- 1CMA Earth System Modeling and Prediction Centre, Beijing, China
- 2State Key Laboratory of Severe Weather, Chinese Academy of Meteorological Sciences, Beijing, China
- 3Laboratory for Climate and Ocean-Atmosphere Studies, Department of Atmospheric and Oceanic Sciences, School of Physics, Peking University, Beijing, China
Low-level jets (LLJ) are a common phenomenon in the atmospheric boundary layer and have been reported worldwide. Additionally, they have considerable relevance in a series of fields. This review aimed to document their implications on air quality, specifically particulate matter, mineral dust, and ozone in recent literature focus on i) generalizing long-range advection of pollutants by the low-level jets; ii) analysing vertical transport due to low-level jets-enhanced turbulence mixing and the corresponding mechanisms for different pollutants; and iii) introducing the performance of state-of-the-art numerical models. Finally, we suggest that high-resolution spatiotemporal observations of the pollutants and turbulence must be conducted, and current parameterization schemes should be improved to better represent the low-level jets and nocturnal boundary layer structures for reproducing the complicated interactions between the low-level jets and pollutants.
1 Introduction
Low-level jets (LLJs) are a common phenomenon in the atmospheric boundary layer (ABL) and have been widely observed worldwide, including in North America (Smith et al., 2019), South America (Sánchez et al., 2022), East Asia (Wei et al., 2014; Miao et al., 2018), Europe (Tuononen et al., 2017), and Africa (King et al., 2021). However, there is no universal consensus on the definition of the LLJs (Fiedler et al., 2013). To summarize the definitions in previous literatures, the term LLJ used here refers to a stream of fast-moving flow with maximum wind speed and vertical wind shear in the lower part of the troposphere (Stull, 1988).
The occurrence and variation of the LLJs are modulated by a combination of mechanisms on different temporal and spatial scales. Blackadar’s (1957) pioneering work proposed that inertial oscillations of ageostrophic components contribute to the diurnal cycle of the LLJs. The “Blocking Theory” (Wexler, 1961) attributed the speeding-up of flows to the blockage of terrains, such as the Rocky Mountains, an analogy to the Gulf Stream in oceanography. The thermal—wind relationship leads to coastal jets because of the differential heating and cooling between the land and oceans (Lima et al., 2019). Sloping terrains and the associated horizontal temperature gradients are other ways for providing baroclinicity that the LLJs need to develop (Gebauer and Shapiro, 2019).
The LLJs are primarily a process in the ABL and are highly relevant in many fields, such as wind energy (Wimhurst and Greene, 2019), precipitation (Du et al., 2012), fog (Wu et al., 2020), urban heat islands (Hu et al., 2013a), wildfires (Čavlina Tomašević et al., 2022), aviation safety (Gultepe et al., 2019), and migration pathways of birds (Wainwright et al., 2016). This review aimed to examine how the LLJs impact pollutant transport locally and inter-regionally, considering mineral dust, fine particulate matter (PM2.5), and ozone (O3). Specifically, this paper focuses on the following:
Present the current state of knowledge of the LLJ—pollutant interaction mechanism.
Document the state-of-the-art methods to analyse the LLJs, including numerical models and signal analysis techniques.
Outline the remaining gaps between the current progress and future research.
2 Regional transport
The belt of high wind speed, one of the dominant features of the LLJs, is a crucial process in the long-range transport of the pollutants. The contribution of LLJ advection to a certain region depends on the season, wind direction, and location of pollutant sources. In the Sahara Desert, dust is primarily advected below 800 hPa to South America in winter and spring and to the Caribbean at 500 hPa in summer and autumn (Gläser et al., 2015). In the southwestern China, up to 80% of the summertime O3 accumulation is because of horizontal transport (Yang et al., 2020). The LLJs are crucial to the redistribution of O3 between source locations and downwind regions (Bao et al., 2008; Klein et al., 2019). Li et al. (2019) revealed that southerly LLJs could transport large amounts of PM2.5 from upstream regions, leading to accumulation of the pollutants in the downstream zones. In contrast, the occurrence of the LLJs positively contributes to the dilution of pollutants in Beijing (Miao et al., 2019). In Tianjin, China, southwesterly LLJs transport polluted air masses from the southern industrial regions, deteriorating local air quality, whereas the northerly or southeasterly LLJs are helpful in improving visibility (Wu et al., 2020). In general, the existence of the LLJs favors the dilution of pollutants, whereas downwind regions suffer from pollutant advection by the LLJs.
In addition, the transport of precursors and moisture by the LLJs facilitates the formation of pollution periods. In the North China Plain, southwesterly LLJs establish a moisture channel from the South China Sea to the Bohai Rim Region, contributing to the frequent PM2.5 events in winter (Ju et al., 2020).
3 Vertical transport of mineral dust
Although the long-range transport of chemical species by the LLJs between different regions has been studied previously, the vertical flux associated with them has not been intensely investigated (Figure 1). Recent studies have revealed that vertical dispersion induced by the LLJs may play a crucial role in the local air quality.
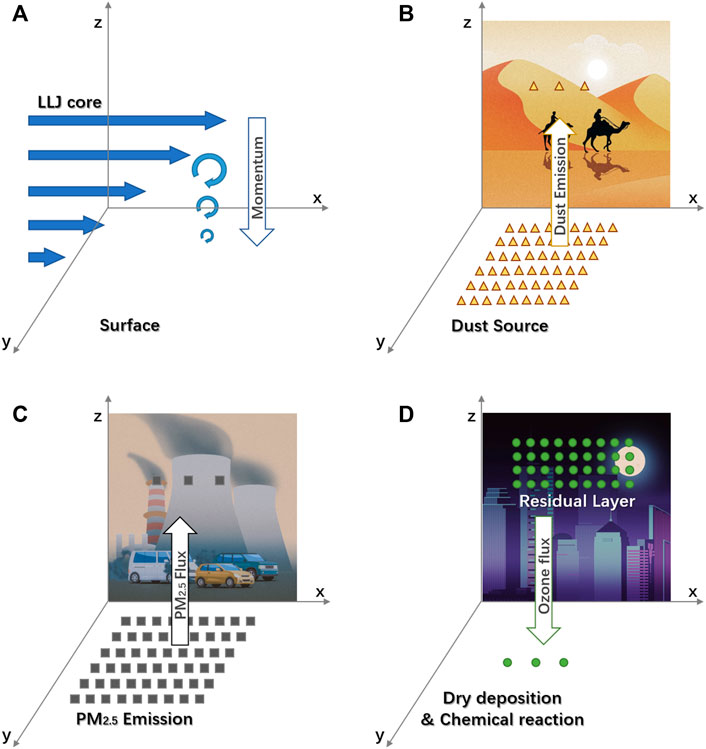
FIGURE 1. Schematic of vertical interaction between LLJs and pollutants with transport of (A) momentum, (B) dust, (C) fine particulate matter, and (D) ozone.
The downward momentum flux caused by the LLJs is recognized as one of the key mechanisms of dust emissions in the Sahara Desert, in addition to monsoon surges (Cuesta et al., 2010), cold pool outflows (Allen et al., 2013), and dry convective plumes (Ansmann et al., 2009). The LLJs develop in the ABL after sunset because of the inertial oscillations (Blackadar, 1957). The wind shear near the jet core creates turbulence in the upper layer and it is transported downward, generating peak winds near the surface. There is a time lag of several hours between the maximal wind speed of the LLJs and that at the surface layer, with the former occurring before sunrise and the latter occurring mid-morning. Dust is emitted once the surface wind exceeds the local dust emission threshold (Allen and Washington, 2014). Observations and model simulations (Todd et al., 2008) have confirmed that LLJ-induced downward momentum initiates mid-morning dust events. Approximately 60% of the total dust can be attributed to the peak wind caused by nocturnal LLJs (Fiedler et al., 2013). The LLJs are a common phenomenon in the Sahara Region and an average of 29% at night was observed (Fiedler et al., 2013). Frequently occurring LLJs are key drivers of the local dust emissions. According to satellite observations from 2006 to 2008 (Schepanski et al., 2009), the surface peak wind associated with the nocturnal LLJs accounted for 65% of dust source activations during the mid-morning across North Africa, which is larger than the values at midday. Once uplifted, the dust is horizontally transported by the LLJs to America, Europe, and beyond.
4 Vertical transport of PM2.5
Due to the consumption of fossil fuels and the stable atmosphere, PM2.5 pollution tends to occur frequently during winter, especially in densely populated metropolises. One feature of PM2.5 pollutions in winter is persistent and it threatens public health (Dominici et al., 2014). Persistent heavy pollution events are divided into two stages (Zhong et al., 2017; Wei et al., 2018; Zhong et al., 2018; Ren et al., 2019a): the cumulative stage during which particles accumulate is due to the high emissions, adverse synoptic weather conditions, regional transport, and secondary pollutant production and the dissipation stage is characterized by an abrupt decline in PM2.5 levels. Numerous studies (Wei et al., 2018; Ren et al., 2019a; Ren et al., 2019c; Li et al., 2020; Wei et al., 2020; Li et al., 2021) have revealed that the LLJs play a key role in the dissipation stage of PM2.5.
Inversion layer frequently develops in winter and traps PM2.5 emitted from the ground within a shallow layer. According to the “barrier effect” theory (Ren et al., 2021), turbulence disappears at some heights and laminar flow develops during heavily polluted periods, which serves as a barrier layer impeding vertical turbulence transport. In the presence of the LLJs, turbulence bursts produced by aloft wind shear enhance vertical mixing, break the decoupled ABL structure, and rebuild the heat/momentum/material exchange between different heights. Field observations (Li et al., 2020) confirmed that the LLJs frequently occurred during the transition between the two stages. Miao et al. (2018) investigated the climatology of the LLJs in the Beijing Region and discovered that they frequently occurred in spring and winter and were mostly concentrated during nighttime. The consistency between the LLJs and severe PM2.5 events implies that they play a crucial role in PM2.5 pollution, which has been confirmed in a wide range of areas in China.
The LLJs can affect the diffusion of PM2.5 indirectly via propagating internal gravity waves (IGWs), in addition to their direct impact on pollutant transport. The IGWs are a type of wavelike motion in the stable boundary layer (SBL) triggered by the LLJs (Jia et al., 2019). One puzzling phenomenon of heavy pollution is that PM2.5 concentrations at different levels experience non-simultaneous drops or inverse variations (Ren et al., 2020). In the presence of the LLJs, the IGWs are generated and propagated downward, intermittently triggering turbulence layer by layer (Wei et al., 2022). The vertical spreading of the IGWs is responsible for short-term PM2.5 removals during the cumulative stage and asynchronous variation at different heights.
5 Vertical transport of tropospheric O3
As a volatile secondary photochemical pollutant, O3 concentration at the surface undergoes a marked diurnal variation: i) High emission of precursors and photodissociation leads to high O3 concentration during the daytime. ii) After sunset, the photochemical production shuts down and O3 is removed via dry deposition and chemical reactions within the surface layer. Meanwhile the aloft residual layer traps O3. iii) The following morning, strong turbulence develops and fully mixes the aloft O3. In this scenario, nocturnal O3 concentration at the surface should be near zero owing to the absence of production, removal processes, and a decoupled SBL. However, nighttime O3 spikes have been frequently reported in different regions since the 1970s (Samson, 1978; Reitebuch et al., 2000).
At night, the surface layer decouples with the aloft residual layer, thus making the residual layer a “reservoir” of daytime pollutants. In the presence of the LLJs, the strong wind shear near the jet core generates turbulence bursts, enhancing the vertical mixing between the two layers. Thus, the O3 restored in the residual layer leaks into the surface layer. Studies (Reitebuch et al., 2000; Hu et al., 2012; Hu et al., 2013b) have verified that the concentration of O3 in the residual layer, accompanied by O3 spikes near the surface, is highly variable, implying active vertical exchanges. This aloft turbulence source associated with the LLJs depicts a reversed vertical structure from the traditional SBL, where most of the turbulence is produced by surface friction and transported upward. The turbulence induced by the LLJs is highly sporadic and is marked by intermittent bursts which remains an intriguing problem. Apart from the LLJs, other mechanisms causing intermittent turbulence include Kelvin–Helmholtz instabilities, gravity waves, wake vortices, and density currents (Wei et al., 2017). Considering that favorable synoptical patterns for the LLJs are also beneficial to heavy O3 periods in some regions (Ryan, 2004), the importance of the LLJs in O3 pollution cannot be ignored.
The maxima O3 concentrations at nighttime may not be comparable to those in the afternoon. However, relevant research still has implications: i) The LLJs are important contributors to the horizontal transport. Considering that the vertical O3 flux leads to a leaky residual-layer reservoir, the impact of pollutants carried by the LLJs downwind may be relieved. ii) O3 dispersed to the surface is permanently removed via dry deposition at night. As photochemical production does not occur, the net effect is that the total amount of O3 is reduced, further easing the next-morning O3 levels. iii) In persistent O3 periods, the amount of O3 removed by nighttime vertical mixing is crucial for the total O3 budget over several days (Banta et al., 2007). iv) Nighttime spikes have been reported in different chemical species, including hydrogen peroxide (Das and Aneja, 1994), isoprene (Starn et al., 1998), volatile organic compounds (VOCs) (Ganzeveld et al., 2008), carbon dioxide (CO2) (Hicks et al., 2015), and PM2.5 (Wei et al., 2018). Therefore, the studies on the LLJ–O3 mechanism also have implications for other pollutants.
6 Models and analyses methods
Model simulation has become a useful tool for investigating the LLJs and their interactions with air pollutions. Based on the inertial oscillation theory, numerical models can reproduce the diurnal variation in the LLJs (van de Wiel et al., 2010). However, the underestimation of the maximal wind speed and deviations of profiles by the numerical models have been widely reported (Smith et al., 2018; Haikin and Castelli, 2022). The simulation bias of the maximal wind strength and profiles could further affect the wind shear and turbulence mixing. The simulation of the nocturnal LLJ is highly sensitive to the vertical resolution, planetary boundary layer (PBL) schemes and surface-layer parameterizations. Refining vertical spacing is helpful to improve the LLJs while the computational cost and model stability should be considered.
The simulation bias in the diurnal variation of PM2.5 levels is largely due to turbulence mixing during nighttime. Considering that the LLJs and serious PM2.5 pollution tend to occur in stabilized atmosphere, local closure PBL schemes are suitable for the simulation of the LLJs and PM2.5 events (Jia and Zhang, 2020). Some literatures (Udina et al., 2020; Yang et al., 2021) have confirmed that the Mellor—Yamada—Janjic scheme (Janjic, 2002) shows better performance compared with other schemes, while it still cannot reproduce the different stages of haze events. The turbulence mixing coefficient is one of the key parameters to control the PBL mixing. Du et al. (2020) revealed that increasing the threshold of turbulence mixing coefficient was helpful to the simulation of diurnal variation of PM2.5. Jia et al. (2021) developed a turbulence diffusion term for aerosols and embedded it into a mesoscale model for the first time.
The high wind speed of the LLJs is crucial to achieving long-range transport of dust. The underestimation of the jet core speed implies a weakened region-to-region transport simulation. In addition, parameterization of the mineral dust budget depends on the simulation of the downward momentum flux (Pérez et al., 2011; Knippertz and Todd, 2012). However, a weakened LLJ strength can lead to an underestimation of the dust emissions, as the surface wind speed is not strong enough to reach the dust emission threshold. Besides, the overestimation of LLJ strength and vertical mixing at night alters the diurnal variation of the wind field in the ABL (Sandu et al., 2013).
The tropospheric O3 concentration during the daytime can be simulated in accordance with the field observations, although distinct differences exist at night. The primary reason for this is the representation of the nighttime turbulence transport by the LLJs. Although the horizontal structure of the LLJs can be reasonably simulated, the results of the simulated LLJ vertical profiles are far from satisfactory. Sensitivity simulations (Hu et al., 2012) elucidated that longer turbulence lengths and long-tail stability functions are more suitable for O3 and LLJs modelling, implying that turbulence mixing during nighttime requires careful treatment.
Turbulence intermittency has still not been effectively considered in the numerical models, although field observations have confirmed its importance in the transport of O3 (Salmond and McKendry, 2002; Salmond and McKendry, 2005), PM2.5 (Wei et al., 2018; Ren et al., 2019b), and CO2 (ElMadany et al., 2014; Hicks et al., 2015). The classical Monin—Obukhov similarity theory (MOST, Monin and Obukhov, 1954), which has been widely adopted in atmospheric and environmental models, is only suitable for unstable ABL or traditional surface-forced SBL. Large uncertainties may exist when applying the MOST-based models to intermittent turbulence. Furthermore, no overarching agreement has yet been reached an appropriate method to describe the turbulence intermittency in the ABL. The traditional Fourier transform cannot be applied to the analysis of non-linear and non-stationary turbulence bursts. The wavelet analysis was used to separate the LLJ-induced intermittent turbulence during O3 periods (Salmond, 2004). Based on the self-adaptive Hilbert—Huang transform (Huang et al., 1998), several indexes have been proposed to quantify the strength of the turbulence intermittency (Wei et al., 2018; Ren et al., 2019b; Ren et al., 2019c).
7 Summary and discussion
This review summarized the current state of interactions between the LLJs and pollutants from the perspective of observations, models, and mechanisms. With the literature of the three most-studied species (i.e., mineral dust, PM2.5, and O3), the effects of the LLJs can be divided into long-range horizontal transport and local vertical dispersion. Recent works have been listed in Table 1. The fast-moving stream of the LLJs is an important pathway for the region-to-region transport of moisture, precursors, and pollutants. Whether the LLJ is a boon or bane for the local air quality depends on the season, wind direction, and location of the pollutant sources. Recently, vertical mixing induced by the LLJs has been under the spotlight for explaining the local variation of pollutant levels. The downward transport of intermittent turbulence resulting from the LLJs is crucial for re-coupling nocturnal SBLs, enhancing vertical mixing, transporting pollutants downward/upward, and worsening/improving the air quality. What the three species have in common is that the downward momentum due to LLJs strengthens the turbulence mixing and pollutants transport. Specifically, O3 dissipates downward from the aloft “reservoir”, leading to O3 peaks at night. The mineral dust was uplifted from the underground the next morning. The PM2.5 is mostly transported upward and diluted over a deeper layer. The intermittency of LLJ-induced turbulence perplexes the mechanism of the vertical dissipation of pollutants.
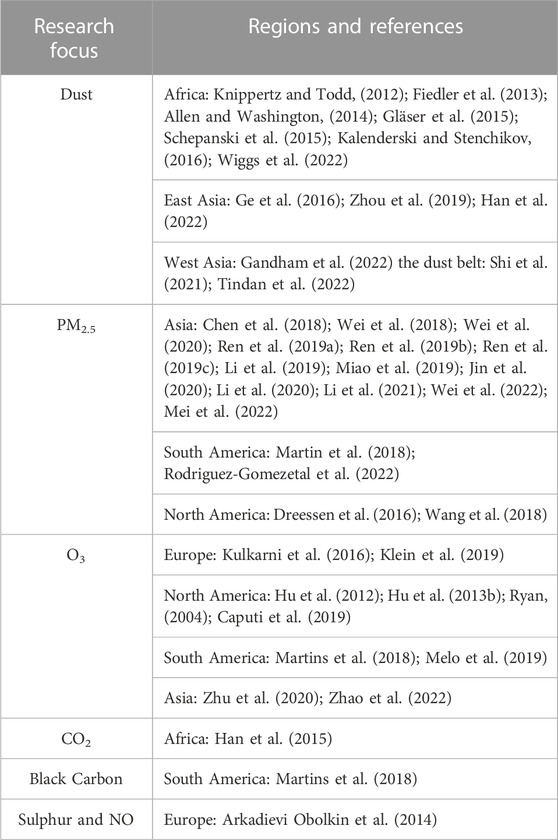
TABLE 1. Summary of main studies (mostly after 2012) on the LLJs and chemical species transport in different regions.
In the future, high-resolution turbulence and pollutant observational data is needed to investigate the complicated vertical LLJ—pollutant interaction and establish the turbulence diffusion relationship of pollutants. Most current work is based on surface observations of pollutants and turbulence. However, to study the fine structure of the SBL, continuous profiles are required. A meteorological tower can provide multilevel observations, however, its spatial resolution is far from satisfactory. Radiosonde data suffers from a low time resolution. Carrying out intensive observation period experiments can be a good way for addressing these problems. Remote sensing technologies, such as Lidar are helpful methods to obtain continuous observation. Furthermore, the current findings were mostly obtained from one site. There is a large scope for further research on the spatial distribution of intermittent turbulence and pollutants based on multi-site observation.
The numerical models perform well in the simulation of the horizontal structure of the LLJs, while the vertical distribution remains unsatisfactory. PBL schemes need to be further improved for better representing nighttime processes. Accurate simulation of the LLJ profiles and development is crucial for nocturnal turbulence mixing. In addition, present models do not consider the turbulence intermittency, and most studies on the LLJ-burst interplay focus on O3 and PM2.5. How this mechanism impacts the variation of other chemical species, such as nitrogen oxides (NO), VOCs, and carbon monoxide, remains to be assessed.
Author contributions
All authors listed have made a substantial, direct, and intellectual contribution to the work and approved it for publication.
Funding
This work is supported by the National Natural Science Foundation of China (42090031, 92044301, 91544216, 41705003) and Natural Science Foundation of Liaoning Province (No.2020-MS-350).
Conflict of interest
The authors declare that the research was conducted in the absence of any commercial or financial relationships that could be construed as a potential conflict of interest.
Publisher’s note
All claims expressed in this article are solely those of the authors and do not necessarily represent those of their affiliated organizations, or those of the publisher, the editors and the reviewers. Any product that may be evaluated in this article, or claim that may be made by its manufacturer, is not guaranteed or endorsed by the publisher.
References
Allen, C. J. T., Washington, R., and Engelstaedter, S. (2013). Dust emission and transport mechanisms in the central Sahara: Fennec groundbased observations from bordj badji mokhtar, june 2011. J. Geophys. Res. Atmos. 118, 6212–6232. doi:10.1002/jgrd.50534
Allen, C. J., and Washington, R. (2014). The low-level jet dust emission mechanism in the central Sahara: Observations from bordj-badji mokhtar during the june 2011 fennec intensive observation period. J. Geophys. Res. Atmos. 119 (6), 2990–3015. doi:10.1002/2013jd020594
Ansmann, A., Tesche, M., Knippertz, P., Bierwirth, E., Althausen, D., Müller, D., et al. (2009). Vertical profiling of convective dust plumes in southern Morocco during SAMUM. Tellus B 61 (1), 340–353. doi:10.3402/tellusb.v61i1.16833
Arkadievich Obolkin, V., Lvovich Potemkin, V., Leonidovich Makukhin, V., Vladimirovna Chipanina, Y., and Iozovna Marinayte, I. (2014). Low-level atmospheric jets as main mechanism of long-range transport of power plant plumes in the Lake Baikal Region. Int. J. Environ. Stud. 71 (3), 391–397. doi:10.1080/00207233.2014.918396
Banta, R. M., Mahrt, L., Vickers, D., Sun, J., Balsley, B. B., Pichugina, Y. L., et al. (2007). The very stable boundary layer on nights with weak low-level jets. J. Atmos. Sci. 64 (9), 3068–3090. doi:10.1175/jas4002.1
Bao, J. W., Michelson, S. A., Persson, P. O. G., Djalalova, I. V., and Wilczak, J. M. (2008). Observed and WRF-simulated low-level winds in a high-ozone episode during the Central California Ozone Study. J. Appl. Meteorology Climatol. 47 (9), 2372–2394. doi:10.1175/2008JAMC1822.1
Blackadar, A. K. (1957). Boundary layer wind maxima and their significance for the growth of nocturnal inversions. Bull. Am. Meteorological Soc. 38, 283–290. doi:10.1175/1520-0477-38.5.283
Caputi, D. J., Faloona, I., Trousdell, J., Smoot, J., Falk, N., and Conley, S. (2019). Residual layer ozone, mixing, and the nocturnal jet in California's San Joaquin Valley. Atmos. Chem. Phys. 19 (7), 4721–4740. doi:10.5194/acp-19-4721-2019
Čavlina Tomašević, I., Cheung, K. K., Vučetić, V., Fox-Hughes, P., Horvath, K., Telišman Prtenjak, M., et al. (2022). The 2017 Split wildfire in Croatia: Evolution and the role of meteorological conditions. Nat. Hazards Earth Syst. Sci. Discuss. 22, 3143–3165. doi:10.5194/nhess-22-3143-2022
Chen, Y., An, J., Sun, Y., Wang, X., Qu, Y., Zhang, J., et al. (2018). Nocturnal low-level winds and their impacts on particulate matter over the Beijing area. Adv. Atmos. Sci. 35 (12), 1455–1468. doi:10.1007/s00376-018-8022-9
Cuesta, J., Lavaysse, C., Flamant, C., Mimouni, M., and Knippertz, P. (2010). Northward bursts of the west african monsoon leading to rainfall over the hoggar massif, Algeria. Q. J. R. Meteorol. Soc. 136, 174–189. doi:10.1002/qj.439
Das, M., and Aneja, V. P. (1994). Measurements and analysis of concentrations of gaseous hydrogen peroxide and related species in the rural central piedmont region of North Carolina. Atmos. Environ. 28, 2473–2483. doi:10.1016/1352-2310(94)90398-0
Dominici, F., Greenstone, M., and Sunstein, C. R. (2014). Particulate matter matters. Science 344 (6181), 257–259. doi:10.1126/science.1247348
Dreessen, J., Sullivan, J., and Delgado, R. (2016). Observations and impacts of transported Canadian wildfire smoke on ozone and aerosol air quality in the Maryland region on June 9–12, 2015. J. Air & Waste Manag. Assoc. 66 (9), 842–862. doi:10.1080/10962247.2016.1161674
Du, Q., Zhao, C., Zhang, M., Dong, X., Chen, Y., Liu, Z., et al. (2020). Modeling diurnal variation of surface PM<sub>2.5</sub> concentrations over East China with WRF-chem: Impacts from boundary-layer mixing and anthropogenic emission. Atmos. Chem. Phys. 20, 2839–2863. doi:10.5194/acp-20-2839-2020
Du, Y., Zhang, Q., Ying, Y., and Yang, Y. (2012). Characteristics of low-level jets in shanghai during the 2008–2009 warm seasons as inferred from wind profiler radar data. J. Meteorological Soc. Jpn. Ser. II 90 (6), 891–903. doi:10.2151/jmsj.2012-603
Fiedler, S., Schepanski, K., Heinold, B., Knippertz, P., and Tegen, I. (2013). Climatology of nocturnal low-level jets over North Africa and implications for modeling mineral dust emission. J. Geophys. Res. Atmos. 118 (12), 6100–6121. doi:10.1002/jgrd.50394
Gandham, H., Dasari, H. P., Karumuri, A., Ravuri, P. M. K., and Hoteit, I. (2022). Three-dimensional structure and transport pathways of dust aerosols over West Asia. npj Clim. Atmos. Sci. 5 (1), 45–15. doi:10.1038/s41612-022-00266-2
Ganzeveld, L., Eerdekens, G., Feig, G., Fischer, H., Harder, H., Konigstedt, R., et al. (2008). Surface and boundary layer exchanges of volatile organic compounds, nitrogen oxides and ozone during the GABRIEL campaign. Atmos. Chem. Phys. 8, 6223–6243. doi:10.5194/acp-8-6223-2008
Ge, J. M., Liu, H., Huang, J., and Fu, Q. (2016). Taklimakan Desert nocturnal low-level jet: Climatology and dust activity. Atmos. Chem. Phys. 16 (12), 7773–7783. doi:10.5194/acp-16-7773-2016
Gebauer, J. G., and Shapiro, A. (2019). Clarifying the baroclinic contribution to the Great Plains low-level jet frequency maximum. Mon. Weather Rev. 147 (9), 3481–3493. doi:10.1175/mwr-d-19-0024.1
Gläser, G., Wernli, H., Kerkweg, A., and Teubler, F. (2015). The transatlantic dust transport from north Africa to the americas—its characteristics and source regions. J. Geophys. Res. Atmos. 120 (21), 11231–11252. doi:10.1002/2015jd023792
Gultepe, I., Sharman, R., Williams, P. D., Zhou, B., Ellrod, G., Minnis, P., et al. (2019). A review of high impact weather for aviation meteorology. Pure Appl. Geophys. 176 (5), 1869–1921. doi:10.1007/s00024-019-02168-6
Haikin, N., and Castelli, S. T. (2022). On the effect of a low-level jet on atmospheric pollutant dispersion: A case study over a coastal complex domain, employing high-resolution modelling. Boundary-Layer Meteorol. 182 (3), 471–495. doi:10.1007/s10546-021-00661-x
Han, Z., Ge, J., Chen, X., Hu, X., Yang, X., and Du, J. (2022). Dust activities induced by nocturnal low-level jet over the taklimakan Desert from WRF-chem simulation. J. Geophys. Res. Atmos. 127 (12), e2021JD036114. doi:10.1029/2021jd036114
Hicks, B. B., O’Dell, D. L., Eash, N. S., and Sauer, T. J. (2015). Nocturnal intermittency in surface CO2 concentrations in sub-Saharan Africa. Agric. For. Meteorology 200, 129–134. doi:10.1016/j.agrformet.2014.09.007
Hu, X. M., Doughty, D. C., Sanchez, K. J., Joseph, E., and Fuentes, J. D. (2012). Ozone variability in the atmospheric boundary layer in Maryland and its implications for vertical transport model. Atmos. Environ. 46, 354–364. doi:10.1016/j.atmosenv.2011.09.054
Hu, X. M., Klein, P. M., Xue, M., Lundquist, J. K., Zhang, F., and Qi, Y. (2013a). Impact of low-level jets on the nocturnal urban heat island intensity in Oklahoma City. J. Appl. Meteorology Climatol. 52 (8), 1779–1802. doi:10.1175/jamc-d-12-0256.1
Hu, X. M., Klein, P. M., Xue, M., Zhang, F., Doughty, D. C., Forkel, R., et al. (2013b). Impact of the vertical mixing induced by low-level jets on boundary layer ozone concentration. Atmos. Environ. 70, 123–130. doi:10.1016/j.atmosenv.2012.12.046
Huang, N. E., Shen, Z., Long, S. R., Wu, M. C., Shih, H. H., Zheng, Q., et al. (1998). The empirical mode decomposition and the Hilbert spectrum for nonlinear and non-stationary time series analysis. Proc. R. Soc. 454, 903–995. doi:10.1098/rspa.1998.0193
Janjic, Z. I. (2002). Nonsingular implementation of the Mellor–Yamada level 2.5 scheme in the NCEP meso model. NCEP Off. note 437, 61.
Jia, M., Yuan, J., Wang, C., Xia, H., Wu, Y., Zhao, L., et al. (2019). Long-lived high-frequency gravity waves in the atmospheric boundary layer: Observations and simulations. Atmos. Chem. Phys. 19 (24), 15431–15446. doi:10.5194/acp-19-15431-2019
Jia, W., and Zhang, X. (2020). The role of the planetary boundary layer parameterization schemes on the meteorological and aerosol pollution simulations: A review. Atmos. Res. 239, 104890. doi:10.1016/j.atmosres.2020.104890
Jia, W., Zhang, X., Zhang, H., and Ren, Y. (2021). Application of turbulent diffusion term of aerosols in mesoscale model. Geophys. Res. Lett. 48 (11), e2021GL093199. doi:10.1029/2021gl093199
Jin, X., Cai, X., Yu, M., Song, Y., Wang, X., Kang, L., et al. (2020). Diagnostic analysis of wintertime PM2. 5 pollution in the North China Plain: The impacts of regional transport and atmospheric boundary layer variation. Atmos. Environ. 224, 117346. doi:10.1016/j.atmosenv.2020.117346
Ju, T., Wu, B., Wang, Z., Liu, J., Chen, D., and Zhang, H. (2020). Relationships between low-level jet and low visibility associated with precipitation, air pollution, and fog in Tianjin. Atmosphere 11 (11), 1197. doi:10.3390/atmos11111197
Kalenderski, S., and Stenchikov, G. (2016). High-resolution regional modeling of summertime transport and impact of African dust over the Red Sea and Arabian Peninsula. J. Geophys. Res. Atmos. 121 (11), 6435–6458. doi:10.1002/2015jd024480
King, J. A., Engelstaedter, S., Washington, R., and Munday, C. (2021). Variability of the Turkana low-level jet in reanalysis and models: Implications for rainfall. J. Geophys. Res. Atmos. 126 (10), e2020JD034154. doi:10.1029/2020jd034154
Klein, A., Ravetta, F., Thomas, J. L., Ancellet, G., Augustin, P., Wilson, R., et al. (2019). Influence of vertical mixing and nighttime transport on surface ozone variability in the morning in Paris and the surrounding region. Atmos. Environ. 197, 92–102. doi:10.1016/j.atmosenv.2018.10.009
Knippertz, P., and Todd, M. C. (2012). Mineral dust aerosols over the Sahara: Meteorological controls on emission and transport and implications for modeling. Rev. Geophys. 50, RG1007. doi:10.1029/2011rg000362
Kulkarni, P. S., Dasari, H. P., Sharma, A., Bortoli, D., Salgado, R., and Silva, A. M. (2016). Nocturnal surface ozone enhancement over Portugal during winter: Influence of different atmospheric conditions. Atmos. Environ. 147, 109–120. doi:10.1016/j.atmosenv.2016.09.056
Li, Q., Wu, B., Liu, J., Zhang, H., Cai, X., and Song, Y. (2020). Characteristics of the atmospheric boundary layer and its relation with PM2.5 during haze episodes in winter in the North China Plain. Atmos. Environ. 223, 117265. doi:10.1016/j.atmosenv.2020.117265
Li, Q., Zhang, H., Cai, X., Song, Y., and Zhu, T. (2021). The impacts of the atmospheric boundary layer on regional haze in North China. npj Clim. Atmos. Sci. 4 (1), 9–10. doi:10.1038/s41612-021-00165-y
Li, X., Hu, X. M., Ma, Y., Wang, Y., Li, L., and Zhao, Z. (2019). Impact of planetary boundary layer structure on the formation and evolution of air-pollution episodes in Shenyang, Northeast China. Atmos. Environ. 214, 116850. doi:10.1016/j.atmosenv.2019.116850
Lima, D. C., Soares, P. M., Semedo, A., Cardoso, R. M., Cabos, W., and Sein, D. V. (2019). A climatological analysis of the Benguela coastal low-level jet. J. Geophys. Res. Atmos. 124 (7), 3960–3978. doi:10.1029/2018jd028944
Martins, L. D., Hallak, R., Alves, R. C., de Almeida, D. S., Squizzato, R., Moreira, C. A., et al. (2018). Long-range transport of aerosols from biomass burning over southeastern South America and their implications on air quality. Aerosol Air Qual. Res. 18 (7), 1734–1745. doi:10.4209/aaqr.2017.11.0545
Mei, M., Ding, Y., Wang, Z., Liu, Y., and Zhang, Y. (2022). Effects of the East Asian subtropical westerly jet on winter persistent heavy pollution in the Beijing–Tianjin–Hebei region. Int. J. Climatol. 42 (5), 2950–2964. doi:10.1002/joc.7400
Melo, A. M., Dias-Junior, C. Q., Cohen, J. C., Sá, L. D., Cattanio, J. H., and Kuhn, P. A. (2019). Ozone transport and thermodynamics during the passage of squall line in Central Amazon. Atmos. Environ. 206, 132–143. doi:10.1016/j.atmosenv.2019.02.018
Miao, Y., Guo, J., Liu, S., Wei, W., Zhang, G., Lin, Y., et al. (2018). The climatology of low-level jet in Beijing and Guangzhou, China. J. Geophys. Res. Atmos. 123 (5), 2816–2830. doi:10.1002/2017jd027321
Miao, Y., Liu, S., Sheng, L., Huang, S., and Li, J. (2019). Influence of boundary layer structure and low-level jet on PM2.5 pollution in beijing: A case study. Int. J. Environ. Res. public health 16 (4), 616. doi:10.3390/ijerph16040616
Monin, A., and Obukhov, A. (1954). Basic laws of turbulent mixing in the surface layer of the atmosphere. Contrib. Geophys Inst. Acad. Sci. USSR 151, 163–187.
Pérez, C., Haustein, K., Janjic, Z., Jorba, O., Huneeus, N., Baldasano, J. M., et al. (2011). Atmospheric dust modeling from meso to global scales with the online NMMB/BSC-Dust model - Part 1: Model description, annual simulations and evaluation. Atmos. Chem. Phys. 11, 13001–13027. doi:10.5194/acp-11-13001-2011
Reitebuch, O., Strassburger, A., Emeis, S., and Kuttler, W. (2000). Nocturnal secondary ozone concentration maxima analysed by sodar observations and surface measurements. Atmos. Environ. 34 (25), 4315–4329. doi:10.1016/s1352-2310(00)00185-0
Ren, Y., Zhang, H., Wei, W., Cai, X., Song, Y., and Kang, L. (2019a). A study on atmospheric turbulence structure and intermittency during heavy haze pollution in the Beijing area. Sci. China Earth Sci. 62 (12), 2058–2068. doi:10.1007/s11430-019-9451-0
Ren, Y., Zhang, H., Wei, W., Wu, B., Cai, X., and Song, Y. (2019b). [Standards and quality control points of alginate-based medical devices]. Atmos. Chem. Phys. 19 (2), 1041–1044. doi:10.7507/1002-1892.201812002
Ren, Y., Zhang, H., Wei, W., Wu, B., Liu, J., Cai, X., et al. (2019c). Comparison of the turbulence structure during light and heavy haze pollution episodes. Atmos. Res. 230, 104645. doi:10.1016/j.atmosres.2019.104645
Ren, Y., Zhang, H., Zhang, X., Wei, W., Li, Q., Wu, B., et al. (2021). Quantitative verification of the turbulence barrier effect during heavy haze pollution events. Sci. Total Environ. 753, 045005. doi:10.1088/2515-7620/ac6381
Rodríguez-Gómez, C., Echeverry, G., Jaramillo, A., and Ladino, L. A. (2022). The negative impact of biomass burning and the Orinoco low-level jet on the air quality of the Orinoco River Basin. Atmósfera 35 (3), 497–520. doi:10.20937/ATM.52979
Ryan, W. F. (2004). The low level jet in Maryland: Profiler observations and preliminary climatology. Maryland: Dept. of the Environment Rep, 43.
Salmond, J. A., and McKendry, I. G. (2005). A review of turbulence in the very stable nocturnal boundary layer and its implications for air quality. Prog. Phys. Geogr. 29 (2), 171–188. doi:10.1191/0309133305pp442ra
Salmond, J. A. (2004). Wavelet analysis of intermittent turbulence in a very stable nocturnal boundary layer: Implications for the vertical mixing of ozone. Boundary-Layer Meteorol. 114 (3), 463–488. doi:10.1007/s10546-004-2422-3
Salmond, J., and McKendry, I. G. (2002). Secondary ozone maxima in a very stable nocturnal boundary layer: Observations from the lower fraser valley, BC. Atmos. Environ. 36 (38), 5771–5782. doi:10.1016/s1352-2310(02)00698-2
Samson, P. J. (1978). Nocturnal ozone maxima. Atmos. Environ. 12 (4), 951–955. doi:10.1016/0004-6981(78)90035-5
Sánchez, M. P., Pereira de Oliveira, A., Varona, R. P., Tito, J. V., Codato, G., Ynoue, R. Y., et al. (2022). Observational investigation of the low-level jets in the metropolitan region of São Paulo, Brazil. Earth Space Sci. 9, e2021EA002190. doi:10.1029/2021ea002190
Sandu, I., Beljaars, A., Bechtold, P., Mauritsen, T., and Balsamo, G. (2013). Why is it so difficult to represent stably stratified conditions in numerical weather prediction (NWP) models? J. Adv. Model. Earth Syst. 5, 117–133. doi:10.1002/jame.20013
Schepanski, K., Knippertz, P., Fiedler, S., Timouk, F., and Demarty, J. (2015). The sensitivity of nocturnal low-level jets and near-surface winds over the Sahel to model resolution, initial conditions and boundary-layer set-up. Q. J. R. Meteorological Soc. 141 (689), 1442–1456. doi:10.1002/qj.2453
Schepanski, K., Tegen, I., Todd, M., Heinold, B., Bönisch, G., Laurent, B., et al. (2009). Meteorological processes forcing Saharan dust emission inferred from MSG-SEVIRI observations of subdaily dust source activation and numerical models. J. Geophys. Res. 114, D10201. doi:10.1029/2008JD010325
Shi, L., Zhang, J., Yao, F., Zhang, D., and Guo, H. (2021). Drivers to dust emissions over dust belt from 1980 to 2018 and their variation in two global warming phases. Sci. Total Environ. 767, 144860. doi:10.1016/j.scitotenv.2020.144860
Smith, E. N., Gebauer, J. G., Klein, P. M., Fedorovich, E., and Gibbs, J. A. (2019). The Great Plains low-level jet during PECAN: Observed and simulated characteristics. Mon. Weather Rev. 147 (6), 1845–1869. doi:10.1175/mwr-d-18-0293.1
Smith, E. N., Gibbs, J. A., Fedorovich, E., and Klein, P. M. (2018). WRF Model study of the Great Plains low-level jet: Effects of grid spacing and boundary layer parameterization. J. Appl. Meteorology Climatol. 57 (10), 2375–2397. doi:10.1175/jamc-d-17-0361.1
Starn, T. K., Shepson, P. B., Bertman, S. B., Riemer, D. D., Zika, R. G., and Olszyna, K. (1998). Nighttime isoprene chemistry at an urban-impacted forest site. J. Geophys. Res. Atmos. 103 (17), 22437–22447. doi:10.1029/98jd01201
Stull, R. B. (1988). An introduction to boundary layer meteorology. Dordrecht: Kluwer Academic Publishers.
Tindan, J. Z. O., Jin, Q., and Pu, B. (2022). Understanding day-night differences in dust activities over the dust belt of North Africa, the Middle East, and Asia. Atmos. Chem. Phys. Discuss., 1–47. doi:10.5194/acp-2022-490
Todd, M. C., Washington, R., Raghavan, S., Lizcano, G., and Knippertz, P. (2008). Regional model simulations of the bodélé low-level jet of northern Chad during the bodélé dust experiment (BoDEx 2005). J. Clim. 21 (5), 995–1012. doi:10.1175/2007JCLI1766.1
Tuononen, M., O’Connor, E. J., Sinclair, V. A., and Vakkari, V. (2017). Low-level jets over Utö, Finland, based on Doppler lidar observations. J. Appl. Meteorology Climatol. 56 (9), 2577–2594. doi:10.1175/jamc-d-16-0411.1
Udina, M., Soler, M. R., Olid, M., Jiménez-Esteve, B., and Bech, J. (2020). Pollutant vertical mixing in the nocturnal boundary layer enhanced by density currents and low-level jets: Two representative case studies. Boundary-Layer Meteorol. 174 (2), 203–230. doi:10.1007/s10546-019-00483-y
van de Wiel, B. J. H., Moene, A. F., Steeneveld, G. J., Baas, P., Bosveld, F. C., and Holtslag, A. A. M. (2010). A conceptual view on inertial oscillations and nocturnal low-level jets. J. Atmos. Sci. 67 (8), 2679–2689. doi:10.1175/2010JAS3289.1
Wainwright, C. E., Stepanian, P. M., and Horton, K. G. (2016). The role of the US Great Plains low-level jet in nocturnal migrant behavior. Int. J. biometeorology 60 (10), 1531–1542. doi:10.1007/s00484-016-1144-9
Wang, S. C., Wang, Y., Estes, M., Lei, R., Talbot, R., Zhu, L., et al. (2018). Transport of central American fire emissions to the US Gulf coast: Climatological pathways and impacts on ozone and PM2. 5. J. Geophys. Res. Atmos. 123 (15), 8344–8361. doi:10.1029/2018jd028684
Wei, W., Zhang, H., Cai, X., Song, Y., Bian, Y., Xiao, K., et al. (2020). Influence of intermittent turbulence on air pollution and its dispersion in winter 2016/2017 over Beijing, China. J. Meteorological Res. 34 (1), 176–188. doi:10.1007/s13351-020-9128-4
Wei, W., Zhang, H. S., Schmitt, F. G., Huang, Y. X., Cai, X. H., Song, Y., et al. (2017). Investigation of turbulence behaviour in the stable boundary layer using arbitrary-order Hilbert spectra. Boundary-Layer Meteorol. 163 (2), 311–326. doi:10.1007/s10546-016-0227-9
Wei, W., Zhang, H. S., and Ye, X. X. (2014). Comparison of low-level jets along the north coast of China in summer. J. Geophys. Res. Atmos. 119 (16), 9692–9706. doi:10.1002/2014jd021476
Wei, W., Zhang, H., Wu, B., Huang, Y., Cai, X., Song, Y., et al. (2018). Intermittent turbulence contributes to vertical dispersion of PM<sub>2.5</sub> in the North China plain: Cases from tianjin. Atmos. Chem. Phys. 18 (17), 12953–12967. doi:10.5194/acp-18-12953-2018
Wei, Z., Zhang, H., Wei, W., Zhang, X., Cai, X., Song, Y., et al. (2022). Mechanism of the effect of vertically propagating internal gravity waves on turbulence barrier and pollutant diffusion during heavy haze episodes. Sci. Total Environ. 845, 157349. doi:10.1016/j.scitotenv.2022.157349
Wexler, H. (1961). A boundary layer interpretation of the low-level jet. Tellus 13, 368–378. doi:10.1111/j.2153-3490.1961.tb00098.x
Wiggs, G. F., Baddock, M. C., Thomas, D. S., Washington, R., Nield, J. M., Engelstaedter, S., et al. (2022). Quantifying mechanisms of aeolian dust emission: Field measurements at etosha Pan, Namibia. J. Geophys. Res. Earth Surf. 127 (8), e2022JF006675. doi:10.1029/2022jf006675
Wimhurst, J. J., and Greene, J. S. (2019). Oklahoma's future wind energy resources and their relationship with the Central Plains low-level jet. Renew. Sustain. Energy Rev. 115, 109374. doi:10.1016/j.rser.2019.109374
Wu, B., Li, Z., Ju, T., and Zhang, H. (2020). Characteristics of Low-level jets during 2015–2016 and the effect on fog in Tianjin. Atmos. Res. 245, 105102. doi:10.1016/j.atmosres.2020.105102
Yang, J., Tang, Y., Han, S., Liu, J., Yang, X., and Hao, J. (2021). Evaluation and improvement study of the Planetary Boundary-Layer schemes during a high PM2. 5 episode in a core city of BTH region, China. Sci. Total Environ. 765, 142756. doi:10.1016/j.scitotenv.2020.142756
Yang, X., Wu, K., Wang, H., Liu, Y., Gu, S., Lu, Y., et al. (2020). Summertime ozone pollution in Sichuan Basin, China: Meteorological conditions, sources and process analysis. Atmos. Environ. 226, 117392. doi:10.1016/j.atmosenv.2020.117392
Zhao, D., Xin, J., Wang, W., Jia, D., Wang, Z., Xiao, H., et al. (2022). Effects of the sea-land breeze on coastal ozone pollution in the Yangtze River Delta, China. Sci. Total Environ. 807, 150306. doi:10.1016/j.scitotenv.2021.150306
Zhong, J., Zhang, X., Dong, Y., Wang, Y., Liu, C., Wang, J., et al. (2018). Feedback effects of boundary-layer meteorological factors on cumulative explosive growth of PM<sub>2.5</sub> during winter heavy pollution episodes in Beijing from 2013 to 2016. Atmos. Chem. Phys. 18 (1), 247–258. doi:10.5194/acp-18-247-2018
Zhong, J., Zhang, X., Wang, Y., Sun, J., Zhang, Y., Wang, J., et al. (2017). Relative contributions of boundary-layer meteorological factors to the explosive growth of PM2. 5 during the red-alert heavy pollution episodes in Beijing in December 2016. J. Meteorological Res. 31 (5), 809–819. doi:10.1007/s13351-017-7088-0
Zhou, C., Mamtimin, A., Yang, F., Huo, W., Wang, M., Pan, H., et al. (2019). Dust uplift potential in the taklimakan Desert: An analysis based on different wind speed measurement intervals. Theor. Appl. Climatol. 137 (1), 1449–1456. doi:10.1007/s00704-018-2678-z
Keywords: low-level jets, regional transport, vertical dispersion, intermittent turbulence, PM2.5, ozone
Citation: Wei W, Zhang H, Zhang X and Che H (2023) Low-level jets and their implications on air pollution: A review. Front. Environ. Sci. 10:1082623. doi: 10.3389/fenvs.2022.1082623
Received: 28 October 2022; Accepted: 12 December 2022;
Published: 04 January 2023.
Edited by:
Chaoyang Xue, UMR7328 Laboratoire de physique et chimie de l’environnement et de l'Espace (LPC2E), FranceReviewed by:
Chen Xu, Institute of Atmospheric Physics (CAS), ChinaQian Chen, Nanjing University of Information Science and Technology, China
Copyright © 2023 Wei, Zhang, Zhang and Che. This is an open-access article distributed under the terms of the Creative Commons Attribution License (CC BY). The use, distribution or reproduction in other forums is permitted, provided the original author(s) and the copyright owner(s) are credited and that the original publication in this journal is cited, in accordance with accepted academic practice. No use, distribution or reproduction is permitted which does not comply with these terms.
*Correspondence: Wei Wei, weiw@cma.gov.cn