- 1College of Water Conservancy, Shenyang Agricultural University/Liaoning Panjin Wetland Ecosystem National Observation and Research Station, Shenyang, Liaoning, China
- 2Liaoning Shuangtai Estuary Wetland Ecosystem Research Station, Panjin, Liaoning, China
- 3Yellow River Engineering Consulting Co., Ltd., Zhengzhou, Henan, China
Estuarine tidal flats are located in the land-sea crisscross-sensitive zone. The degraded and shrinking state of major tidal flat landscapes weakens their ecological role in maintaining the safety of coastal wetlands in the world. However, little attention has been paid to tidal flat landscape evolution and ecological risks due to the tidal flats being located in the periphery of estuarine wetlands. This study was conducted in the tidal flats of the Liaohe estuary wetlands. The GIS spatial analysis and a transition matrix were combined to explore the tidal flat landscape evolution characteristics. The ecological risk faced by the tidal flats was evaluated by a landscape ecological risk model constructed by landscape indexes. The key driving factors and threshold values that affect the ecological risks were clarified using geographical detectors and regression analysis methods. The results showed that the tidal flats mainly evolved into a natural wetland before 2000 and rapidly changed into an artificial landscape between 2000 and 2010; the proportion of artificial landscapes then began to decline after 2010. Over the past 30 years, the landscape ecological risk in this area has generally been at a medium risk level. The ecological risk index showed a decreasing trend with the increases in landscape diversity. The low-risk artificial plaques have gradually replaced high-risk and medium-risk plaques of exposed tidal flats and natural wetlands. The study showed that human development activities were the key factors affecting the landscape ecological risks of the tidal flats. River sediment discharge was the key natural factor in maintaining the landscape ecological risk at a stable level. Therefore, the human development activities should be controlled within an appropriate limit, and the material supply of the upstream basin should be guaranteed to maintain the stability of the tidal flat landscape form in the protection and utilization of estuarine tidal flats in the future.
1 Introduction
Estuary tidal flats are important land–sea material exchange belts, and are located at the outermost periphery of coastal estuarine wetlands (Yao, 2013; Li et al., 2021a). Tidal flats play an important ecological role in the security of estuarine wetland ecosystems, shoreline stability, reserving coastal land, and conserving biodiversity (Kim and Ryu, 2020; Bao and Gao, 2021; Costanza et al., 2021; Jackson et al., 2021). However, 16% of the world’s tidal flats were lost between 1984 and 2016 due to natural and human factors such as watershed climates, water conservancy construction, and industrial and agricultural production weakening the buffering capacity of tidal flats as a defense against coastal erosion (Murray et al., 2019; Liu et al., 2020). This phenomenon leads to the structural degradation of coastal wetland ecosystems and the reduction of ecological functions (Sun et al., 2017; Cui et al., 2021), which is not conducive to the sustainable development of coastal cities (Ma et al., 2014). Thus, of importance to maintaining the stability of estuarine wetland shorelines and wetland ecological security is exploring the spatial evolution of, and ecological risks to, tidal flats.
The temporal and spatial evolution of wetlands has been the result of changes in regional landscape patterns (Jiang et al., 2017; Murray et al., 2019). Landscape patterns reflect a combination of different ecological processes, climate, land-use changes, and biodiversity changes (Zhan et al., 2019; Zhang et al., 2021). Studies have shown that wetland landscape patterns depend on the geographical distribution and composition of wetland resources, and are closely related to the robustness to disturbance, resilience, stability, and biodiversity of wetland ecosystems (Fu, 1995). External disturbance is an important ecological and landscape-scale process, and a key driving force affecting landscape structure and spatial organization (Li et al., 2021b; Duan et al., 2022). Nearly three-quarters of the global ecological landscape has been altered by human disturbance over the past millennium (Dadashpoor et al., 2019; Grafius et al., 2020; Winkler et al., 2021). For example, the transfer rate of the tidal flats landscape around the Nile Estuary to the ocean waters reaches 30 m/a (Zucker et al., 2021), and other tidal flats landscapes such as the Mississippi, Ebro, and Colorado have been showing a trend in shifting to marine waters due to the intensification of external disturbances (Walling, 2006; Xu et al., 2019). In the coastal areas of China, almost 53% of the tidal flats along the coast were lost due to land reclamation (Zhou et al., 2020). In particular from 2000 to 2010, the area of coastal tidal flats transformed into such artificial landscapes as construction land and cultivated land reached 320,000 hm2 (Yu and Zhang, 2020), and the area of tidal flats was reduced by 25.2% (Ma et al., 2019). Changes in natural factors, such as river conditions, estuary size, and estuary hydrodynamic environments have also had an important impact on the evolution of coastal tidal flats landscapes (Paul et al., 2002; Strayer et al., 2003). For example, 5.2 km2 of the coastal tidal flats landscape in South Korea transformed into marine waters due to the reduction of river sediment input from 2013 to 2018 (Kim et al., 2021). The tidal flats landscape of the Yangtze River estuary has also been continuously turned into marine waters due to the decline of the annual sediment discharge from the Yangtze River after 2000 (Shen et al., 2013; Liu et al., 2020). In addition to Qingbacha, the tidal flats landscapes of the Yellow River estuary have been transforming into marine waters due to the reduction of river runoff and thus sediment discharge (Wang et al., 2017).
Ongoing landscape changes have caused a range of environmental and ecological problems globally, including habitat destruction, soil degradation, primary productivity decline, and biodiversity loss (Imhoff et al., 2004; Guida Johnson and Zuleta, 2013; Zhang et al., 2016; Zhou et al., 2018). Especially in recent decades, the dominant landscape of coastal wetlands has been gradually replaced by artificial landscapes with the expansion of construction land, resulting in regional ecosystem changes and the formation of more scattered and complex landscape patterns (Dadashpoor et al., 2019; Roussel and Alexandre, 2021). This makes landscape fragmentation a common feature of the evolution of coastal tidal flats landscapes (Plexida et al., 2014; Pace et al., 2017; Skilleter et al., 2017). These problems have greatly curtailed the ability of wetland ecosystems to provide ecological services for humans, and have brought serious threats to the sustainable development of human society (Jiyuan et al., 2010; Zhou et al., 2018). Therefore, it is crucial to assess the ecological risks arising from the current evolution of wetland landscapes correctly to alleviate the conflict between regional sustainable development and environmental protection. Landscape ecological risk is a landscape pattern-based perspective that reflects the possible adverse effects of human activities or natural conditions on landscape composition, structure, function, and processes comprehensively (McEachran et al., 2018). It can characterize comprehensive ecological risks from multiple stressors through landscape features, and provides an ecological risk mitigation pathway based on landscape pattern optimization (Goussen et al., 2016; Wang et al., 2021). Landscape ecological risk analyzes the impact of ecological risk on landscape composition and function, emphasizing the spatiotemporal heterogeneity of that risk (Wang et al., 2021; Zhang et al., 2022). Landscape ecological risk is used in watersheds, coastal wetlands, and for the ecological risk assessment of ecologically fragile areas and cities. However, there is still a lack of research based on ecological risk assessments of tidal flats landscapes around estuarine wetlands, and research on landscape patterns, ecological risks, and their driving factors is limited.
In summary, the landscape patterns of estuarine wetlands are in continuous evolution under the influence of human or natural environment factors, which affects the ecological security and ecological functions of regional wetlands. Relevant studies mainly focus on the ecological degradation and restoration of wetlands within tidal flats, ignoring that tidal flats are an important peripheral defense line of estuarine wetlands, and thus there is a lack of understanding of the ecological role of tidal flats on coastal wetlands security. Therefore, since findings from the research to date on the evolution of estuarine tidal flats landscapes are unclear, the present study was conducted at the tidal flats in the Liaohe estuary wetlands (abbreviated as LEW), representing a typical international estuarine wetland, to achieve the following objectives: 1) Analyze the tidal flats landscape pattern around the estuary wetland; 2) quantitatively assess the ecological risks faced by estuarine tidal flats; 3) clarify the key driving forces and thresholds of ecological risks affecting tidal flats landscapes, and provide a theoretical basis for the protection and utilization of estuarine wetlands. The results will provide a theoretical basis for the protection and utilization of estuarine wetlands.
2 Materials and methods
2.1 Study area
This study was conducted on the tidal flats at the boundary between the land and sea of the LEW in Panjin City, northern China (Figure 1). This area is an important zone of material exchange in Bohai Bay, which is both boundaries of the wetlands and the city. The tidal flat area identified in this study is the area before the boundary of the vegetation zone and the boundary of the ocean low tide in the LEW. To discover the evolution of the estuary tidal flats landscape, the study area was chosen as between the upper and lower boundaries of the tidal flats of the LEW in 1985 and 2017. The area of the tidal flats was 223.30 km2 in 2017. The main material source of the continuous tidal flats deposition in the LEW is the sediment discharge of the Liaohe River. The study area was in a warm, temperate continental, semi-humid monsoon climate zone with four distinct seasons, including rain and high temperatures in the same season, and also dry and cold weather in the same period. The annual average temperature was 8.40°C, the annual average precipitation was 623.20 mm, and the annual average evaporation was 1669.60 mm.
2.2 Data sources
The main indicators and data sources involved in this study are shown in Table 1.
2.3 Landscape ecological risk assessment
The main methods and technical processes used in the current research to explore the ecological risks to tidal flat landscapes were shown in Figure 2. The research was completed through the following steps: step 1, obtain remote sensing images of the study area, and apply GIS spatial analysis and a transition matrix comprehensively to explore landscape evolution characteristics; step 2, calculate and analyze the relevant indices based on the results of landscape interpretation: 1) calculate the landscape vulnerability index (LVI) through sensitivity analysis; 2) calculate the landscape disturbance index (LDI) using Fragstats_V4.2 (Chen et al., 2014; Plexida et al., 2014; Wang et al., 2020); 3) construct a landscape ecological risk model based on integrating the two parameters, and evaluate the ecological risk of the study area; step 3, explore the key driving factors that affect ecological risk. Specifics about the methods of analysis and calculations undertaken are presented in the Supplementary Materials.
To finely evaluate the ecological risks to this regional landscape, this study established a 500 × 500 m grid according to the spatial distribution characteristics of the landscape patches, and divided the study area into 2,963 grid evaluation units. The values of the landscape disturbance index (LDI) and the landscape vulnerability index (LVI) for each evaluation unit were calculated and then the landscape ecological risk index (ERIk) of the corresponding grid assessment unit was calculated using the following formula.
In the formula, ERIk is the landscape ecological risk index of the kth evaluation unit; LDIi is the landscape disturbance index; LVIi is the landscape vulnerability index; n is the number of landscape types; Aki is the area of the ith landscape type of the kth evaluation unit; Ak is the total area of the kth evaluation unit. The larger the ERIk value, the higher the ecological risk of the corresponding grid.
To analyze the spatial and temporal distribution differences of the ERI, the values of ERI were divided into low ecological risk areas, medium ecological risk areas, and high ecological risk areas using the Jenks natural breaks classification method (Chen et al., 2013; Gong et al., 2021). Then, the spatial distribution characteristics of the ERI were analyzed using the kriging interpolation method, and the spatial distribution changes of the number of high-risk, medium-risk, and low-risk grids were statistically analyzed.
2.4 Driving factors analysis
According to the current environmental status of the tidal flats in the LEW, the eight main driving factors of patch density (PD), landscape diversity (SHDI), artificial disturbance intensity index (LHAI), Gross Domestic Product (GDP), Gross Value of Agricultural Production (GVAP), mean annual flow, flow during the flood season, and annual sediment discharge were screened out by principal component analysis based on a comprehensive analysis of the 14 impact indicators shown in Table 1. Among them, the LHAI, GDP, GVAP, PD, and SHDI in the first principal component had factor loads greater than 0.8, while the mean annual flow, flow during the flood season, and annual sediment discharge in the second principal component had factor loads greater than 0.8.
Based on the main initiating factors screened, the factor detector in the geographic detector (Wang et al., 2010, 2016) was used to analyze the effect of the main driving factors affecting the ERI on the tidal flats landscape in the LEW, based on the main initiating factors that were screened. Then, the q value was used to judge and explain the driving force of each index with regards the range of change of the ERI. Regression analysis was used to explore the relationship between each driving factor and the ERI, and to analyze the effect of different driving factors on the ecological risk to the regional landscape.
3 Results
3.1 The evolution of the tidal flats landscape
The tidal flats of the LEW has been silting up to the sea gradually over the past 30 years, meanwhile, the tidal flats landscape has evolved into natural wetlands and artificial landscapes such as roads, towns, and aquaculture (Figure 3). From 1985 to 2017, 16.11% of the natural waters gradually evolved into tidal flats in the study area. 17.66% of the tidal flats have evolved into natural wetlands, of which 15.44% and 2.22% have evolved into reed and Suaeda salsa wetlands respectively; 24.47% of the tidal flats have been developed into mariculture, and 6.85% have been developed into paddy fields. 11.08% evolved into other artificial landscapes. In terms of artificial landscape, 24.47%, 6.85%, and 11.08% of the tidal flats were developed into mariculture, paddy fields, and other artificial landscapes, respectively. Of the natural wetlands, 68.72% were developed into paddy fields, of which 70.71% and 11.01% of the reed and Suaeda salsa wetlands were transformed into paddy fields, respectively. In addition, 7.38% of the natural wetlands were developed for aquaculture, of which 13.40% and 7.17% of the Suaeda salsa and reed wetlands were developed for aquaculture, respectively.
The characteristics of landscape change can be roughly divided into the natural evolution period (1985–2000), the artificial landscape rapid increase period (2000–2010), and the ecological restoration period (2010–2017). In terms of the period 1985 to 2000, the tidal flats in the LEW mainly turned to Suaeda salsa and reed wetlands by natural succession, and the average proportion of artificial landscapes such as marine aquaculture and roads was 2.30%. The proportion of natural wetlands being developed into paddy fields increased year by year. From 2000 to 2010, the proportion of artificial landscapes increased rapidly. 6.40% of tidal flats turned into natural wetlands, 13.72% was developed for mariculture, and only 0.2% was turned into other artificial landscapes. Of the natural wetlands, 7.18% were turned into such artificial landscapes such as for mariculture, roads, and other artificial landscapes. During this period, the area that can be developed for mariculture reached its limit, and the scale of mariculture accounted for 13.18% of the study area, which was the largest artificial landscape. At this time, the tidal flats were in a state of erosion, and 35.06% of the area was transformed into natural water, while 57.82% and 14.79% of Suaeda salsa and reed wetlands, respectively, were degraded into the exposed tidal flats. From 2010 to 2017, 28.29% of the tidal flats were transformed into natural wetlands, of which 18.65% were transformed into Suaeda salsa wetlands. The proportion of tidal flats developed for aquaculture was reduced to 1.17%, and 5.47% of the tidal flats were developed. In paddy fields, the proportion of other artificial landscapes was reduced to 0.83% during the period, and 9.72% of the aquaculture landscapes were cleared and restored to natural wetlands, of which 8.41% of aquaculture was transformed into Suaeda salsa wetlands. The results show that the implementation of the “Environmental Grayscale Project around the Bohai Bay” has promoted improvement of the landscape pattern in the study area, and the increased rate of the artificial landscape has begun to decline. However, during this period, the tidal flats were still eroded, shrinking by 6.84%, and 5.84% of Suaeda salsa degenerated into exposed tidal flats.
In general, the proportion of tidal flats transformed into such artificial landscapes as paddy fields and aquaculture between 1985 and 2017 was greater than that of natural wetlands such as reeds and Suaeda salsa. The regional tidal flats landscape generally showed a tendency to transform into an artificial landscape, in which, in 2017, the average increase in rate of mariculture reached 11.39%, the proportion of mariculture reached 11.55%, and the natural wetlands accounted for 8.02%. The reed and Suaeda salsa natural wetlands in the region were mainly developed into paddy fields, and the transfer rate increased to 7.60% in 2017.
3.2 The ecological risk of the tidal flats landscape
The tidal flats of the estuary have evolved into salt marsh wetlands and reed wetlands through the gradual but continuous process of silting, and swelling towards the sea. The landscape of the estuary has evolved from a single tidal flats landscape into a variety of wetlands. The landscape diversity has increased, but with the impact of human activities, the evolution of the tidal flats has gradually been replaced by the artificial landscape. The ecological risk faced by the regional landscape has changed with the evolution of the landscape. The evaluation results of ecological risk in the study area over the past 30 years are shown in Figure 4.
The change of the ERI reflects the superposition effect of the changes of various types of landscape utilization in the region (Ran et al., 2022). With the evolution of landscape patterns, the spatial distribution of the ERI in the study area was different (Figure 4). The ERI fluctuated with changes in the distribution of exposed tidal flats, with high ecological sensitivity. From 1985 to 2017, the high ecological risk zones were mainly distributed in the exposed tidal flats located outside of the LEW; the medium ecological risk zones were mainly distributed in the largest natural waters; the low-risk zones were mainly distributed in the artificial landscapes with strong anti-interference capacity, and the low-risk zones increased to a certain extent with the increase in landscape diversity. This demonstrates that the increase of regional landscape types can alleviate ecological risk to a certain extent.
From 1985 to 1990, the high ecological risk and medium ecological risk zones dominated the study area, with a small proportion of low-risk zones. This shows that the regional landscape type was dominated by exposed tidal flats at the beginning of the research period. The overall landscape type also had high ecological sensitivity and thus the study area suffered from high landscape ecological risk. After 1990, as the tidal flats silted up to the sea, the areas of high ecological risk increased. From 1995 to 2005, the high ecological risk areas increased, spreading from the periphery to the inside of the study area, and the value of the ERI increased. The low-risk areas were mainly in mariculture, paddy fields, and reservoir landscapes, and the medium-risk areas decreased. Since 2005, the natural water landscape has evolved into medium ecological risk zones, indicating that the continuous strengthening of artificial developments has increased the ecological risk of natural waters. By 2010, the area of high ecological risk was decreasing, and mainly distributed in the peripheral areas of the tidal flats. The low ecological risk zones were mainly distributed in artificial landscapes with strong robustness against interference such as paddy fields, mariculture, and reservoirs. Since 2010, the spatial distribution of the ERI has not changed much, the area of high-risk zones has decreased, and the medium-risk and low-risk zones have not changed significantly. The area of high-risk zones increased by 2017, however, which may be related to the large-scale degradation of salt marsh wetlands caused by the disconnection of tidal flats waterways which in turn were caused by tourism developments from 2015 to 2017.
Considering the changes in the spatial distribution of the ERI in the study area from 1985 to 2017, in the process of natural succession of the tidal flats from a single landscape to a natural wetland landscape, with the increase of landscape diversity, the ERI decreased. Later in this period, with the increase in the proportion of artificial landscapes and the influence of tidal flats erosion, the ERI showed a fluctuating trend of increasing and decreasing. Overall, the ecological risk of the tidal flats landscape in the LEW has been at a medium-risk level over the past 30 years, the high-risk and medium-risk zones have declined to some extent, and the low-risk zones have been slowly increasing. The results of this study have showed that the stability of the estuarine wetland landscape can be improved when the regional landscape evolved from a single exposed tidal flats landscape to a diverse landscape structure. The ability of ecologically fragile areas to resist ecological risks also improved. This landscape evolution improves its ability to resist ecological risks in ecologically fragile areas.
3.3 The driving factors affecting the ecological risk of the tidal flats
3.3.1 Screening of key factors
The staged comprehensive analysis by the factor detection method (Figure 5) showed that the order of explanatory power of the driving factors affecting the changes of the tidal flats ERI in the LEW was PD > SHDI > LHAI > GDP > sediment discharge > GVAP > mean annual runoff > flow during the flood season. The results showed that the PD, SHDI, and LHAI have a greater impact on the landscape ecological risk index in the study area, indicating that the change in structure of the tidal flats was the key process that caused changes in landscape ecological risk. However, the main force driving the change in regional landscape structure was human development (Jat et al., 2008; Bryan et al., 2018). On the whole, the q value of the socioeconomic driving factor reflecting the influence of human activities was greater than that of the natural factors, which indicates that human development activities were the key factors driving the changes in ecological risk of the tidal flats landscape in the LEW.
3.3.2 Effects of changes in landscape spatial structure on ecological risks
The ERI increased first and then decreased with the increase of SHDI (Figure 6A). The ERI increased when the SHDI changed when the SHDI value was less than 1.0. The ERI decreased when the SHDI increased from a value changed from 1.0 to 1.4. The ERI increased again when the SHDI continued to increase after exceeding 1.4. The ERI slowly decreased with the increase of the PD (Figure 6B). The influence of the PD on the ERI stabilized with no obvious change beyond a PD of 0.3. The results showed that the environmental risks faced by a single landscape patch were decreased when the tidal flat landscape evolved from a single landscape patch to multiple other landscape patches. Thus, the ERI decreased to a certain extent as the SHDI and PD increased. However, the ecological risk of the landscape tended to be stable or increase when this changing trend exceeded a certain limit. This indicates that the continuous increase of SHDI and PD will be unfavorable to reducing the ecological risk of the regional landscape. This limitation may be related to the increase of landscape diversity in the study area mainly due to the contribution of the artificial landscape. However, the increase of artificial landscapes will exacerbate the fragmentation of regional landscapes (Zhou et al., 2018), making the landscape pattern unstable and affecting the regional landscape and its ecological security.
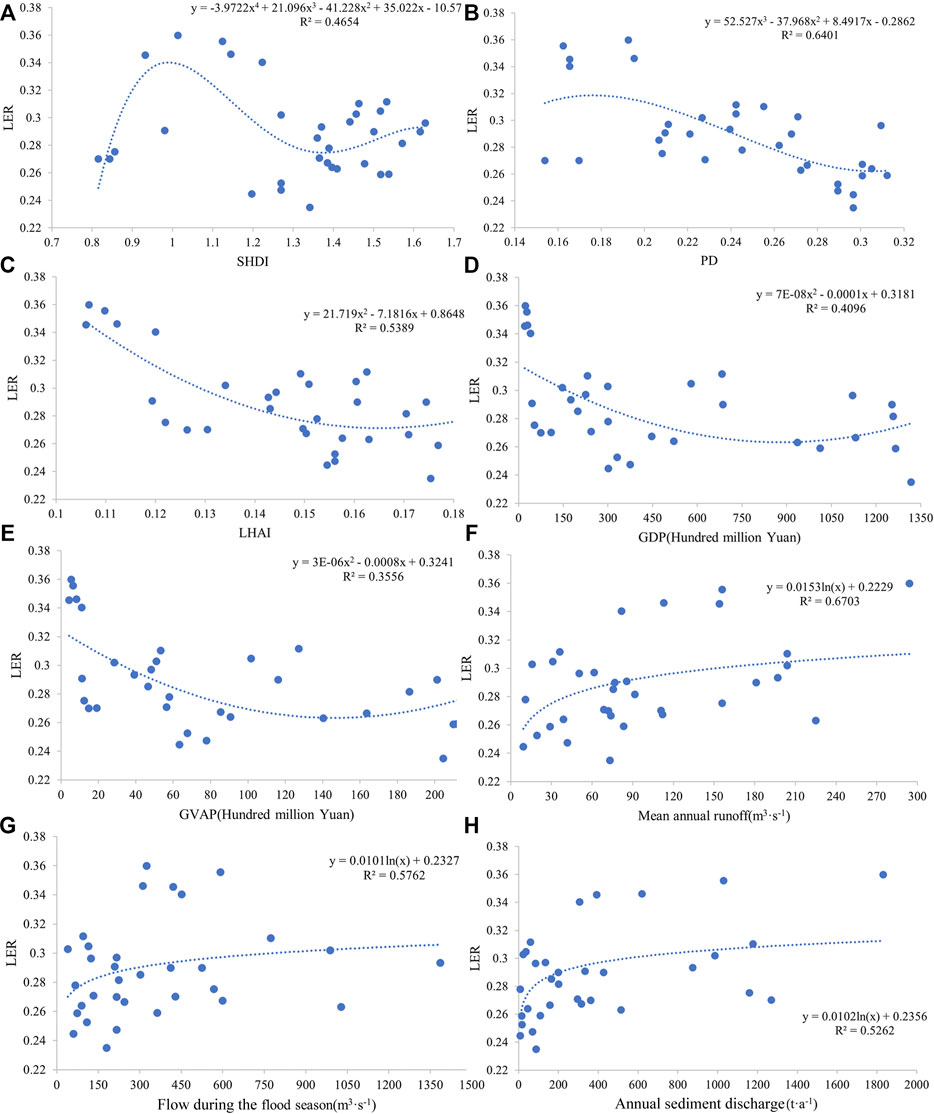
FIGURE 6. Different kinds of driving forces on Ecological risk in the tidal flat: the labels (A–H) represent SHDI, PD, LHAI, GDP, GVAP, mean annual flow, flow during the flood season, and annual sediment discharge respectively.
3.3.3 The impact of human disturbance activities on ecological risks
The LHAI, GDP, and GVAP all have a quadratic polynomial relationship with the ERI (Figures 6C–E). The regional ERI decreased with increases in the LHAI, when the value was less than 0.15. This indicates that the ecological risk faced by the single landscape of tidal flats would be reduced if the single tidal flats landscape was transformed into an artificially developed landscape. The ERI became stable when human disturbance continued to increase. However, the ERI changed to a trend of increasing with increasing LHAI when the LHAI value was greater than 0.17. The ERI decreased with increases in regional GDP and GVAP but once economic development exceeds a certain limit, the ERI switched to increasing with increases in GDP and GVAP. These results show that the environmental risks faced by a single tidal flats landscape would be reduced to a certain extent in the face of human development controlled within a certain range.
3.3.4 The impact of watershed hydrology on landscape ecological risk
The Liaohe River flowing into the estuary has a logarithmic relationship between the mean annual flow, flow during the flood season, annual sediment discharge, and the ERI (Figures 6F–H). The ERI increased rapidly with changes in the mean annual flow and the flow during the flood season when the mean annual flow was less than 90 m3 s−1 and the flow during the flood season was less than 300 m3 s−1 in the low runoff years. The ERI tended to be stable with no obvious change when the mean annual flow and the flow during the flood season continued to increase beyond 180 m3 s−1 and 600 m3 s−1, respectively. The regional ERI clearly increased with the change of the sediment discharge when the annual sediment discharge of the Liaohe River was less than 400 t y−1. Beyond this, The ERI tended to be stable when the annual sediment discharge further increased. In addition, there was little effect on the ERI when the annual sediment discharge was greater than 1,200 t y−1.
4 Discussion
4.1 The evolution of estuarine tidal flats landscapes
The estuary tidal flats are located in the land–ocean interlaced, sensitive zone, which is the most vulnerable type of landscape in coastal estuaries (Li et al., 2021a; Xie et al., 2021). They are potential development areas for reeds, salina, and other types of wetlands that exist in coastal estuaries. The coastal and estuarine tidal flats have mainly evolved into salt marsh wetlands under the dominant action of certain natural factors. Regional landscape diversity has increased, which has improved the anti-disturbance ability of coastal estuarine wetland ecosystems (Cao et al., 2020; Liu et al., 2020; Zhao et al., 2020). However, tidal flats in coastal estuaries are an important element of the land reserve resources of coastal cities, and the evolution of this landscape has been deeply affected by human activities (Lin et al., 2018), which have intensified the evolution of natural tidal flats into diversified artificial landscapes (Zhang et al., 2022). This has led to the fragmentation of wetland landscapes, reducing the connectivity between patches of landscape types (Zhou et al., 2018), and increasing the complexity and heterogeneity of patch shapes (Liu et al., 2016).
The research showed that the tidal flats and natural water landscapes of coastal wetlands were mainly transformed into construction land and aquaculture land, the natural wetlands were turned into artificial wetlands and aquaculture, and the artificial wetlands were transformed into construction land (Lin et al., 2018). These results were consistent with the results of the tidal flats in the LEW. The tidal flats landscape has mainly evolved into a natural wetland landscape under the influence of natural factors. In contrast, the tidal flats landscape has rapidly turned into an artificial landscape due to human interference, which causes the fragmentation of the regional landscape pattern. In general, the evolution of the landscape pattern of coastal estuarine tidal flats indicated that landscape types with a high degree of human disturbance replaced various landscape types with low and medium levels of human disturbance (Li et al., 2017; Zhou et al., 2018), and the intensity of human disturbance was the main reason for the instability of the landscape pattern in the study area. It showed that the continuous increase of human disturbance intensity was the main reason for the rapid changes in the estuarine tidal flat landscape pattern.
4.2 Ecological risks faced by estuarine tidal flats landscape evolution
In terms of time dynamics, the ERI of the tidal flats landscape in the study area changed in stages, and the ERI level differed at different stages of landscape evolution. The ERI was established based on the landscape index, and its value was determined by the change in the combination of landscape type and its vulnerability (Xu and Kang, 2017; Lin et al., 2019). Therefore, the degree of transfer between the patches with different ecological risk levels was different in the process of landscape evolution of the tidal flats. The LEW was mainly affected by agricultural production at the beginning of the study period when the main landscape type was exposed tidal flats (Figure 7). The ERI was relatively high due to the exposed tidal flats with a high degree of vulnerability. Whereafter, with the increase of landscape diversity, the tidal flats landscape evolved into natural wetlands such as reeds and Suaeda salsa. As a result, the ERI has decreased, and the high-risk zones have shifted to become medium and low-risk zones. More recently, with the implementation of China’s reform and development policy, the Liaohe Estuary region experienced rapid urbanization and social and economic development, which promoted the rapid transformation of such landscapes of exposed tidal flats, natural waters, natural wetlands, and others to artificial landscapes with low vulnerability (Cheng et al., 2019). By the end of the study period, the overall ERI decreased due to the area of high-risk and medium-risk patches decreasing by 24.90% and 16.83% respectively, and the area of low-risk patches increasing by 20.24%.
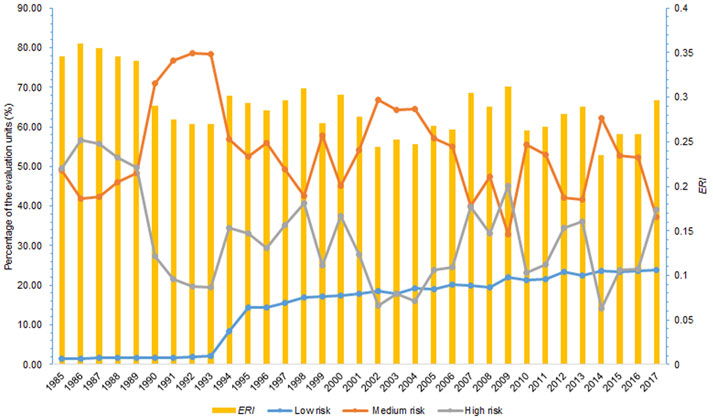
FIGURE 7. Interannual changes in ERI and percentage of assessment units with different ecological risk levels.
Previous studies concluded that urban sprawl and increased land fragmentation were the main driving factors leading to increased ecological risks in regional landscapes (Tang et al., 2018; Lin et al., 2021). However, in terms of the environmental conditions, the artificial reclamation of coastal tidal flats has played a role to some extent in protecting the fragile ecological environment of the islands and the coastal tidal flats (Lin et al., 2018). The expansion of artificial landscapes increased landscape diversity and reduced landscape sensitivity (Yang et al., 2022). It showed that there has a double nature of the impact of human disturbance on the ecological landscape structures. The anti-interference ability of the fragile ecosystem will be improved due to the increase in landscape diversity of the regional ecosystem under moderate interference. Nevertheless, the ecological risk of the regional ecosystem will be increased due to the destruction and imbalance of landscape structures under excessive artificial interference (Osman, 2008, 2015). The overall distribution of the ERI in the tidal flats of the LEW (Figure 7) exhibited a shift from high-risk patches with high sensitivity to medium-risk patches with low sensitivity and low-risk ones with strong robustness against interference. The LER values decreased to a certain extent with the increase of artificial landscape components generally. But a continued increase in artificial disturbance would lead to an increase in regional ecological risk.
4.3 The impact thresholds of driving factors
The results showed that in the evolution of the tidal flats landscape in the LEW, there seems to be a critical value for the impact of human disturbance factors and natural factors on the ERI in the study area. Studies on the Yellow River Estuary and Yancheng Beach showed that the main artificial disturbance activities should be controlled within a reasonable threshold to ensure the stability and ecological security of the estuary and the pattern of the tidal flats landscape (Tian et al., 2022; Yang et al., 2022). Other studies determined that the vehicular roads in the tidal flats of the LEW should be limited to at or below 770.92 hm2 to ensure the ecological stability of the tidal flat structure (Li et al., 2021a). This study found that the LHAI should be controlled to be no higher than 0.17 in the development of the tidal flats, otherwise, if the LHAI exceeds this value, the ERI will increase due to the continuous artificial disturbance. It showed that the management of the estuarine tidal flat landscape should follow the guidance of the intermediate disturbance hypothesis (Lubchenco and Sousa, 2020; Murdoch and Sousa, 2020). The proportion of artificial landscapes can be increased appropriately in the development of tidal flat resources. But to take maximum advantage of human interference to increase the heterogeneity and diversity of regional landscapes its development needs to be controlled within a reasonable limit. As such, the ecological risks faced by a single landscape structure will be reduced (Molino and Sabatier, 2001; Sasaki et al., 2009; Liu et al., 2019).
A local government in Italy dredged 10 × 104 m3 of sand from Viareggio to nourish the tidal flats in order to maintain the relative stability of the Tuscan estuary landscape (Pranzini et al., 2020). Research on the Hudson River estuary clarified that the upstream sediment input to the estuary of the Mohawk and Hudson Rivers should not be less than 36.13 × 104 and 10.95 × 104 t⋅y−1, respectively, to maintain the stability of the tidal flats landscape (Ralston and Geyer, 2009). Research into the Yellow River Estuary showed that to maintain the stability of the tidal flats landscape, the sediment discharge of the Yellow River should not be less than 441 × 106 and 167 × 106 t⋅y−1 before and after artificial water and sediment regulation, respectively (Kong et al., 2015). Previous studies proposed that the river sediment transported to the tidal flats should be no less than 792.91 × 104 t⋅y−1 to maintain the stability of the landscape macro-structure of the Liaohe Estuary wetlands (Li et al., 2021a). It showed that the material supply of the upstream basins restricted the stability of the morphological structure of the estuarine tidal flat landscape (Giosan et al., 2014). If the material supply of the basins was insufficient, the tidal flat landscape ecosystem will be degraded directly, hence the ecological risk level aggravated faced by the estuary tidal flats (Wei et al., 2020; Yu et al., 2021). This paper also found that there was a threshold range for the impact of changes in sediment input upstream of the Liaohe River in terms of the ecological risk to tidal flats landscapes. The annual sediment discharge, the mean annual flow, and the flow during the flood season should not be less than 400 t·y−1, 180 m3·s−1, or 600 m3·s−1, respectively. If any of these factors are below these thresholds, the ecological risk index increases rapidly. The results showed that the sediment and water input from the upstream of the Liaohe River was an important material source to ensure the stability of the estuary tidal flat landscape structure, which can alleviate the ecological risk pressure of the tidal flat landscape effectively. Therefore, to maintain the ecological risk of tidal flat landscapes within an available range, two aspects should be paid attention to in the protection and utilization of estuary tidal flat resources in the future. The regional human development activities should be controlled within an appropriate range. On the other hand, it was necessary to coordinate the allocation of water and sediment material supply from upstream to ensure the stability of the estuary tidal flat landscape morphology.
5 Conclusion
The evolution of the tidal flats landscape in the LEW has changed in stages, and seems to be turning into mainly an artificial landscape. Between 1985 and 2000, the exposed tidal flats landscape mainly evolved into natural wetlands such as Suaeda salsa and reeds. In the period 2000 to 2010, the exposed tidal flats landscape rapidly transformed into paddy fields and aquaculture, and landscape fragmentation continued to intensify. From 2010 to 2017, the proportion of artificial landscapes began to decrease, changing into natural landscapes, and the tidal flats landscapes in the study area developed naturally due to ecological restoration projects.
The landscape pattern of tidal flats in the LEW has generally been at a medium level of ecological risk over the past 30 years. The ecological risk tended to decrease as the single exposed tidal flats landscape evolved into a diverse landscape. The areas of high-risk and medium-risk patches decreased to a certain extent, and the areas of low-risk patches gradually increased. The high ecological risk patches were mainly distributed in the exposed tidal flats zones, with high sensitivity in the periphery of the estuary.
The key factors affecting the landscape ecological risk of the tidal flats were PD, SHDI, LHAI, GDP, and sediment discharge. Among them, the PD, SHDI, and LHAI were the key processes that caused changes in landscape ecological risk. Human disturbance was the key factor affecting changes to landscape ecological risk. In regional wetland management, the LHAI should be controlled to be no higher than 0.17, and the SHDI and PD should be kept within 0.14 and 0.3, respectively, to preserve a reasonable landscape structure and the ecological security of regional tidal flats. River sediment input was a key natural factor affecting landscape ecological risk, to ensure the continuing evolution of the regional tidal flats landscape.
Data availability statement
The original contributions presented in the study are included in the article/Supplementary Materials, further inquiries can be directed to the corresponding author.
Author contributions
Writing—original draft preparation, HL; writing—review and editing, HL and FS; investigation, HL and LD; data curation, FS and CW; supervision, CG; project administration, FS.
Funding
This research was funded by the National Key Research and Development Program of China (2022YFF1301004); the China Postdoctoral Science Foundation (2021MD703856); the Liaoning Science and technology innovation leader projects (XLYC2002054).
Acknowledgments
Thanks to all the teachers who participated in the research process for their technical guidance, the master and doctoral students for their help in data collection and interpretation, and the support of Liaoning Panjin Wetland Ecosystem National Observation and Research Station and Liaoning Shuangtai Estuary Wetland Ecosystem Research Station.
Conflict of interest
LD was employed by the Yellow River Engineering Consulting Co., Ltd.
The remaining authors declare that the research was conducted in the absence of any commercial or financial relationships that could be construed as a potential conflict of interest.
Publisher’s note
All claims expressed in this article are solely those of the authors and do not necessarily represent those of their affiliated organizations, or those of the publisher, the editors and the reviewers. Any product that may be evaluated in this article, or claim that may be made by its manufacturer, is not guaranteed or endorsed by the publisher.
Supplementary material
The Supplementary Material for this article can be found online at: https://www.frontiersin.org/articles/10.3389/fenvs.2022.1070009/full#supplementary-material
References
Bao, J., and Gao, S. (2021). Wetland utilization and adaptation practice of a coastal megacity: A case study of chongming island, shanghai, China. Front. Environ. Sci. 9, 627963. doi:10.3389/fenvs.2021.627963
Bryan, B. A., Gao, L., Ye, Y., Sun, X., Connor, J. D., Crossman, N. D., et al. (2018). China’s response to a national land-system sustainability emergency. Nature 559, 193–204. doi:10.1038/s41586-018-0280-2
Cao, W., Zhou, Y., Li, R., and Li, X. (2020). Mapping changes in coastlines and tidal flats in developing islands using the full time series of Landsat images. Remote Sens. Environ. 239, 111665. doi:10.1016/j.rse.2020.111665
Chen, A., Yao, L., Sun, R., and Chen, L. (2014). How many metrics are required to identify the effects of the landscape pattern on land surface temperature? Ecol. Indic. 45, 424–433. doi:10.1016/j.ecolind.2014.05.002
Chen, J., Dong, B., Li, H., Zhang, S., Peng, L., Fang, L., et al. (2020). Corrigendum to ‘Study on landscape ecological risk assessment of Hooded Crane breeding and overwintering habitat’ [Volume 187, August 2020, 109649]. Environ. Res. 191, 110076. doi:10.1016/j.envres.2020.110076
Chen, J., Yang, S., Li, H., Zhang, B., and Lv, J. (2013). “Research on geographical environment unit division based on the method of natural breaks (jenks),” in Isprs/igu/ica joint workshop on borderlands modelling and understanding for global sustainability 2013. Editors J. Chen, Y. Ge, and Y. Cheng (Gottingen: Copernicus Gesellschaft Mbh), 47–50. doi:10.5194/isprsarchives-XL-4-W3-47-2013
Cheng, R., Chen, W., Wu, S., and Lin, J. (2019). Spatiotemporal variation characteristics of ecosystems and their driving forces in the min delta urban agglomeration. IRSPSD. Int. 7, 177–194. doi:10.14246/irspsda.7.1_177
Costanza, R., Anderson, S. J., Sutton, P., Mulder, K., Mulder, O., Kubiszewski, I., et al. (2021). The global value of coastal wetlands for storm protection. Glob. Environ. Change 70, 102328. doi:10.1016/j.gloenvcha.2021.102328
Cui, L., Li, G., Chen, Y., and Li, L. (2021). Response of landscape evolution to human disturbances in the coastal wetlands in northern jiangsu province, China. Remote Sens. 13, 2030. doi:10.3390/rs13112030
Dadashpoor, H., Azizi, P., and Moghadasi, M. (2019). Land use change, urbanization, and change in landscape pattern in a metropolitan area. Sci. Total Environ. 655, 707–719. doi:10.1016/j.scitotenv.2018.11.267
Duan, H., Yu, X., Zhang, L., Xia, S., Liu, Y., Mao, D., et al. (2022). An evaluating system for wetland ecological risk: Case study in coastal mainland China. Sci. Total Environ. 828, 154535. doi:10.1016/j.scitotenv.2022.154535
Fu, B. (1995). The spatial pattern analysis of agricultural landscape in the loess are. Acta Ecol. Sin. 15, 113–120. doi:10.2307/2997695
Giosan, L., Syvitski, J., Constantinescu, S., and Day, J. (2014). Climate change: Protect the world’s deltas. Nature 516, 31–33. doi:10.1038/516031a
Gong, J., Cao, E., Xie, Y., Xu, C., Li, H., and Yan, L. (2021). Integrating ecosystem services and landscape ecological risk into adaptive management: Insights from a Western mountain-basin area, China. J. Environ. Manage 281, 111817. doi:10.1016/j.jenvman.2020.111817
Goussen, B., Price, O. R., Rendal, C., and Ashauer, R. (2016). Integrated presentation of ecological risk from multiple stressors. Sci. Rep. 6, 36004. doi:10.1038/srep36004
Grafius, D. R., Edmondson, J. L., Norton, B. A., Clark, R., Mears, M., Leake, J. R., et al. (2020). Estimating food production in an urban landscape. Sci. Rep. 10, 5141. doi:10.1038/s41598-020-62126-4
Guida Johnson, B., and Zuleta, G. A. (2013). Land-use land-cover change and ecosystem loss in the Espinal ecoregion, Argentina. Agric. Ecosyst. Environ. 181, 31–40. doi:10.1016/j.agee.2013.09.002
Imhoff, M. L., Bounoua, L., DeFries, R., Lawrence, W. T., Stutzer, D., Tucker, C. J., et al. (2004). The consequences of urban land transformation on net primary productivity in the United States. Remote Sens. Environ. 89, 434–443. doi:10.1016/j.rse.2003.10.015
Jackson, M. V., Fuller, R. A., Gan, X., Li, J., Mao, D., Melville, D. S., et al. (2021). Dual threat of tidal flat loss and invasive Spartina alterniflora endanger important shorebird habitat in coastal mainland China. J. Environ. Manag. 278, 111549. doi:10.1016/j.jenvman.2020.111549
Jat, M. K., Garg, P. K., and Khare, D. (2008). Modelling of urban growth using spatial analysis techniques: A case study of ajmer city (India). Int. J. Remote Sens. 29, 543–567. doi:10.1080/01431160701280983
Jiang, W., Lv, J., Wang, C., Chen, Z., and Liu, Y. (2017). Marsh wetland degradation risk assessment and change analysis: A case study in the zoige plateau, China. Ecol. Indic. 82, 316–326. doi:10.1016/j.ecolind.2017.06.059
Jiyuan, L., Zengxiang, Z., Xinliang, X., Wenhui, K., Wancun, Z., Shuwen, Z., et al. (2010). Spatial patterns and driving forces of land use change in China during the early 21st century. J. Geogr. Sci. 20, 483–494. doi:10.1007/s11442-010-0483-4
Kim, J.-H., Kim, S.-Y., and Yoo, S.-H. (2021). South Koreans’ willingness to pay for restoration of gomsoman tidal flat. Ocean Coast. Manag. 199, 105388. doi:10.1016/j.ocecoaman.2020.105388
Kim, K.-L., and Ryu, J.-H. (2020). Mapping oyster reef distribution using kompsat-2/3 and linear spectral unmixing algorithm - a case study at hwangdo tidal flat. J. Coast. Res. 102, 246–253. doi:10.2112/SI102-030.1
Kong, D., Miao, C., Wu, J., Jiang, L., and Duan, Q. (2015). Bi-Objective analysis of water–sediment regulation for channel scouring and delta maintenance: A study of the lower Yellow River. Glob. Planet. Change 133, 27–34. doi:10.1016/j.gloplacha.2015.07.007
Li, H., Li, L., Su, F., Wang, T., and Gao, P. (2021a). Ecological stability evaluation of tidal flat in coastal estuary: A case study of Liaohe estuary wetland, China. Ecol. Indic. 130, 108032. doi:10.1016/j.ecolind.2021.108032
Li, H., Peng, J., Yanxu, L., and Yi’na, H. (2017). Urbanization impact on landscape patterns in beijing city, China: A spatial heterogeneity perspective. Ecol. Indic. 82, 50–60. doi:10.1016/j.ecolind.2017.06.032
Li, Y., Mao, D., Wang, Z., Wang, X., Tan, X., Jia, M., et al. (2021b). Identifying variable changes in wetlands and their anthropogenic threats bordering the Yellow Sea for water bird conservation. Glob. Ecol. Conservation 27, e01613. doi:10.1016/j.gecco.2021.e01613
Lin, J., Lin, M., Chen, W., Zhang, A., Qi, X., and Hou, H. (2021). Ecological risks of geological disasters and the patterns of the urban agglomeration in the Fujian Delta region. Ecol. Indic. 125, 107475. doi:10.1016/j.ecolind.2021.107475
Lin, W., Cen, J., Xu, D., Du, S., and Gao, J. (2018). Wetland landscape pattern changes over a period of rapid development (1985-2015) in the ZhouShan Islands of Zhejiang province, China. Estuar. Coast. Shelf Sci. 213, 148–159. doi:10.1016/j.ecss.2018.08.024
Lin, Y., Hu, X., Zheng, X., Hou, X., Zhang, Z., Zhou, X., et al. (2019). Spatial variations in the relationships between road network and landscape ecological risks in the highest forest coverage region of China. Ecol. Indic. 96, 392–403. doi:10.1016/j.ecolind.2018.09.016
Liu, X., Liu, X., Wu, L., and Tian, Z. (2019). Diversity in phytoplankton communities: A field test of the intermediate disturbance hypothesis. Ecol. Eng. 129, 54–60. doi:10.1016/j.ecoleng.2018.12.009
Liu, Y.-F., Ma, J., Wang, X.-X., Zhong, Q.-Y., Zong, J.-M., Wu, W.-B., et al. (2020). Joint effect of spartina alterniflora invasion and reclamation on the spatial and temporal dynamics of tidal flats in Yangtze River Estuary. Remote Sens. (Basel) 12, 1725. doi:10.3390/rs12111725
Liu, Y., He, Q., Tan, R., Liu, Y., and Yin, C. (2016). Modeling different urban growth patterns based on the evolution of urban form: A case study from huangpi, central China. Appl. Geogr. 66, 109–118. doi:10.1016/j.apgeog.2015.11.012
Lubchenco, J., and Sousa, W. P. (2020). Joseph H. Connell (1923–2020). Nature 586, 670. doi:10.1038/d41586-020-02990-2
Ma, Z., Chen, Y., David, S. Me., Fan, J., Li, J., Dong, J., et al. (2019). Changes in area and number of nature reserves in China. Conserv. Biol. 33, 1066–1075. doi:10.1111/cobi.13285
Ma, Z., Melville, D. S., Liu, J., Chen, Y., Yang, H., Ren, W., et al. (2014). Rethinking China’s new great wall. Science 346, 912–914. doi:10.1126/science.1257258
McEachran, Z. P., Slesak, R. A., and Karwan, D. L. (2018). From skid trails to landscapes: Vegetation is the dominant factor influencing erosion after forest harvest in a low relief glaciated landscape. For. Ecol. Manage 430, 299–311. doi:10.1016/j.foreco.2018.08.021
Molino, J. F., and Sabatier, D. (2001). Tree diversity in tropical rain forests: A validation of the intermediate disturbance hypothesis. Science 294, 1702–1704. doi:10.1126/science.1060284
Murdoch, W. W., and Sousa, W. P. (2020). Joseph H. Connell (1923-2020). Science 370, 410. doi:10.1126/science.abe8992
Murray, N. J., Phinn, S. R., DeWitt, M., Ferrari, R., Johnston, R., Lyons, M. B., et al. (2019). The global distribution and trajectory of tidal flats. Nature 565, 222–225. doi:10.1038/s41586-018-0805-8
Osman, R. W. (2008). “Intermediate disturbance hypothesis,” in Encyclopedia of ecology. Editors S. E. Jørgensen, and B. D. Fath (Oxford: Academic Press), 1986–1994. doi:10.1016/B978-008045405-4.00510-3
Osman, R. W. (2015). “The intermediate disturbance hypothesis☆,” in Encyclopedia of ecology (Second Edition), ed. B. Fath (Oxford: Elsevier), 441–450. doi:10.1016/B978-0-12-409548-9.09480-X
Pace, M., Borg, J. A., Galdies, C., and Malhotra, A. (2017). Influence of wave climate on architecture and landscape characteristics of Posidonia oceanica meadows. Mar. Ecol. Berl. 38, e12387. doi:10.1111/maec.12387
Paul, K. I., Polglase, P. J., Nyakuengama, J. G., and Khanna, P. K. (2002). Change in soil carbon following afforestation. For. Ecol. Manag. 168, 241–257. doi:10.1016/S0378-1127(01)00740-X
Plexida, S. G., Sfougaris, A. I., Ispikoudis, I. P., and Papanastasis, V. P. (2014). Selecting landscape metrics as indicators of spatial heterogeneity-A comparison among Greek landscapes. Int. J. Appl. Earth Obs. Geoinf 26, 26–35. doi:10.1016/j.jag.2013.05.001
Pranzini, E., Cinelli, I., Cipriani, L. E., and Anfuso, G. (2020). An integrated coastal sediment management plan: The example of the tuscany region (Italy). JMSE 8, 33. doi:10.3390/jmse8010033
Ralston, D. K., and Geyer, W. R. (2009). Episodic and long-term sediment transport capacity in the Hudson River Estuary. Estuaries Coasts 32, 1130–1151. doi:10.1007/s12237-009-9206-4
Ran, P., Hu, S., Frazier, A. E. E., Qu, S., Yu, D., and Tong, L. (2022). Exploring changes in landscape ecological risk in the Yangtze River Economic Belt from a spatiotemporal perspective. Ecol. Indic. 137, 108744. doi:10.1016/j.ecolind.2022.108744
Roussel, F., and Alexandre, F. (2021). Landscape ecological enhancement and environmental inequalities in peri-urban areas, using flora as a socio-ecological indicator-The case of the greater Paris area. Landsc. Urban Plan. 210, 104062. doi:10.1016/j.landurbplan.2021.104062
Sasaki, T., Okubo, S., Okayasu, T., Jamsran, U., Ohkuro, T., and Takeuchi, K. (2009). Management applicability of the intermediate disturbance hypothesis across Mongolian rangeland ecosystems. Ecol. Appl. 19, 423–432. doi:10.1890/08-0144.1
Shen, F., Zhou, Y., Li, J., He, Q., and Verhoef, W. (2013). Remotely sensed variability of the suspended sediment concentration and its response to decreased river discharge in the Yangtze estuary and adjacent coast. Cont. Shelf Res. 69, 52–61. doi:10.1016/j.csr.2013.09.002
Skilleter, G. A., Loneragan, N. R., Olds, A., Zharikov, Y., and Cameron, B. (2017). Connectivity between seagrass and mangroves influences nekton assemblages using nearshore habitats. Mar. Ecol. Prog. Ser. 573, 25–43. doi:10.3354/meps12159
Strayer, D. L., Beighley, R. E., Thompson, L. C., Brooks, S., Nilsson, C., Pinay, G., et al. (2003). Effects of land cover on stream ecosystems: Roles of empirical models and scaling issues. Ecosystems 6, 407–423. doi:10.1007/pl00021506
Sun, X., Li, Y., Zhu, X., Cao, K., and Feng, L. (2017). Integrative assessment and management implications on ecosystem services loss of coastal wetlands due to reclamation. J. Clean. Prod. 163, S101–S112. doi:10.1016/j.jclepro.2015.10.048
Tang, L., Wang, L., Li, Q., and Zhao, J. (2018). A framework designation for the assessment of urban ecological risks. Int. J. Sustain. Dev. World Ecol. 25, 387–395. doi:10.1080/13504509.2018.1434570
Tian, P., Cao, L., Li, J., Pu, R., Liu, Y., Zhang, H., et al. (2022). Ecosystem stability assessment of Yancheng coastal wetlands, a world natural heritage site. Land 11, 564. doi:10.3390/land11040564
Walling, D. E. (2006). Human impact on land–ocean sediment transfer by the world’s rivers. Geomorphology 79, 192–216. doi:10.1016/j.geomorph.2006.06.019
Wang, H., Liu, X., Zhao, C., Chang, Y., Liu, Y., and Zang, F. (2021). Spatial-temporal pattern analysis of landscape ecological risk assessment based on land use/land cover change in Baishuijiang National nature reserve in Gansu Province, China. Ecol. Indic. 124, 107454. doi:10.1016/j.ecolind.2021.107454
Wang, H., Wu, X., Bi, N., Li, S., Yuan, P., Wang, A., et al. (2017). Impacts of the dam-orientated water-sediment regulation scheme on the lower reaches and delta of the Yellow River (huanghe): A review. Glob. Planet. Change 157, 93–113. doi:10.1016/j.gloplacha.2017.08.005
Wang, J.-F., Zhang, T.-L., and Fu, B.-J. (2016). A measure of spatial stratified heterogeneity. Ecol. Indic. 67, 250–256. doi:10.1016/j.ecolind.2016.02.052
Wang, J., Li, X., Christakos, G., Liao, Y., Zhang, T., Gu, X., et al. (2010). Geographical detectors-based health risk assessment and its application in the neural tube defects study of the heshun region, China. Int. J. Geogr. Inf. Sci. 24, 107–127. doi:10.1080/13658810802443457
Wang, L., Wang, S., Zhou, Y., Zhu, J., Zhang, J., Hou, Y., et al. (2020). Landscape pattern variation, protection measures, and land use/land cover changes in drinking water source protection areas: A case study in danjiangkou reservoir, China. Glob. Ecol. Conserv. 21, e00827. doi:10.1016/j.gecco.2019.e00827
Wei, W., Dai, Z., Pang, W., Wang, J., and Gao, S. (2020). Sedimentary zonation shift of tidal flats in a meso-tidal estuary. Sediment. Geol. 407, 105749. doi:10.1016/j.sedgeo.2020.105749
Winkler, K., Fuchs, R., Rounsevell, M., and Herold, M. (2021). Global land use changes are four times greater than previously estimated. Nat. Commun. 12, 2501. doi:10.1038/s41467-021-22702-2
Xie, W., Wang, X., Guo, L., He, Q., Dou, S., and Yu, X. (2021). Impacts of a storm on the erosion process of a tidal wetland in the Yellow River Delta. Catena 205, 105461. doi:10.1016/j.catena.2021.105461
Xu, J., and Kang, J. (2017). Comparison of ecological risk among different urban patterns based on system dynamics modeling of urban development. J. Urban Plan. Dev. 143, 04016034. doi:10.1061/(ASCE)UP.1943-5444.0000365
Xu, K., Bentley, S. J., Day, J. W., and Freeman, A. M. (2019). A review of sediment diversion in the Mississippi River Deltaic Plain. Estuar. Coast. Shelf Sci. 225, 106241. doi:10.1016/j.ecss.2019.05.023
Yang, D., Zhang, P., Jiang, L., Zhang, Y., Liu, Z., and Rong, T. (2022). Spatial change and scale dependence of built-up land expansion and landscape pattern evolution—case study of affected area of the lower Yellow River. Ecol. Indic. 141, 109123. doi:10.1016/j.ecolind.2022.109123
Yao, H. (2013). Characterizing landuse changes in 1990–2010 in the coastal zone of Nantong, Jiangsu province, China. Ocean Coast. Manag. 71, 108–115. doi:10.1016/j.ocecoaman.2012.09.007
Yu, D., Han, G., Wang, X., Zhang, B., Eller, F., Zhang, J., et al. (2021). The impact of runoff flux and reclamation on the spatiotemporal evolution of the Yellow River estuarine wetlands. Ocean. Coast. Manag. 212, 105804. doi:10.1016/j.ocecoaman.2021.105804
Yu, X., and Zhang, L. (2020). Green paper of China’s coastal wetland conservation. Beijing, China: Science Press.
Zhan, J., Zhang, F., Chu, X., Liu, W., and Zhang, Y. (2019). Ecosystem services assessment based on emergy accounting in Chongming Island, Eastern China. Ecol. Indic. 105, 464–473. doi:10.1016/j.ecolind.2018.04.015
Zhang, S., Zhong, Q., Cheng, D., Xu, C., Chang, Y., Lin, Y., et al. (2022). Landscape ecological risk projection based on the PLUS model under the localized shared socioeconomic pathways in the Fujian Delta region. Ecol. Indic. 136, 108642. doi:10.1016/j.ecolind.2022.108642
Zhang, X., Wang, G., Xue, B., Zhang, M., and Tan, Z. (2021). Dynamic landscapes and the driving forces in the Yellow River Delta wetland region in the past four decades. Sci. Total Environ. 787, 147644. doi:10.1016/j.scitotenv.2021.147644
Zhang, Y., Wang, T., Cai, C., Li, C., Liu, Y., Bao, Y., et al. (2016). Landscape pattern and transition under natural and anthropogenic disturbance in an arid region of northwestern China. Int. J. Appl. Earth Obs. Geoinf 44, 1–10. doi:10.1016/j.jag.2015.06.013
Zhao, Y., Liu, Q., Huang, R., Pan, H., and Xu, M. (2020). Recent evolution of coastal tidal flats and the impacts of intensified human activities in the modern radial sand ridges, east China. Int. J. Environ. Res. Public Health 17, 3191. doi:10.3390/ijerph17093191
Zhou, Y., Dou, Y., Yu, X., Zhang, L., Huang, C., Wang, Y., et al. (2020). Examining health of wetlands with multiple ecosystem services as targets in China’s coastal regions. Chin. Geogr. Sci. 30, 600–613. doi:10.1007/s11769-020-1137-9
Zhou, Y., Ning, L., and Bai, X. (2018). Spatial and temporal changes of human disturbances and their effects on landscape patterns in the Jiangsu coastal zone, China. Ecol. Indic. 93, 111–122. doi:10.1016/j.ecolind.2018.04.076
Keywords: coastal estuary, tidal flats, landscape evolution, ecological risk, impact thresholds
Citation: Li H, Su F, Guo C, Dong L, Song F, Wei C and Zheng Y (2022) Landscape ecological risk assessment and driving mechanism of coastal estuarine tidal flats—A case study of the liaohe estuary wetlands. Front. Environ. Sci. 10:1070009. doi: 10.3389/fenvs.2022.1070009
Received: 14 October 2022; Accepted: 14 November 2022;
Published: 28 November 2022.
Edited by:
Pedzisai Kowe, Midlands State University, ZimbabweReviewed by:
Tatenda Musasa, Midlands State University, ZimbabweMing Jiang, Northeast Institute of Geography and Agroecology (CAS), China
Copyright © 2022 Li, Su, Guo, Dong, Song, Wei and Zheng. This is an open-access article distributed under the terms of the Creative Commons Attribution License (CC BY). The use, distribution or reproduction in other forums is permitted, provided the original author(s) and the copyright owner(s) are credited and that the original publication in this journal is cited, in accordance with accepted academic practice. No use, distribution or reproduction is permitted which does not comply with these terms.
*Correspondence: Fangli Su, c3lhdWxoZkBzeWF1LmVkdS5jbg==