- 1Institute of Landscape Planning and Ecology ILPÖ, Faculty of Architecture and Urban Planning, University of Stuttgart, Stuttgart, Germany
- 2Department of Architecture, Faculty of Engineering, Ain Shams University, Cairo, Egypt
- 3Department of Urban Design and Planning, Faculty of Engineering, Ain Shams University, Cairo, Egypt
- 4Integrated Urbanism and Sustainable Design (IUSD) Program, Ain Shams University, Cairo, Egypt
The building sector is one of the most resource-intensive industries. In Egypt, buildings consume 60% of electricity, produce 8% of CO2 emissions, and anthropize agricultural land, peri-urban and urban landscapes. To compensate for these consumption patterns, building envelopes can become productive in terms of greening and energy production. This encompasses the implementation of productive building systems that include (a) greening systems such as building-integrated vegetation and agriculture systems and (b) solar energy systems such as building-applied and integrated photovoltaics. For Egypt, the transformation toward more productive buildings still lacks a holistic understanding of their status and implementation requirements. This paper undergoes a comprehensive analysis of the two systems’ classifications, benefits, challenges, and implementation aspects based on a thorough assessment of 121 studies and 20 reports addressing them. This is coupled with a contextual analysis using questionnaires (n = 35) and semi-structured interviews (n = 13) with Egyptian experts and suppliers. Results showed that a large variety of systems is studied in literature and exists in the local market. Among the most purchased productive building systems in the Egyptian market, according to experts, are hydroponics (selected by 75% of respondents), planter boxes/pots (50%), roof-mounted photovoltaic panels (95%), and solar water heaters (55%). The main benefits of greening and solar energy systems are identified as enjoying the greenery view (95%) and reducing energy expenses (100%), respectively. The high initial cost was considered the main barrier for both systems. Multiple commonalities between the two systems in terms of spatial and environmental applicability aspects (e.g., accessibility and safety, net useable area, sun exposure, wind exposure) and environmental performance aspects (e.g., energy demand and emissions reduction, heat flow reduction) were identified. Lastly, we highlight the importance of analyzing integrated solutions that make use of the identified synergies between the systems and maximize the production potentials.
1 Introduction
Globally, with the extensive urbanization trends, the building sector is becoming one of the most resource-intensive industries (Gokarakonda et al., 2019), that is responsible for almost 30% of the global CO2 emissions (International Energy Agency IEA, 2015; Benis et al., 2017b). By 2050, 68% of the global population will be living in urban areas1. The high urban growth rates are leading to excessive demand for resources including food and energy (Roehr and Laurenz, 2008), diminishing of urban/peri-urban landscapes (Johnson, 2001), anthropization of agricultural land (Glaeser and Kahn, 2004), increase of energy consumption and greenhouse gas emissions (Glaeser and Kahn, 2004; Kahn, 2009), and decrease of urban green areas (Tan et al., 2020).
In Egypt, around 43% of the population currently lives in urban areas (CAPMAS, 2021), and by 2041, the urban population will exceed the rural one1. To cope with these rapid changes, the Egyptian government introduced several new cities and embarked on large-scale construction projects as part of the sustainable development strategy: Egypt’s vision 2030. The vision included the construction of the “1-million units” social housing project, massive road networks, and fourth-generation cities that expand into Egypt’s desert (El-Megharbel, 2015; CAPMAS, 2016; Abdel Raheem et al., 2020). 20 new cities are currently under construction to accommodate around 30 million inhabitants, and 17 more cities are in the planning phases (Attia et al., 2018; Reda, 2018).
The expanding new cities lead to high resource consumption patterns by the building sector. In Egypt, this sector alone consumes almost 60% of all electricity voltages (AbdelAzim et al., 2017; EEHC, 2020; Abdollah et al., 2021), 8% of total water consumption (The Trade Council, 2014), and produces around 8% of CO2 emissions (Abdallah, 2020; Al-Ayouty et al., 2021). It further anthropizes much of the agricultural land (Youssef et al., 2020). According to Radwan et al. (2019), from the year 1992–2015, more than 900 km2 of fertile agricultural land in Egypt was covered by urban areas, which is almost equal to the size of Berlin city (Germany).
Diminishing agricultural land and decreasing local food production have negative implications on the health and wellbeing of a large segment of the Egyptian population (UNICEF Country Office, 2015). Egypt witnesses high levels of malnutrition and obesity at 21.4% and 16% of the population, respectively, due to unhealthy dietary components and not consuming enough fresh vegetables and fruits2. Also, an increase in the level of food insecurity from 14% of the population to 17.2% was witnessed in 2011 (World Food Programme WFP, 2013; UNICEF Country Office, 2015).
For urban populations in Egypt, the dense urban areas’ expansions and the population increase lead to a decrease in the per capita share of green areas (Riad et al., 2020; Aly and Dimitrijevic, 2022). This has multiple negative impacts on health and wellbeing that usually improves when having contact with greenery or practicing gardening (Ghanbari, 2015; Soga et al., 2017). It also has many environmental implications such as the increasing greenhouse gas emissions coupled with the deteriorating air quality (El-Hattab et al., 2018; El-Kenawy et al., 2020). According to WHO target figures, the air quality is qualified as “moderate” in Egypt, but it is considered the 56th most polluted country out of 98 countries in total3. Greenhouse gas emissions are in continuous increase and their impacts not only affect the air quality but also contribute greatly to the urban heat island effect (Hassanein et al., 2014; El-Hattab et al., 2018; Sattler et al., 2020). This effect is very evident in Egyptian cities; it could reach 4.5°C—6.5°C higher temperatures in central Cairo, for example, compared to its peripheries depending on the season (El-Kenawy et al., 2020).
A compilation of the outlined phenomena results in a continuously increasing cooling demand in the Egyptian urban areas to maintain indoor thermal comfort for building users and face the increasing temperatures (Caponigro et al., 2020; Abdollah et al., 2021). Accordingly, the energy consumption by the building sector is speculated to continue increasing and is likely to follow a similar trend as when it increased from 150 GWh in 2009 to 217 GWh in 2017 (IRENA, 2018; Abdollah et al., 2021). This also implies an increase in greenhouse gas emissions and the global warming potential, pushing toward an endless cycle that could only be broken by improving the building sector’s performance.
It is thus necessary to find innovative ways of compensating for the high consumption patterns of buildings as well as reducing their ecological footprint. One way to achieve this is by functionalizing building envelopes and recognizing their productivity potential when integrating greening and solar energy systems into their components (Gorgolewski and Straka, 2017; Tablada and Chaplin, 2017; Kim et al., 2019; Kosorić et al., 2019). The building envelope was defined by Elder (2007) as “building components, which enclose conditioned spaces and through which thermal energy is transferred to/from the outdoor environment” (Abdel-Rahman, 2021; Singh et al., 2021), its elements (components) are mainly the rooftop, façade, and balcony. This study addresses the concept of productive buildings through adding new functions to their envelopes using Productive Building Systems (PBS).
The term “productive buildings” in literature usually refers to the productivity of building users and the building performance improvement to guarantee that (Clements-Croome, 2006, 2015; Harris, 2019). However, few studies used the term as a connotation of the buildings’ potential to produce resources such as food, vegetation, energy, etc. The term “productive façades” was introduced in a series of studies that integrate photovoltaics and farming systems on façades of residential buildings in Singapore (Tablada and Chaplin, 2017; Tablada and Kosoric, 2017; Kosorić et al., 2019; Tablada et al., 2020). Likewise, “productive rooftops” as a term was occasionally used to refer to different food and energy generation systems that can be installed on the roof (Benis et al., 2018; Corcelli et al., 2019). Also, the notion of “productive urban landscapes” covered concepts that integrate food and energy generation but in urban areas (Viljoen et al., 2005; Abbasi, 2017).
Similarly, our study uses the term “Productive Building Systems (PBS)” to refer to systems that could be implemented on the building envelopes (rooftop, façade, and balcony) to emulate ecosystem services and produce multiple resources. This includes, but is not limited to, producing vegetation that is also a habitat or foraging resource to species, producing food, generating renewable energy, purifying air and soil, mitigating greenhouse gas emissions, and compensating at least in parts for the land and habitat the building has replaced. In this sense, we understand the building as a part of the many heterogeneous environmental and spatial settings of cities that can serve as novel kinds of ecosystems for a range of species (Lundholm and Richardson, 2010; Kowarik and von der Lippe, 2018).
Beside their direct productivity benefits, PBSs have many other indirect benefits to offer. These include contributing to the buildings’ efficiency by reducing heat/solar gains and energy consumption, as well as improving the indoor thermal and visual comforts (Gupta et al., 2011; Cucchiella and Dadamo, 2012; Attoye et al., 2017; Tablada and Kosoric, 2017). This study defines PBSs to include two prevailing productive systems (Figure 1) that are defined below:
(a) Greening systems such as Building-Integrated Vegetation (BIVs) and Building-Integrated Agriculture (BIAs) systems.
(b) Solar energy systems such as Building-Applied Photovoltaics (BAPVs) and Building-Integrated Photovoltaics (BIPVs).
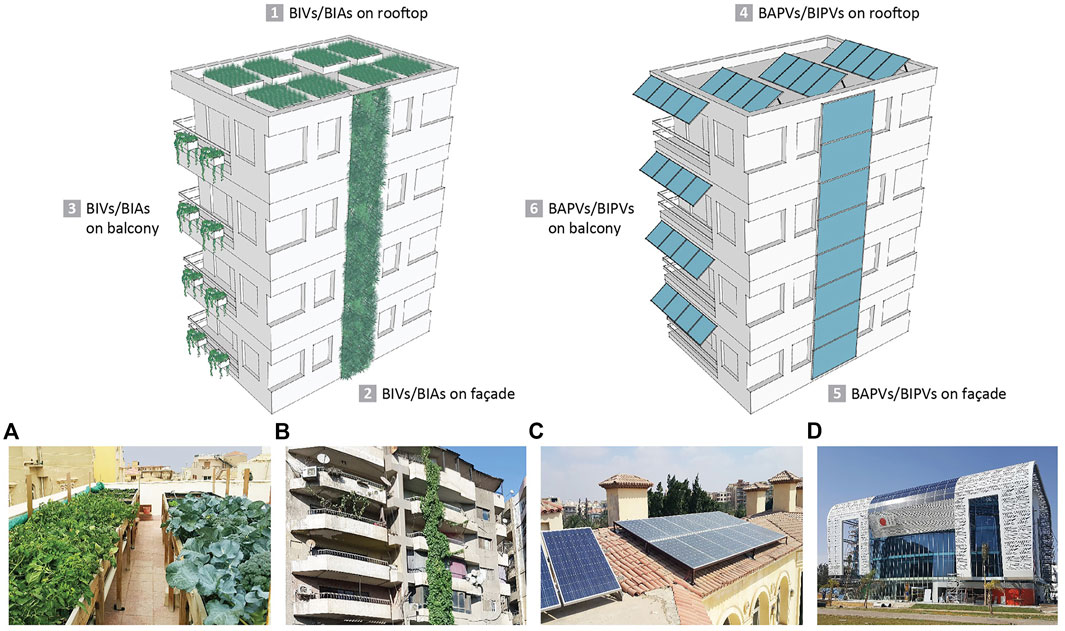
FIGURE 1. Productive Building Systems (PBSs) types (greening and solar energy systems) and their installation options onto building envelopes (rooftop, façade, and balcony). BIVs, Building-Integrated Vegetation systems; BIAs, Building-Integrated Agriculture Systems; BAPVs, Building-Applied Photovoltaics; and BIPVs, Building-Integrated Photovoltaics. (A): BIAs on a rooftop in “New Cairo”, (B): BIVs on a residential building façade in “Haram”, (C): BAPVs on a rooftop in “New Cairo”, (D): BIPVs on as a double skin façade in the “Smart Village”. Copyright of photos (A)–(C): Mai Marzouk, photo (D): Summit (2018).
The first type of production is growing vegetation and food using different greening systems. BIAs were first defined by Caplow and Nelkin (2007) as high-performance farming and gardening systems, implemented on/in buildings using renewable, local sources of energy and water (Gould and Caplow, 2012; Eigenbrod and Gruda, 2015; Benis et al., 2017b; 2017a). The term was also used to refer to the application of horizontal planters on the façade element and balcony railings (Tablada and Chaplin, 2017; Tablada and Kosoric, 2017). In our study, it refers to all farming and gardening systems spanning from the simplest Do-It-Yourself (DIY) to the most high-tech systems that are implemented on the building envelope elements to produce edible plants (e.g., vegetables, fruits, herbs, etc.).
The study further differentiates BIAs from BIVs whose main aim is to plant vegetation and decorative plants (e.g., flowers, succulents, foliage plants, etc.) on building envelope elements (Gupta et al., 2011; Lee, 2014). Often, this is done to compensate for the limited green areas on ground, produce more oxygen, improve air quality, and achieve multiple aesthetical purposes (Gupta et al., 2011; Lee, 2014). It is also referred to as vertical greening systems when systems are implemented on the building façade (Gupta et al., 2011; Radić et al., 2019; Tan et al., 2020).
The second type of production covered by our research is energy production through the implementation of solar energy systems on the building envelope elements. This type is defined by two forms (Anduła and Heim, 2020; Corti et al., 2020; Krawczak, 2020; Reddy et al., 2020; Singh et al., 2021): BAPVs where the system is just mounted or externally attached to the building envelope (usually used in existing buildings; Corti et al., 2020), and BIPVs where the system replaces some of the building envelope components and becomes an integral part of the envelope (usually used in new buildings; Tripathy et al., 2016). In both cases, the systems could be applied to the building rooftops, façades (including windows), or balconies (Singh et al., 2021).
In light of the above, the main goal of our study is to investigate the situation and opportunities available for the identified PBSs with special reflection on the context of Egypt. It aims at creating a comprehensive inventory of the four systems’ types (BIVs, BIAs, BAPVs, and BIPVs), a clear outline of their benefits and challenges, and a framework of their manifold implementation requirements. To the best of our knowledge, such a compilation of these three topics was not identified in the reviewed studies from the literature. Previous studies have partially conducted analyses for greening and solar energy systems, but mostly individually and in temperate or humid tropical climates. However, perceiving them as several components of productive systems and comparing their status, requirements, and performances is understudied. Moreover, there are hardly any studies with a similar focus, of comparing both systems, for the context of Egypt as an example of an arid and highly urbanizing region.
Against this background, the paper replies to three research questions:
(1) What are the available types and classifications of Productive Building Systems (PBSs; including BIVs, BIAs, BAPVs, and BIPVs) in theory and practice in Egypt?
(2) What are the benefits achieved and challenges faced by PBSs in theory and practice in Egypt?
(3) What are the key aspects considered for PBSs implementation on building envelopes?
Updating the current knowledge of available PBSs in the Egyptian market to better understand the different options and opportunities is one of the study’s main outcomes. In addition, it could be considered as a guide to the building sector stakeholders; architects, engineers, residents, and government officials to ensure higher implementation rates and productivity of the systems. We anticipate that the study outcomes are beneficial for those involved in sustainable city planning in countries also beyond Egypt. The theoretical analysis outcomes based on literature could be useful to different countries being of an international focus. While, the contextual analysis of the Egyptian case could benefit countries of similar climatic, social, and economic conditions. Our assessment can thus help support the transformation to productive buildings that achieve several economic, social, and environmental benefits and contribute to overcoming many challenges facing the built environment.
2 Methodology
The study covered three main topics regarding greening and solar energy systems based on insights from theory and practice in Egypt. With a mixed-methods approach, it identified for each system (a) the types and classifications, (b) the expected benefits and challenges, and then outlined (c) the key applicability and performance aspects related to implementation requirements.
2.1 Literature assessment
We conducted a thorough assessment of English language studies and reports of similar focus to the study topics on Google Scholar, Scopus, and Google search engine. Multiple keywords were used to identify the starting set. It continued using snowball sampling to identify other relevant studies. Keywords used for the productive building envelope topic were “productive building systems”, “productive rooftops”, and “productive façades”. For the greening systems, we used “Building-Integrated Vegetation (BIV)”, “Building-Integrated Agriculture (BIA)”, “green wall/façade”, “living wall/façade”, “green roof”, and “rooftop agriculture”. For the solar energy systems, the keywords were “Building-Applied Photovoltaics (BAPV)”, “Building-Integrated Photovoltaics (BIPV)”, “solar façade”, and “solar rooftop”.
The studies were selected to cover one or more of the three main topics outlined above. They could cover one of the two systems, or one or more subsystems in detail, or cover the two types of systems together if any. Applying the aforementioned criteria, the selected studies totaled 121 from literature, which provided a good basis for our analysis. 104 studies had an international focus, while 17 studies were focused on Egypt, developing countries, or similar climates. Supplementary research was done for technical reports, project reports, and guidelines of both systems. 20 reports and guidelines were consulted with the rationale of having an additional layer of practice input. Such reports usually build on knowledge from implemented projects or market-driven experiences.
2.2 Questionnaires and interviews
To contextualize the analysis, the literature assessment was followed by an investigation of the systems’ status in the Egyptian market. The methods used were an online questionnaire and semi-structured interviews with Egyptian experts and suppliers of both systems. Using SurveyMonkey, we developed two online surveys following the same structure but with system-related differences when needed, for the greening and solar energy systems’ experts. The surveys were developed in English, and translated into Arabic. Respondents had the chance to select the language they prefer for the questions and answers. The surveys were composed of 32 questions in total that investigated the multifaceted views of key market players on the systems’ present and future status. This study reported on the results of three main questions related to the scope of the conducted analysis whereas the first is a question about the systems of highest sales in the market with a special focus on residential buildings. The second and third questions ask about the customer’s aims when purchasing the systems, and the barriers that might hinder their successful implementation. This reflects the local perspective of the benefits and challenges facing the systems’ implementation in the context.
The survey was tested with a small test sample of five experts (with whom we initiated a first contact) to check the clarity and validity of the questions. Existing systems’ suppliers and companies in the market were mapped, and purposive sampling was used to guarantee the representation of the most relevant and influential market players in the sample. Desktop research and snowballing techniques were used to reach the final list of contact persons in the selected companies. Contact was initiated via phone and they were invited to participate in the 10-min online survey. Some of the interested experts and suppliers were further interviewed to ask more questions about the available systems and the current market trends. Semi-structured interviews were carried out online, over the phone, or in person depending on the experts’ preference and COVID-19-related regulations. In total, 45 experts and suppliers were purposively selected and contacted, 35 survey responses were received for both systems (n = 15 for greening and n = 20 for solar) and 13 interviews were conducted (n = 6 for greening and n = 7 for solar). 28 experts responded only to the survey, six experts responded only to the interview, and seven experts participated in both with a total of 41 participating experts (response rate of 90%). The surveys and interviews were carried out over the period from 18 March to 5 June 2022. Data collected from both tools was compiled to serve the purpose of the analysis.
2.3 Data preparation and analyses
Assessed studies were thematically classified according to their relevance to the three study topics: systems’ types and classifications, expected benefits and challenges, and key implementation aspects. Collected data was then analyzed and synthesized to identify connections and gaps across the studies, and compare their results about the different systems and subsystems. The survey had a qualitative nature being interested in the value of each response and its contribution to the research. Therefore, participants were recruited so that they could provide in-depth detailed information about the systems’ status in the market. Data from the survey was complemented by the responses retrieved from the follow-up semi-structured interview. All responses were transcribed and the systems’ types identified, at least once, by the interviewees were recorded, mapped, and reported on.
The outcome of all data sources was overlaid to formulate the two study sections (Figure 2). Section 3.1, gives an overview of the PBSs, which is divided into two subsections, (a) Building-Integrated Vegetation and Agriculture Systems, and (b) Building-Applied and Integrated Photovoltaics. In this section, the assessed studies and reports (n = 82) were used to map the existing types and classifications of each system and to give an outlook on their potential benefits and challenges. The contextual layer was added by identifying the types existing in the local market as well as the main benefits and challenges from this perspective. Section 3.2, focuses on outlining the main aspects that identify the PBSs applicability on a specific building, and aspects that guide the detailed systems’ selection based on their expected performance. It further aims at categorizing these aspects with the two systems in focus to highlight the arising synergies or competitions. Out of the 139 total studies, 67 were partially or fully concerned with the manifold implementation aspects related to applicability and/or performance.
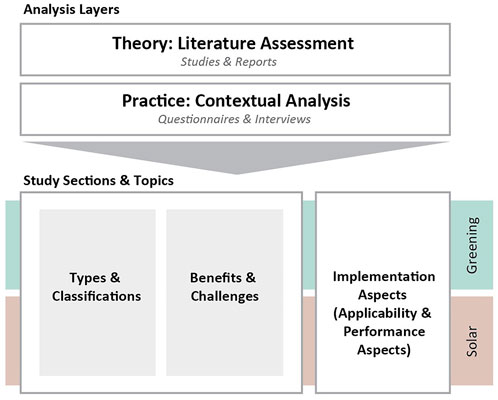
FIGURE 2. Research methodology combining the literature assessment of relevant studies and reports, and the contextual analysis based on questionnaires and semi-structured interviews. The results are reported in two sections. Section 3.1 gives an overview of PBSs types and classifications, benefits, and challenges. Section 3.2 covers the applicability and performance aspects that guide the implementation. In both sections, a comparison of the greening and solar systems is illustrated.
3 Results
3.1 Section 1: Overview of productive building systems (PBSs)
Our study attempted to systematically build the classifications of the greening and solar energy systems on different building envelope elements from literature with an additional reflection on the Egyptian local context. It makes a crucial differentiation between BIVs and BIAs and between BAPVs and BIPVs with a thematic grouping of their benefits and challenges.
3.1.1 Building-integrated vegetation and agriculture systems (BIVs and BIAs)
3.1.1.1 Types and classifications
Our assessment of studies showed that BIVs aim at adding vegetation (greenery or decorative plants) to the envelope elements for aesthetic and environmental purposes (Gupta et al., 2011; Lee, 2014). BIVs were classified into green roofs that can be intensive, semi-intensive, or extensive, and green walls that have multiple subsystems (DEPI, 2014; Lee, 2014; Raji et al., 2015; Besir and Cuce, 2018; Figures 3, 4). The most common subsystems of green walls are the Green façades that grow climbing plants, and the Living Wall Systems (LWS) that use continuous panels or modular containers (Perini et al., 2011; GWG, 2013; Raji et al., 2015; Besir and Cuce, 2018; Figure 4).
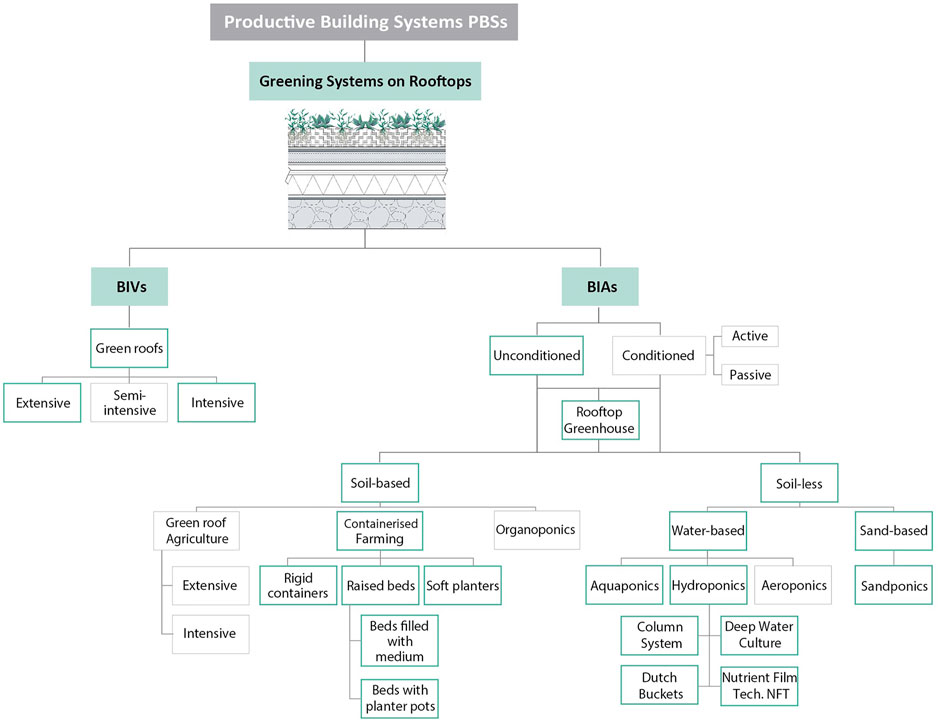
FIGURE 3. Inventory of greening systems (BIVs and BIAs) on rooftops, compiling the types and classifications identified from assessed studies and reports to create the body of classification (all boxes). Structured based on (Savvas 2013; Raji et al., 2015; Caputo et al., 2017; Rodríguez-Delfín et al., 2017; Benis et al., 2018; Ledesma et al., 2020) with additions. Systems existing in the Egyptian market are highlighted by the green-bordered boxes. PBSs: Productive Building Systems, BIVs, Building-Integrated Vegetation systems; BIAs, Building-Integrated Agriculture Systems; DWC, Deep Water Culture; NFT, Nutrient Film Technique.
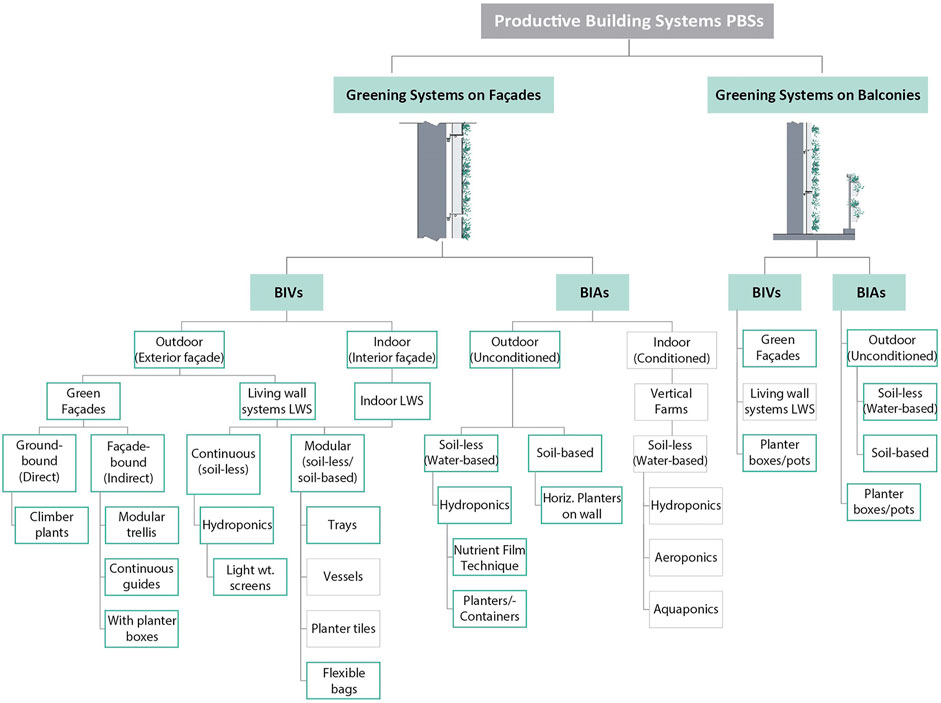
FIGURE 4. Inventory of greening systems (BIVs and BIAs) on façades and balconies, compiling the types and classifications identified from assessed studies and reports to create the body of classification (all boxes). Structured based on (Perini et al., 2011; Gould and Caplow, 2012; GWG, 2013; DEPI, 2014; Raji et al., 2015; Tablada and Kosoric, 2017; Besir and Cuce, 2018) with additions. Systems existing in the Egyptian market are highlighted by the green-bordered boxes. For balconies, all systems can be installed on the railing/parapet, and the planter boxes/pots can also be laid on the floor. PBSs: Productive Building Systems, BIVs, Building-Integrated Vegetation systems; BIAs, Building-Integrated Agriculture Systems; LWS, Living Wall System; NFT, Nutrient Film Technique.
The green balcony is briefly tackled in some studies (Raji et al., 2015; Besir and Cuce, 2018). It has the potential of maximizing the benefits by combining the systems’ implementation on the façade (acting as a balcony wall) with their implementation on railings and parapets (Figure 4). We identified that the subsystems’ terms are not always consistent across studies (Radić et al., 2019) and need further clarification (Supplementary Table S1; Supplementary Table S3).
On the other side of production, BIAs come in a wide array of subsystems differentiated based on the growing medium into soil-based versus soil-less systems (Lab, 2011; Rodríguez-Delfín et al., 2017). Three overarching types of BIAs on rooftops were identified (Caputo et al., 2017). Firstly, informal rooftop agriculture where low-tech and low-cost containers and wooden raised-beds are installed directly on the waterproofed roof deck (Lab, 2011; Goldstein et al., 2016; Marzouk, 2016). Secondly, formal rooftop referring to intensive and extensive options for food production (Benis et al., 2018; Ledesma et al., 2020). Thirdly, technological rooftop indicating environmentally controlled soil-less systems installed in rooftop greenhouses to maximize production (Savvas, 2013; Rodríguez-Delfín et al., 2017; Nexus, 2021). To complement that, some studies listed detailed types of hydroponic systems that intensify production including the Nutrient Film Technique (NFT), Deep Water Culture (DWC), Dutch buckets, and column systems (Savvas, 2013; George and George, 2016; Gould, 2019; Figure 3, Supplementary Table S1, Supplementary Table S3).
Assessed studies barely discussed the different types of BIAs on façades, yet each study focused on one specific system. Based on these, we classified them into firstly, indoor conditioned systems (vertical farms) that are implemented as double-skin façades combining different soil-less systems for indoor crop cultivation (Gould and Caplow, 2012; Despommier, 2013; Eigenbrod and Gruda, 2015; Jenkins et al., 2015; Benis et al., 2017b; Saxena, 2021). Secondly, outdoor unconditioned systems that are installed as modular systems on external façades, balcony walls, and railings/parapets (Tablada and Chaplin, 2017; Tablada and Kosoric, 2017; Tablada, 2018, 2020).
Pinpointing the systems’ status in the Egyptian market was sought in the semi-structured interviews by asking experts and suppliers about the types that are currently existing in the market. The identified systems were depicted on the figures using bold green borders (Figure 3, Figure 4). For the rooftops, all identified systems from the literature are said to exist in the market except for the green roof agriculture and organoponics. In addition, the conditioned systems and aeroponics exist for large-scale on-ground farms but not for rooftops. For the façades and balconies, the identified living wall system categories mainly refer to indoor applications with very few attempts for the outdoor ones. The rest of the façade types witnessed limited local implementation attempts by a few suppliers.
Responses to the survey question about “greening systems of highest sales in the market” were used to verify the interviews’ insights. Sales is defined as the number of systems sold annually and the respondents could select multiple options of systems (X = 41 total responses; Figure 5). As shown, the NFT hydroponic system is claimed to achieve the highest sales in the market by 75% of the respondents, followed by the more conventional planter boxes and pots, selected by 50% of the respondents, and the low-tech raised beds (soil-based) system by almost 42%.
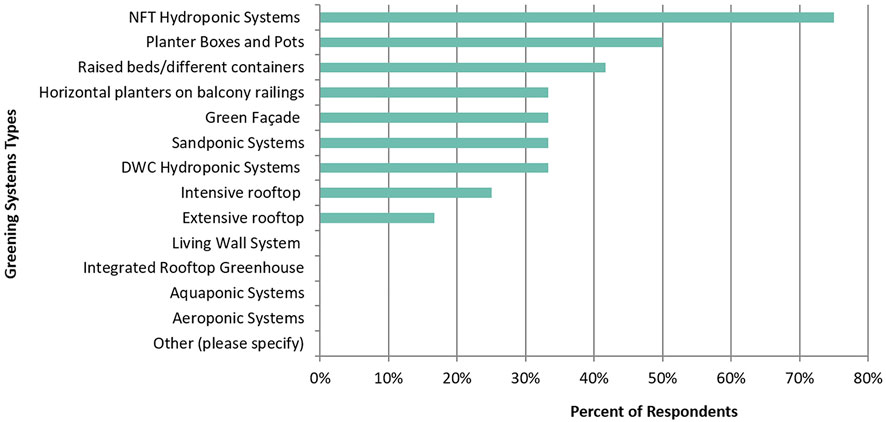
FIGURE 5. Results from the online survey targeting greening systems’ experts and suppliers. It shows the greening systems that achieve the highest sales in the Egyptian market based on the responses (n = 15 out of 15 total respondents). Sales was defined as the number of systems sold annually, and the respondents could select multiple options of systems (X = 41 total responses). NFT, Nutrient Film Technique; DWC, Deep Water Culture.
3.1.1.2 Benefits and challenges
BIVs and BIAs offer multiple benefits to the urban population regarding health and social life. BIAs can guarantee urban residents’ access to healthier food products thus improving health and wellbeing (Astee and Kishnani, 2008; Gould and Caplow, 2012; GWG, 2013). Better health conditions extend beyond this to the psychological and mental gains of nature exposure and having better aesthetics in the built environment (Gould and Caplow, 2012; Lee, 2014; Besir and Cuce, 2018; Tan et al., 2020). In addition, such building-integrated gardens and farms allow for community engagement and social connections among neighbors (Astee and Kishnani, 2008; DEPI, 2014) as well as provide multiple educational activities for the community’s children (Eigenbrod and Gruda, 2015; Radić et al., 2019; Ledesma et al., 2020).
A long list of environmental benefits of BIVs and BIAs can be identified from the literature (see performance aspects in Table 2). A brief list includes: increasing the share of green spaces (Tan et al., 2020) and improving the microclimate and air quality (Birkeland, 2009; Gould and Caplow, 2012; Lee, 2014). On the urban scale, they alleviate the urban heat island effect (Birkved et al., 2016; Besir and Cuce, 2018; Tan et al., 2020), reduce stormwater runoff (Birkeland, 2009; Lee, 2014), increase biodiversity, and provide habitat for multiple species (Perini et al., 2011; GWG, 2013; Besir and Cuce, 2018; Radić et al., 2019). On the building scale, they improve the envelope insulation (DEPI, 2014; Raji et al., 2015; Besir and Cuce, 2018; Tan et al., 2020), reduce air temperature and cooling/heating demand, reduce energy demand (Gupta et al., 2011; Perini et al., 2011; GWG, 2013; Raji et al., 2015), and reduce greenhouse emissions (Birkeland, 2009; Gould and Caplow, 2012). BIAs further reduce the emissions resulting from food transportation owing to on-site production (Gould and Caplow, 2012; Birkved et al., 2016; Benis et al., 2017b, 2018; Gorgolewski and Straka, 2017).
Economically, BIAs contribute to reducing household food expenses by on-site food production for self-sufficiency (Attia and Amer, 2009; Rodríguez-Delfín et al., 2017). Also, they reduce electricity bill expenses because of their role in decreasing the energy demand (Gould and Caplow, 2012). Such systems are argued to increase property values as a result of improved aesthetics and environmental performance (Birkeland, 2009; DEPI, 2014; Benis et al., 2018; Radić et al., 2019). Selling the produce to gain revenue is another potential benefit that proved feasibility under certain conditions including the system area, production yield, and access to the market (Attia and Amer, 2009; Marzouk, 2018).
Our assessed studies show that multi-layered challenges are hindering the systems’ wide implementation. It is claimed that many systems are not yet financially feasible, especially the high-tech BIAs that aim at maximizing productivity gains (Gould and Caplow, 2012; Reese, 2014; Eigenbrod and Gruda, 2015; Ledesma et al., 2020). In addition, the lack of financial incentives and governmental policy support slows down the uptake (Kortright, 2001; Birkeland, 2009). Limited awareness of the systems’ manifold benefits, costs, and performances, coupled with the lack of demonstration pilot projects are key challenges among the communities (Kortright, 2001; Birkeland, 2009; Reese, 2014). Attention is usually given to the environmental benefits and production capacity, while overlooking the social aspects and people’s preferences, which hinders the uptake (Ledesma et al., 2020). Lastly, having no adequate guidelines that set the systems’ implementation standards, challenges their successful adoption by buildings (Birkeland, 2009; Reese, 2014).
Zooming in on the Egyptian practice, experts and suppliers were asked in the survey about the main motivators and barriers for customers when implementing the greening systems (Figure 6). The respondents were asked to select the four most important options in each question (X = 35 total responses for the benefits and X = 36 for the barriers). Interestingly, enjoying the aesthetic view of greenery came as the customers’ first priority, selected by more than 95% of the respondents. The self-sufficiency aims and production of healthier produce came second. The economic benefit of reducing household expenses came at a later stage, only selected by 44% of the respondents. On the other hand, the systems’ high initial costs were perceived as the main barrier faced by the customers as indicated by almost 80% of their responses. The lack of trust in the systems’ quality and high demand for knowledge of the operation were selected by 55% of the respondents.
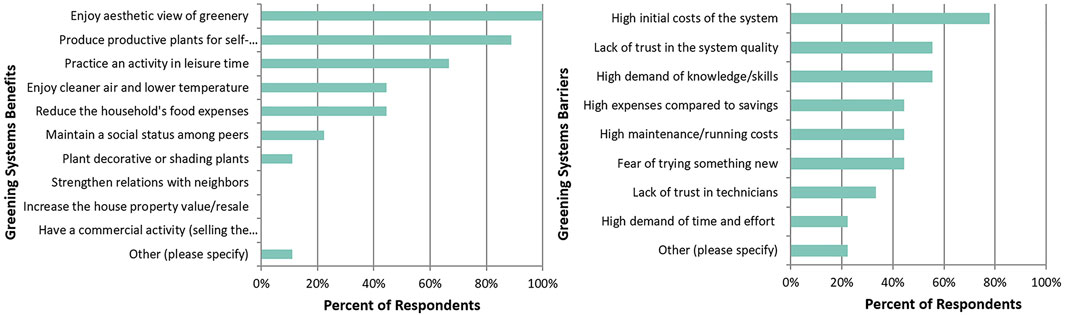
FIGURE 6. Results from the online survey showing the opinions of greening systems’ experts and suppliers about the customers’ perceptions of the main benefits and barriers facing the systems (n = 12 out of 15 total respondents). The respondents were asked to select the four most important options in each question (X = 35 total responses for the benefits question and X = 36 for the barriers).
3.1.2 Building-applied and building-integrated photovoltaic systems (BAPVs and BIPVs)
3.1.2.1 Types and classifications
Our study classifies solar energy systems based on two aspects identified from the assessed studies: the application that differs according to the installation mechanism on the building envelope (BAPV, BIPV), and the technology that differs according to the production outcome (electrical energy by photovoltaics (PVs), thermal energy by solar thermal systems (STs), electrical and thermal energy by Photovoltaic thermal systems (PVTs; Figure 7).
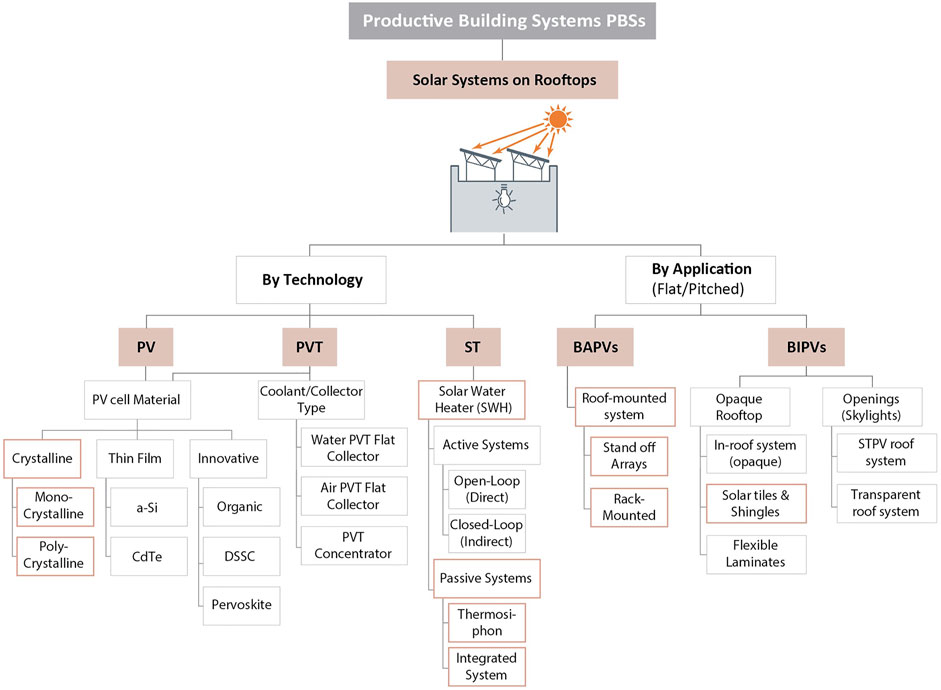
FIGURE 7. Inventory of solar energy systems (BAPVs and BIPVs) on rooftops, compiling the types and classifications identified from assessed studies and reports to create the body of classification (all boxes). Structured based on (Stephen and James, 2001; Tyagi et al., 2012; Tripathy et al., 2016; Ghosh, 2020; Reddy et al., 2020; Dehghan et al., 2021; Singh et al., 2021) with additions. Systems existing in the Egyptian market are highlighted by the brown-bordered boxes. PBSs, Productive Building Systems; BAPVs, Building-Applied Photovoltaics; BIPVs, Building-Integrated Photovoltaics; PVs, Photovoltaic panels; PVTs, Photovoltaic thermal systems; STs, Solar Thermal systems; SWH, Solar Water Heaters; a-Si, Amorphous silicon cells; CdTe, Cadmium telluride cells; DSSC, Dye-synthesized solar cell; STPV, Semi-Transparent Photovoltaics.
BAPVs are considered add-ons to the envelope element, mounted using a structural system (Stephen and James, 2001; Wu et al., 2015; Kumar et al., 2019; Anduła and Heim, 2020). Two subsystems of rooftop BAPVs were identified (Figure 7): standoff arrays (parallel to pitched roof), and rack-mounted arrays (tilted to flat roofs; Stephen and James, 2001; Samir and Ali, 2017; Cho et al., 2019; Ghosh, 2020). Façade BAPVs are almost undiscussed in the studies, they are only referred to as systems directly mounted using different mechanisms (Saber et al., 2014; Ghosh, 2020). The balcony has the additional privilege of mounting the panels on its parapets, railings, or as tilted shading devices to increase the production (Boemi et al., 2016; Tablada and Zhao, 2016; Tablada and Chaplin, 2017; Figure 8).
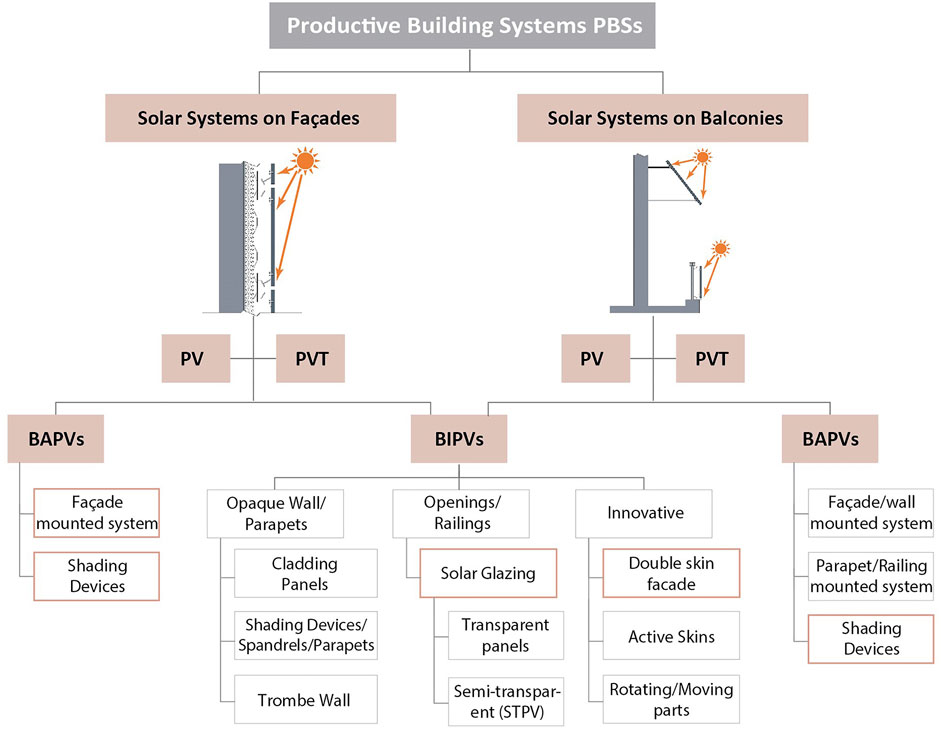
FIGURE 8. Inventory of solar energy systems (BAPVs and BIPVs) on façades and balconies, compiling the types and classifications identified from assessed studies and reports to create the body of classification (all boxes). Structured based on (Saber et al., 2014; Boemi et al., 2016; Attoye et al., 2017; Biyik et al., 2017; Shukla et al., 2017; Ghosh, 2020; Singh et al., 2021) with additions. Systems existing in the Egyptian market are highlighted by the brown-bordered boxes. PBSs, Productive Building Systems; BAPVs, Building-Applied Photovoltaics; BIPVs, Building-Integrated Photovoltaics; PVs, Photovoltaic panels; PVTs, Photovoltaic thermal systems; STPV, Semi-Transparent Photovoltaics.
BIPVs are installed by replacing the envelope’s components (Kumar et al., 2019; Anduła and Heim, 2020; Corti et al., 2020; Singh et al., 2021). Five main categories were outlined for BIPVs depending on the material and position on the rooftop or façade (Figure 7, Figure 8): 1) In-roof systems for flat or pitched roofs, 2) semi-transparent PVs for skylights, windows, and curtain walls, 3) cladding systems for opaque walls, 4) PV tiles and shingles for pitched roofs or façade cladding, and 5) flexible laminates that could be pasted to different surfaces (Tripathy et al., 2016; Shukla et al., 2017; Reddy et al., 2020; Supplementary Table S2; Supplementary Table S3). A systematic study by Attoye et al. (2017) added the following to the already mentioned façade BIPVs: external solar devices (such as shading elements, spandrels, balconies parapets, etc.) and innovative systems (such as double skin façades, rotating and moving systems, etc.). In addition, several studies focused on PV shading devices that are implemented on balconies (Tablada and Chaplin, 2017; Tablada and Kosoric, 2017; Kosorić et al., 2018; Tablada, 2018, 2020).
PV technology options encompass first generation PVs (crystalline silicon cells), second generation PVs (thin films), as well as third generation PVs (innovative cells; Cucchiella and Dadamo, 2012; Biyik et al., 2017; Anduła and Heim, 2020; Reddy et al., 2020; Singh et al., 2021; Figure 7 and Supplementary Table S2). According to Reddy et al. (2020), the first generation PVs (crystalline silicon cells) are used for both BAPVs and BIPVs, while the second and third generations are more commonly used for BIPV applications. Ghosh (2020) claims that the first and second generations are primarily used for rooftop and façade BAPV and BIPV applications, however; the third generation (Almosni et al., 2018; Diwania et al., 2020; Mariotti et al., 2020) is not yet common for BAPV or BIPV façades.
Other technologies include Photovoltaic Thermal Systems (PVTs) and Solar Thermal Systems (STs). We have adopted the PVT systems’ classification into water flat collector, air flat collector, and PVT concentrator (Othman et al., 2005; Tyagi et al., 2012; Babu and Ponnambalam, 2017; Diwania et al., 2020), which could use one of the three PV generations (Babu and Ponnambalam, 2017; Diwania et al., 2020). Multiple studies consider PVs and PVTs as being suitable for the same applications: either mounted on or integrated into the building envelope (BAPVT, BIPVT; Yang and Athienitis, 2016; Biyik et al., 2017; Anduła and Heim, 2020; Kaplanis et al., 2022). For STs, we focused on Solar Water Heaters (SWHs), which are established technologies usually implemented on rooftops and can be active or passive depending on the water heating method (Ogueke et al., 2009; Tyagi et al., 2012; Dehghan et al., 2021; Figure 7, Supplementary Table S2; Supplementary Table S3).
Interviews with experts and suppliers investigated the solar energy systems that exist in the Egyptian market, which are highlighted in bold brown boxes (Figure 7, Figure 8). For the technology classification, first generation crystalline PVs were identified to exist in the market in addition to the passive SWHs, while photovoltaic thermals were not locally found (Figure 7). For the application classification, all rooftop BAPVs are said to have been implemented before, while the rooftop BIPVs are not sold in the Egyptian market except for the solar tiles and shingles. For the façades and balconies, BAPVs have few implementation attempts such as façade mounted systems and shading devices. Solar glazing and double skin façades were the only façade BIPVs mentioned in the interviews (one application), the rest do not exist.
Systems’ experts and suppliers were asked the same survey question about the “systems that have the highest sales”. Sales is defined as the number of systems sold annually and the respondents could select multiple options of systems (X = 30 total responses). In this case, only three systems were selected to have the highest sales, which are the PVs on rooftops (BAPVs) selected by almost 95% of respondents, followed by SWHs, a common solar thermal system selected by 55%, while the solar rooftop tiles, usually on pitched roofs, were the choice of only 11% of the respondents. The rest of the options (e.g., solar glazing, solar shading elements, cladding tiles, PVT systems, etc.) were not selected by the respondents (Figure 9).
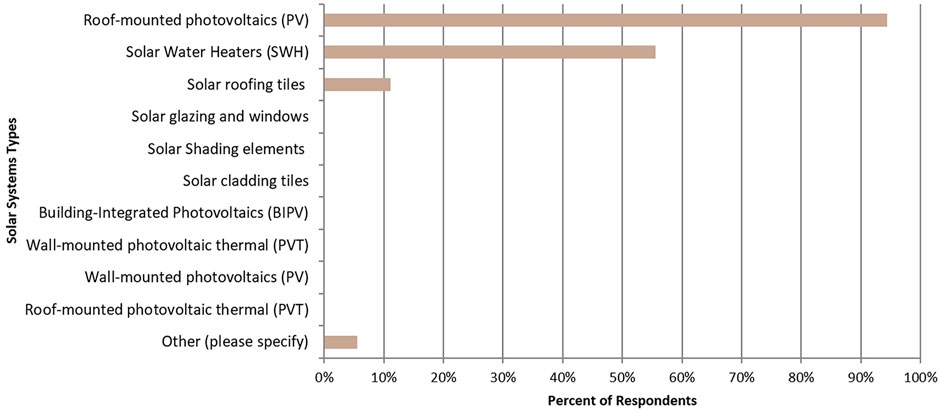
FIGURE 9. Results from the online survey targeting solar systems’ experts and suppliers. It shows the solar systems that achieve the highest sales in the Egyptian market based on the responses (n = 20 out of 20 total respondents). Sales was defined as the number of systems sold annually and the respondents could select multiple options of systems (X = 30 total responses). BIPV, Building-Integrated Photovoltaics; PV, Photovoltaic panels; PVT, Photovoltaic Thermal systems; SWH, Solar Water Heaters.
3.1.2.2 Benefits and Challenges
Renewable energy generation from solar resources to achieve self-sufficiency is the most valuable technical performance benefit provided by the systems (Attoye et al., 2017; Asfour, 2018; Corcelli et al., 2019; Corti et al., 2020; Ghosh, 2020). BAPV and BIPV systems can provide the dual function of producing energy as well as reducing energy demand in buildings (Singh et al., 2021). When installed on rooftops/façades, they act as an insulation layer from external conditions and reduce heat flow through the envelope (Attoye et al., 2017; Martín-Chivelet et al., 2018; Ghosh, 2020; Reddy et al., 2020; Singh et al., 2021). Thus, indoor thermal comfort is improved, cooling demand is reduced (Elsayed, 2016), and energy demand decreased becoming more reasonable to be covered by the amount of generated renewable energy (Corcelli et al., 2019; Corti et al., 2020; Ghosh, 2020; Reddy et al., 2020). Transparency of semi-transparent/transparent PV panels allows for their integration as solar glazing without obstructing the internal views to the outside and allows for daylight transmission inside the space, thus reducing the lighting demand as well (Attoye et al., 2017; Ghosh, 2020).
Such capacities can be translated to multiple environmental benefits and gains. Renewable energy generation reduces greenhouse gas emissions and contributes to climate change mitigation (Cucchiella and Dadamo, 2012; Attoye et al., 2017; Anduła and Heim, 2020; Ghosh, 2020). Cutting down energy demand leads to further emissions reduction, which maximizes the environmental gains and results in overall air quality improvement (Attoye et al., 2017; Corcelli et al., 2019; see performance aspects in Table 2).
From an economic perspective, self-sufficiency of electricity production on the building scale reduces household expenses on electricity bills (Attoye et al., 2017). If the system is connected to the grid with financial incentives in place, it could also achieve cost savings and financial gains by selling the extra electricity to the national network (Anduła and Heim, 2020; Ghosh, 2020; Dehghan et al., 2021). On a larger scale, on-site energy generation can reduce the costs of energy transmittance from central generation plants (Attoye et al., 2017; Ghosh, 2020). In addition, the process of production, installation, and maintenance creates a large number of job opportunities for the community (Boemi et al., 2016).
According to Attoye et al. (2017), challenges are faced at different implementation phases starting from the limited knowledge of the system’s design and installation, to the lack of systems’ marketing, to the cost issues (Ghosh, 2020; Reddy et al., 2020; Dehghan et al., 2021). Financially, the systems’ costs are still not competitive in comparison to conventional energy sources, with unattractive long payback periods (Cucchiella and Dadamo, 2012; Ghosh, 2020; Dehghan et al., 2021). On the community side, the lack of awareness of the systems’ production capacity and the limited demonstration projects slow down their market uptake (Attoye et al., 2017; Ghosh, 2020; Dehghan et al., 2021). On a technical level, the panels’ low efficiency is a real challenge for some climates where it is highly impacted by the increase in surface temperature leading to overall productivity reduction (Cucchiella and Dadamo, 2012; Tripathy et al., 2016; Ghosh, 2020).
In the survey, experts and suppliers were asked to select the four most important motivators and barriers for customers when implementing the systems (X = 68 total responses for each of the benefits and barriers). For solar energy systems, reducing household expenses was identified as the main motivator for customers when implementing the systems, it was selected by almost all surveyed experts. Related to that, the production aims came next where the electricity production for self-consumption was selected by 82% and hot water generation for the household was selected by 65%. Operating the house off-grid seems to be currently the least priority. The foreseeable barrier of high initial costs was the most selected by 94% of respondents. Fear of trying something new was the second barrier followed by the lack of trust in the system quality selected by 82% and 76% of respondents, respectively (Figure 10).
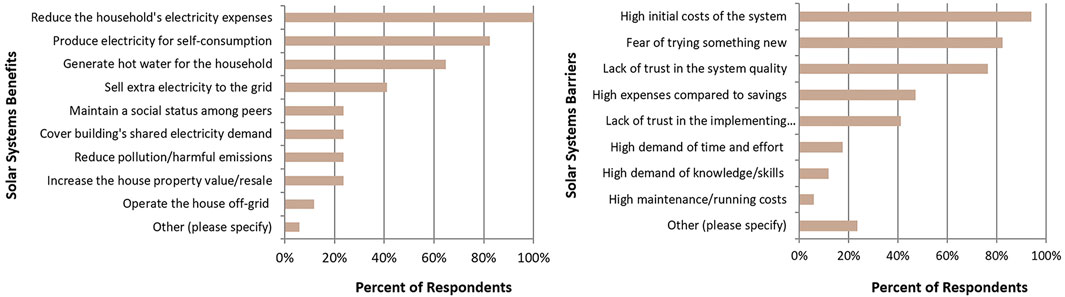
FIGURE 10. Results from the online survey showing the opinions of solar energy systems’ experts and suppliers about the customers’ perceptions of the main benefits and barriers facing the systems (n = 18 out of 20 total respondents). The respondents were asked to select the four most important options in each question (X = 68 total responses for each of the benefits and barriers questions).
3.2 Section 2: Implementation aspects for productive building systems (PBSs)
Based on the assessed studies and reports, the study at hand mapped and compiled several aspects related to the systems’ implementation requirements. It then created a holistic framework where the aspects are mapped against the type of system (greening or solar energy) and the building envelope element (rooftop or façade).
3.2.1 Overview of key implementation aspects
Reese (2014) has identified a methodology for determining the greening systems’ potential on rooftops by considering the available rooftop area, food production potential (produce yield), and revenue potential. Several spatial aspects were further defined such as the building use, building height, rooftop construction, rooftop area, load bearing capacity, existence of obstruction elements on the roof, rooftop accessibility, and solar potential (Ackerman, 2012; Berger, 2013; Reese, 2014; Marzouk, 2022). Other studies went beyond the basic site assessment to cover necessary design elements such as compliance with building/planning codes, suitable building structure, structural loads, and resources’ use, that is, water and power inputs needed for the farm operation (Caputo, Iglesias, and Rumble 2017; Gorgolewski and Straka 2017; Table 1).
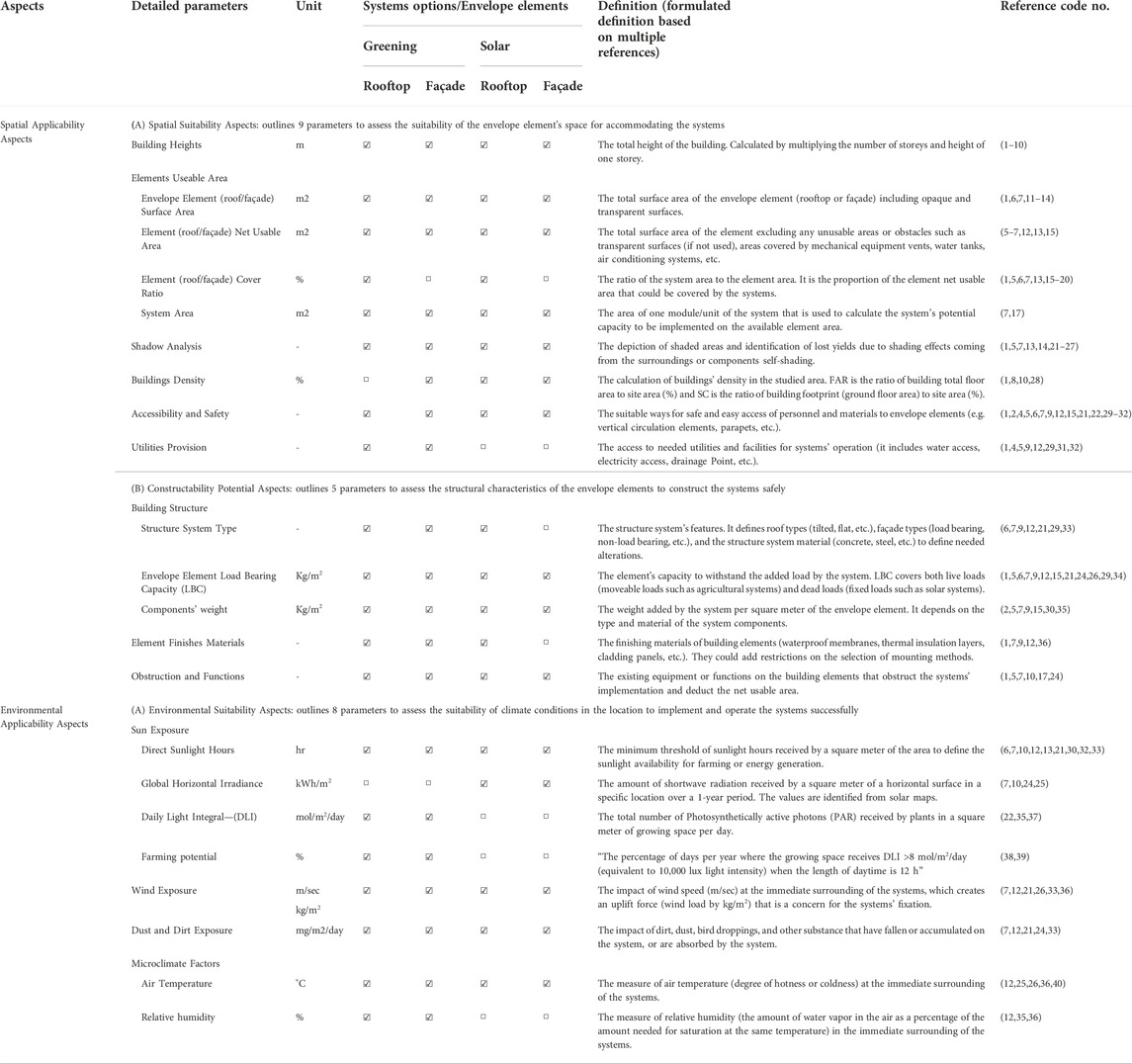
TABLE 1. Pillar 1 of applicability aspects for PBSs Implementation mapped against systems’ options (greening or solar) on different envelope elements (rooftop or façade). Detailed aspects and parameters were defined for each category, the measurement units, and the definitions are outlined as well. FAR, Floor Area Ratio; SC, Site Coverage; LBC, Load Bearing Capacity; DLI, Daily Light Integral; PAR, Photosynthetically Active Photons. List of References corresponding to the reference code numbers is in Supplementary Table S4.
Site assessment aspects for façade greening systems were defined to cover the climatic factors (such as wind, solar radiation, temperature, etc.), façade available area, finishing materials, façade access, structural factors, building load bearing capacity, and irrigation/drainage existence (DEPI, 2014). Other requirements include the type of vegetation, sunlight/shading levels, maintenance requirements, and services provision (power and water; GWG, 2013). In a further analysis, the environmental benefits and performance aspects of the façade systems were extensively outlined (Perini et al., 2011; GWG, 2013; Manso, 2021; Table 2). Improvement of air quality by reducing fine dust particulates, reduction of urban heat island effect by radiation absorption and evapotranspiration, and reduction of air temperature were also discussed.
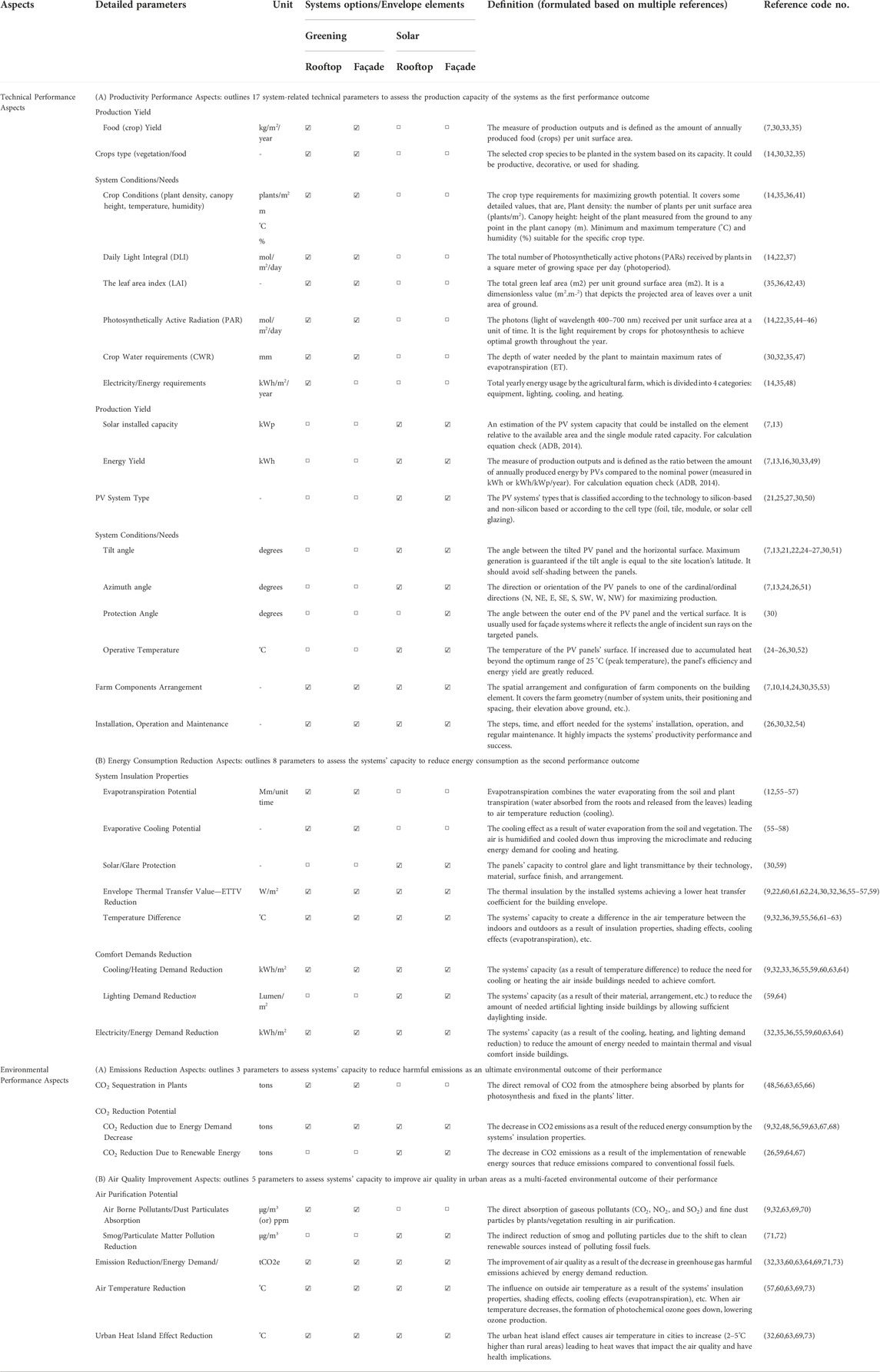
TABLE 2. Pillar 2 of performance aspects for PBSs Implementation mapped against systems’ options (greening or solar) on different envelope elements (rooftop or façade). Detailed aspects and parameters were defined for each category, the measurement units, and the definitions are outlined as well. DLI, Daily Light Integral; LAI, Leaf Area Index; PAR, Photosynthetically Active Radiation; CWR, Crop Water Requirements; PVs, Photovoltaic panels; ETTV, Envelope Thermal Transfer Value.
For implementing rooftop solar energy systems, site assessments should identify the rooftop configuration, structure and materials, rooftop accessibility, shading, and electrical loads (Schallenberg-Rodríguez, 2013; ADB, 2014; Gagnon, 2016; Table 1). It should also identify the solar resource sufficiency, the installed capacity potential, as well as the generated energy (produce yield; ADB, 2014; Saber et al., 2014). Methodologies for estimating the suitable rooftop area for solar energy systems were explained thoroughly in multiple studies and can be summarized as (a) identifying the shaded areas and sunlight hours, (b) defining the tilt and azimuth angles, (c) applying the minimum area threshold to define the rooftops of reasonable installed capacity, and (d) estimating the system productivity performance for the eligible rooftops (Melius et al., 2013; Schallenberg-Rodríguez, 2013; Gagnon, 2016).
For façade solar systems, spatial configuration and buildings’ density are crucial aspects that impact the implementation potential (Lee et al., 2016). Characteristics such as building typology, site coverage, and building heights had to be coupled with building density to depict the true applicability (Lee et al., 2016). Other system-related key aspects include the panels’ tilt angle to maximize production, structural loads on cantilevered panels (if tilted), and self-shading between panels (Kim et al., 2019). Façade orientation, shading impact, panels’ thermal performance, panels’ inclination angle, and climatic conditions are stated as of high impact on performance as well (Saber et al., 2014; Kim et al., 2019).
3.2.2 Applicability and performance aspects framework
To create the implementation aspects framework, we compiled, filtered, classified, and re-grouped all previously outlined aspects. Based on that, we proposed two pillars for the framework (Figure 11): The applicability aspects that define the systems suitable for implementation on the building envelope in a specific location, and the performance aspects that define the systems to be selected based on their expected performance. To reach a successful implementation, the process goes through the pillars sequentially, starting with the selection of eligible buildings or site locations, followed by the selection of the most suitable and promising systems.
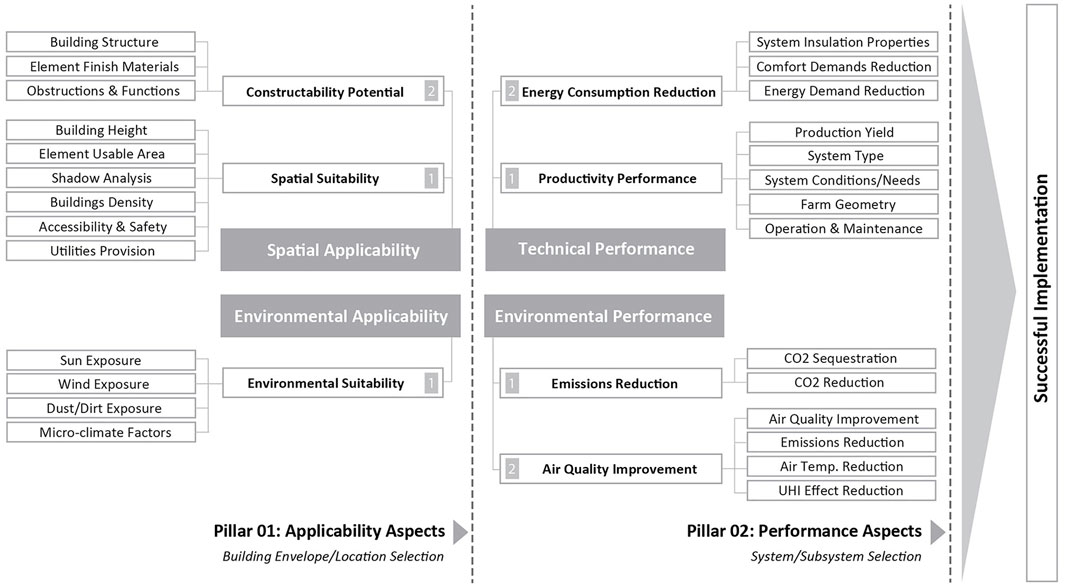
FIGURE 11. A comprehensive framework of implementation aspects compiled from assessed studies and reports and grouped into two pillars: applicability aspects that define the suitability of systems’ implementation on a specific building envelope and location, and performance aspects that guide the selection of the most promising systems for implementation. For each category, subcategories were formulated and the detailed parameters outlined. The framework core is structured based on several studies (Perini et al., 2011; Ackerman, 2012; ADB, 2014; DEPI, 2014; Attoye et al., 2017; Caputo et al., 2017; Besir and Cuce, 2018; U.S. Environmental Protection Agency, 2018; Kim et al., 2019) with additions from many supporting studies and reports (see Supplementary Table S4).
The first pillar, that is, the applicability aspects, is grouped into two main categories (a) spatial applicability aspects that describe specific spatial characteristics of the targeted building envelope elements (rooftop or façade) to be thoroughly assessed for accommodating the studied systems (Figure 11; Table 1). The second category is (b) environmental applicability aspects, which define location-specific climatic factors to be assessed to guarantee the systems’ productivity. Assessment of the surrounding environment and its suitability for hosting the systems is as crucial as the spatial assessments.
The second pillar, that is, the performance aspects, is classified into (a) technical performance aspects, which are system-related technical aspects that allow measuring the systems’ performance outcomes (Figure 11; Table 2). Performance does not stop at the technical level of production and consumption reduction but extends to a wider scale of environmental outcomes. Therefore, the second category (b) environmental performance aspects are concerned with aspects that allow quantifying the systems’ environmental gains. This is not meant as an exhaustive list of the system benefits but rather a list of the key quantifiable parameters that define two key environmental outcomes of the systems’ implementation (Figure 11).
Tracking the two systems across the first pillar of applicability aspects (Table 1) revealed that for the spatial suitability aspects, the majority of detailed parameters (6 out of 9) were identified to apply to all options (rooftop/façade greening systems and rooftop/façade solar energy systems) but at varying thresholds. The remaining dissimilar parameters are the element cover ratio (unidentified for façade systems), the building density (unapplied to rooftop greening), and the utilities provision (undiscussed for solar systems). Some of these parameters could be considered applicable to the missing options by similarity, however; literature validation is still missing. For the constructability potential aspects, all five parameters apply to both systems, yet the structure system type and element finishing material were not tackled for both façade systems. However, the façade’s structural suitability for systems’ mounting is a crucial aspect overlooked by the assessed studies. Half (4 out of 8) of the environmental suitability parameters apply to all options, the rest are system-specific climate factors needed for productivity. For instance, greening systems require assessing the daily light integral and farming potential values that impact the plants’ photosynthesis while solar energy systems need an assessment of global horizontal irradiance, which is a main productivity determinant.
More differences between the two systems were determined in the second pillar of performance aspects that is more system-specific (Table 2). Productivity parameters follow the same logic for both systems (production yield parameters, system types, and system conditions/needs) but are different in the detailed parameters. Only two parameters (2 out of 17) apply to all systems, which are the farm components arrangement and the installation, operation, and maintenance requirements. For the energy consumption reduction parameters, both systems have common aspects (4 out of 8) where both reduce heat flow through the envelope elements (Envelope Thermal Transfer Value ETTV), make a difference in the indoor temperature, reduce the cooling/heating demand, and eventually reduce the electricity demand. Yet, some system-related aspects include the evapotranspiration and evaporative cooling potentials of greening systems and the solar/glare protection and lighting demand reduction by solar systems. Similarly, the environmental performance aspects have a balance between the common and different parameters (4 versus 4). Mutual impacts achieved by both systems include the CO2 and emissions reduction due to energy demand reduction as well as air temperature reduction and urban heat island effect alleviation.
4 Discussion
Ongoing global and local urbanization trends lead to multiple adverse impacts such as the extensive resource consumption by buildings, the increase in greenhouse gas emissions, and the anthropization of agricultural landscapes. Functionalizing underutilized building envelopes by adding productive functions of PBSs is one way forward to compensate for the consuming and polluting patterns of growing cities. Analyzing and comparing the status, benefits, challenges, and requirements of the different PBSs (BIVs, BIAs, BAPVs, and BIPVs) was not conducted in that sense before, especially in the Egyptian context, as we provide here. Accordingly, this study identified the need for a comprehensive understanding of the systems in the arid climate and high urbanization rates of Egypt, to push their future implementation. The study’s practical analysis outcomes (contextual assessment) based on Egypt, could be extended to neighboring or developing counties of rapidly urbanizing contexts. They are foreseen to support PBSs implementation in countries of similar climatic, social, and economic conditions where the systems are still considered emerging markets. While the theoretical analysis outcomes (literature assessment) could be useful to different countries (international focus) as they reveal the current status of the systems’ technological advancement, global benefits and challenges, and general implementation requirements as discussed in literature.
4.1 Productive building systems (PBSs) between theory and practice
This research is an addition to the body of knowledge, given that previous relevant studies had limited focus. Some studies mapped the systems’ types by their hosting envelope element (on the rooftop and/or façade; DEPI, 2014; Raji et al., 2015; Tripathy et al., 2016; Singh et al., 2021), others by their production type (greening and/or solar energy; e.g. Benis et al., 2018; Tablada, 2018; Tablada et al., 2020), or just focused on only one category in detail (Stephen and James, 2001; Perini et al., 2011; Attoye et al., 2017; Rodríguez-Delfín et al., 2017). Therefore, this study created an updated comprehensive inventory based on the assessed studies and reports. It covers the identified types and classifications of the four PBSs (BIV, BIA, BAPV, and BIPV) that could be implemented on different envelope elements such as rooftops, façades, and balconies. The study further outlined the systems’ key benefits and challenges, and culminated the analysis with insights from the Egyptian local market that is expected to grow in the future. Creating the inventory as well as mapping the benefits and challenges provide a reply to the first two outlined research questions about PBSs in theory and the practice in Egypt.
4.1.1 Building-integrated vegetation and agriculture systems (BIVs and BIAs)
For rooftops, compared to available BIVs (extensive, semi-intensive, and intensive), BIAs have more options and are of varying complexity, reaching from low-tech and low-cost systems (e.g., soil-based containerized farming) to high-tech and high-cost systems (e.g., soil-less conditioned greenhouses). A potential reason is that BIAs serve multiple aims: a social purpose of engaging communities and ensuring food self-sufficiency (lower end) or a commercial purpose that requires efficiently maximizing production (higher end). For outdoor façade systems, BIVs were more discussed in the literature for their aesthetic and environmental gains. Their types (green façade and living wall systems) were extensively analyzed compared to BIA types that were slightly touched upon, mainly limited to the indoor vertical farm applications.
Comparing the assessment of studies to the Egyptian market insights from the interviews reveals that a wide range of BIVs and BIAs exist in the market with varying intensities. Locally, more focus is on rooftop systems where almost all their types identified in the literature exist in the Egyptian market (Figure 3). Several studies confirmed the popularity of rooftop systems in different regions (Raji et al., 2015; Benis et al., 2018), yet some façade systems were highlighted to exist in the local market only by few suppliers and limited small-scale applications (Figure 4). The reason could be the perceived complexity of installing such high-tech systems as well as their high costs. A body of literature supported this inference, especially for Living Wall Systems (LWS) and vertical farms (Perini et al., 2011; Raji et al., 2015; Benis et al., 2017b; Radić et al., 2019).
The survey findings that Nutrient Film Technique (NFT) system is achieving the highest sales (Figure 5) could be attributed to having the highest productivity and feasibility despite its high initial costs, an interpretation backed up by literature analyzing the system (Savvas, 2013; Rodríguez-Delfín et al., 2017). In contrast, the secondary preference of conventional planter boxes/pots and raised beds (soil-based systems) points to the inclination of a large number of customers in Egypt toward the DIY or low-cost, low-tech techniques for self-sufficiency purposes, as supported by Rodríguez-Delfín et al. (2017) for different contexts. This conforms with the discussion on the diffusion of simpler and lower-tech technologies in developing countries, for their reasonable costs, efficient use of resources, and reasonable productivity (Orsini et al., 2013; Eigenbrod and Gruda, 2015).
Balconies, though being traditional building elements of multifunctional use, were not extensively studied and are usually mentioned briefly in studies (Vazhacharickal, 2014; Raji et al., 2015; Besir and Cuce, 2018). Locally, balconies are identified to host greening systems as a cultural conduct by customers where implementation is facilitated by their high accessibility. Yet, residents still incline toward conventional solutions (such as horizontal planters on walls and/or railings, planter boxes and pots, etc.). The easy access to systems implemented in balconies, especially on railings/parapets, privileges them over the extending façade systems (Figure 4), yet they differ in the crop type selection, maintenance frequency, irrigation systems, etc.
Our literature assessment highlighted that enormous efforts were exerted in the greening systems’ studies to quantify the environmental benefits, slight efforts for the economic benefits, and minimal efforts for the social ones. This is in contrast with the survey results (Figure 6) that demonstrated that environmental benefits got less interest from the customers. In fact, customers’ prioritization of health-related and social benefits was clearly expressed by the top selections, which are, “enjoying the greenery view”, “producing healthier produce”, and “practicing a productive activity”. In addition, except for the financial benefit of “reducing the household’s food expenses”, the other economic benefits do not seem to be marketed well or exist on-ground. The interviewees also mentioned the customers’ increased awareness of the importance of consuming healthier food products to avoid prevailing health problems such as obesity and vitamin deficiency. In addition, the customers’ interest in enjoying greenery was highlighted, especially with the decrease in urban green spaces in Egypt. They also stressed that commercializing small-scale farms to gain revenue is of low feasibility. These claims could interpret the survey results and are supported by several studies (Gould and Caplow, 2012; GWG, 2013; DEPI, 2014; Gawad, 2018; Rosasco and Perini, 2019).
Economic challenges were recognized by the assessed studies as the most influential barriers facing greening systems. Similar to the literature, high initial costs were identified by the experts as the main challenge facing the local implementation progress, especially for lower-income groups. A body of literature mentions and analyzes this financial barrier (Benis et al., 2017b; Rosasco and Perini, 2019). To counterbalance the financial challenge, better knowledge is needed about the systems’ feasibility and the compensation of initial costs by long-term savings (e.g. food expenses reduction and electricity bill reduction due to lower energy demand). Financial incentives and payment facilitations by suppliers would also support the uptake until market competitiveness is increased.
4.1.2 Building-applied and building-integrated photovoltaic systems (BAPVs and BIPVs)
For rooftops, BAPVs have limited subsystems (standoff arrays, rack-mounted arrays) identified from the literature (Figure 7). In parallel, BIPVs offer multiple integration options within the building envelopes to achieve different design and aesthetic purposes, thus, a longer list of their types was identified from the literature (e.g. in roof systems and tiles). Similarly, BIPV façades provide diverse options for integration (e.g. solar cladding and solar glazing), yet there are hardly any BAPVs classifications. In general, when compared to the well-established BAPVs, BIPVs are considered to be emerging, thus studies focus more on introducing their types. Several studies supported this inference about the BAPVs advancement over BIPVs (Boemi et al., 2016; Shukla et al., 2017).
Despite the multiple types of rooftop BIPVs in the literature, the local market is still confined to BAPVs that are considered emerging, gaining popularity only recently as discussed by Kumar et al. (2019). For the façade systems, market penetration is much less for both BAPVs and BIPVs. This is proved by studies where usually the split is such that 80% of the market share is for rooftops versus 20% for facades (Shukla et al., 2017; Reddy et al., 2020). Locally, façade BAPVs and BIPVs have limited implementation trials (Figure 8). We conclude that this could be accounted for façade BAPVs and BIPVs being a niche market with high initial costs, low feasibility, and low productivity. Productivity is affected by the varying orientations, limited exposure to sunlight, and shading problems as explained by the interviewees and further depicted in past studies from other regions (Cheng et al., 2006; Gupta et al., 2011; Raji et al., 2015; Lee et al., 2016; Tablada and Shashwat, 2016).
On the technology level, Crystalline PV modules are the most widely spread in the local market being the most conventional option, with relatively lower costs, which is confirmed by literature (Cucchiella and Dadamo, 2012; Tripathy et al., 2016; Reddy et al., 2020). Solar thermal technology used in solar water heaters (SWH) is also well-established in the local market based on the interviews and supported by Dehghan et al. (2021).
In line with the assessed studies, the survey findings showed that Roof-mounted PVs (BAPVs) are sold the most by the respondents (Figure 9). Locally, the preference for BAPVs over BIPVs is due to the complexity of BIPVs implementation and maintenance as explained by Samir and Ali (2017). BAPVs are followed by solar thermals (SWHs) selected by 50% of respondents. The lower sales of SWHs has some discrepancy with the expected uptake in the local market where they have lower costs and longer market existence than PVs (since the 1980s; Dehghan et al., 2021). Currently, a financial mechanism is in place (net metering scheme) to support the adoption of PV systems but not SWHs, which could further lower their potential uptake, also backed up by the evaluation of SWHs status in Egypt reported by Dehghan et al. (2021).
Quantification of energy generation capacity (technical performance) is the main focus of the benefits analysis in most studies. Some include environmental gains and economic calculations (cost-benefit analyses, etc.). Yet, the social dimension is barely discussed, as are the positive health-related impacts. Unlike the literature focus on the role played by the solar energy systems to meet the continuously increasing electricity demand while reducing the reliance on polluting fossil fuels (Samir and Ali, 2017; Asfour, 2018; Ghosh, 2020; Singh et al., 2021), the survey responses revealed that economic benefits were the first priority for customers (Figure 10). “Reducing household expenses” was the ultimate selection by the respondents followed by “electricity production”, “hot water generation”, and “selling extra electricity to the grid”. Prioritizing technical and economic gains could be understood in light of the current electricity sector reforms and the gradual electricity subsidy removal taking place in Egypt (IRENA, 2018; EEHC, 2020). “Reducing emissions” was among the low ranks pointing to its lower priority for customers.
According to the survey, the “systems’ high initial costs” is a key obstacle facing local implementation (Figure 10). Even though globally, PV technology prices went down over the years (Baumann et al., 2019), locally, they are still considered high-cost products and need financial incentives. The claim of not being economically viable yet for Egypt is discussed by Samir and Ali (2017). The second identified barrier “fear of trying something new” is a common societal response to the introduction of new technologies, which can be faced by the systems’ marketing, demonstration projects, and awareness raising. To support the on-ground implementation, it is necessary to calculate the systems’ financial feasibility (long-term savings) and clearly communicate it to the customers. A monetary value needs to be added to the systems’ environmental and social benefits to calculate the true feasibility. In addition, a set of financial schemes is needed to support the market and compensate for the current high system costs.
4.2 Productive building systems (PBSs) implementation framework
Facilitating PBSs implementation on building envelopes requires a deep understanding of all aspects related to each system’s applicability and performance. Based on the assessed studies, in most cases, there is no categorization of the aspects or only a partial classification of them (Gorgolewski and Straka, 2017; Singh et al., 2021). Also, most studies cover one or two of the applicability and performance categories (Gagnon, 2016; Caputo et al., 2017), which impedes having a complete vision of all the systems’ needs and outcomes. Therefore, compiling scattered implementation aspects and grouping them into a comprehensive framework was considered of added value, especially for different stakeholders involved in implementation. In addition, covering both systems (greening and solar energy) in this investigation, as PBSs components, allows for identifying their commonalities and differences as well as the potential competition between them. By creating the framework (Figure 11), this study comprehensively replies to the third research question asking about the key aspects considered for PBSs implementation on building envelopes.
The majority of applicability aspects need to be assessed for both systems when considering a specific building envelope, but with varying thresholds. Aspects such as the envelope elements’ useable area, shadow analysis, accessibility and safety, building structure, sun exposure, and wind exposure are common spatial and environmental applicability parameters between greening and solar energy systems (Table 1). On the other hand, many performance aspects are different for each system, yet they follow the same logic and organization (Table 2). Food yield, crop type, Photosynthetically active radiation (PAR), evapotranspiration, and CO2 sequestration are technical and environmental performance parameters specific to greening systems. While, energy yield, tilt angle, operative temperature, glare protection, and CO2 reduction by renewable energy generation are technical and environmental performance parameters specific to solar energy systems.
In all, our study determines that despite the different production aims, the two systems share a lot of commonalities when it comes to the applicability aspects (spatial and environmental) with slightly varying thresholds. This leads to the inference that, in most cases, the space suitable for one system can be considered suitable for the other with some minor verifications needed. A similar argument was identified in the literature, when the rooftop is assumed to be suitable for solar systems, it was also considered suitable for accommodating agricultural systems (Berger, 2013; Reese, 2014). This backs up the main logic behind our study where the two systems are considered as potential components of PBS, which can be implemented side-by-side on the same building envelope. It also opens the room for the next level of the systems’ potential integration on the same elements or surfaces. The systems share multiple environmental performance aspects. A fact that highlights the potential behind their combination as components of PBSs or their future integration. For instance, maximizing the emission reduction, air quality improvement, and temperature reduction that are achieved by both systems allow them to effect a sensed change in the surrounding urban areas leading to multiplied positive impacts on the building and urban scales.
5 Conclusion
The continuously expanding building sector poses real challenges to the environment and the everyday life of urban populations. Accordingly, functionalizing the traditional building envelopes can contribute to minimizing the buildings’ negative environmental impacts and high consumption rates. Productive Building Systems (PBSs) combining greening and solar energy interventions on building envelopes provide a promising solution for producing multiple resources by the building sector. In the study at hand, we analyzed four PBSs with the main motive of supporting their successful implementation in the Egyptian building sector.
The first section highlighted that hydroponic systems (selected by 75% of the greening systems’ survey respondents), planter boxes (50%), raised beds (42%), PV panels (selected by 95% of the solar systems’ survey respondents), and solar water heaters (55%) are the most purchased PBSs in the Egyptian market. Local customers’ reasons for implementing the systems differed in nature where enjoying greenery (social benefit) and reducing energy expenses (economic benefit) were identified as the main aims for greening and solar systems by 95% and 100% of the respondents, respectively. While the high initial cost (economic barrier) was considered the main challenge for both systems (80% for the greening and 94% for the solar energy systems). The second section pointed to several commonalities between the two systems regarding the applicability and performance aspects as outlined in the implementation framework.
Building on the added value of comparing the theoretical and practical statuses of the two systems (greening and solar energy) regarding the three topics of the study—types and classifications, benefits and challenges, and applicability and performance aspects of implementation – we shed light on the importance of investigating their integration potential into PBS packages. The identified commonalities regarding the two systems’ applicability on building envelopes facilitate their integration on the same space. In addition, the common performance benefits could maximize the production and environmental gains when integrating the systems, and thus achieving maximum exploitation of the underutilized resource of building envelopes. Analysis and optimization of the systems’ integration potential and mutual benefits could be covered in future research with a special focus on the local context of the Egyptian building sector.
Data availability statement
The raw data supporting the conclusions of this article will be made available by the authors, without undue reservation.
Ethics statement
Ethical review and approval was not required for the study on human participants in accordance with the local legislation and institutional requirements. Written informed consent for participation was not required for this study in accordance with the national legislation and the institutional requirements.
Author contributions
MM, MS, and LF: substantial contributions to the study conception and design. MM: substantial contributions to the acquisition and interpretation of data. MM: drafting the work. MM, MS, and LF: revising the drafts critically. All authors contributed to the paper and approved the submitted version.
Acknowledgments
We would like to thank all local experts and suppliers who participated in the surveys and interviews and contributed with valuable inputs to the formulation of this study.
Conflict of interest
The authors declare that the research was conducted in the absence of any commercial or financial relationships that could be construed as a potential conflict of interest.
Publisher’s note
All claims expressed in this article are solely those of the authors and do not necessarily represent those of their affiliated organizations, or those of the publisher, the editors and the reviewers. Any product that may be evaluated in this article, or claim that may be made by its manufacturer, is not guaranteed or endorsed by the publisher.
Supplementary material
The Supplementary Material for this article can be found online at: https://www.frontiersin.org/articles/10.3389/fenvs.2022.1056382/full#supplementary-material
Footnotes
1UN DESA (2018) 68% of the world population projected to live in urban areas by 2050, says UN. Available at: https://www.un.org/development/desa/en/news/population/2018-revision-of-world-urbanization-prospects.html (Accessed: 26 September 2022).
2WFP (2021) Egypt|World Food Programme. Available at: https://www.wfp.org/countries/egypt (Accessed: 1 December 2021).
3IQAir (2021) Egypt Air Quality Index (AQI) and Air Pollution information. Available at: https://www.iqair.com/egypt (Accessed: 2 June 2022).
References
Abbasi, H. A. (2017). Productive landscape. Amsterdam, Netherlands: Elsevier Ltd. 37, 131–140. doi:10.1016/j.proenv.2017.03.029
Abdallah, L. (2020). Egypt’s nationally determined contributions to paris agreement: Review and recommendations. Int. J. Industry Sustain. Dev. 1 (1), 49–59. doi:10.21608/ijisd.2020.73503
Abdel Raheem, A., Mohamed, U., and Abdel Hafez, O. (2020). Analyzing affordable housing programs and projects in Egypt in the light of the positives of global experiences. J. Mod. Res. 0, 0. doi:10.21608/jmr.2020.23593.1020
Abdel-Rahman, W. S. M. (2021). Thermal performance optimization of parametric building envelope based on bio-mimetic inspiration. Ain Shams Eng. J. 12 (1), 1133–1142. doi:10.1016/j.asej.2020.07.007
AbdelAzim, A. I., Ibrahim, A. M., and Aboul-Zahab, E. M. (2017). Development of an energy efficiency rating system for existing buildings using Analytic Hierarchy Process – the case of Egypt. Renew. Sustain. Energy Rev. 71 (2016), 414–425. doi:10.1016/j.rser.2016.12.071
Abdollah, M. A. F., Scoccia, R., Filippini, G., and Motta, M. (2021). Cooling energy use reduction in residential buildings in Egypt accounting for global warming effects. Climate 9 (3), 45. doi:10.3390/cli9030045
Ackerman, K. (2012). The potential for urban agriculture in New York City: Growing capacity, food security, and green infrastructure. Available at: http://www.urbandesignlab.columbia.edu/sitefiles/file/urban_agriculture_nyc.pdf.
ADB (2014). Handbook for rooftop solar development in asia. Philippines. Available at: www.adb.org.
Al-Ayouty, I., Hassaballa, H., and El Hini, M. (2021). Carbon dioxide emissions: A dynamic panel analysis of energy intensive industries in Egypt. Eur. J. Sustain. Dev. 10 (3), 109. doi:10.14207/ejsd.2021.v10n3p109
Almosni, S., Delamarre, A., Jehl, Z., Suchet, D., Cojocaru, L., Giteau, M., et al. (2018). Material challenges for solar cells in the twenty-first century: Directions in emerging technologies. Sci. Technol. Adv. Mater. 19 (1), 336–369. doi:10.1080/14686996.2018.1433439
Aly, D., and Dimitrijevic, B. (2022). Public green space quantity and distribution in Cairo, Egypt. J. Eng. Appl. Sci. 69 (1), 15–23. doi:10.1186/s44147-021-00067-z
Anduła, A., and Heim, D. (2020). Photovoltaic systems - types of installations, materials, monitoring and modeling - review. Acta Innovations [Preprint].
Asfour, O. S. (2018). Solar and shading potential of different configurations of building integrated photovoltaics used as shading devices considering hot climatic conditions. Sustain. Switz. 10 (12). doi:10.3390/su10124373
Astee, L. Y., and Kishnani, N. T. (2008). Building integrated agriculture: Utilising rooftops for sustainable food crop cultivation in Singapore. J. Green Build. 5 (2), 105–113. doi:10.3992/jgb.5.2.105
Attia, S., and Amer, A. (2009). “Green roofs in cairo: A holistic approach for healthy productive cities,” in Proceedings of 7th annual greening rooftops for sustainable communities, 1–11.
Attia, S., Shafik, Z., and Ibrahim, A. (2018) New cities and community extensions in Egypt and the Middle East: Visions and challenges, new cities and community extensions in Egypt and the Middle East: Visions and challenges. doi:10.1007/978-3-319-77875-4
Attoye, D. E., Aoul, K. A. T., and Hassan, A. (2017). A review on building integrated photovoltaic façade customization potentials. Sustain. Switz. 9 (12), 2287. doi:10.3390/su9122287
Babu, C., and Ponnambalam, P. (2017). The role of thermoelectric generators in the hybrid PV/T systems: A review. Energy Convers. Manag. 151, 368–385. doi:10.1016/j.enconman.2017.08.060
Baumann, T., Nussbaumer, H., Klenk, M., Dreisiebner, A., Carigiet, F., and Baumgartner, F. (2019). Photovoltaic systems with vertically mounted bifacial PV modules in combination with green roofs. Sol. Energy 190, 139–146. doi:10.1016/j.solener.2019.08.014
Benis, K., Reinhart, C., and Ferrão, P. (2017a). Building-integrated agriculture ( BIA ) in urban contexts : Testing A simulation-based decision support workflow, 1798–1807. doi:10.26868/25222708.2017.47915th Int. Build. Perform. Simul. Assoc.
Benis, K., Reinhart, C., and Ferrão, P. (2017b). Development of a simulation-based decision support workflow for the implementation of Building-Integrated Agriculture (BIA) in urban contexts. J. Clean. Prod. 147, 589–602. doi:10.1016/j.jclepro.2017.01.130
Benis, K., Turan, I., Reinhart, C., and Ferrao, P. (2018). Putting rooftops to use – a Cost-Benefit Analysis of food production vs. energy generation under Mediterranean climates. Cities 78, 166–179. doi:10.1016/j.cities.2018.02.011
Berger, D. (2013). A GIS suitability analysis of the potential for rooftop agriculture in New York city. Master’s thesis. Columbia University. doi:10.7916/D85T3SN1
Besir, A. B., and Cuce, E. (2018). Green roofs and facades: A comprehensive review. Renew. Sustain. Energy Rev. 82 (2017), 915–939. doi:10.1016/j.rser.2017.09.106
Birkeland, J. (2009). “Eco-retrofitting with building integrated living systems,” in Proceedings of : The 3rd CIB international conference on smart and sustainable built environment : SASBE09 (Delft, Netherlands: Aula Congress Centre), 1–9.
Birkved, M., Hauschild, M., and Fernandez, J. (2016). Testing the environmental performance of urban agriculture as a food supply in northern climates. J. Clean. Prod. 135, 984–994. doi:10.1016/j.jclepro.2016.07.004
Biyik, E., Araz, M., Hepbasli, A., Shahrestani, M., Yao, R., Shao, L., et al. (2017). A key review of building integrated photovoltaic (BIPV) systems. Eng. Sci. Technol. Int. J. 20 (3), 833–858. doi:10.1016/j.jestch.2017.01.009
Boemi, S.-N., Irulegi, O., and Santamouris, M. (2016). Energy performance of buildings: Energy efficiency and built environment in temperate climates, energy performance buildings. Springer. doi:10.1201/9781351071697
Caplow, T., and Nelkin, J. (2007). 2nd PALENC conference and 28th AIVC conference on building low energy cooling and advanced ventilation technologies in the 21st century. Greece.Building-integrated greenhouse systems for low energy cooling Building-integrated greenhouse systems for low energy cooling
Capmas, (2021). Statistical yearbook 2021 - Egypt in figures. Available at: https://www.capmas.gov.eg/Pages/StaticPages.aspx?page_id=5035 (Accessed: December 1, 2021).
Capmas, (2016). Sustainable development strategy (SDS): Egypt vision 2030. Available at: https://arabdevelopmentportal.com/sites/default/files/publication/sds_egypt_vision_2030.pdf.
Caponigro, M., Manoloudis, A., and Papadopoulos, A. M. (2020). “Developing a strategy for energy efficiency in the Egyptian building sector Developing a strategy for energy efficiency in the Egyptian building sector,” in IOP conference series: Earth and environmental science. doi:10.1088/1755-1315/410/1/012076
Caputo, S., Iglesias, P., and Rumble, H. (2017). “Elements of rooftop agriculture design,” in Rooftop urban agriculture, urban agriculture. Editor F. Orsini (Springer International Publishing AG 2017), 39–59. doi:10.1007/978-3-319-57720-3_4
Cheng, V., Steemers, K., Montavon, M., and Compagnon, R. (2006). “Urban form, density and solar potential,”. Preprint in Plea 2006 - 23rd international conference on passive and low energy architecture (Geneva, Switzerland: Conference Proceedings).
Cho, E. I. E. I., Aung, T. I. N. Z. A. R., and Aung, T. (2019). Stand alone solar PV system with battery backup system for gonpinaing village , mandalay division. IRE Journals 3 (2), 225–231. Available at: https://irejournals.com/formatedpaper/1701501.pdf.
Clements-Croome, D. (2006). Creating the productive workplace. Taylor & Francis. Available at: https://books.google.com/books/about/Creating_the_Productive_Workplace.html?id=J5R5AgAAQBAJ (Accessed July 7, 2022).
Clements-Croome, D. (2015). Creative and productive workplaces: A review. Intell. Build. Int. 7 (4), 164–183. doi:10.1080/17508975.2015.1019698
Corcelli, F., Fiorentino, G., Petit-Boix, A., Rieradevall, J., and Gabarrell, X. (2019). Transforming rooftops into productive urban spaces in the Mediterranean. An LCA comparison of agri-urban production and photovoltaic energy generation. Resour. Conservation Recycl. 144, 321–336. doi:10.1016/j.resconrec.2019.01.040
Corti, P., Capannolo, L., Bonomo, P., De Berardinis, P., and Frontini, F. (2020). Comparative analysis of BIPV solutions to define energy and cost-effectiveness in a case study. Energies 13 (15). doi:10.3390/en13153827
Cucchiella, F., and Dadamo, I. (2012). Estimation of the energetic and environmental impacts of a roof-mounted building-integrated photovoltaic systems. Renew. Sustain. Energy Rev. 16 (7), 5245–5259. doi:10.1016/j.rser.2012.04.034
Dehghan, M., Pfeiffer, C. F., Rakhshani, E., and Bakhshi-Jafarabadi, R. (2021). A review on techno-economic assessment of solar water heating systems in the Middle East. Energies 14 (16), 4944. doi:10.3390/en14164944
DEPI (2014). in National library of Australia cataloguing-in-publication data. Available at: https://202020vision.com.au/media/41918/growing_green_guide_ebook_130214.pdf.A Guide to green roofs, walls and facades. State of victoria.
Despommier, D. (2013). Farming up the city: The rise of urban vertical farms. Trends Biotechnol. 31 (7), 388–389. doi:10.1016/j.tibtech.2013.03.008
Diwania, S., Agrawal, S., Siddiqui, A. S., and Singh, S. (2020). Photovoltaic–thermal (PV/T) technology: A comprehensive review on applications and its advancement. Int. J. Energy Environ. Eng. 11 (1), 33–54. doi:10.1007/s40095-019-00327-y
EEHC (2020). Annual report of the Egyptian electricity holding company2019/2020. Egyptian Electricity Holding CompanyAvailable at: http://www.eehc.gov.eg/.
Eigenbrod, C., and Gruda, N. (2015). Urban vegetable for food security in cities. A review. Agron. Sustain. Dev. 35 (2), 483–498. doi:10.1007/s13593-014-0273-y
El-Hattab, M., Amany, S. M., and Lamia, G. E. (2018). Monitoring and assessment of urban heat islands over the Southern region of Cairo Governorate, Egypt. Egypt. J. Remote Sens. Space Sci. 21 (3), 311–323. doi:10.1016/j.ejrs.2017.08.008
El-Kenawy, A. M., Hereher, M., Robaa, S. M., McCabe, M. F., Lopez-Moreno, J. I., Dominguez-Castro, F., et al. (2020). Nocturnal surface urban heat island over greater cairo: Spatial morphology, temporal trends and links to land-atmosphere influences. Remote Sens. 12 (23). doi:10.3390/rs12233889
El-Megharbel, N. (2015)., 27. New York)-May, 2015.Sustainable development strategy: Egypt’s vision 2030 and planning reformMinist. Plan. Monit. Adm. Reform, Integr. Approaches Sustain. Dev. Plan. Implement.
Elder, K. E. (2007). “Building envelope,” in Energy management handbook. Sixth. Editors W. C. Turner, and S. Doty (Lilburn, GA, United States: The Fairmont Press, Inc.), 236–262.
Elsayed, M. S. (2016). Optimizing thermal performance of building-integrated photovoltaics for upgrading informal urbanization. Energy Build. 116, 232–248. doi:10.1016/j.enbuild.2016.01.004
Gagnon, P., Margolis, R., Melius, J., Phillips, C., and Elmore, R. (2016). Rooftop solar photovoltaic technical potential in the United States: A detailed assessment, nrel technical report. Available at: http://www.nrel.gov/docs/fy16osti/65298.pdf.
Gawad, I. O. (2018). “The use of living walls in Egyptian residential buildings : Identifying the perceptions and challenges,” in AMPS Proceedings Series 11. Health: The Design, Planning and Politics of How and Where We Live (Bristol, United Kingdom: University of the West of England), 99–110.
George, P., and George, N. (2016). Hydroponics-(Soilless cultivation of plants) for biodiversity conservation. Int. J. Mod. Trends Eng. Sci. 03 (06), 97–104.
Ghanbari, S., Jafari, F., Bagheri, N., Neamtolahi, S., and Shayanpour, R. (2015). Study of the effect of using purposeful activity (gardening) on depression of female resident in golestan dormitory of ahvaz jundishapur university of medical sciences. J. Rehabilitation Sci. Res. 2 (1), 8–11. Available at: https://jrsr.sums.ac.ir/article_41066.html.
Ghosh, A. (2020). Potential of building integrated and attached/applied photovoltaic (BIPV/BAPV) for adaptive less energy-hungry building’s skin: A comprehensive review. J. Clean. Prod. 276, 123343. doi:10.1016/j.jclepro.2020.123343
Glaeser, E. L., and Kahn, M. E. (2004). “Sprawl and urban growth,” in Handbook of regional and urban economics. Editors J. V. Henderson, and J. F. Thisse (Elsevier), 2481–2527. doi:10.1016/S1574-0080(04)80013-0
Gokarakonda, S., Shrestha, S., Caleb, P. R., Rathi, V., Jain, R., Thomas, S., et al. (2019). Decoupling in India’s building construction sector: Trends, technologies and policies. Build. Res. Inf. 47 (1), 91–107. doi:10.1080/09613218.2018.1490054
Goldstein, B., Hauschild, M., Fernandez, J., and Birkved, M. (2016). Urban versus conventional agriculture, taxonomy of resource profiles: A review. Agron. Sustain. Dev. 36 (1), 9–19. doi:10.1007/s13593-015-0348-4
Gorgolewski, M., and Straka, V. (2017). “Integrating rooftop agriculture into urban infrastructure,” in Rooftop urban agriculture, urban agriculture. (Springer International Publishing AG), 113–126. doi:10.1007/978-3-319-57720-3_8
Gould, D., and Caplow, T. (2012). Building-integrated agriculture: A new approach to food production. Sawston, Cambridge, United Kingdom: Woodhead Publishing, 147–170. doi:10.1533/9780857096463.2.147
Gould, S. (2019). Internal design of a hydroponics greenhouse for tri cycle farms. Available at: https://scholarworks.uark.edu/baeguht/61.
Gupta, A., Hall, M. R., Hopfe, C. J., and Rezgui, Y. (2011). Building integrated vegetation as an energy conservation measure applied to non-domestic building typology in the UK. In Proceedings of building simulation 2011: 12th conference of international building performance simulation association, 949–956.
GWG (2013). UK guide to green walls an introductory guide to designing and constructing green walls in the UK.
Harris, R. (2019). Defining and measuring the productive office. J. Corp. Real Estate 21 (1), 55–71. doi:10.1108/JCRE-05-2018-0016
Hassanein, M. K., Fahim, M. A., Khalil, A. A., Abolmaty, S. M., Refaie, K. M., Taqi, M. O., et al. (2014). Impact of urbanization on agricultural land losses and climate change Case study: Gharbiah Governorate, Egypt. J. Am. Sci. 10 (1), 99–107.
International Energy Agency IEA (2015). Building energy performance metrics-supporting energy efficiency progress in major economies. Paris, France: Energy Efficiency, 1–110.
Jenkins, A., Keeffe, G., and Hall, N. (2015). “Facade farm: Solar mediation through food production,” in EuroSun 2014 conference proceedings, 1–10. doi:10.18086/eurosun.2014.18.02
Johnson, M. P. (2001). Environmental impacts of urban sprawl: A survey of the literature and proposed research agenda. Environ. Plan. A 33 (4), 717–735. doi:10.1068/a3327
Kahn, M. E. (2009). Urban growth and climate change. Annu. Rev. Resour. Econ. 1 (1), 333–350. doi:10.1146/annurev.resource.050708.144249
Kaplanis, S., Kaplani, E., and Kaldellis, J. K. (2022). PV temperature and performance prediction in free-standing, BIPV and BAPV incorporating the effect of temperature and inclination on the heat transfer coefficients and the impact of wind, efficiency and ageing, 181. Amsterdam, Netherlands: Elsevier Ltd., 235–249. doi:10.1016/j.renene.2021.08.124
Kim, A. A., Reed, D. A., Choe, Y., Wang, S., and Recart, C. (2019). New building cladding system using independent Tilted BIPV panels with battery storage capability. Sustain. Switz. 11 (20), 5546. doi:10.3390/su11205546
Kortright, R. (2001). Evaluating the potential of green roof agriculture: A demonstration project. Master’s thesis, Trent University.
Kosorić, V., Huang, H., Tablada, A., Lau, S. K., and Tan, H. T. (2019). Survey on the social acceptance of the productive façade concept integrating photovoltaic and farming systems in high-rise public housing blocks in Singapore. Renew. Sustain. Energy Rev. 111, 197–214. doi:10.1016/j.rser.2019.04.056
Kosorić, V., Lau, S. K., Tablada, A., and Lau, S. S. Y. (2018). General model of Photovoltaic (PV) integration into existing public high-rise residential buildings in Singapore – challenges and benefits. Renew. Sustain. Energy Rev. 91, 70–89. doi:10.1016/j.rser.2018.03.087
Kowarik, I., and von der Lippe, M. (2018). Plant population success across urban ecosystems: A framework to inform biodiversity conservation in cities. J. Appl. Ecol. 55 (5), 2354–2361. doi:10.1111/1365-2664.13144
Krawczak, E. (2020). BAPV system modeling for the single-family house: A case study. Inf. Autom. Pomiary w Gospod. i Ochr. Środowiska 10 (3), 44–47. doi:10.35784/iapgos.2106
Kumar, N. M., Sudhakar, K., and Samykano, M. (2019). Performance comparison of BAPV and BIPV systems with c-Si, CIS and CdTe photovoltaic technologies under tropical weather conditions. Case Stud. Therm. Eng. 13 (2018), 100374. doi:10.1016/j.csite.2018.100374
Lab, L. (2011). Building integrated agriculture: A qualitative comparative analysis of methods for commercial food production using buildings as an agricultural settlement. Sheffield, United Kingdom: University of Sheffield. MA2. Available at: https://issuu.com/libalab/docs/building_integrated_agriculture/1 (Accessed: July 16, 2022).
Ledesma, G., Nikolic, J., and Pons-Valladares, O. (2020). Bottom-up model for the sustainability assessment of rooftop-farming technologies potential in schools in Quito, Ecuador. J. Clean. Prod. 274, 122993. doi:10.1016/j.jclepro.2020.122993
Lee, D.-K. (2014). Building integrated vegetation systems into the new sainsbury’s building based on BIM. J. KIBIM 4 (2), 25–32. doi:10.13161/kibim.2014.4.2.025
Lee, K. S., Lee, J. W., and Lee, J. S. (2016). Feasibility study on the relation between housing density and solar accessibility and potential uses. Renew. Energy 85, 749–758. doi:10.1016/j.renene.2015.06.070
Lundholm, J. T., and Richardson, P. J. (2010). MINI-REVIEW: Habitat analogues for reconciliation ecology in urban and industrial environments. J. Appl. Ecol. 47, 966–975. doi:10.1111/j.1365-2664.2010.01857.x
Manso, M., Teotónio, I., Silva, C. M., and Cruz, C. O. (2021). Green roof and green wall benefits and costs: A review of the quantitative evidence. Amsterdam, Netherlands: Elsevier Ltd., 135. doi:10.1016/j.rser.2020.110111
Mariotti, N., Bonomo, M., and Barolo, C. (2020). “Emerging photovoltaic technologies and EcoDesign—criticisms and potential improvements,” in Reliability and ecological aspects of photovoltaic modules (London: IntechOpen). doi:10.5772/intechopen.88327
Martín-Chivelet, N., Gutierrez, J., Alonso-Abella, M., Chenlo, F., and Cuenca, J. (2018). Building retrofit with photovoltaics: Construction and performance of a BIPV ventilated façade. Energies 11 (7), 1719. doi:10.3390/en11071719
Marzouk, M. A., Salheen, M. A., Stokman, A., and Faggal, A. A. (2022). “Applicability of PV rooftops versus agriculture rooftops in the residential buildings of nasr city, cairo,” in SUPTM 2022 conference proceedings sciforum-053134, 1–4. doi:10.31428/10317/10592
Marzouk, M. (2018). Harvesting crops versus solar energy on cairo’s residential rooftops – status-quo analysis. Trialog 2/2017, 32–42. Available at: shorturl.at/ADHQT.
Marzouk, M. (2016). Rooftops from wasted to scarce resource; the competition between harvesting crops and solar energy in nasr city , Cairo. Stuttgart, Germany and Cairo, Egypt: University of Stuttgart and Ain Shams University. Master’s thesis. Available at: shorturl.at/cjvy9.
Melius, J., Margolis, R., and Ong, S. (2013). Estimating rooftop suitability for PV : A review of methods , patents , and validation techniques, 35. NREL Technical Report, (December)Available at: www.nrel.gov/publications.
Nexus, P. R. I. M. A. (2021). Awesome - managing water, ecosystems and food across sectors and scales in the South Mediterranean.
Ogueke, N. V., Anyanwu, E. E., and Ekechukwu, O. V. (2009). A review of solar water heating systems. J. Renew. Sustain. Energy 1 (4), 043106. doi:10.1063/1.3167285
Orsini, F., Kahane, R., Nono-Womdim, R., and Gianquinto, G. (2013). Urban agriculture in the developing world: A review. Agron. Sustain. Dev. 33 (4), 695–720. doi:10.1007/s13593-013-0143-z
Othman, M. Y. H., Yatim, B., Sopian, K., and Abu Bakar, M. N. (2005). Performance analysis of a double-pass photovoltaic/thermal (PV/T) solar collector with CPC and fins. Renew. Energy 30 (13), 2005–2017. doi:10.1016/j.renene.2004.10.007
Perini, K., Ottele, M., Haas, E. M., and Raiteri, R. (2011). Greening the building envelope, facade greening and living wall systems. Open J. Ecol. 01 (01), 1–8. doi:10.4236/oje.2011.11001
Radić, M., Dodig, M. B., and Auer, T. (2019). Green facades and living walls-A review establishing the classification of construction types and mapping the benefits. Sustain. Switz. 11 (17). doi:10.3390/su11174579
Radwan, T. M., Blackburn, G. A., Whyatt, J. D., and Atkinson, P. M. (2019). Dramatic loss of agricultural land due to urban expansion threatens food security in the Nile Delta, Egypt. Remote Sens. 11 (3). doi:10.3390/rs11030332
Raji, B., Tenpierik, M. J., and Van Den Dobbelsteen, A. (2015). The impact of greening systems on building energy performance: A literature review. Renew. Sustain. Energy Rev. 45, 610–623. doi:10.1016/j.rser.2015.02.011
Reda, L. (2018). Egypt to construct 20 fourth-generation cities - EgyptToday. EgyptToday. Available at: https://www.egypttoday.com/Article/1/57378/Egypt-to-construct-20-fourth-generation-cities (Accessed: December 2, 2021).
Reddy, P., Gupta, M. V. N. S., Nundy, S., Karthick, A., and Ghosh, A. (2020). Status of BIPV and BAPV system for less energy-hungry building in India-a review. Appl. Sci. Switz. 10 (7). doi:10.3390/app10072337
Reese, N. M. (2014). An assessment of the potential for urban rooftop agriculture in west oakland, California. San Francisco, CA, United States: University of San Francisco. Master’s thesis. Available at: https://repository.usfca.edu/capstone/17.
Riad, P., Graefe, S., Hussein, H., and Buerkert, A. (2020). Landscape transformation processes in two large and two small cities in Egypt and Jordan over the last five decades using remote sensing data. Landsc. Urban Plan. 197, 103766. doi:10.1016/J.LANDURBPLAN.2020.103766
Rodríguez-Delfín, A., Gruda, N., Eigenbrod, C., Orsini, F., and Gianquinto, G. (2017). “Soil based and simplified hydroponics rooftop gardens,” in Rooftop urban agriculture, urban agriculture. (Springer International Publishing AG), 61–81. doi:10.1007/978-3-319-57720-3_5
Roehr, D., and Laurenz, J. (2008). “Green living envelopes for food and energy production in cities,” in The sustainable city V. WIT transactions on ecology and the environment. Editors A. Gospodini, C. A. Brebbia, and E. Tiezzi, 663–671. doi:10.2495/SC080621
Rosasco, P., and Perini, K. (2019). Selection of (green) roof systems: A sustainability-based multi-criteria analysis. Buildings 9 (5), 134. doi:10.3390/buildings9050134
Saber, E. M., Lee, S. E., Manthapuri, S., Yi, W., and Deb, C. (2014). PV (photovoltaics) performance evaluation and simulation-based energy yield prediction for tropical buildings. Energy 71, 588–595. doi:10.1016/j.energy.2014.04.115
Samir, H., and Ali, N. A. (2017). Applying building-integrated photovoltaics (BIPV) in existing buildings. Oppor. Constrains Egypt 37, 614–625. doi:10.1016/j.proenv.2017.03.048
Sattler, S., Zluwa, I., and Österreicher, D. (2020). The “PV rooftop garden”: Providing recreational green roofs and renewable energy as a multifunctional system within one surface area. Appl. Sci. Switz. 10 (5), 1791. doi:10.3390/app10051791
Savvas, D., Gianquinto, G., Tuzel, Y., and Gruda, N. (2013). “Soilless culture,” in Good Agricultural Practices for greenhouse vegetable crops, Principles for Mediterranean climate areas. Editor W. Baudoin (Rome: FAO), 137–148. Available at: https://www.researchgate.net/publication/259842465_Soilless_culture.
Saxena, N. N. (2021). The review on techniques of vertical farming. Int. J. Mod. Agric. 10 (1), 732–738.
Schallenberg-Rodríguez, J. (2013). Photovoltaic techno-economical potential on roofs in regions and islands: The case of the Canary Islands. Methodological review and methodology proposal. Renew. Sustain. Energy Rev. 20, 219–239. doi:10.1016/j.rser.2012.11.078
Shukla, A. K., Sudhakar, K., and Baredar, P. (2017). Recent advancement in BIPV product technologies: A review. Energy Build. 140, 188–195. doi:10.1016/j.enbuild.2017.02.015
Singh, D., Chaudhary, R., and Karthick, A. (2021). Review on the progress of building-applied/integrated photovoltaic system. Environ. Sci. Pollut. Res. 28 (35), 47689–47724. doi:10.1007/s11356-021-15349-5
Soga, M., Gaston, K. J., and Yamaura, Y. (2017). Gardening is beneficial for health: A meta-analysis. Prev. Med. Rep. 5, 92–99. doi:10.1016/j.pmedr.2016.11.007
Stephen, F., and James, P. (2001). “Discussion of strategies for mounting photovoltaic arrays on rooftops,” in Proceedings of solar forum 2001, solar energy: The power to choose (Washington, DC.
Tablada, A., Chaplin, I., Huajing, H., Kosoric, V., Lau, S. K., Chao, Y., et al. (2017). “Assessment of solar and farming systems integration into tropical building facades,” in Proceedings ISES solar world congress 2017 - IEA SHC international conference on solar heating and cooling for buildings and industry 2017, 655–665. doi:10.18086/swc.2017.12.11
Tablada, A., Chaplin, I., Huang, H., Lau, S. K., Yuan, C., and Lau, S. S. (2018). “Simulation algorithm for the integration of solar and farming systems on tropical façades,” in Caadria 2018 - 23rd international conference on computer-aided architectural design research in asia: Learning, prototyping and adapting, 2, 123–132.
Tablada, A., Kosoric, V., Lau, S. K., Yuan, C., and Lau, S. (2017). “Productive facade systems for energy and food harvesting: A prototype optimisation framework,” in Proceedings of 33rd PLEA international conference: Design to thrive, PLEA 2017, 3, 3595–3602.
Tablada, A., Kosoric, V., Huang, H., Lau, S. S., and Shabunko, V. (2020). Architectural quality of the productive façades integrating photovoltaic and vertical farming systems: Survey among experts in Singapore. Front. Archit. Res. 9 (2), 301–318. doi:10.1016/j.foar.2019.12.005
Tablada, A., and Shashwat, S. (2016). “Potential use of building facades for potential use of building facades for food and energy harvesting in Singapore,” in PLEA 2016 los angeles - 32th international conference on passive and low energy architecture. Cities, buildings, people: Towards regenerative environments.
Tablada, A., and Zhao, X. (2016). Sunlight availability and potential food and energy self-sufficiency in tropical generic residential districts. Sol. Energy 139, 757–769. doi:10.1016/j.solener.2016.10.041
Tan, H., Hao, X., Long, P., Xing, Q., Lin, Y., and Hu, J. (2020). Building envelope integrated green plants for energy saving. Energy Explor. Exploitation 38 (1), 222–234. doi:10.1177/0144598719875529
The Trade Council, (2014). Egypt: Water sector, embassy of Denmark in Cairo. Cairo. Available at: http://www.iberglobal.com/files/egipto_agua.pdf.
Tripathy, M., Sadhu, P. K., and Panda, S. K. (2016). A critical review on building integrated photovoltaic products and their applications. Renew. Sustain. Energy Rev. 61, 451–465. doi:10.1016/j.rser.2016.04.008
Tyagi, V. V., Kaushik, S. C., and Tyagi, S. K. (2012). Advancement in solar photovoltaic/thermal (PV/T) hybrid collector technology. Renew. Sustain. Energy Rev. 16 (3), 1383–1398. doi:10.1016/j.rser.2011.12.013
UNICEF Country Office (2015). Nutrition agenda for action: A policy paper on scaling up nutrition intervention in Egypt. Available at: https://www.unicef.org/egypt/media/296/file.
U.S. Environmental Protection Agency (2018). Estimating the environmental effects of green roofs A case study in Kansas city. Missouri. Available at: https://www.epa.gov/heat-islands/using-green-roofs-reduce-heat-islands.
Vazhacharickal, P. J. (2014). Balcony and terrace gardens in urban greening and local food production: Scenarios from Mumbai metropolitan region (mmr), India. Int. J. Food 4 (2), 149–162.
Viljoen, A., Bohn, K., and Howe, J. (2005). Continuous productive urban landscapes : Designing urban agriculture for sustainable cities. Architectural Press, an imprint of Elsevier. Available at: https://books.google.com.eg/books?id=2qwrBgAAQBAJ (Accessed July 7, 2022).
World Food Programme Wfp, (2013). The status of poverty and food security in Egypt: Analysis and policy recommendations, Preliminary Summary Report. Available at: https://documents.wfp.org/stellent/groups/public/documents/ena/wfp257467.pdf.
Wu, X., Liu, Y., Xu, J., Lei, W., Si, X., Du, W., et al. (2015). Monitoring the performance of the building attached photovoltaic ( BAPV ) system in Shanghai. Energy Build. 88, 174–182. doi:10.1016/j.enbuild.2014.11.073
Yang, T., and Athienitis, A. K. (2016). A review of research and developments of building-integrated photovoltaic/thermal (BIPV/T) systems. Renew. Sustain. Energy Rev. 66, 886–912. doi:10.1016/j.rser.2016.07.011
Keywords: building-applied photovoltaics, building-integrated agriculture, building-integrated photovoltaics, building-integrated vegetation, productive buildings envelopes, solar energy generation, sustainable architecture, urban green infrastructure
Citation: Marzouk MA, Salheen MA and Fischer LK (2022) Functionalizing building envelopes for greening and solar energy: Between theory and the practice in Egypt. Front. Environ. Sci. 10:1056382. doi: 10.3389/fenvs.2022.1056382
Received: 28 September 2022; Accepted: 14 November 2022;
Published: 05 December 2022.
Edited by:
Salvador García-Ayllón Veintimilla, Technical University of Cartagena, SpainReviewed by:
Siddhita Yadav, Indian Institute of Technology Roorkee, IndiaSuprava Chakraborty, Vellore Institute of Technology (VIT), India
Felicia Jefferson, Fort Valley State University, United States
Copyright © 2022 Marzouk, Salheen and Fischer. This is an open-access article distributed under the terms of the Creative Commons Attribution License (CC BY). The use, distribution or reproduction in other forums is permitted, provided the original author(s) and the copyright owner(s) are credited and that the original publication in this journal is cited, in accordance with accepted academic practice. No use, distribution or reproduction is permitted which does not comply with these terms.
*Correspondence: Mai A. Marzouk, mai-adel-fathy.marzouk@ilpoe.uni-stuttgart.de