- 1School of Geographic Sciences, Hunan Normal University, Changsha, China
- 2CAS Key Laboratory of Soil Environment and Pollution Remediation, Institute of Soil Science, Chinese Academy of Sciences, Nanjing, China
- 3Rural Vitalization Research Institute, Changsha University, Changsha, China
Root exudates are carriers for the transfer of material, energy and information between plant roots and soils. Plants encountering environmental stresses such as heavy metal pollution adapt to the environment by producing and secreting root exudates. In this study, laboratory soil culture experiment and pot experiment with Sedum plumbizincicola were used to study the effects of single and combined application of three root exudates, citric acid, glycine, and fructose, on the Cd-activation and phytoremediation of Cd-contaminated paddy soil. Results from the soil culture experiment showed that for the single application of root exudates, all three root exudates significantly activated the Cd in soil as presented by the increased content of diethylenetriamine pentaacetic acid extracted Cd (DTPA-Cd). In Particular, citric acid (SC) at a relatively low concentration (2 mmol/kg) exhibited the best Cd activation efficiency by increasing DTPA-Cd in the soil by 66.12%. For the combined application of root exudates, citric acid in combination with glycine (SC + G, 1:3) had the best activation effect on the Cd in the soil. In the phytoremediation pot experiment, both the single application of citric acid at a low concentrate (1 mmol/kg) and the combined application of citric acid and glycine (1:1) significantly reduced the total Cd and DTPA-Cd in the soil and increased the biomass and the content of Cd in S. plumbizincicola; thus, the phytoremediation efficiency of Cd-contaminated soil increased by 42.33% and 35.61%. The results from this study suggest that citric acid plays a crucial role in Cd activation and phytoremediation with single or combined applications with glycine. However, the mechanisms under the synergetic interaction between citric acid and glycine require further investigation.
1 Introduction
With the development of modern industry and agriculture, environmental pollution is becoming increasingly serious, among which heavy metal pollution is an important aspect. Cadmium (Cd) is a heavy metal with inherent toxicity, non-degradability, persistence, and frequent detection in different matrices, which directly or indirectly plays a critical role in human health due to its bioaccumulation and biomagnification through the food chain and its carcinogenic effects (Wu et al., 2020). According to the National Soil Pollution Survey Bulletin of China issued in 2014, cadmium is one of the most concerning heavy metal pollutants in farmland soil. Thus, green and efficient remediation materials and technologies are urgently needed for the sustainable remediation of Cd-polluted farmland soil.
Sedum plumbizincicola has been recognized as a typical Cd-hyperaccumulating plant since 2006 (Wu et al., 2006). Due to its high Cd uptake ability, large biomass, and suitability for mowing, S. plumbizincicola has been confirmed as a promising plant for the phytoremediation of Cd-contaminated soils (Shen et al., 2011). Plants can directly or indirectly change metal availability and promote the uptake of heavy metals through different mechanisms, such as changes in root exudates and rhizosphere pH (Hammer and Keller, 2002). Root exudates are important plant metabolites that are released from plant roots into the rhizosphere soil to facilitate the uptake of nutrients (Jiang et al., 2023). The composition of root exudates is diverse; for example, Sun et al. (2020) identified 155 compounds in the root exudates of S. plumbizincicola, i.e., 12 organic acids, 14 amino acids, 10 fatty acids, 10 amines, 17 polyols, 17 lipids, 11 carbohydrates, and 64 other substances. The secretion of low-molecular-weight organic acids plays a crucial role in improving plant growth and development and it also significantly alters the speciation and mobility of heavy metals in the soil, thus affecting the stress resistance and/or the phytoremediation efficiency of plants (Jiang et al., 2023). Citric acid is a type of common but important component of root exudates. It can form chelates with Cd, thereby reducing the pH of plant rhizosphere and improving Cd availability in soil. Activated Cd in rhizosphere soil can adsorb to the surface of root cells and transmembranes in root cells and be transported to the aboveground parts, thus improving the efficiency of phytoremediation (Liu et al., 2022). Amino acids are another type of root exudates that are involved in various activities during plant development, including responses to biotic and abiotic stresses (Galili et al., 2016). Total amino acids (AAs) and free amino acids (FAAs) secreted by plant roots are essential for mitigating the toxicity of heavy metals in crops (Xue et al., 2022). In addition, saccharides can improve the activities of particular soil microorganisms, which can effectively increase the activity of heavy metals in the soil through the secretion of protons and organic matter (Zhu et al., 2003). However, previous studies only focused on the effect of one particular root exudate on soil Cd species; the effect of combined root exudates on the remediation efficiency of S. plumbizincicola has rarely been referred.
Therefore, in this study, citric acid, glycine (Gly), and fructose were selected as three typical root exudates to investigate their single and combined effects on Cd activation and phytoremediation efficiency in Cd-contaminated soil. A soil culture experiment, as well as a phytoremediation pot experiment with S. plumbizincicola, was conducted to 1) compare the effects of the three root exudates (citric acid, Gly, fructose) on the activation of total and bioavailable Cd in the soil under single addition treatment; 2) explore the optimal combination of the mixed application of different root exudates; and 3) elucidate the effects of different root exudates on the soil Cd removal, biomass and remediation efficiency of S. plumbizincicola. The results of this study could help enhance the Cd phytoremediation efficiency of S. plumbizincicola, and may provide a theoretical reference for the sustainable remediation of heavy metal-polluted farmland soil.
2 Materials and methods
2.1 Materials
The tested soil was collected from the surface (0–20 cm) of a heavy metal-polluted paddy soil in Xiangtan city, Hunan Province, China. The parent material of the soil was Quaternary red clay. After air drying, the soil was ground and passed through a 2 mm sieve before use. The pH of the soil was 5.12, the organic matter content was 25.2 g/kg, and the contents of total N, total P, and total K were 1.47 g/kg, 0.77 g/kg, and 13.1 g/kg. The total Cd content in the soil was 0.81 mg/kg, which exceeds the national standard for Cd in paddy soil (0.3 mg/kg, GB15618-2018). The available Cd content in the soil was 0.34 mg/kg, which was presented as diethylenetriamine pentaacetic acid extracted Cd (DTPA-Cd). The tested Cd hyperaccumulator was S. plumbizincicola, which was also collected from an experimental base in Xiangtan city. Healthy plants of uniform size were cut to about 10 cm for cutting. The average biomass as measured by the dry mass of S. plumbizincicola cuttings was 1.03 g/pot, and the total Cd was 41.30 mg/kg. Citric acid, glycine (Gly), and fructose were of analytical pure grade and were purchased from the Shanghai Sinophosphoric Medicine Group.
2.2 Soil culture experimental design
The effects of different components, contents and pairwise combinations of S. plumbizincicola’s root exudates on soil pH and DTPA-Cd were studied using laboratory soil culture experiments. It was carried out in a greenhouse at Hunan Normal University for 1 month. Citric acid, Gly and fructose were selected as the target root exudates of S. plumbizincicola according to our previous study (Sun et al., 2020). Four concentrations (0, 2, 4, and 8 mmol/kg) were set for each root exudate in their single application treatment, which was based on both our previous studies (Deng et al., 2020a; Bai et al., 2020; Sun et al., 2020; Liu et al., 2022) and related literature (Wu et al., 2016; Wei, 2017; He et al., 2022). The selected concentrations include the properties of different root exudates and the tolerance of S. plumbizincicola. In the combination experiment, the total concentration of different exudates was 4 mmol/kg, but the specific ratio of the two components varied. The specific experimental design is shown in Table 1. Each treatment was replicated three times.
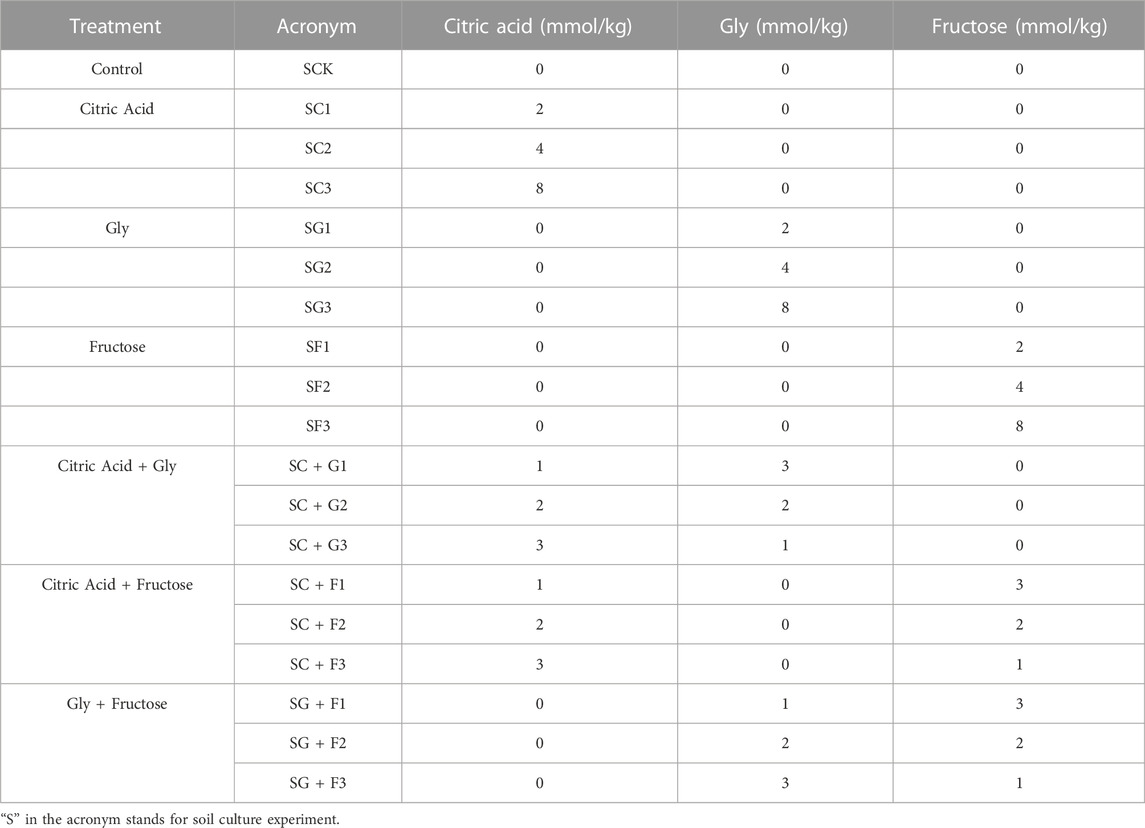
TABLE 1. Experimental design of single and in combination addition of citric acid, Gly and fructose at different concentrations.
For the soil cultivation experiment, 100 g (dry weight) of prepared soil was added to a 250 ml beaker. The soil was first mixed with 10 ml of deionized water, followed by 10 ml of the prepared S. plumbizincicola root exudate. The soil water content was maintained at about 20% during the incubation period.
2.3 Experimental design for pot phytoremediation
A pot experiment was also carried out in a greenhouse at Hunan Normal University for 5 months. It was conducted to study the effects of the single application of citric acid (CA1 = 1 mmol/kg; CA2 = 2 mmol/kg; CA3 = 4 mmol/kg) or the combination with Gly (C + G1 = 1 mmol/kg + 3 mmol/kg; C + G2 = 2 mmol/kg + 2 mmol/kg; C + G3 = 3 mmol/kg + 1 mmol/kg) on the phytoremediation efficiency of S. plumbizincicola in Cd-contaminated soil. Seven treatments were designed in this experiment with three replicates per treatment. One kilogram of the prepared soil (dry weight) was added to each pot, and the soil moisture was maintained at about 70% of the field water-holding capacity. Three cuttings of S. plumbizincicola were planted in each pot. On days 15 and 30 before the end of the experiment, 10 ml citric acid solution and a mixed citric acid + Gly solution were added to the soil by spraying. The same amount of deionized water was also added by spraying in the control treatment (CK). Water and fertilizer management was performed according to field conditions throughout the experiment.
2.4 Sample collection and determination
2.4.1 Sample collection
For the soil culture experiment with single root exudate treatments, soil samples were collected on the days 1, 3, 7, 14 and 28 after the start of the experiment, and for the soil culture experiment with combined root exudate treatments, soil samples were collected on the days 1, 14, and 28. All soil samples were air-dried, ground, and passed through 10, 20, and 100 mesh nylon sieves before use.
For the pot experiment, the S. plumbizincicola samples were harvested at the end of the experiment, washed with tap water to remove soil and rotting leaves, and then washed with deionized water three times. The washed plant samples were deactivated in an oven at 105 °C for 30 min and then dried at 70 °C to constant weight. The dry mass was weighed and recorded as the biomass of whole S. plumbizincicola plant. The dried plant samples were pulverized, passed through a 60-mesh sieve, and stored in a sealed bag until analysis. After the plant samples were removed, the soil in each pot was mixed and air dried and then ground and passed through 10, 20, and 100 mesh nylon sieves before analysis.
2.4.2 Analysis of soil pH
Soil pH was determined by the extraction of soil to water at a ratio of 1:2.5 (m:v) and measured by a PHS-4CT digital pH meter (Deng et al., 2020b). Five grams of soil samples were collected to determine the soil pH in each treatment.
2.4.3 Analysis of Cd in plants and soil
For the analysis of the total Cd in soil and plant samples, digestion was conducted according to the US EPA3051a standard method. The content of heavy metals in S. plumbizincicola was digested by HNO3 (10 ml), and the total amount of heavy metals in the soil was digested by HNO3: HCl (3:1, v/v) mixed acid solution. Digestion was carried out with a microwave digestion instrument (CEM MARS6, Matthews, NC, USA). Cd concentrations in plants and soils were determined using an atomic absorption spectrometer (PinAAcle 900T, Perkin Elmer, USA). In the process of sample testing, a blank test and national standard substances (soil: GBW07406; plant: GBW07603) were used for quality control (Deng et al., 2020a).
Soil available Cd was extracted with 0.05 mol/L DTPA at a soil-to-liquid ratio of 1:5. A PinAAcle 900T atomic absorption spectrometer was used for determination, and national reference materials (GSS, GBE) were used for quality control (Sun, 2021). Cd species in the soil were analyzed by the four-step BCR sequential extraction of a European reference plant (Liu et al., 2021). The method for determination of Cd was the same as that reported above.
2.5 Data processing and statistical analysis
The remediation efficiency was calculated as the amount of Cd absorbed by the plant (PRE). It was estimated as follows, Eqss. 1, 2 (Cao et al., 2019):
Where Q p is the amount of Cd absorbed by S. plumbizincicola, mg/pot; CP2 is the Cd content in S. plumbizincicola after phytoremediation, mg/kg; WP2 is the biomass of S. plumbizincicola after phytoremediation, kg/pot; CP1 is the Cd content in S. plumbizincicola before phytoremediation, mg/kg; WP1 is the biomass of S. plumbizincicola before phytoremediation, kg/pot; WS is the quality of soil in each pot, kg; TC before remediation is the total Cd content in the soil before remediation, mg/kg.
The remediation efficiency was calculated as the amount of reduced Cd in the soil (SRE). It was calculated by the difference in total Cd concentration (mg/kg) before and after remediation following Eq. 3 (Xue et al., 2022):
Excel 2019 and Origin 2021 were used to process data and draw diagrams, and SPSS 20.0 software was used to check the statistical significance of the data among different treatments.
3 Results and discussion
3.1 Effects of single root exudates on soil pH and DTPA-Cd
All three root exudate fractions reduced the soil pH to some extent, and the decrease was more obvious with the increase in their dosage. Figure 1 shows the changes in soil pH under different fractions and dosages of single citric acid, Gly, and fructose. Compared with the control soil (SCK), single citric acid treatment (SC) significantly reduced the soil pH within 1 week after its application, especially on the first day when the pH decreased markedly by 1 unit. Moreover, the decrease in soil pH was positively dosage dependent. Similarly, the pH of soil treated with Gly (SG) also decreased within 1 week after application, with the most significant decrease in soil pH on the first day, and the soil pH in the SG1, SG2, and SG3 treatments decreased by 0.14, 0.26 and 0.43 units, respectively. However, the Gly treatment had no significant effects on soil pH on days 14 and 28. In contrast to citric acid and Gly, the addition of fructose (SF) significantly reduced the soil pH only on day14, and a dose-dependent effect was not observed.
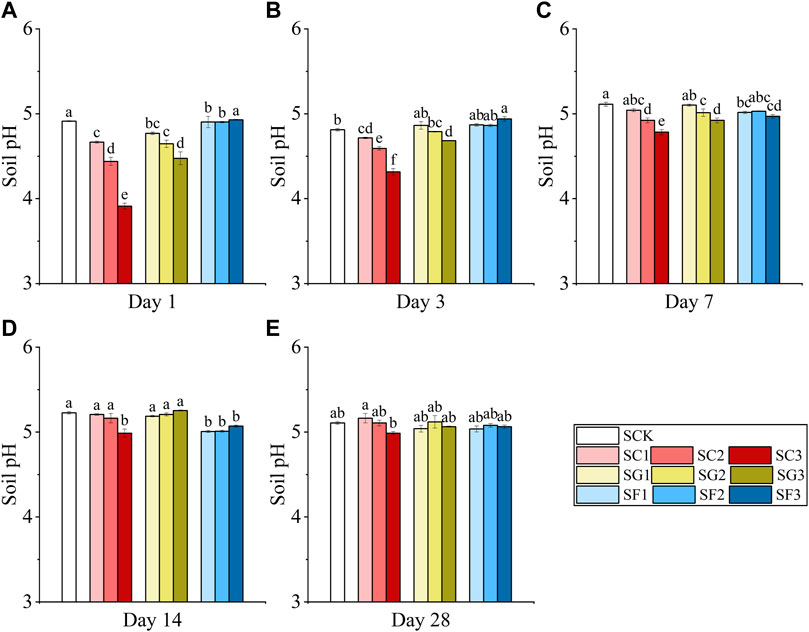
FIGURE 1. Changes in soil pH under different fractions and dosages of single citric acid (SC1, SC2, SC3), Gly (SG1, SG2, SG3), and fructose (SF1, SF2, SF3) application on days 1 (A), 3 (B), 7 (C), 14 (D), and 28 (E) (Different letters in each sampling timepoint show significant differences, p < 0.05).
All three root exudate fractions activated the Cd in the soil and increased the content of available Cd (DTPA-Cd). Figure 2 shows the changes in DTPA-Cd in soil under different fractions and dosages of single citric acid, Gly and fructose. In the citric acid treatment, the content of DTPA-Cd peaked at 0.67 mg/kg on the first day, and the activation efficiency reached 66.12%. Next, DTPA-Cd content continued to decrease until the seventh day. On the seventh day, citric acid was even less effective in reactivating Cd in soil than the Gly and fructose treatments. After day 7, the activation efficiency of citric acid increased and reached a stable state. On the 28th day, the activation efficiency of citric acid was 22.35% higher than that of Gly, 24.16% higher than that of fructose, and 31.27% higher than that of SCK. Furthermore, the DTPA-Cd under a low concentration of citric acid application (SC1 and SC2) was higher than that under a high concentration (SC3). Similarly, Gly also effectively increased the DTPA-Cd content in the soil within 1 week after addition, but there were no significant differences among different dosages. The contents of DTPA-Cd in soil increased by 25.66%–27.87%, 12.15%–18.79% and 21.22%–26.47% on the first, third, and seventh days, respectively, compared with SCK. However, the increase in DTPA-Cd in the soil gradually weakened after 1 week. In addition, the activation effect of fructose on Cd increased on days 1 (0.58 mg/kg) and 3 (0.66 mg/kg), then decreased on days 14 (0.42 mg/kg) and was generally higher than that in the SCK group during the whole experimental period. The activation effect of Cd by citric acid was significantly stronger than that of Gly and fructose during the whole incubation, except for the third and seventh days.
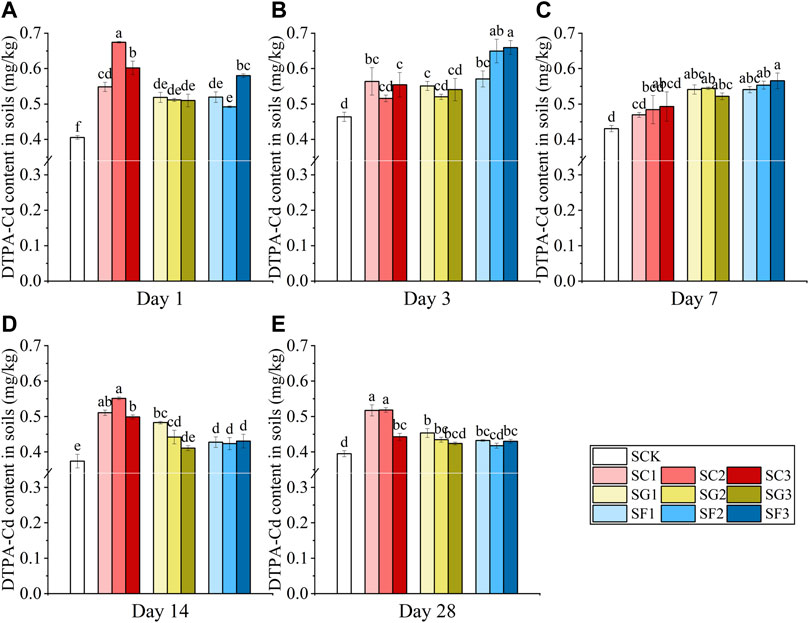
FIGURE 2. Changes in DTPA-Cd in soil under different fractions and dosages of single citric acid (SC1, SC2, SC3), Gly (SG1, SG2, SG3), and fructose (SF1, SF2, SF3) application on days 1 (A), 3 (B), 7 (C), 14 (D), and 28 (E) (Different letters in each sampling timepoint show significant differences, p < 0.05).
Some organic acids, amino acids and other substances in the S. plumbizincicola root exudates can change the acidity and alkalinity of the rhizosphere soil. The concentration of H+ in an aqueous solution was positively correlated with the concentration of organic acids and amino acids. The release of more H+ in the aqueous solution reduces the electronegativity of the soil surface, weakens the adsorption capacity of soil for heavy metals, and thus promotes the desorption of heavy metals (Tang et al., 2022). In this experiment, different concentrations of citric acid and Gly rapidly decreased the pH of the soil in a short period, and with the increase in the applied concentration, the soil pH decreased accordingly. However, with the extension of the culture time, the soil pH gradually increased in all treatments, and finally reached a new stable state. This is consistent with the findings of Chen et al. (2003) and Jiang et al. (2023), which may be due to the degradation of citric acid by microorganisms in the soil and the adsorption and deposition of citric acid by soil particles.
A lower soil pH range is more conducive to the desorption of heavy metals. Among the three additives, citric acid is a low molecular organic acid with stronger acidity, which can effectively reduce the pH and electronegativity of the surface soil and thus can active heavy metals in the soil. However, the activation of heavy metals by citric acid mainly relies on chelation to form relatively stable ring structures rather than a decrease in soil pH only (Wu et al., 2016). Wei (2017) also found that the chelation contribution of citric acid accounted for 72.79% while that of oxalate accounted for only 1.42% when using various rhizosphere organic acids to activate cadmium carbonate, and the ability of citric acid to activate cadmium carbonate was much greater than that of oxalate. Therefore, although the content of DTPA-Cd under citric acid treatment was slightly lower than that under the Gly and fructose treatments on days 3 and 7, the advantage of citric acid application was gradually increased with the increase in the culture time (28 days), and the content of DTPA-Cd in the soil was significantly higher than that of other treatments.
Furthermore, our study also found that higher a concentration of citric acid (SC3) resulted in less Cd activation, than the lower concentration ones (SC1 and SC2). This is because when the concentration of organic acid is lower, the pH and negative charges are significantly reduced, which promotes the desorption of heavy metals. However, with the increase of organic acid concentration, organic acids, Cd and Mn form ternary surface complexes on the soil surface, which promote adsorption and inhibit desorption (Xu et al., 2005). Under the fructose treatment, different dosages of fructose did not significantly reduce the soil pH, but the activation of heavy metals still had a positive effect on the first, third and seventh days. On the one hand, fructose is a reducing sugar with reducing groups (C = O), which mainly changes the speciation and availability of heavy metals through the oxidation-reduction redox rather than the soil pH (Wang et al., 2022). On the other hand, the addition of fructose to soil may affect the activity of soil microorganisms and the production of their metabolites, thus effectively enhancing the activation of heavy metals in the soil. Fructose supplementation promoted carbohydrate metabolism (1.13-fold increase in isomaltose). Although fructose application decreased the alpha diversity of bacterial species in rhizosphere soil by 12.95%, it increased the relative abundance of actinomycetes in rhizosphere soil by 94.60%. Actinomycetes can secrete protons, organic matter and other substances, thus effectively enhancing the activation of heavy metals (Jiang, 2021).
3.2 Effects of combined root exudates on soil pH and DTPA-Cd
The variation in soil pH under the three different combinations is shown in Figure 3. The combination of citric acid + Gly and citric acid + fructose significantly reduced soil pH on the first day, especially the combination of 2 mmol/kg citric acid +2 mmol/kg Gly (SC + G2) and 2 mmol/kg citric acid +2 mmol/kg fructose (SC + F2). However, the combination of Gly + fructose increased the soil pH. With the increase in the culture time, all three combinations increased the soil pH, and there was no significant difference in the pH value compared with SCK.
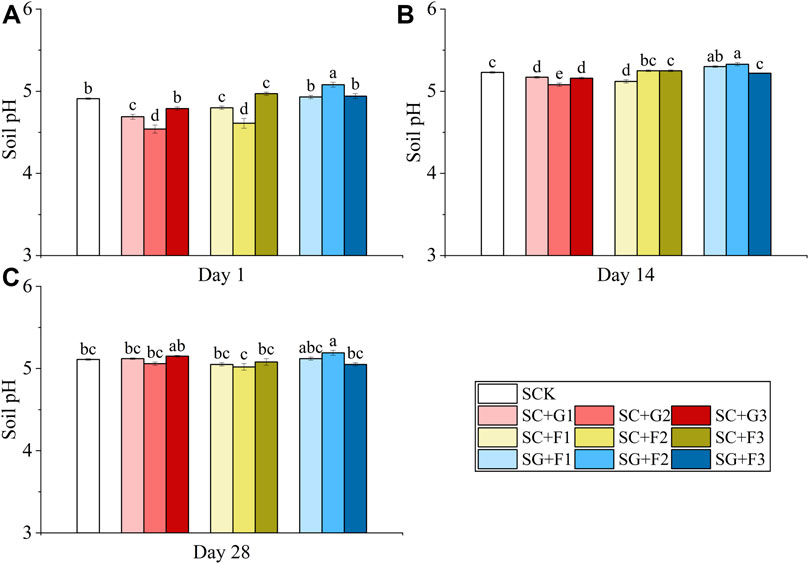
FIGURE 3. Changes in soil pH under the combined treatment of citric acid, Gly and fructose on days 1 (A), 14 (B), and 28 (C). The combination of citric acid + Gly: SC + G1, SC + G2, SC + G3; the combination of citric acid + fructose: SC + F1, SC + F2, SC + F3; the combination of Gly + fructose: SG + F1, SG + F2, SG + F3 (Different letters in each sampling time point show significant differences, p < 0.05).
Figure 4 shows the changes in DTPA-Cd in soil under different dosages of combinations of citric acid, Gly and fructose. Except for SC + G3, all other combinations effectively activated Cd in soil and increased the content of DTPA-Cd during the first 14 days. Among all combinations, the SC + G1 had the best Cd-activation rate, which reached 56.18%. The content of DTPA-Cd in soil under the three combinations showed a downward trend during the experiment.
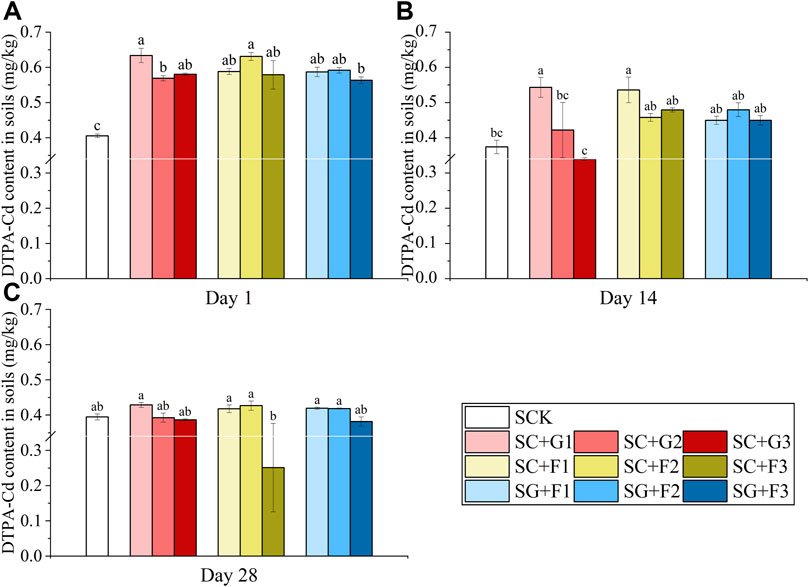
FIGURE 4. Changes in DTPA-Cd in soil under the combined treatment of citric acid, Gly and fructose on days 1 (A), 14 (B), and 28 (C). The combination of citric acid + Gly: SC + G1, SC + G2, SC + G2; the combination of citric acid + fructose: SC + F1, SC + F2, SC + F3; the combination of Gly + fructose: SG + F1, SG + F2, SG + F3 (Different letters in each sampling timepoint show significant differences, p < 0.05).
The synergistic effect of various chelators effectively increased the DTPA-Cd content in the soil in a short time. First, the combination of two chelators increased the solubility of metals by lowering the pH of the soil. Second, in the process of the interaction between metals and different chelators, one metal reacts with one chelator, and its solubility is increased by the addition of another chelator. This is because it can reduce the solubility of another metal in the soil and its competitiveness effects between these matters (Zheng and Zhu, 2009). The significant increase in the DTPA-Cd content on the first day of the experiment was due to a large extent to the added compound solution that effectively reduced the soil pH and reduced the electronegativity of the soil surface, thus promoting the desorption of heavy metals. In the combination experiment, we also found that instead of lowering the soil pH, the combination of Gly + fructose slightly increased it. Non-etheless, the combination of Gly + fructose still significantly increased soil DTPA-Cd in the short term, and the effect was not different from that of the other two combination modes. This indicates that the removal effect of citric acid on heavy metals is related to its acidity to a certain extent, but it mainly depends on the complexation ability of the acid radical, and the activation process can be carried out under mild acidic conditions (Chen, 2011). When SG1 was compared with SC + G2 and SF1 was compared with SC + F2, it was found that the activation efficiency of heavy metals by adding 2 mmol/kg citric acid based on 2 mmol/kg Gly or 2 mmol/kg fructose was further increased by 12.34% and 27.36%, respectively. This may be because the addition of Gly and fructose reduces the competitive cations for citric acid to capture trace metals, such as soil-soluble Ca, thus increasing the solubility of Cd in soil (Tandy et al., 2004; Yuan, 2021).
3.3 Sedum plumbizincicola root exudates enhance the efficiency of phytoremediation in Cd-contaminated soil
3.3.1 Sedum plumbizincicola root exudates enhance the reduction of soil Cd
In the soil culture experiment, single citric acid application and its combination with Gly (SC + G) exhibited the best Cd activation efficiency in the soil. To further verify their effects on phytoremediation, a pot experiment was conducted with S. plumbizincicola. The changes in soil Cd species under single citric acid treatment and the combination of citric acid and Gly are shown in Figure 5. The percentage of the acid soluble Cd fraction in CA1, CA2, and CA3 increased by 5.63%, 8.68%, and 9.14% when compared with CK, which was positively related to the citric acid concentration. Whilst the percentage of the residual fraction decreased in all CA treatments (1.69%–5.71%). Similarly, for the combined application of citric acid and Gly, with the increasing ratio of the citric acid proportion, the percentage of the acid soluble fraction increased, and the residual fraction decreased accordingly when compared with CK. However, when compared with the single application of citric acid at the same dosage (CA3, 4 mmol/kg), only the C + G2 and C + G3 treatments resulted in increased acid soluble fraction and a decreased residual fraction. This indicated that the higher ratio of citric acid proportion in the combined application of citric acid and Gly was helpful in transforming the residual fraction into the acid soluble fraction and reducible fraction in the soil with higher bioavailability.
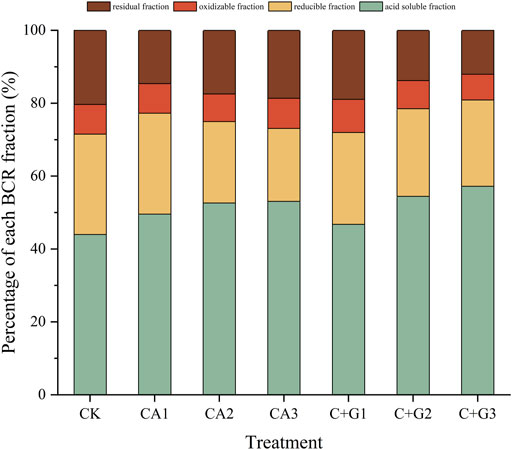
FIGURE 5. Variation of soil Cd species under single citric acid applies and in combination with Gly.
The contents of total Cd and DTPA-Cd in soil were determined after 30 days of incubation in all treatments (Figure 6). For the single citric acid addition (CA), the total amount of Cd in CA1 treated soil was the lowest (0.44 mg/kg), which was 46.16% lower than that in the original soil (0.81 mg/kg). With the increase in the citric acid concentration, the total Cd content in the soil gradually increased. A similar trend was observed for the content of DTPA-Cd with the increasing concentration of citric acid, the concentration of DTPA-Cd in the CA2 and CA3 treatments was significantly higher than that of CK, by 53.85% and 69.23%, respectively. For the combination of citric acid and Gly treatment (C + G), all treatments could significantly reduce the total soil Cd content, among which the C + G2 treatment (2 mmol/kg citric acid +2 mmol/kg Gly) showed the best effect. The total Cd concentration was significantly reduced by 46.11% compared with the original soil (0.81 mg/kg) (Figure 6). Similar to the addition of single citric acid, there was also a positive correlation between soil DTPA-Cd and the citric acid concentration in the C + G combination treatment. These results indicated that citric acid played a dose-dependent facilitation effect on the activation of Cd in the soil, and Gly played a synergistic role in the activation.
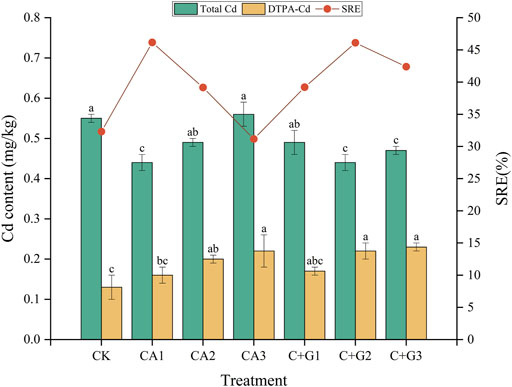
FIGURE 6. Changes in total Cd and DTPA-Cd in soils under different fractions and dosages of single citric acid application and its combination with Gly. The remediation efficiency of Cd was evaluated by calculating the removal rate of Cd in the soil (Different letters indicate significant differences, p < 0.05).
SRE was used to evaluate the remediation efficiency of Cd by calculating the removal rate of Cd in the soil. Except for CA3, all treatments showed a higher SRE than CK, among which CA1 and C + G2 showed the highest remediation efficiency (Figure 6).
Low-molecular-weight organic acids in root exudates, such as citric acid, carboxylic acid, amino acid and phenolic acid, can promote the activation of heavy metals in the soil by reducing the soil pH, changing the soil redox potential and complexing with heavy metals, thus affecting the bioavailability and uptake of heavy metals by plants (Guo, 2017). The H+ released by organic acids can destroy the bonding forms of heavy metals in the soil and, thus promote the conversion of the heavy metals from the reduced state to the acid-soluble state (Jelusic et al., 2014). In this study, a higher concentration of citric acid in both the single and combined treatments induced the conversion of stable Cd (residual Cd) into exchangeable Cd (acid soluble Cd) in the soil, resulting in an increase in the concentration of bioavailable Cd (DTPA-Cd) in the soil. This is in consist with the results reported by Qian and Liu (2012). Perez-Esteban et al. (2013) also found that higher concentrations of citric acid and tartaric acid significantly decreased soil pH, thus promoting the dissolution of iron and manganese oxide bonded copper and zinc in the soil and increasing the desorption of heavy metals in the soil in a mining area.
For the Cd removal efficiency among different treatments, CA1 and C + G2 had the best effect. This likely indicated that although a higher concentration of citric acid may increase the availability of Cd in the soil, the activated Cd could not be taken up and removed by the plants effectively. In addition, Gly may also play an important role in regulating the Cd removal efficiency, especially in an equal proportion with CA. The potential reason for these results will be explained in detail in the next section.
3.3.2 Sedum plumbizincicola root exudates enhance Cd uptake by plants
The biomass and concentration of Cd in S. plumbizincicola were determined after 30 days of incubation under the addition of different doses of single citric acid (CA) and its combination with Gly (C + G) (Figure 7). For the single citric acid addition (CA), the CA1 treatment resulted in the greatest biomass (Figure 7A) and the highest Cd concentration in S. plumbizincicola (Figure 7B), so the phytoremediation efficiency was the highest (42.33%) (Figure 7B). But in the CA3 treatment with the highest concentration of citric acid, the Cd concentration in S. plumbizincicola decreased significantly, which lead to a marked decrease in the phytoremediation efficiency (25.74%) compared with that of CA1. For the combined treatment (C + G), C + G1 had the highest plant biomass (Figure 7A), while C + G2 had the best phytoremediation efficiency (35.61%) (Figure 7B), despite its biomass was lower than that of C + G1. Moreover, C + G3 treatment with the highest concentration of citric acid had the lowest phytoremediation efficiency (29.43%).
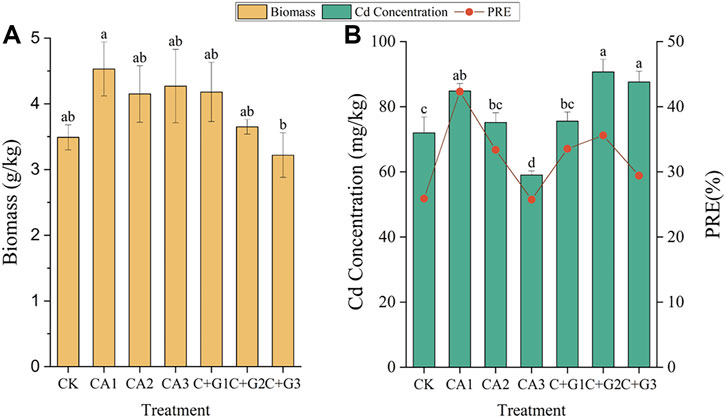
FIGURE 7. Changes in the biomass (A) and Cd concentration in S. plumbizincicola (B) under different fractions and dosages of single citric acid application and its combination with Gly. The remediation efficiency of Cd was evaluated by calculating the absorption rate of Cd in S. plumbizincicola (B) (Different letters indicate significant differences. Biomass: p < 0.1; Cd concentration: p < 0.05).
A low concentration of citric acid significantly increased the biomass of S. plumbizincicola and thus promoted the uptake and transport of heavy metals by the plant via the “biological dilution effect” (Zhang et al., 2015). Hence, the lowest dosage of citric acid used in this study (C1 and C + G1 treatments) resulted in the increased phytoremediation efficiency of Cd by S. plumbizincicola. When the concentration of citric acid increases (CA3 and C + G3), it may cause growth inhibition or even damaged to the plant tissues, thus decreasing the plant biomass and the absorption of Cd by plant roots, and finally affecting the phytoremediation efficiency (Qiao, 2010). In addition to the biological dilution effect, it is possible that Gly also plays a synergetic role in Cd phytoremediation via two pathways. On the one hand, under Cd stress, plants can synthesize and secrete a series of nitrogenous metabolites, such as amino acids, plant chelates, and glutathione, to help plants cope with environmental stress and alleviate the toxic effects (Ma, 2019). On the other hand, exogenous addition of Gly may provide beneficial nutrient elements for the rhizosphere microorganisms, thus regulating the microbial community structure and function and indirectly affecting the phytoremediation efficiency (Wang et al., 2022). Therefore, in the combined application of citric acid and Gly, when citric acid and Gly were in equal proportions (C + G2), both SRE (46.11%) and PRE (35.61%) had the highest efficiency, which verified the importance of glycine in promoting the Cd phytoremediation effect of S. plumbizincicola.
4 Conclusion
In this study, the effects of S. plumbizincicola’s root exudates and their combination patterns on the activation and remediation of Cd-contaminated soils were evaluated. The results showed that, compared with the single application of Gly and fructose, citric acid had the best effect on Cd activation by increasing the DTPA extracted Cd in the soil. Lower concentration of citric acid application resulted in a significantly higher content of DTPA-Cd than the higher one. Furthermore, the combined application of citric acid and Gly, especially at the ratio of 1: 3, had the best activation effect on the Cd in the soil. In addition, the single application of citric acid at lower concentration could significantly reduce the total Cd and DTPA-Cd in the soil and enhance the biomass and the content of Cd in S. plumbizincicola, thus increase the phytoremediation efficiency of Cd-contaminated soil. While for the combined treatment, citric acid and Gly had the best effects of Cd removal and the phytoremediation efficiency. In conclusion, citric acid plays a crucial role in the phytoremediation of Cd by the activation of Cd in the soil, as well as the accumulation of Cd in S. plumbizincicola. Gly was also synergistically involved in relieving the environmental stress on S. plumbizincicola growth and enhancing the uptake of Cd. The detailed mechanisms of the synergetic interaction between citric acid and Gly need further investigation in the future.
Data availability statement
The original contributions presented in the study are included in the article/supplementary material, further inquiries can be directed to the corresponding authors.
Author contributions
QX and LS are responsible for the conduct of the experiment and the writing of the manuscript. XC, YD and JY also contributed partially in designing and conducting of the experiment. CTa and CTu have made contributions to the revision of the logic and language of the manuscript.
Funding
This research was financially supported by the National Natural Science Foundation of China (42107018), the Hunan Innovative Province Construction Special Fund (2020NK 2001), the Natural Science Foundation of Hunan Province (2021JJ40630), and the Independent Project of Institute of Soil Science, Chinese Academy of Sciences (ISSASIP2204).
Conflict of interest
The authors declare that the research was conducted in the absence of any commercial or financial relationships that could be construed as a potential conflict of interest.
Publisher’s note
All claims expressed in this article are solely those of the authors and do not necessarily represent those of their affiliated organizations, or those of the publisher, the editors and the reviewers. Any product that may be evaluated in this article, or claim that may be made by its manufacturer, is not guaranteed or endorsed by the publisher.
References
Bai, J., Tan, C. Y., Cao, X. Y., Zhou, Q., Huang, S. P., Peng, X., et al. (2020). Effect of three organic acids on the remediation efficiency of Sedum plumbizincicola and soil microbial quantity. J. Soil Water Conservation 34, 318–324. doi:10.13870/j.cnki.stbcxb.2020.02.045
Cao, X. Y., Tan, C. Y., Xie, Y., Dai, B., Zhu, S., and Wang, Y. (2019). Effect of soil pH and total cadmium concentration of soil on the remediation efficiency of Sedum plumbizincicola. Res. Environ. Sci. 32, 1604–1612. doi:10.13198/j.issn.1001-6929.2019.05.08
Chen, J. J. (2011). Eeffects of added maize straw and citric acid on Cu and Cd availability in soil. Zhengzhou: Henan Agricultural University.
Chen, Y. X., Lin, Q., Luo, Y. M., He, Y. F., Zhen, S. J., Yu, Y. L., et al. (2003). The role of citric acid on the phytoremediation of heavy metal contaminated soil. Chemosphere 50, 807–811. doi:10.1016/S0045-6535(02)00223-0
Deng, Y. Q., Cao, X., Tan, C., Sun, L., Peng, X., Bai, J., et al. (2020a). Effect of organic materials on phytoremediation efficiency of Cd-contaminated acid soil by Sedum plumbizincicola. J. Agro-Environment Sci. 39, 2762–2770. doi:10.11654/jaes.2020-0605
Deng, Y. Q., Cao, X., Tan, C., Sun, L., Peng, X., Bai, J., et al. (2020b). Strengthening the effect of Bacillus megaterium on remediation of Cd-contaminated farmland soil by Sedum plumbizincicola. Chin. J. Appl. Ecol. 31, 3111–3118. doi:10.13287/j.1001-9332.202009.036
Galili, G., Amir, R., and Fernie, A. R. (2016). The regulation of essential amino acid synthesis and accumulation in plants. Annu. Rev. Plant Biol. 67, 153–178. doi:10.1146/annurev-arplant-043015-112213
Guo, S. H. (2017). Effect of salt stress on soil Cd mobility and Cd uptake by edible amaranth (Amaranthus mangostanus L.) cultivar. Guangzhou: Jinan University.
Hammer, D., and Keller, C. (2002). Changes in the rhizosphere of metal-accumulating plants evidenced by chemical extractants. J. Environ. Qual. 31, 1561–1569. doi:10.2134/jeq2002.1561
He, M. F., Li, Z. B., Chen, C., and Mei, P. (2022). Activation and remediation of Cu2+ and Cd2+ in soil by root exudates and plants. Environ. Sci. Technol. 45, 198–205. doi:10.19672/j.cnki.1003-6504.0039.22.338
Jelusic, M., Vodnik, D., and Lestan, D. (2014). Revitalization of EDTA-remediated soil by fertilization and soil amendments. Ecol. Eng. 73, 429–438. doi:10.1016/j.ecoleng.2014.09.068
Jiang, O. Y., Li, L. Y., Duan, G. L., Gustave, W., Zhai, W. W., Zou, L. N., et al. (2023). Root exudates increased arsenic mobility and altered microbial community in paddy soils. J. Environ. Sci. 127, 410–420. doi:10.1016/j.jes.2022.05.036
Jiang, Y. Y. (2021). Effect of cadmium stress on symbiont combined with AM fungi and medicago truncatula and the effect of additional fructose. Chongqing: Southwest University of Science and Technology. doi:10.27415/d.cnki.gxngc.2021.000933
Liu, L. L., Tan, C. Y., Cao, X. Y., Peng, X., Bai, J., Cheng, X. Q., et al. (2022). Remediation effects of citric acid activation combined with Sedum plumbizincicola intercropped with maize on slightly Cd-contaminated soil. Trans. Chin. Soc. Agric. Eng. 38, 257–263. doi:10.1016/j.jes.2022.05.036
Liu, S. H., Chen, H. Y., Ji, X. H., Liu, S. B., and Xie, Y. H. (2021). Remediation potential of rice with high cadmium accumulation to cadmium contaminated farmland. Trans. Chin. Soc. Agric. Eng. Trans. CSAE) 37, 175–181. doi:10.11975/j.issn.1002-6819.2021.10.021
Ma, Q. X. (2019). The uptake of amino acids and the effects of pH and Cd stress on plants absorption. Hangzhou: Zhejiang University.
Perez-Esteban, J., Escolastico, C., Moliner, A., and Masaguer, A. (2013). Chemical speciation and mobilization of copper and zinc in naturally contaminated mine soils with citric and tartaric acids. Chemosphere 90, 276–283. doi:10.1016/j.chemosphere.2012.06.065
Qian, L., and Liu, Y. (2012). Effects of single and combined organic acids on form of Cd in soil. Chin. J. Soil Sci. 43, 186–189. doi:10.19336/j.cnki.trtb.2012.01.036
Qiao, D. M. (2010). Phytoremediation mechanism of lead pollution base on organic acid exudation from ryegrass root. Beijing: Chinese Academy of Agricultural Sciences.
Shen, L. B., Wu, L. H., Han, X. R., Tan, W. N., Huang, Y. J., Luo, Y. M., et al. (2011). Effects of nutrient regulation and control on plant growth and Zn/Cd uptack by hyperaccmulator Sedum plumbizincicola. Soils 43, 221–225. doi:10.13758/j.cnki.tr.2011.02.009
Sun, L. J., Cao, X. Y., Tan, C. Y., Deng, Y. Q., Cai, R. Z., Peng, X., et al. (2020). Analysis of the effect of cadmium stress on root exudates of Sedum plumbizincicola based on metabolomics. Ecotoxicol. Environ. Saf. 205, 111152. doi:10.1016/j.ecoenv.2020.111152
Sun, L. J. (2021). Effects of Sedum plumbizincicola root exudates and its components in Cd-contaminated soil remediation. Changsha: Hunan Normal University. doi:10.27137/d.cnki.ghusu.2021.002504
Tandy, S., Bossart, K., Mueller, R., Ritschel, J., Hauser, L., Schulin, R., et al. (2004). Extraction of heavy metals from soils using biodegradable chelating agents. Environ. Sci. Technol. 38, 937–944. doi:10.1021/es0348750
Tang, D. Y., Xiang, Q., Lei, W. D., and Sun, J. (2022). Effects of organic acids on the desorption of Cd, Cr and Mn ions in heavy metal contaminated soil. J. South⁃Central Univ. Natl. Nat. Sci. Ed. 41, 44–50. doi:10.12130/znmdzk.20220108
Wang, Y., Feng, F. Y., Ge, J., Li, Y., and Yu, X. Y. (2022). Effects and mechanisms of plant root exudates on soil remediation. Acta Ecol. Sin. 42, 829–842. doi:10.5846/stxb202101030011
Wei, J. (2017). A comparative study on insoluble cadmium mobilized by various rhizosphere organic acids. Guangzhou: Jinan University.
Wu, J., Lu, J., Zhang, C., Zhang, Y. X., Lin, Y. C., and Xu, J. (2020). Pollution, sources, and risks of heavy metals in coastal waters of China. Hum. Ecol. Risk Assess. Int. J. 26, 2011–2026. doi:10.1080/10807039.2019.1634466
Wu, L. H., Zhou, S. B., Bi, D., Guo, X. H., Qin, W. H., Wang, H., et al. (2006). Sedum plumbizincicola, A new species of the crassulaceae from zhejiang, China. Soils. Thesis. Beijing 38, 632–633. doi:10.13758/j.cnki.tr.2006.05.022
Wu, L. S., Xian, S. Y., Kong, D., C Ou, M. Y., and Deng, Q. G. (2016). Remediation of Cd polluted soil by Co-leaching of tannic acid and citric acid. Environ. Eng. 34, 178–181+165. doi:10.13205/j.hjgc.201608037
Xu, R. K., Xiao, S. C., and Ji, G. L. (2005). Mechanisms of low molecular weight organic acids affecting Cu adsorption by variable charge soils. China Environ. Sci. 25, 334–338.
Xue, W., Zhang, C., Huang, Y., Wang, C., Zhang, X., and Liu, Z. (2022). Rice organs concentrate cadmium by chelation of amino acids containing dicarboxyl groups and enhance risks to human and environmental health in Cd-contaminated areas. J. Hazard Mater 426, 128130. doi:10.1016/j.jhazmat.2021.128130
Yuan, K. (2021). Effects of amino acids and calcium magnesium phosphate fertilizer on characteristic of cadmium accumulation in rice and their mechanism thesis. Beijing: Chinese Academy of Agricultural Sciences.
Zhang, X., Chen, B. D., and Ohtomo, R. (2015). Mycorrhizal effects on growth, P uptake and Cd tolerance of the host plant vary among different AM fungal species. Soil Sci. Plant Nutr. 61, 359–368. doi:10.1080/00380768.2014.985578
Zheng, X. L., and Zhu, K. (2009). The application of chelating agents in the phytoremediation of heavy mental contaminated soils. Environ. Sci. Manag. 34, 106–109. doi:10.13292/j.1000-4890.2009.0317
Keywords: Sedum plumbizincicola, root exudates, citric acid, glycine, fructose
Citation: Xing Q, Cao X, Tan C, Sun L, Deng Y, Yang J and Tu C (2023) Effects of single and combined applications of three root exudates of Sedum plumbizincicola on the phytoremediation efficiency of paddy soil contaminated with Cd. Front. Environ. Sci. 10:1086753. doi: 10.3389/fenvs.2022.1086753
Received: 01 November 2022; Accepted: 12 December 2022;
Published: 04 January 2023.
Edited by:
Jun Wu, Harbin Engineering University, ChinaReviewed by:
Xiaobing Wang, Yangzhou University, ChinaGuoyong Huang, Huazhong Agricultural University, China
Huidan Jiang, Hunan Academy of Agricultural Sciences (CAAS), China
Copyright © 2023 Xing, Cao, Tan, Sun, Deng, Yang and Tu. This is an open-access article distributed under the terms of the Creative Commons Attribution License (CC BY). The use, distribution or reproduction in other forums is permitted, provided the original author(s) and the copyright owner(s) are credited and that the original publication in this journal is cited, in accordance with accepted academic practice. No use, distribution or reproduction is permitted which does not comply with these terms.
*Correspondence: Changyin Tan, chytan@hunnu.edu.cn; Chen Tu, ctu@issas.ac.cn