- 1Nature Coast Biological Station, Institute of Food and Agricultural Sciences, University of Florida, Cedar Key, FL, United States
- 2Dauphin Island Sea Lab, Dauphin Island, AL, United States
- 3Louisiana Universities Marine Consortium, Chauvin, LA, United States
Introduction
The health of coastal environments is at risk due to exploitation of economically valuable minerals, including oil and natural gas. Impacts to biota occur both directly (e.g., chemical toxicity, foraging/growth changes, behavioral alterations; McDonald et al., 2022) and indirectly (e.g., environmental conditions, cleanup/mitigation activities, food web alterations; McCann et al., 2017; Lewis et al., 2022). Despite acknowledgement of adverse effects, our understanding of oil spill impacts has been driven by unplanned disasters, including Exxon Valdez (Peterson et al., 2003), Ixtoc (Soto et al., 2014), Arthur Kill (Burger 1994), Falmouth Harbor (Reddy et al., 2002), and numerous smaller spills (Rozas et al., 2000; Roth and Baltz 2009). Smaller spills are frequent, with hundreds reported monthly in the United States (USCG National Response Center reports >20,000 calls per year) and many are sufficiently small enough to not initiate management response. In Louisiana (United States), oil spill response efforts are coordinated by the Louisiana Oil Spill Coordinator’s Office (LOSCO) and in 2021, 90 spills triggered on-scene response activities by LOSCO (https://data.losco.org/).
On 20-April-2010, an explosion aboard the Deepwater Horizon (DwH) drilling platform off the Louisiana coast created fires resulting in the uncontrolled release of petroleum into the Gulf of Mexico (GoM). The depth, ∼1500 m, was an obstacle preventing the immediate capping of the damaged wellhead, which leaked for 87 days. A significant portion of the five million+ barrels released (McNutt et al., 2012) inundated wetlands throughout Louisiana, Mississippi, Alabama, and Florida (Michel et al., 2013; Nixon et al., 2016). The volume, temporal and spatial heterogeneity in oil coverage, disproportionate weathering and unknown organism tolerances, plus unintended impacts from mitigation activities, all limited accurate damage assessments. To better understand DwH impacts, the Gulf of Mexico Research Initiative (GoMRI) was established and administered approximately $500 M USD, largely for DwH research, data archival efforts, and outreach over a 10-year period (Shepherd et al., 2016).
Here, we draw on archived GOMRI studies of DwH impacts in nearshore, coastal ecosystems to: 1) highlight the volume and diversity of information obtained via funding, 2) discuss major uncertainties and variability in impact determinations, and 3) provide recommendations to improve future environmental assessments. Given continued and growing coastal development and exploitation of nearshore resources in GoM waters, unexpected disasters will likely reoccur, and discussion of major challenges will guide future experimental and empirical determinations.
What have we learned? Success stories post-Deepwater Horizon
Numerous revelations resulted from GoMRI-funded research. The GoMRI enterprise, as a funding instrument, was successful in creating an unprecedented network of researchers focused on coastal issues ranging from oil/human impacts and petroleum safety to ecological effects. GoMRI funding supported over 1,500 peer-reviewed articles, of which almost 400 focused on inshore/nearshore ecosystems (Figure 1). These studies were used in our assessment below to further identify variability in study designs.
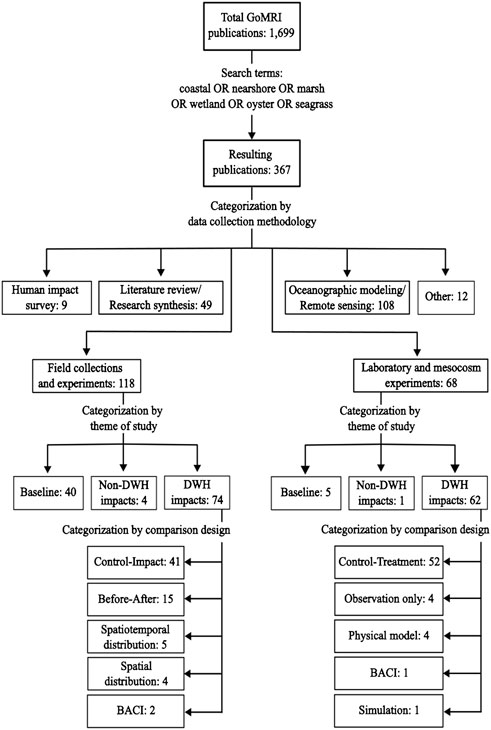
FIGURE 1. Publications deposited in the online GoMRI publication repository database were searched for nearshore studies using the Boolean search terms coastal OR nearshore OR marsh OR wetland OR oyster OR seagrass. The resulting 367 publications were then categorized by data collection methodology, with publications that could be described as “Field collections and experiments” and “Laboratory and mesocosm experiments” subsequently categorized by general study theme. Field collection/experiment and lab/mesocosm studies that investigated DWH impacts were then further categorized by comparison design, resulting in five general comparison categories for each of these two collection methods. Note: Although not listed out in this figure, the ‘Other’ collection method category consisted of the following method descriptions: public outreach (4 publications), perspective (2 publications), dataset (1 publication), and technical advancement (1 publication). Additionally, DWH was a study theme in all other collection method categories, with 9 DWH themed publications in “Human impact survey,” 36 publications in “Literature review/Research synthesis,” 25 publications in “Oceanographic modeling/Remote sensing,” and 7 publications in “Other.”
GoMRI funding improved our understanding of the region’s physical, biological, and geochemical importance-a demonstrable success story. This included collections of the first baseline inventories in decades, critical documentation that can serve as baseline reference sites for future stressors. Other noteworthy contributions highlighted the enhanced understanding of deep sea population dynamics and community resilience following this disturbance, quantification of nearshore ecosystem properties, and improved understanding of oil chemistry, especially as it relates to physical/meteorological forcings, weathering/degradation, and effects in coastal ecosystems (Hsing et al., 2013; Felder et al., 2014; Prouty et al., 2016; Rabalais and Turner 2016; Murawski et al., 2021; Overton et al., 2022).
The unprecedented findings were driven by large, collaborative research teams (“consortia”). Funding for consortia supported over 4,000 scientists and support personnel, including faculty (1,106), postdoctoral researchers (367), graduate (1,205) and undergraduate (1,050) students, and research staff (718). The consortia concept was novel and intended to create multidisciplinary teams, and linked researchers from 43 states and 18 different countries. These consortia also facilitated rapid responses to other disasters including the Hercules gas well blowout (Joye et al., 2014) and Hurricanes Isaac and Harvey (Curcic et al., 2016; Steichen et al., 2020), as well as ongoing research collaborations not related to DwH. As a result of GOMRI funding, we now have a greater understanding of the benefits of large and diverse research networks, and the roadblocks historically limiting product generation.
Lessons learned: Improving oil impact assessments
Incorporating confounding variables
Due to the mensurative, observational nature of field measurements, the possibility of unconsidered/unmeasured drivers exists and potentially obfuscated DwH impact assessments (sensu Osenberg and Schmitt 1996; Stewart-Oaten et al., 1992). In other words, the lack of controlled comparisons reduces the ability to assign causality. Potentially confounding variables include the inconsistencies in oiling intensity, weathering, and inconsistent analytical methods, including often-overlooked indirect connections (e.g., food web alterations, changes in connectivity, habitat loss, and other environmental responses). Here, we provide a few examples of possible confounding variables that should be considered in future assessments.
DwH oil (e.g., Sweet Louisiana Crude, MC-252, etc.) was a unique blend of >10,000 chemical compounds, ranging from more toxic, lighter aromatics to less toxic, heavier, and stable compounds (Overton et al., 2022). Lighter compounds (e.g., naphthalenes) precipitate rapidly during the weathering process (via wave action, ultraviolet light degradation, and other chemical processes), while more stable compounds (e.g., asphaltenes) resist weathering. Remedial management actions following the release of oil offshore were largely focused on increasing oil weathering (e.g., Corexit dispersant application to increase oil surface area and expedite microbial degradation, burning of surface oil, and application of booms for oil collection). As oil moved inshore, its chemical composition changed, with some exceptions. Oil protected inside of tar balls and later resuspended from buried sediments were less weathered, as documented in remobilized oil after Hurricane Isaac in 2012 (Zengel et al., 2015; Bam et al., 2018). Overall, however, oil that arrived inshore became highly weathered and compositionally different than when initially released at the wellhead. This variable oil weathering is an important aspect that merits consideration in future impact assessments and accounting for during lab-based trials, as important differences in responses can result (Martin 2017). Moreover, oil characterization techniques (total petroleum hydrocarbons, fingerprinting, etc.) varies considerably among studies, making cross-study comparisons difficult. Compounds measured in total petroleum estimates can also vary among labs and employed instruments have variable detection limits and accuracy.
Several non-oil factors can also introduce confounding variables. Prevailing meteorological and hydrological conditions controlled DwH oil delivery to coastal wetlands, and deposition may thus occur in areas already prone to erosion from wind/wave energy or in areas where recruitment of specific benthic organisms may be prevalent. To keep oil out of coastal wetlands, the State of Louisiana diverted Mississippi River water into estuaries, an action of great importance because salinity is a key driver of biological composition, and this also caused physiological stress to estuarine organisms (e.g., oysters, Powers et al., 2017). Crews conducting remedial (Martinez et al., 2012) or cleaning activities of oiled marshes often trampled vegetation causing additional harm unnecessary in lightly oiled areas (Zengel et al., 2015). At higher trophic levels, cessation of commercial/recreational fishing likely had important cascading effects throughout GoM food webs (Fodrie et al., 2014; Schaefer et al., 2016; Martin et al., 2020). Thus, not all effects were due to oil toxicity.
Of the total 177 DwH-related GoMRI publications that focused on nearshore habitats (excluding literature reviews), field-based and laboratory/mesocosm comparisons respectively comprised just 42% and 38% of published research (Figure 1), making the need for consideration of previously mentioned study concerns even more important. Revealing field patterns is often difficult due to multicollinearity (Graham 2003), but reduced realism in laboratory environments offers a philosophical tradeoff for determining true effects (Diamond, 1986). We suggest that pluralistic approaches can take advantage of positive aspects of each technique (e.g., pairing field and lab to account for variability while retaining realism) and provide more definitive results.
Appropriate study designs
The unpredictable spatial/temporal heterogeneity of unplanned disturbances make robust assessments difficult (Stewart-Oaten et al., 1992; Schmitt and Osenberg 1996). DwH studies often lacked unimpacted baseline information (only ∼23% of field studies incorporated baseline data, Figure 1) thus relying on control-impact comparisons, many of which lacked appropriate controls and/or sufficient experimental designs to conduct impact assessments. Few field assessments employed before-after-control impact (BACI) studies, or their suggested alternative approaches, often preferred for determining impacts (∼3%, Figure 1). Given the lack of baseline data, characterizing temporal variability in oiling is critical as many comparisons were made years after DwH. Many field studies (∼52%) inferred oiling status from anecdotal or broad oil categorization assessments (e.g., Shoreline Cleanup Assessment Team, SCAT; Michel et al., 2013) to determine treatment identities. In other cases, researchers leveraged prior available information to serve as surrogate baseline (pre-impact) data. These before-after studies represented only ∼20% of publications in our survey of nearshore GoMRI publications (Figure 1). Given the numerous anthropogenic stressors affecting GoM estuaries, coupled with oil response/mitigation impacts (described above), before-after comparisons should be considered as the successional trajectory of many ecosystems change naturally (Schmitt and Osenberg 1996). The presence of multiple, interacting, and often non-additive stressors also challenge the power of studies sacrificing time or space components. Thus, other stressors (e.g., dredging, climate factors, etc.) may be incorrectly attributed to oil impacts. This supports the use of paired laboratory experiments to investigate potential confounding factors.
Similarly, the design of laboratory assessments requires careful consideration. Oil has a variety of effects, making it difficult to design rigorous laboratory studies. For example, degrading oil can produce air-borne chemicals that influence non-oil treatments leading to questionable replicate independence (Hurlbert, 1984). Moreover, oil can interact with plastics used in tanks to produce novel toxic chemicals that may impact the survival of experimental organisms (de Soysa et al., 2012). Oil treatments should be reflective of natural conditions, with relevant concentrations, compositions, and weathering to mimic impacted areas (Overton et al., 2022). Trial duration should also be considered, as oil effects in contained systems may overestimate exposure by reducing oil dilution or preventing emigration that occurs in nature. Representative species that minimize handling stress, an important experimental artifact, while maximizing ecological relevance (e.g., species that are appropriate as indicators, or have some other intrinsic value due to abundance or importance) should be considered. Done appropriately, lab studies provide great insight and supplement field observations, thereby lending increased confidence in mensurative field patterns (Diamond, 1986; Schmitt and Osenberg 1996).
Discussion: A way forward and future considerations
In the years following DwH, researchers have made significant contributions to our understanding of nearshore GoM ecosystems, and in the process made advances in diverse areas of study. Petroleum exploitation in the GoM will only increase due to international tension, and thus it is in the best interest of researchers, managers, conservationists, and the general public alike to reflect on the DwH experience, both positive and negative, to better improve the response to future spills. Given this, we conclude with these recommendations based on our assessment of work to date on nearshore GoM ecosystems.
The novel use of large consortia stimulated multi-institution collaboration, and future efforts should determine when and why these efforts were successful (or not), including best practices, obstacles, and optimization of group dynamics and participation.
While a greater understanding of oil chemistry has been achieved, significant variability in measurement techniques, groupings (e.g., total petroleum hydrocarbons), and analytical detection limits can be improved by adopting standardized approaches. This will facilitate future cross-study comparisons and meta-analyses.
Attribution of oil concentrations in field conditions can be problematic without appropriate caution. For example, large scale oil patterns (e.g., SCAT) may be inappropriate when used to determine small plot impacts or inappropriate oil treatments due to temporal factors when oil concentrations are known to change.
Field assessments are hampered by multiple unmeasured/unconsidered variables and these additional stressors require consideration and acknowledgement.
A system of environmental monitoring, including experiments, to determine baseline conditions across a broad geographic area should be implemented to avoid lack of pre-disturbance information which led to suboptimal designs during DwH. This will facilitate preferred designs (e.g., BACI) and is especially true given the continued shifting baselines and frequency of disturbances.
Laboratory studies should seek appropriate species, methods, and oil concentrations/weatherings to maintain realism.
The pairing of observational field data with appropriate lab studies can generate robust assessments.
Author contributions
CM framed the study with input from JV and BR. CM wrote the initial draft of the text. AM created and edited the figure. All authors contributed portions of the text, reviewed, edited, and revised content.
Funding
This research was made possible by a grant from The Gulf of Mexico Research Initiative. Studies used in the analysis are publicly available through the Gulf of Mexico Research Initiative Information and Data Cooperative (GRIIDC) at https://data.gulfresearchinitiative.org. Authors served as members of two consortia: the Alabama Center for Ecological Resilience (CM and JV) and the Coastal Waters Consortium (BR, CM, and AM).
Acknowledgments
We thank the leadership of GoMRI for discussions leading to this text, attendees of GoMRI and GOMOSES conferences, and all our colleagues that worked on GoMRI-funded research for productive discussions that advanced our understanding of Gulf ecosystems.
Conflict of interest
The authors declare that the research was conducted in the absence of any commercial or financial relationships that could be construed as a potential conflict of interest.
Publisher’s note
All claims expressed in this article are solely those of the authors and do not necessarily represent those of their affiliated organizations, or those of the publisher, the editors and the reviewers. Any product that may be evaluated in this article, or claim that may be made by its manufacturer, is not guaranteed or endorsed by the publisher.
Supplementary material
The Supplementary Material for this article can be found online at: https://www.frontiersin.org/articles/10.3389/fenvs.2022.1092097/full#supplementary-material
References
Bam, W., Hooper-Bui, L. M., Strecker, R. M., Adhikari, P. L., and Overton, E. B. (2018). Coupled effects of oil spill and hurricane on saltmarsh terrestrial arthropods. Plos ONE 13 (4), e0194941. doi:10.1371/journal.pone.0194941
J. Burger (Editor) (1994). Before and after an oil spill: the Arthur Kill (New Jersey: Rutgers University Press).
Curcic, M., Chen, S. S., and Özgökmen, T. M. (2016). Hurricane-induced ocean waves and stokes drift and their impacts on surface transport and dispersion in the Gulf of Mexico. Geophys. Res. Lett. 43 (6), 2773–2781. doi:10.1002/2015gl067619
De Soysa, T. Y., Ulrich, A., Friedrich, T., Pite, D., Compton, S. L., Ok, D., et al. (2012). Macondo crude oil from the Deepwater Horizon oil spill disrupts specific developmental processes during zebrafish embryogenesis. BMC Biol. 10 (1), 40–25. doi:10.1186/1741-7007-10-40
Diamond, J. (1986). Laboratory experiments, field experiments, and natural experiments. Community Ecol. 3–22.
Felder, D. L., Thoma, B. P., Schmidt, W. E., Sauvage, T., Self-Krayesky, S. L., Chistoserdov, A., et al. (2014). Seaweeds and decapod crustaceans on Gulf deep banks after the Macondo oil spill. Bioscience 64 (9), 808–819. doi:10.1093/biosci/biu119
Fodrie, F. J., Able, K. W., Galvez, F., Heck, K. L., Jensen, O. P., López-Duarte, P. C., et al. (2014). Integrating organismal and population responses of estuarine fishes in Macondo spill research. BioScience 64 (9), 778–788. doi:10.1093/biosci/biu123
Graham, M. H. (2003). Confronting multicollinearity in ecological multiple regression. Ecology 84 (11), 2809–2815. doi:10.1890/02-3114
Hsing, P. Y., Fu, B., Larcom, E. A., Berlet, S. P., Shank, T. M., Govindarajan, A. F., et al. (2013). Evidence of lasting impact of the Deepwater Horizon oil spill on a deep Gulf of Mexico coral community. Elem. Sci. Anthropocene 1. doi:10.12952/journal.elementa.000012
Hurlbert, S. H. (1984). Pseudoreplication and the design of ecological field experiments. Ecol. Monogr. 54 (2), 187–211.
Joye, S. B., Montoya, J. P., Murawski, S. A., Özgökmen, T. M., Wade, T. L., Montuoro, R., et al. (2014). A rapid response study of the Hercules gas well blowout. Eos, Trans. Am. Geophys. Union 95 (38), 341–342. doi:10.1002/2014eo380002
Lewis, K. A., Christian, R. R., Martin, C. W., Allen, K. L., McDonald, A. M., Roberts, V. M., et al. (2022). Complexities of disturbance response in a marine food web. Limnol. Oceanogr. 67, S352–S364. doi:10.1002/lno.11790
Martin, C. W., Lewis, K. A., McDonald, A. M., Spearman, T. P., Alford, S. B., Christian, R. C., et al. (2020). Disturbance-driven changes to northern Gulf of Mexico nekton communities following the Deepwater Horizon oil spill. Mar. Pollut. Bull. 155, 111098. doi:10.1016/j.marpolbul.2020.111098
Martin, C. W. (2017). Avoidance of oil contaminated sediments by estuarine fishes. Mar. Ecol. Prog. Ser. 576, 125–134. doi:10.3354/meps12084
Martínez, M. L., Feagin, R. A., Yeager, K. M., Day, J., Costanza, R., Harris, J. A., et al. (2012). Artificial modifications of the coast in response to the deepwater horizon oil spill: quick solutions or long-term liabilities? Front. Ecol. Environ. 10 (1), 44–49. doi:10.1890/100151
McCann, M. J., Able, K. W., Christian, R. R., Fodrie, F. J., Jensen, O. P., Johnson, J. J., et al. (2017). Key taxa in food web responses to stressors: the deepwater horizon oil spill. Front. Ecol. Environ. 15 (3), 142–149. doi:10.1002/fee.1474
McDonald, A. M., Martin, C. W., Rieucau, G., and Roberts, B. J. (2022). Prior exposure to weathered oil influences foraging of an ecologically important saltmarsh resident fish. PeerJ 9, e12593. doi:10.7717/peerj.12593
McNutt, M. K., Camilli, R., Crone, T. J., Guthrie, G. D., Hsieh, P. A., Ryerson, T. B., et al. (2012). Review of flow rate estimates of the Deepwater Horizon oil spill. Proc. Natl. Acad. Sci. 109 (50), 20260–20267. doi:10.1073/pnas.1112139108
Michel, J., Owens, E. H., Zengel, S., Graham, A., Nixon, Z., Allard, T., et al. (2013). Extent and degree of shoreline oiling: Deepwater Horizon oil spill, Gulf of Mexico, USA. PloS ONE 8 (6), e65087. doi:10.1371/journal.pone.0065087
Murawski, S. A., Kilborn, J. P., Bejarano, A. C., Chagaris, D., Donaldson, D., Hernandez, F. J., et al. (2021). Acidification in the U.S. Southeast: Causes, potential consequences and the role of the southeast ocean and coastal acidification network. Front. Mar. Sci. 7, 1–548. doi:10.3389/fmars.2020.00548
Nixon, Z., Zengel, S., Baker, M., Steinhoff, M., Fricano, G., Rouhani, S., et al. (2016). Shoreline oiling from the Deepwater Horizon oil spill. Mar. Pollut. Bull. 107 (1), 170–178. doi:10.1016/j.marpolbul.2016.04.003
Osenberg, C. W., and Schmitt, R. J. (1996). “Detecting ecological impacts caused by human activities,” in Detecting ecological impacts (Cambridge: Academic Press), 3–16.
Overton, E. B., Adhikari, P. L., Radović, J. R., and Passow, U. (2022). Fates of petroleum during the deepwater horizon oil spill: A chemistry perspective. Front. Mar. Sci. 1403. doi:10.3389/fmars.2022.928576
Peterson, C. H., Rice, S. D., Short, J. W., Esler, D., Bodkin, J. L., Ballachey, B. E., et al. (2003). Long-term ecosystem response to the Exxon Valdez oil spill. Science 302 (5653), 2082–2086. doi:10.1126/science.1084282
Powers, S. P., Grabowski, J. H., Roman, H., Geggel, A., Rouhani, S., Oehrig, J., et al. (2017). Consequences of large-scale salinity alteration during the Deepwater Horizon oil spill on subtidal oyster populations. Mar. Ecol. Prog. Ser. 576, 175–187. doi:10.3354/meps12147
Prouty, N. G., Fisher, C. R., Demopoulos, A. W., and Druffel, E. R. (2016). Growth rates and ages of deep-sea corals impacted by the Deepwater Horizon oil spill. Deep Sea Res. Part II Top. Stud. Oceanogr. 129, 196–212. doi:10.1016/j.dsr2.2014.10.021
Rabalais, N. N., and Turner, R. E. (2016). Effects of the Deepwater Horizon oil spill on coastal marshes and associated organisms. Oceanography 29 (3), 150–159. doi:10.5670/oceanog.2016.79
Reddy, C. M., Eglinton, T. I., Hounshell, A., White, H. K., Xu, L., Gaines, R. B., et al. (2002). The West Falmouth oil spill after thirty years: the persistence of petroleum hydrocarbons in marsh sediments. Environ. Sci. Technol. 36 (22), 4754–4760. doi:10.1021/es020656n
Roth, A. M. F., and Baltz, D. M. (2009). Short-term effects of an oil spill on marsh-edge fishes and decapod crustaceans. Estuaries Coasts 32 (3), 565–572. doi:10.1007/s12237-009-9135-2
Rozas, L. P., Minello, T. J., and Henry, C. B. (2000). An assessment of potential oil spill damage to salt marsh habitats and fishery resources in Galveston Bay, Texas. Mar. Pollut. Bull. 40 (12), 1148–1160. doi:10.1016/s0025-326x(00)00075-8
Schaefer, J., Frazier, N., and Barr, J. (2016). Dynamics of near-coastal fish assemblages following the Deepwater Horizon oil spill in the northern Gulf of Mexico. Trans. Am. Fish. Soc. 145 (1), 108–119.
Schmitt, R. J., and Osenberg, C. W. (Editors) (1996). Detecting ecological impacts: Concepts and applications in coastal habitats (Cambridge: Academic Press).
Shepherd, J., Benoit, D. S., Halanych, K. M., Carron, M., Shaw, R., and Wilson, C. (2016). Introduction to the special issue: An overview of the Gulf of Mexico research initiative. Oceanography 29 (3), 26–32. doi:10.5670/oceanog.2016.58
Soto, L. A., Botello, A. V., Licea-Durán, S., Lizárraga-Partida, M. L., and Yáñez-Arancibia, A. (2014). The environmental legacy of the Ixtoc-I oil spill in Campeche Sound, southwestern Gulf of Mexico. Front. Mar. Sci. 1, 57. doi:10.3389/fmars.2014.00057
Steichen, J. L., Labonté, J. M., Windham, R., Hala, D., Kaiser, K., Setta, S., et al. (2020). Microbial, physical, and chemical changes in Galveston Bay following an extreme flooding event, Hurricane Harvey. Front. Mar. Sci. 186. doi:10.3389/fmars.2020.00186
Stewart-Oaten, A., Bence, J. R., and Osenberg, C. W. (1992). Assessing effects of unreplicated perturbations: no simple solutions. Ecology 73 (4), 1396–1404. doi:10.2307/1940685
Keywords: Macondo, MC-252, Gulf of Mexico, disturbance, stressor, impact assessment
Citation: Martin CW, McDonald AM, Valentine JF and Roberts BJ (2023) Towards relevant ecological experiments and assessments of coastal oil spill effects: Insights from the 2010 Deepwater Horizon oil spill. Front. Environ. Sci. 10:1092097. doi: 10.3389/fenvs.2022.1092097
Received: 07 November 2022; Accepted: 19 December 2022;
Published: 06 January 2023.
Edited by:
Jaan H. Pu, University of Bradford, United KingdomReviewed by:
David Hala, Texas A&M University at Galveston, United StatesCopyright © 2023 Martin, McDonald, Valentine and Roberts. This is an open-access article distributed under the terms of the Creative Commons Attribution License (CC BY). The use, distribution or reproduction in other forums is permitted, provided the original author(s) and the copyright owner(s) are credited and that the original publication in this journal is cited, in accordance with accepted academic practice. No use, distribution or reproduction is permitted which does not comply with these terms.
*Correspondence: Charles W. Martin, bWFydGluLmNoYXJsZXMud0BnbWFpbC5jb20=
†Present address: Charles W. Martin, University of South Alabama, Mobile, AL, United States Dauphin Island Sea Lab, Dauphin Island, AL, United States