- 1School of Material and Environmental Engineering, Shenzhen Polytechnic, Shenzhen, China
- 2College of Civil and Transportation Engineering, The Underground Polis Academy, Shenzhen University, Shenzhen, China
- 3Shenzhen Key Laboratory of Green, Efficient and Intelligent Construction of Underground Metro Station, Shenzhen, Guangdong, China
- 4School of Civil and Environmental Engineering, Shenyang Jianzhu University, Shenyang, China
UV/sulfite process without oxygen has been extensively explored and used for the degradation of many micro-pollutants. In the present work, a UV/sulfite process in the presence of oxygen was applied to degrade a widely used pharmaceutical compound, metformin (MET). The results showed that the oxygen-involved UV/sulfite process could efficiently degrade MET. At an initial concentration of 2.5 mg/L, 86.0% of MET was removed from the contaminated water within 180 min irradiation at a sulfite dosage of 10 mM, solution pH of 9, and UV intensity of 4,092 μW/cm2. In addition, sulfate radical (SO4•-), hydroxide radical (•OH), hydrogen atom (•H), and hydrated electron (eaq−) were found to be the dominant active species contributing to MET removal in the studied process through scavenging experiments. The increase in UV intensity, sulfite dosage, solution pH, and reaction temperature enhanced MET degradation in the investigated process to a certain extent, whereas the introduction of bicarbonate and fulvic acid slightly suppressed MET degradation. Finally, the degradation products of MET were identified. The oxygen-involved UV/sulfite process exhibited a remarkable denitrification capacity (>80%) in MET. The findings of this study may offer a novel approach for treating emerging contaminants.
1 Introduction
Pharmaceutical and personal care products (PPCPs) comprise diverse organic substances, including antibiotics, analgesics, hormones for pharmaceuticals and cosmetics, antibacterial agent, preservatives for personal care products (Zhang et al., 2022). Metformin (MET), a common antidiabetic drug used for type Ⅱ diabetes treatment, has been ranked among the most widely used PPCPs because more than 200 million people are suffering from diabetes worldwide (Yu et al., 2022). The widespread use of MET inevitably results in its emission into the environment through wastewater discharge as conventional wastewater treatment approaches (i.e., flocculation, activated carbon filtration, and bio-degradation) are ineffective for MET removal (Scheurer et al., 2009). Thus, the occurrence of MET in sewage, effluent in sewage treatment plants, and surface water is frequently reported (Ambrosio-Albuquerque et al., 2021). For example, a relatively high concentration of MET ranging from 70 to 325 μg/L was found in the effluent of a Portuguese sewage treatment plant (Gaffney et al., 2017). The potential bio-toxicity of MET, such as causing estrogenic effects or acting as an endocrine disruptor, has been reported in some literature (Niemuth and Klaper, 2015; Crago et al., 2016). Hence, MET has become an emerging pollutant in aquatic environments.
Several attempts have been made to alleviate MET contamination, including graphene oxide adsorption, photo-oxidation, and electro-oxidation (Zhu et al., 2017; Karimiana et al., 2020). Owing to the operational simplicity and cost-efficiency of light sources, photo-oxidation methods (i.e., UV/Fenton, UV/H2O2, and UV/TiO2) have become the most favored treatment techniques for organic pollutants degradation (Zhou et al., 2017; George et al., 2022).
In recent decades, the UV/sulfite system has gained increasing attention as an often-mentioned advanced reduction process (ARP) because of the production of various reductive species, such as hydrated electron (eaq−, E = –2.9 V) and hydrogen atom (•H, E = –2.3 V) (Vellanki et al., 2013; Ding et al., 2022). Several halogenated organic pollutants decomposition by the UV/sulfite system have already been investigated. For instance, Song et al. (2013) reported that perfluorooctanoic acid (PFOA), which is an extremely recalcitrant halogenated contaminant, can be completely decomposed in the UV/sulfite system after 1 h reaction, and a high defluorination efficiency of approximately 90% could be obtained by extending the reaction time to 24 h. Liu et al. (2015) achieved nearly 40% degradation efficiency of atenolol (20 μM) even at a neutral solution pH. In the above-mentioned degradation process, eaq− was manifested the dominant reductive species contributing to the decomposition of the target pollutants, and oxygen was previously removed from the reaction system by purging nitrogen, because oxygen can react with eaq− and lead to superoxide radical (O2•–) generation. Nevertheless, once oxygen is applied in the sulfite mediated UV photolysis system, another mild radical, namely sulfite radical (SO3•–), is likely to be transformed into a highly reactive oxidant sulfate radical (SO4•–) (Deister and Warneck, 1990), indicating that the oxygen-involved UV/sulfite system can be regarded as a prospective advanced oxidation process (AOP) (Huang et al., 2018; Li et al., 2020). Recently, (Chen et al. (2021) developed a synergetic-photoelectric sulfite mediated system where oxygen was continuously produced through water electrolysis. The combined effect of electrolysis and photolysis on sulfite brings about a large number of active species generation. The steady-state SO4∙– concentration was determined as ranging from .2 × 10–12 to 1.1 × 10–12 M. Correspondingly, this reaction system had extraordinary oxidative capacity in removing micro-pollutants such as ibuprofen and bisphenol A. Although several researchers have explored treatment efficiency of the oxygen-involved UV/sulfite system towards some emerging contaminants (i.e., atrazine, bisphenol A, trichlorophenol) (Cao et al., 2021a), the systematic investigation on the degradation efficiency of the antidiabetic drug, namely MET, was still scanty. Furthermore, investigation of the oxygen-involved UV/sulfite system on target pollutant removal in the real water matrix was also scarce.
Herein, a UV/sulfite system with the co-presence of oxygen was built and utilized for MET degradation. The crucial species contributing to MET degradation were ascertained. The impact of several common factors such as pH value of testing solution, reaction temperature, sulfite dosage and co-present compounds on MET degradation was evaluated. In addition, MET degradation in a real water matrix was monitored. The degradation products of MET as well as the bio-toxicity change of the testing solution were also determined. Outcomes in the present work suggests a distinctive treatment technology for emerging contaminants.
2 Materials and methods
2.1 Materials
Metformin hydrochloride (MET, 97.0%, C4N5H11•HCl), sodium sulfite (98.0%, Na2SO3), tertiary-butanol (TBA, ≥99.5%, C4H10O), methanol (MeOH, >99.9%, CH3OH), fulvic acid (85.0%, FA), potassium nitrate (>99.0%, KNO3), superoxide dismutase (SOD, ≥1,400 units/mg dry weight), and sodium bicarbonate (99.7%, NaHCO3) were purchased from Aladdin Co., LTD. (Shanghai, China) or Sigma-Aldrich Co., LTD. (Guangzhou, China). A Millipore purification system (Millli-Q IQ 7000, Darmstadt, Germany) was employed for the generation of ultrapure water and subsequent preparation for diverse reaction solution.
2.2 MET degradation experiments
MET degradation experiments were conducted in a 3-L cylindrical glass vessel (Supplementary Figure S1). A mixed solution containing MET and sulfite was filled into the vessel and magnetically stirred during the degradation process. In addition, the testing solution was purged with air or nitrogen gas (1 L/min) for 180 min. Apart from investigating the impact of solution pH on MET removal, the solution pH was maintained at approximately 9 with 10 mM phosphate buffer. A monochromatic low-pressure lamp (λ = 254 nm, 10 W, Dongguan, China) with a quartz sleeve enclosure was used as the irradiation source. The UV lamp was ignited and warmed for 30 min before commencing the irradiation experiments to obtain a stable output. MET batch degradation experiments were carried out at a constant temperature (25°C) through a thermostatic recirculation system (THD-1015, Ningbo, China). KNO3 (5 mM), NaNO2 (5 mM), MeOH (300 mM), TBA (300 mM), and SOD (3.3 mg/L) were introduced as scavengers to ascertain the contribution of different active species to MET degradation. Sulfite dosage was changed from 2.5 mM to 15 mM to study the impact of the sulfite dosage on MET removal. To reveal the impact of UV intensity on MET degradation efficiency, the UV lamp was wrapped with a few aluminum foil to change UV light emitted into the reaction solution (Li et al., 2014). The maximum UV intensity (100% I0) entering into the reaction solution was measured to be 4,092 μW/cm2 using a UV irradiatometer (Linshang Co., LTD., Shenzhen, China). Additionally, effect of the coexisting constituents such as HCO3− and natural organic matter (NOM) on MET removal was monitored by adding diverse concentrations of HCO3− (0–2.0 mM) and FA (0–15 mg/L) to the reaction system. Additionally, two surface water samples from Dasha River in Nanshan District (Shenzhen, China) was obtained and used to evaluate MET degradation efficiency in a real water matrix. Water quality parameters of the surface water samples were listed in Supplementary Table S1. Samples were withdrawn at the preset intervals for immediate analysis. All experiments were performed in triplicate, and the mean values were presented.
2.3 Analysis procedures
MET concentration was analyzed by using a Waters high-performance liquid chromatography which was equipped with a reversed C18 column (5 μm, 4.6 × 250 mm, Phenomenex, Torrance, United States) and a UV detector. The separation conditions were set as follows: A mixed solution which contained .1% acetic acid (80%) and MeOH (20%) was employed as mobile phase. The flow rate of mobile phase was determined as 1.0 mL/min. The wavelength of the UV detector for MET concentration measurement was 246 nm.
A pH meter (Mettler Toledo, Shanghai, China) was employed for solution pH determination. Inorganic degradation products of MET such as nitrite and ammonia nitrogen were detected through a colorimetric method. The detection of organic intermediates was performed in a ultra high-performance liquid chromatography (UPLC, Agilent 1290, CA, United States) coupled with time-of-flight mass spectrometry (AB SICEX Tripe TOF 4600, CA, United States). The mobile phase was a mixture of acetonitrile (10%) and .1% formic acid (90%) with a flow rate of .8 mL/min. The column temperature and injection volume of sample were set at 30°C and 10 μL, respectively. Bio-toxicity of the testing solution was determined by the luminescent bacteria toxicity test referring to the guideline from ISO standard (quality, 2007), and the relative inhibition rate towards luminescent bacteria was used as an indicator to reflect bio-toxicity of the testing solution.
3 Results and discussion
3.1 MET degradation in direct UV and UV/sulfite systems
MET degradation in four different reaction systems was monitored, and the results were shown in Figure 1A, B. 62.0% MET removal was obtained after 180 min irradiation in the oxygen-involved UV/sulfite system at sulfite dosage of 10 mM, whereas negligible MET removal (<5%) was achieved in direct UV and sulfite systems, which was ascribed to the weak absorption of MET in the UV-C region and poor reduction capacity of sulfite (Maćerak et al., 2018; Karimiana et al., 2020). Although the oxygen-free UV/sulfite system behaved better MET treatment efficiency, it needed constant purging of nitrogen gas, which made it unpractical for real wastewater treatment. MET degradation kinetics in the two UV/sulfite system were found to fit the pseudo first-order model, and observed rate constants (kobs) were .0205 min−1 and .0318 min−1, respectively. Comparison between this work and previous investigations concerning about MET removal by photolytic methods was conducted (Table 1), and kobs of MET degradation in this work was a bit faster than that of previous studies. Additionally, energy consumption needed for MET removal to half of its initial concentration was calculated as .1 kJ/μmol, which was at least 1–2 order of magnitude lower than that of other emerging contaminants (i.e., PFOA, ICMs) degraded by photolytic techniques (Vecitis et al., 2009; Gu et al., 2022). The obtained results suggested the studied oxygen-involved UV/sulfite process was promising for practical MET-containing wastewater treatment.
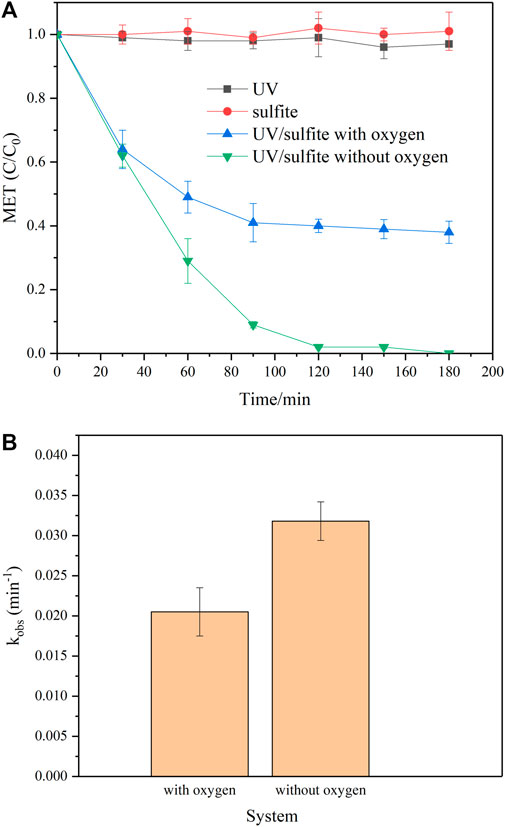
FIGURE 1. (A) MET degradation efficiency in different reaction processes; (B) Pseudo first-order rate constants for MET degradation with or without oxygen by the UV/sulfite process. Experimental conditions: [MET]0 = 10 mg/L, [SO32-]0 = 10 mM, pH = 9.
The extraordinary MET degradation performance in the UV/sulfite system was ascribed to the generation of reactive species. When sulfite undergoes UV photolysis, reactive species like eaq−,∙H, and SO3∙– have been reported to be produced before (Milh et al., 2021). In addition, the studied system was continuously purged with air, thus oxygen was likely to react with SO3∙– and lead to SO4∙– generation (Eqs. 1–4). According to Yang et al. (2015), hydroxyl radical (∙OH) can be transformed from SO4∙– in the testing solution. Hence, at least five kinds of reactive species, namely eaq−,∙H, SO3∙–, SO4∙–, and ∙OH, were likely to exist in the studied system. Scavenging reactions were conducted to determine the primary active species that contributed to MET removal.
As shown in Figure 2, the introduction of both MeOH and TBA inhibited MET degradation. However, the inhibition effect of MeOH was more obvious, indicating that both SO4∙– and ∙OH contributed to MET degradation. Because MeOH reacted quickly with both SO4∙– and ∙OH at a comparable rate constant, whereas TBA merely efficiently quenched ∙OH (Supplementary Table S2). Moreover, nitrate and nitrite were employed in the reaction system to uncover the role of reductive species (i.e., eaq−,∙H) played in MET removal, and MET degradation was apparently suppressed in the presence of nitrate and nitrite, where nitrite exhibited a more obvious inhibition. When 5 mM of nitrate and nitrite were introduced into the reaction system individually, MET degradation ratio decreased by 13.0% and 44.7% after 180 min irradiation, respectively. It is well documented that both nitrate and nitrite can efficiently scavenge eaq− at 109 M−1s−1 level (Buxton et al., 1988). Nevertheless, nitrite reacts with ∙H approximately 500 times as fast as that for nitrate (Buxton et al., 1988), which led to a similar eaq− concentration but a much lower ∙H concentration. The different ∙H and eaq− concentration was thus used to identify their impact on MET removal. Results in Figure 2 indicated both ∙H and eaq− participated in the MET degradation process. Considering another mild oxidant, SO3∙– (E0 = .63 V, pH = 7) (Buxton et al., 1988), many studies have reported its negligible contribution to target pollutant decomposition in sufite-based UV photolytic system (Li et al., 2012; Gu et al., 2016). Notably, the generated SO3∙– can be transformed into highly oxidative SO4∙– when co-present with oxygen, as demonstrated in the above discussion by alcohol inhibition experiments. Consequently, participation of SO3∙– in MET degradation can be excluded. In the presence of oxygen, eaq− may be quickly quenched and resulted in O2− generation (Deister and Warneck, 1990). Therefore, SOD was selected as the quencher and applied to the reaction solution to evaluate the contribution of O2∙– to MET degradation. Negligible MET suppression (<5%) was observed when SOD was introduced into the UV/sulfite process, suggesting an insignificant role of O2∙–. In summary, it is highly probable that reactive species, including SO4∙–, ∙OH, ∙H and eaq−, mainly accounted for MET degradation in the oxygen-involved UV/sulfite system.
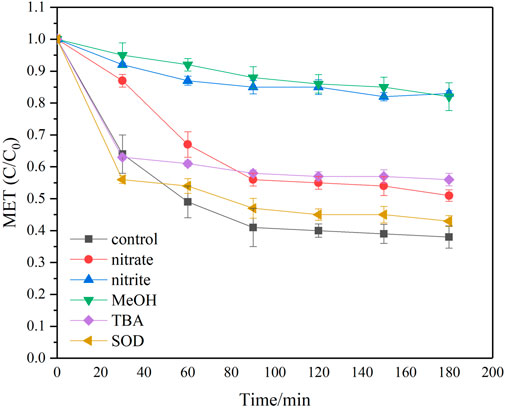
FIGURE 2. The effect of different scavengers on MET degradation. Experimental conditions: [MET]0 = 10 mg/L, [SO32-]0 = 10 mM, pH = 9, [TBA]0 = 300 mM, [MeOH]0 = 300 mM, [KNO3]0 = 5 mM, [NaNO2]0 = 5 mM, and [SOD]0 = 3.3 mg/L.
3.2 Influence of incident UV intensity
In the UV photolytic process, the incident UV intensity is a major factor determining the reaction rate between target pollutant and reactive species generated. Zhang et al. (2013) found methylene blue removal rate in a UV/TiO2/H2O2 system was positively proportional to the UV intensity. Similar results were reported by Liu et al. (2014) A higher UV intensity was found beneficial for enhancing dichloroethane removal in a UV/sulfite system, and the observed rate constant of dichloroethane removal showed a linear relationship with UV intensity (R2 > .99). MET degradation experiments at different UV intensity were carried out and results were presented in Figure 3A. MET degradation ratio was obviously enhanced by increasing UV intensity. MET degradation efficiency altered from 47.6% to 62.0% after 180 min reaction when UV intensity varied from 33% I0 to 100% I0. MET degradation in the UV/sulfite system followed pseudo first-order kinetics (R2 > .98) (Supplementary Table S2). The observed rate constants of MET degradation at 100% I0 was almost doubled when compared with that at 33% I0. When UV intensity entering the testing solution increased, the amount of generated radicals increased accordingly, which in consequence led to a faster MET removal. Referring to previous investigations, the generation rates of eaq− and SO3∙– are proportional to UV intensity under alkaline pH conditions when sulfite undergoes UV photolysis (Song et al., 2013). eaq− and SO4∙– which was produced through chain reactions between O2 and SO3∙–, were manifested the governing active species accounting for MET degradation in Section 3.1. Thus an increase in UV intensity would benefit MET transformation in the studied reaction system.
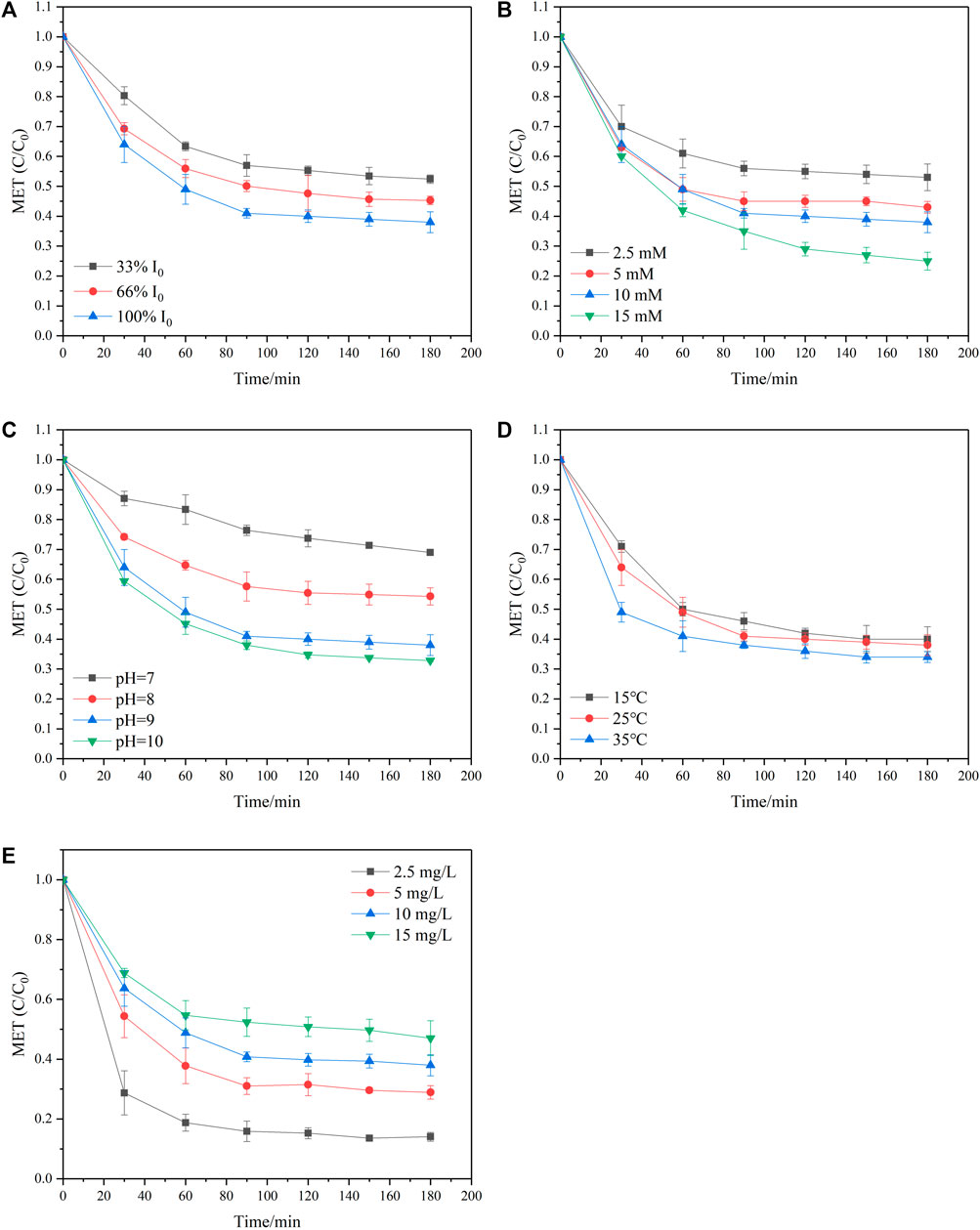
FIGURE 3. The effect of different water quality or operating parameters (A) UV intensity; (B) sulfite dosage; (C) solution pH; (D) reaction temperature; (E) initial MET concentration on MET degradation by the oxygen-involved UV/sulfite system. Experimental conditions: [MET]0 = 10 mg/L, [SO32-]0 = 10 mM, pH = 9, T = 25°C.
3.3 Influence of sulfite dosage
Different sulfite concentrations were introduced to monitor their influence on MET removal at an initial MET concentration of 10 mg/L, and the results were presented in Figure 3B. MET degradation was apparently enhanced by increasing sulfite dosage. As depicted in Figure 3B, increasing sulfite dosage from 2.5 mM to 15 mM led to a higher degradation ratio of MET, increasing from 46.9% to 75.5% after 180 min irradiation. The obvious promotion on MET removal at higher sulfite dosage further confirmed the negligible role of direct photolysis. If direct photolysis governs the MET degradation process, the augmentation of sulfite dosage may result in a decrease in UV intensity, which otherwise would be absorbed by MET, and thus causing a reduction in kobs (Liu et al., 2014). As depicted in Figure 3B and Supplementary Table S3, the optimal sulfite dosage was 10 mM, and further increasing the sulfite concentration slowed MET degradation rate. At a constant solution pH and UV intensity, the reactive species (i.e., eaq− and SO3∙–) production was positively correlated with sulfite dosage (Yoon et al., 2014), thereby causing the difference in MET degradation kinetics. When sulfite dosage was changed from 2.5 mM to 10 mM, a 1.1 fold increase in kobs was observed (Supplementary Table S3). Nevertheless, a further increase in sulfite dosage to 15 mM led to a decline in kobs to .0175 min−1. At higher sulfite dosages, the scavenging reactions between the generated reactive species (e.g., ∙H, eaq−) and co-present constituents became vigorous, thus enormous reactive species were quenched, and their use in MET degradation was limited (Fu et al., 2010; Yazdanbakhsh et al., 2018). Consequently, sulfite dosage exhibited less of enhancement effect on MET degradation, which was reflected by the reduction in kobs.
3.4 Influence of solution pH
Apart from sulfite dosage, the solution pH also affected the degradation of target pollutants in the sulfite mediated photolysis process. Figure 3C presented the effect of solution pH on MET removal at a sulfite dosage of 10 mM. MET degradation was substantially facilitated when the solution pH was changed from neutral to alkaline conditions. The optimal solution pH was at 9, further increasing the solution pH brought about slight enhancement both in degradation efficiency and rate of MET. Moreover, MET degradation kinetics in the investigated solution pH range was well depicted by the pseudo first-order model (Supplementary Table S3), which was in accordance with previous studies (Li et al., 2012; Liu et al., 2015).
Two main reasons can be used to explain the impact of solution pH on MET removal in the UV/sulfite system. On one hand, sulfite distribution would change along with the alteration of solution pH. As shown in Supplementary Table S4, the major form of sulfite at neutral pH condition is HSO3−, and its proportion gets declined with the increase of solution pH, which means the reactive species generated from HSO3− photolysis would accordingly decreased. On the contrary, the production of eaq− can be enhanced because of the increase of SO32– proportion. Therefore, a dramatic promotion on MET degradation was obtained when solution pH was adjusted from pH seven to pH 9. SO32– species accounts for more than 98% of sulfite species at pH 9, thus further increase of solution pH shows little effect on target pollutant degradation. On the other hand, solution pH also affected the transformation between ∙H and eaq−. The transformation from ∙H to eaq− was promoted at a higher pH condition (Eq. 5). Since eaq− was verified an important contributor for MET removal in the investigated system, the higher eaq− concentration would certainly lead to better MET treatment efficiency.
Moreover, the distribution of MET molecule was strongly dependent on the solution pH. As reported by (Scheurer et al. (2009), the principal existing form of MET was its neutral molecule when solution pH was above 12, whereas the protonation reaction occurred at two amino groups accompanied with a decrease in solution pH, which was nearly accomplished at pH 8. The change in the charged MET species may also impact its reaction with various oxidative or reductive species in the studied system, and then caused different MET degradation efficiency.
3.5 Influence of reaction temperature
Temperature is of great importance when determining the rates of chemical reactions. Hence the impact of reaction temperature on MET degradation was explored, and the results were shown in Figure 3D. MET degradation was remarkably enhanced with the increase in reaction temperature. When we transformed Figure 3D to semilog plots, it was found that during the studied temperature range, MET degradation was well fitted with a pseudo first-order model (R2 > .97) (Supplementary Table S3). Additionally, the MET degradation rate increased 1.1–1.8 folds when the reaction temperature was raised by increments of 10°C. Based on the Van’t Hoff equation, the reaction rate of a common chemical process increases approximately 2–4 folds when the reaction temperature is increased by 10°C. Apparently, the impact of temperature on MET removal in the oxygen-involved UV/sulfite system was weakened, hinting the photo-chemical process played a key role during MET degradation. Moreover, the activation energy was calculated as 27.8 kJ/mol, which was comparable to that of a commonly used PPCPs, atenolol, treated by the sulfite-mediated UV irradiation process (Liu et al., 2015).
3.6 Influence of initial MET concentration
MET concatenation was varied in different water matrix (Ambrosio-Albuquerque et al., 2021), thus it’s necessary to evaluate the effect of initial MET concentration on MET degradation in the present system. As depicted in Figure 3E, approximately 86.0% MET was removed from the aqueous media at initial MET concentration of 2.5 mg/L, and the degradation ratio gradually decreased to 53.0% as the initial MET concentration increased to 15 mg/L. Under the circumstance of lower MET concentration, the produced reactive species in the oxygen-involved UV/sulfite system were such sufficient that most of MET could be degraded. As the initial MET concentration increased, the effective collision frequency between MET molecule and the reactive species was decreased. Additionally, more intermediates of MET would be generated when a higher initial MET concentration was employed in the investigated system, which also might compete for the reactive species with MET and accordingly slackened MET degradation (Shah et al., 2014).
3.7 Influence of co-present compounds
In real aqueous environments, many compounds are co-present with MET, and their presence may impact MET degradation capacity in the UV/sulfite system. HCO3− and FA are ubiquitously present in natural water, thus they were selected as representative inorganic and organic substance, respectively, to investigate the water quality effect on MET degradation. The results are presented in Figure 4. In general, the introduction of HCO3− to the UV/sulfite system slightly suppressed MET removal, and the suppression effect of HCO3− was aggravated with an increase in HCO3− concentration. MET degradation ratio decreased from 62.0% to 52.6% when HCO3− dosage was increased by 2.0 mM after 180 min irradiation. The inhibitory effect of HCO3− on MET removal was ascribed to the following reasons. Firstly, it was reported that HCO3− can act as a quencher for ∙OH (Eq 6) (Buxton et al., 1988), thus the introduction of HCO3− to the reaction solution may result in ∙OH consumption, which was attested to be responsible for MET degradation and further caused the reduction of MET degradation efficiency. Secondly, HCO3− could also react with another vigorous species, eaq−, in the studied system, thus a certain amount of eaq− may be consumed by HCO3− and consequently cause an inhibition effect on MET removal. Nevertheless, the rate constant between HCO3− and eaq− (or ∙OH) was relatively slow (k ≈ 106 M−1 s−1) (Buxton et al., 1988), which was at least 10 times smaller than that between perfluorooctanoic acid, a notorious persistent contaminant, and eaq− (Huang et al., 2007). Therefore, the co-presence of 2 mM HCO3− only led to a 10.2% decrease in MET removal in the UV/sulfite process during 180 min reaction.
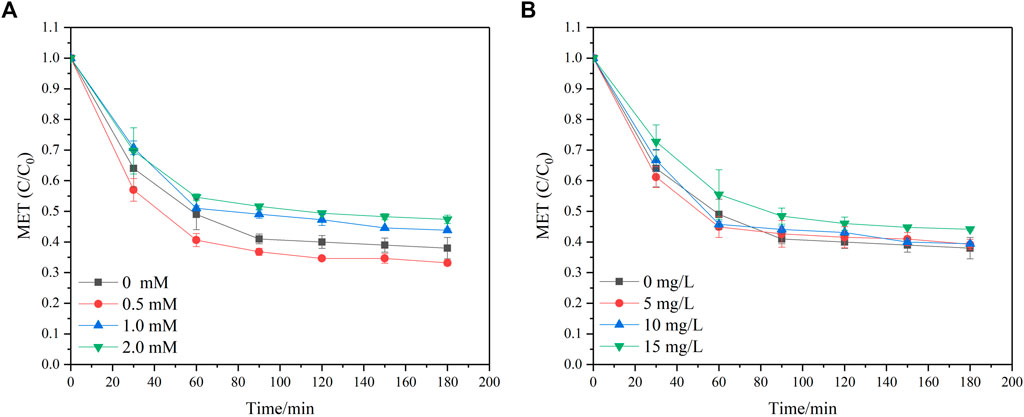
FIGURE 4. MET degradation with co-presence of (A) HCO3− and (B) FA. Experimental conditions: [MET]0 = 10 mg/L, [SO32-]0 = 10 mM, UV intensity = 100% I0, and pH = 9.
Similarly, the introduction of FA resulted in a weak suppression on MET degradation. For instance, at the highest FA dosage of 15 mg/L, MET removal ratio was reduced by only 6.2%. These results differed slightly from those of other researches, which reported that the introduction of NOM to the sulfite-mediated UV photolysis process considerably reduced the target pollutant removal. Xiao et al. (2017) stated BrO3− removal ratio was evidently reduced in the sulfite-mediated UV photolysis process when humic acid was simultaneously added. They reported that the BrO3− removal ratio dramatically decreased from approximately 60.0%–44.8% and 17.0% at humic acid concentrations of 2 mg/L and 5 mg/L, respectively. The distinguishing behavior of the target pollutant removal in the investigated system was caused by the various water characteristics (i.e., DO and pH) and the different NOM used (Peldszus et al., 2004). The slight suppression of NOM in our study can be attributed to the following reasons. Firstly, NOM is rich in chromophores (Lyu et al., 2015), hence behaved competitively for UV light, which resulted in less UV light being absorbed by sulfite and the subsequent reduction of produced oxidative or reductive species. Moreover, several oxidative reactive species (i.e., ∙OH, 1O2, H2O2) were produced in the process of NOM photolysis, which may react quickly with reductive species such as eaq− (Lester et al., 2013) and reduce the proportion of eaq− used for MET degradation, thus causing an inhibition effect on MET removal. Nevertheless, some of the generated oxidative reactive species, such as ∙OH, have been demonstrated to be beneficial for MET removal in the oxygen-involved UV/sulfite processes. Consequently, the impact of NOM on MET removal during the investigated process is complicated.
MET degradation experiments in a real water matrix were also performed, and the results were portrayed in Figure 5. In general, MET degradation was partly restrained in surface water samples when compared to the previous studied synthetic matrix. Several reasons may account for this phenomenon. Firstly, the solution pH of two surface water samples were less than 9 (Supplementary Table S1), which may result in less sulfite exist in the form of SO32- and accordingly less eaq− generation. Secondly, NOM and inorganic ions were co-present in the surface water samples with certain extent (Supplementary Table S1). Their detrimental effect on target pollutants degradation in the UV/sulfite system has also been reported by other researchers (Cao et al., 2021b; Liu et al., 2022). Hence, MET degradation by the studied process in real water samples was impaired. Nevertheless, nearly 80% of MET was removed after 180 min irradiation, which suggested the studied oxygen-involved UV/sulfite process was prospective for MET-bearing wastewater treatment.
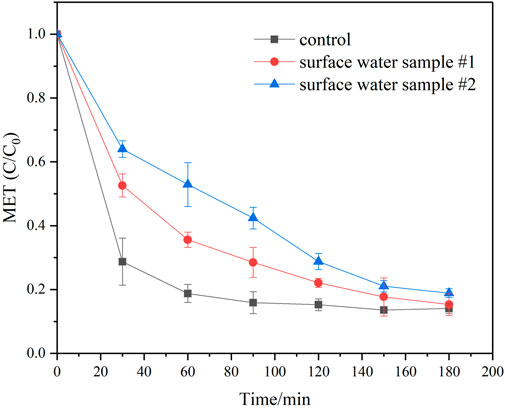
FIGURE 5. MET degradation in the real water matrix. Experimental conditions: [MET]0 = 2.5 mg/L, [Sulfite]0 = 10 mM, UV intensity = 100% I0.
3.8 MET degradation products
In order to investigate the degradation pathway of MET in the oxygen-involved UV/sulfite system, the degradation products were detected. Nitrite and ammonium ions were the dominant inorganic degradation products (Supplementary Figure S2), among which ammonium accounted for the majority. The calculation of nitrogen balance indicated most of the nitrogen (>80%) in MET molecule was transformed into inorganic nitrogen species. Due to the relatively low concentration and small molecule weight, the organic intermediates of MET degradation were difficult to be determined. Therefore, MET degradation experiment at a higher initial MET concentration (1 g/L) and longer reaction time (8 h) was conducted to better ascertain these organic intermediates, and the results were shown in Supplementary Table S5. Three organic N-containing products with lower molecule weight were detected with m/z at 125, 115, and 87, respectively. Referring to previous investigation (Badran et al., 2019; Carbuloni et al., 2020), their molecule structures were also given. The same degradation intermediates were also reported in another literature (Quintão et al., 2016), where MET degradation occurred in three oxidative reaction systems (i.e., sodium hypochlorite, ozonation, photocatalysis). A previous study by Trouillas et al. (2013) have explored the ∙OH induced oxidative degradation of MET, in which ∙OH was produced through water radiolysis reaction. There were totally four kinds of intermediates determined by using HPLC/MS, which included 4-amino-2-imino-1-methyl-1,2-dihydro-1,3,5-triazine (compound 2 in this study), methylbiguanide (compound 3 in this study), hydroperoxide of metformin and 2-amino-4-methylamino-1,3,5-triazine. ∙H abstraction, electron transfer and ∙OH addition were three main reaction pathways when MET was primarily attacked by ∙OH. The bond dissociation enthalpies at C8 and C9 in MET molecule were very close, which hinted the two methyl functional groups were equal targets for ∙OH attacking, and further causing MET converted to methylbiguanide. In addtion, the production of methylbiguanide was reported primarily occurred in the alkaline pH value, which was in good agreement with the reaction condition of our study (pH 9). Additionally, the bio-toxicity of the testing solution during the target pollutant degradation was monitored and presented in Figure 6. The bio-toxicity of the testing solution gradually declined along with MET degradation in general. Overall, the oxygen-involved UV/sulfite process exhibited a remarkable denitrification capacity in MET.
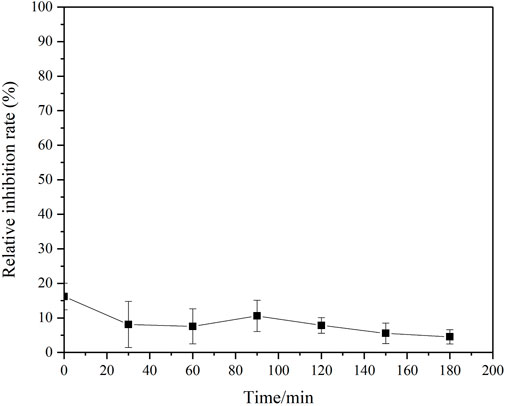
FIGURE 6. Bio-toxicity change of the testing solution during MET degradation process. Experimental conditions: [MET]0 = 10 mg/L, [Sulfite]0 = 10 mM, UV intensity = 100% I0.
4 Conclusion
This work explored MET degradation in the oxygen-involved UV/sulfite process under different experimental parameters. A relatively higher degradation efficiency and kobs were achieved in the investigated process, where SO4∙–, ∙OH, eaq− and ∙H were manifested the major contributors to MET removal. Alkaline conditions (pH ≥ 9) were favorable to the degradation of MET. The MET degradation efficiency was positively correlated with the incident UV intensity, sulfite dosage and reaction temperature, and negatively correlated with the initial MET concentration. The co-existed bicarbonate and FA slightly suppressed MET degradation. The oxygen-involved UV/sulfite system exhibited an extraordinary denitrification capacity in MET. Nitrite, ammonium ions and three kinds of N-containing organic compounds were detected as the dominant intermediates for MET degradation. This study offered a novel approach for treating emerging contaminants.
Data availability statement
The original contributions presented in the study are included in the article/Supplementary Material, further inquiries can be directed to the corresponding author.
Author contributions
YG Conceptualization, Methodology, Investigation, Software, writing—original draft preparation, Writing—review and editing, Validation, Funding acquisition. YZ Conceptualization, Methodology, Data curation. CJ Conceptualization, Writing—review and editing, Funding acquisition ZD Conceptualization, Methodology, Investigation, Data curation, Writing—review and editing, Supervision. Funding acquisition, Visualization. XB Investigation, Validation, Data curation All authors have read and agreed to the published version of the manuscript.
Funding
We thank the National Natural Science Foundation of China (42107396, 52170007), Shenzhen Science and Technology Program (JCYJ20220531094416037), Shenzhen Sustainable Development Project (KCXFZ202002011006362, KCXFZ20201221173413037) for financial support of the present work.
Conflict of interest
The authors declare that the research was conducted in the absence of any commercial or financial relationships that could be construed as a potential conflict of interest.
Publisher’s note
All claims expressed in this article are solely those of the authors and do not necessarily represent those of their affiliated organizations, or those of the publisher, the editors and the reviewers. Any product that may be evaluated in this article, or claim that may be made by its manufacturer, is not guaranteed or endorsed by the publisher.
Supplementary material
The Supplementary Material for this article can be found online at: https://www.frontiersin.org/articles/10.3389/fenvs.2022.1071963/full#supplementary-material
References
Ambrosio-Albuquerque, E. P., Cusioli, L. F., Bergamasco, R., Gigliolli, A. A., Lupepsa, L., and Paupitz, B. R. (2021). Metformin environmental exposure: A systematic review. Environ. Toxi. Col. Phar. Metformin Environ. Expo. A Syst. Rev. 83, 103588.
Badran, I., Manasrah, A. D., and Nassar, N. N. (2019). A combined experimental and density functional theory study of metformin oxy-cracking for pharmaceutical wastewater treatment. Rsc. Adv. 9, 13403–13413. doi:10.1039/c9ra01641d
Buxton, G. V., Greenstock, C. L., Helman, W. P., and Ross, A. B. (1988). Critical review of rate constants for reactions of hydrated electrons, hydrogen atoms and hydroxyl radicals (∙OH/∙O-) in aqueous solution. J. Phys. Chem. Ref. Data. 17 (2), 513–886. doi:10.1063/1.555805
Cao, Y., Qiu, W., Li, J., Jiang, J., and Pan, S. Y. (2021). Review on UV/sulfite process for water and wastewater treatments in the presence or absence of O2. Sci. Total. Environ. 765, 142762. doi:10.1016/j.scitotenv.2020.142762
Cao, Y., Qiu, W., Li, J., Zhao, Y. M., Jiang, J., and Pang, S. Y. (2021). Sulfite enhanced transformation of iopamidol by UV photolysis in the presence of oxygen: Role of oxysulfur radicals. Water. Res. 189, 116625. doi:10.1016/j.watres.2020.116625
Carbuloni, C. F., Savoia, J. E., Santos, J. S., Pereira, C. A., Marques, R. G., Ribeiro, V. A., et al. (2020). Degradation of metformin in water by TiO2–ZrO2 photocatalysis. J. Environ. Manage. 262, 110347. doi:10.1016/j.jenvman.2020.110347
Chen, L., Xue, Y. F., Luo, T., Wu, F., and Alshawabkeh, A. N. (2021). Electrolysis-assisted UV/sulfite oxidation for water treatment with automatic adjustments of solution pH and dissolved oxygen. Chem. Eng. J. 403, 126278. doi:10.1016/j.cej.2020.126278
Crago, J., Bui, C., Grewal, S., and Schlenk, D. (2016). Age-dependent effects in fathead minnows from the anti-diabetic drug metformin. Gen. Comp. Endocr. 232, 185–190. doi:10.1016/j.ygcen.2015.12.030
Cruz, N. D., Pulgarin, C., Giménez, J., Esplugas, S., Grandjean, D., and Alencastro, L. F. (2012). Degradation of 32 emergent contaminants by UV and neutral photo-fenton in domestic wastewater effluent previously treated by activated sludge. Water. Res. 46, 1947–1957. doi:10.1016/j.watres.2012.01.014
Deister, U., and Warneck, P. (1990). Photooxidation of SO32- in aqueous solution. J. Phys. Chem. 94, 2191–2198. doi:10.1021/j100368a084
Ding, Y. C., Han, J. L., Feng, H. J., Liang, Y. X., Jiang, W. L., Liu, S, H., et al. (2022). Source prevention of halogenated antibiotic resistance genes proliferation: UV/sulfite advanced reduction process achieved accurate and efficient elimination of florfenicol antibacterial activity. Sci. Total. Environ. 849, 157844. doi:10.1016/j.scitotenv.2022.157844
Fu, J., Zhang, Z. M., Tang, J. Y., Zeng, Q. F., An, S. Q., and Zhu, H. L. (2010). Photoreduction of reactive brilliant red X-3B by ultraviolet irradiation/potassium borohydride/sodium bisulfite. J. Environ. Eng. 136, 1314–1319. doi:10.1061/(asce)ee.1943-7870.0000251
Gaffney, V. J., Cardoso, V. V., Cardoso, E., Teixeira, A. P., Martins, J., Benoliel, M. J., et al. (2017). Occurrence and behaviour of pharmaceutical compounds in a Portuguese wastewater treatment plant: Removal efficiency through conventional treatment processes. Environ. Sci. Pollut. Res. 24, 14717–14734. doi:10.1007/s11356-017-9012-7
George, N. K., Wols, B. A., Santoro, D., Borboundakis, M., Bell, K., and Gernjak, W. (2022). A novel approach to interpret quasi-collimated beam results to support design and scale-up of vacuum UV based AOPs. J. Water. Res. 17, 100158. doi:10.1016/j.wroa.2022.100158
Gu, Y. R., Dong, W. Y., Luo, C., and Liu, T. Z. (2016). Efficient reductive decomposition of perfluorooctanesulfonate in a high photon flux UV/sulfite system. Environ. Sci. Technol. 50, 10554–10561. doi:10.1021/acs.est.6b03261
Gu, Y. R., Qi, Han., Dong, Z. J., Zhu, J. X., Jiang, C. C., and Qi, J. K. (2022). Enhanced degradation and deiodination of diatrizoate in a sulfite mediated photolytic system: Performance, products and possible pathways. J. Hazard. Maters. Adv. 8, 100144.
Huang, L., Dong, W. B., and Hou, H. Q. (2007). Investigation of the reactivity of hydrated electron toward perfluorinated carboxylates by laser flash photolysis. Chem. Phys. Lett. 436, 124–128. doi:10.1016/j.cplett.2007.01.037
Huang, Y., Han, C., Liu, Y. Q., Nadagouda, M. N., Machala, L., O’Shea, K. E., et al. (2018). Degradation of atrazine by ZnxCu1−xFe2O4 nanomaterial-catalyzed sulfite under UV-vis light irradiation: Green strategy to generate SO4. J. Appl. Catal. B 221, 380–392. doi:10.1016/j.apcatb.2017.09.001
Karimiana, S., Moussavia, G., Fanaeia, F., Mohammadia, S., Shekoohiyana, S., and Giannakis, S. (2020). Shedding light on the catalytic synergies between Fe(II) and PMS in vacuum UV (VUV/Fe/PMS) photoreactors for accelerated elimination of pharmaceuticals: The case of metformin. Chem. Eng. J. 400, 125896. doi:10.1016/j.cej.2020.125896
Lester, Y., Sharpless, C. M., Mamane, H., and Linden, K. G. (2013). Production of photo-oxidants by dissolved organic matter during UV water treatment. Environ. Sci. Technol. 47, 11726–11733. doi:10.1021/es402879x
Li, G., Wang, C., Yan, Y. P., Yan, X. R., Li, W. T., Feng, X. H., et al. (2020). Highly enhanced degradation of organic pollutants in hematite/sulfite/photo system. Chem. Eng. J. 386, 124007. doi:10.1016/j.cej.2019.124007
Li, X. C., Fang, J. Y., Liu, G. F., Zhang, S. J., Pan, B. C., and Ma, J. (2014). Kinetics and efficiency of the hydrated electroninduced dehalogenation by the sulfite/UV process. Water. Res. 62, 220–228. doi:10.1016/j.watres.2014.05.051
Li, X. C., Ma, J., Liu, G. F., Fang, J. Y., Yue, S. Y., Guan, Y. H., et al. (2012). Efficient reductive dechlorination of monochloroacetic acid by sulfite/UV process. Environ. Sci. Technol. 46, 7342–7349. doi:10.1021/es3008535
Liu, S. H., Han, J. L., Ding, Y. C., Gao, X. X., Chen, H. Y., Wang, H. C., et al. (2022). Advanced reduction process to achieve efficient degradation of pyridine. Chemosphere 287, 132240. doi:10.1016/j.chemosphere.2021.132240
Liu, X., Vellanki, B. P., Batchelor, B., and Abdel-Wahab, A. (2014). Degradation of 1, 2-dichloroethane with advanced reduction processes (ARPs): Effects of process variables and mechanisms. Chem. Eng. J. 237, 300–307. doi:10.1016/j.cej.2013.10.037
Liu, X. W., Zhang, T. Q., Wang, L. L., Shao, Y., and Fang, L. (2015). Hydrated electron-based degradation of atenolol in aqueous solution. Chem. Eng. J. 260, 740–748. doi:10.1016/j.cej.2014.08.109
Lyu, X. J., Li, W. W., Lam, P. K. S., and Yu, H. Q. (2015). Photodegradation of perfluorooctane sulfonate in environmental matrices. Sep.Purif. Technol. 151 (13), 172–176. doi:10.1016/j.seppur.2015.07.049
Maćerak, A. L., Kerkez, Đ., Bečelić-Tomin, M., Pilipović, D. T., Kulić, A., Jokić, J., et al. (2018). Removal of diclofenac and metformin from water in laboratory photo reactor. Digit. Publ. Inst. Proc. 2 (20), 1288.
Milh, H., Yu, X. Y., Cabooter, D., and Dewil, R. (2021). Degradation of ciprofloxacin using UV-based advanced removal processes: Comparison of persulfate-based advanced oxidation and sulfite-based advanced reduction processes. Sci. Total. Environ. 764, 144510. doi:10.1016/j.scitotenv.2020.144510
Neamţu, M., Grandjean, D., Sienkiewicz, A., Faucheur, S. L., Slaveykova, V., Colmenares, J. J., et al. (2014). Degradation of eight relevant micropollutants in different water matrices by neutral photo-Fenton process under UV254 and simulated solar light irradiation - a comparative study. Appl. Catal. B Environ. 158-159, 30–37.
Nezar, S., and Laoufi, N. A. (2018). Electron acceptors effect on photocatalytic degradation of metformin under sunlight irradiation. Sol. Energy. 164, 267–275. doi:10.1016/j.solener.2018.02.065
Niemuth, N. J., and Klaper, R. D. (2015). Emerging wastewater contaminant metformin causes intersex and reduced fecundity in fish. Chemosphere 135, 38–45. doi:10.1016/j.chemosphere.2015.03.060
Peldszus, S., Andrews, S. A., Souza, R., Smith, F., Douglas, I., Bolton, J., et al. (2004). Effect of medium-pressure UV irradiation on bromate concentrations in drinking water, a pilot-scale study. Water Res. 38, 211–217. doi:10.1016/j.watres.2003.09.010
quality, Water (2007). Determination of the inhibitory effect of water samples on the light emission of Vibrio fifischeri (Luminescent bacteria test)-Part 3: Method using freeze-dried bacteria. Geneva, Switzerland: International Organization for Standardization. ISO 11348-3.
Quintão, F. J., Freitas, J. R., Machado, C. F., Aquino, S. F., Silva, S. Q., and Afonso, R. J. (2016). Characterization of metformin by-products under photolysis, photocatalysis, ozonation and chlorination by high-performance liquid chromatography coupled to high-resolution mass spectrometry. Rapid. Commun. Mass. Sp. 30 (21), 2360–2368.
Scheurer, M., Sacher, F., and Brauch, H. J. (2009). Occurrence of the antidiabetic drug metformin in sewage and surface waters in Germany. J. Environ. Monit. 11, 1608–1613. doi:10.1039/b909311g
Shah, N. S., Khan, J. A., Nawaz, S., and Khan, H. M. (2014). Role of aqueous electron and hydroxyl radical in the removal of endosulfan from aqueous solution using gamma irradiation. J. Hazard. Mat. 278, 40–48. doi:10.1016/j.jhazmat.2014.05.073
Song, Z., Tang, H. Q., Wang, N., and Zhu, L. H. (2013). Reductive defluorination of perfluorooctanoic acid by hydrated electrons in a sulfite-mediated UV photochemical system. J. Hazard. Mat. 262, 332–338. doi:10.1016/j.jhazmat.2013.08.059
Trouillas, P., Marchetti, C., Bonnefont-Rousselot, D., Lazzaroni, R., Jore, D., Gardès-Albert, M., et al. (2013). Mechanism of one-electron oxidation of metformin in aqueous solution. Phys. Chem. Chem. Phys. 15, 9871–9878. doi:10.1039/c3cp50602a
Vecitis, C. D., Park, H., Cheng, J., Mader, B. T., and Hoffmann, M. R. (2009). Treatment technologies for aqueous perfluorooctanesulfonate (PFOS) and perfluorooctanoate (PFOA). Front. Environ. Sci. Engin. China. 3 (2), 129–151. doi:10.1007/s11783-009-0022-7
Vellanki, B. P., Batchelor, B., and Abdel-Wahab, A. (2013). Advanced reduction processes: A new class of treatment processes. Enivron. Eng. Sci. 30 (5), 264–271.
Xiao, Q., Ren, Y. F., and Yu, S. L. (2017). Pilot study on bromate reduction from drinking water by UV/sulfite systems: Economic cost comparisons, effects of environmental parameters and mechanisms. Chem. Eng. J. 330, 1203–1210. doi:10.1016/j.cej.2017.08.071
Yang, Y., Jiang, J., Lu, X., Ma, J., and Liu, Y. (2015). Production of sulfate radical and hydroxyl radical by reaction of ozone with peroxymonosulfate: A novel advanced oxidation process. Environ. Sci. Technol. 49, 7330–7339. doi:10.1021/es506362e
Yazdanbakhsh, A., Eslami, A., Moussavi, G., and Rafiee, M. (2018). Photo-assisted degradation of 2, 4, 6-trichlorophenol by an advanced reduction process based on sulfite anion radical: Degradation, dechlorination and mineralizationPhoto-assisted degradation of 2, 4, 6-trichlorophenol by an advanced reduction process based on sulfite anion radical: Degradation, dechlorination and mineralization. Chemosphere 191, 156–165. doi:10.1016/j.chemosphere.2017.10.023
Yoon, S., Han, D. S., Liu, X., Batchelor, B., and Abdel-Wahab, A. (2014). Degradation of 1, 2-dichloroethane using advanced reduction processes. J. Environ. Chem. Eng. 2, 731–737. doi:10.1016/j.jece.2013.11.013
Yu, H., Yang, R. T., Wu, J. H., Wang, S. Y., Qin, X. Y., Wu, T., et al. (2022). Association of metformin and depression in patients with type 2 diabetes. J. Affect. Disord. 318, 380–385. doi:10.1016/j.jad.2022.09.015
Zhang, Q., Li, C. L., and Li, T. (2013). Rapid photocatalytic decolorization of methylene blue using high photon flux UV/TiO2/H2O2 process. Chem. Eng. J. 217, 407–413. doi:10.1016/j.cej.2012.11.106
Zhang, W. Q., Huang, T., Ren, Y., Yang, S., Zhao, X. Y., Yuan, M. S., et al. (2022). A multifunctional chitosan composite aerogel for PPCPs adsorption. Carbohydr. Polym. 298, 120102. doi:10.1016/j.carbpol.2022.120102
Zhou, L., Ferronato, C., Chovelon, J. M., Sleiman, M., and Richard, C. (2017). Investigations of diatrizoate degradation by photo-activated persulfate. Chem. Eng. J. 311, 28–36. doi:10.1016/j.cej.2016.11.066
Keywords: sulfite, UV, oxygen, metformin, AOPs
Citation: Gu Y, Zhang Y, Jiang C, Dong Z and Bai X (2023) Efficient metformin transformation in sulfite/UV process co-present with oxygen. Front. Environ. Sci. 10:1071963. doi: 10.3389/fenvs.2022.1071963
Received: 17 October 2022; Accepted: 29 December 2022;
Published: 10 January 2023.
Edited by:
Tao Zhang, China Agricultural University, ChinaReviewed by:
Changyin Zhu, Nanjing University, ChinaOmar Mohammad Atta, Al-Azhar University, Egypt
Chengcheng Xia, Sichuan University, China
Tongzhou Liu, Harbin Institute of Technology, China
Copyright © 2023 Gu, Zhang, Jiang, Dong and Bai. This is an open-access article distributed under the terms of the Creative Commons Attribution License (CC BY). The use, distribution or reproduction in other forums is permitted, provided the original author(s) and the copyright owner(s) are credited and that the original publication in this journal is cited, in accordance with accepted academic practice. No use, distribution or reproduction is permitted which does not comply with these terms.
*Correspondence: Zijun Dong, ZG9uZ3ppanVuQHN6dS5lZHUuY24=