Corrigendum: The roles for branch shelters and sheep manure to accelerate the restoration of degraded grasslands in northern China
- 1School of Agriculture, Ningxia University, Yinchuan, Ningxia, China
- 2Ningxia Grassland and Animal Husbandry Engineering Technology Research Centre, Yinchuan, Ningxia, China
- 3Department of Nature Resources, College of Agriculture and Life Sciences, Cornell University, Ithaca, NY, United States
- 4Baijitan Nature Reserve Administration, Lingwu, Ningxia, China
- 5College of Geographical Sciences and Planning, Ningxia University, Yinchuan, Ningxia, China
New strategies are desperately needed for restoring the millions of hectares of degraded grasslands in arid and semiarid areas of northern China. This study evaluated using different combinations of manure amendments and shrub branch shelters for their impacts on soil moisture, nutrient availability, and plant growth over two growing seasons in a degraded grassland in Ningxia, China. A two-factor experiment was conducted, with three concentrations of 1.2 g m−2, 442 g m−2, and 884 g m−2 native Tan sheep manure as the main plots. Cut caragana (Caragana intermedia) branches were used to create branch shelters covering 0%, 20%, 40%, and 60% of ground area, and these acted as sub-main plots. Soil water storage, soil temperature, manure decomposition, branch decomposition, soil nutrients, and plant growth were monitored for 2 years. Results indicated that soil water storage was significantly increased, and soil temperature decreased, under the 40% and 60% branch shelters. Decomposition rate of manure and shrub branches also increased with increasing soil water availability associated with the higher branch sheltering effects, although soil carbon and nitrogen concentrations were primarily driven by the decomposing manure. The combination of high levels of shrub branch shelter and manure application significantly enhanced plant production, although the bulk of the biomass was concentrated in one species, Artemisia scoparia. In conclusion, our study successfully demonstrated feasible and inexpensive solution for the restoration of degraded grasslands, which takes advantage of resources associated with overgrazing Tan sheep and Caragana shrub encroachment in arid and semiarid areas.
1 Introduction
Grassland ecosystems account for approximately 40% of the Earth’s surface (Luan et al., 2014), and fulfil important functions and services in agricultural systems (Liu et al., 2011). Grasslands provide the basis for agriculture with 3.38 billion ha (26%) used as livestock pasturing and 1.53 billion ha (12%) for cropland (Foley et al., 2011). However, due to climate change and human disturbance, grassland degradation has become a serious global ecological problem (Akiyama and Kawamura, 2007; Dong et al., 2019). Clearing of natural vegetation followed by chronic tillage for crops degrades soil structure, oxidizes soil organic matter, and kills the soil microbial communities that help bind the soil, resulting in increased erosion (Reichert et al., 2022). Overgrazing also kills the plants whose roots bind the soil and contribute to soil organic matter in grasslands (Klumpp et al., 2009). In summary, both systems lead to grassland degradation which has been described as an inverse succession that combines the deterioration of vegetation and soil (Gang et al., 2014). Therefore, many practices such as reseeding, fertilization, irrigation, organic amendments, and enclosure have been adopted to restore degraded grasslands (Zhang Q et al., 2021).
Presently, overgrazing is widely regarded as a major factor contributing to grassland degradation. However, with appropriate grazing practices, manure deposition and decomposition by livestock can play a positive role in grassland soil nutrient cycling and grassland restoration (Schuman et al., 2002), since about 50% (range 40%–60%) of total animal N excretion is voided in grasslands (Oenema and Tamminga, 2005). Numerous studies have demonstrated that returned or applied livestock manure to grasslands can effectively improve grassland soil fertility and productivity (Min et al., 2014; Duffková et al., 2015; Zhang et al., 2015; Bai et al., 2017). Moreover, livestock manure has high concentrations of easily-decomposable C and N compounds, providing readily-accessible nutrients to the soil micro- and macrobiota decomposers (Knops et al., 2002; Bakker et al., 2004). Conversely, chronic export of animal manure from the grassland ecosystem can result in deterioration of soil fertility and decline of grassland primary productivity (Xu et al., 2012). However, in degraded grassland systems, manure application alone is generally insufficient to restore the soil health and other types of amendments are needed. In particular, strategies are needed to improve soil water availability in these dry environments, since the activities of microbial manure decomposers are usually positively correlated with increasing soil moisture in arid areas (Zhu et al., 2020). Hence, the measures for improving soil water condition may have benefit for livestock manure decomposition and soil nutrient improvement in grazed grasslands.
In natural, undisturbed grasslands, the thick layer of leaf litter that forms across the surface has been shown to increase rain capture and shade and cool the soil (Deutsch et al., 2010). Similarly, the use of mulch and other surface organic amendments is also gaining recognition for their value in shading and cooling soils, and reducing evaporative losses. For instance, addition of a thick layer of wood chips as mulch over the soil surface, can cool the soil, reduce evaporative loss, and enhance plant growth in arid areas (Li et al., 2018, 2019). Mulch is also used as a common landscaping practice, to prevent weed germination and growth, which it does by inhibiting light from reaching the surface (Cochran et al., 2009). Alternative strategies are needed that help shade and cool, but also allow some light penetration to help seed germination.
Previous research has shown that piling branches over the soil can shade and cool the soil yet allow seed germination and plant growth (Li et al., 2018, 2019; Leger et al., 2022). And unlike wood chip mulch, the sturdier longer branches facilitate the formation of a loose three-dimensional structure of material above the soil surface. One potential source of woody material, namely invading shrubs, may be a useful source of material for these amendments, while countering shrub encroachment, which has become another factor which is contributing to grassland degradation worldwide (Wilson et al., 2022). The proliferation of shrubs in grasslands can substantially change the pattern of soil water resources, which in turn leads to changes in the structure and function of the ecosystem (Jia et al., 2018; D’Odorico et al., 2012). Presently, about 10%–20% of arid and semi-arid grasslands are undergoing shrub encroachment (Watson et al., 2018). This phenomenon has been documented in southwestern North America (Caracciolo et al., 2016), the Mediterranean Basin (Maestre et al., 2009), Australia (Eldridge and Koen, 2003), and South Africa (Kerley and Whitford, 2009). Some grasslands in Inner Mongolia, Gansu, Ningxia, and other provinces of northern China are also exhibiting shrub encroachment (Wei et al., 2019). In eastern Ningxia, China, caragana (Caragana intermedia) were extensively planted in grasslands (accounting for about 33% of grassland area) for wind prevention and sand stabilization (Zheng et al., 2019). In the period immediately following planting, the caragana was deemed successful in stabilizing the sandy soils, however it was subsequently found to consume large amounts of water, decreasing overall soil water availability (Dan et al., 2020). As a result, with increasing time since planting of the caragana, a suite of problems occurs, including reduction in perennial herbs, water deficit, and soil degradation gradually developed in local grasslands (Zhao et al., 2020). Therefore, fire management and other measurements have being extensively adopted to reduce shrub encroachment for grassland restoration (Killgorea et al., 2009; Wang et al., 2018; Wilcox et al., 2018). However, fire management may also cause environment pollution and waste of potentially valuable resources.
Interestingly, previous research has demonstrated that tree branch shelters were successful in achieving benefits to cool temperatures and increase soil moisture contents in forests that had been thinned (Morreale and Sullivan, 2010). Even when laid directly atop forest soils coarse woody debris also has benefits in improving soil moisture, increasing soil organic matter, and reducing bulk density (Bulmer et al., 2007). Our earlier studies in semi-arid environments have also demonstrated that tree branch shelters effectively and immediately decrease evaporation, retain more precipitation, and enhance plant growth in desertified grassland soils (Li et al., 2018, 2019). Moreover, over longer term periods of three or more years, tree branch shelters were found to improve soil physical properties and increase soil nutrients of desertified grassland (Li et al., 2019). In addition, moderate shading level, for example <60%, had minimal negative effects on overall annual grassland net herbage accumulation (Dodd et al., 2005). Furthermore, unlike light organic materials such as straw and other herbaceous materials that may easily decompose in as little as 2 years even in more arid regions, woody materials (for instance shrub and tree branches) can last for years to decades (Weedon et al., 2009) when used for soil restoration. Therefore, woody materials have an additional advantage of reducing the cost of soil restoration over the long term, since constant re-application is not needed.
Given this framework, it is likely that caragana branches can also be viable for use as in-situ shelters to improve grassland soil moisture and jump-start restoration of degraded grasslands in Ningxia, where the climate is semi-arid and this shrub is abundant. We hypothesize that (a) caragana branch shelters can facilitate nutrient cycling of livestock manure in grassland by improving soil moisture availability, and (b) then enhancing plant growth via improving soil moisture and nutrient availability. The overall goal of this study was to evaluate the potential of utilizing various combinations of two readily available resources, caragana branch shelters and sheep manure, to improve the soil environment and support increased native plant productivity in highly degraded arid landscapes of northern China. The specific objectives of this study were to: (a) analyze the effect of using caragana branch shelters versus manure on the soil physical environment, including soil temperatures and soil water storage in the study site; (b) evaluate the interactive effects of different sheltering levels and amounts of manure amendment on decomposition rates of the two materials, as well as soil nutrient levels, and (c) evaluate interactive effects of manure and branch shelter treatments on plant production, as indicated by biomass, average plant height, and cover.
2 Materials and methods
2.1 Site description
The study site is located in Yanchi County, Ningxia (106° 30′—107° 47′ E, 37° 04′—38° 10′ N), Northern China. The regional climate is a typical temperate continental and monsoonal climate. The average annual temperature is 8.1°C. The annual precipitation is 295 mm, and the average annual potential evapotranspiration is 2,132 mm, and the frost-free period is about 162 days (Zhang Y et al., 2021). The soil texture is primary sandy loam, and the soil characteristics of the 0–20 cm layer as follows: pH was 8.69, 0.18 g kg−1 total N, 0.53 g kg−1 total P, 2.29 g kg−1 organic matter content. The natural vegetation of the site is desert steppe, and the plant species mainly consist of Artemisia scoparia, Corispermum mongolicum, Heteropappus altaicus, Agropyron cristatum, Salsola ruthenica, Agropyron mongolicum, Bassia dasyphylla, and planted Caragana intermedia. However, caragana shrubs were extensively planted in grasslands (account for about 33% of 3,327 km2 grassland area) for wind prevention and sand stabilization in this area, and the total above-ground caragana biomass (dry weight) in grassland can be as high as 8.4 × 104 kg km−2 (Zheng et al., 2019).
2.2 Field experiment design
The field experiment was set up in October in 2016 in a degraded 60 hm2 grassland, and the measurements were conducted during the two subsequent growing seasons of 2017 and 2018. Prior to the experiment, this grassland was intensively grazed by Tan sheep, a native species to this region. The grazing density was 3–4 Tan sheep·hm−2, and the whole grassland was enclosed by fences with a gate. The vegetation of the site consisted of mixed grass species and shrub caragana. The cumulative amount of oven-dried (85°C) sheep manure was 872 g m−2 at the gate and 1.2 g m−2 at 800 m from the gate, based on an initial sampling of the surface soil (0–5 cm depth) throughout the field.
In order to ensure the homogeneity both for soil properties and vegetation, all experimental plots were set up 200 m far away from the gate, i.e., at sites where the concentration of sheep manure was approximately 442 g m−2. Then, a split-plot experiment was designed (Figure 1), and the main plots were comprised of three treatments where the cumulative manure concentrations were 1.2 g m−2 (thus near to zero), 442 g m−2, and 884 g m−2 for low (L), medium (M) and high (H) concentrations, respectively. Thus, for L, almost all manure had been removed from the plots, by shoveling. Whereas, for H, the manure removed from L plots were added into H plots. The M plots had no manure removed or added. The sub-main plots were comprised of four different branch shelters based on our previous studies (Li et al., 2018, 2019). Thus 0%, 20%, 40%, and 60% of grassland surface area were overlaid by the cut caragana branch shelters. Caragana branches of ∼0.7 cm diameter were obtained from throughout the study site. Then branches, with length of ∼1 m, were selected and layered over the soil surface, criss-crossing to form a lattice type structure, after the manure plots were set up. The sheltering levels were adjusted and measured by LAI-2200C plant canopy analyzer (Li-COR Inc., United States) in October, when there was no aboveground plant foliage to interfere with the measurements. The branch shelter treatments were randomly distributed in each main block. The shelter treatments of 20%, 40%, and 60% surface coverage corresponded approximately to 660 g m−2, 1,320 g m−2, and 1980 g m−2 (dry weight) caragana branches, respectively. Increasing extent of the branch shelters provided increasing amounts of shade, a thicker boundary layer above the soil surface which would act to reduce evaporative losses, as well as a greater amount of wood for potential decomposition into the plot.
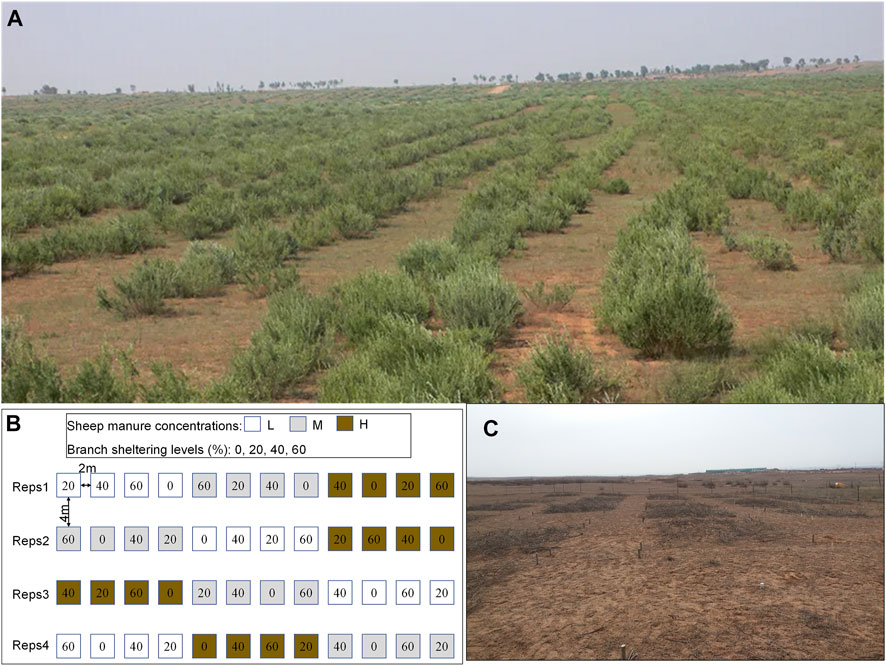
FIGURE 1. Shrub encroached grassland (A) in study site and schematic (B) and photo (C) showing layout of the experimental study site and plots. L, M and H in figure b are low, medium, and high amount of manure accumulated in grassland surface.
In total, 12 treatments with four replicate plots per treatment were set up for the experiment. Each plot size was 16 m2 (4 × 4 m), and each pair of plots were separated from each other by 2 m spacing, and 4 m alleys between the two main blocks. There was no grazing allowed in the field plots during the experimental period, and the whole experiment site was enclosed with fences to prevent intrusion by Tan sheep and other large herbivores. The organic carbon content of manure and branch are 198.55 g kg−1and 453.94 g kg−1, respectively; the total N of manure and branch are 12.27 g kg−1 and 14.82 g kg−1, respectively.
2.3 Monitoring of soil water content and associated soil temperature
The soil volumetric water content within the 0–20 cm soil layer was monitored using AZS - 100 Handheld Meter (Aozuo Ecology Instrumentation Ltd., Beijing China), but calibrated by the gravimetric method. Measurements were made every 10 days from April to October both in 2017 and 2018. Finally, the soil water storage was calculated with the following equation:
where SWS (mm) is soil water storage; VW is volumetric water; H (mm) is soil depth = 200 mm.
Additionally, soil temperature at the depth of 20 cm was monitored at 14:00 h on the same day as the soil moisture measurements were made, using a soil thermometer (Changzhou Rui Ming Instrument and meter plant thermometer, Changzhou China).
2.4 Measurement of decompositions and nutrient inputs both for manure and caragana branches
Decomposition rates of manure and caragana branches were measured using nylon bags (with 2 mm mesh). For manure bags, 100 g oven-dried (85°C) and intact sheep manure were placed into a bag, then two bags were laid on the surface of the soil within each plot (totally, four sheltering levels × 3 manure concentrations × 2 sampling dates × 4 replicates = 96 bags). For branch bags, 100 g oven-dried (85°C) air-dried caragana branches (∼0.7 cm in diameter and 10 cm in length) were placed into each bag, then two bags were placed into each plot for which there was branch sheltering (thus totally, three sheltering levels × 3 manure concentrations × 2 sampling dates × 4 replicates = 72 bags).
Both for manure and branches, one bag in each plot was collected at the end of September in 2017, and another was collected at the end of September in 2018. Then the decomposition rate was determined as the change in oven-dried weight loss determined after oven drying at 85°C. Organic carbon was assayed by the acidified potassium dichromate (K2Cr2O7-H2SO4) heating method (Bao, 2013). Total nitrogen (N) was determined using the Kjeldahl procedure (Bao, 2013). Considering the influence of soil to interfere accurate evaluation for manure decomposition, ash correction was applied according to Rashid et al. (2017). Thus, a muffle furnace was used to determine ash content in bags by loss-on ignition at 550°C for 4 h, and the soil dry weight that contaminated the manure calculated as following equations:
where CDW is soil dry weight (g) than contaminated the manure bag, ASR is ash content (mg) that remained in manure bag, AIC is the initial ash content (mg) in manure bag and ASL is the soil ash content (mg g–1). After ash correction, cumulative decomposition rate of manure and branches, as well as the potential maximum C and N which manure and branches input into soil were evaluated with the following equations:
where, DR (%) is cumulative decomposition rate of manure or branches after one or 2 years; DMinitial (g m−2) is the initial dry matter weight of manure or sheltered branches in each plot; DMend is the dry matter weight of manure or sheltered branches were decomposed after one or 2 years in each plot; Creleased or Nreleased (g m−2) is cumulative released C or N from decomposed manure or branches after one or 2 years in each plot; Ccontent (g kg−1) or Ncontent (g kg−1) is the concentration of C or N in manure or branches; Cinput (g kg−1) or Ninput (g kg−1) is the potential maximum C or N which manure or branches cumulatively input into soil; BD (g cm−3) is soil bulk density, and in this study BD = 1.4 g cm−3; S (m2) is soil area in calculation, and 1 m2 soil area was applied in calculation in this study; H is soil depth, H = 20 cm in this study.
2.5 Soil sampling and soil chemical property measurement
Samples were also collected at the end of September in both 2017 to 2018. Three soil samples between 0 and 20 cm were collected in each plot, and then mixed together to get about a 500-g composite sample for each plot. Four replicate samples were then analyzed from each composite for each treatment. Soil samples were sieved through a 2-mm mesh and oven-dried for chemical property analysis.
Soil organic carbon (SOC) was assayed by the acidified potassium dichromate (K2Cr2O7-H2SO4) heating method (Bao, 2013). Total nitrogen (N) was determined using the Kjeldahl procedure (Bao, 2013); total phosphorus (P) was determined using H2SO4 + HClO4 digestion (Bao, 2013); available N was determined by the alkaline diffusion method (Bao, 2013); available P was exacted with 0.5 mol/L NaHCO3 (pH 8.5) (Bao, 2013).
2.6 Investigation of grassland vegetation
Within each plot, a 1 × 1 m quadrat was set aside for analyzed vegetation in September in 2017 and again in 2018. The total coverage and average height of vegetation was evaluated according to point frame method (Byrne et al., 2011), then the aboveground biomass was harvested, dried at 60°C for 48 h, and weighed (Bloor, 2015).
2.7 Statistical analyses
Two-way ANOVAs were applied to detect the effects of shelters, sheep manure, and shelters × manure on each determined soil and plant index, respectively. And when the results of two-way ANOVAs shown interaction effect is significant, the simple effect analysis, thus One-way analysis of variance (ANOVA), was used to determine the difference between the effects of one factor at different levels of another factor. Regression analysis was applied to reveal the correlation of manure decomposition rate and soil water storage, as well as branch decomposition rate and soil water storage. Correlation analysis was used to reveal the relationships between plant growth parameters and tested soil properties. All above analyses were carried out using the “vegan” package in R. In all tests, a p-value ≤0.05 was considered significant.
3 Results
There was slightly more rainfall in 2018 (374.6 mm) than in 2017 (353.4 mm), however in 2017 it was distributed as smaller but more frequent storms throughout the growing season (Figures 2A, B). Average air temperature was hotter in 2017 than in 2018 (Figures 2C, D). Soil temperatures reached a maximum of 45°C in 2017, but maximums were 10° lower in 2018, at 35°C (Figures 2E, F).
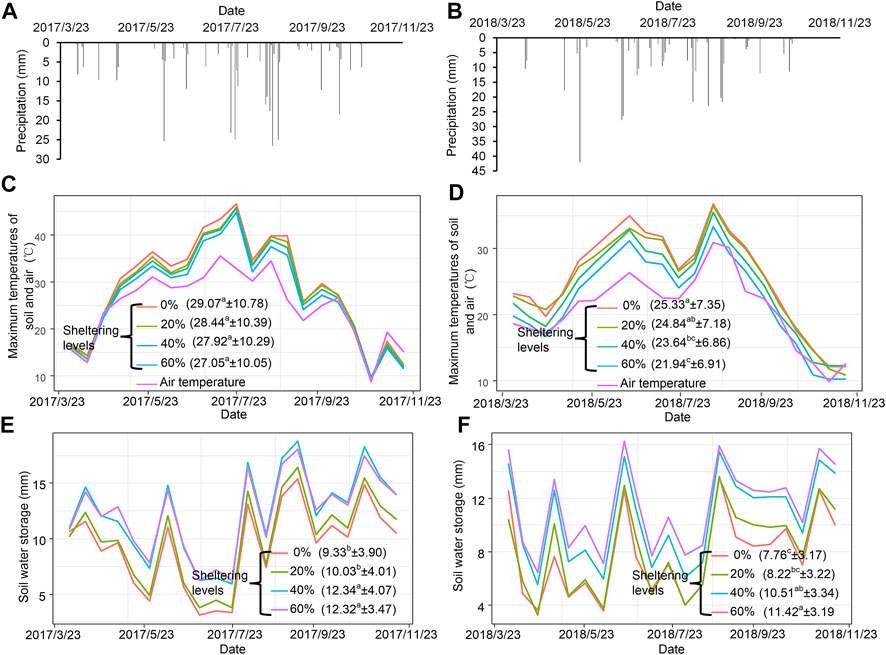
FIGURE 2. The precipitation (A,B) over the two growing seasons, and the maximum soil temperatures (C,D) and soil water storages (E,F) of degraded grassland under different branch sheltering levels in 2017 and 2018. Data in parentheses were average soil temperature ±SE (C,D) and average soil water storage ±SE (E,F), and different letters indicate significant differences among different treatments at the 0.05 level.
3.1 Effect of shelters on soil water storage and soil temperature of grassland
Branch shelters significantly decreased the average daily soil maximum temperature in both 2017 and 2018 (Figures 2C, D). Increasing the extent of branch shelter up to 60% resulted in 2°C cooler average soil temperatures in 2017, and in 3.4°C cooler soil temperatures in 2018, when compared with unsheltered exposed soil.
Soil water storage was overall slightly higher in 2017 than in 2018. There was a significant increase in average soil water storage associated with the 40% and 60% branch shelters as compared to the 0% Control in both 2017 and 2018 (Figures 2E, F). The 60% shelter increased the average soil water storage by 32% and 47.2% over the Controls in 2017 and 2018, respectively. The 20% branch shelter also increased soil water storage above the Control on several days, but was not statistically significant on average. In contrast, increasing the amount of sheep manure, without an associated shelter, was not found to significantly increase soil water storage or decrease soil temperature in either 2017 or 2018 (p > 0.05) (Supplementary Figure S1).
3.2 Effect of branch shelters on decomposition and nutrient releases of manure and branches
Two-way ANOVAs indicated that branch shelters significantly impacted both the manure and branch decomposition rates both in 2017 and 2018 (p < 0.001), evaluated as % weight loss from the initial weight. Manure concentrations also significantly impacted its own decomposition in 2018 (p < 0.001), and branch shelters × manure concentrations significantly impacted manure decomposition as well both in 2017 and 2018 (p < 0.05 or 0.001) (Figure 3). By the end of 2017, Control manure bags had lost ∼25% of their initial mass, while treatments with shelters of 40% and 60% significantly increased the decomposition loss rate of the manure to roughly 50% (Figure 3A). In 2018, manure bags with 0% or 20% shelters had only lost an additional 5% of their initial weight but bags in the 40% and 60% shelters dramatically lost up to 65% of their initial weights (Figure 3C).
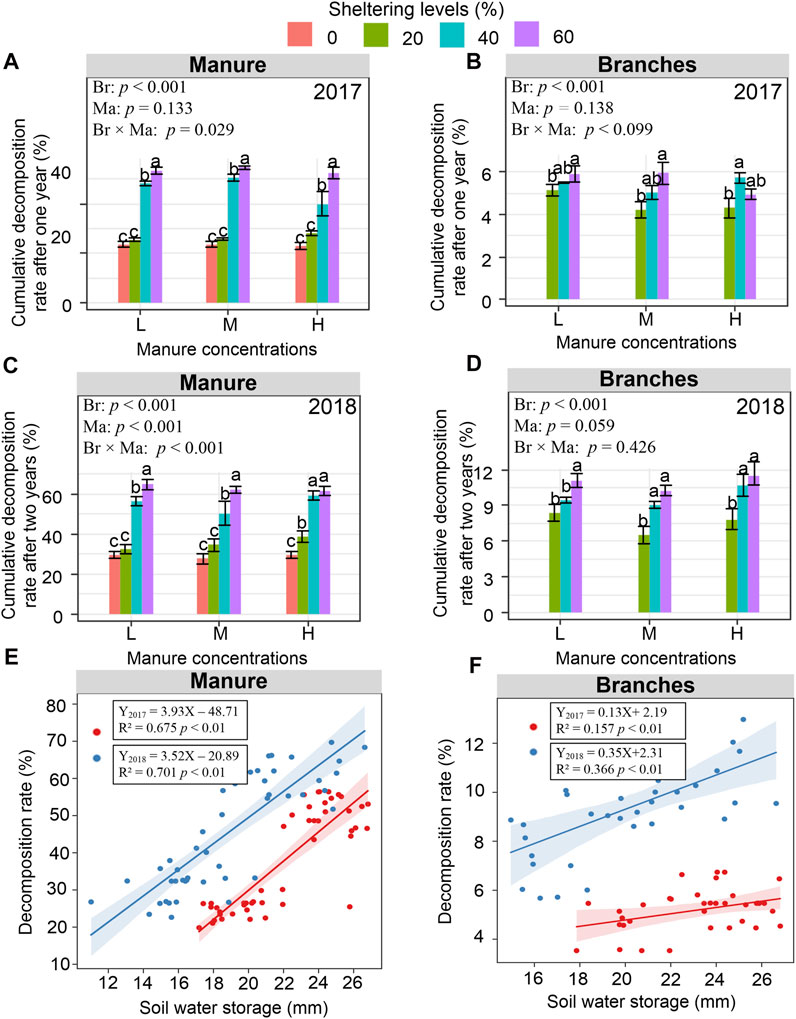
FIGURE 3. Decomposition rates of manure (A,C) and caragana branches (B,D) under different treatments evaluated as % weight loss from the initial weight, as well as regressions of decomposition rate against soil water storage (mm) for manure treatments (E) and caragana treatment (F) in 2017 and 2018. Different letters above columns in Figs a, b, c, and d indicate significant differences among different sheltering levels in Figs a and b (p < 0.05). Different p values in Figs a, b, c, and d show the effects of branch sheltering (Br), manure concentration (Ma), and their interaction (Br × Ma) on decomposition by two-way ANOVAs.
In contrast, caragana branches had very low decomposition rates, with less than 5% loss rate at the end of 2017 (Figure 3B). Branch bags had only lost an additional 5% more after the next 2018 growing season (Figure 3D). However, increasing density of the shelter did slightly increase the decomposition rate of the caragana bags. Further analysis showed that, as a whole, the decomposition rates of manure were increased with increasing branch sheltering levels both in 2017 and 2018. In addition, the decomposition rate of caragana branches also increased with increasing sheltering levels both in 2017 and 2018. Regression analysis revealed that both the manure decomposition and branch decomposition rates were positively correlated with soil water storage (p < 0.05 or 0.01) (Figure 3E and Figure 3F).
The potential maximum C and N which manure and branches inputted into soil were estimated based on the rates of decomposition (Table 1). Branch treatments had no substantial effect in inputting C and N into soil during the whole experimental period. However, with increased sheltering levels, the potential of manure inputted C and N did increase. Manure had potential input of maximum C into the soil between 0.07—0.33 g kg−1 in 2017 and 0.06 g kg−1—0.29 g kg−1 C in 2018. Potential maximum inputs of Nitrogen into the soil from manure were 0.01–0.02 g kg−1 both in 2017 and in 2018.
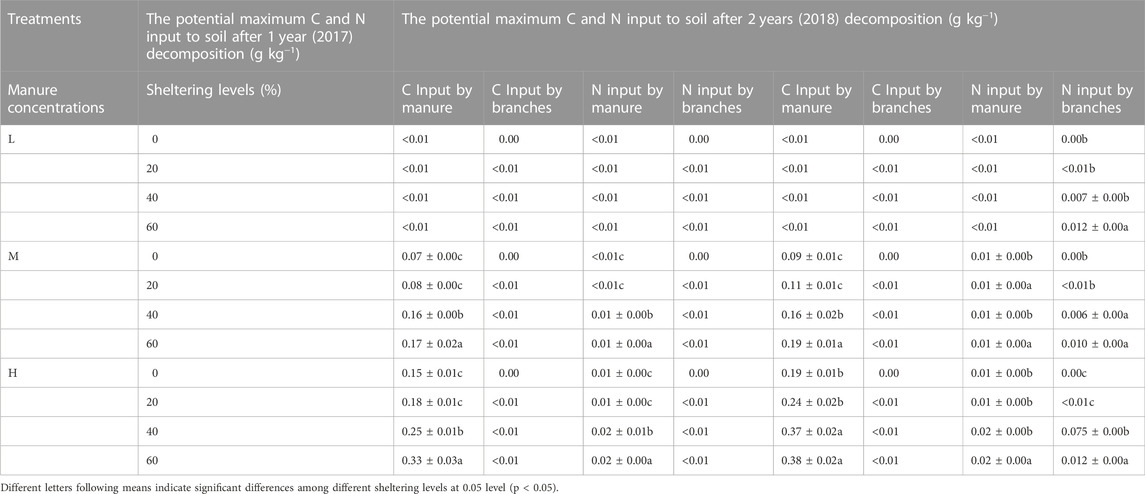
TABLE 1. The evaluation of potential maximum C and N which decomposed sheep manure and caragana branches input to soil.
3.3 Effect of branch shelters on soil nutrients of grassland
Overall, branch shelter, sheep manure, and branch shelter × sheep manure generally had a positive effect on enhancing soil nutrients (Figure 4). However, patterns differed depending on year and on chemical species. SOC started with a low concentration of approximately 2.5 g kg−1 in the Controls in 2017. SOC was significantly increased in the High manure treatments, when there were increases in branch sheltering levels. SOC responded more strongly to the increase in branch shelters in 2018 with a maximum concentration of 3.7 g kg−1g under High manure and 60% shelter. Increasing manure amounts, and especially increasing sheltering levels, resulted in increased concentrations of Total P, Available N, and Available P in both 2017 and 2018. However, Total N concentrations overall decreased by half in the second year, whereas Available N almost doubled in the second year. In contrast, phosphorus concentrations exhibited the reverse pattern, with Total P increasing from year one to year two while Available P was reduced to less than 10% in the second year.
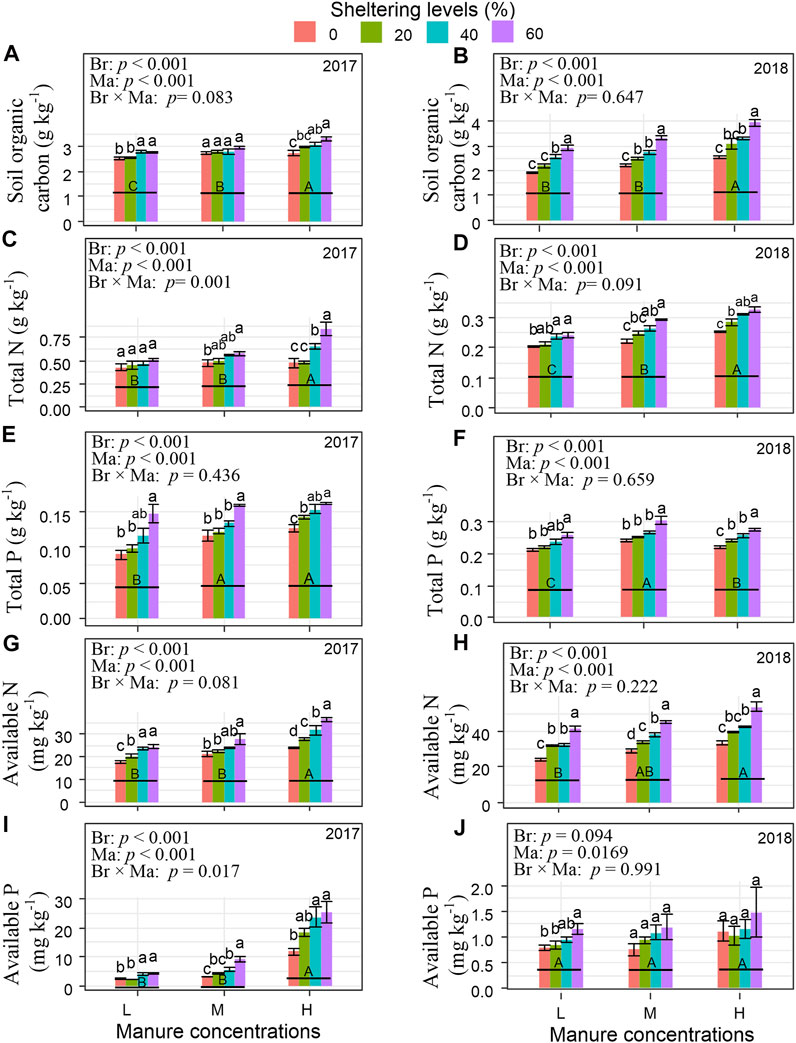
FIGURE 4. Soil organic carbon (A,B), total N (C,D), total P (E,F), available N (G,H), and available P (I,J) between 0–20 cm depth under different shrub branch sheltering levels and cumulative sheep manure concentrations in degraded grassland in 2017 and 2018. L, M, and H denote sheep manure with low, medium, and high concentration in grasslands. Different uppercase letters indicate significant differences among different concentrations of sheep manure (p < 0.05), while lowercase letters indicate significant differences among different levels of shrub branch shelters (p < 0.05). Different p values show the effects of branch sheltering (Br), manure concentration (Ma), and their interaction (Br × Ma) on soil nutrients by two-way ANOVAs.
3.4 Effect of branch shelters on plant growth of grassland
Under the lowest manure treatment, and without branch shelters, plant growth at the end of 2017, was extremely low, measured respectively as average coverage (20%), average height (12 cm), and biomass (30 g m−2) (Figures 5A, C, E). Without the addition of branch shelters, increasing the amount of sheep manure from Low to High in year 1, during the plant establishment phase, resulted in limited plant growth and only slightly increased plant biomass (Low avg 32.1 g m−2; High avg 69.7 g m−2; Figure 5E). Without shelters in year 2, the final biomass was approximately four-fold higher in the Low manure treatment (Avg 128.4 g m−2) as compared with year 1, but the plant growth was significantly higher in the Medium manure treatment (Avg 167.4 g m−2) and High manure treatment (Avg 192 g m−2) than Low manure treatment. Addition of branch shelters to the Low manure treatment, significantly increased the plant biomass production in year 1 as compared with no shelters (60% and Low manure in year 1: avg 134.3 g m−2).
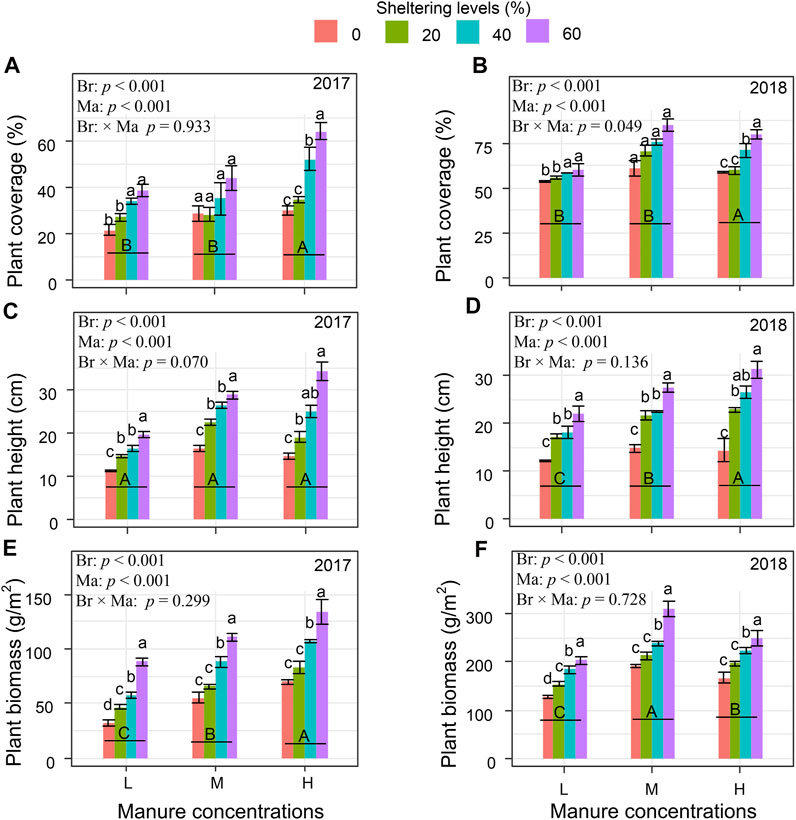
FIGURE 5. Plant coverage (A,B), height (C,D), and biomass (E,F) under different shrub branch sheltering levels and cumulative sheep manure concentrations in degraded grassland in 2017 and 2018. L, M, and H denote sheep manure with low, medium, and high concentration in grasslands. Different uppercase letters indicate significant differences among different concentrations of sheep manure (p < 0.05), while lowercase letters indicate significant differences among different levels of shrub branch shelter (p < 0.05). Different p values show the effects of branch sheltering (Br), manure concentration (Ma), and their interaction (Br × Ma) on plant growth by two-way ANOVAs.
When manure and shelter treatments were combined, there were impressive increases in production. During the plant establishment phase in year 1, High amounts of manure combined with 60% sheltering level resulted in an average 134.3 g m−2 of biomass (Figure 5E), and by the end of year 2, biomass under Medium manure had reached 238.4 g m−2 and 310.9 g m−2 under the 40% and 60% shelters respectively (Figure 5F). Plant biomass in year two under High manure was comparable or slightly lower than Medium manure, under the same sheltering levels. Similar patterns were observed for the two other indices of plant response, i.e., plant coverage and plant height. However in all cases, plant biomass was dominated by, or consisted solely of one native annual species, Artemisia scoparia.
Correlation analysis revealed that all tested soil properties positively correlated with plant heigh and biomass (p < 0.01), yet not correlated with plant coverage (p > 0.05) in 2017 (Table 2). However, in 2018, all tested soil properties (except available P) were positively correlated with plant coverage, height, and biomass (p <0.01 or 0.05; Table 2).
4 Discussion
Overall, the results of this study demonstrated that combining two readily available and inexpensive resources, sheep manure and caragana branches in the form of a branch shelter, can significantly improve the soil environment and foster native plant growth in the severely degraded grassland soils, such as those of Ningxia, China. The branch shelters act to shade and cool the soil, and help maintain significantly higher soil moisture availability. This pattern was consistent across the two growing seasons. Addition of manure significantly increased the availability of nitrogen and phosphorus for plant growth. Application of manure alone, which is the traditional amendment approach, only had a minor effect on soil properties and plant growth, even at the highest levels. In contrast, increasing the branch sheltering level up to 60%, at the lowest manure rate, doubled plant production which suggests that limited water availability may be a stronger driver in these semi-arid environments. In fact, after 2 years, treatments with the highest amounts of both branch shelters and manure amendments combined resulted in a nearly doubling of the soil organic carbon and greatly boosted plant production to 311 g m−2 (3,110 kg ha−1) by the end of year 2. Continued observations of the plants after the study ended indicated that they maintained enhanced production under the amendments in the subsequent year as well.
Notably, plant biomass in the treatment plots was dominated by a single native annual, wormwood Artemisia scoparia. Although the substantial increase in plant production can help jumpstart ecosystem processes and begin the self-healing of the grassland, additional inputs would likely be needed, either in the form of seeds or seedlings, to more quickly re-establish the diverse grassland flora communities key to a stable and resilient ecosystem. This simple and inexpensive solution employing readily available amendment materials has strong potential for application in other severely degraded landscapes. The research findings also provide new insights into the valuable benefits of a different form of organic amendment, namely branch shelters derived from encroaching shrubs, to help restored degraded grasslands.
In arid and semiarid regions, agricultural production is being exacerbated by increasing droughts associated with climate change (Pachauri and Meyer 2014). Hence, improvement of soil water capture and availability is pivotal in these water-limited regions and our research provides useful new insights about the role and efficacy of woody amendments in this regard. This study demonstrated that soil water availability can be significantly increased by the use of branch shelters in degraded soils. Our previous research demonstrated that branch shelters combined with woodchip incorporation into the soil could increase average soil water content by as much as 5 mm above the unamended soil, (from 7 to 11 mm in year 2012 and 11–16 mm in the same plots 3 years later) and by ∼ 2 mm above the unamended soil in the woodchip treatment without branches in the same time periods (Li et al., 2019). This was a substantial improvement in soil water availability given the annual rainfall of ∼150 mm during both growing seasons. In comparison, the current study demonstrated that only using branch shelters, with no woodchip incorporation, had an average increase of ∼3.5 mm in soil water storage, under conditions of more than twice the total rainfall, and also decreased the maximum soil temperatures by at least 2°. Although less effective at improving soil moisture than the combined branch plus woodchips, the branch shelters alone are a simpler solution to implement than incorporation of woodchips into the soil. The successful use of branch shelters provides a new and complementary type of organic amendment, along with mulch, composts, and woodchip incorporation, that can help to restore degraded grasslands.
The impacts of the woody amendments are apparently complicated by the specific temperature and precipitation regimes experienced in a given year. The study year 2018 was significantly cooler and only slightly dryer than 2017, which may explain why the branch shelter treatments exhibited greater success in reducing soil temperatures and increasing soil water storage in the second year. But, when the results of all these studies are integrated, it is clear that woody amendments can play a key role in restoring grassland soils, with significant reductions in soil temperatures and increases in soil water availability. These results can be achieved with just the use of branch shelters, with greater benefits achieved by combining shelters with woodchip or manure incorporation into the soil.
A few other studies have also corroborated this finding. For example, the use of long plum tree branches increased soil moisture in the jujube orchard in the Loess Plateau of China (Yang et al., 2010). Even loose piles of wood latticed above the forest floor achieved similar benefits of cooler temperatures and higher soil moisture contents (Morreale and Sullivan, 2010). It is likely that the use of branch shelters retains soil moisture through a combination of processes including increasing the boundary layer thickness, which acts to reduce evaporation, and by shading (Flerchinger et al., 2003; Fuchs and Hadas, 2011). However, more research is needed to understand the underlying mechanisms so that appropriate restoration applications can be developed. For example, plastic branch shelters may not provide the same benefits as wood branches, because they can’t absorb and release water and contribute to evaporative cooling. In a similarly vein, our previous research showed that rubber woodchips did not provide the same benefits as true woodchips incorporated into the soil because they couldn’t absorb and then release water (Menzies-Pluer et al., 2020).
In all, the utility of small branch material for ecological restoration is particularly useful given that shrub encroachment has become a global nuisance contributing to grassland degradation. For instance, previous studies have showed that caragana growth depleted or even exhausted soil water of the grassland in our study site (Zhao et al., 2020). Historically, these invasive shrubs are removed mechanically, or herbicides and fire are used to destroy the shrubs (Killgorea et al., 2009; Wang et al., 2018). However, our study suggests that shrubs can be cut and used for shelter for grassland restoration in the future.
This study also revealed that utilizing Tan sheep manure as a soil amendment proved to be a vital strategy for soil nutrient enhancement in a region where this material is widely available. Previous studies have shown that 75%–95% of the nitrogen of the forages consumed by grazing livestock is returned to the grassland in the form of both manure and urine (Cai et al., 2014). Schuman et al. (2002) also reported that manure deposition by grazing livestock has a significant impact on grassland soil nutrient cycling. However, as has been demonstrated elsewhere, manure decomposition and increased nutrient availability were positively correlated with soil water availability (Zhu et al., 2020). In this study, branch shelter levels of 40%–60% significantly improved soil water content and accelerated manure decomposition, and manure decomposition rate was significantly positively correlated with soil water condition. Manure inputs of C and N into the soil increased as branch sheltering levels increased, and with inputs ranging from 0.07—0.33 g kg−1 C in 2017 and 0.06 g kg−1—0.29 g kg−1 C in 2018, and maximum N inputs were 0.01–0.02 g kg−1 both in 2017 and in 2018. It is acknowledged that the manure used in this study was already 1 year old when it was re-distributed and the experiment started. Much of the nutrients are leached out and/or volatilized after the first few weeks and months post animal defecation (Zhu et al., 2020), so decomposition rates and nutrient inputs of fresher manure would likely be more extreme.
In contrast, decomposition rates of the caragana branches were extremely slow, at approximately 3–6% weight loss per year. They were therefore a limited source of nutrients to the soil and their key role appeared to be increasing soil moisture retention. Serendipitously, the low decomposition rate of branches also translated to a low-cost application for soil restoration underscoring our previous observation that one of the advantages of woody amendments over other organic materials is that the benefits achieved are maintained more than 5 years post implementation (Li et al., 2019). So, we speculate that shrub branch shelters will also benefit grassland restoration for a comparatively long period without requiring replenishment.
Ultimately, our study demonstrated that the use of shrub branch shelters and sheep manure had significant positive effects on plant growth in this degraded grassland. Under the combined treatment of High manure levels and 40—60% coverage by branch shelters, aboveground plant biomass reached ∼300 g m−2 in the second year. This biomass is comparable to levels reported from short-grass prairie steppes of comparable climate in the western Great Plains (Reeves et al., 2021). The current condition of the soil at this site is so degraded that, when unamended, there are insufficient nutrients and water to allow plants to get established and grow. The system had degraded to such a level that it had reached a new stable state or equilibrium condition (Scheffer et al., 2015). Inputs were needed to jumpstart the ecosystem to a different equilibrium. So, this is a feasible solution for plant production in grassland in arid and semiarid areas of China, since water and soil nutrients are regarded as most limiting factors for plant/crop production in these areas (Wang et al., 2016). Actually, this research also found that caragana branch shelter significantly improved soil water content and reduced soil temperature in a degraded grassland, and created a beneficial environment for reseeded forage growth (Zhang R et al., 2021). Our previous studies had also demonstrated that poplar branch shelters improved soil water condition and enhanced plant growth in arid areas (Li et al., 2018, 2019). Similarly, Fehmi et al. (2020) showed that adding a thin layer of wood chips on the surface increased plant growth. Besides, return of livestock manure in typical grasslands (Zhang et al., 2015), alpine grasslands (Min et al., 2014), dry grasslands (Duffková et al., 2015), and desertified grasslands (Bai et al., 2017) had also showed the effectively potential in improving productivity. Wang et al. (2016) also found that, dry matter accumulation and rainfall use efficiency of crop significantly increased as chicken manure application rate increasing (from 750 g m−2–2,250 g m−2) in semiarid areas of China. Our experimental design allowed us to separate the influence of the two different amendment types and demonstrated that it was the combination of cooler temperatures, higher moisture availability and increased nutrients that resulted in the highest plant production. Actually, there was also a very similar study demonstrated that woody branch shelter combined with a thin layer of compost can improve success rates of revegetation in dryland ecosystems by moderating the soil microclimate (such as improving soil moisture and enhancing soil nutrients) (Leger et al., 2022). In this study, the correlation analysis also showed that plant growth was significantly influenced by all tested indices including soil water, soil organic carbon, Total N, Total P, Available N, and Available P during whole period. In conclusion, this study revealed that the improved soil moisture promoted the transformation of manure nutrients and finally enhanced plant growth of grassland (Bang et al., 2005; Aarons et al., 2009; Saarijärvi and Virkajärvi, 2009).
5 Conclusion
This study demonstrated that 40%–60% cover of shrub branch shelters effectively increased soil water availability of degraded grassland, and consequently promoted decomposition of manure and shrub branches. The soil C and N were substantially inputted by decomposed manure rather than shrub branches, while the low decomposition rate of branches also means low-cost application in soil restoration. The combination of shrub branch shelter and manure application also enhanced plant growth via improving soil water availability and soil nutrients. In summary, our study provided a template using shrub branches and livestock manure to restore degraded grassland in arid and semiarid areas.
Data availability statement
The raw data supporting the conclusions of this article will be made available by the authors, without undue reservation.
Author contributions
ZL designed the research. JL and ZL performed the research and wrote the manuscript. RLS and SJM wrote the manuscript. HW, RW, and FW analyzed the data. All authors contributed to the article and approved the submitted version.
Funding
This work was supported by the Key Research and Development Plan of Ningxia (2021BEG03010; 2021BEB04002), Ningxia Natural Science Foundation (2022AAC05013), the National Natural Science Foundation of China (32,260348; 42067022) and the Science and Technology Research Project of Ningxia Higher Education Institutions (NGY2018008).
Conflict of interest
The authors declare that the research was conducted in the absence of any commercial or financial relationships that could be construed as a potential conflict of interest.
Publisher’s note
All claims expressed in this article are solely those of the authors and do not necessarily represent those of their affiliated organizations, or those of the publisher, the editors and the reviewers. Any product that may be evaluated in this article, or claim that may be made by its manufacturer, is not guaranteed or endorsed by the publisher.
Supplementary material
The Supplementary Material for this article can be found online at: https://www.frontiersin.org/articles/10.3389/fenvs.2022.1089645/full#supplementary-material
References
Akiyama, T., and Kawamura, K. (2007). Grassland degradation in China: Methods of monitoring, management and restoration. Grassl. Sci. 53, 1–17. doi:10.1111/j.1744-697X.2007.00073.x
Aarons, S. R., O’Connor, C. R., Hosseini, H. M., and Gourley, C. J. P. (2009). Dung pads increase pasture production, soil nutrients and microbial biomass carbon in grazed dairy systems. Nutr. Cycl. Agroecosyst. 84, 81–92. doi:10.1007/s10705-008-9228-5
Bai, Y., Wei, Z., Lv, S., Yan, R., Wu, R., Wang, T., et al. (2017). Effects of fertilizer application on the yield of forage and nutrient content on desert grassland. Grassl. Prataculture 29, 10–16. CNKI:SUN:NMCY.0.2017-02-002
Bakker, E. S., Olff, H., Boekhoff, M., Gleichman, J. M., and Berendse, F. (2004). Impact of herbivores on nitrogen cycling: Contrasting effects of small and large species. Oecologia 138, 91–101. doi:10.1007/s00442-003-1402-5
Bang, H. S., Lee, J. H., Kwon, O. S., Na, Y. E., Jang, Y. S., and Kim, W. H. (2005). Effects of paracoprid dung beetles (Coleoptera: Scarabaeidae) on the growth of pasture herbage and on the underlying soil. Appl. Soil Ecol. 29, 165–171. doi:10.1016/j.apsoil.2004.11.001
Bloor, J. M. G. (2015). Additive effects of dung amendment and plant species identity on soil processes and soil inorganic nitrogen in grass monocultures. Plant Soil 396, 189–200. doi:10.1007/s11104-015-2591-5
Bulmer, C., Venner, K., and Prescott, C. (2007). Forest soil rehabilitation with tillage and wood waste enhances seedling establishment but not height after 8 years. Can. J. For. Res. 37, 1894–1906. doi:10.1139/X07-063
Byrne, K. M., Lauenroth, W. K., Adler, P. B., and Byrne, C. M. (2011). Estimating aboveground net primary production in grasslands: A comparison of nondestructive methods. Rangel. Ecol. Manag. 64, 498–505. doi:10.2111/REM-D-10-00145.1
Cai, Y., Wang, X., Tian, L., Zhao, H., Lu, X., and Yan, Y. (2014). The impact of excretal returns from yak and Tibetan sheep dung on nitrous oxide emissions in an alpine steppe on the qinghai-Tibetan plateau. Soil Biol. Biochem. 76, 90–99. doi:10.1016/j.soilbio.2014.05.008
Caracciolo, D., Istanbulluoglu, E., Noto, L. V., and Collins, S, L. (2016). Mechanisms of shrub encroachment into Northern Chihuahuan Desert grasslands and impacts of climate change investigated using a cellular automata model. Adv. Water Resour. 91, 46–62. doi:10.1016/j.advwatres.2016.03.002
Cochran, D. R., Gilliam, C. H., Eakes, D. J., Wehtje, G. R., Knight, P. R., and Olive, J. (2009). Mulch depth affects weed germination. J. Environ. Hortic. 27, 85–90. doi:10.24266/0738-2898-27.2.85
Dan, Y., Du, L. T., Wang, L., Ma, L. L., Qiao, C. L., Wu, H. Y., et al. (2020). Effects of planted shrub encroachment on evapotranspiration and its components in desert steppe: A case study in Yanchi county, Ningxia hui autonomous region. Acta Ecol. Sin. 40, 5638–5648. doi:10.5846/stxb201910032066
Deutsch, E. S., Bork, E. W., and Willms, W. D. (2010). Soil moisture and plant growth responses to litter and defoliation impacts in Parkland grasslands. Agric. Ecosyst. Environ. 135, 1–9. doi:10.1016/j.agee.2009.08.002
Dodd, M. B., McGowan, A. W., Power, I. L., and Thorrold, B. S. (2005). Effects of variation in shade level, shade duration and light quality on perennial pastures. N. Z. J. Agric. Res. 48, 531–543. doi:10.1080/00288233.2005.9513686
D’Odorico, P., Okin, G. S., and Bestelmeyer, B. (2012). A synthetic review of feedbacks and drivers of shrub encroachment in arid grasslands. Ecohydrology 5, 520–530. doi:10.1002/eco.259
Dong, C., Wang, W., Liu, H., Xu, X., and Zeng, H. (2019). Temperate grassland shifted from nitrogen to phosphorus limitation induced by degradation and nitrogen deposition: Evidence from soil extracellular enzyme stoichiometry. Ecol. Indic. 101, 453–464. doi:10.1016/j.ecolind.2019.01.046
Duffková, R., Hejcman, M., and Libichová, H. (2015). Effect of cattle slurry on soil and herbage chemical properties, yield, nutrient balance and plant species composition of moderately dry arrhenatherion, grassland. Agric. Ecosyst. Environ. 213, 281–289. doi:10.1016/j.agee.2015.07.018
Eldridge, D. J., and Koen, T. B. (2003). Detecting environmental change in eastern Australia: Rangeland health in the semi-arid woodlands. Sci. Total Environ. 310, 211–219. doi:10.1016/S0048-9697(02)00641-1
Fehmi, J. S., Rasmussen, C., and Gallery, R. E. (2020). Biochar and woodchip amendments alter restoration outcomes, microbial processes, and soil moisture in a simulated semi-arid ecosystem. Restor. Ecol. 28, 355–364. doi:10.1111/rec.13100
Flerchinger, G. N., Sauer, T. J., and Aiken, R. A. (2003). Effects of crop residue cover and architecture on heat and water transfer at the soil surface. Geoderma 116, 217–233. doi:10.1016/S0016-7061(03)00102-2
Foley, J. A., Ramankutty, N., Brauman, K. A., Cassidy, E. S., Gerber, J. S., Johnston, M., et al. (2011). Solutions for a cultivated planet. Nature 478, 337–342. doi:10.1038/nature10452
Fuchs, M., and Hadas, A. (2011). Mulch resistance to water vapor transport. Agric. Water Manag. 98, 990–998. doi:10.1016/j.agwat.2011.01.008
Gang, C., Zhou, W., Chen, Y., Wang, Z., Sun, Z., Li, J., et al. (2014). Quantitative assessment of the contributions of climate change and human activities on global grassland degradation. Environ. Earth Sci. 72, 4273–4282. doi:10.1007/s12665-014-3322-6
Jia, X., Shao, M., Yu, D., Zhang, Y., and Binley, A. (2018). Spatial variations in soil-water carrying capacity of three typical revegetation species on the Loess Plateau, China. Agric. Ecosyst. Environ. 273, 25–35. doi:10.1016/j.agee.2018.12.008
Kerley, G. I. H., and Whitford, W. G. (2009). Can kangaroo rat graminivory contribute to the persistence of desertified shrublands. J. Arid Environ. 73, 651–657. doi:10.1016/j.jaridenv.2009.01.001
Killgorea, A., Jacksonb, E., and Whitfordc, W. G. (2009). Fire in chihuahuan desert grassland: Short-term effects on vegetation, small mammal populations, and faunal pedoturbation. J. Arid Environ. 73, 1029–1034. doi:10.1016/j.jaridenv.2009.04.016
Klumpp, K., Fontaine, S., Attard, E., Le Roux, X., Gleixner, G., and Soussana, J. F. (2009). Grazing triggers soil carbon loss by altering plant roots and their control on soil microbial community. J. Ecol. 97, 876–885. doi:10.1111/j.1365-2745.2009.01549.x
Knops, J. M. H., Bradley, K. L., and Wedin, D. A. (2002). Mechanisms of plant species impacts on ecosystem nitrogen cycling. Ecol. Lett. 5, 454–466. doi:10.1046/j.1461-0248.2002.00332.x
Leger, A. M., Ball, K. R., Rathke, S. J., and Blankinship, J. C. (2022). Mulch more so than compost improves soil health to reestablish vegetation in a semiarid rangeland. Restor. Ecol. 30, e13698. doi:10.1111/rec.13698
Li, Z., Schneider, R. L., Morreale, S. J., Xie, Y., Li, C., and Li, J. (2018). Woody organic amendments for retaining soil water, improving soil properties and enhancing plant growth in desertified soils of Ningxia, China. Geoderma 310, 143–152. doi:10.1016/j.geoderma.2017.09.009
Li, Z., Schneider, R. L., Morreale, S. J., Xie, Y., Li, J., Li, C., et al. (2019). Using woody organic matter amendments to increase water availability and jump-start soil restoration of desertified grassland soils of Ningxia, China. Land Degrad. Dev. 30, 1313–1324. doi:10.1002/ldr.3315
Liu, K., Sollenberger, L. E., Newman, Y. C., Vendramini, J. M. B., Interrante, S. M., and White-Leech, R. (2011). Grazing management effects on productivity, nutritive value, and persistence of ’Tifton 85’ Bermudagrass. Crop Sci. 51, 353–360. doi:10.2135/cropsci2010.02.0122
Luan, J., Cui, L., Xiang, C., Wu, J., Song, H., Ma, Q., et al. (2014). Different grazing removal exclosures effects on soil C stocks among alpine ecosystems in east Qinghai-Tibet Plateau. Ecol. Eng. 64, 262–268. doi:10.1016/j.ecoleng.2013.12.057
Maestre, F. T., Bowker, M. A., Puche, M. D., Hinojosa, M. B., Martínez, I., García-Palacios, P., et al. (2009). Shrub encroachment can reverse desertification in semi-arid Mediterranean grasslands. Ecol. Lett. 12, 930–941. doi:10.1111/j.1461-0248.2009.01352.x
Menzies-Pluer, E. G., Schneider, R. L., Morreale, S. J., Liebig, M. A., Li, J., Li, C. X., et al. (2020). Returning degraded soils to productivity: An examination of the potential of coarse woody amendments for improved water retention and nutrient holding capacity. Water Air Soil Pollut. 231, 15. doi:10.1007/s11270-019-4380-x
Min, X., Ma, Y., Li, S., and Wang, Y. (2014). Effects of sheep manure on productivity and nutrition of soil for Poa pratensis cv. Qinghai pasture. Pratacultural Sci. 31, 1039–1044. doi:10.11829/j.issn.1001-0629.2013-0572
Morreale, S. J., and Sullivan, K. L. (2010). Community-level enhancements of biodiversity and ecosystem services. Front. Earth Sci. China 4, 14–21. doi:10.1007/s11707-010-0015-7
Oenema, O., and Tamminga, S. (2005). Nitrogen in global animal production and management options for improving nitrogen use efficiency. Sci. China. C Life Sci. 48, 871–887. doi:10.1007/BF03187126
Pachauri, R. K., and Meyer, L. A. (2014). “Contribution of working groups I, II and III to the fifth assessment report of the intergovernmental panel on climate change,” in Climatic change 2014 synthesis report (Geneva: IPCC).
Rashid, M. I., Lantinga, E. A., Brussaard, L., and de Goede, R. G. M. (2017). The chemical convergence and decomposer control hypotheses explain solid cattle manure decomposition in production grasslands. Appl. Soil Ecol. 113, 107–116. doi:10.1016/j.apsoil.2017.02.009
Reeves, C. R., Hanberry, B. B., Wilmer, H., Kaplan, N. E., and Lauenroth, W. K. (2021). An assessment of production trends on the Great Plains from 1984 to 2017. Rangel. Ecol. Manag. 78, 165–179. doi:10.1016/j.rama.2020.01.011
Reichert, J. M., Gubiani, P. I., dos Santos, D. R., Reinert, D. J., Aita, C., and Giacomini, S. J. (2022). Soil properties characterization for land-use planning and soil management in watersheds under family farming. Int. Soil Water Conservation Res. 10, 119–128. doi:10.1016/j.iswcr.2021.05.003
Saarijärvi, K., and Virkajärvi, P. (2009). Nitrogen dynamics of cattle dung and urine patches on intensively managed boreal pasture. J. Agric. Sci. 147, 479–491. doi:10.1017/S0021859609008727
Scheffer, M., Carpenter, S. R., Dakos, V., and van Nes, E. H. (2015). Generic indicators of ecological resilience: Inferring the chance of a critical transition. Annu. Rev. Ecol. Evol. Syst. 46, 145–167. doi:10.1146/annurev-ecolsys-112414-054242
Schuman, G. E., Janzen, H. H., and Herrick, J. E. (2002). Soil carbon dynamics and potential carbon sequestration by rangelands. Environ. Pollut. 116, 391–396. doi:10.1016/S0269-7491(01)00215-9
Wang, G., Li, J., Sujith, R., David, D., Gonzales, H. B., and Sankey, J. B. (2018). Post-fire redis-tribution of soil carbon and nitrogen at a grassland– shrubland ecotone. Ecosystems 22, 174–188. doi:10.1007/s10021-018-0260-2
Wang, X., Jia, Z., Liang, L., Yang, B., Ding, R., Nie, J., et al. (2016). Impacts of manure application on soil environment, rainfall use efficiency and crop biomass under dryland farming. Sci. Rep. 6, 20994. doi:10.1038/srep20994
Watson, P. A., Alexander, H. D., and Moczygemba, J. D. (2018). Coastal prairie recovery in response to shrub removal method and degree of shrub encroachment. Rangel. Ecol. Manag. 72, 275–282. doi:10.1016/j.rama.2018.11.005
Weedon, J. T., Cornwell, W. K., Cornelissen, J. H. C., Zanne, A. E., Wirth, C., and Coomes, D. A. (2009). Global meta-analysis of wood decomposition rates: A role for trait variation among tree species? Ecol. Lett. 12, 45–56. doi:10.1111/j.1461-0248.2008.01259.x
Wei, N., Zhao, L. P., Tan, S. T., and Zhao, F. R. (2019). Research progress on shrub encroachment in grasslands. Ecol. Sci. 38, 208–216. doi:10.14108/j.cnki.1008-8873.2019.06.030
Wilcox, B. P., Birt, A., Fuhlendorf, S. D., and Archer, S. R. (2018). Emerging frameworks for understanding and mitigating woody plant encroachment in grassy biomes. Curr. Opin. Environ. Sustain. 32, 46–52. doi:10.1016/j.cosust.2018.04.005
Wilson, B. S., Jensen, W. E., Houseman, G. R., Jameson, M. L., Reichenborn, M. M., Watson, D. F., et al. (2022). Cattle grazing in CRP grasslands during the nesting season: Effects on avian abundance and diversity. J. Wildl. Manage. 86. doi:10.1002/jwmg.22188
Xu, S., Sayer, E. J., Eisenhauer, N., Lu, X., wang, J., and Liu, C. (2021). Aboveground litter inputs determine carbon storage across soil profiles: A meta-analysis. Plant Soil 462, 429–444. doi:10.1007/s11104-021-04881-5
Xu, Z., Gao, L., Wang, L., Cheng, S., Zhang, X., and Wu, J. (2012). Impacts of dung combustion on carbon cycle of grassland ecosystem. Resour. Sci. 34, 1062–1069. doi:10.1007/7588(2012)06-1062-08
Yang, Z., Wang, Y., Zhao, Y., Li, P., and Dan, X. (2010). Influence of branch cover and water retaining agent on soil water. J. Irrigation Drainage 29, 97–99. doi:10.1016/S1002-0160(10)60014-8
Zhang, Q., Liu, K., Shao, X., Li, H., He, Y., Sirimuji, Wang B., et al. (2021). Microbes require a relatively long time to recover in natural succession restoration of degraded grassland ecosystems. Ecol. Indic. 129, 107881. doi:10.1016/j.ecolind.2021.107881
Zhang, R., Li, J., Peng, W., Wang, F., and Li, Z. (2021). Effects of mulching with caragana (Caragana intermedia) branches on soil moisture content and temperature and reseeded forage biomass in desertified grassland in Ningxia Province, China. Acta Prataculturae Sin. 30, 58–67. doi:10.11686/cyxb2020172
Zhang, Y., Xie, Y., Ma, H., Zhang, J., Jing, L., Wang, Y., et al. (2021). The responses of soil respiration to changed precipitation and increased temperature in desert grassland in northern China. J. Arid Environ. 193, 104579. doi:10.1016/j.jaridenv.2021.104579
Zhang, Y., Yang, S., Fu, M., Cai, J., Zhang, Y., Wang, R., et al. (2015). Sheep manure application increases soil exchangeable base cations in a semi-arid steppe of Inner Mongolia. J. Arid. Land 7, 361–369. doi:10.1007/s40333-015-0004-5
Zhao, Y., Yu, L., Zhou, Y., Wang, H., Ma, Q., and Qi, L. (2020). Soil moisture dynamics and deficit of desert grassland with anthropogenic introduced shrub encroachment in the eastern Ningxia, China. Acta eco. Sin. 40, 1305–1315. doi:10.5846/stxb201812152735
Zheng, Q., Du, L., Gong, F., Dan, Y., and Wang, L. (2019). Landscape characteristics of caragana intermedia plantation based on GF-1 remote sensing image in Yanchi. J. southwest For. Univ. Nat. Sci. 39, 152–159. doi:10.11929/j.swfu.201808028
Keywords: caragana branch shelters, soil water availability, sheep manure decomposition, soil nutrients, plant growth, degraded grassland
Citation: Liu J, Schneider RL, Morreale SJ, Wang H, Wang R, Wang F and Li Z (2023) The roles for branch shelters and sheep manure to accelerate the restoration of degraded grasslands in northern China. Front. Environ. Sci. 10:1089645. doi: 10.3389/fenvs.2022.1089645
Received: 04 November 2022; Accepted: 02 December 2022;
Published: 04 January 2023.
Edited by:
Jianping Li, Ningxia University, ChinaReviewed by:
Shaokun Wang, Northwest Institute of Eco-Environment and Resources (CAS), ChinaHao Qu, Cold and Arid Regions Environmental and Engineering Research Institute, Chinese Academy of Sciences, China
Copyright © 2023 Liu, Schneider, Morreale, Wang, Wang, Wang and Li. This is an open-access article distributed under the terms of the Creative Commons Attribution License (CC BY). The use, distribution or reproduction in other forums is permitted, provided the original author(s) and the copyright owner(s) are credited and that the original publication in this journal is cited, in accordance with accepted academic practice. No use, distribution or reproduction is permitted which does not comply with these terms.
*Correspondence: Zhigang Li, bGl6ZzAwMUBzaW5hLmNvbQ==