- Department of Civil Engineering, University of Asia Pacific, Dhaka, Bangladesh
One of the most persistent issues affecting individuals in developing countries is the lack of access to safe drinking water and sanitary facilities. The adoption of centralized water, energy, and cost-intensive technology has proven ineffective in addressing the complex water-related challenges that have arisen as a consequence of growing urbanization in developing nations. Constructed wetlands have emerged as an effective wastewater treatment solution with natural applications. The fundamental goal of this study is to offer a complete overview of the wide variety of practices, uses, and investigations of constructed wetlands systems for eliminating different pollutants from wastewater in developing countries leading to placing them in the context of climate change, environmental resource planning, and sustainable wastewater treatment systems. CWs offer significant levels of treatment performances with hybrid systems achieving contaminant removal efficiencies up to 93.82% for total suspended solids, 85.65% for chemical oxygen demand, and 80.11% for ammonia nitrogen which is adequate with respect to other viable alternatives. In terms of Biological Oxygen Demand (BOD5), the highest elimination (84.06%) was achieved in hybrid systems when compared to Free water surface CWs (65.34%), Horizontal sub-surface CWs (75.1%), and Floating treatment wetland (55.29%). The maximum power density generation through the microbial fuel cell-based constructed wetlands ranges between 50 and 86 mW/m2 in Bangladesh (integrated tidal flow) and 852 mW/m3 in China (vertical flow), and the production of bioenergy has been evidenced up to 1,836.5 GJ/hector/year. Annually, wastewater treatment plant systems (WTPs) generate around a hundred times more Methane (CH4), Nitrous oxide (N2O), and carbon dioxide (CO2) than CWs. In metropolitan cities, WTPs may lead to a considerable increase in upstream land use, which could be minimized by promoting CWs in these areas. The potential utility of different CWs in protecting and preserving estuarine quality within the present regulatory framework is finally addressed in the study, emphasizing that it can balance the impacts of industrial expansions in developing countries for subsequent mitigation and adaptation to climate change.
1 Introduction
Insufficient access to safe water has become one of the pressing concerns impacting human health in developing nations, and water deficiencies are predicted to intensify in the future decades (Stavi et al., 2021). Developing nations are classified based on their annual Gross National Income (GNI) per capita. Developing countries have a GDP of $11,905 or less (World Bank, 2012). More than 780 million people in the world in 2010 were using unsafe water for drinking, according to a study from the World Health Organization and UNICEF (2013). Even more severe is the problem of poor sanitation. Sub-Saharan Africa and Southern Asia account for the vast majority of the world’s population. Nevertheless, sanitation coverage remains below 50% in both regions, leaving approximately 2.5 billion people without access to better sanitation facilities. Millions of people every year, including 3,900 children, succumb to illnesses that are spread by ingesting contaminated water or coming into contact with human waste (Klein, 2022). Many emerging nations have overexploited water sources (such as groundwater) that are simple to develop from a geological and technical standpoint. This has led to a shortage of available safe water despite increased demand (Boretti and Rosa, 2019). Potentially negative environmental consequences of this short-term approach include saltwater intrusion and ecosystem decline (Markandya, 2022). Additionally, many cities in the developing world have slacked in developing and managing sewage treatment systems. Wastewater treatment is often ranked toward the bottom of the list of proposed priorities in improvements (Caban and Stepnowski, 2021). As a direct consequence, many developing countries routinely discharge significant volumes of untreated wastewater straight into the streams and lakes (Čegar et al., 2022). In demand for low-cost and imperishable wastewater treatment systems in developing countries, ecologically friendly technologies like constructed wetlands for wastewater treatment offer novel and emerging solutions for environmental conservation and restoration that are designed to treat wastewater that includes a variety of treatment modules that closely resemble physical, chemical, and biological processes (Manikandan et al., 2022). CWs have been effectively utilized to reduce pollution by eliminating a broad range of contaminants from wastewater, including organic chemicals, suspended particles, pathogens, metals, and nutrients (Bhatt et al., 2022), in addition to pharmaceutical and personal care solutions (Priya et al., 2022).
Two of the foremost concerns to sustainable development throughout the world, especially in developing nations, are the energy crisis and global warming. The use of different engineered wetland types, such as those used to produce biofuels, as an alternative to fossil fuels is something to keep an eye on. Microalgae (Song et al., 2022) and low-input high-diversity (LIHD) (Ottoy et al., 2022) are only some of the methods used to lessen the need for nitrogen. The recycling of waste nitrogen is an appealing notion explored via microalgae biofuel trials (Ummalyma et al., 2021); however, current microalgae systems are still hampered by their modest production scale due to sophisticated technology (Ali et al., 2022). As a result, other techniques to make better use of waste nitrogen are required. To treat wastewater, engineers create wetlands, which are artificial ecosystems comprised of plants and rhizosphere bacteria (Xu et al., 2016). Around the world, thousands of large-scale artificial wetlands are used to combat worsening wastewater contamination. As an alternative to Wastewater Treatment Plant Systems (WTPs), which rely on physical, chemical, and biological (microorganism-based) processes to purge wastewater of contaminants, the constructed wetland has emerged as a viable treatment option (Das and Kumar, 2022). The WTP is still the preferred method for centralized, highly efficient treatment in densely populated regions with significant energy usage and Green House Gas (GHG) emissions. On the other hand, constructed wetlands are less expensive and offer more ecosystem services such as carbon sequestration, biodiversity protection, and aesthetics (Ge et al., 2007; Xu et al., 2016; Saeed et al., 2022). In addition, Microbial fuel cells (MFC) are effective additions in the constructed wetland that incorporates new energy-producing enhanced wastewater treatment methods derived from conventionally planned plant and media-based wetland systems (Saeed and Miah, 2021; Saeed et al., 2022). Electrochemical oxidation of organic molecules occurs in the anaerobic anode compartment of a microbial fuel cell-based engineered wetland (Saeed et al., 2022). When oxygen is present, the reactions at the anode are completed when electrons are accepted and transmitted to the cathode electrode (Chai et al., 2021). Classical denitrification and other methods for ridding the environment of microbiological contaminants benefit from the electrons created in this process (Sari et al., 2022). Wastewater reclamation in open systems (such as a lagoon, pond, or lake) has gotten a lot of emphasis in recent years, and self-buoyant hydroponic root CW mats have been at the center of this interest (Colares et al., 2020). Improved mitigation capability of FTWs may be attained via the addition of specialized microbes and the proper selection of macrophytes. Therefore, Nawaz et al. (2021) demonstrated that textile wastewater was effectively cleaned up by bioaugmentation of plant-growth-encouraging and pollutant-degrading bacteria. As a corollary, a favorable correlation was found between the effectiveness of FTWs and the high persistence of inoculation bacteria in the wetland plant’s water, root interior, and shoot interior. Total dissolved solids, total suspended solids, chemical oxygen demand, biochemical oxygen demand, electric conductivity, color, and hazardous metals in dye-polluted wastewater were effectively decreased, especially with the improvement of rhizospheric and endophytic bacteria. Furthermore, the toxicity of the textile effluent was reduced, and plant development was enhanced, both of which contributed to the plants’ enhanced resistance to the harmful effects of pollution. In light of this, Fahid et al. (2020) showed that diesel oil in FTWs might be better cleaned up by a combination of plant- and hydrocarbon degrading bacteria.
To improve water quality, constructed wetlands utilize natural processes, including wetland plants, soils, and their associated microbial communities (Barancheshme and Munir, 2018). These systems have gained popularity because of their user-friendliness, low price, and high productivity (Fang et al., 2017; Tilak et al., 2017). Several plant species, including Canna, Eichhornia, Phragmites, Typha, and Ageratum, have shown promise in the treatment of wastewater, the elimination of heavy metals, and the regulation of antibiotic-resistant genes in constructed wetlands (Jamwal et al., 2021; Rampuria et al., 2021). Because of their high removal efficiency, low cost, simple operation, ability to produce energy, and substantial potential for reusing water and nutrients, CWs have grown to be an increasingly attractive option for wastewater treatment (Kadlec, 2009; Saeed and Miah, 2021). However, insufficient information regarding the use of CWs in developing countries is available yet, and the acceptance of the CW system has been unexpectedly sluggish (Jiang et al., 2022). However, certain potentials and amazing applications have been demonstrated in Saeed et al. (2022) studies in Bangladesh for making natural treatment offer great solutions to the ever-increasing pollution phenomenon. Considering that CWs have proliferated in the last decade, this study aims to offer a complete overview of the wide variety of practices, uses, and investigations of constructed wetland systems for eliminating different pollutants from wastewater in developing countries leading to placing them in the context of combating climate change impacts, environmental resource planning, and sustainable wastewater treatment systems.
2 Manuscript formatting
2.1 Constructed wetlands in developing countries
Over the last several decades, CWs have emerged as a popular option for wastewater treatment since these are viable alternatives to more conventional methods. Its potential to significantly increase water recycling rates, simplicity of operation and maintenance, minimum energy requirements, ability to offer electricity production, and significant wildlife habitat are all major contributing factors (Manikandan et al., 2022). Although CWs have been used mainly to treat domestic wastewater in impoverished nations (Mburu et al., 2013), CWs are increasingly being utilized in recent times to treat many types of wastewater, including industrial wastewater (Maine et al., 2007; Saeed et al., 2012), liquid wastewater from agricultural farms (Licata et al., 2021), river water (Zhang et al., 2021), effluent from sludge (Ahmed et al., 2008), the flow of rainwater during a storm (Ávila et al., 2013), wastewater from hospitals (Shrestha et al., 2001), liquid waste produced in laboratories (Khalish et al., 2022), and drainage water from agricultural farms (Yang et al., 2008). Most countries in need may be found in warmer, tropical, or subtropical climates. It is generally agreed that CWs operate better and are more cost-effective for treating wastewater in these types of environments (Developing countries) than in colder climes (Abdel-Shafy et al., 2022). Due to sustained plant growth and enhanced microbial activity, a warmer environment improves treatment success (Kaseva, 2004). Better plant production all year round and a shorter time necessary for microbial biodegradation are characteristics of tropical wetland ecosystems because of their exposure to higher temperatures and constant sunlight. This leads to more effective removal of pollutants (Zhang et al., 2014).
2.2 Types of CWs
There are different kinds of CW treatment systems; however, the three main ones are free water subsurface (FWS), subsurface flow (SSF), and hybrid CWs (Kadlec and Knight, 1996). Based on the direction of flow, SSF CWs are further subdivided into vertical subsurface flow (VSSF) and horizontal subsurface flow (HSSF) systems. The components of treatment, the location, the cost, the available space, and the treatment goals all have a role in determining the flow regime (Horner et al., 2012). Sections 2.2.1 through 2.2.4 provide an overview of the mentioned types of CWs in different applications published in prominent research works.
2.2.1 Free water surface (FWS) CWs
In free water surface (FWS) type of CWs, which are essentially shallow basins filled with water, the treatment processes occur due to the complex interactions between the plants and the associated biofilms in the liquid phase (Kadlec and Wallace, 2008). It has been shown that free water surface constructed wetlands (FWS CWs) may remove several harmful substances from water (Manikandan et al., 2022). The therapeutic effectiveness and claimed operational features of FWS systems are summarized in Table 1. Microbial degradation and filtration processes may allow the FWS CWs to effectively remove suspended particles and organics (Kadlec and Wallace, 2008). In FWS CWs, removal rates of more than 70% are often achieved for total suspended solids (TSS), chemical oxygen demand (COD), 5 days-biochemical oxygen demand (BOD5), and pathogens (Kadlec and Wallace, 2008). Nitrogen removal efficiency typically varies between 40 and 50% (Vymazal, 2007), despite the fact that these fluctuations greatly depend on a variety of criteria such as input concentration, forms of nitrogen species, dissolved oxygen content, organic carbon availability, season, and water temperature (Kadlec, 2009). FWS CWs have a range of 40%–90% phosphorus removal efficiency, this still being a sustainable rate since phosphorus removal is more difficult in FWS systems because of the low depth of the water table (Vymazal, 2007).
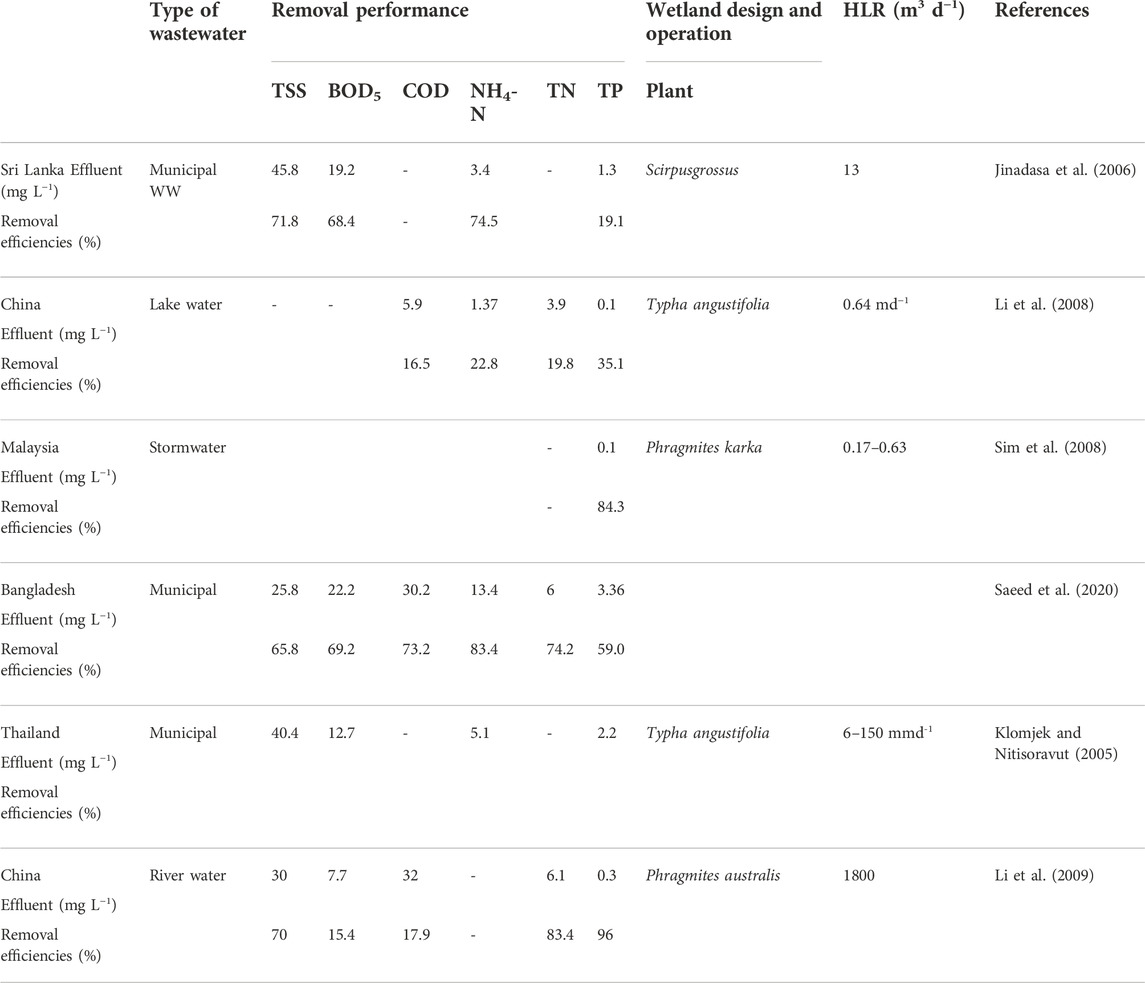
TABLE 1. An overview of the FWS systems’ operating characteristics and treatment performance in Asian countries.
Current evidence suggests that the rates at which TSS (66.1% of samples) and BOD5 (65.34%) are being removed are subpar. Research showed that FWS CWs were the least successful in removing COD (removal efficiency of 44.9%). Furthermore, it was shown that free water surface CWs were capable of effectively filtering out the water of nitrate (NO3-N) (51.63%), ammonium (NH4-N) (60.87%), and total nitrogen (TN) (43.1%). Table 1 displays the results of assessed studies, showing that the percentage of total phosphorus (TP) removed from the environment ranges from 19.5% to 96%.
2.2.2 Subsurface flow (SSF) systems
Systems that use subsurface flow (SSF) are planned for subsurface flow in either a horizontal or vertical direction via a permeable material (Gravel, sand, or crushed rock) (Dittrich et al., 2021). There are two types of sub-surface flows: HSSF and VSSF. HSSF constructed wetlands configurations are used as the most popular systems. While VSSF constructed wetlands systems are becoming increasingly common, more operational data is available for HSSF wetlands (Vymazal, 2007). Table 2 offers a summary of the operational characteristics and treatment efficiencies of HSSF CWs in Asian countries. The current analysis indicates that removal efficiencies for BOD5 are 75.10% for HSSF and 89.29% for VSSF based on this analysis, whereas for COD, those are 66.02% (HSSF) and 64.41% (VSSF). When it comes to treating municipal sewage, SSF CWs often showed excellent treatment effectiveness of BOD5 and COD, as shown by a global study conducted by Puigagut et al. (2007). Results for biological oxygen demand (BOD5) reduction were between 75% and 93%, while those for chemical oxygen demand (COD) removal ranged from 64% to 82%. Since refractory constituents are not often present in high amounts in domestic wastewater, the majority of organics in this stream are relatively pliable and straightforward to break down (Doğan et al., 2022).
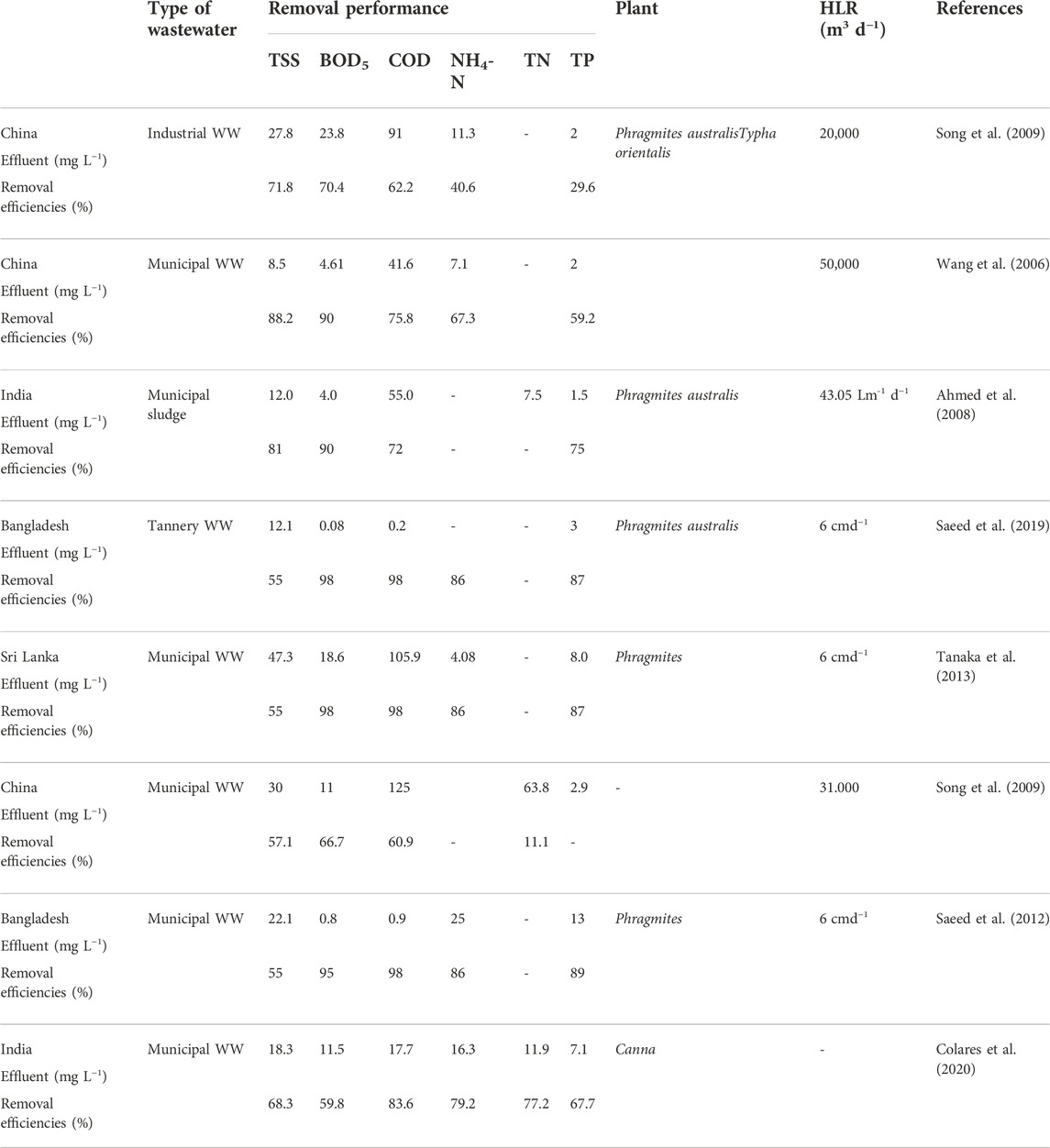
TABLE 2. An overview of the HSSF systems’ operating characteristics and treatment performance in Asian countries.
Since VSSF CWs are infrequently loaded and carry unsteady flow, which results in an increased transfer of oxygen to the filter media compared to HSSF CWs, those are generally predicted to perform better than the HFCW CWs for the reduction of BOD5 (Kadlec and Wallace, 2008). In comparison with HSSF CWs (removal performance of 79.93% for TSS, 75.1% for BOD5, and 66.02% for COD), VSSF CWs exhibit excellent removal performance for COD (66.14%), BOD5 (89.29%), and TSS (85.25%), respectively. In a statistical comparison utilizing the student’s t-test, we found no statistically significant differences (p > 0.05) between the VSSF and HSSF CWs in their ability to remove TSS, BOD5, and COD. These findings are comparable with those of Caban and Stepnowski (2021) who employed a recirculating aquaculture system in the Mekong Delta of Vietnam to clean fishpond water using CWs comprised of HSSF CWs and VSSF CWs propagated with Oreochromis niloticus and Cyprinus carpio. While HSSF CWs usually have a low capacity for nitrifying ammonia, they offer perfect denitrification circumstances. The current meta-analysis found no statistically significant difference (p > 0.05) between VSSF CWs’ removal of NH4-N (61.20%) and HSSF CWs’ removal of NH4-N (53.52%). To treat high-strength wastewater from 80 homes (400 people equivalent) in Nepal, Singh et al. (2009) studied the effectiveness of a model for decentralized wastewater treatment employing a hybrid system consisting of HSSF and VSSF CWs. It has been observed that both HSSF (51.97%) and VSSF (50.55%) CW systems are somewhat effective for removing total nitrogen (TN). TN removal ranged from 40% to 55%, depending on the CW type and influent loading. The amount of TN removed from the wastewater was also shown with HSSF CWs, which were also more effective in removing TP (65.96%) than VSSF CWs (59.61%) (Vymazal, 2007) (Table 2).
A comparison of their respective treatment performances was conducted to evaluate the FWS and SSF system’s potential for cleaning up the eutrophic water in China’s Taihu Lake (Li et al., 2008). The growth of Typha angustifolia in three parallel pilot-scale CWs (a VSSF, HSSF, and FWS CW) was analysed. The treatment effectiveness for the primary parameters in the FWS CW was found to be inadequate (16.5% for COD, 22.8% for NH4-N, 19.8% for TN, and 35.1% for TP), especially in comparison with VSSF and HSSF CWs, which has shown statistically better potential for COD removal (39.6% for HSSF and 40.4% for VSSF CW), NH4-N removal (45.9% for VSSF and 32.0% for HSSF), TN removal (52.1% for HSSF and 51.6% for VSSF CW), and removal of TP (64.3% for VSSF CW, and 65.7% for HSSF). The water quality of the polluted Xinyi River in Jiangsu Province, China, was improved via an integrated system developed by Ruan et al. (2006) that included FWS and VSSF CW planted with Typha latifolia and Scirpus lacustris. The scientists showed that the VSSF CW removed COD (77.38%) more effectively than the FWS CW (61.1% for COD).
2.2.3 Floating treatment wetland (FTW)
Instead of being rooted in the sediments, the rooted, emergent Macrophytes employed in floating treatment wetlands (FTWs) grow on a floating mat (Headley and Tanner., 2012). One of the key benefits of FTWs over traditional sediment-rooted wetlands is their capacity to deal with changing depth of the water that is characteristic of event-driven stormwater systems (Kerr-Upal et al., 2000). In addition, this quality allows the FTW system to be fashioned as a prolonged detention basin, whereby massive runoff events may be absorbed and distributed gradually over the course of the following days. Table 3 provides an overview of the FTW systems’ operating characteristics and treatment performances as applied in Asian countries. In comparison to Free water surface (FWS) CW systems (65.34% for BOD5, 44.9% for COD, 43% for TN, and 49.16% for TP), FTW systems exhibited improved removal efficiencies for these parameters (79.31%, 55.20%, 73.45%, 62.45%, and 49.58%, respectively). Compared to a wetland with sediment-rooted plants, it is possible that plants in an FTW system absorb more nutrients and other substances (such as heavy metals) (Headley and Tanner., 2012). Because the roots of the plants in an FTW never touch the benthic sediments or soil, they must rely on the nutrients in the floating mat and the surrounding water (Kadlec and Wallace., 2008). In an FWS CW or other sediment-bound wetland, plant roots reach down into the soil to draw nutrients, but in this case, the water is the only source of water and nutrients for the plants. Roots, rhizomes, and the hanging root biofilm network provide a biologically active surface area below the floating mat for the biochemical transformation of pollutants and physical activities, including filtering and entrapping particles (Li et al., 2009).
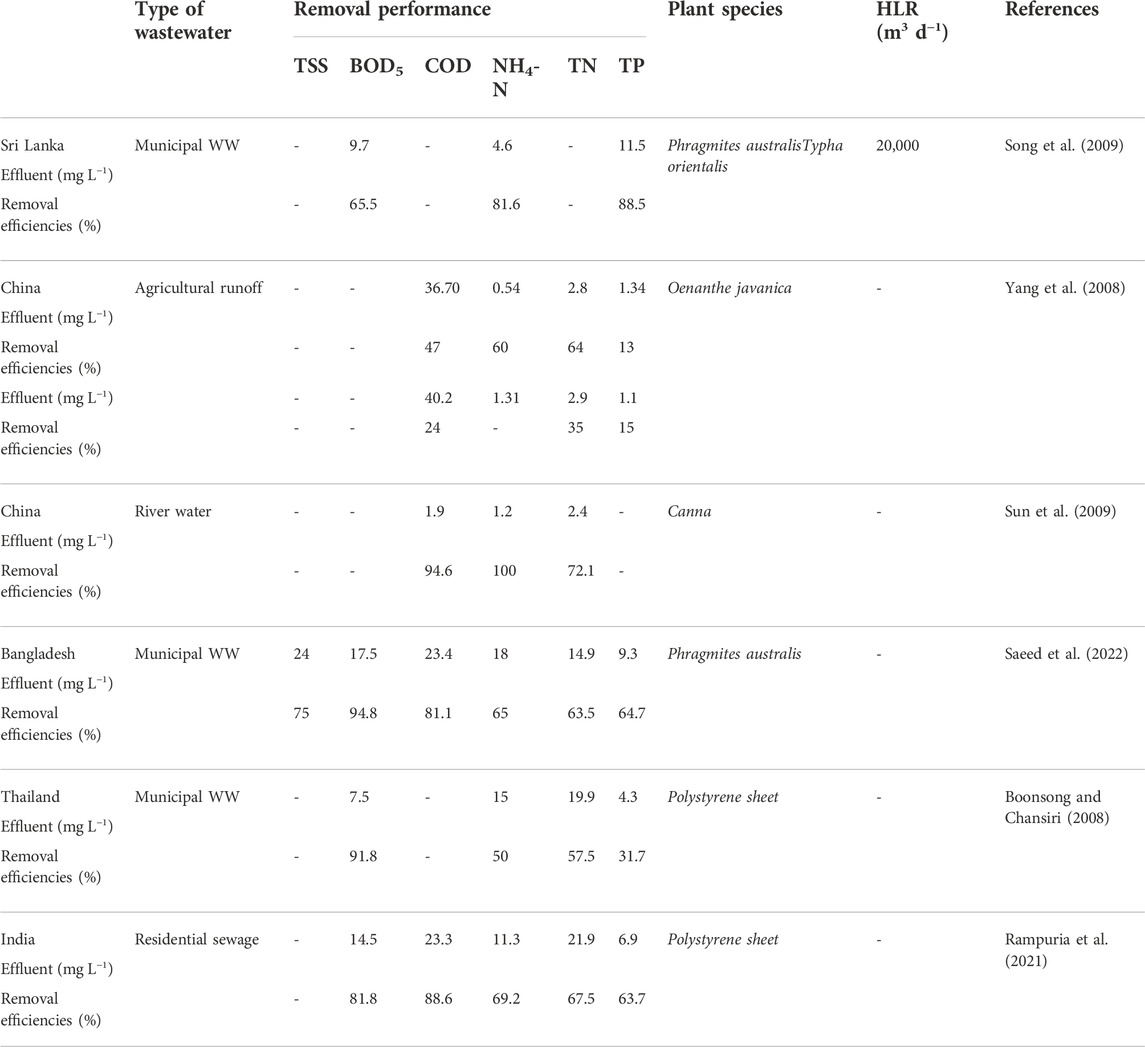
TABLE 3. An overview of the FTW system operating characteristics and treatment performances in Asian Countries.
As of now, FTWs have been implemented in ornamental ponds and lakes to better water quality, enhance habitat, and enhance aesthetics (Headley and Tanner, 2012). In Bangkok (Thailand), Boonsong and Chansiri (2008) investigated the effectiveness of V. ziznnioides (L.) Nash grown using the floating platform procedures for treating household wastewater at three different HRTs (3, 5, and 7 days). For total nitrate-N (TN) and ammonium nitrogen (NH4-N), the average removal efficiencies were 9.97%–62.48% and 13.35%–58.62%, respectively. Based on the data, it was determined that 7 days of HRT resulted in the highest removal efficiency for BOD5 (90.5–91.5%), TN (61.0%–62.5%), and TP (17.8%–35.9%). Yang et al. (2008) in China studied the efficacy of an FTW hydroponic system for cleaning up a nitrate-rich agricultural runoff with influent TN and TP concentrations of 3.8–7.9 gm/m3 and 1.2 to 1.5 gm/m3, respectively. Maximum removal efficiencies of 17%–47% for COD and 8%–15% for TP were observed. The removal efficiencies for total nitrogen (72.1%), nitrogen oxides (75.7%), nitrates (95.7%), and chemical oxygen demand (94.6%) were all reported by Sun et al. (2009), who studied the removal of TN from polluted river water (inlet concentration of 8.7 gm/m3) in Guangzhou, China.
2.2.4 Hybrid system
Single-stage CWs are limited in their capacity to remove TN since those cannot provide both aerobic and anaerobic conditions at the same time (Vymazal, 2007). As such, it is possible to mix distinct CW types to maximize the benefits of each. Hybrid systems, which consist of a sequence of different kinds of artificial wetlands, have been established because of the difficulty of treating several wastewater in a single-stage system. The majority of hybrid setups are composed of a staggering combination of VSSF and HSSF systems (Dittrich et al., 2021). Table 4 illustrates an overview of hybrid systems’ operational characteristics and treatment efficiency. Four of the eight investigated hybrid systems were established to treat municipal sewage, while the other hybrid systems were created to treat lake waters, hospital wastewaters, laboratory wastewaters, and up-flow anaerobic sludge blanket reactor effluent, among other forms of wastewater. The removal performance of TSS (93.82%), BOD5 (84.06%), COD (85.65%), and NH4-N (80.11%) was highly successful in the eight hybrid systems evaluated, whereas the removal performance of TP (54.75%), NO3-N (63.50%), and TN (66.88%) was moderate and reliable. The removal efficiencies for TP (99%) and TN (31.9%–91%) were very variable, nonetheless, and were dependent on the system architecture, HLR, and species of plants.
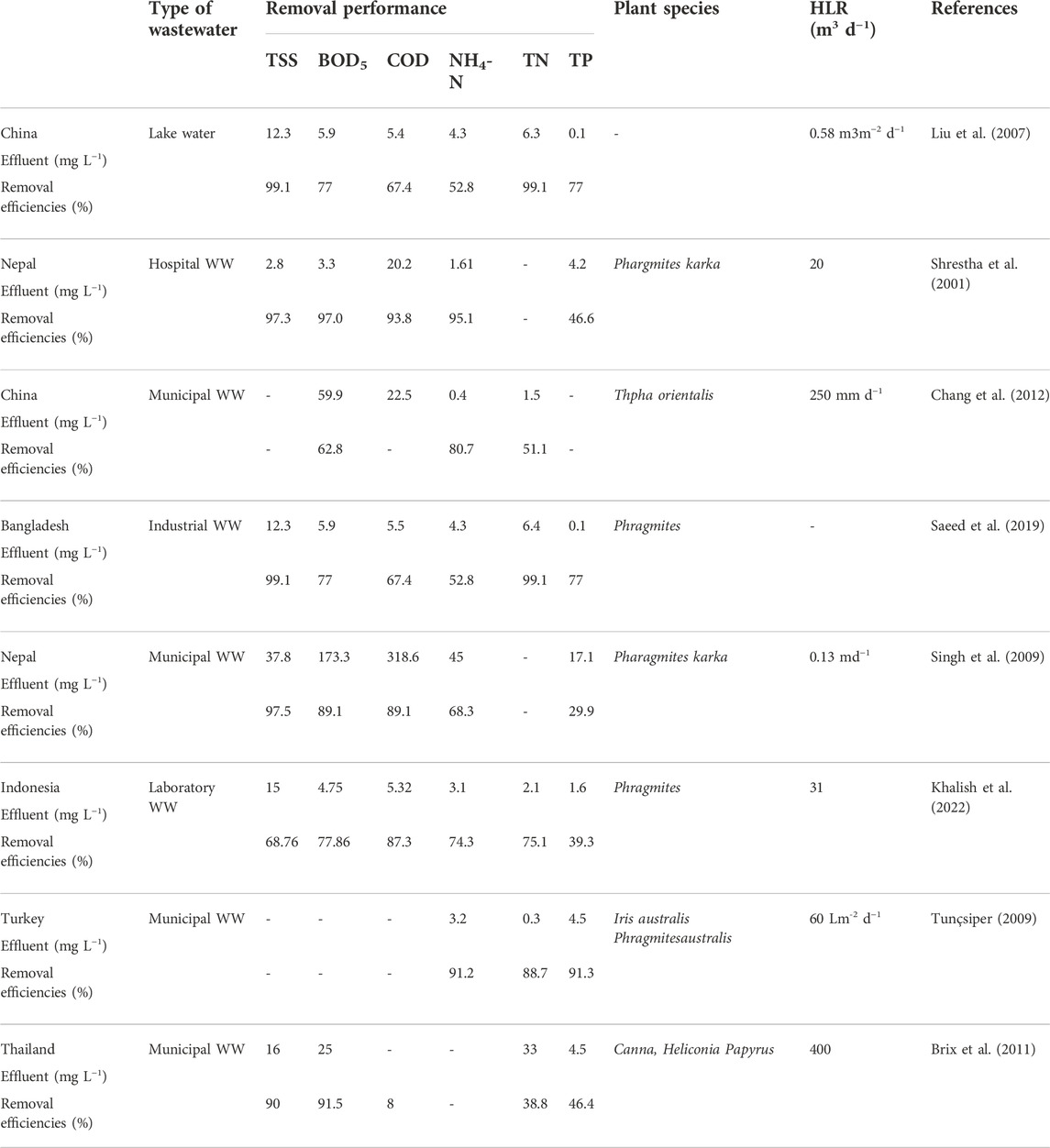
TABLE 4. An overview of the hybrid systems’ operating characteristics and treatment performances in Asian countries.
A large-scale hybrid CW system was envisioned and assembled in Nepal to treat hospital and grey sewage. It had a three-chamber septic tank, a 140-square-meter HSSF bed (at 0.75 m depth), and a 12-square-meter VSSF bed (1 m depth) (Shrestha et al., 2001). Shrestha et al. (2001), who monitored the system for a full year, found that during the studied time, it consistently showed high treatment efficiency. In Nepal, Singh et al. (2009) assessed an approach for decentralized wastewater treatment employing a hybrid CW system to deal with high-strength effluent from 80 residences (400 people). In this study, wastewater was first treated in an anaerobic baffled reactor, then in two HSSF CWs, and finally in two VSSF CWs that were planted with Phragmites Karka and Canna Latifolia to perform as secondary treatment. The authors reported the removal rates of these contaminants from the water system to be 96% for TSS, 90% for BOD5, 90% for COD, 70% for NH4-N, and 26% for TP, respectively (Singh et al., 2009), suggesting that this hybrid system was rather successful. Hybrid CWs may often have an FWS stage in addition to the HSSF to VSSF and VSSF to HSSF systems (Vymazal, 2007). Surrounded by hotels, restaurants, and stores on Koh Phi, a popular tourist destination in Southern Thailand, Brix et al. (2011) detailed the planning, construction, and operation of a CW system. Units of the VSSF, HSSF, and FWS were all a part of the treatment process training. The input was found to be chemically similar to sewage from homes, although at a higher concentration than expected (due to the lack of pre-treatment). In terms of total suspended solids and total phosphorus, the system’s effluent was within the acceptable range for Thailand; however, the BOD5 contents were somewhat higher than the effluent limit (average of 25 mg L−1). For the treatment of river or lake water, hybrid systems consisting of FWS CWs and SSF CWs have also been proven to be effective. At a wastewater treatment facility in Hangzhou City, Zhejiang, China, Xiong et al. (2011) investigated the effectiveness of an integrated CW in removing nitrogen from the secondary effluent. Vetiver Zizanioides was planted in a VSSF CW, and Coislacryma-Jobi was seeded in an FWS CW in this system. The findings revealed that the integrated CW had excellent potential for N removal and exhibited superior removal efficiency for NH4-N (95.60%) and TN (92.41%).
In summary, four different types of constructed wetland have been assessed in terms of natural treatment approaches for wastewater in developing countries. Moreover, the present review also compared their efficiency and observed that hybrid constructed wetland system performed significantly better compared to the other wetland systems.
2.3 Overall performance of CWs in the context of developing countries
According to the current review, compared to the other configurations of CWs, the elimination of TSS (93.82%), COD (85.65%), NH4-N (80.11%), and TN (66.88%) seems to be most effective when using a hybrid system for municipal wastewater. The percentage of TP that was eliminated by HSSF (65.96%) and VSSF (59.61%) CWs was higher than that of FWS (49.16%), hybrid (54.75%), and FTW (49.58%) CWs. When compared to FWS CWs (65.34%), HSSF CWs (75.1%), hybrid systems (84.06%), and FTW (55.29%), VSSF systems had the highest BOD5 (89.29%) elimination. The average BOD5 removal efficiencies for FWS CWs, HSSF CWs, VSSF CWs, and hybrid systems in developing nations were obtained at 65.34, 75.10, 89.29, and 84.06%, respectively. In contrast, the efficiency of European treatment wetlands was 79.1% (Haberl et al., 1995). According to available data, the majority of treatment wetlands in developing nations have similar performance to those in Europe. On the other hand, most CW systems in developing nations performed moderately when compared to the average COD removal efficiency in Europe (69.5%), with removal efficiencies of 44.90% for FWS CWs, 66.02% for HSSF CWs, 66.41% for VSSF CWs, and 85.65% for hybrid systems. In terms of industrial wastewater, removal efficiencies of TSS in Shandong, China, were 71.8% for HSSF CW and 99.1% for hybrid systems in Bangladesh, which was considerably better than Europe (30.3%) (Haberl et al., 1995). The BOD5 removal efficiency was 70.4% for HSSF CWs in China, and 77% for hybrid systems in Bangladesh, which are better than the average BOD5 removal rate of 39.61% for European treatment wetlands (Haberl et al., 1995). To the same end, the average removal efficiencies for COD in treatment wetlands in China were found to be 62.2% for HSSF CWs and 67.4% for hybrid systems in Bangladesh. This conspicuously indicates that the system of wetlands performs in a more lucrative way in Asian countries for industrial wastewater treatment.
2.4 Impacts on power generation from CWs
Table 5 provides information about the results of a comparative assessment of studies that employed CW-MFCs to produce electricity. Electrodes were placed initially in the CW’s aerobic and anaerobic zones, leading to the development of the first combined CW-MFC system (Zhang et al., 2014).
The current performance of a microcosmic floating macrophyte-oriented ecosystem linked with fuel cells with a capacity of 224.9 mA/m2 achieved 86.7% COD removal and 72.3% volatile fatty acids removal reported by Mohan et al. (2011). One more vertical flow, a CW-MFC, was established and implemented by Yadav et al. (2012) in India for the treatment of effluent-containing dye, with results including a power density of 15.7 mW/m2 and a removal efficiency of 74.9%. Using the root exudates of Ipomoea Aquatica as fuel, Liu et al. (2013) introduced a built wetland combined with MFC based on the fundamentals of photosynthetic MFC. They were able to increase the power density of the CW-MFC planted using I. Aquatica by 142%, reaching a maximum of 12.42 mW/m2 compared to the 5.13 mW/m2 generated from the CW-MFC without plants (Liu et al., 2013). It has been reported that treating initial wastewater in continuous up flow in a CW-MFC system results in a power density of 9.4 mW/m2 and a 76.5% COD elimination (Zhao et al., 2013) (Table 5). Fang et al. (2013) established another CW-MFC and utilized it to process wastewater containing dye with an initial COD content of 180 mg/L, resulting in a power density of 302 mW/m3 and 86% removal for COD in China. For the treatment of wastewater containing azo dyes, Fang et al. (2015) discovered that an MFC-coupled built wetland system achieved a power density of 852 mW/m3 and a COD removal of 72.5%.
In order to treat wastewater, Saeed et al. (2022) established a CW-MFC with a vertical flow, which allowed them to remove 80%–100% of COD at a maximum power density of 86 mW/m2. The Alum Sludge’s feasibility of CW-MFCs for treating swine slurry wastewater and generating power was evaluated in another study (Doherty et al., 2015). In this experiment, the slurry wastewater was treated to a maximum power density of 268 mW/m3 and a maximum COD removal of 64%. Using CW-MFCs for cogeneration of electricity and wastewater treatment, Doherty et al. (2015) investigated the impact of electrode spacing and wastewater flow direction. When wastewater flowed upwards into the anode and downwards into the cathode simultaneously, 276 mW/m3 of electricity was generated. In order to cleanse wastewater and generate energy simultaneously, Oon et al. (2015) developed an up-flow constructed wetland-microbial fuel cell (UFCW-MFC). The investigation found that the highest power density was 6.12 mW/m2, with 100% COD removal, 40% NO3–removal, and 91% NH4+ removal efficiency.
2.5 A potential biofuel production scenario
Table 6 depicts a comparison of production in terms of biofuel ecosystems. According to the present review, Liu et al. (2012) have the highest potential efficiency through a constructed wetland system of all the biofuel plant species analyzed, with a maximum of 1,836 GJ ha−1yr−1 (Table 6). Some biofuel plant species have also been published to have high potential productivity; for example, Napier grass (Pennisetum purpureum) has reported productivity of 1,628 GJ ha−1yr−1 and 1,850 GJ ha−1yr−1 from Echinochloa Polystachy in tropical zones (Somerville et al., 2010). Although high biomass production is not a primary concern in wastewater treatment, the majority of constructed wetlands have average productivity, the microalgae system. Nevertheless, by using discharged waste nitrogen, adjusting hydrologic flow behavior, and adopting competitive plant species, the bioenergy production of constructed wetlands could be significantly boosted. Several aspects need to be taken into account for constructed wetlands to be an efficient and considerable Biofuel producing system (Agusdinata et al., 2011). Constructed wetlands demonstrated to achieve a greater mean net energy balance (NEB) (253 GJ ha−1yr−1) than the other five biofuel production systems (switchgrass, corn, microalgae, LIHD grassland, and soybean) during their entire life (NEB = energy output-input). Constructed wetlands also had the highest NEB ratio (4.29) of the five systems, surpassing even the corn/soybean (Hill et al., 2006) and algae systems because of their relatively large energy input (76.9 GJ ha−1yr−1) (Clarens et al., 2010). Constructed wetlands still hold significant promise as a sustainable biofuel production method when both NEB and NEB ratios are taken into account.
According to the Intergovernmental Panel on Climate Change (IPCC), the short-cycle CO2 produced during the breakdown of organic matter in wastewater in constructed wetlands does not contribute to the greenhouse effect. Although in this study, CO2 sequestration was determined to be an average of 31 Mg ha−1yr−1 due to the collection of organic matter from wastewater and plants (Table 1). Because of the high nitrogen content, N2O emission from wetlands (3.76 Mg CO2 equivalent to ha−1yr−1 on average) is 710% from corn/soybean, 1,800% from LIHD grassland, and 4,600% from microalgae. While grassland and corn/soybean act as sinks for methane, wetlands and algal systems act as sources (18.71 Mg CO2 equivalent ha−1yr−1 CH4 from engineered wetlands, 1,700% from microalgae). Overall, in comparison to the Water treatment plants (WTP), constructed wetlands only released 0.08%, 3.4%, and 7.7% of CH4, N2O, and fossil fuel CO2, respectively, on an annual basis (Liu et al., 2012).
2.6 Impact implications from treatment purposes and scenario analysis
Table 7 illustrates the estimated volume of treated wastewater in different developing countries. Using T. Angustifolia (a species of plan), Klomjek and Nitisoravut (2005) introduced a built, constructed wetland that treats wastewater from Lake in Thailand. The system processed over 2000 m3 of wastewater (approximately), with the wastewater, flow in each created wetland initially set at 3 m3/day and held unchanged throughout the study period, leading to a hydraulic loading rate of 6 cm/day. Using the Phragmites karka (a species of plan), Dhulikhel hospital has established a constructed wetland system in Nepal, which treats wastewater from the hospital. The system was designed to treat 20 m3/day of wastewater. During the design phase, it was assumed that the hospital capacity was 60 beds and 250 people were using the hospital on a daily basis. The Dhulikhel Hospital constructed wetland system consists of a three-chambered septic tank with a volume of 16.7 m3. The area of the horizontal bed and the vertical bed is 140 m2 and 120 m2, respectively. Both beds were planted with Phragmites karka, a local variety of reeds readily available and able to be treated nearly 500,000 m3 of wastewater in 5 years. The system does not need any electric energy as the wastewater is fed hydro-mechanically into the beds. It was estimated that roughly US$ 50,000 could be potentially saved after using the constructed wetland instead of using a wastewater treatment plant (Poh, 2003). Pilot-scale wetland system consisting of a series of stabilization ponds, HSSF CWs, with T. Angustifolia, and Chrysanthemum cineraria folium was implemented by Belmont et al. (2004) in the small community of Santa Mara Nativitas to promote the wastewater treatment and reuse of domestic wastewater as a water management solution in the Rio Texcoco watershed in Mexico. A flow rate of two lectures per minute was used in the treatment system, which resulted in a hydraulic retention time (HRT) of 2.3 days in each SSF CW. The daily average treated wastewater volume was 2.88 m3 per day. CW wastewater treatment facilities have been demonstrated to have a less environmental footprint than their centralized counterparts in previous research (Austin and Nivala, 2009). In addition, it has been demonstrated that decentralized wastewater treatment facilities use less energy and produce less carbon dioxide than their centralized counterparts (Chen et al., 2011; Shao et al., 2013). Furthermore, CWs are preferred to centralized wastewater treatment facilities because of the various ecological advantages they provide, including the maintenance of biodiversity and the sequestration of carbon (Shaharuddin et al., 2013). However, significant concern continues to remain as an issue regarding the fact that expanding CWs’ deployment may raise tremendous demands on the availability of land resources.
Present study reviews a number of the study conducted in China to treat urban wastewater discharge and treatment volume since 2010 represented in Figure 1 as a case study to assess the land requirement aspects.
The China Environmental Statistics Yearbook reports that by the end of 2017, cities in China had constructed a total of 2,209 urban centralized wastewater treatment plants with a wastewater treatment capability of 157.43 million m3 per day (CSP, 2018). To recapitulate, while treating 1 m3 of wastewater, the CW uses 0.05 m2 less space than the centralized WTS. As a result, it is possible to make a rough estimation that a massive amount of land usage caused (about 2.17 billion m2) may be eliminated if CWs substituted all centralized urban WTSs in 2017 (Fan et al., 2021). Figure 2 shows the amount of land conserved by each provincial area and the land saved per capita by the province in 2017 if Chinese urban WTSs were substituted with CWs. Due to the growing trend of blurring of physical borders between towns and provinces and the formation of a cross-division of the manufacturing chains, it is essential to remember that a process physically located in a city/province may require power, materials, and instrument supplies from the remaining of the nation in general. As a result, the enormous onsite space needed to promote CWs in metropolitan areas is severely constrained. While it may seem at first glance that centralized WTSs need less onsite acreage than CWs, Fan et al. (2021) found that widespread deployment of WTSs in municipalities may cause a significantly more amount of land usage in upstream operations, which might put further strain on the accessible land resources.
2.7 Mitigation of climate change impacts by natural treatment
One billion people still use open defecation, and 80% of wastewater is directly dumped into the environment without treatment worldwide (Wastewater, 2017). Human health, ecological balance, energy consumption, and GHG emissions are just a few facets of wastewater treatment. Modern wastewater treatment facilities seem essential but are undoubtedly expensive, complicated, centralized and need a lot of power and specialized workers to run and maintain (McCarty et al., 2011). As a result of its energy-intensive nature, conventional wastewater treatment has been mostly phased out. Approximately 3% of the total power used in the United States is used for wastewater treatment (Conservation, 2006). There is an energy cost of up to 2.2 MJ (Megajoules) per cubic meter of water treated in municipal wastewater treatment facilities (McCarty et al., 2011). Instead of using expensive and high-maintenance conventional methods, many people have turned to constructed wetlands (CW) as a cost-effective and low-maintenance option for treating wastewater in centralized and decentralized settings (Zhang et al., 2014). This environmentally friendly technology uses the root zones of wetland Macrophytes to treat various wastewater through biological and physical processes (Kadlec and Wallace, 2008).
CWs are low-cost options for treating a wide range of wastewater types in underdeveloped nations, and those are rightfully earning their reputation as a tried-and-tested wastewater treatment technology. There was a significant difference in BOD5 removal efficiency between VSSF systems and other CWs, with the former producing an 89.29% improvement. In every metric studied, FTW systems accomplished better than FWS CWs, proving to be an innovative new approach to therapy. By 2035, it is estimated that biomass energy, a kind of renewable energy, may provide up to 50 EJ (Exajoules, 1018 J), or almost 10% of the world’s energy consumption (Slade et al., 2014). Nevertheless, terrestrial energy crops would be the principal source of biomass for this energy (Slade et al., 2014). A present review of the available literature reveals that the maximum yearly output of above-ground biofuel (energy produced by burning a certain amount of dry biomass) is lower than algae but much greater than the maximum production of other systems (Table 1). Most constructed wetlands continue to operate at a lower productivity level due to the fact that high biomass yield is not a primary concern in wastewater treatment, resulting in a wide range of values for the bioenergy productivity of existing constructed wetlands around the world [from 11 to 1,836 GJ ha−1yr−1 (1 GJ = 109 J)]. Nevertheless, by using discharged waste nitrogen, regulating hydrologic flow patterns, and choosing productive plant species, the bioenergy production of wetlands may be significantly enhanced. Using wastewater treatment plants (WTP) as a baseline, new wetlands might reduce GHG emissions by 591 kg, equivalent to CO2 per kilogram of nitrogen removal (Slade et al., 2014). This is because WTP generates relatively large GHG emissions, whereas biofuel production generates no additional GHG emissions (Table 1). In addition to biofuel generation, constructed wetlands have the potential to provide several other ecosystem services, such as protecting the environment and offering opportunities for energy generation. Over 31 million biogas units were used in India and China alone by 2011 (Vickers, 2017) and 45 million globally (Mungwe et al., 2016).
CW may also play a role in the electricity production sector (Hülsmann and Ardakanian, 2014). Oon et al. (2015) reported a maximum power density that ranged between 12 and 93 mW/m3 in two-stage (i.e., a combination of vertical and horizontal flow systems) MFC-based wetlands. In addition, Saeed et al. (2022) also reported a considerable power density of 86 mW/m2 with biofuel-based constructed wetlands in Bangladesh. Present findings of such research may have far-reaching implications, especially in the areas of rapid CW development requiring 25%–30% fewer aerial footprints than centralized water treatment plants (Fan et al., 2021).
3 Conclusion
The present study combed through the relevant literature to assess the importance of constructed wetlands as natural treatment systems and the energy resources obtained from those. Recovering pure water and energy from CW systems from wastewater treatment has been demonstrated to be possible and feasible. As the intersection among urban infrastructure, high density of population, and agricultural lands has caused conflict for space and resources, the integrated strategy of this may be highly advantageous in preurban regions, which will provide a long-term answer to the issue of food and energy production resource requirements. Requirements for conventional forms of energy could be met by energy generation from wetlands. The use of biomass harvested from wetlands may reduce our dependency on fossil fuels. If biomass is utilized as fuel, the byproduct of the conversion could be used as fertilizer and can reduce the need for synthetic fertilizers. This means that sustainable development and climate change mitigation depends critically on managing natural resources, the natural environment, and the wetlands in developing countries and worldwide. According to the current assessment, the ecological significance of wetlands has been emphasized, and it is important to set aside specific locations to preserve them at the regional and state levels. There is a knowledge gap among academics, legislators, and wetland users on the connection between human impacts on wetlands and the subsequent effects of global warming and climate change, particularly in developing nations. Consequently, future studies need to look into the identification and evaluation of the links between wetland health and climate change.
Author contributions
Conceptualization, NM and MASI; Investigation, MASI and NM; Data Collection and Analysis, MASI; Writing—original draft preparation, MASI; Writing—review and editing, MASI and NM; Supervision; NM and TS. All authors have read and agreed to the published version of the manuscript.
Conflict of interest
The authors declare that the research was conducted in the absence of any commercial or financial relationships that could be construed as a potential conflict of interest.
Publisher’s note
All claims expressed in this article are solely those of the authors and do not necessarily represent those of their affiliated organizations, or those of the publisher, the editors and the reviewers. Any product that may be evaluated in this article, or claim that may be made by its manufacturer, is not guaranteed or endorsed by the publisher.
References
Abdel-Shafy, H. I., El-Khateeb, M. A., and Mansour, M. S. (2022). Constructed wetlands for wastewater management in Egypt: An overview of 30-years experiences in small/medium-size treatment plants. Constr. Wetl. Wastewater Treat. Hot Arid Clim. 71–99. doi:10.1007/978-3-031-03600-2_5
Agusdinata, D. B., Zhao, F., Ileleji, K., and DeLaurentis, D. (2011). Life cycle assessment of potential biojet fuel production in the United States. Environ. Sci. Technol. 45 (21), 9133–9143. doi:10.1021/es202148g
Ahmed, S., Popov, V., and Trevedi, R. C. (2008). Constructed wetland as tertiary treatment for municipal wastewater. Proc. institution Civ. engineers-waste Resour. Manag. 161 (2), 77–84. doi:10.1680/warm.2008.161.2.77
Ali, S. S., El-Sheekh, M., Manni, A., Ruiz, H. A., Elsamahy, T., Sun, J., et al. (2022). Microalgae-mediated wastewater treatment for biofuels production: A comprehensive review. Microbiol. Res. 265, 127187. doi:10.1016/j.micres.2022.127187
Austin, D., and Nivala, J. (2009). Energy requirements for nitrification and biological nitrogen removal in engineered wetlands. Ecol. Eng. 35 (2), 184–192. doi:10.1016/j.ecoleng.2008.03.002
Ávila, C., Salas, J. J., Martin, I., Aragón, C., and García, J. (2013). Integrated treatment of combined sewer wastewater and stormwater in a hybrid constructed wetland system in southern Spain and its further reuse. Ecol. Eng. 50, 13–20. doi:10.1016/j.ecoleng.2012.08.009
Barancheshme, F., and Munir, M. (2018). Strategies to combat antibiotic resistance in the wastewater treatment plants. Front. Microbiol. 8, 2603. doi:10.3389/fmicb.2017.02603
Belmont, M. A., Cantellano, E., Thompson, S., Williamson, M., Sánchez, A., and Metcalfe, C. D. (2004). Treatment of domestic wastewater in a pilot-scale natural treatment system in central Mexico. Ecol. Eng. 23 (4-5), 299–311. doi:10.1016/j.ecoleng.2004.11.003
Bhatt, P., Bhandari, G., Bhatt, K., and Simsek, H. (2022). Microalgae-based removal of pollutants from wastewaters: Occurrence, toxicity and circular economy. Chemosphere 306, 135576. doi:10.1016/j.chemosphere.2022.135576
Boonsong, K., and Chansiri, M. (2008). Domestic wastewater treatment using vetiver grass cultivated with floating platform technique. AU J. Technol. 12 (2), 73–80.
Boretti, A., and Rosa, L. (2019). Reassessing the projections of the world water development report. NPJ Clean. Water 2 (1), 15–16. doi:10.1038/s41545-019-0039-9
Brix, H., Koottatep, T., Fryd, O., and Laugesen, C. H. (2011). The flower and the butterfly constructed wetland system at Koh Phi Phi—system design and lessons learned during implementation and operation. Ecol. Eng. 37 (5), 729–735. doi:10.1016/j.ecoleng.2010.06.035
Caban, M., and Stepnowski, P. (2021). How to decrease pharmaceuticals in the environment? A review. Environ. Chem. Lett. 19 (4), 3115–3138. doi:10.1007/s10311-021-01194-y
Čegar, S., Denona Bogović, N., and Jugović, A. (2022). Impact of intersectoral dependencies in national production on wastewater discharges: An extended input–output study of the Croatian economy. Water 14 (13), 2122. doi:10.3390/w14132122
Chai, F., Li, L., Xue, S., Xie, F., and Liu, J. (2021). Electrochemical system for anaerobic oxidation of methane by DAMO microbes with nitrite as an electron acceptor. Sci. Total Environ. 799, 149334. doi:10.1016/j.scitotenv.2021.149334
Chang, J. J., Wu, S. Q., Dai, Y. R., Liang, W., and Wu, Z. B. (2012). Treatment performance of integrated vertical-flow constructed wetland plots for domestic wastewater. Ecol. Eng. 44, 152–159. doi:10.1016/j.ecoleng.2012.03.019
Chen, G. Q., Chen, H., Chen, Z. M., Zhang, B., Shao, L., Guo, S., et al. (2011). Low-carbon building assessment and multi-scale input–output analysis. Commun. Nonlinear Sci. Numer. Simul. 16 (1), 583–595. doi:10.1016/j.cnsns.2010.02.026
Clarens, A. F., Resurreccion, E. P., White, M. A., and Colosi, L. M. (2010). Environmental life cycle comparison of algae to other bioenergy feedstocks. Environ. Sci. Technol. 44 (5), 1813–1819. doi:10.1021/es902838n
Colares, G. S., Dell'Osbel, N., Wiesel, P. G., Oliveira, G. A., Lemos, P. H. Z., da Silva, F. P., et al. (2020). Floating treatment wetlands: A review and bibliometric analysis. Sci. Total Environ. 714, 136776. doi:10.1016/j.scitotenv.2020.136776
Conservation, E. (2006). Wastewater management fact sheet. Washington: Office of Water, United States Environmental Protection Agency.
Das, G., and Kumar, A. (2022). Wetland flora of West Bengal for phytoremediation: Physiological and biotechnological studies—a review. Biotechnol. Innovations Environ. Bioremediation, 455–485. doi:10.1007/978-981-16-9001-3_19
Dittrich, E., Klincsik, M., Somfai, D., Dolgos-Kovács, A., Kiss, T., and Szekeres, A. (2021). Application of divided convective-dispersive transport model to simulate variability of conservative transport processes inside a planted horizontal subsurface flow constructed wetland. Environ. Sci. Pollut. Res. 28 (13), 15966–15994. doi:10.1007/s11356-020-10965-z
Doğan, E. C., Arslan, A., Genç, N., Dağaşan, L., and Hung, Y. T. (2022). “Treatment and minimization of waste in baker’s yeast industry,” in Waste treatment in the biotechnology, agricultural and food industries (Cham: Springer), 391–470. doi:10.1007/s11356-021-17992-4
Doherty, L., Zhao, X., Zhao, Y., and Wang, W. (2015). The effects of electrode spacing and flow direction on the performance of microbial fuel cell-constructed wetland. Ecol. Eng. 79, 8–14. doi:10.1016/j.ecoleng.2015.03.004
Fahid, M., Ali, S., Shabir, G., Rashid Ahmad, S., Yasmeen, T., Afzal, M., et al. (2020). Cyperus laevigatus L. enhances diesel oil remediation in synergism with bacterial inoculation in floating treatment wetlands. Sustainability 12 (6), 2353. doi:10.3390/su12062353
Fan, Y., Wu, X., Shao, L., Han, M., Chen, B., Meng, J., et al. (2021). Can constructed wetlands be more land efficient than centralized wastewater treatment systems? A case study based on direct and indirect land use. Sci. Total Environ. 770, 144841. doi:10.1016/j.scitotenv.2020.144841
Fang, Z., Song, H. L., Cang, N., and Li, X. N. (2013). Performance of microbial fuel cell coupled constructed wetland system for decolorization of azo dye and bioelectricity generation. Bioresour. Technol. 144, 165–171. doi:10.1016/j.biortech.2013.06.073
Fang, Z., Song, H. L., Cang, N., and Li, X. N. (2015). Electricity production from Azo dye wastewater using a microbial fuel cell coupled constructed wetland operating under different operating conditions. Biosens. Bioelectron. 68, 135–141. doi:10.1016/j.bios.2014.12.047
Fang, H., Zhang, Q., Nie, X., Chen, B., Xiao, Y., Zhou, Q., et al. (2017). Occurrence and elimination of antibiotic resistance genes in a long-term operation integrated surface flow constructed wetland. Chemosphere 173, 99–106. doi:10.1016/j.chemosphere.2017.01.027
Ge, Y., Li, S. P., Niu, X. Y., Yue, C. L., Xu, Q. S., and Chang, J. (2007). Sustainable growth and nutrient uptake of plants in a subtropical constructed wetland in southeast China. Fresenius Environ. Bull. 16 (9), 1023–1029. doi:10.4236/ajps.2016.78121
Haberl, R., Perfler, R., and Mayer, H. (1995). Constructed wetlands in Europe. Water Sci. Technol. 32 (3), 305–315. doi:10.2166/wst.1995.0153
Headley, T. R., and Tanner, C. C. (2012). Constructed wetlands with floating emergent macrophytes: An innovative stormwater treatment technology. Crit. Rev. Environ. Sci. Technol. 42 (21), 2261–2310. doi:10.1080/10643389.2011.574108
Hill, J., Nelson, E., Tilman, D., Polasky, S., and Tiffany, D. (2006). Environmental, economic, and energetic costs and benefits of biodiesel and ethanol biofuels. Proc. Natl. Acad. Sci. U. S. A. 103 (30), 11206–11210. doi:10.1073/pnas.0604600103
Horner, J. E., Castle, J. W., Rodgers, J. H., Gulde, C. M., and Myers, J. E. (2012). Design and performance of pilot-scale constructed wetland treatment systems for treating oilfield produced water from Sub-Saharan Africa. Water Air Soil Pollut. 223 (5), 1945–1957. doi:10.1007/s11270-011-0996-1
Hülsmann, S., and Ardakanian, R. (2014). White book on advancing a Nexus approach to the sustainable management of water, soil and waste. Dresden: UNU-FLORES.
Jamwal, P., Raj, A. V., Raveendran, L., Shirin, S., Connelly, S., Yeluripati, J., et al. (2021). Evaluating the performance of horizontal sub-surface flow constructed wetlands: A case study from southern India. Ecol. Eng. 162, 106170. doi:10.1016/j.ecoleng.2021.106170
Jiang, S., Xu, J., Wang, H., and Wang, X. (2022). Study of the effect of pyrite and alkali-modified rice husk substrates on enhancing nitrogen and phosphorus removals in constructed wetlands. Environ. Sci. Pollut. Res. 29, 54234–54249. doi:10.1007/s11356-022-19537-9
Jinadasa, K. B. S. N., Tanaka, N., Mowjood, M. I. M., and Werellagama, D. R. I. B. (2006). Free water surface constructed wetlands for domestic wastewater treatment: A tropical case study. Chem. Ecol. 22 (3), 181–191. doi:10.1080/02757540600658849
Kadlec, R. H. (2009). Comparison of free water and horizontal subsurface treatment wetlands. Ecol. Eng. 35 (2), 159–174. doi:10.1016/j.ecoleng.2008.04.008
Kaseva, M. E. (2004). Performance of a sub-surface flow constructed wetland in polishing pre-treated wastewater—A tropical case study. Water Res. 38 (3), 681–687. doi:10.1016/j.watres.2003.10.041
Kerr-Upal, M., Seasons, M., and Mulamoottil, G. (2000). Retrofitting a stormwater management facility with a wetland component. J. Environ. Sci. Health Part A 35 (8), 1289–1307. doi:10.1080/10934520009377037
Khalish, M., Utami, A., Lukito, H., and Herlambang, S. (2022). Evaluation of textile industry wastewater treatment as an effort to control river water pollution in central java. KnE Life Sci. 1, 48–61. doi:10.18502/kls.v7i3.11106
Klein, A. R. (2022). Death before sentencing: Ending rampant suicide, overdoses, brutality, and malpractice in America's jails. Rowman & Littlefield.
Klomjek, P., and Nitisoravut, S. (2005). Constructed treatment wetland: A study of eight plant species under saline conditions. Chemosphere 58 (5), 585–593. doi:10.1016/j.chemosphere.2004.08.073
Li, Q., Mahendra, S., Lyon, D. Y., Brunet, L., Liga, M. V., Li, D., et al. (2008). Antimicrobial nanomaterials for water disinfection and microbial control: Potential applications and implications. Water Res. 42 (18), 4591–4602. doi:10.1016/j.watres.2008.08.015
Li, M., Wu, Y. J., Yu, Z. L., Sheng, G. P., and Yu, H. Q. (2009). Enhanced nitrogen and phosphorus removal from eutrophic lake water by Ipomoea aquatica with low-energy ion implantation. Water Res. 43 (5), 1247–1256. doi:10.1016/j.watres.2008.12.013
Licata, M., Ruggeri, R., Iacuzzi, N., Virga, G., Farruggia, D., Rossini, F., et al. (2021). Treatment of combined dairy and domestic wastewater with constructed wetland system in sicily (Italy). Pollutant removal efficiency and effect of vegetation. Water 13 (8), 1086. doi:10.3390/w13081086
Liu, C., Du, G., Huang, B., Meng, Q., Li, H., Wang, Z., et al. (2007). Biodiversity and water quality variations in constructed wetland of Yongding River system. Acta Ecol. Sin. 27 (9), 3670–3677. doi:10.1016/S1872-2032(07)60080-8
Liu, D., Wu, X., Chang, J., Gu, B., Min, Y., Ge, Y., et al. (2012). Constructed wetlands as biofuel production systems. Nat. Clim. Chang. 2 (3), 190–194. doi:10.1038/nclimate1370
Liu, S., Song, H., Li, X., and Yang, F. (2013). Power generation enhancement by utilizing plant photosynthate in microbial fuel cell coupled constructed wetland system. Int. J. Photoenergy 2013, 1–10. doi:10.1155/2013/172010
Maine, M. A., Suñe, N., Hadad, H., and Sánchez, G. (2007). Temporal and spatial variation of phosphate distribution in the sediment of a free water surface constructed wetland. Sci. total Environ. 380 (1-3), 75–83. doi:10.1016/j.scitotenv.2006.11.036
Manikandan, S., Subbaiya, R., Saravanan, M., Ponraj, M., Selvam, M., and Pugazhendhi, A. (2022). A critical review of advanced nanotechnology and hybrid membrane based water recycling, reuse, and wastewater treatment processes. Chemosphere 289, 132867. doi:10.1016/j.chemosphere.2021.132867
Markandya, A. (2022). Marine ecosystems and the blue economy: Policies for their sustainable exploitation. Blue Econ., 37–55. doi:10.1007/978-3-030-96519-8_3
Mburu, N., Tebitendwa, S. M., Rousseau, D. P., Van Bruggen, J. J. A., and Lens, P. N. (2013). Performance evaluation of horizontal subsurface flow–constructed wetlands for the treatment of domestic wastewater in the tropics. J. Environ. Eng. New. York. 139 (3), 358–367. doi:10.1061/(ASCE)EE.1943-7870.0000636
McCarty, P. L., Bae, J., and Kim, J. (2011). Domestic wastewater treatment as a net energy producer–can this be achieved? Environ. Sci. Technol. 45, 7100. doi:10.1021/es2014264
Mohan, S. V., Mohanakrishna, G., and Chiranjeevi, P. (2011). Sustainable power generation from floating macrophytes based ecological microenvironment through embedded fuel cells along with simultaneous wastewater treatment. Bioresour. Technol. 102 (14), 7036–7042. doi:10.1016/j.biortech.2011.04.033
Mungwe, J. N., Colombo, E., Adani, F., and Schievano, A. (2016). The fixed dome digester: An appropriate design for the context of Sub-Sahara Africa? Biomass Bioenergy 95, 35–44. doi:10.1016/j.biombioe.2016.09.007
Nawaz, A., Mishra, R. K., Sabbarwal, S., and Kumar, P. (2021). Studies of physicochemical characterization and pyrolysis behavior of low-value waste biomass using thermogravimetric analyzer: Evaluation of kinetic and thermodynamic parameters. Bioresour. Technol. Rep. 16, 100858. doi:10.1016/j.biteb.2021.100858
Oon, Y. L., Ong, S. A., Ho, L. N., Wong, Y. S., Oon, Y. S., Lehl, H. K., et al. (2015). Hybrid system up-flow constructed wetland integrated with microbial fuel cell for simultaneous wastewater treatment and electricity generation. Bioresour. Technol. 186, 270–275. doi:10.1016/j.biortech.2015.03.014
Ottoy, S., Truyers, E., De Block, M., Lettens, S., Swinnen, W., Broothaerts, N., and Vancampenhout, K. (2022). Digital mapping of soil organic carbon hotspots in nature conservation areas in the region of Flanders, Belgium. Geoderma Reg. 30, e00531. doi:10.1016/j.geodrs.2022.e00531
Panwar, R. S., and Makvana, K. S. (2017). Reed-Phragmitis karka based constructed wetland for the treatment of domestic wastewater in Ujjain city of Central India. Int. J. Sci. Res. Biol. Sci. 4, 1–5.
Poh, S. C. (2003). Assessment of constructed wetland systems in Nepal. Massachusetts Avenue, Cambridge: Doctoral dissertation, Massachusetts Institute of Technology.
Priya, A. K., Gnanasekaran, L., Rajendran, S., Qin, J., and Vasseghian, Y. (2022). Occurrences and removal of pharmaceutical and personal care products from aquatic systems using advanced treatment-A review. Environ. Res. 204, 112298. doi:10.1016/j.envres.2021.112298
Puigagut, J., Villaseñor, J., Salas, J. J., Bécares, E., and García, J. (2007). Subsurface-flow constructed wetlands in Spain for the sanitation of small communities: A comparative study. Ecol. Eng. 30 (4), 312–319. doi:10.1016/j.ecoleng.2007.04.005
Rampuria, A., Gupta, A. B., Kulshreshtha, N. M., and Brighu, U. (2021). Microbiological analysis of two deep constructed wetlands with special emphasis on the removal of pathogens and antibiotic-resistant bacteria. Water Air Soil Pollut. 232, 174. doi:10.1007/s11270-021-05121-3
Ruan, X., Xue, Y., Wu, J., Ni, L., Sun, M., and Zhang, X. (2006). Treatment of polluted river water using pilot-scale constructed wetlands. Bull. Environ. Contam. Toxicol. 76 (1), 90–97. doi:10.1007/s00128-005-0893-7
Saeed, T., and Miah, M. J. (2021). Organic matter and nutrient removal in tidal flow-based microbial fuel cell constructed wetlands: Media and flood-dry period ratio. Chem. Eng. J. 411, 128507. doi:10.1016/j.cej.2021.128507
Saeed, T., Afrin, R., Al Muyeed, A., and Sun, G. (2012). Treatment of tannery wastewater in a pilot-scale hybrid constructed wetland system in Bangladesh. Chemosphere 88 (9), 1065–1073. doi:10.1016/j.chemosphere.2012.04.055
Saeed, T., Al-Muyeed, A., Afrin, R., Rahman, H., and Sun, G. (2014). Pollutant removal from municipal wastewater employing baffled subsurface flow and integrated surface flow-floating treatment wetlands. J. Environ. Sci. 26 (4), 726–736. doi:10.1016/S1001-0742(13)60476-3
Saeed, T., Haque, I., and Khan, T. (2019). Organic matter and nutrients removal in hybrid constructed wetlands: Influence of saturation. Chem. Eng. J. 371, 154–165. doi:10.1016/j.cej.2019.04.030
Saeed, T., Miah, M. J., Khan, T., and Ove, A. (2020). Pollutant removal employing tidal flow constructed wetlands: Media and feeding strategies. Chem. Eng. J. 382, 122874. doi:10.1016/j.cej.2019.122874
Saeed, T., Miah, M. J., and Yadav, A. K. (2022). Free-draining two-stage microbial fuel cell integrated constructed wetlands development using biomass, construction, and industrial wastes as filter materials: Performance assessment. Chem. Eng. J. 437, 135433. doi:10.1016/j.cej.2022.135433
Sari, T., Akgul, D., and Mertoglu, B. (2022). Accumulation of TiO2 nanoparticles in the anammox bioreactor: Their effects on treatment performance and microbial dynamics. Biochem. Eng. J. 187, 108595. doi:10.1016/j.bej.2022.108595
Schmer, M. R., Vogel, K. P., Mitchell, R. B., and Perrin, R. K. (2008). Net energy of cellulosic ethanol from switchgrass. Proc. Natl. Acad. Sci. U. S. A. 105 (2), 464–469. doi:10.1073/pnas.0704767105
Shaharuddin, S., Zakaria, N. A., Ghani, A. A., and Chang, C. K. (2013). “Performance evaluation of constructed Wetland in Malaysia for water security enhancement,” in Proceedings of 2013 IAHR world congress China.
Shao, L., Wu, Z., Zeng, L., Chen, Z. M., Zhou, Y., and Chen, G. Q. (2013). Embodied energy assessment for ecological wastewater treatment by a constructed wetland. Ecol. Model. 252, 63–71. doi:10.1016/j.ecolmodel.2012.09.004
Shrestha, R. R., Haberl, R., Laber, J., Manandhar, R., and Mader, J. (2001). Application of constructed wetlands for wastewater treatment in Nepal. Water Sci. Technol. 44 (11-12), 381–386. doi:10.2166/wst.2001.0855
Sim, C. H., Yusoff, M. K., Shutes, B., Ho, S. C., and Mansor, M. (2008). Nutrient removal in a pilot and full scale constructed wetland, Putrajaya city, Malaysia. J. Environ. Manag. 88 (2), 307–317. doi:10.1016/j.jenvman.2007.03.011
Singh, S., Haberl, R., Moog, O., Shrestha, R. R., Shrestha, P., and Shrestha, R. (2009). Performance of an anaerobic baffled reactor and hybrid constructed wetland treating high-strength wastewater in Nepal—a model for DEWATS. Ecol. Eng. 35 (5), 654–660. doi:10.1016/j.ecoleng.2008.10.019
Slade, M., Amering, M., Farkas, M., Hamilton, B., O'Hagan, M., Panther, G., et al. (2014). Uses and abuses of recovery: Implementing recovery-oriented practices in mental health systems. World Psychiatry 13 (1), 12–20. doi:10.1002/wps.20084
Somerville, C., Youngs, H., Taylor, C., Davis, S. C., and Long, S. P. (2010). Feedstocks for lignocellulosic biofuels. science 329 (5993), 790–792. doi:10.1126/science.1189268
Song, H. L., Li, X. N., Lu, X. W., and Inamori, Y. (2009). Investigation of microcystin removal from eutrophic surface water by aquatic vegetable bed. Ecol. Eng. 35 (11), 1589–1598. doi:10.1016/j.ecoleng.2008.04.005
Song, Y., Wang, L., Qiang, X., Gu, W., Ma, Z., and Wang, G. (2022). The promising way to treat wastewater by microalgae: Approaches, mechanisms, applications and challenges. J. Water Process Eng. 49, 103012. doi:10.1016/j.jwpe.2022.103012
Srivastava, P., Yadav, A. K., and Mishra, B. K. (2015). The effects of microbial fuel cell integration into constructed wetland on the performance of constructed wetland. Bioresour. Technol. 195, 223–230. doi:10.1016/j.biortech.2015.05.072
Stavi, I., Paschalidou, A., Kyriazopoulos, A. P., Halbac-Cotoara-Zamfir, R., Siad, S. M., Suska-Malawska, M., et al. (2021). Multidimensional food security nexus in drylands under the slow onset effects of climate change. Land 10 (12), 1350. doi:10.3390/land10121350
Sun, L., Liu, Y., and Jin, H. (2009). Nitrogen removal from polluted river by enhanced floating bed grown canna. Ecol. Eng. 35 (1), 135–140. doi:10.1016/j.ecoleng.2008.09.016
Tanaka, N., Jinadasa, K. B. S. N., Werellagama, D. R. I. B., Mowjood, M. I. M., and Ng, W. J. (2006). Constructed tropical wetlands with integrated submergent-emergent plants for sustainable water quality management. J. Environ. Sci. Health, Part A 41 (10), 2221–2236. doi:10.1080/10934520600867581
Tanaka, S., Terasaki, M., Hayashi, N., Oka, S., and Chayama, K. (2013). Warning for unprincipled colorectal endoscopic submucosal dissection: Accurate diagnosis and reasonable treatment strategy. Dig. Endosc. 25 (2), 107–116. doi:10.1111/den.12016
Tilak, A. S., Wani, S. P., Datta, A., Patil, M. D., Kaushal, M., and Reddy, K. R. (2017). Evaluation of Ageratum conyzoides in field scale constructed wetlands (CWs) for domestic wastewater treatment. Water Sci. Technol. 75, 2268–2280. doi:10.2166/wst.2017.119
Tilman, D., Hill, J., and Lehman, C. (2006). Carbon-negative biofuels from low-input high-diversity grassland biomass. Science 314 (5805), 1598–1600. doi:10.1126/science.1133306
Tunçsiper, B. (2009). Nitrogen removal in a combined vertical and horizontal subsurface-flow constructed wetland system. Desalination 247 (1-3), 466–475. doi:10.1016/j.desal.2009.03.003
Ummalyma, S. B., Sahoo, D., and Pandey, A. (2021). Resource recovery through bioremediation of wastewaters and waste carbon by microalgae: A circular bioeconomy approach. Environ. Sci. Pollut. Res. 28 (42), 58837–58856. doi:10.1007/s11356-020-11645-8
Vickers, N. J. (2017). Animal communication: When i’m calling you, will you answer too? Curr. Biol. 27 (14), R713–R715. doi:10.1016/j.cub.2017.05.064
Vymazal, J. (2007). Removal of nutrients in various types of constructed wetlands. Sci. total Environ. 380 (1-3), 48–65. doi:10.1016/j.scitotenv.2006.09.014
Wang, L., Peng, J., Wang, B., and Yang, L. (2006). Design and operation of an eco-system for municipal wastewater treatment and utilization. Water Sci. Technol. 54 (11-12), 429–436. doi:10.2166/wst.2006.923
World Water Assessment Programme (2017). Wastewater: The untapped resource. Nairobi: The United Nations World Water Development Report.
World Bank (2012). World Bank. Available at: http://data.worldbank.org/(accessed 02 28, 14).
World Health Organization, and UNICEF (2013). Progress on sanitation and drinking-water. Geneva: World Health Organization.
Xiong, J., Guo, G., Mahmood, Q., and Yue, M. (2011). Nitrogen removal from secondary effluent by using integrated constructed wetland system. Ecol. Eng. 37 (4), 659–662. doi:10.1016/j.ecoleng.2010.12.025
Xu, L., Zhao, Y., Doherty, L., Hu, Y., and Hao, X. (2016). Promoting the bio-cathode formation of a constructed wetland-microbial fuel cell by using powder activated carbon modified alum sludge in anode chamber. Sci. Rep. 6 (1), 26514–26519. doi:10.1038/srep26514
Yadav, A. K., Dash, P., Mohanty, A., Abbassi, R., and Mishra, B. K. (2012). Performance assessment of innovative constructed wetland-microbial fuel cell for electricity production and dye removal. Ecol. Eng. 47, 126–131. doi:10.1016/j.ecoleng.2012.06.029
Yang, Z., Zheng, S., Chen, J., and Sun, M. (2008). Purification of nitrate-rich agricultural runoff by a hydroponic system. Bioresour. Technol. 99 (17), 8049–8053. doi:10.1016/j.biortech.2008.03.040
Zhang, D., Gersberg, R. M., Ng, W. J., and Tan, S. K. (2014). Removal of pharmaceuticals and personal care products in aquatic plant-based systems: A review. Environ. Pollut. 184, 620–639. doi:10.1016/j.envpol.2013.09.009
Zhang, H., Tang, W., Wang, W., Yin, W., Liu, H., Ma, X., et al. (2021). A review on China's constructed wetlands in recent three decades: Application and practice. J. Environ. Sci. 104, 53–68. doi:10.1016/j.jes.2020.11.032
Keywords: constructed wetlands, treatment performance, bioenergy generation, climate change, developing countries
Citation: Islam MAS, Saeed T and Majed N (2022) Role of constructed wetlands in mitigating the challenges of industrial growth and climate change impacts in the context of developing countries. Front. Environ. Sci. 10:1065555. doi: 10.3389/fenvs.2022.1065555
Received: 09 October 2022; Accepted: 04 November 2022;
Published: 21 November 2022.
Edited by:
Yuanchun Zou, Northeast Institute of Geography and Agroecology (CAS), ChinaCopyright © 2022 Islam, Saeed and Majed. This is an open-access article distributed under the terms of the Creative Commons Attribution License (CC BY). The use, distribution or reproduction in other forums is permitted, provided the original author(s) and the copyright owner(s) are credited and that the original publication in this journal is cited, in accordance with accepted academic practice. No use, distribution or reproduction is permitted which does not comply with these terms.
*Correspondence: Nehreen Majed, nehreen-ce@uap-bd.edu