- 1College of Environment and Safety Engineering, Qingdao University of Science and Technology, Qingdao, China
- 2Institute of Coastal Environmental Pollution Control, College of Environmental Science and Engineering, Key Laboratory of Marine Environment and Ecology, Ministry of Education, Ocean University of China, Qingdao, China
- 3State Environmental Protection Key Laboratory of Environmental Pollution Health Risk Assessment, South China Institute of Environmental Sciences, Ministry of Ecology and Environment, Guangzhou, China
- 4Department of Environmental Science, Zhejiang University, Hangzhou, China
- 5Department of Biomedicine and Health Sciences, Shanghai Vocational College of Agriculture and Forestry, Shanghai, China
- 6Department of Environmental Science, University of Narowal, Narowal, Pakistan
The salinization of several hundred million hectares of land poses a serious threat to global food security and biodiversity. Biochar and wood vinegar amendment has been suggested as a possible way to promote plant productivity and improve soil qualities, whereas less emphasis has been placed on the application of biochar in saline−alkaline soils to increase plant productivity. Herein, the single and composite application of sawdust biochar, wood vinegar, and acid-modified biochar on the restoration of coastal saline−alkali soil and plant growth of strawberry seedlings was investigated. Proximate and ultimate analyses and different characterization techniques such as FTIR and scanning electron microscopy were applied to study the physicochemical characteristics of raw and modified biochar. A pot experiment was conducted to explore the effects of different soil additives on soil properties and strawberry growth. Our results revealed that acid-modified biochar shows the most significant promoting effect on strawberry growth, which could increase the weight gain rate, root length, root specific surface area, and root volume of strawberry seedlings by 41.2%, 18.9%, 23.0%, and 34.7%, respectively. Moreover, the application of acid-modified biochar can effectively alleviate soil salinization by reducing soil pH (7.65) and CEC (19.5 cmol/kg). Furthermore, the addition of biochar can significantly improve the community structure of microorganisms (Proteobacteria) and increase the content of bacteria related to soil nutrient cycling (Pseudomonadaceae and Sphingomonadaceae). Preliminary findings from this study suggested that acid-modified biochar is a potentially effective means of preventing soil salinization and increasing economic crop yields.
1 Introduction
Over the past few decades, the rising population has boosted the food requirements resulting in the intensification of agriculture, leading to soil degradation. Various methods have been used to increase crop yields, such as applying soil fertilizers (Cai et al., 2019) or improving crop varieties (Tadele, 2017). At present, soil salinization is a global issue that contributes to soil degradation, leading to the loss of agricultural productivity (Shrivastava and Kumar, 2015) and food shortages (Ullah et al., 2021). Globally, 10 million ha of agricultural land is affected each year as a result of irrigation with salt-affected water (Machado and Serralheiro, 2017), and about 7% of the world’s land surface is threatened by salinization.
Coastal saline soil is an important land resource widely distributed in eastern China. Jiangsu Province, for example, has cultivated about 2 million hectares of reclaimed tidal flats, and the land area is growing steadily at a rate of 1,300 ha per year. As a result of nutrient deficiency, groundwater salt content, sodium accumulation, freshwater scarcity, and reduced microbial diversity, crop growth in coastal saline−alkali lands is eventually hindered. This problem is further aggravated by the changing climate, intensive farming, reduced leaching, and poor drainage (Hassani et al., 2021). It was predicted that about 50% of arable land in the world will be salt affected by 2050 (Kumar and Sharma, 2020). Coastal saline soils have a number of remediation alternatives, which can be categorized into three categories: leaching, vegetative bioremediation with halophyte, and addition of either chemical or organic amendments. However, leaching requires a lot of fresh water and bioremediation takes a too long time, making it challenging to treat the saline soil quickly and affordably (Sun et al., 2020). Soil salinity requires reclamation of the root zone of the plant to enhance agricultural productivity, which is time-consuming and expensive and requires an abundance of high-quality water and well-drained soil (Stavi et al., 2021). Therefore, it is not always possible to have sufficient time duration and collection of water of sufficient quality for the reclamation of saline soils. On the other hand, plants grown in salt-affected soil face soil osmotic pressure, nutrient deficiency, and water deficiency that will cause metabolic changes in plants leading to stunted growth (Meena et al., 2017). It was evident from the literature that soil salinity decreased CO2 availability, photosynthetic pigments, chlorophyll contents, and plant biomass and caused ion toxicities, leading to the generation of reactive oxygen species (ROS) (Delfine et al., 1999; Ashraf and Harris, 2013). In addition, understanding the structure and function of the microbial community in salt-affected soil is an important ecological goal, as it will aid in elucidating the biological control mechanisms for nutrient cycles ongoing in saline soils. Because any impact on these microbial processes will create a greater implication for the concentration of soil organic matter and availability of nutrients. It was evident from previous studies that soil respiration, enzyme activities, and soil microbial biomass were greatly influenced by salinity (Zhang et al., 2019). Therefore, it is necessary to find alternatives that will help to boost soil health and agricultural production. Biochar and wood vinegar, the products of biomass pyrolysis at high temperatures, showed excellent performance in the remediation of soil pollution, improvement of soil characteristics, and promotion of plant growth (Y. Zhang et al., 2020). However, as the pH of biochar and wood vinegar is alkaline, they are not suitable for coastal saline soil restoration. Acid-modified biochar is widely used in the field of pollutant remediation due to its low pH value and developed pore structure (Chen et al., 2019). Phosphoric acid-activated biochar and nitrogen-containing modified biochar showed an excellent performance in the adsorption of pollutants (Lu et al., 2021). At present, we do not know the effects of the use of acid biochar alone or in combination with wood vinegar on the restoration of offshore degraded soil and the growth of cash crops. Therefore, this research investigated the effects of wood vinegar, sawdust biochar, and its acid-modified biochar alone and in combination on the growth of strawberry seedlings in coastal saline−alkali soil to study the changes in soil microbial diversity after the amendments of raw and modified biochar.
2 Materials and methods
2.1 Preparation of wood vinegar and biochar
The feedstock material of poplar sawdust obtained from the local market was used for the production of biochar in the present study (Wang et al., 2022). The feedstock (sawdust) was pyrolyzed at 450°C for 2 h at a heating rate of 10°C/min in an anaerobic environment to prepare sawdust wood vinegar (WV) and raw biochar (BC), while acid-modified biochar (ABC) was derived from sawdust (3.6 kg) soaked in nitric acid and phosphoric acid (180 ml) under constant pyrolytic parameters. The previous study has shown that 5% is the optimal amount to ensure maximum loading of N and P elements and prevent the destruction of biochar structure (Zheng et al., 2018). The prepared soil additives were stored in a cool and dark environment.
2.2 Characterization
The composition of functional groups in BC and ABC samples was tested by Fourier transform infrared spectroscopy (FTIR, Tensor 27, Bruker, Germany). A scanning electron microscope (SEM, S-4800, Hitachi, Japan) was applied to analyze the morphological structure of biochars. Other basic properties of WV, BC, and ABC were described in detail in our previous article (Wang et al., 2022).
2.3 Pot experiment
The sample area is located in the okra growing area without chemical fertilizers in past years, and the soil samples are randomly collected from the topsoil (0–20 cm). A measure of 200 g of air-dried soil and 1.5% (w:w) of different soil additives were mixed in the ceramic pots (Wang et al., 2022). Four strawberry seedlings which germinated in the incubator were put in the pot. Then, the strawberry seedlings were planted in these pots. The pots without any material addition were set up as the control or blank. The experimental groups were BC, ABC, WV, BC + WV (BWV), and ABC + WV (AWV), while the control group was soil without biochar. Each treatment was replicated three times. These treatments were incubated for 60 days in a greenhouse, and the temperature was maintained between 22 and 26°C, and the humidity was maintained at 60%–80%. The deionized water was added to the strawberry seedlings every day to reach 60% of the field water capacity.
2.4 Analysis of soil properties
After collecting water (soil−water ratio 1:1:2.5) from soil samples treated with different treatments, the pH value was measured using a pH meter. The spectrophotometer (UV-8000T, Shanghai Metash Instrument Co., LTD.) was applied to measure the nutrient content in the soil (Zheng et al., 2013). The content of soil organic carbon (SOC) was determined by the oxidation method with the potassium dichromate–concentrated sulfuric acid high-temperature external heating method (Zheng et al., 2018). Furthermore, soil microbial genomic DNA was extracted from approximately 1 g (wet weight) of soil using a MoBio PowerSoil DNA Isolation Kit (MoBio Laboratories Inc. Carlsbad, CA, United States) following the manufacturer’s instructions. The V3−V4 hypervariable region of the bacterial 16S rRNA gene was amplified by PCR with primers 338F (5 ′-barcode-CTCCtacGGgaggCAGAG-3 ′) and 806R (5' -GGACTACHVGGGTWTCTAAT-3 ′). The PCR products were pyrosequenced on an Illumina MiSeq platform (PE300, Illumina, Inc., San Diego, CA, United States). High-quality sequence reads are then processed and analyzed using QIIME (version 1.8.0). After DNA extraction from soil samples, the extracted genomic DNA was detected by 1% agarose gel electrophoresis. The PCR products were detected by 2% agarose gel electrophoresis. Samples were mixed in equal amounts according to PCR product concentration. After thorough mixing, the target band product was recovered using the gel recovery kit provided by Qiagen. Finally, TruSeq®DNA PCR-Free Sample Preparation Kit was used to construct the library. The constructed library was quantitatively analyzed using Qubit and Q-PCR. Upon eligibility, libraries were sequenced on a computer using HiSeq2500 PE250.
2.5 Statistical analysis
The significant difference between different treatments was tested by one-way analysis of variance using Duncan’s multiple range test (p < 0.05) with the help of Statistical Product and Service Solutions software 20.0 (SPSS 20.0).
3 Results and discussion
3.1 Characterization of biochar
The morphology of biochar and acid-modified biochar was determined by scanning electron microscopy (SEM). As shown in Figure 1A, BC has a smooth surface and regular porous structure with a plant vascular bundle structure, which was similar to the corresponding precursor; the results were consistent with the previous study (Fu et al., 2018). The morphology and porosity of ABC showed a significant variation, as shown in Figure 1B. The surface of ABC was obviously damaged, which resulted in more irregular pore structures. The phenomenon could be explained by the fact that nitric acid and phosphoric acid could destroy the original surface structure of biochar and make its pore structure more developed (Peng et al., 2017).
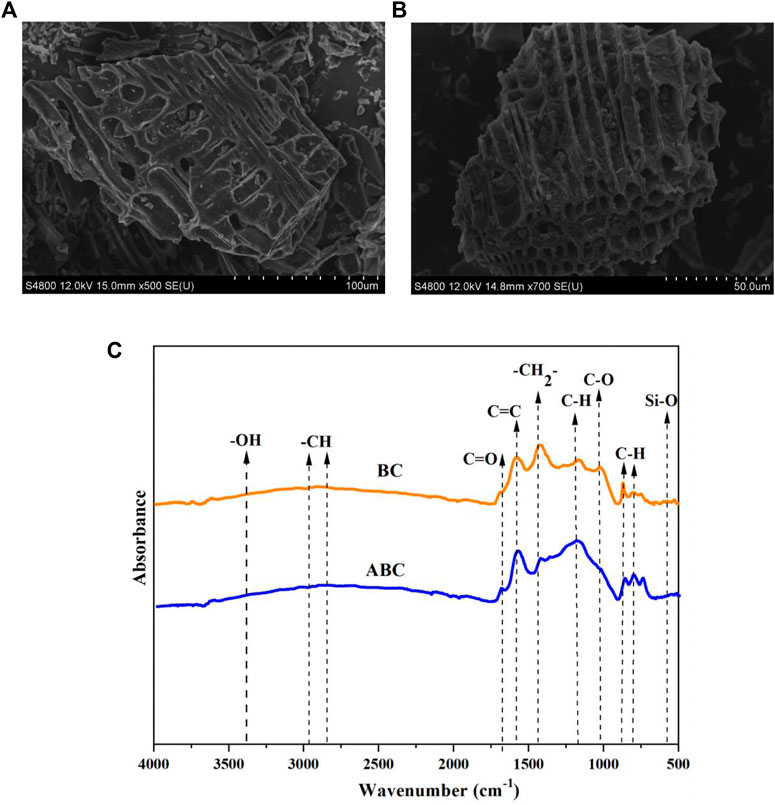
FIGURE 1. SEM images of the sawdust-derived biochar (BC) (A) and acid-modified biochar (ABC) (B). FTIR spectra of biochar (C).
To investigate the effects of nitric acid and phosphoric acid on the functional groups of BC and ABC, the normalized FTIR spectrum of biochar samples was investigated. As shown in Figure 1C, the peaks around 1740 cm−1 and 1640 cm−1 were related to the C=O bond of lipids and ketones. The C=C bond mainly appeared at 1521 cm−1 and 1440 cm−1, which mainly represented the asymmetric bending vibration of -CH2-, while the O−H bond of the phenolic hydroxyl group mainly appeared at 1385 cm−1. In addition, the peaks at 1335 cm−1 represented C−O bonds in the carboxyl group, while the peaks at 1050 and 1041 cm−1 were related to the C−O−C and P−O bonds, respectively. The intensity of O−H and C=O peaks in ABC was significantly higher than that in BC. The results indicated that the acid modification did not show a noticeable modification in the types of oxygenated functional groups in biochar. Nevertheless, the acid modification could affect the content of oxygenated functional groups of biochar. This was consistent with the previous study by Liu et al. (2021).
3.2 Evaluation of the strawberry in response to various soil amendments
The single and combined effects of sawdust wood vinegar, raw biochar, and its acid-modified biochar on the growth of strawberry seedlings are shown in Figure 2. The results revealed that compared to CK, wood vinegar alone did not improve the weight gain rate of strawberry seedlings but inhibited the growth of strawberry seedlings to some extent as depicted in Figure 2A. The application of BC showed no significant effect on the weight gain rate of strawberry seedlings. Compared to CK, the use of acid-modified biochar alone or combined with wood vinegar showed a significant effect (p < 0.05), which could promote the growth of strawberry seedlings, and the weight gain rate of strawberry seedlings increased by 36.0% and 41.2%. Moreover, the strawberry seedlings' height in different treatments showed non-significant differences with CK. Furthermore, the individual application of wood vinegar or biochar had no significant effect on the root growth of strawberry seedlings as shown in Figures 2C–F. Among all the treatments, ABC showed the most significant effect on the root growth of strawberry seedlings. Compared with CK, ABC significantly increased root length, root specific surface area, root volume, and root tip number of strawberry seedlings by 18.9%, 23.0%, 34.7%, and 10.4%, respectively. In general, ABC has the most obvious growth promotion effect on strawberry seedlings, which may be because ABC can better neutralize the pH of saline−alkali soil and adsorb metal ions to alleviate soil salt stress (He et al., 2020). In addition, ABC can provide more nutrient elements such as N and P to meet the needs of plant growth. All these results indicated that owing to the abundant nutrient elements, developed pore structure, and lower pH, ABC could promote the growth of strawberry seedlings.
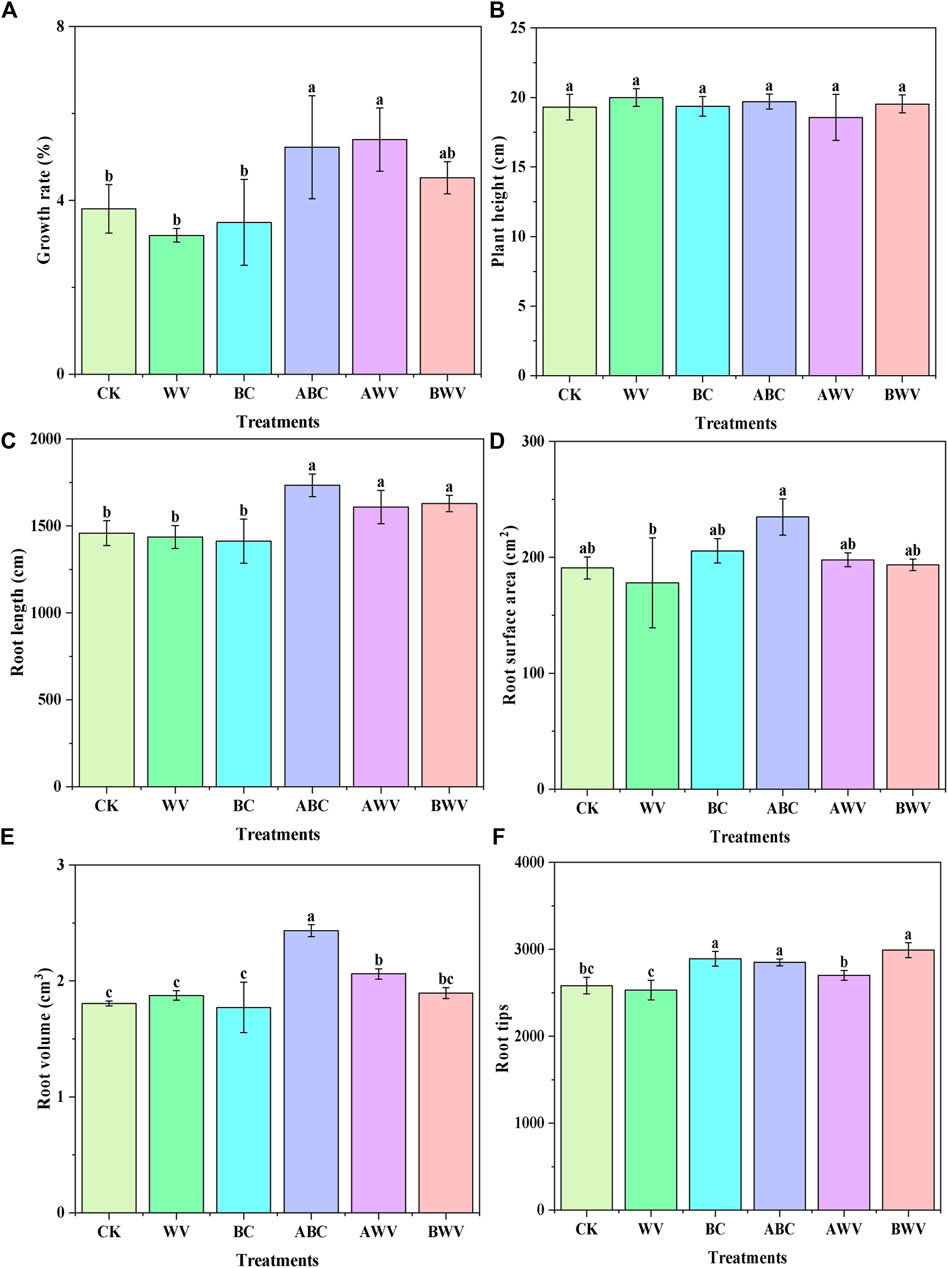
FIGURE 2. The effect of WV, BC, ABC, AWV and BWV on the Growth rate (A), Plant height (B), Root length (C), Root surface area (D), Root volume (E) and Root tips (F) of strawberry seedlings in coastal saline-alkali soil. The different letters among different treatments indicate significant differences, which were analyzed by Duncan test (p = 0.05).
3.3 Evaluation of the soil properties in response to various soil amendments
The remediation effects of single and combined use of sawdust wood vinegar, raw biochar, and its acid-modified biochar on soil characters are illustrated in Figure 3. As shown in Figure 3A, WV and BC had little effect on soil pH, while ABC significantly reduced soil pH to 7.65, and a previous study showed a similar result (Shetty et al., 2021). This phenomenon may affect the bioavailability of soil nutrient elements, soil microbial community structure, and soil organic matter content (Rajapaksha et al., 2016). WV and BC significantly decreased the EC value to 69.5% and 69.4%, respectively. Owing to the large amount of aromatic carbon, each treatment increased the SOC content, and ABC has the most obvious effect. Moreover, the independent or combined application of BC, WV, and ABC could increase the content of soil nutrients (total nitrogen, total phosphorus, and total potassium) to varying degrees. Notably, ABC and AWV treatments significantly improved the bioavailability of nutrients (available phosphorus, available nitrogen, and available potassium). This may be attributed to ABC containing abundant N and P after being modified by H3PO4 and HNO3. These results confirmed that biochar could be an effective supplement to provide nutrients for the growth of plants. Moreover, previous literature works also indicated that the bioavailability of soil nutrients was related to pH; changes in soil pH also influence the composition of nutrient elements (Zhang et al., 2022). In general, the improvement of soil properties by ABC is not only attributed to the additional nutrient elements provided by ABC but also because ABC can reduce soil pH and alleviate soil salinization.
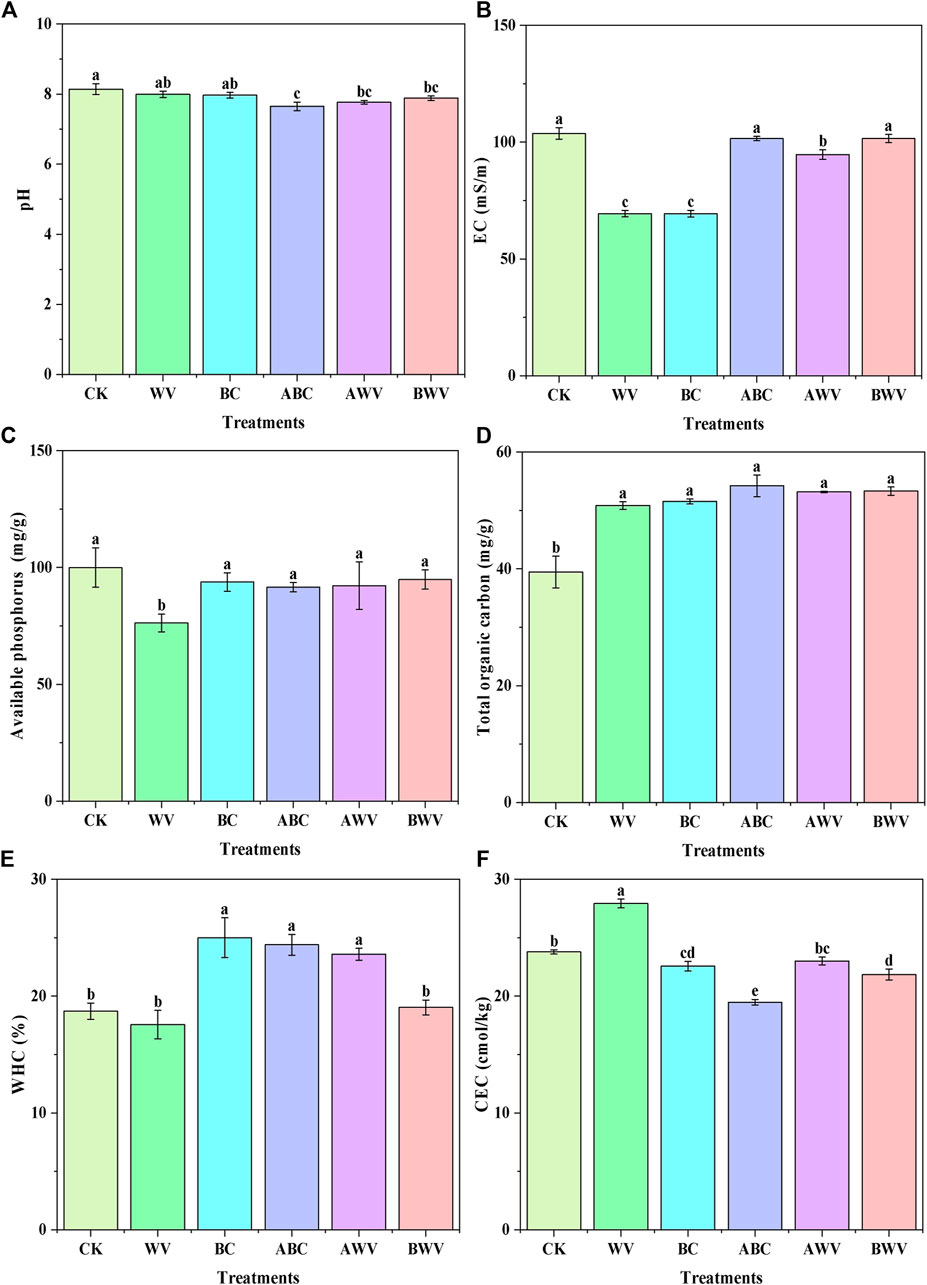
FIGURE 3. Transformations in pH (A), electrical conductivity (B), available phosphorus (C), total organic carbon (D), water-holding capacity (E), and cation exchange capacity (F) in different treatments (CK, WV, BC, ABC, AWV, and BWV) of the coastal saline−alkali soil.
3.4 Evaluation of the soil microbial community in response to various soil amendments
To determine the independent or combined effect of BC, ABC, and WV on the strawberry seedlings in saline−alkali soil, the microbial community composition was measured. Microbial community responses to the application of BC, ABC, WV, AWV, and BWV amendments were further investigated to estimate their amelioration effects on the saline−alkali soil. Alpha diversity analysis (Figures 4A,B) showed that bacterial community richness and diversity of the single use of BC and ABC or the combined application with WV were much more than those of CK, while WV showed a weak effect on bacterial abundance. This phenomenon could be attributed to the fact that the amendment of BC or ABC could efficiently alleviate the salinization of soil to provide a suitable environment for microbes to grow. This was consistent with a previous study (Mitchell et al., 2015). Consistently, the Venn diagram further revealed that the independent or combined use of BC and ABC with WV influences the soil microbial community (Figure 4C). The common number of OTU in different treatments was 2278, and the particular OTU number of CK, BC, ABC, WVB, and WVA was 283, 316, 545, 315, and 482, respectively. This phenomenon further confirmed that the amendment of BC or ABC modified the composition of the microbial community in saline−alkali soil and led to more particular bacterial species. This was attributed to the fact that biochar promotes the growth and reproduction of the soil bacterial community by applying more nutrients, which was consistent with the obvious aggregation of the soil bacterial community in our present study (Figure 4D). For the soil ecosystem to operate and survive throughout time, soil bacterial diversity is crucial. Rich soil biodiversity can also improve soil performance and biological activity while stabilizing the soil ecosystem (Tang et al., 2020). In the present study, analysis using Chao1 and ACE showed that the OTU numbers for BC and ABC treatments were significantly higher than those for the CK treatment, which further indicates that the use of biochar leads to a modification in the soil bacterial composition.
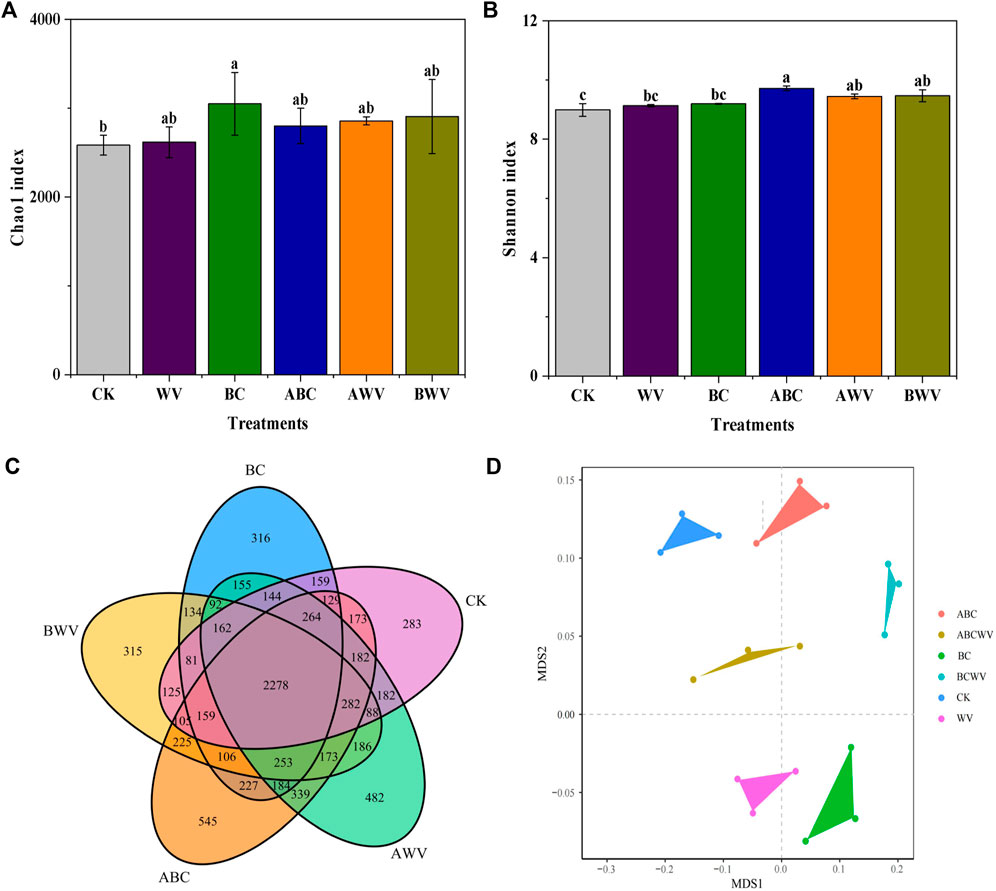
FIGURE 4. Profiles of the bacterial community in the coastal saline−alkali soil with different treatments. (A) Richness (Chao1) and (B) diversity (Shannon) index for the soil bacterial community. (C) Venn diagram showed the shared bacterial OTUs. (D) Non-metric multidimensional scaling (NMDS) ordination plots of the bacterial community composition based on the Bray−Curtis similarity.
The sequencing data from the coastal saline soils (control + five treatments) categorized at the phylum level were linked to eight bacterial phyla as depicted in Figure 5A. Proteobacteria, Bacteroidota, Acidobacteria, Gemmatimonadetes, Myxococcota, Actinobacteria, Patescibacteria, and Chloroflexi were the dominant species of phylum microorganisms with the highest abundance in soil, and these dominant species accounted for 95.3–98.2% of the soil microorganisms. As for the strawberry seedling-growing environment, the application of BC and ABC increased the abundance of Proteobacteria from those soils without biochar, showing that BC and ABC significantly altered the composition of the soil bacterial community. As Proteobacteria was positively correlated with the content of nutrient elements in soil, this result further proved that the application of biochar could be a promising approach to increase soil fertility. Moreover, raw biochar and its acid-modified biochar significantly increased the abundance of Acidobacteria which was positively correlated with soil acidity, showing that the reduction of soil pH could be more suitable for the growth of Acidobacteria (Zheng et al., 2018). Figure 5B illustrates that the use of BC, ABC, and WV leads to an increase in the relative contents of specific families (Pseudomonadaceae and Sphingomonadaceae). The contributions of bacterial community diversity and the composition and properties of amendments to soil properties were further evaluated by redundancy analysis (RDA). As shown in Figure 5C, the first two components could account for 64.52% of the variation in the relationship between the examined genera and soil chemical characteristics (Figure 5C). The first component (RDA1) distinguished between the CK and BWV treatments and explained 51.14% of the variance, while the second component (RDA2) distinguished between the samples based on different sampling periods and explained 13.38% of the variance. The results suggested that the transformation of the soil microbial community was negatively correlated with pH and CEC. This was consistent with previous studies for high pH, and salt pressures were not suitable for microbial growth (Zhang et al., 2022). Furthermore, the results showed that the microorganisms are also affected by the bioavailability of soil nutrients (AK, AP, AN, and TOC). Figure 5C illustrates that the change in microflora is highly correlated with the bio-availability of nutrient elements (AK, SAK, AN, and TOC); abundant nutrients could accelerate the growth of microorganisms and promote soil circulation (Zhu et al., 2017). Notably, with its well-developed pore structure, acidity, and numerous oxygen-containing functional groups, acid-modified biochar can significantly alleviate soil salinization. All these results confirmed that ABC could be a promising approach for the restoration of coastal saline−alkali soils, which could accelerate the carbon, nitrogen, and phosphorus cycle and organic matter degradation.
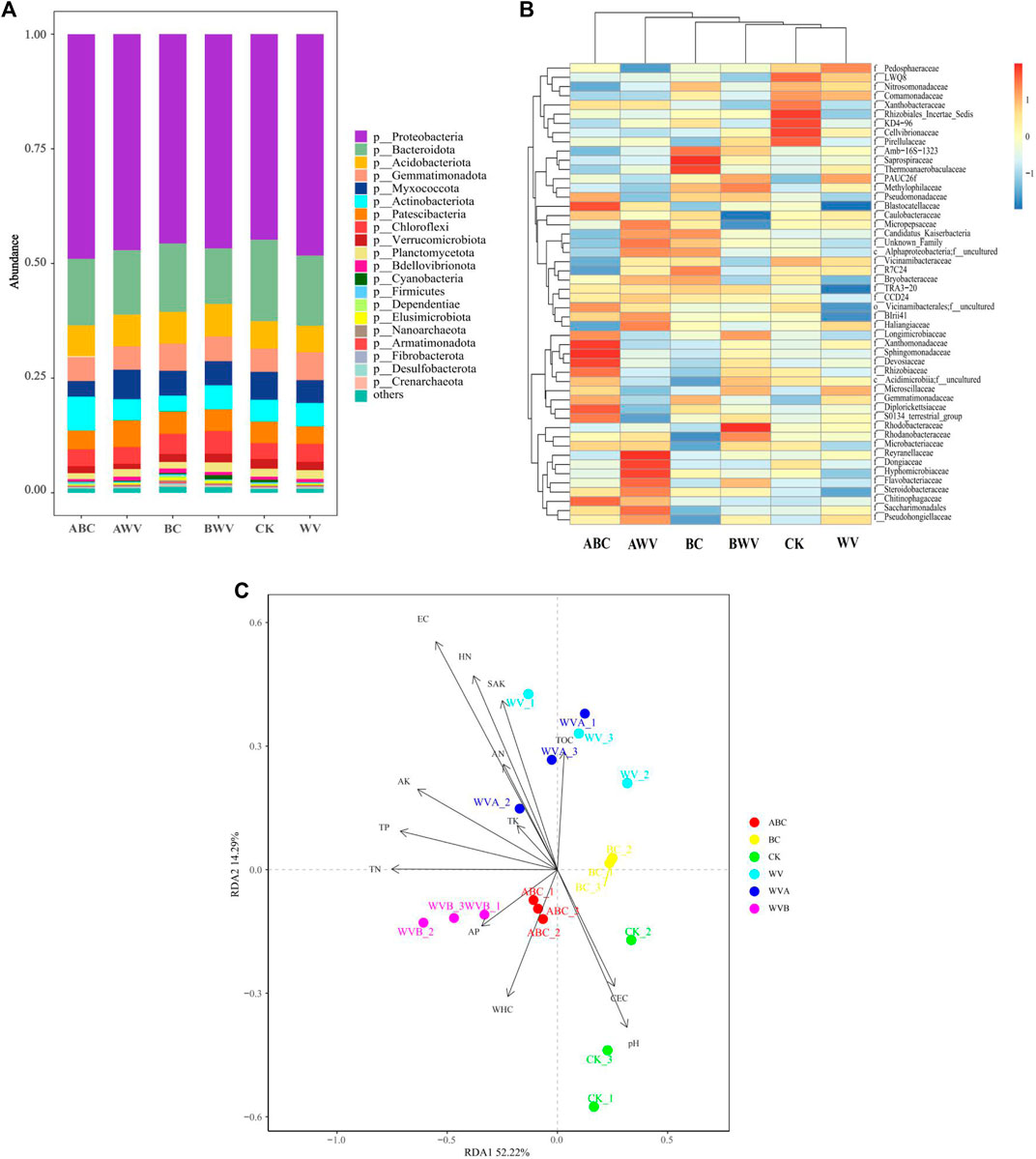
FIGURE 5. Relative abundance of the top 10 bacterial phyla in the coastal saline−alkali soil and the heat map of the top 35 bacteria at the family level (A). Color intensity of the scale demonstrates the relative abundance of each genus (B). Redundancy analysis (RDA) between the microbial family relative abundance and soil properties in different treatments (C).
4 Conclusion
The potential amelioration efficacy of biochar, wood vinegar, and acid-modified biochar on the amelioration of saline−alkali soil and the growth of strawberries was investigated in this study. The results indicated that the application of sawdust biochar modified by acid (H3PO4 and HNO3) showed the best effects which could alleviate soil salinization (reduced soil pH and electrical conductivity) and promote the growth of strawberry seedlings thanks to the unique structure (developed pore structure and abundant functional groups) of acid-modified biochar. Furthermore, acid-modified biochar could enhance the soil biodiversity, increase the relative contents of specific families (Pseudomonadaceae and Sphingomonadaceae), and increase the abundance of Proteobacteria by influencing the nutrient element (C, N, and P) cycle. This work proved that the application of modified biochar-based technology could be a good choice to promote the production of strawberries by remediation of saline−alkali soil.
Data availability statement
The original contributions presented in the study are included in the article/Supplementary Material; further inquiries can be directed to the corresponding authors.
Author contributions
Conceptualization, XP; methodology, MS; validation, XC; resources, SK; data curation, HL and SK; writing—original draft preparation, XP; writing—review and editing, LR; project administration, HU; funding acquisition, SK.
Funding
The present research was supported and funded by the Major Scientific and Technological Innovation Project of Shandong Province (No. 2021CXGC011206).
Acknowledgments
The authors thank the editors and reviewers for their valuable comments to improve this article.
Conflict of interest
The authors declare that the research was conducted in the absence of any commercial or financial relationships that could be construed as a potential conflict of interest.
Publisher’s note
All claims expressed in this article are solely those of the authors and do not necessarily represent those of their affiliated organizations, or those of the publisher, the editors, and the reviewers. Any product that may be evaluated in this article, or claim that may be made by its manufacturer, is not guaranteed or endorsed by the publisher.
Supplementary material
The Supplementary Material for this article can be found online at: https://www.frontiersin.org/articles/10.3389/fenvs.2022.1057384/full#supplementary-material
References
Ashraf, M., and Harris, P. J. (2013). Photosynthesis under stressful environments: An overview. Photosynthetica 51, 163–190. doi:10.1007/s11099-013-0021-6
Cai, A., Xu, M., Wang, B., Zhang, W., Liang, G., Hou, E., et al. (2019). Manure acts as a better fertilizer for increasing crop yields than synthetic fertilizer does by improving soil fertility. Soil Tillage Res. 189, 168–175. doi:10.1016/j.still.2018.12.022
Chen, H., Li, W., Wang, J., Xu, H., Liu, Y., Zhang, Z., et al. (2019). Adsorption of cadmium and lead ions by phosphoric acid-modified biochar generated from chicken feather: Selective adsorption and influence of dissolved organic matter. Bioresour. Technol. 292, 121948. doi:10.1016/j.biortech.2019.121948
Delfine, S., Alvino, A., Villani, M. C., and Loreto, F. (1999). Restrictions to carbon dioxide conductance and photosynthesis in spinach leaves recovering from salt stress. Plant Physiol. 119, 1101–1106. doi:10.1104/pp.119.3.1101
Fu, Y., Zhang, N., and Shen, Y. (2018). Micro-mesoporous carbons from original and pelletized rice husk via one-step catalytic pyrolysis[J]. Bioresource technology 269, 67–73. doi:10.1016/j.biortech.2018.08.083
Hassani, A., Azapagic, A., and Shokri, N. (2021). Global predictions of primary soil salinization under changing climate in the 21st century. Nat. Commun. 12, 6663–6717. doi:10.1038/s41467-021-26907-3
He, K., He, G., Wang, C., Zhang, H., Xu, Y., Wang, S., et al. (2020). Biochar amendment ameliorates soil properties and promotes Miscanthus growth in a coastal saline-alkali soil. Appl. Soil Ecol. 155, 103674. doi:10.1016/j.apsoil.2020.103674
Kumar, P., and Sharma, P. K. (2020). Soil salinity and food security in India[J]. Frontiers in Sustainable Food Systems 4, 533781. doi:10.3389/fsufs.2020.533781
Liu, Y., Li, F., Deng, J., Wu, Z., Lei, T., Tan, M., et al. (2021). Mechanism of sulfamic acid modified biochar for highly efficient removal of tetracycline. J. Anal. Appl. Pyrolysis 158, 105247. doi:10.1016/j.jaap.2021.105247
Lu, Z., Zhang, H., Shahab, A., Zhang, K., Zeng, H., Bacha, A. U. R., et al. (2021). Comparative study on characterization and adsorption properties of phosphoric acid activated biochar and nitrogen-containing modified biochar employing Eucalyptus as a precursor. J. Clean. Prod. 303, 127046. doi:10.1016/j.jclepro.2021.127046
Machado, R. M. A., and Serralheiro, R. P. (2017). Soil salinity: Effect on vegetable crop growth. Management practices to prevent and mitigate soil salinization. Horticulturae 3, 30. doi:10.3390/horticulturae3020030
Meena, K. K., Sorty, A. M., Bitla, U. M., Choudhary, K., Gupta, P., Pareek, A., et al. (2017). Abiotic stress responses and microbe-mediated mitigation in plants: the omics strategies. Frontiers in plant science 8, 172. doi:10.3389/fpls.2017.00172
Mitchell, P. J., Simpson, A. J., Soong, R., and Simpson, M. (2015). Shifts in microbial community and water-extractable organic matter composition with biochar amendment in a temperate forest soil. Soil Biol. Biochem. 81, 244–254. doi:10.1016/j.soilbio.2014.11.017
Peng, H., Gao, P., Chu, G., Pan, B., Peng, J., and Xing, B. (2017). Enhanced adsorption of Cu (II) and Cd (II) by phosphoric acid-modified biochars. Environ. Pollut. 229, 846–853. doi:10.1016/j.envpol.2017.07.004
Rajapaksha, A. U., Chen, S. S., Tsang, D. C., Zhang, M., Vithanage, M., Mandal, S., et al. (2016). Engineered/designer biochar for contaminant removal/immobilization from soil and water: Potential and implication of biochar modification. Chemosphere 148, 276–291. doi:10.1016/j.chemosphere.2016.01.043
Shetty, R., Vidya, C. S.-N., Prakash, N. B., Lux, A., and Vaculik, M. (2021). Aluminum toxicity in plants and its possible mitigation in acid soils by biochar: A review. Sci. Total Environ. 765, 142744. doi:10.1016/j.scitotenv.2020.142744
Shrivastava, P., and Kumar, R. (2015). Soil salinity: A serious environmental issue and plant growth promoting bacteria as one of the tools for its alleviation. Saudi J. Biol. Sci. 22, 123–131. doi:10.1016/j.sjbs.2014.12.001
Stavi, I., Thevs, N., and Priori, S. (2021). Soil salinity and sodicity in drylands: A review of causes, effects, monitoring, and restoration measures. Front. Environ. Sci. 330, 712831. doi:10.3389/fenvs.2021.712831
Sun, Y., Yang, J., Yao, R., Chen, X., and Wang, X. (2020). Biochar and fulvic acid amendments mitigate negative effects of coastal saline soil and improve crop yields in a three year field trial. Sci. Rep. 10 (1), 8946–9012. doi:10.1038/s41598-020-65730-6
Tadele, Z. (2017). Raising crop productivity in africa through intensification. Agronomy 7 (1), 22. doi:10.3390/agronomy7010022
Tang, J., Zhang, S., Zhang, X., Chen, J., He, X., and Zhang, Q. (2020). Effects of pyrolysis temperature on soil-plant-microbe responses to Solidago canadensis L.-derived biochar in coastal saline-alkali soil. Sci. Total Environ. 731, 138938. doi:10.1016/j.scitotenv.2020.138938
Ullah, A., Bano, A., and Khan, N. (2021). Climate change and salinity effects on crops and chemical communication between plants and plant growth-promoting microorganisms under stress. Front. Sustain. Food Syst. 5, 161. doi:10.3389/fsufs.2021.618092
Wang, Z., Pan, X., Kuang, S., Chen, C., Wang, X., Xu, J., et al. (2022). Amelioration of coastal salt-affected soils with biochar, acid modified biochar and wood vinegar: Enhanced nutrient availability and bacterial community modulation. Int. J. Environ. Res. Public Health 19 (12), 7282. doi:10.3390/ijerph19127282
Zhang, W.-w., Chong, W., Rui, X., and Wang, L.-j. (2019). Effects of salinity on the soil microbial community and soil fertility. J. Integr. Agric. 18, 1360–1368. doi:10.1016/s2095-3119(18)62077-5
Zhang, Y., Wang, X., Liu, B., Liu, Q., Zheng, H., You, X., et al. (2020). Comparative study of individual and Co-Application of biochar and wood vinegar on blueberry fruit yield and nutritional quality. Chemosphere 246, 125699. doi:10.1016/j.chemosphere.2019.125699
Zhang, P., Bing, X., Jiao, L., Xiao, H., Li, B., and Sun, H. (2022). Amelioration effects of coastal saline-alkali soil by ball-milled red phosphorus-loaded biochar. Chem. Eng. J. 431, 133904. doi:10.1016/j.cej.2021.133904
Zheng, H., Wang, Z., Deng, X., Zhao, J., Luo, Y., Novak, J., et al. (2013). Characteristics and nutrient values of biochars produced from giant reed at different temperatures. Bioresour. Technol 130, 463–471. doi:10.1016/j.biortech.2012.12.044
Zheng, H., Wang, X., Chen, L., Wang, Z., Xia, Y., Zhang, Y., et al. (2018). Enhanced growth of halophyte plants in biochar‐amended coastal soil: Roles of nutrient availability and rhizosphere microbial modulation. Plant Cell Environ. 41 (3), 517–532. doi:10.1111/pce.12944
Keywords: biochar, soil remediation, coastal saline soil, strawberry seedlings, microbial community
Citation: Pan X, Shi M, Chen X, Kuang S, Ullah H, Lu H and Riaz L (2022) An investigation into biochar, acid-modified biochar, and wood vinegar on the remediation of saline−alkali soil and the growth of strawberries. Front. Environ. Sci. 10:1057384. doi: 10.3389/fenvs.2022.1057384
Received: 29 September 2022; Accepted: 20 October 2022;
Published: 10 November 2022.
Edited by:
Muhammad Azeem, Institute of Urban Environment (CAS), ChinaReviewed by:
Asfandyar Shahab, Guilin University of Technology, ChinaAudil Rashid, University of Gujrat, Pakistan
Copyright © 2022 Pan, Shi, Chen, Kuang, Ullah, Lu and Riaz. This is an open-access article distributed under the terms of the Creative Commons Attribution License (CC BY). The use, distribution or reproduction in other forums is permitted, provided the original author(s) and the copyright owner(s) are credited and that the original publication in this journal is cited, in accordance with accepted academic practice. No use, distribution or reproduction is permitted which does not comply with these terms.
*Correspondence: Shaoping Kuang, kuangshaoping@126.com; Luqman Riaz, luman.riaz@uon.edu.pk
†These authors have contributed equally to this work