- 1Beijing Key Laboratory for Source Control Technology of Water Pollution, College of Environmental Science and Engineering, Beijing Forestry University, Beijing, China
- 2School of Environment, Tsinghua University, Beijing, China
- 3School of Energy and Environmental Engineering, University of Science and Technology Beijing, Beijing, China
- 4Australian Centre for Water and Environmental Biotechnology, The University of Queensland, St Lucia, QLD, Australia
Urine source separation, a kind of new sewage management concept, has made great progress in technology development and application in the past 30 years. However, understanding of the potential microbial risks in reuse of urine-derived fertilizer products (UDFPs) in agriculture is still lacking. Outbreak of pandemic of Coronavirus Disease 2019 and more deadly disease caused by Monkeypox strongly sounds the alarm bell to the attention on pathogens in urine and their fate in UDFPs. Therefore, this study presented a comprehensive review on pathogens inactivation in nutrient recovery technologies. The review suggests that technologies using alkaline or heating treatment can effectively reduce pathogens in UDFPs. However, technologies with characteristics such as membrane rejection of nutrients or nutrient adsorption may even concentrate pathogens in their fertilizer products. Based on an overall assessment, connections of technologies and the pathogens inactivation in their UDFPs have been established. This would help to provide a perspective on development of urine treatment technology and management of microbial risks in reusing urine nutrients in agriculture.
1 Introduction
Urine source separation, a novel concept of wastewater management, was proposed at the end of the 19th century (Larsen and Gujer, 1996). Human urine contains abundant amounts of macro-nutrients, accounting for approximately 79% of nitrogen, 49% of phosphorus, and 58% of potassium in household wastewater (Vinnerås et al., 2006). Resource recycling from urine benefits closing the loop of nutrients. This would provide a sustainable approach to ease the tension between the increasing demand of food and fertilizer and the shortage of nutrients while preventing water pollution (Larsen et al., 2016; Badeti et al., 2022). Thus, it could be an effective tool to help eradicating hunger and ensuring environmental sustainability in the Millennium Development Goals (MDGs) of United Nations, and achieving zero hunger, clean water and sanitation, and sustainable cities and communities in the Sustainable Development Goals (SDGs).
Various technologies have been developed to reuse or recover nutrients from urine. This has been reviewed in several recent publications (Simha and Ganesapillai, 2017; Harder et al., 2019; Patel et al., 2020; Cheng et al., 2022; Liu et al., 2022). Moreover, dozens of pilot projects of urine source separation have been constructed and evaluated in schools, villages or modern buildings in both developed and developing countries (Zhang et al., 2015). Most of these technologies and projects mainly focus on the technical and economic efficiency of nutrient recycling while only a few studies reported the hygienic aspect of urine. Actually, urine is not sterile and it contains culture-positive microbiological communities (Hilt et al., 2014). Several bacterial and viral pathogens were detected in the source-separated urine collected at the eThekwini Water and Sanitation municipality in South Africa (Bischel et al., 2015). Users of toilet and urine-derived fertilizer product (UDFP) may be exposed to pathogens-carrying urine and UDFPs. Moreover, consumers also may be exposed to food fertilized with UDFPs. All these possible exposure pathways can bring health risks to people who may be infected through vehicleborne transmission defined as one of the indirect transmission modes (US Department of Health and Human Services, 2012).
Quantitative Microbial Risk Assessment (QMRA) has been used to assess the microbial risk in handling of urine and eating vegetables fertilized by urine (Hoglund et al., 2002; Ahmed et al., 2017; Bischel et al., 2019; Oishi et al., 2020). Typical pathogens examined included Campylobacter jejuni, Enterotoxigenic Escherichia coli (E. coli), Shigella spp., Cryptosporidium parvum and rotavirus, etc. All these studies reported health risks to users, workers and consumers when pathogens-containing urine was collected, transported and handled without any pretreatment. Storage for 2–6 months is usually recommended for effective inactivation of pathogens in urine, which is caused by high alkaline pH and high free ammonia due to urea hydrolysis (World Health Organization (WHO), 2006; Vinnerås et al., 2008). Moreover, pathogens in urine can also be effectively inactivated through alkaline treatment by ash and lime (Randall et al., 2016; Senecal et al., 2018). However, up to date, there still lacks a connection of urine treatment to its effect on pathogens inactivation, which might make researchers ignore the microbial risks of urine source separation especially developing new technologies for urine treatment. Particularly, the pandemic of Coronavirus Disease 2019 (https://www.who.int/emergencies/diseases/novel-coronavirus-2019) and the outbreak of more deadly disease caused by Monkeypox (https://www.who.int/emergencies/situations/monkeypox-oubreak-2022) strongly reminds the possible microbial risks in the recycling of urine nutrients in agriculture.
Therefore, this study aimed to review the inactivation of pathogens in urine treatment technologies. Pathogens in urine reported in publications were summarized. The inactivation of pathogens in various technologies and UDFPs were evaluated based on a comprehensive review of publications. Then, an overall assessment was carried out to build a connection of urine treatment to its effect on pathogens inactivation. Finally, an outlook was proposed for the microbial risk management in urine source separation and nutrient recovery.
2 Pathogens in urine
Only a few publications reported pathogens detected in urine collected from toilets while many reported findings in urine collected from patients in medical research. Bacteria and viruses detected in urine in literature have been briefly summarized in Supplementary Table S1. Therefore, urine is not sterile. Possible approaches for pathogens introducing to urine were discussed as following.
2.1 Pathogens excretion with urine
Human urine forms by filtration of blood plasma through glomerular filtration barrier into nephron in kidneys (Rose, 1966). The barrier commonly has nanoscale pores while sizes of bacteria are approximately of micron scale (Goswami and Pugazhenthi, 2020). For example, the length and the diameter of E. coli is approximately 2 µm and 0.5 µm, respectively (Sherbet and Lakshmi, 1973). Thus, urine often does not contain bacteria. However, urine will be contaminated by pathogenic bacteria when infection occurs in the urinary system of patients. Studies have reported several pathogenic bacteria in urine, which often cause some clinical urinary tract infection and inflammation, such as E. coli (Hinata et al., 2004), Proteus mirabilis, Pseudomonas aeruginosa (Qiao et al., 2013), a variety of gram-negative and gram-positive bacteria (Shigemura et al., 2005). Sun et al. (2020) identified more than 105/ml of bacterial particles in clinical urine samples through analysis by mass spectrometry combined with UF‒5000i flow cytometry, and the pathogens were all single microbial species (Sun et al., 2020). Chen et al. (2018) successfully detected E. coli, Proteus mirabilis, Pseudomonas aeruginosa and Staphylococcus aureus in urine samples, with the detection limit of 100 colony-forming unit (CFU)/mL, using multiplex recombinase polymerase chain reaction (Chen et al., 2018).
Infection of urinary system can also introduce viruses to urine similar as the introduction of bacteria. In addition, viruses usually range in size from 5 to 300 nm (Goldsmith and Miller, 2009) and most of them lies in the lower margin of the scale as summarized by (Goswami and Pugazhenthi, 2020). Thus, a part of viruses can pass through the glomerular filtration barrier in kidneys, thereby contaminating urine. For example, researchers reported that the diameter of SARS-CoV-2 ranged in 60–140 nm (Zhu et al., 2020). The virus detection rate in the serum and urine samples collected from 74 COVID-19 patients were 2.8% (9/323) and 0.8% (2/247), with mean viral load of 1210 ± 1861 and 79 ± 30 copies/mL, respectively (Kim et al., 2020). Kim et al. (2020) did not report urinary system infection by SARS-CoV-2. This indicates that virus in serum passed through the barrier to urine. While the viral load in urine was low and the SARS-COV-2 could not be isolated from the virus-positive samples (Kim et al., 2020), the microbiological risk of viral infection still cannot be ruled out. In addition, researchers have found many types of viruses in urine, such as Zika virus (Wiwanitkit, 2016), SARS virus (Xu et al., 2005), dengue virus (Van den Bossche et al., 2015), BK virus (Konietzny et al., 2012), Rift Valley fever and yellow fever virus (Li M. et al., 2019), etc. Therefore, urine would inevitably contain pathogens due to pathogen permeation from serum and infection of urinary system when patients use toilets.
2.2 Fecal contamination
Besides urinal, urine diverting toilet, which is divided into two parts through a partition, is employed to collect urine separately from feces. This toilet is quite different from a conventional toilet, which may make users puzzled especially when there is insufficient instruction. Incorrect use or cleaning of the toilet may cause the feces to enter the urine, which is a common unavoidable phenomenon (Schonning et al., 2002). Moreover, insect vectors (e.g., mosquitoes and flies) can also be carriers of microbes from feces to urine. The detection of intestinal microbes such as E. coli in source-separated urine would be an evidence for the cross contamination by feces (An et al., 2020). A study found that the corresponding level of fecal contamination of urine samples in eco-villages was 38% and at public places (i.e., work places and schools) 58% of the urine samples were contaminated when the contamination was determined based on the content of fecal sterols (Schonning et al., 2002). Therefore, cross contamination of urine by feces can be confirmed to be another route to introduce pathogens to urine. Feces contain more than 100 different varieties of viruses, bacteria, and helminthes (Senecal et al., 2018). However, only a few kinds of bacteria have been detected in source-separated urine because of the lack of related studies.
Urine contamination by feces would enlarge the microbial risks of reusing urine in agriculture. As far as the SARS-COV-2 is concerned, it may replicate in human gastrointestinal tract, exist in fecal excrement for a certain period of time, and may have infectious pathogenicity (Senecal et al., 2018). Zhang et al. (2020) investigated the feces of 23 patients, 83% of those were positive and the average duration of virus shedding was 22 days. During this period, the average titer of virus in feces was 5,623 copies/mL, but the highest titer reached 105.8 copies/mL at the peak (Zhang et al., 2020). Although the viral load in urine was quite low in the clinical study of sampling in aseptic environment (Kim et al., 2020), the viral load and its potential risk would increase a lot when urine was contaminated by feces in the field-scale projects of source separation. As such, cross contamination of feces is considered as a dominating risk of disease transmission when handling and reusing urine (Senecal et al., 2018). Therefore, due care should be taken in the design of new urine-diverting toilets, instructions of toilet use and training of urine collection users.
3 Pathogens in urine treatment and UDFPs
3.1 Direct use of urine after storage
Direct use of urine as liquid fertilizer is a commonly recommended simple approach for nutrient recycling from urine to agriculture. This has been successfully applied for the cultivation of microalgae (Adamsson, 2000; Golder et al., 2007), zooplankton (Adamsson, 2000) and various plants such as cucumbers (Heinonen-Tanski et al., 2007), lettuce, tomatoes (Adamsson, 2000; Mnkeni et al., 2008; Pradhan et al., 2009), okra (Akpan-Idiok et al., 2012), beet (Mnkeni et al., 2008; Pradhan et al., 2010a), pumpkin (Pradhan et al., 2010b), carrot (Mnkeni et al., 2008), maize (Guzha et al., 2005; Mnkeni et al., 2008; Antonini et al., 2012a), cabbage (Pradhan et al., 2007), water spinach (Yang et al., 2015), Lolium multiflorum Lam (Antonini et al., 2012a), wheat (Tidåker, 2003) and barley (Tidåker, 2003). However, the presence of pathogens may pose a potential risk for the users of fertilizer and the consumers of agriculture products. Particularly, the regrowth of some microorganisms such E. coli were even reported in fresh urine because of its appropriate pH and nutrients (Lahr et al., 2016; Ahmed et al., 2017).
To reduce the microbial risks of urine application, storage for 2–6 months is commonly recommended as a hygienic treatment method (Schonning, 2001; Jönsson et al., 2004; World Health Organization (WHO), 2006). Table 1 summarizes the inactivation of pathogens in urine after storage, including Aeromonas hydrophila (Höglund et al., 1998), Enterococci (Höglund et al., 2000), Enterococcus faecalis (Chandran et al., 2009; Nordin et al., 2013; Almeida et al., 2019), E. coli (Höglund et al., 1998; Höglund et al., 2000; Chandran et al., 2009; Wohlsager et al., 2010; Nordin et al., 2013; Ahmed et al., 2017; Zhou et al., 2017; Almeida et al., 2019; Oishi et al., 2020), fecal coliforms (Höglund et al., 2000; Wohlsager et al., 2010; Zhou et al., 2017; Almeida et al., 2019), fecal streptococci (Höglund, 2001; Wohlsager et al., 2010), human adenovirus (Decrey and Kohn, 2017), Mycobacterium tuberculosis (Vinnerås et al., 2011), Mycobacterium bovis (Vinnerås et al., 2011), Pseudomonas aeruginosa (Höglund, 2001), Salmonella typhimurium (Höglund et al., 1998; Vinnerås et al., 2008; Chandran et al., 2009; Wohlsager et al., 2010), Salmonella senftenberg (Höglund et al., 1998), sulphite reducing Clostridia (Höglund et al., 2000; Almeida et al., 2019), BK Human Polyomavirus (Goetsch et al., 2018), Rhesus rotavirus (Höglund et al., 2002), Ascaris suum, Ascaris suum egg (Nordin et al., 2009), and Cryptosporidium parvum oocysts (Höglund and Stenström, 1999). Furthermore, inactivation experiments sometimes used bacteriophage as virus surrogate, such as bacteriophage T3 (Goetsch et al., 2018), coliphage ФX174 (Vinnerås et al., 2008; Nordin et al., 2013; Decrey and Kohn, 2017), coliphage MS2 (Chandran et al., 2009; Decrey et al., 2015; Ahmed et al., 2017; Decrey and Kohn, 2017), coliphage T4 (Decrey and Kohn, 2017), Enterobacteria phage MS2 (Vinnerås et al., 2008; Nordin et al., 2013), and Salmonella typhimurium phage 28B (Höglund et al., 2002; Vinnerås et al., 2008). Most of these pathogens were reduced to an acceptable limit after 6 months.
Several factors may respond for the pathogens inactivation, of which free ammonia (NH3) induced by the hydrolysis of urea in the storage is considered as the dominating reason (Vinnerås et al., 2008). NH3 is one of the most important basic species in stored urine inducing the genome cleavage via alkaline transesterification, which results in the inactivation of the single-stranded (ss)RNA viruses (Decrey et al., 2015). Based on the same mechanism, other bases (carbonate, phosphate, and hydroxide) should also be taken into account for the viruses inactivation (Decrey et al., 2015). Furthermore, high temperature and high pH can accelerate the inactivation because these two factors also determine the concentrations of NH3 and other bases (Höglund et al., 1998; Höglund and Stenström, 1999; Hoglund et al., 2002; Vinnerås et al., 2008; Ahmed et al., 2017). For example, the inactivation rate constant K of E. coli increased from 0.20/d to 3.04/d at increasing temperature from 15°C to 35°C in storage of undiluted urine (Ahmed et al., 2017). 20°C or higher temperature was recommended for the current recommended storage time of 6 months (Vinnerås et al., 2008). Moreover, urine dilution brought by water flushing or pretreatment before fertilization (Jönsson et al., 2004) can decrease the concentration of NH3, thereby slowing the pathogens inactivation and extending the storage time for hygienic treatment (Vinnerås et al., 2008).
Notably, the types of pathogens of which the inactivation was experimentally studied (Table 1) are far less than that detected in urine (Supplementary Table S1). The inactivation of pathogens will be surely determined by its type. For example, the mean time for 1 log10 reduction (t90) of S. typhimuriuma, Enterococcus Faecalis, MS2, and ФX174 was <0.1 d, <1.1 d, <1.6 d, and <5.7 d, respectively, during the storage of urine at 34°C (Vinnerås et al., 2008). Some pathogens were still in high concentrations and even enriched after several months of storage. The concentration of Bacillus spores did not decrease during the urine storage because some microorganisms in the sporulated form have a higher resistance (Almeida et al., 2019). Moreover, the growth of streptococci was even found in urine collecting pipes and tank (Höglund et al., 1998). In addition, heterogeneity among viruses in their susceptibility toward NH3 is driven by the genome type. It is more difficult to inactivate the double-stranded (ds)RNA reovirus and Rhesus rotavirus as well as the dsDNA Salmonella phage 28B than ssRNA viruses (Decrey et al., 2015). Therefore, it is essential to carry out a comprehensive evaluation of the inactivation of possible pathogens particularly in areas with typical infectious diseases when urine was directly used as liquid fertilizer after storage.
In addition, several pretreatment methods were developed to accelerate the urea hydrolysis, which may accelerate the pathogens inactivation. These methods included dosage of urease (Kabdasli et al., 2006; Engelhardt et al., 2020), fecal incubation (Hotta and Funamizu, 2008), incubation of stale urine (Liu et al., 2008), increasing temperature (Liu et al., 2008) and microbial electrochemical current (Chen et al., 2017). However, incubation of fecal matter can simultaneously bring the contamination of urine by pathogens whether more pathogenic types or higher concentrations. As such, the pretreatment using fecal incubation should be re-considered taking the microbial risks into account.
3.2 Urine stabilization
Urea hydrolysis driven by the catalysis of urease occurred quickly in urine because many bacteria contaminated by feces or nature can produce urease (Udert et al., 2003a). More than half of urea hydrolyzed in the urine collecting pipes and storage tank (Udert et al., 2003b; 2003c). Although urea hydrolysis promotes the pathogens inactivation, it also brings the loss of nitrogen due to ammonia volatilization and the loss of phosphorus due to phosphate precipitation at alkaline pH. To inhibit urea hydrolysis, stabilization has been developed as a pretreatment before urine reuse or dewatering (Randall et al., 2016).
3.2.1 Acidification/alkalinization
Acidification (pH < 5 (Hellstrom et al., 1999; Saetta et al., 2020; Moharramzadeh et al., 2022)) or alkalinization (11 < pH < 13 (Randall et al., 2016)) can be used to inhibit the hydrolysis of urea due to the inactivation of urease-producing bacteria or urease. The inactivation of pathogens after urine acidification has been rarely reported maybe because the disinfection is not significant. By comparison, alkalinization of urine is considered as a promising method to inactivating pathogens as well as stabilizing urine (Randall et al., 2016). Lime is a common alkaline agent used for urine stabilization (Randall et al., 2016), sometimes combining with biochar (Simha et al., 2020a) and wood ash (Dutta and Vinnerås, 2016; Simha et al., 2020b). Pathogens inactivation in urine using lime has not been experimentally examined. However, lime is a well-known disinfection method for water and sewerage sludge because high pH was highly effective in the inactivation of pathogens (Bujoczek et al., 2002; Keller et al., 2004; Hansen et al., 2007). Moreover, bacteria Enterococcus faecalis and Salmonella spp. and bacteriophages MS2 and ФX 174 were reduced to below the detection limit when urine was alkalized using wood ash after 4 d at 20°C (Senecal et al., 2018) while the mean time for t90 of Enterococcus Faecalis, MS2, and ФX174 was 2.3, 15, and 12 d, respectively, during urine storage at 24°C (Vinnerås et al., 2008). Therefore, alkalinization would be much quicker and more effective than urine storage.
3.2.2 Chemical oxidation
Chemical oxidation using hydrogen peroxide or heat-activated peroxydisulfate could effectively inhibit urea hydrolysis due to the irreversible destruction of urease (Zhang et al., 2013; Lv et al., 2020). The two oxidants are normally used in disinfection of water (Jin et al., 2020; Xiao et al., 2020), providing a basis for pathogens inactivation of urine. However, chemical oxidation also brought the loss of nitrogen because of the oxidation of ammonium to nitrogen gas (Lv et al., 2020). This might limit the application of chemical oxidation for urine stabilization.
3.2.3 Biological nitrification
Urine after urea hydrolysis can also be stabilized through biological nitrification. Ammonium in hydrolyzed urine can be oxidized to nitrite or nitrate while the pH decreases to a weak acidic pH (i.e., 5–7) (Udert et al., 2003d; Feng et al., 2008; Jiang et al., 2011). This would reduce the volatilization of ammonia and the precipitation of phosphate salts. Furthermore, this produces a stabilized liquid fertilizer (Feng et al., 2008) or solid fertilizer when combining with distillation (Udert and Wächter, 2012; Fumasoli et al., 2016). However, the impact of nitrification on pathogens inactivation is not an interest even since nitrification was proposed for biological nitrogen removal from wastewater (Gujer, 2010). This means that biological nitrification may not be an effective technology on pathogens inactivation in urine. Notably, a recent study achieved the nitritation of urine in an acidic nitrifying bioreactor (Li et al., 2020). This may provide an opportunity to inactivate pathogens in urine because the product free nitrous acid is biocidal to a broad range of microorganisms (Jiang and Yuan, 2014) despite the target of nitritation was to obtain nitrite rather than recover nutrients from urine.
3.3 Dewatering urine to obtain concentrated fertilizer products
Despite being rich in nutrients, urine still has high content of water (approximately 97%) when urine is collected in a dry toilet. Urine will be further highly diluted when a water flushing toilet is used. Several dewatering technologies have been developed to concentrate urine to yield concentrated fertilizer products as well as clean water.
3.3.1 Membrane filtration
Urine can be dewatered to produce concentrated liquid fertilizer through membrane filtration including nanofiltration (Ray et al., 2020a; Courtney and Randall, 2022), reverse osmosis (Ek et al., 2006; Ray et al., 2020a; Courtney and Randall, 2022), forward osmosis (Zhang et al., 2014; Engelhardt et al., 2020; Jiang et al., 2022; Pocock et al., 2022), membrane distillation (Zhao et al., 2013; Volpin et al., 2019a; Khumalo et al., 2019) and electrodialysis (Brewster et al., 2017; Wang et al., 2017; De Paepe et al., 2018). Nutrients are rejected in the concentrate while water passes through the membrane filter to the other side in most of the filtration processes except the electrodialysis which concentrates nutrients in the permeate side.
Membrane filtration is a common technology to remove pathogens from water. The separation of bacteria and viruses from wastewater using polymeric and ceramic membrane has been summarized (Goswami and Pugazhenthi, 2020). The rejection of pathogens by filter mainly depends on the filter pore size due to the size exclusion mechanisms. Moreover, virus adsorption, electrostatic interaction and hydrophobic interaction also respond for the removal of pathogens from water (Goswami and Pugazhenthi, 2020). A nanofilter with pore size of 20 nm (Viresolve NFP filter, Millipore, United States) was employed to remove viruses from urine aiming to obtain virus free urokinase solution. The log reduction factors for porcine parvovirus (18–26 nm), human hepatitis A virus (25–30 nm), murine encephalomyocarditis virus (28–30 nm), bovine viral diarrhoea virus (40–60 nm), and bovine herpes virus (120–300 nm) were ≥4.86, ≥4.60, ≥6.87, ≥4.60, and ≥5.44, respectively (Kim et al., 2004). The rejection of pathogens by filter also means that pathogens would be concentrated in the liquid urine fertilizer. A recent study reported that concentration of urine can also lead to the inactivation of E. coli due to the high osmotic pressure under high salt contents (Oishi et al., 2020). 1-day storage was necessary for the safe reuse of 5-fold concentrated hydrolyzed urine whereas 91-h storage was required for non-concentrated urine when the initial numbers of E. coli were the same in these two urine solutions. However, the inactivation of pathogens might be inhibited due to the low concentration of free ammonia at low pH when acidification pretreatment was used to reduce the loss of ammonia to the water permeate (Tun et al., 2016; Xu et al., 2019a; Ray et al., 2020a).
Electrodialysis may pose an advantage than other membrane filtrations for dewatering urine. Nutrients were concentrated in the permeate side as the ionic species passed through selective ion-exchange membranes from the urine to the permeate side driven by an electric field (Pronk et al., 2006; De Paepe et al., 2018). Thus, pathogens would be retained in urine while yielding a pathogen free fertilizer product. However, this has not been examined by experiments.
Forward osmosis and membrane distillation were also employed to separate ammonia and urea from urine, producing ammonia- or urea-rich solution in the permeate side (Xu et al., 2019a; Ray et al., 2019; Ray et al., 2020b; Han et al., 2022). The N-rich solution would have low pathogen concentrations due to the rejection by the filter. Furthermore, a P-rich solid product could be obtained in a pretreatment using struvite precipitation before the filtration to avoid fouling on the membrane (Volpin et al., 2018; Volpin et al., 2019b). The combined processes successfully would achieve the recovery of N and P from urine. However, special attention should be paid to microbial risks of the struvite product formed in the urine with concentrated pathogens.
3.3.2 Evaporation/distillation
Urine can be passively evaporated at ambient temperature to produce concentrated fertilizer solution or solid fertilizer products (Bethune et al., 2014). The evaporation was further accelerated by solar irradiation (Antonini et al., 2012b; Bethune et al., 2015) or heating at 25–65°C (Dutta and Vinnerås, 2016; Senecal and Vinnerås, 2017; Simha et al., 2018; Simha et al., 2020a). The purpose of urine distillation at boiling temperature is mainly to obtain solid fertilizer products (Udert and Wächter, 2012; Fumasoli et al., 2016; Jiang et al., 2017). The boiling temperature even increased to 130.1°C when the salts in urine was highly concentrated following the distillation (Udert and Wächter, 2012). However, loss of the total ammonia was observed due to the volatilization of free ammonia during the evaporation of water (Bethune et al., 2014,2016). Therefore, stabilization pretreatment such as acidification (Jiang et al., 2017), alkalinization (Dutta and Vinnerås, 2016; Senecal and Vinnerås, 2017; Simha et al., 2018; Simha et al., 2020a) and nitrification (Udert and Wächter, 2012; Fumasoli et al., 2016) was usually employed prior to the evaporation or distillation process.
Several factors involved in the evaporation/distillation of urine can inactivate pathogens. High temperature is highly effective to inactivate pathogens as reported in the fields of waste composting or water disinfection (Chu et al., 2019; Hu et al., 2020). Moreover, Bethune et al. (2014) suggested that the urine-derived solid product was sterile because high salinity in the concentrated product might respond for the inactivation of microorganisms. In addition, solar thermal evaporation may further enhance the pathogen inactivation because sunlight radiation is considered as a unconventional disinfection technology to obtain safe drinking water (Chu et al., 2019). The inactivation of pathogens would be further enhanced when alkaline pretreatment was employed to inhibit the urea hydrolysis before evaporation/distillation. In an alkaline dehydration of urine using ash at 42°C, five fecal pathogens including Ascaris suum, Enterococcus faecalis, bacteriophages MS2 and ФX 174, and Salmonella spp. were inactivated to below the detection limit within 10 d (Senecal et al., 2018), which met the World Health Organization (WHO) guidelines for the reuse of excreta (World Health Organization (WHO), 2006). Although acidification or biological nitrification may be not effective on the pathogen inactivation, the following evaporation/distillation can play a dominating role (Bischel et al., 2015).
The evaporation/distillation of urine can also produce clean water for reuse. Distillation at boiling temperature would also promise a sterile water product because boiling temperature is very efficient on disinfection (Chu et al., 2019). However, reclaimed water collected after evaporation at ambient temperature or high temperature around 35–65°C may still pose microbial risks. Pathogens in the concentrated fertilizer solution or the solid product may be inactivated by the synergistic effects of high salinity, pH and temperature. But single effect of temperature on the water disinfection may not act as effectively as on the solid product disinfection. Thus, the reuse of the reclaimed water after the evaporation/distillation of urine should be further addressed.
3.3.3 Freezing-thawing
Freeze crystallization can recover water in the form of ice from urine as well as concentrating the fertilizer solution. Low temperature ranges of −18 to −4°C were examined for freezing urine (Lind et al., 2001; Gulyas et al., 2004; Schmidt and Alleman, 2005; Ganrot et al., 2007a; Yu et al., 2007; Ganrot et al., 2008; Moharramzadeh et al., 2022). A thermodynamic model was further developed providing a theoretical understanding on obtaining solid salts and water from stored urine (Randall and Nathoo, 2018). After thawing, the reclaimed water and the concentrated fertilizer product can be obtained.
The influence of freezing and thawing on pathogens seems to be unclear. Freezing creates damage to cell structure of microorganisms (Sanin et al., 1994). It was found that the reduction of pathogenic bacteria (Salmonella), virus (Poliovirus) and parasites (Ascaris suum and Cryptosporidium parvum) was effectively achieved by freezing-thawing coupled with anaerobic digestion of sludge in a wastewater treatment plant, meeting the pathogen reduction requirements for sludge land disposal by US regulations (Sanin et al., 1994). Furthermore, freezing is widely used in food storage because of its negative effect on microbial community. For examples, storage for more than 3 days at −20°C can effectively inactivate T. gondii in contaminated meat (Alizadeh et al., 2018). However, freeze-drying is also considered as a common cryopreservation method to preserve pathogens because the ultra-low temperature can fix the cells in powder and nearly completely inhibit the cell metabolism (Perry, 1998). For example, the freeze-drying was sustainable for the long-term preservation of marine pathogen V. anguillarum (Yu et al., 2019). Furthermore, the stability and retention of infectivity of viruses was confirmed after frozen storage of foods, indicating negative effect on killing viruses (Sánchez and Bosch, 2016). In addition, it was reported that sublethally injured pathogens (E. coli) caused by freezing during food storage can recover after thawing, presenting a potential threat on food safety (Zhang R. et al., 2021). Therefore, the related publications can’t show a clear understanding whether freeze-drying is bad or good to effectively deactivate pathogens in urine.
3.4 Extraction of nutrients from urine
3.4.1 Stripping-absorption of ammonia to obtain liquid N-products
Ammonia separated from urine by air (Behrendt et al., 2002; Basakcilardan-Kabakci et al., 2007; Antonini et al., 2011; Morales et al., 2013; Liu et al., 2015; Xu et al., 2017; Jagtap and Boyer, 2018; Jagtap and Boyer, 2020) or membrane stripping (Tarpeh et al., 2018a; Christiaens et al., 2019; Pradhan et al., 2019) can be absorbed in water or sulfate acid solution to yield liquid N-products such as ammonia water or ammonium sulfate solution. However, an autofluorescent E. coli spiked in the urine was also observed in the absorbent (Christiaens et al., 2019), which should be caused by the transfer of microorganisms from urine to the absorbent via aerosols induced by air stripping (Benami et al., 2016). This indicates potential microbial risks of using the ammonia absorbent products in agriculture. Alkalinization and heating is usually used to improve ammonia stripping efficiency from urine. pH >12 maintained by lime increased the removal efficiency by 8% for the stripping of pure urine at 30°C (Pradhan et al., 2017). Furthermore, high temperature ranging in 40°C–65°C could significantly increase the stripping efficiency because of the formation of more free ammonia (Pradhan et al., 2017; Wei et al., 2018; Tao et al., 2019; Tian et al., 2019). High pH and temperature would meanwhile benefit the inactivation of pathogens in urine influent as well as reducing the pathogens in absorbent.
Membrane stripping provide another approach to reduce the transfer of pathogens to absorbent. Free ammonia in the urine side pass through a gas permeate membrane (GPM) to the absorbent side. Pore size of the GPM commonly ranges in 0.1–0.59 µm (Tarpeh et al., 2018a; Xu et al., 2019a; Christiaens et al., 2019). As such, most pathogenic bacteria and some viruses would be rejected by the membrane, producing N-products with less pathogens in the permeate side. A study by Tarpeh et al. (2018a) confirmed that E. coli was not observed in the absorbent of a membrane stripping process when its concentration was 108.00–8.78 events/mL in the urine feed. However, it was also found that very small microorganisms reported in urine (<0.1 µm) could pass through the membrane with an average measured pore size of 0.274 µm (Dong et al., 2011). Thus, stripping via hydrophobic GPM does not lead to a pathogens-free absorbent. Similar as that in the air stripping process, high pH and temperature intending for high ammonia transfer efficiency in membrane stripping (Xu et al., 2019a) would probably inactivate pathogens in the feed side and reduce the transfer of pathogens to the permeate side.
Concentrating ammonia using cation exchange membrane (CEM) in electrodialysis can increase the concentration of free ammonia before stripping (Christiaens et al., 2017; Tarpeh et al., 2018a; Christiaens et al., 2019). Tarpeh et al. (2018a) developed a novel process combining electrodialysis and membrane stripping to yield an E. coli-free fertilizer product from urine for potential safe reuse. Pore size of CEM ranges from 1.5 to 200 nm (Kononenko et al., 2017), which would benefit rejecting the transfer of pathogens with small sizes such as nanoscale viruses.
3.4.2 Adsorption of nutrients to obtain solid fertilizer products
Urea, ammonium, phosphate and potassium in urine can be adsorbed by various adsorbents such as activated carbon (Ganrot et al., 2007a), biochar (Bai et al., 2018; Xu et al., 2018; Xu et al., 2019b; Zhang X. et al., 2021), gastropod shell (Saliu et al., 2020), ion exchange resin (O’Neal and Boyer, 2013; O’Neal and Boyer, 2015; Tarpeh et al., 2017; Tarpeh et al., 2018b), layered double hydroxides (LDH) (Dox et al., 2019a; Dox et al., 2019b; Dox et al., 2022), magnetic Fe3O4@ZrO2 nanoparticles (Guan et al., 2020a), metal organic frameworks (MOF) (Lin et al., 2015; Guan et al., 2020b), natural loess (Jiang et al., 2016), porous organic polymer (Zhang et al., 2020), wollastonite (Lind et al., 2000), zeolite (Ban and Dave, 2004; Beler-Baykal et al., 2004; Ganrot et al., 2007a; Leung et al., 2007; Ganrot et al., 2008; Baykal et al., 2009; Beler-Baykal et al., 2011; Huang et al., 2014; Xu S. et al., 2015; Mitrogiannis et al., 2018; Makgabutlane et al., 2020; Regmi and Boyer, 2020). Some of these adsorbents i.e., biochar, gastropod shell, natural loess, and zeolite after nutrient adsorption can be directly used as fertilizer in agriculture (Ganrot et al., 2008; Beler-Baykal et al., 2011; Xu S. et al., 2015; Jiang et al., 2016; Bai et al., 2018; Xu et al., 2018; Saliu et al., 2020; Zhang X. et al., 2021). Other adsorbents should be desorbed in solutions containing NaOH (Beler-Baykal et al., 2004; O'Neal and Boyer, 2015; Mitrogiannis et al., 2018; Guan et al., 2020a; Guan et al., 2020b), NaHCO3 (Mitrogiannis et al., 2018; Dox et al., 2019a; Dox et al., 2019b), NaCl (Lin et al., 2015; O'Neal and Boyer, 2015; Mitrogiannis et al., 2018; Zhang et al., 2020), HCl (Mitrogiannis et al., 2018; Guan et al., 2020b), or H2SO4 (Tarpeh et al., 2017; Tarpeh et al., 2018b) to obtain phosphate-rich solution, after which a chemical precipitation process such as struvite precipitation was usually conducted to yield solid phosphate fertilizer easy to be collected and applied for agriculture. However, to our best knowledge, the behavior of urine pathogens during the nutrient adsorption has not been reported.
Normally, some adsorbents such as zeolites and biochar can uptake aquatic microorganisms, forming biofilm on the surface (Perez-Mercado et al., 2019; Wang et al., 2020). The uptake of microorganisms is mainly driven by weak electrostatic or binding forces to the carrier surface and the intra-grain and intra-crystallite pores (Lameiras et al., 2008). Experiments confirmed the uptake of fecal coliforms and E. coli from wastewater by clinoptilolite, a naturally occurring zeolite, with an active uptake of 3989 CFU/g and 2732 CFU/g, respectively (Ferronato et al., 2015). Furthermore, a correlation model based on experiments also showed that biochar filters could significantly remove bacteria and viruses with the reduction rate of ≥2 log10 and ≥1 log10, respectively, because these microbes were enriched on biochar due to adsorption (Perez-Mercado et al., 2019). This indicates that the microbial risks would even be magnified especially when the nutrient-enriched adsorbents are directly used as fertilizer in agriculture.
Various metal ions have been used as biocidal agents such as Ag, Zn, Cu, Ni, Co, Ti and Bi (Wyszogrodzka et al., 2016). Zeolite exchanged with Cu and Zn can be used as antimicrobial material for efficient disinfection of contaminated water with bacteria because it was effective to reduce the concentrations of E. coli and Staphylococcus aureus (Yao et al., 2019). This may provide a potential approach to reduce the microbial risks of nutrient-adsorbed solid fertilizer but pose a potential risk of heavy metals. Post treatment in an alkaline desorption solution may further inactivate pathogens adsorbed on adsorbents because alkaline treatment is effective in the disinfection of urine pathogens (Senecal et al., 2018). However, it is unclear that whether desorption of nutrients from adsorbents in acid solution could inactivate pathogens.
3.4.3 Chemical precipitation to obtain solid products
Chemical precipitation is a commonly reported approach to recover nutrients from urine by the formation of non-soluble phosphate salts. Struvite precipitation is the most concerned phosphorus recovery technology which can also simultaneously recover part of ammonium from urine (Krähenbühl et al., 2016; Zamora et al., 2017; Wei et al., 2018; Zheng et al., 2018; Aguado et al., 2019; Li P. et al., 2019; Pinatha et al., 2020; Oztekin et al., 2022). The precipitation of struvite-K, an analogue of struvite, can simultaneously recover phosphate and potassium from ammonium-depleted urine (Xu et al., 2011; Xu et al., 2012; Xu K. et al., 2015; Xu et al., 2017; Zhang et al., 2017; Zhang et al., 2018; Huang et al., 2019). Furthermore, phosphate can also be recovered by the precipitation of calcium phosphate (Pradhan et al., 2017). Finally, filtration and drying following the precipitation process is essential to obtain solid phosphate products. Struvite and struvite-K can be directly used as fertilizer in agriculture because they are both slow-release fertilizer while calcium phosphate is considered as a raw material for phosphate fertilizer industry (Ganrot et al., 2007b; Liu et al., 2020). There are standards for microbial risk management in industrial fertilizer (Ministry of Industry and Information Technology of the People’s Republic of China, 2019). Therefore, this review mainly addresses the potential microbial risk of the recovered phosphate products which can be directly used in agriculture.
Decrey et al. (2011) found that the struvite precipitation process did not considerably inactivate the pathogen indicators phage ΦX174 and Ascaris suum eggs in the urine liquid fraction. The pH value during the struvite precipitation in urine was approximately 9 (Etter et al., 2011), which is similar as that in the storage process. This indicates that free ammonia formed at such alkaline pH can inactivate pathogens like that in the storage process. However, the reaction time for struvite formation was only 10 min in their study (Decrey et al., 2011). Some other studies used longer precipitation time ranging from 15 to 125 min (Ronteltap et al., 2007; Ronteltap et al., 2010; Liu et al., 2013), which is still much less that the storage time of 2‒6 months (Jönsson et al., 2004; Vinnerås et al., 2008). As such, the pathogen inactivation can be neglected within such a short precipitation time. At a longer precipitation time of 24 h, the number of coliforms was reduced by 56% during the struvite precipitation at pH 9 in anaerobically digested chicken slurry (Muhmood et al., 2018). Furthermore, they also reported a greater reduction of coliforms (73%) at pH 10. The viability of spiked E. coli cells also significantly decreased during the struvite precipitation at pH of 11 in source-diverted blackwater (Yee et al., 2019). Non-etheless, optimum pH of the struvite precipitation was approximately 9 and higher pH decreased the struvite purity (Harada et al., 2006). Thus, high pH for effective pathogen inactivation may be suitable for precipitation of calcium phosphate rather than the struvite precipitation. Complete inactivation of E. coli, Salmonella enterica serovar typhymurium, and Porcine circovirus type 2 was achieved when removing phosphate from swine slurry at pH 10 using Ca(OH)2 (Viancelli et al., 2015). The coliforms was also completely inactivated after 6 h of treatment using wood ash to remove phosphate from urban wastewater and landfill leachate at pH of 10.1–12.7 (Ivanković et al., 2014). In addition, the optimal pH in ranges of 10–11 for the precipitation of struvite-K (Xu et al., 2011; Xu et al., 2012; Xu K. et al., 2015) may also improve the pathogens inactivation in the liquid fraction despite this has not been reported.
It is essential to evaluate the pathogens in the solid nutrient product obtained from urine especially when the product is directly used as fertilizer. Pathogen indicators phage ΦX174 and Ascaris suum eggs were both detected in the struvite product filtered by nylon fabric while no considerable inactivation was observed in the precipitation process in urine (Decrey et al., 2011). There was no significant difference on concentration of phage ΦX174 in both the solid and the liquid fraction. However, the Ascaris eggs even accumulated by 100 fold within the solid product because the filter with irregular pore diameters of 18–240 µm rejected the Ascaris eggs having a size of 35–50 × 45–70 µm in the solid fractions. Bischel et al. (2016) also reported the accumulation of heterotrophs and total bacteria in the struvite product obtained by the filtration with nylon or cloth filters from urine. Non-etheless, these two publications cannot clearly display an actual behavior of the pathogens in the solid fraction of struvite precipitation because of the effect of filtration on the pathogens accumulation. Electrostatic attraction and repulsion may play an important role for behavior of pathogens in solid struvite. Struvite crystals are negatively charged with zeta-potential of −17.5 to −27.6 mV at pH from 8.5 to 10.5 (Le Corre et al., 2007). Yee et al. (2019) reported that the electrostatic attraction between C. perfringens with positive zeta potential of 0–3 mV and struvite particles increased the cell aggregation in the final solid product. However, there was an electrostatic repulsion between struvite and phage ΦX174 with isoelectric point of 6.6 (Michen and Graule, 2010) carrying negative surface charge at pH around 9 (Muhmood et al., 2018). Moreover, isoelectric points of most viruses ranged from 1.9 to 8.4 (Michen and Graule, 2010). Thus, these viruses would present negative surface charge in the struvite precipitation system of urine, indicating electrostatic repulsion between struvite and viruses.
Factors affecting the surface charge of struvite may change the adsorption of pathogens onto struvite particles. High concentration of residual Mg2+ lowered the negative charge of struvite which could lead to more adsorption of pathogens with low isoelectric point (Decrey et al., 2011). Coagulants and flocculants may also result in more adsorption of pathogens when they were used to promote the agglomeration of struvite crystals (Le Corre et al., 2007). The adsorption of pathogens would probably be enhanced during the precipitation of struvite on metal-modified biochar (Xu et al., 2018; Liu et al., 2020) or zeolite (Huang et al., 2014; Mitrogiannis et al., 2018) with positive surface charge. In addition, the tendency of bacteria growth on these carrier (Perez-Mercado et al., 2019; Wang et al., 2020) would further lead to accumulation of more pathogens in the struvite product.
Although there lacks understanding on the adsorption of pathogens on struvite and the following filtration rejects some pathogens with large size in the struvite filter cake, pathogens in the solid products can still be effectively inactivated in the following drying process (Bischel et al., 2016). Temperature and humidity were important factors determining the efficiency of pathogens inactivation. No significant inactivation of Ascaris suum eggs in struvite obtained from urine were observed at 5 and 20°C while the eggs was inactivated by more than 99% at 35–36°C after drying for 3 days (Decrey et al., 2011). Furthermore, inactivation of Ascaris suum eggs was only 1.2 log10 after 3 days drying at temperature of 35°C and relative humidity of 85% while it reached more than 2 log10 after 1 day of drying at temperature of 36°C and relative humidity of 36%. Thus, inactivation of Ascaris suum eggs in struvite drying was enhanced at low relative humidity (Decrey et al., 2011). Moreover, the reduction of phage ΦX174 in struvite was 0.03, 0.07 and 0.09 log10/day in the drying process at 5°C/85% (temperature/relative humidity), 20°C/93% and 5°C/35%, respectively. As such, its inactivation was enhanced at high temperatures and low relative humidity. Experimental results further showed that the inactivation of heterotrophic bacteria, total bacteria, Enterococcus spp. and Salmonella typhimurium accumulated in struvite from urine was also improved with increasing temperature for drying at a constant relative humidity (Bischel et al., 2016). The reduction of heterotrophs even reached 3 log10 at optimized drying conditions within 100 h. Therefore, drying, also as an essential process to obtain final solid struvite product, is recommended as an effective method for the disinfection of the final product. Experimental results confirmed that the concentrations of total coliforms and E. coli were under the detection limits when struvite formed in anaerobically digested chicken slurry was dried at 40°C without control of humidity for 48 h after a filtration using 0.45 μm membrane (Muhmood et al., 2018; Muhmood et al., 2019). Drying at higher temperature than the ambient temperature already examined in experiments will reasonably improve the pathogens inactivation of struvite product. However, this may also result in the loss of crystal water and ammonia in the struvite crystals due to its decomposition (Bhuiyan et al., 2008).
Drying struvite by sunlight is also an option especially for small-scale reactor. However, Decrey et al. (2011) found that no significant inactivation of phage ΦX174 or Ascaris suum eggs was observed when struvite was exposed to sunlight within 5 h at 31°C with relative humidity of <35%. The short exposure time may be one of the dominating reasons for ineffective inactivation. Exposure to sunlight will increase the temperature of struvite and accelerate the loss of struvite moisture, thereby theoretically introducing the disinfection of struvite. Moreover, sunlight radiation in ultraviolet range is known to be effective in pathogens inactivation (Chu et al., 2019). Therefore, struvite disinfection is expected to be realized to some extent for long time of exposure in sunlight.
Decomposition of struvite (Eq. 1) can release ammonia which can be recovered through absorption in water or acid solution and the solid decomposition product can be employed to remove ammonia from urine through the re-precipitation of struvite (Eq. 2) (Chen et al., 2020). The decomposition was commonly conducted in ranges of 100–150°C using calcination (Huang et al., 2016), ultrasound radiation (Huang et al., 2017), and microwave radiation (Chen et al., 2020), the latter two processes of which were even carried out in alkaline solution containing NaOH. High temperature and combination of alkaline condition would probably inactivate pathogens in struvite, thus promising clean products of solid struvite and liquid ammonia-rich solution.
3.5 An overall assessment
Table 2 summarizes the overall assessment of pathogens inactivation in different urine treatment processes. Storage for 2–6 months was considered as an effective pretreatment technology to inactive pathogens when urine was used as liquid fertilizer (Schonning, 2001; Jönsson et al., 2004; World Health Organization (WHO), 2006). Urine dewatering and dehydration technologies are developed to obtain concentrated liquid fertilizer or solid fertilizer. Pathogens could be retained in concentrated urine fertilizer by membrane treatment using reverse osmosis, forward osmosis, and nanofiltration. This would bring strong negative effect on pathogens inactivation. However, electrodialysis may effectively remove pathogens because concentrated liquid fertilizer is obtained in the permeate side and pathogens are retained in the urine feed side. Moreover, membrane distillation should have strong positive effect on inactivating pathogens in concentrated urine fertilizer because of alkalinization or heating of urine feed. Evaporation under heating or distillation will probably yield sterile concentrated liquid or solid fertilizer products because high temperature is effective for pathogens inactivation.
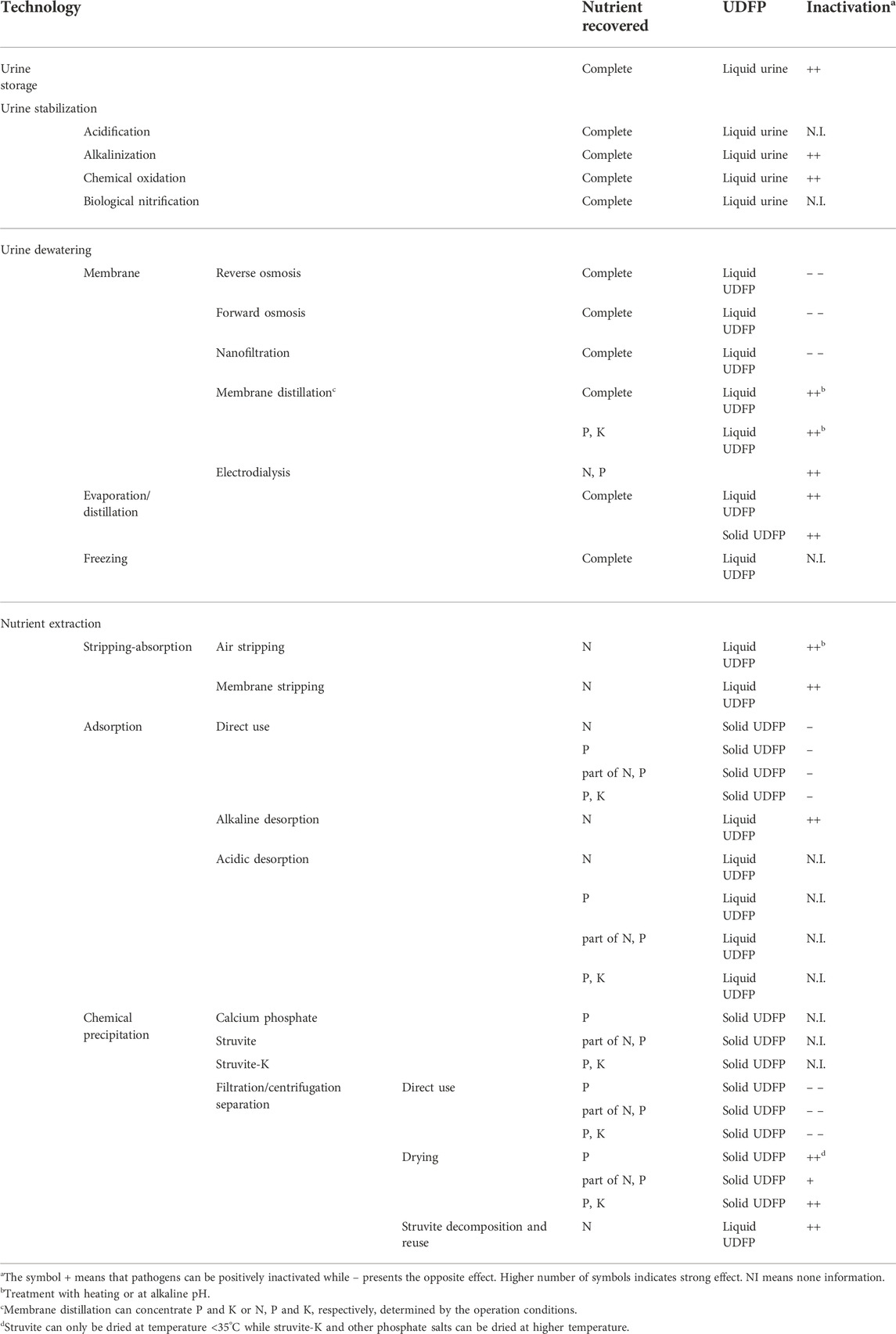
TABLE 2. An overall assessment of pathogens inactivation in urine treatment technologies and the related UDFPs.
Urine stabilization is commonly essential before urine dewatering to prevent loss of ammonia due to urea hydrolysis. Alkalinization can be used to enhance the pathogens inactivation as a pretreatment process, which may be particularly important for dewatering technologies without inactivation effect such as reverse osmosis, forward osmosis, nanofiltration and freezing. Pathogens would also be effectively inactivated by chemical oxidation. However, the effect of chemical oxidation on the characteristics of UDFPs is unclear. Moreover, it is also unclear whether acidification or biological nitrification can inactivate pathogens in urine. Thus, these two technologies would be suitable for urine stabilization before urine dewatering through membrane distillation, electrodialysis, evaporation and distillation while may be unsuitable prior to urine dewatering using reverse osmosis, forward osmosis, nanofiltration and freezing.
Absorption of ammonia by water or acid solution following stripping can recover N and yield liquid ammonia fertilizer. Air stripping with heating or at alkaline pH and membrane stripping would probably have strong positive effect on pathogens inactivation. Adsorption can recover specific nutrients from urine determined by the adsorbents’ characteristics. However, direct use of the solid nutrients-enriched adsorbents in agriculture may pose microbial risks because pathogens may also be adsorbed on the UDFP. Alkaline desorption of nutrient from the solid UDFP to yield liquid fertilizer may be a promising post-treatment process to reduce the microbial risks. Non-etheless, alkaline desorption may be only suitable for ammonia recovery from urine. None information could be obtained on the pathogens inactivation through acidic desorption despite this post-treatment process can recover N, P and K in liquid UDFP. There lacks understanding on the inactivation of pathogens in the chemical precipitations of calcium phosphate, struvite, and struvite-K. However, the following filtration or centrifugation separation of precipitates from urine would retain pathogens in the solid UDFP. This may pose strong negative effect on pathogens inactivation in UDFP. Post-treatment through drying with heating could effectively inactivate pathogens in the solid UDFP. Moreover, recovery of total ammonia in urine through recycling use of struvite precipitation and decomposition may also yield sterile liquid ammonia fertilizer because of struvite decomposition with heating and alkaline treatment.
Combination of different processes is usually essential to extract N, P and K from urine. Adsorption and precipitation was combined to yielding solid N-P enriched UDFP (Lind et al., 2000; Ban and Dave, 2004; Ganrot et al., 2007a; Ganrot et al., 2008; Xu S. et al., 2015; Zheng et al., 2018). This combined processes would have negative effect on pathogens inactivation because each separated process has negative effect. Moreover, precipitation of struvite and stripping-absorption can be combined to recover N and P producing liquid ammonia fertilizer and solid struvite. Pathogens in the liquid ammonia fertilizer would be reduced because of heating and alkaline treatment of urine. However, the pathogens in struvite were determined by the order in the combination. Pathogens in urine can be inactivated in the stripping process. Thus, struvite obtained in a precipitation process following the stripping (Pradhan et al., 2017) would have lower contents of pathogens than that yielded in a precipitation process followed by stripping(Antonini et al., 2011; Morales et al., 2013; Wei et al., 2018; Tao et al., 2019; Yang et al., 2022; Zhang et al., 2022). However, pathogens in solid product obtained in combined processes of stripping and precipitation of struvite-K may be inactivated because the operating pH in this precipitation was approximately 11 which benefits pathogens inactivation (Xu et al., 2017). This indicates that more attention should be paid to the interaction effects of processes combination on pathogens inactivation in further studies.
It should be noted that this study has not reviewed the treatment technologies which are developed to remove pollutants or recover energy from urine. Biological processes including nitrification-denitrification (Udert et al., 2003d), partial nitrification-denitrification (Yao et al., 2017) and nitritation-anammox (Buergmann et al., 2011) can remove nitrogen from urine. Microbial electrolysis cell can remove organics in urine (Boggs et al., 2009; Barbosa et al., 2018; Barbosa et al., 2019). The removal of N and organics has been examined through electrochemical oxidation processes (Amstutz et al., 2012; Dbira et al., 2015; Zoellig et al., 2015; Zollig et al., 2017; Cotillas et al., 2018a; Dbira et al., 2019). Energy production has been achieved via microbial fuel cells (You et al., 2016; Salar-Garcia et al., 2017; Gajda et al., 2019; Sabin et al., 2022). Advanced oxidation processes including chemical oxidation (Sun et al., 2018; Li et al., 2022), photocatalytic oxidation (Zhang et al., 2016), and electrochemical oxidation (Antonin et al., 2015; Cotillas et al., 2018b) have been developed to remove micropollutants. Pathogens inactivation in these technologies has not been discussed in this review. Microbial risks in UDFPs should be paid more attention when these technologies are combined with nutrient recovery technologies.
In addition, only the pathogens inactivation in storage and alkaline stabilization has been experimentally examined. Most of the cases were assessed based on publications about their effects on pathogens inactivation in other fields rather than in urine treatment. Moreover, none information on the pathogens inactivation could be obtained when urine was treated using acidic stabilization, stabilization via biological nitrification, dewatering via freezing or desorption after nutrient adsorption. This means that systematic and meticulous research work is urgently needed to provide technical support for better and safe development of the concept of urine source separation. This is particularly important for achieving U.N. SDGs in developing countries and regions lacking sanitation.
4 Outlook on microbial risk management of urine source separation
Disinfection of UDFPs and agricultural products is essential if recovery technology can’t effectively inactivate pathogens in urine and the fertilizer product. Conventional technologies including high-temperature sterilization, ultraviolet disinfection and chemical oxidation disinfection have been proved to be effective for product disinfection (US Centers for Disease Control and Prevention, 2008). Cooking agricultural products before eating will be preferred to reduce the microbial risk by consumers. However, few publications reported the disinfection of UDFPs. Due care should be taken if these disinfection technologies would affect the fertilizer characteristics in further studies.
Moreover, it is essential to set standards on pathogen limitations in UDFPs and technical standards from the aspect of microbial risk management. The related standards lacks despite the concept of urine source separation has been proposed for nearly 30 years. The WHO guidelines recommends that the number of E. coli in water is less than 104/100 ml in the reuse of water in drip irrigation (World Health Organization (WHO), 2006). The Environmental Protection Agency regulations suggest that the number of E. coli should be less than 102/100 ml in the reused water (Environmental Protection Agency, 2012). The maximum allowable concentration of bacteria is 1000 CFU/g in recycled organic waste fertilizer products following the European Animal By-Products Regulation (The European Parliament and of the Council, 2002). These regulations may provide references for UDFPs which are also used for agriculture. However, this has to be addressed in further studies.
Effective inactivation of pathogens in UDFPs would reduce the microbial risk to farmworkers and consumers of agricultural products. However, urine collection and transportation can also bring health risk to users. It is commonly assumed that the direct accidental ingestion of urine was 1 ml per case in recycling of urine in agriculture (Hoglund et al., 2002; Ahmed et al., 2017). Non-etheless, a recent study found that indirect ingestion also played a role in the exposure of urine collection users to urine (Bischel et al., 2019). The indirect ingestion refers to hand to mouth contacts with pathogens when hands, skin and clothes are contaminated and then hands bring pathogens to mouth. Furthermore, operating struvite precipitation reactor also resulted in health risk due to direct and indirect contacts with pathogens-carrying substances (Bischel et al., 2019). This indicates that microbial risk in urine source separation should consider all possible exposure pathways besides the exposure to UDFPs. Therefore, it is recommended that personal protective equipment (facemasks, gloves, work clothes, etc.) is essential to reduce the exposure of urine collection users and farmworkers to urine and UDFPs.
5 Conclusion
Pathogens in source-separated urine can be introduced through two approaches including excretion with urine and fecal contamination. A summary on bacteria and viruses detected in urine confirms that urine is not sterile. Then, this study reviewed and evaluated pathogens inactivation in urine treatment technologies classified based on their UDFPs. Storage and alkaline stabilization have experimentally proved effective effects on pathogens inactivation of urine. Technologies including membrane distillation, electrodialysis, evaporation, distillation, air stripping, and membrane stripping should have positive effect on pathogens inactivation of UDFPs because alkaline treatment, high temperature and membrane separation in these technologies could reduce pathogens. However, pathogens might be concentrated when urine is dewatered using reverse osmosis, forward osmosis, and nanofiltration. Moreover, adsorption and precipitates separation using filtration and centrifugation may also present negative effect on pathogens inactivation in UDFPs. Non-etheless, post treatment through alkaline desorption, precipitates drying, and struvite decomposition and recycle would benefit the disinfection of UDFPs. Finally, an overall assessment was carried out to show a clear map of the connection of technologies and the pathogens inactivation in UDFPs. Based on the assessment, this review discussed the lack of experimental studies on pathogens inactivation in urine treatment and presented outlooks on development of new urine treatment technology and management of microbial risk in urine recycling in agriculture.
Author contributions
KX: investigation and writing—original draft. JLu and HL: investigation, writing—review and editing. JLi: conceptualization, writing—review and editing, funding acquisition. SC: conceptualization, writing—review and editing. MZ: writing—review and editing. CW: conceptualization.
Funding
This study was supported by the National Key Research and Development Plan of China (No. 2020YFD1100102).
Conflict of interest
The authors declare that the research was conducted in the absence of any commercial or financial relationships that could be construed as a potential conflict of interest.
Publisher’s note
All claims expressed in this article are solely those of the authors and do not necessarily represent those of their affiliated organizations, or those of the publisher, the editors and the reviewers. Any product that may be evaluated in this article, or claim that may be made by its manufacturer, is not guaranteed or endorsed by the publisher.
Supplementary material
The Supplementary Material for this article can be found online at: https://www.frontiersin.org/articles/10.3389/fenvs.2022.1056019/full#supplementary-material
References
Adamsson, M. (2000). Potential use of human urine by greenhouse culturing of microalgae (Scenedesmus acuminatus), zooplankton (Daphnia magna) and tomatoes (Lycopersicon). Ecol. Eng. 16 (2), 243–254. doi:10.1016/S0925-8574(00)00064-1
Aguado, D., Barat, R., Bouzas, A., Seco, A., and Ferrer, J. (2019). P-recovery in a pilot-scale struvite crystallisation reactor for source separated urine systems using seawater and magnesium chloride as magnesium sources. Sci. Total Environ. 672, 88–96. doi:10.1016/j.scitotenv.2019.03.485
Ahmed, W., Hamilton, K. A., Vieritz, A., Powell, D., Goonetilleke, A., Hamilton, M. T., et al. (2017). Microbial risk from source-separated urine used as liquid fertilizer in sub-tropical Australia. Microb. Risk Anal. 5 (SI), 53–64. doi:10.1016/j.mran.2016.11.005
Akpan-Idiok, A. U., Udo, I. A., and Braide, E. I. (2012). The use of human urine as an organic fertilizer in the production of okra (Abelmoschus esculentus) in South Eastern Nigeria. Resour. Conservation Recycl. 62, 14–20. doi:10.1016/j.resconrec.2012.02.003
Alizadeh, A. M., Jazaeri, S., Shemshadi, B., Hashempour-Baltork, F., Sarlak, Z., Plievar, Z., et al. (2018). A review on inactivation methods of Toxoplasma gondii in foods. Pathogens Glob. Health 112 (6), 306–319. doi:10.1080/20477724.2018.1514137
Almeida, J. A. S., Azevedo, A. L., Brett, M., Tadeu, A., Silva-Afonso, A., Costa, A., et al. (2019). Urine recovery at the building level. Build. Environ. 156, 110–116. doi:10.1016/j.buildenv.2019.04.006
Almeida, J. A. S., Azevedo, A. L., Brett, M., Tadeu, A., Silva-Afonso, A., Costa, A., et al. (2019). Urine recovery at the building level. Build. Environ. 156, 110–116. doi:10.1016/j.buildenv.2019.04.006
Amstutz, V., Katsaounis, A., Kapalka, A., Comninellis, C., and Udert, K. M. (2012). Effects of carbonate on the electrolytic removal of ammonia and urea from urine with thermally prepared IrO2 electrodes. J. Appl. Electrochem. 42 (9SI), 787–795. doi:10.1007/s10800-012-0444-y
An, X., Wang, J., Pu, Q., Li, H., Pan, T., Li, H., et al. (2020). High-throughput diagnosis of human pathogens and fecal contamination in marine recreational water. Environ. Res. 190, 109982. doi:10.1016/j.envres.2020.109982
Antonin, V. S., Santos, M. C., Garcia-Segura, S., and Brillas, E. (2015). Electrochemical incineration of the antibiotic ciprofloxacin in sulfate medium and synthetic urine matrix. Water Res. 83, 31–41. doi:10.1016/j.watres.2015.05.066
Antonini, S., Arias, M. A., Eichert, T., and Clemens, J. (2012a). Greenhouse evaluation and environmental impact assessment of different urine-derived struvite fertilizers as phosphorus sources for plants. Chemosphere 89 (10), 1202–1210. doi:10.1016/j.chemosphere.2012.07.026
Antonini, S., Paris, S., Eichert, T., and Clemens, J. (2011). Nitrogen and phosphorus recovery from human urine by struvite precipitation and air stripping in Vietnam. Clean. Soil Air Water 39 (12), 1099–1104. doi:10.1002/clen.201100036
Antonini, S., Phong, T. N., Arnold, U., Eichert, T., and Clemens, J. (2012b). Solar thermal evaporation of human urine for nitrogen and phosphorus recovery in Vietnam. Sci. Total Environ. 414, 592–599. doi:10.1016/j.scitotenv.2011.11.055
Badeti, U., Jiang, J., Almuntashiri, A., Pathak, N., Dorji, U., Volpin, F., et al. (2022). Impact of source-separation of urine on treatment capacity, process design, and capital expenditure of a decentralised wastewater treatment plant. Chemosphere 300, 134489. doi:10.1016/j.chemosphere.2022.134489
Bai, X., Li, Z., Zhang, Y., Ni, J., Wang, X., and Zhou, X. (2018). Recovery of ammonium in urine by biochar derived from faecal sludge and its application as soil conditioner. Waste Biomass Valor. 9 (9), 1619–1628. doi:10.1007/s12649-017-9906-0
Ban, Z., and Dave, G. (2004). Laboratory studies on recovery of N and P from human urine through struvite crystallisation and zeolite adsorption. Environ. Technol. 25 (1), 111–121. doi:10.1080/09593330409355443b
Barbosa, S. G., Peixoto, L., Soares, O. S. G. P., Pereira, M. F. R., Ter Heijne, A., Kuntke, P., et al. (2018). Influence of carbon anode properties on performance and microbiome of Microbial Electrolysis Cells operated on urine. Electrochimica Acta 267, 122–132. doi:10.1016/j.electacta.2018.02.083
Barbosa, S. G., Rodrigues, T., Peixoto, L., Kuntke, P., Alves, M. M., Pereira, M. A., et al. (2019). Anaerobic biological fermentation of urine as a strategy to enhance the performance of a microbial electrolysis cell (MEC). Renew. Energy 139, 936–943. doi:10.1016/j.renene.2019.02.120
Barzon, L., Pacenti, M., Franchin, E., Pagni, S., Martello, T., Cattai, M., et al. (2013). Excretion of West Nile virus in urine during acute infection. J. Infect. Dis. 208 (7), 1086–1092. doi:10.1093/infdis/jit290
Basakcilardan-Kabakci, S., Ipekoglu, A. N., and Talini, I. (2007). Recovery of ammonia from human urine by stripping and absorption. Environ. Eng. Sci. 24 (5), 615–624. doi:10.1089/ees.2006.0412
Baykal, B. B., Kocaturk, N. P., Allar, A. D., and Sari, B. (2009). The effect of initial loading on the removal of ammonium and potassium from source-separated human urine via clinoptilolite. Water Sci. Technol. 60 (10), 2515–2520. doi:10.2166/wst.2009.614
Behrendt, J., Arevalo, E., Gulyas, H., Niederste-Hollenberg, J., Niemiec, A., Zhou, J., et al. (2002). Production of value added products from separately collected urine. Water Sci. Technol. 46 (6-7), 341–346. doi:10.2166/wst.2002.0698
Beler-Baykal, B., Allar, A. D., and Bayram, S. (2011). Nitrogen recovery from source-separated human urine using clinoptilolite and preliminary results of its use as fertilizer. Water Sci. Technol. 63 (4), 811–817. doi:10.2166/wst.2011.324
Beler-Baykal, B., Bayram, S., Akkaymak, E., and Cinar, S. (2004). Removal of ammonium from human urine through ion exchange with clinoptilolite and its recovery for further reuse. Water Sci. Technol. 50 (6), 149–156. doi:10.2166/wst.2004.0371
Benami, M., Busgang, A., Gillor, O., and Gross, A. (2016). Quantification and risks associated with bacterial aerosols near domestic greywater-treatment systems. Sci. Total Environ. 562, 344–352. doi:10.1016/j.scitotenv.2016.03.200
Bercovici, M., Kaigala, G. V., Mach, K. E., Han, C. M., Liao, J. C., and Santiago, J. G. (2011). Rapid detection of urinary tract infections using isotachophoresis and molecular beacons. Anal. Chem. 83 (11), 4110–4117. doi:10.1021/ac200253x
Berger, J. R., Miller, C. S., Mootoor, Y., Avdiushko, S. A., Kryscio, R. J., and Zhu, H. (2006). JC virus detection in bodily fluids: Clues to transmission. Clin. Infect. Dis. 43 (1), e9–e12. doi:10.1086/504947
Bethune, D. N., Chu, A., and Ryan, M. C. (2014). Passive evaporation of source-separated urine from dry toilets: A lab study. J. Water Sanit. Hyg. De. 4 (4), 654–662. doi:10.2166/washdev.2014.058
Bethune, D. N., Chu, A., and Ryan, M. C. (2015). Passive evaporation of source-separated urine from dry toilets: Prototype design and field testing using municipal water. J. Water, Sanitation Hyg. Dev. 5 (3), 392–401. doi:10.2166/washdev.2015.158
Bethune, D. N., Chu, A., and Ryan, M. C. (2016). Passive evaporation of source-separated urine from dry toilets: UES optimization and dry product accumulation over time. J. Water Sanit. Hyg. De. 6 (1), 96–103. doi:10.2166/washdev.2016.103
Bhuiyan, M., Mavinic, D. S., and Koch, F. A. (2008). Thermal decomposition of struvite and its phase transition. Chemosphere 70 (8), 1347–1356. doi:10.1016/j.chemosphere.2007.09.056
Bischel, H. N., Caduff, L., Schindelholz, S., Kohn, T., and Julian, T. R. (2019). Health risks for sanitation service workers along a container-based urine collection system and resource recovery value chain. Environ. Sci. Technol. 53 (12), 7055–7067. doi:10.1021/acs.est.9b01092
Bischel, H. N., Duygan, B. D. O., Strande, L., Mcardell, C. S., Udert, K. M., and Kohn, T. (2015). Pathogens and pharmaceuticals in source-separated urine in eThekwini, South Africa. Water Res. 85, 57–65. doi:10.1016/j.watres.2015.08.022
Bischel, H. N., Schindelholz, S., Schoger, M., Decrey, L., Buckley, C. A., Udert, K. M., et al. (2016). Bacteria inactivation during the drying of struvite fertilizers produced from stored urine. Environ. Sci. Technol. 50 (23), 13013–13023. doi:10.1021/acs.est.6b03555
Boan, P., Hewison, C., Swaminathan, R., Irish, A., Warr, K., Sinniah, R., et al. (2016). Optimal use of plasma and urine BK viral loads for screening and predicting BK nephropathy. BMC Infect. Dis. 16, 342. doi:10.1186/s12879-016-1652-6
Boggs, B. K., King, R. L., and Botte, G. G. (2009). Urea electrolysis: Direct hydrogen production from urine. Chem. Commun. (32), 4859–4861. doi:10.1039/b905974a
Bonaldo, M. C., Ribeiro, I. P., Lima, N. S., Dos Santos, A. A., Menezes, L. S., da Cruz, S. O., et al. (2016). Isolation of infective Zika virus from urine and saliva of patients in Brazil. PLoS Negl. Trop. Dis. 10 (6), e0004816. doi:10.1371/journal.pntd.0004816
Brewster, T. E., Jermakka, J., Freguia, S., and Batstone, D. J. (2017). Modelling recovery of ammonium from urine by electro-concentration in a 3-chamber cell. Water Res. 124, 210–218. doi:10.1016/j.watres.2017.07.043
Buergmann, H., Jenni, S., Vazquez, F., and Udert, K. M. (2011). Regime shift and microbial dynamics in a sequencing batch reactor for nitrification and anammox treatment of urine. Appl. Environ. Microbiol. 77 (17), 5897–5907. doi:10.1128/AEM.02986-10
Bujoczek, G., Oleszkiewicz, J., Danesh, S., and Sparling, R. (2002). Co-processing of organic fraction of municipal solid waste and primary sludge--stabilization and disinfection. Environ. Technol. 23 (2), 227–241. doi:10.1080/09593332508618423
Chandran, A., Pradhan, S. K., and Heinonen-Tanski, H. (2009). Survival of enteric bacteria and coliphage MS2 in pure human urine. J. Appl. Microbiol. 107 (5), 1651–1657. doi:10.1111/j.1365-2672.2009.04353.x
Chen, J., Xu, Y., Yan, H., Zhu, Y., Wang, L., Zhang, Y., et al. (2018). Sensitive and rapid detection of pathogenic bacteria from urine samples using multiplex recombinase polymerase amplification. Lab. Chip 18 (16), 2441–2452. doi:10.1039/c8lc00399h
Chen, S., Yang, Y., Zheng, M., Cheng, X., Xu, K., and Dou, X. (2020). Thermal decomposition of struvite pellet by microwave radiation and recycling of its product to remove ammonium and phosphate from urine. Environ. Res. 188, 109774–109779. doi:10.1016/j.envres.2020.109774
Chen, X., Gao, Y., Hou, D., Ma, H., Lu, L., Sun, D., et al. (2017). The microbial electrochemical current accelerates urea hydrolysis for recovery of nutrients from source-separated urine. Environ. Sci. Technol. Lett. 4 (7), 305–310. doi:10.1021/acs.estlett.7b00168
Cheng, S., Long, J., Evans, B., Zhan, Z., Li, T., Chen, C., et al. (2022). Non-negligible greenhouse gas emissions from non-sewered sanitation systems: A meta-analysis. Environ. Res. 212, 113468. doi:10.1016/j.envres.2022.113468
Christiaens, M. E. R., Gildemyn, S., Matassa, S., Ysebaert, T., De Vrieze, J., and Rabaey, K. (2017). Electrochemical ammonia recovery from source-separated urine for microbial protein production. Environ. Sci. Technol. 51 (22), 13143–13150. doi:10.1021/acs.est.7b02819
Christiaens, M. E. R., Udert, K. M., Arends, J. B. A., Huysman, S., Vanhaecke, L., Mcadam, E., et al. (2019). Membrane stripping enables effective electrochemical ammonia recovery from urine while retaining microorganisms and micropollutants. Water Res. 150, 349–357. doi:10.1016/j.watres.2018.11.072
Chu, C., Ryberg, E. C., Loeb, S. K., Suh, M., and Kim, J. (2019). Water disinfection in rural areas demands unconventional solar technologies. Acc. Chem. Res. 52 (5), 1187–1195. doi:10.1021/acs.accounts.8b00578
Corman, V. M., Albarrak, A. M., Omrani, A. S., Albarrak, M. M., Farah, M. E., Almasri, M., et al. (2015). Viral shedding and antibody response in 37 patients with Middle East respiratory syndrome coronavirus infection. Clin. Infect. Dis. 62 (4), 477–483. doi:10.1093/cid/civ951
Cotillas, S., Lacasa, E., Saez, C., Canizares, P., and Rodrigo, M. A. (2018a). Disinfection of urine by conductive-diamond electrochemical oxidation. Appl. Catal. B Environ. 229, 63–70. doi:10.1016/j.apcatb.2018.02.013
Cotillas, S., Lacasa, E., Saez, C., Canizares, P., and Rodrigo, M. A. (2018b). Electrolytic and electro-irradiated technologies for the removal of chloramphenicol in synthetic urine with diamond anodes. Water Res. 128, 383–392. doi:10.1016/j.watres.2017.10.072
Courtney, C., and Randall, D. G. (2022). A hybrid nanofiltration and reverse osmosis process for urine treatment: Effect on urea recovery and purity. Water Res. 222, 118851. doi:10.1016/j.watres.2022.118851
Dbira, S., Bensalah, N., Ahmad, M. I., and Bedoui, A. (2019). Electrochemical oxidation/disinfection of urine wastewaters with different anode materials. Materials 12 (12548), 1254. doi:10.3390/ma12081254
Dbira, S., Bensalah, N., Bedoui, A., Caizares, P., and Rodrigo, M. A. (2015). Treatment of synthetic urine by electrochemical oxidation using conductive-diamond anodes. Environ. Sci. Pollut. Res. 22 (8), 6176–6184. doi:10.1007/s11356-014-3831-6
De Paepe, J., Lindeboom, R. E. F., Vanoppen, M., De Paepe, K., Demey, D., Coessens, W., et al. (2018). Refinery and concentration of nutrients from urine with electrodialysis enabled by upstream precipitation and nitrification. Water Res. 144, 76–86. doi:10.1016/j.watres.2018.07.016
Decrey, L., Kazama, S., Udert, K. M., and Kohn, T. (2015). Ammonia as an in situ sanitizer: Inactivation kinetics and mechanisms of the ssRNA virus MS2 by NH3. Environ. Sci. Technol. 49 (2), 1060–1067. doi:10.1021/es5044529
Decrey, L., and Kohn, T. (2017). Virus inactivation in stored human urine, sludge and animal manure under typical conditions of storage or mesophilic anaerobic digestion. Environ. Sci. Water Res. Technol. 3 (3), 492–501. doi:10.1039/c6ew00311g
Decrey, L., Udert, K. M., Tilley, E., Pecson, B. M., and Kohn, T. (2011). Fate of the pathogen indicators phage Phi X174 and Ascaris suum eggs during the production of struvite fertilizer from source-separated urine. Water Res. 45 (16), 4960–4972. doi:10.1016/j.watres.2011.06.042
Dong, Q., Nelson, D., Toh, E., Diao, L., Gao, X., Fortenberry, J., et al. (2011). The microbial communities in male first catch urine are highly similar to those in paired urethral swab specimens. Plos One 6 (5), e19709. doi:10.1371/journal.pone.0019709
Dox, K., Everaert, M., Merckx, R., and Smolders, E. (2019a). Optimization of phosphate recovery from urine by layered double hydroxides. Sci. Total Environ. 682, 437–446. doi:10.1016/j.scitotenv.2019.05.181
Dox, K., Martin, T., Houot, S., Merckx, R., and Smolders, E. (2022). Superior residual fertiliser value in soil with phosphorus recycled from urine in layered double hydroxides. Sci. Rep. 12, 8092. doi:10.1038/s41598-022-11892-4
Dox, K., Pareijn, R., Everaert, M., and Smolders, E. (2019b). Phosphorus recycling from urine using layered double hydroxides: A kinetic study. Appl. Clay Sci. 182, 105255. doi:10.1016/j.clay.2019.105255
Drosten, C., Seilmaier, M., Corman, V. M., Hartmann, W., Scheible, G., Sack, S., et al. (2013). Clinical features and virological analysis of a case of Middle East respiratory syndrome coronavirus infection. Lancet Infect. Dis. 13 (9), 745–751. doi:10.1016/s1473-3099(13)70154-3
Ducancelle, A., Legrand, M. C., Pivert, A., Veillon, P., Le Guillou-Guillemette, H., De Brux, M. A., et al. (2014). Interest of human papillomavirus DNA quantification and genotyping in paired cervical and urine samples to detect cervical lesions. Arch. Gynecol. Obstet. 290 (2), 299–308. doi:10.1007/s00404-014-3191-y
Dutta, S., and Vinnerås, B. (2016). Fertilizer from dried human urine added to ash and lime a potential product from eco-sanitation system. Water Sci. Technol. 74 (6), 1436–1445. doi:10.2166/wst.2016.324
Ek, M., Bergstrom, R., Bjurhem, J. E., Bjorlenius, B., and Hellstrom, D. (2006). Concentration of nutrients from urine and reject water from anaerobically digested sludge. Water Sci. Technol. 54 (11-12), 437–444. doi:10.2166/wst.2006.924
Eksi, F., Karsligil, T., Gundes, I., Saglam, M., Kirik, M. P., Budeyri, M. S., et al. (2018). Investigation of BK virus by real-time polymerase chain reaction in patients with allogeneic bone marrow transplantation. Transplant. Proc. 50 (5), 1510–1513. doi:10.1016/j.transproceed.2017.12.056
El Ansary, M., Abd Elhamid, S., Saadi, G., Ismail, W., Ibrahim, N., Bahaa El-Din, N., et al. (2016). Prevalence of polyoma BK virus infection among living-donor renal transplant recipients. Transpl. Infect. Dis. 18 (4), 529–537. doi:10.1111/tid.12557
Engelhardt, S., Vogel, J., Duirk, S. E., Moore, F. B., and Barton, H. A. (2020). Assessment of urea hydrolysis as a pretreatment strategy to improve total nitrogen rejection from urine using aquaporin-based membranes in forward osmosis. J. Water Process Eng. 34, 101135. doi:10.1016/j.jwpe.2020.101135
Etter, B., Tilley, E., Khadka, R., and Udert, K. M. (2011). Low-cost struvite production using source-separated urine in Nepal. Water Res. 45 (2), 852–862. doi:10.1016/j.watres.2010.10.007
Feng, D. L., Wu, Z. C., and Xu, S. H. (2008). Nitrification of human urine for its stabilization and nutrient recycling. Bioresour. Technol. 99 (14), 6299–6304. doi:10.1016/j.biortech.2007.12.007
Ferronato, C., Vianello, G., and Antisari, L. V. (2015). Adsorption of pathogenic microorganisms, NH4+ and heavy metals from wastewater by clinoptilolite using bed laminar flow. Clay Min. 50 (1), 1–10. doi:10.1180/claymin.2015.050.1.01
Fumasoli, A., Etter, B., Sterkele, B., Morgenroth, E., and Udert, K. M. (2016). Operating a pilot-scale nitrification/distillation plant for complete nutrient recovery from urine. Water Sci. Technol. 73 (1), 215–222. doi:10.2166/wst.2015.485
Gajda, I., Greenman, J., Santoro, C., Serov, A., Atanassov, P., Melhuish, C., et al. (2019). Multi-functional microbial fuel cells for power, treatment and electro-osmotic purification of urine. J. Chem. Technol. Biotechnol. 94 (7), 2098–2106. doi:10.1002/jctb.5792
Ganrot, Z., Dave, G., Nilsson, E., and Li, B. (2007b). Plant availability of nutrients recovered as solids from human urine tested in climate chamber on Triticum aestivum L. Bioresour. Technol. 98 (16), 3122–3129. doi:10.1016/j.biortech.2007.01.003
Ganrot, Z., Dave, G., and Nilsson, E. (2007a). Recovery of N and P from human urine by freezing, struvite precipitation and adsorption to zeolite and active carbon. Bioresour. Technol. 98 (16), 3112–3121. doi:10.1016/j.biortech.2006.10.038
Ganrot, Z., Slivka, A., and Dave, G. (2008). Nutrient recovery from human urine using pretreated zeolite and struvite precipitation in combination with freezing-thawing and plant availability tests on common wheat. Clean. Soil Air Water 36 (1), 45–52. doi:10.1002/clen.200700074
Goetsch, H. E., Zhao, L., Gnegy, M., Imperiale, M. J., Love, N. G., and Wigginton, K. R. (2018). Fate of the urinary tract virus BK Human Polyomavirus in source-separated urine. Appl. Environ. Microbiol. 84 (7), e02374-17. doi:10.1128/AEM.02374-17
Golder, D., Rana, S., Sarkar, D., and Jana, B. B. (2007). Human urine is an excellent liquid waste for the culture of fish food organism, Moina micrura. Ecol. Eng. 30 (4), 326–332. doi:10.1016/j.ecoleng.2007.04.002
Goldsmith, C. S., and Miller, S. E. (2009). Modern uses of electron microscopy for detection of viruses. Clin. Microbiol. Rev. 22 (4), 552–563. doi:10.1128/CMR.00027-09
Goswami, K. P., and Pugazhenthi, G. (2020). Credibility of polymeric and ceramic membrane filtration in the removal of bacteria and virus from water: A review. J. Environ. Manag. 268, 110583. doi:10.1016/j.jenvman.2020.110583
Guan, T., Kuang, Y., Li, X., Fang, J., Fang, W., and Wu, D. (2020a). The recovery of phosphorus from source-separated urine by repeatedly usable magnetic Fe3O4@ZrO2 nanoparticles under acidic conditions. Environ. Int. 134, 105322. doi:10.1016/j.envint.2019.105322
Guan, T., Li, X., Fang, W., and Wu, D. (2020b). Efficient removal of phosphate from acidified urine using UiO-66 metal-organic frameworks with varying functional groups. Appl. Surf. Sci. 501, 144074. doi:10.1016/j.apsusc.2019.144074
Gujer, W. (2010). Nitrification and me – a subjective review. Water Res. 44 (1), 1–19. doi:10.1016/j.watres.2009.08.038
Gulyas, H., Bruhn, P., Furmanska, M., Hartrampf, K., Kot, K., Luttenberg, B., et al. (2004). Freeze concentration for enrichment of nutrients in yellow water from no-mix toilets. Water Sci. Technol. 50 (6), 61–68. doi:10.2166/wst.2004.0360
Guzha, E., Nhapi, I., and Rockstrom, J. (2005). An assessment of the effect of human faeces and urine on maize production and water productivity. Phys. Chem. Earth Parts A/B/C 30 (11-16), 840–845. doi:10.1016/j.pce.2005.08.028
Han, C., Yuan, X., Ma, S., Li, Y., Feng, Y., and Liu, J. (2022). Simultaneous recovery of nutrients and power generation from source-separated urine based on bioelectrical coupling with the hydrophobic gas permeable tube system. Sci. Total Environ. 824, 153788. doi:10.1016/j.scitotenv.2022.153788
Han, M. S., Seong, M. W., Kim, N., Shin, S., Im Cho, S., Park, H., et al. (2020). Virological assessment of hospitalized patients with COVID-2019. Nature 26 (10), 465–469. doi:10.1038/s41586-020-2196-x
Hanaoka, N., Ito, S., Konagaya, M., Nojiri, N., Yasuda, M., Fujimoto, T., et al. (2019). Infectious human adenoviruses are shed in urine even after disappearance of urethral symptoms. PLoS One 14 (3), e0212434. doi:10.1371/journal.pone.0212434
Hansen, J. J., Warden, P. S., and Margolin, A. B. (2007). Inactivation of adenovirus type 5, rotavirus Wa and male specific coliphage (MS2) in biosolids by lime stabilization. Int. J. Env. Res. Pub. He. 4 (1), 61–67. doi:10.3390/ijerph2007010010
Harada, H., Shimizu, Y., Miyagoshi, Y., Matsui, S., Matsuda, T., and Nagasaka, T. (2006). Predicting struvite formation for phosphorus recovery from human urine using an equilibrium model. Water Sci. Technol. 54 (8), 247–255. doi:10.2166/wst.2006.720
Harder, R., Wielemaker, R., Larsen, T. A., Zeeman, G., and Oberg, G. (2019). Recycling nutrients contained in human excreta to agriculture: Pathways, processes, and products. Crit. Rev. Environ. Sci. Technol. 49 (8), 695–743. doi:10.1080/10643389.2018.1558889
Heinonen-Tanski, H., Sjoblom, A., Fabritius, H., and Karinen, P. (2007). Pure human urine is a good fertiliser for cucumbers. Bioresour. Technol. 98 (1), 214–217. doi:10.1016/j.biortech.2005.11.024
Hellstrom, D., Johannson, E., and Grennberg, K. (1999). Storage of human urine: Acidification as a method to inhibit decomposition of urea. Ecol. Eng. 12 (3-4), 253–269. doi:10.1016/S0925-8574(98)00074-3
Hilt, E. E., Mckinley, K., Pearce, M. M., Rosenfeld, A. B., Zilliox, M. J., Mueller, E. R., et al. (2014). Urine is not sterile: Use of enhanced urine culture techniques to detect resident bacterial flora in the adult female bladder. J. Clin. Microbiol. 52 (3), 871–876. doi:10.1128/JCM.02876-13
Hinata, N., Shirakawa, T., Okada, H., Shigemura, K., Kamidono, S., and Gotoh, A. (2004). Quantitative detection of Escherichia coli from urine of patients with bacteriuria by real-time PCR. Mol. Diagn. 8 (3), 179–184. doi:10.1007/BF03260062
Höglund, C., Ashbolt, N., Stenström, T. A., and Svensson, L. (2002). Viral persistence in source-separated human urine. Adv. Environ. Res. 6 (3), 265–275. doi:10.1016/S1093-0191(01)00057-0
Höglund, C. (2001). Evaluation of microbial health risks associated with the reuse of source-separated human urine. Stockholm: Royal Institute of Technology.
Hoglund, C., Stenstrom, T. A., and Ashbolt, N. (2002). Microbial risk assessment of source-separated urine used in agriculture. Waste Manag. Res. 20 (2), 150–161. doi:10.1177/0734242X0202000207
Höglund, C., Stenström, T. A., Jönsson, H., and Sundin, A. (1998). Evaluation of faecal contamination and microbial die-off in urine separating sewage systems. Water Sci. Technol. 38 (6), 17–25. doi:10.2166/wst.1998.0232
Höglund, C., and Stenström, T. A. (1999). Survival of <i>Cryptosporidium parvum</i> oocysts in source separated human urine. Rev. Can. Microbiol. 45 (9), 740–746. doi:10.1139/cjm-45-9-740
Höglund, C., Vinnerås, B., Stenström, T. A., and Jönsson, H. (2000). Variation of chemical and microbial parameters in collection and storage tanks for source separated human urine. J. Environ. Sci. Health Part A 35 (8), 1463–1475. doi:10.1080/10934520009377047
Höglund, C., Vinnerås, B., Stenström, T. A., and Jönsson, H. (2000). Variation of chemical and microbial parameters in collection and storage tanks for source separated human urine. J. Environ. Sci. Health Part A 35 (8), 1463–1475. doi:10.1080/10934520009377047
Hotta, S., and Funamizu, N. (2008). Evolution of ammonification potential in storage process of urine with fecal contamination. Bioresour. Technol. 99 (1), 13–17. doi:10.1016/j.biortech.2006.12.001
Hu, X., Han, M., Wang, C., Yang, N., Wang, Y., Duan, E., et al. (2020). A short review of bioaerosol emissions from gas bioreactors: Health threats, influencing factors and control technologies. Chemosphere 253, 126737. doi:10.1016/j.chemosphere.2020.126737
Huang, H., Li, J., Li, B., Zhang, D., Zhao, N., and Tang, S. (2019). Comparison of different K-struvite crystallization processes for simultaneous potassium and phosphate recovery from source-separated urine. Sci. Total Environ. 651 (1), 787–795. doi:10.1016/j.scitotenv.2018.09.232
Huang, H., Liu, J., Xu, C., and Gao, F. (2016). Recycling struvite pyrolysate obtained at negative pressure for ammonia nitrogen removal from landfill leachate. Chem. Eng. J. 284, 1204–1211. doi:10.1016/j.cej.2015.09.080
Huang, H., Xiao, D., Pang, R., Han, C., and Ding, L. (2014). Simultaneous removal of nutrients from simulated swine wastewater by adsorption of modified zeolite combined with struvite crystallization. Chem. Eng. J. 256, 431–438. doi:10.1016/j.cej.2014.07.023
Huang, H., Zhang, P., Xiao, J., Xiao, D., and Gao, F. (2017). Repeatedly using the decomposition product of struvite by ultrasound stripping to remove ammonia nitrogen from landfill leachate. Ultrason. Sonochemistry 38, 622–628. doi:10.1016/j.ultsonch.2016.08.019
Hung, I. F. N., Cheng, V. C. C., Wu, A. K. L., Tang, B. S. F., Chan, K. H., Chu, C. M., et al. (2004). Viral loads in clinical specimens and SARS manifestations. Emerg. Infect. Dis. 10 (9), 1550–1557. doi:10.3201/eid1009.040058
Iseri, E., Biggel, M., Goossens, H., Moons, P., and van der Wijngaart, W. (2020). Digital dipstick: Miniaturized bacteria detection and digital quantification for the point-of-care. Lab. Chip 20 (23), 4349–4356. doi:10.1039/d0lc00793e
Ivanković, T., Hrenović, J., Itskos, G., Koukouzas, N., Kovačević, D., and Milenković, J. (2014). Alkaline disinfection of urban wastewater and landfill leachate by wood fly ash. Arh. za Hig. rada i Toksikol. 65 (4), 365–375. doi:10.2478/10004-1254-65-2014-2546
Jagtap, N., and Boyer, T. H. (2018). Integrated, multi-process approach to total nutrient recovery from stored urine. Environ. Sci. Water Res. Technol. 4 (10), 1639–1650. doi:10.1039/c8ew00004b
Jagtap, N. S., and Boyer, T. H. (2020). Urine collection in a multi-story building and opportunities for onsite recovery of nutrients and non-potable water. J. Environ. Chem. Eng. 8, 103964. doi:10.1016/j.jece.2020.103964
Jain, S., Su, Y. H., Su, Y. P., McCloud, S., Xue, R., Lee, T. J., et al. (2018). Characterization of the Hepatitis B virus DNA detected in urine of chronic Hepatitis B patients. BMC Gastroenterol. 18 (1), 40. doi:10.1186/s12876-018-0767-1
Jeong, H. W., Kim, S. M., Kim, H. S., Kim, Y. I., Kim, J. H., Cho, J. Y., et al. (2020). Viable SARS-CoV-2 in various specimens from COVID-19 patients. Clin. Microbiol. Infect. 26 (11), 1520–1524. doi:10.1016/j.cmi.2020.07.020
Jiang, F., Chen, Y., Mackey, H. R., Chen, G. H., and van Loosdrecht, M. C. M. (2011). Urine nitrification and sewer discharge to realize in-sewer denitrification to simplify sewage treatment in Hong Kong. Water Sci. Technol. 64 (3), 618–626. doi:10.2166/wst.2011.491
Jiang, G., and Yuan, Z. (2014). Inactivation kinetics of anaerobic wastewater biofilms by free nitrous acid. Appl. Microbiol. Biotechnol. 98 (3), 1367–1376. doi:10.1007/s00253-013-5031-6
Jiang, Q., Liu, J., Song, X., Qiu, Y., Xue, J., Shao, Y., et al. (2022). Energy efficient bioelectro-concentration and recovery system of nutrients from human urine by integrating forward osmosis. Resour. Conservation Recycl. 181, 106253. doi:10.1016/j.resconrec.2022.106253
Jiang, S., Wang, X., Yang, S., and Shi, H. (2016). Characteristics of simultaneous ammonium and phosphate adsorption from hydrolysis urine onto natural loess. Environ. Sci. Pollut. Res. 23 (3), 2628–2639. doi:10.1007/s11356-015-5443-1
Jiang, S., Wang, X., Yang, S., and Shi, H. (2017). Effect of initial pH and pH-adjusted acid on nutrient recovery from hydrolysis urine by combining acidification with evaporation-crystallization. Environ. Sci. Pollut. Res. 24 (4), 3872–3881. doi:10.1007/s11356-016-8052-8
Jin, Y., Shi, Y., Chen, Z., Chen, R., Chen, X., Zheng, X., et al. (2020). Combination of sunlight with hydrogen peroxide generated at a modified reticulated vitreous carbon for drinking water disinfection. J. Clean. Prod. 252, 119794. doi:10.1016/j.jclepro.2019.119794
Jones, D. L., Baluja, M. Q., Graham, D. W., Corbishley, A., McDonald, J. E., Malham, S. K., et al. (2020). Shedding of SARS-CoV-2 in feces and urine and its potential role in person-to-person transmission and the environment-based spread of COVID-19. Sci. Total Environ. 749, 141364. doi:10.1016/j.scitotenv.2020.141364
Jönsson, H., Richert Stinzing, A., Vinnerås, B., and Salomon, E. (2004). “Guidelines on the use of urine and faeces in crop production,” in EcoSanRes publications. (Stockholm: EcoSanRes Publications).
Judice, C. C., Tan, J. J. L., Parise, P. L., Kam, Y. W., Milanez, G. P., Leite, J. A., et al. (2018). Efficient detection of Zika virus RNA in patients' blood from the 2016 outbreak in Campinas, Brazil. Sci. Rep. 8 (1), 4012. doi:10.1038/s41598-018-22159-2
Kabdasli, I., Tunay, O., Islek, C., Erdinc, E., Huskalar, S., and Tatli, M. B. (2006). Nitrogen recovery by urea hydrolysis and struvite precipitation from anthropogenic urine. Water Sci. Technol. 53 (12), 305–312. doi:10.2166/wst.2006.433
Kashi, A. H., De la Rosette, J., Amini, E., Abdi, H., Fallah-Karkan, M., and Vaezjalali, M. (2020). Urinary viral shedding of COVID-19 and its clinical associations: A systematic review and meta-analysis of observational studies. medRxiv. doi:10.1101/2020.05.15.20094920
Keller, R., Passamani-Franca, R. F., Cassin, S. T., and Goncalves, F. R. (2004). Disinfection of sludge using lime stabilisation and pasteurisation in a small wastewater treatment plant. Water Sci. Technol. 50 (1), 13–17. doi:10.2166/wst.2004.0005
Khumalo, N., Nthunya, L., Derese, S., Motsa, M., Verliefde, A., Kuvarega, A., et al. (2019). Water recovery from hydrolysed human urine samples via direct contact membrane distillation using PVDF/PTFE membrane. Sep. Purif. Technol. 211, 610–617. doi:10.1016/j.seppur.2018.10.035
Kim, I. S., Choi, Y. W., and Lee, S. R. (2004). Optimization and validation of a virus filtration process for efficient removal of viruses from urokinase solution prepared from human urine. J. Microbiol. Biotechn. 14 (1), 140–147.
Kim, J., Kim, H. M., Lee, E. J., Jo, H. J., Yoon, Y., Lee, N., et al. (2020). Detection and isolation of SARS-CoV-2 in serum, urine, and stool specimens of COVID-19 patients from the Republic of Korea. Osong Public Health Res. Perspect. 11 (3), 112–117. doi:10.24171/j.phrp.2020.11.3.02
Kim, J. M., Kim, H. M., Lee, E. J., Jo, H. J., Yoon, Y., Lee, N. J., et al. (2020). Detection and isolation of SARS-CoV-2 in serum, urine, and stool specimens of COVID-19 patients from the Republic of Korea. Osong Public Health Res. Perspect. 11 (3), 112–117. doi:10.24171/j.phrp.2020.11.3.02
Konietzny, R., Fischer, R., Ternette, N., Wright, C. A., Turney, B. W., Chakera, A., et al. (2012). Detection of BK virus in urine from renal transplant subjects by mass spectrometry. Clin. Proteom. 9 (1), 4. doi:10.1186/1559-0275-9-4
Kononenko, N., Nikonenko, V., Grande, D., Larchet, C., Dammak, L., Fomenko, M., et al. (2017). Porous structure of ion exchange membranes investigated by various techniques. Adv. Colloid Interface Sci. 246, 196–216. doi:10.1016/j.cis.2017.05.007
Krähenbühl, M., Etter, B., and Udert, K. M. (2016). Pretreated magnesite as a source of low-cost magnesium for producing struvite from urine in Nepal. Sci. Total Environ. 542, 1155–1161. doi:10.1016/j.scitotenv.2015.08.060
Lahr, R. H., Goetsch, H. E., Haig, S. J., Noe-Hays, A., Love, N. G., Aga, D. S., et al. (2016). Urine bacterial community convergence through fertilizer production: Storage, pasteurization, and struvite precipitation. Environ. Sci. Technol. 50 (21), 11619–11626. doi:10.1021/acs.est.6b02094
Lameiras, S., Quintelas, C., and Tavares, T. (2008). Biosorption of Cr (VI) using a bacterial biofilm supported on granular activated carbon and on zeolite. Bioresour. Technol. 99, 801–806. doi:10.1016/j.biortech.2007.01.040
Larsen, T. A., and Gujer, W. (1996). Separate management of anthropogenic nutrient solutions (human urine). Water Sci. Technol. 34 (3-4), 87–94. doi:10.2166/wst.1996.0420
Larsen, T. A., Hoffmann, S., Luthi, C., Truffer, B., and Maurer, M. (2016). Emerging solutions to the water challenges of an urbanizing world. Science 352 (6288), 928–933. doi:10.1126/science.aad8641
Le Corre, K. S., Valsami-Jones, E., Hobbs, P., Jefferson, B., and Parsons, S. A. (2007). Agglomeration of struvite crystals. Water Res. 41 (2), 419–425. doi:10.1016/j.watres.2006.10.025
Leung, S., Barrington, S., Wan, Y., Zhao, X., and El-Husseini, B. (2007). Zeolite (clinoptilolite) as feed additive to reduce manure mineral content. Bioresour. Technol. 98 (17), 3309–3316. doi:10.1016/j.biortech.2006.07.010
Li, J., Xu, K., Liu, T., Bai, G., Liu, Y., Wang, C., et al. (2020). Achieving stable partial nitritation in an acidic nitrifying bioreactor. Environ. Sci. Technol. 54, 456–463. doi:10.1021/acs.est.9b04400
Li, M., Wang, B., Li, L., Wong, G., Liu, Y., Ma, J., et al. (2019a). Rift Valley fever virus and yellow fever virus in urine: A potential source of infection. Virol. Sin. 34 (3), 342–345. doi:10.1007/s12250-019-00096-2
Li, P., Chen, L., Ding, Y., Tian, X., Guan, D., Zhang, Z., et al. (2019b). Phosphorus recovery from urine using cooling water system effluent as a precipitant. J. Environ. Manag. 244, 391–398. doi:10.1016/j.jenvman.2019.05.057
Li, Z., Zhang, R., and Sun, P. (2022). Simultaneous removal of phosphate and antibiotic from hydrolyzed urine by novel spherical particles. Chemosphere 300, 134637. doi:10.1016/j.chemosphere.2022.134637
Liao, J. C., Mastali, M., Gau, V., Suchard, M. A., Moller, A. K., Bruckner, D. A., et al. (2006). Use of electrochemical DNA biosensors for rapid molecular identification of uropathogens in clinical urine specimens. J. Clin. Microbiol. 44 (2), 561–570. doi:10.1128/JCM.44.2.561-570.2006
Lin, K. A., Chen, S., and Jochems, A. P. (2015). Zirconium-based metal organic frameworks: Highly selective adsorbents for removal of phosphate from water and urine. Mater. Chem. Phys. 160, 168–176. doi:10.1016/j.matchemphys.2015.04.021
Lind, B. B., Ban, Z., and Byden, S. (2000). Nutrient recovery from human urine by struvite crystallization with ammonia adsorption on zeolite and wollastonite. Bioresour. Technol. 73 (2), 169–174. doi:10.1016/S0960-8524(99)90157-8
Lind, B. B., Ban, Z., and Byden, S. (2001). Volume reduction and concentration of nutrients in human urine. Ecol. Eng. 16 (4), 561–566. doi:10.1016/S0925-8574(00)00107-5
Liu, B., Giannis, A., Zhang, J., Chang, V. W. C., and Wang, J. (2015). Air stripping process for ammonia recovery from source-separated urine: Modeling and optimization. J. Chem. Technol. Biotechnol. 90 (12), 2208–2217. doi:10.1002/jctb.4535
Liu, J., Zheng, M., Wang, C., Liang, C., Shen, Z., and Xu, K. (2020). A green method for the simultaneous recovery of phosphate and potassium from hydrolyzed urine as value-added fertilizer using wood waste. Resour. Conservation Recycl. 157, 104793. doi:10.1016/j.resconrec.2020.104793
Liu, X., Hu, Z., Zhu, C., Wen, G., Meng, X., and Lu, J. (2013). Influence of process parameters on phosphorus recovery by struvite formation from urine. Water Sci. Technol. 68 (11), 2434–2440. doi:10.2166/wst.2013.514
Liu, Y., He, L., Deng, Y., Zhang, Q., Jiang, G., and Liu, H. (2022). Recent progress on the recovery of valuable resources from source-separated urine on-site using electrochemical technologies: A review. Chem. Eng. J. 442, 136200. doi:10.1016/j.cej.2022.136200
Liu, Z. G., Zhao, Q. L., Wang, K., Lee, D. J., Qiu, W., and Wang, J. F. (2008). Urea hydrolysis and recovery of nitrogen and phosphorous as MAP from stale human urine. J. Environ. Sci. 20 (8), 1018–1024. doi:10.1016/S1001-0742(08)62202-0
Lv, Y., Li, Z., Zhou, X., Cheng, S., and Zheng, L. (2020). Stabilization of source-separated urine by heat-activated peroxydisulfate. Sci. Total Environ. 749, 142213. doi:10.1016/j.scitotenv.2020.142213
Madden, K., Janitell, C., Sower, D., and Yang, S. (2018). Prediction of BK viremia by urine viral load in renal transplant patients: An analysis of BK viral load results in paired urine and plasma samples. Transpl. Infect. Dis. 20 (5), e12952. doi:10.1111/tid.12952
Makgabutlane, B., Nthunya, L. N., Musyoka, N., Dladla, B. S., Nxumalo, E. N., and Mhlanga, S. D. (2020). Microwave-assisted synthesis of coal fly ash-based zeolites for removal of ammonium from urine. RSC Adv. 10 (4), 2416–2427. doi:10.1039/C9RA10114D
Michen, B., and Graule, T. (2010). Isoelectric points of viruses. J. Appl. Microbiol. 109 (2), 388–397. doi:10.1111/j.1365-2672.2010.04663.x
Ministry of Industry and Information Technology of the People's Republic of China (2019). Limitation requirements of toxic and harmful substance in fertilizers. GB 38400-2019).
Mitrogiannis, D., Psychoyou, M., Koukouzas, N., Tsoukalas, N., Palles, D., Kamitsos, E., et al. (2018). Phosphate recovery from real fresh urine by Ca(OH)(2) treated natural zeolite. Chem. Eng. J. 347, 618–630. doi:10.1016/j.cej.2018.04.102
Mnkeni, P. N. S., Kutu, F. R., Muchaonyerwa, P., and Austin, L. M. (2008). Evaluation of human urine as a source of nutrients for selected vegetables and maize under tunnel house conditions in the Eastern Cape, South Africa. Waste Manag. Res. 26 (2), 132–139. doi:10.1177/0734242x07079179
Moharramzadeh, S., Ong, S. K., Alleman, J., and Cetin, K. S. (2022). Stabilization and concentration of nitrogen in synthetic urine with peracetic acid and progressive freeze concentration. J. Environ. Chem. Eng. 10, 107768. doi:10.1016/j.jece.2022.107768
Morales, N., Boehler, M. A., Buettner, S., Liebi, C., and Siegrist, H. (2013). Recovery of N and P from urine by struvite precipitation followed by combined stripping with digester sludge liquid at full scale. Water 5 (3), 1262–1278. doi:10.3390/w5031262
Muhmood, A., Lu, J., Kadam, R., Dong, R., Guo, J., and Wu, S. (2019). Biochar seeding promotes struvite formation, but accelerates heavy metal accumulation. Sci. Total Environ. 652, 623–632. doi:10.1016/j.scitotenv.2018.10.302
Muhmood, A., Wu, S., Lu, J., Ajmal, Z., Luo, H., and Dong, R. (2018). Nutrient recovery from anaerobically digested chicken slurry via struvite: Performance optimization and interactions with heavy metals and pathogens. Sci. Total Environ. 635, 1–9. doi:10.1016/j.scitotenv.2018.04.129
Musso, D., Teissier, A., Rouault, E., Teururai, S., de Pina, J. J., and Nhan, T. X. (2016). Detection of chikungunya virus in saliva and urine. Virol. J. 13, 102. doi:10.1186/s12985-016-0556-9
Nomoto, H., Ishikane, M., Katagiri, D., Kinoshita, N., Nagashima, M., Sadamasu, K., et al. (2020). Handheld ultrasound devices: An emerging technology to reduce viral spread during the Covid-19 pandemic. Am. J. Infect. Control 48 (8), 968–969. doi:10.1016/j.ajic.2020.05.041
Nordin, A., Niwagaba, C., Jonsson, H., and Vinnerås, B. (2013). Pathogen and indicator inactivation in source-separated human urine heated by the sun. J. Water Sanit. Hyg. De. 3 (2), 181–188. doi:10.2166/washdev.2013.174
Nordin, A., Nyberg, K., and Vinnerås, B. (2009). Inactivation of Ascaris eggs in source-separated urine and feces by ammonia at ambient temperatures. Appl. Environ. Microbiol. 75 (3), 662–667. doi:10.1128/AEM.01250-08
Novazzi, F., Cassaniti, I., Piralla, A., Di Sabatino, A., Bruno, R., Baldanti, F., et al. (2020). Detection of the SARS-CoV-2 in different biologic specimens from positive patients with COVID-19, in Northern Italy. Pediatr. Allergy Immunol. 31 (S26), 72–74. doi:10.1111/pai.13366
O'Neal, J. A., and Boyer, T. H. (2013). Phosphate recovery using hybrid anion exchange: Applications to source-separated urine and combined wastewater streams. Water Res. 47 (14), 5003–5017. doi:10.1016/j.watres.2013.05.037
O'Neal, J. A., and Boyer, T. H. (2015). Phosphorus recovery from urine and anaerobic digester filtrate: Comparison of adsorption–precipitation with direct precipitation. Environ. Sci. Water Res. Technol. 1 (4), 481–492. doi:10.1039/C5EW00009B
Oishi, W., Kato, I., Hijikata, N., Ushijima, K., Ito, R., Funamizu, N., et al. (2020). Inactivation kinetics modeling of Escherichia coli in concentrated urine for implementing predictive environmental microbiology in sanitation safety planning. J. Environ. Manag. 268, 110672. doi:10.1016/j.jenvman.2020.110672
Olanrewaju, A. O., Ng, A., DeCorwin-Martin, P., Robillard, A., and Juncker, D. (2017). Microfluidic capillaric circuit for rapid and facile bacteria detection. Anal. Chem. 89 (12), 6846–6853. doi:10.1021/acs.analchem.7b01315
Oztekin, E., Colak, S., and Inan, H. (2022). Comparison of classic and chemometric methods used for phosphate removal from fresh human urine under optimum conditions. Water sa. 48 (3), 286–294. doi:10.17159/wsa/2022.v48.i3.3933
Pang, X. L., Martin, K., and Preiksaitis, J. K. (2008). The use of unprocessed urine samples for detecting and monitoring BK viruses in renal transplant recipients by a quantitative real-time PCR assay. J. Virological Methods 149 (1), 118–122. doi:10.1016/j.jviromet.2007.12.015
Patel, A., Mungray, A. A., and Mungray, A. K. (2020). Technologies for the recovery of nutrients, water and energy from human urine: A review. Chemosphere 259, 127372. doi:10.1016/j.chemosphere.2020.127372
Perez-Mercado, L. F., Lalander, C., Joel, A., Ottoson, J., Dalahmeh, S., and Vinnerås, B. (2019). Biochar filters as an on-farm treatment to reduce pathogens when irrigating with wastewater-polluted sources. J. Environ. Manag. 248, 109295. doi:10.1016/j.jenvman.2019.109295
Perry, S. F. (1998). Freeze-drying and cryopreservation of bacteria. Mol. Biotechnol. 9, 59–64. doi:10.1007/BF02752697
Pinatha, Y., Polprasert, C., and Englande, (2020). Product and cost perspectives of phosphorus recovery from human urine using solid waste ash and sea salt addition - a case of Thailand. Sci. Total Environ. 713 (136514), 136514. doi:10.1016/j.scitotenv.2020.136514
Pocock, J., Muzhingi, A., Mercer, E., Velkushnova, K., Septien, S., and Buckley, C. A. (2022). Water and nutrient recovery from stored urine by forward osmosis with an ammonium bicarbonate draw solution. Front. Environ. Sci. 10, 937456. doi:10.3389/fenvs.2022.937456
Pradhan, S. K., Holopainen, J. K., and Heinonen-Tanski, H. (2009). Stored human urine supplemented with wood ash as fertilizer in tomato (Solanum lycopersicum) cultivation and its impacts on fruit yield and quality. J. Agric. Food Chem. 57 (16), 7612–7617. doi:10.1021/jf9018917
Pradhan, S. K., Holopainen, J. K., Weisell, J., and Heinonen-Tanski, H. (2010a). Human urine and wood ash as plant nutrients for red beet (Beta vulgaris) cultivation: Impacts on yield quality. J. Agric. Food Chem. 58 (3), 2034–2039. doi:10.1021/jf9029157
Pradhan, S. K., Mikola, A., Heinonen-Tanski, H., and Vahala, R. (2019). Recovery of nitrogen and phosphorus from human urine using membrane and precipitation process. J. Environ. Manag. 247, 596–602. doi:10.1016/j.jenvman.2019.06.046
Pradhan, S. K., Mikola, A., and Vahala, R. (2017). Nitrogen and phosphorus harvesting from human urine using a stripping, absorption, and precipitation Process. Environ. Sci. Technol. 51 (9), 5165–5171. doi:10.1021/acs.est.6b05402
Pradhan, S. K., Nerg, A., Sjoeblom, A., Holopainen, J. K., and Heinonen-Tanski, H. (2007). Use of human urine fertilizer in cultivation of cabbage (Brassica oleracea)- Impacts on chemical, microbial, and flavor quality. J. Agric. Food Chem. 55 (21), 8657–8663. doi:10.1021/JF0717891
Pradhan, S. K., Pitkanen, S., and Heinonen-Tanski, H. (2010b). Fertilizer value of urine in pumpkin (Cucurbita maxima L.) cultivation. AFSci. 19 (1), 57–67. doi:10.2137/145960610791015032
Pronk, W., Biebow, M., and Boller, M. (2006). Electrodialysis for recovering salts from a urine solution containing micropollutants. Environ. Sci. Technol. 40 (7), 2414–2420. doi:10.1021/es051921i
Prusty, B. K., Kumar, A., Arora, R., Batra, S., and Das, B. C. (2005). Human papillomavirus (HPV) DNA detection in self-collected urine. Int. J. Gynecol. Obstetrics 90 (3), 223–227. doi:10.1016/j.ijgo.2005.06.004
Qiao, L., Chen, S., Yang, Y., Zhang, K., Zheng, B., Guo, H., et al. (2013). Characteristics of urinary tract infection pathogens and their in vitro susceptibility to antimicrobial agents in China: Data from a multicenter study. Bmj Open 3 (12), e004152. doi:10.1136/bmjopen-2013-004152
Qiao, L. D., Chen, S., Yang, Y., Zhang, K., Zheng, B., Guo, H. F., et al. (2013). Characteristics of urinary tract infection pathogens and their in vitro susceptibility to antimicrobial agents in China: Data from a multicenter study. BMJ Open 3 (12), e004152. doi:10.1136/bmjopen-2013-004152
Rahbar, M., Amiri, M., Poormand, G., Poortahmasebi, V., Karkhaneh, M. M., Jazayeri, A., et al. (2019). Simultaneous detection of opportunistic viral infections among renal transplant patients from Sina Hospital, Tehran. Future Virol. 14 (6), 419–426. doi:10.2217/fvl-2018-0192
Randall, D. G., Krahenbuhl, M., Kopping, I., Larsen, T. A., and Udert, K. M. (2016). A novel approach for stabilizing fresh urine by calcium hydroxide addition. Water Res. 95, 361–369. doi:10.1016/j.watres.2016.03.007
Randall, D. G., and Nathoo, J. (2018). Resource recovery by freezing: A thermodynamic comparison between a reverse osmosis brine, seawater and stored urine. J. Water Process Eng. 26, 242–249. doi:10.1016/j.jwpe.2018.10.020
Ray, H., Perreault, F., and Boyer, T. H. (2020b). Ammonia recovery from hydrolyzed human urine by forward osmosis with acidified draw solution. Environ. Sci. Technol. 54 (18), 11556–11565. doi:10.1021/acs.est.0c02751
Ray, H., Perreault, F., and Boyer, T. H. (2020a). Rejection of nitrogen species in real fresh and hydrolyzed human urine by reverse osmosis and nanofiltration. J. Environ. Chem. Eng. 8, 103993. doi:10.1016/j.jece.2020.103993
Ray, H., Perreault, F., and Boyer, T. H. (2019). Urea recovery from fresh human urine by forward osmosis and membrane distillation (FO-MD). Environ. Sci. Water Res. Technol. 5 (11), 1993–2003. doi:10.1039/c9ew00720b
Regmi, U., and Boyer, T. H. (2020). Ammonium and potassium removal from undiluted and diluted hydrolyzed urine using natural zeolites. Chemosphere 268, 128849. doi:10.1016/j.chemosphere.2020.128849
Ronteltap, M., Maurer, M., and Gujer, W. (2007). Struvite precipitation thermodynamics in source-separated urine. Water Res. 41 (5), 977–984. doi:10.1016/j.watres.2006.11.046
Ronteltap, M., Maurer, M., Hausherr, R., and Gujer, W. (2010). Struvite precipitation from urine - influencing factors on particle size. Water Res. 44 (6), 2038–2046. doi:10.1016/j.watres.2009.12.015
Rose, D. P. (1966). Excretion of xanthurenic acid in the urine of women taking progestogen-oestrogen preparations. Nature 210 (5032), 196–197. doi:10.1038/210196a0
Sabin, J. M., Leverenz, H., and Bischel, H. N. (2022). Microbial fuel cell treatment energy-offset for fertilizer production from human urine. Chemosphere 294 (133594), 133594. doi:10.1016/j.chemosphere.2022.133594
Saetta, D., Zheng, C., Leyva, C., and Boyer, T. H. (2020). Impact of acetic acid addition on nitrogen speciation and bacterial communities during urine collection and storage. Sci. Total Environ. 745, 141010. doi:10.1016/j.scitotenv.2020.141010
Salar-Garcia, M. J., Ortiz-Martinez, V. M., Gajda, I., Greenman, J., Hernandez-Fernandez, F. J., and Ieropoulos, I. A. (2017). Electricity production from human urine in ceramic microbial fuel cells with alternative non-fluorinated polymer binders for cathode construction. Sep. Purif. Technol. 187, 436–442. doi:10.1016/j.seppur.2017.06.025
Saliu, T. D., Ali, J., Ololade, I. A., and Oladoja, N. A. (2020). Preparation and characterization of a decentralized modular yellow water nutrient recovery system. J. Environ. Manag. 276, 111345. doi:10.1016/j.jenvman.2020.111345
Sánchez, G., and Bosch, A. (2016). “Survival of enteric viruses in the environment and food,” in Viruses in foods. Viruses in foods. Editors S. M. Goyal, and J. L. Cannon. 2nd ed (Springer), 367–392.
Sanin, F. D., Vesilind, P. A., and Martel, C. J. (1994). Pathogen reduction capabilities of freeze/thaw sludge conditioning. Water Res. 28 (11), 2393–2398. doi:10.1016/0043-1354(94)90055-8
Schmidt, J., and Alleman, J. (2005). Urine processing for water recovery via freeze concentration. Trans. J. Aerosp. 114 (1), 2005–013032. doi:10.4271/2005-01-3032
Schonning, C., Leeming, R., and Stenstrom, T. A. (2002). Faecal contamination of source-separated human urine based on the content of faecal sterols. Water Res. 36 (8), 1965–1972. doi:10.1016/S0043-1354(01)00427-4
Scutari, R., Piermatteo, L., Ciancio Manuelli, M., Iannetta, M., Salpini, R., Bertoli, A., et al. (2020). Long-term SARS-CoV-2 infection associated with viral dissemination in different body fluids including bile in two patients with acute cholecystitis. Life 10 (11), 302. doi:10.3390/life10110302
Senecal, J., Nordin, A., Simha, P., and Vinnerås, B. (2018). Hygiene aspect of treating human urine by alkaline dehydration. Water Res. 144, 474–481. doi:10.1016/j.watres.2018.07.030
Senecal, J., and Vinnerås, B. (2017). Urea stabilisation and concentration for urine-diverting dry toilets: Urine dehydration in ash. Sci. Total Environ. 586, 650–657. doi:10.1016/j.scitotenv.2017.02.038
Sherbet, G. V., and Lakshmi, M. S. (1973). Characterisation of Escherichia coli cell surface by isoelectric equilibrium analysis. Biochimica Biophysica Acta - Biomembr. 298 (1), 50–58. doi:10.1016/0005-2736(73)90008-4
Shigemura, K., Shirakawa, T., Okada, H., Tanaka, K., Kamidono, S., Arakawa, S., et al. (2005). Rapid detection and differentiation of Gram-negative and Gram-positive pathogenic bacteria in urine using TaqMan probe. Clin. Exper. Med. 4 (4), 196–201. doi:10.1007/s10238-004-0056-x
Si-Mohamed, A., Goff, J. L., Desire, N., Maylin, S., Glotz, D., and Belec, L. (2006). Detection and quantitation of BK virus DNA by real-time polymerase chain reaction in the LT-ag gene in adult renal transplant recipients. J. Virological Methods 131 (1), 21–27. doi:10.1016/j.jviromet.2005.06.025
Simha, P., and Ganesapillai, M. (2017). Ecological sanitation and nutrient recovery from human urine: How far have we come? A review. Sustain. Environ. Res. 27 (3), 107–116. doi:10.1016/j.serj.2016.12.001
Simha, P., Karlsson, C., Viskari, E., Malila, R., and Vinneras, B. (2020b). Field testing a pilot-scale system for alkaline dehydration of source-separated human urine: A case study in Finland. Front. Environ. Sci. 8 (570637). doi:10.3389/fenvs.2020.570637
Simha, P., Lalander, C., Nordin, A., and Vinnerås, B. (2020a13931). Alkaline dehydration of source -separated fresh human urine: Preliminary insights into using different dehydration temperature and media. Sci. Total Environ. 733, 139313. doi:10.1016/j.scitotenv.2020.139313
Simha, P., Senecal, J., Nordin, A., Lalander, C., and Vinnerås, B. (2018). Alkaline dehydration of anion-exchanged human urine: Volume reduction, nutrient recovery and process optimisation. Water Res. 142, 325–336. doi:10.1016/j.watres.2018.06.001
Su, L. C., Tian, Y. C., Chang, Y. F., Chou, C., and Lai, C. S. (2014). Rapid detection of urinary polyomavirus BK by heterodyne-based surface plasmon resonance biosensor. J. Biomed. Opt. 19 (1), 011013. doi:10.1117/1.JBO.19.1.011013
Sun, J., Zhu, A., Li, H., Zheng, K., Zhuang, Z., Chen, Z., et al. (2020). Isolation of infectious SARS-CoV-2 from urine of a COVID-19 patient. Emerg. MICROBES Infect. 9 (1), 991–993. doi:10.1080/22221751.2020.1760144
Sun, P., Li, Y., Meng, T., Zhang, R., Song, M., and Ren, J. (2018). Removal of sulfonamide antibiotics and human metabolite by biochar and biochar/H2O2 in synthetic urine. Water Res. 147, 91–100. doi:10.1016/j.watres.2018.09.051
Tao, W., Bayrakdar, A., Wang, Y., and Agyeman, F. (2019). Three-stage treatment for nitrogen and phosphorus recovery from human urine: Hydrolysis, precipitation and vacuum stripping. J. Environ. Manag. 249, 109435. doi:10.1016/j.jenvman.2019.109435
Tarpeh, W. A., Barazesh, J. M., Cath, T. Y., and Nelson, K. L. (2018a). Electrochemical stripping to recover nitrogen from source-separated urine. Environ. Sci. Technol. 52 (3), 1453–1460. doi:10.1021/acs.est.7b05488
Tarpeh, W. A., Udert, K. M., and Nelson, K. L. (2017). Comparing ion exchange adsorbents for nitrogen recovery from source-separated urine. Environ. Sci. Technol. 51 (4), 2373–2381. doi:10.1021/acs.est.6b05816
Tarpeh, W. A., Wald, I., Wiprachtiger, M., and Nelson, K. L. (2018b). Effects of operating and design parameters on ion exchange columns for nutrient recovery from urine. Environ. Sci. Water Res. Technol. 4 (6), 828–838. doi:10.1039/c7ew00478h
Tian, X., Gao, Z., Feng, H., Zhang, Z., Li, J., and Wang, A. (2019). Efficient nutrient recovery/removal from real source-separated urine by coupling vacuum thermal stripping with activated sludge processes. J. Clean. Prod. 220, 965–973. doi:10.1016/j.jclepro.2019.02.181
Tidåker, P. (2003). Life Cycle Assessment of grain production using source-separated human urine and mineral fertilizer. Uppsala: Department of Agricultural Engineering, Swedish University of Agricultural Sciences.
Tun, L. L., Jeong, D., Jeong, S., Cho, K., Lee, S., and Bae, H. (2016). Dewatering of source-separated human urine for nitrogen recovery by membrane distillation. J. Membr. Sci. 512, 13–20. doi:10.1016/j.memsci.2016.04.004
Udert, K. M., Fux, C., Munster, M., Larsen, T. A., Siegrist, H., and Gujer, W. (2003d). Nitrification and autotrophic denitrification of source-separated urine. Water Sci. Technol. 48 (1), 119–130. doi:10.2166/wst.2003.0031
Udert, K. M., Larsen, T. A., Biebow, M., and Gujer, W. (2003a). Urea hydrolysis and precipitation dynamics in a urine-collecting system. Water Res. 37 (11), 2571–2582. doi:10.1016/S0043-1354(03)00065-4
Udert, K. M., Larsen, T. A., and Gujer, W. (2003b). Biologically induced precipitation in urine-collecting systems. Water Sci. Technol. Water Supply 3 (3), 71–78. doi:10.2166/ws.2003.0010
Udert, K. M., Larsen, T. A., and Gujer, W. (2003c). Estimating the precipitation potential in urine-collecting systems. Water Res. 37 (11), 2667–2677. doi:10.1016/S0043-1354(03)00071-X
Udert, K. M., and Wächter, M. (2012). Complete nutrient recovery from source-separated urine by nitrification and distillation. Water Res. 46 (2), 453–464. doi:10.1016/j.watres.2011.11.020
US Centers For Disease Control And Prevention (2008). Guideline for disinfection and sterilization in healthcare facilities. United States.
U. S. Department of Health and Human Services (2012). Principles of epidemiology in public health practice. 3rd ed. Atlanta, GA 30333: Centers for Disease Control and Prevention (CDC) Office of Workforce and Career Development, 1–62.
Van den Bossche, D., Cnops, L., and Van Esbroeck, M. (2015). Recovery of dengue virus from urine samples by real-time RT-PCR. Eur. J. Clin. Microbiol. Infect. Dis. 34 (7), 1361–1367. doi:10.1007/s10096-015-2359-0
Viancelli, A., Kunz, A., Fongaro, G., Kich, J. D., Barardi, C. R. M., and Suzin, L. (2015). Pathogen inactivation and the chemical removal of phosphorus from swine wastewater. Water Air Soil Pollut. 226 (8), 263. doi:10.1007/s11270-015-2476-5
Vinnerås, B., Bolske, G., Wahlstrom, H., and Albihn, A. (2011). Survival of Mycobacterium tuberculosis and Mycobacterium bovis in human urine. Water Sci. Technol. 63 (6), 1075–1080. doi:10.2166/wst.2011.344
Vinnerås, B., Nordin, A., Niwagaba, C., and Nyberg, K. (2008). Inactivation of bacteria and viruses in human urine depending on temperature and dilution rate. Water Res. 42 (15), 4067–4074. doi:10.1016/j.watres.2008.06.014
Vinnerås, B., Palmquist, H., Balmér, P., and Jönsson, H. (2006). The characteristics of household wastewater and biodegradable solid waste-A proposal for new Swedish design values. Urban Water J. 3 (1), 3–11. doi:10.1080/15730620600578629
Volpin, F., Chekli, L., Phuntsho, S., Cho, J., Ghaffour, N., Vrouwenvelder, J. S., et al. (2018). Simultaneous phosphorous and nitrogen recovery from source-separated urine: A novel application for fertiliser drawn forward osmosis. Chemosphere 203, 482–489. doi:10.1016/j.chemosphere.2018.03.193
Volpin, F., Chekli, L., Phuntsho, S., Ghaffour, N., Vrouwenvelder, J. S., and Shon, H. K. (2019a). Optimisation of a forward osmosis and membrane distillation hybrid system for the treatment of source-separated urine. Sep. Purif. Technol. 212, 368–375. doi:10.1016/j.seppur.2018.11.003
Volpin, F., Heo, H., Johir, M. A. H., Cho, J., Phuntsho, S., and Shon, H. K. (2019b). Techno-economic feasibility of recovering phosphorus, nitrogen and water from dilute human urine via forward osmosis. Water Res. 150, 47–55. doi:10.1016/j.watres.2018.11.056
Wang, R., Wang, X., Deng, C., Chen, Z., Chen, Y., Feng, X., et al. (2020). Partial nitritation performance and microbial community in sequencing batch biofilm reactor filled with zeolite under organics oppression and its recovery strategy. Bioresour. Technol. 305, 123031. doi:10.1016/j.biortech.2020.123031
Wang, Y., Geng, Y., Pan, X., and Sheng, G. (2017). In situ utilization of generated electricity for nutrient recovery in urine treatment using a selective electrodialysis membrane bioreactor. Chem. Eng. Sci. 171, 451–458. doi:10.1016/j.ces.2017.06.002
Wang, Y., Li, H., and Chen, B. (2016). Pathogen distribution and drug resistance of nephrology patients with urinary tract infections. Saudi Pharm. J. 24 (3), 337–340. doi:10.1016/j.jsps.2016.04.013
Wei, S. P., van Rossum, F., van de Pol, G. J., and Winkler, M. H. (2018). Recovery of phosphorus and nitrogen from human urine by struvite precipitation, air stripping and acid scrubbing: A pilot study. Chemosphere 212, 1030–1037. doi:10.1016/j.chemosphere.2018.08.154
Wiwanitkit, V. (2016). Urine-based molecular diagnosis of Zika virus. Int. Urol. Nephrol. 48 (12), 2023. doi:10.1007/s11255-016-1417-6
Wohlsager, S., Clemens, J., Nguyet, P. T., Rechenburg, A., and Arnold, U. (2010). Urine - a valuable fertilizer with low risk after storage in the tropics. Water Environ. Res. 82 (9), 840–847. doi:10.2175/106143010x12609736967125
Wohlsager, S., Clemens, J., Nguyet, P. T., Rechenburg, A., and Arnold, U. (2010). Urine--a valuable fertilizer with low risk after storage in the tropics. Water Environ. Res. 82 (9), 840–847. doi:10.2175/106143010x12609736967125
World Health Organization (WHO), (2006). Guidelines for the safe use of the wastewater, excreta and grey water. Vol 2, Wastewater use in agriculture. Geneva, Switzerland: WHO.
Wyszogrodzka, G., Marszałek, B., Gil, B., and Dorożyński, P. (2016). Metal-organic frameworks: Mechanisms of antibacterial action and potential applications. Drug Discov. Today 21 (6), 1009–1018. doi:10.1016/j.drudis.2016.04.009
Xiao, R., Bai, L., Liu, K., Shi, Y., Minakata, D., Huang, C., et al. (2020). Elucidating sulfate radical-mediated disinfection profiles and mechanisms of Escherichia coli and Enterococcus faecalis in municipal wastewater. Water Res. 173 (115552), 115552. doi:10.1016/j.watres.2020.115552
Xu, D., Zhang, Z., Jin, L., Chu, F., Mao, Y., Wang, H., et al. (2005). Persistent shedding of viable SARS-CoV in urine and stool of SARS patients during the convalescent phase. Eur. J. Clin. Microbiol. Infect. Dis. 24 (3), 165–171. doi:10.1007/s10096-005-1299-5
Xu, K., Glanton, V., Johnson, S. R., Beck-Sague, C. O. N. S. U. E. L. O., Bhullar, V., Ccandal, D. H., et al. (1998). Detection of neisseria gonorrhoeae infection by ligase chain reaction testing of urine among adolescent women with and without chlamydia trachomatis infection. Sex. Transm. Dis. 25 (10), 533–538. doi:10.1097/00007435-199811000-00007
Xu, K., Li, J., Zheng, M., Zhang, C., Xie, T., and Wang, C. (2015b). The precipitation of magnesium potassium phosphate hexahydrate for P and K recovery from synthetic urine. Water Res. 80, 71–79. doi:10.1016/j.watres.2015.05.026
Xu, K., Lin, F., Dou, X., Zheng, M., Tan, W., and Wang, C. (2018). Recovery of ammonium and phosphate from urine as value-added fertilizer using wood waste biochar loaded with magnesium oxides. J. Clean. Prod. 187, 205–214. doi:10.1016/j.jclepro.2018.03.206
Xu, K., Qu, D., Zheng, M., Guo, X., and Wang, C. (2019a). Water reduction and nutrient reconcentration of hydrolyzed urine via direct contact membrane distillation: Ammonia loss and its control. J. Environ. Eng. 145 (3), 04018144. doi:10.1061/(ASCE)EE.1943-7870.0001496
Xu, K., Wang, C., Liu, H., and Qian, Y. (2011). Simultaneous removal of phosphorus and potassium from synthetic urine through the precipitation of magnesium potassium phosphate hexahydrate. Chemosphere 84 (2), 207–212. doi:10.1016/j.chemosphere.2011.04.057
Xu, K., Wang, C., Wang, X., and Qian, Y. (2012). Laboratory experiments on simultaneous removal of K and P from synthetic and real urine for nutrient recycle by crystallization of magnesium-potassium-phosphate-hexahydrate in a draft tube and baffle reactor. Chemosphere 88 (2), 219–223. doi:10.1016/j.chemosphere.2012.02.061
Xu, K., Zhang, C., Dou, X., Ma, W., and Wang, C. (2019b). Optimizing the modification of wood waste biochar via metal oxides to remove and recover phosphate from human urine. Environ. Geochem. Health 41, 1767–1776. doi:10.1007/s10653-017-9986-6
Xu, K., Zhang, C., Li, J., Cheng, X., and Wang, C. (2017). Removal and recovery of N, P and K from urine via ammonia stripping and precipitations of struvite and struvite-K. Water Sci. Technol. 75 (1), 155–164. doi:10.2166/wst.2016.494
Xu, S., Luo, L., He, H., Liu, H., and Cui, L. (2015a). Nitrogen and phosphate recovery from source-separated urine by dosing with magnesite and zeolite. Pol. J. Environ. Stud. 24 (5), 2269–2275. doi:10.15244/pjoes/43611
Yam, W. C., Chan, K. H., Chow, K. H., Poon, L. L., Lam, H. Y., Yuen, K. Y., et al. (2005). Clinical evaluation of real-time PCR assays for rapid diagnosis of SARS coronavirus during outbreak and post-epidemic periods. J. Clin. Virology 33 (1), 19–24. doi:10.1016/j.jcv.2004.09.029
Yang, D., Zhang, J., Xie, M., Tong, X., Jiang, T., Yu, W., et al. (2022). An integrated hollow fiber membrane contactor and chemical precipitation to recover N, P and K from human urine wastewater. J. Environ. Chem. Eng. 10, 107844. doi:10.1016/j.jece.2022.107844
Yang, L., Giannis, A., Chang, V. W. C., Liu, B., Zhang, J., and Wang, J. (2015). Application of hydroponic systems for the treatment of source-separated human urine. Ecol. Eng. 81, 182–191. doi:10.1016/j.ecoleng.2015.04.013
Yao, G., Lei, J., Zhang, W., Yu, C., Sun, Z., Zheng, S., et al. (2019). Antimicrobial activity of X zeolite exchanged with Cu2+ and Zn2+ on Escherichia coli and Staphylococcus aureus. Environ. Sci. Pollut. Res. 26 (3), 2782–2793. doi:10.1007/s11356-018-3750-z
Yao, S., Chen, L., Guan, D., Zhang, Z., Tian, X., Wang, A., et al. (2017). On-site nutrient recovery and removal from source-separated urine by phosphorus precipitation and short-cut nitrification-denitrification. Chemosphere 175, 210–218. doi:10.1016/j.chemosphere.2017.02.062
Yee, R. A., Alessi, D. S., Ashbolt, N. J., Hao, W., Konhauser, K., and Liu, Y. (2019). Nutrient recovery from source-diverted blackwater: Optimization for enhanced phosphorus recovery and reduced co-precipitation. J. Clean. Prod. 235, 417–425. doi:10.1016/j.jclepro.2019.06.191
Yoon, J. G., Yoon, J., Song, J. Y., Yoon, S. Y., Lim, C. S., Seong, H., et al. (2020). Clinical significance of a high SARS-CoV-2 viral load in the Saliva. J. Korean Med. Sci. 35 (20), e195. doi:10.3346/jkms.2020.35.e195
You, J., Greenman, J., Melhuish, C., and Ieropoulos, I. (2016). Electricity generation and struvite recovery from human urine using microbial fuel cells. J. Chem. Technol. Biotechnol. 91 (3), 647–654. doi:10.1002/jctb.4617
Yu, T., Ma, J. Z., and Li, Q. (2007). Factors affecting ice crystal purity during freeze concentration process for urine treatment. J. Harbin Inst. Technol. 14 (5), 593–597.
Yu, Y., Zhang, Z., Wang, Y., Liao, M., Rong, X., Li, B., et al. (2019). Effects of different preservation methods on physicochemical property of marine pathogen Vibrio anguillarum. J. Ocean. Univ. China 18 (6), 1417–1426. doi:10.1007/s11802-019-4050-z
Zamora, P., Georgieva, T., Salcedo, I., Elzinga, N., Kuntke, P., and Buisman, C. J. N. (2017). Long-term operation of a pilot-scale reactor for phosphorus recovery as struvite from source-separated urine. J. Chem. Technol. Biotechnol. 92 (5), 1035–1045. doi:10.1002/jctb.5079
Zhang, B., Tian, S., and Wu, D. (2022). An integrated strategy for nutrient harvesting from hydrolyzed human urine as high-purity products: Tracking of precipitation transformation and precise regulation. Sci. Total Environ. 854, 158721. doi:10.1016/j.scitotenv.2022.158721
Zhang, C., Xu, K., Li, J., Wang, C., and Zheng, M. (2017). Recovery of phosphorus and potassium from source-separated urine using a fluidized bed reactor: Optimization operation and mechanism modeling. Ind. Eng. Chem. Res. 56 (11), 3033–3039. doi:10.1021/acs.iecr.6b04819
Zhang, C., Xu, K., Su, F., and Wang, C. (2015). Review on pilot projects of source separation system. China Water & Wastewater 31 (2), 28–33.
Zhang, C., Xu, K., Zheng, M., Li, J., and Wang, C. (2018). Factors affecting the crystal size of struvite-K formed in synthetic urine using a stirred reactor. Ind. Eng. Chem. Res. 57, 17301–17309. doi:10.1021/acs.iecr.8b03328
Zhang, J., She, Q., Chang, V. W. C., Tang, C. Y., and Webster, R. D. (2014). Mining nutrients (N, K, P) from urban source-separated urine by forward osmosis dewatering. Environ. Sci. Technol. 48 (6), 3386–3394. doi:10.1021/es405266d
Zhang, R., Lan, L., and Shi, H. (2021a). Sublethal injury and recovery of Escherichia coli O157:H7 after freezing and thawing. Food control. 120, 107488. doi:10.1016/j.foodcont.2020.107488
Zhang, R., Yang, Y., Huang, C., Zhao, L., and Sun, P. (2016). Kinetics and modeling of sulfonamide antibiotic degradation in wastewater and human urine by UV/H2O2 and UV/PDS. Water Res. 103, 283–292. doi:10.1016/j.watres.2016.07.037
Zhang, X., Gang, D. D., Sun, P., Lian, Q., and Yao, H. (2021b). Goethite dispersed corn straw-derived biochar for phosphate recovery from synthetic urine and its potential as a slow-release fertilizer. Chemosphere 262, 127861. doi:10.1016/j.chemosphere.2020.127861
Zhang, Y., Aguila, B., Ma, S., and Zhang, Q. (2020). Comparison of the use of functional porous organic polymer (POP) and natural material zeolite for nitrogen removal and recovery from source-separated urine. J. Environ. Chem. Eng. 8, 104296. doi:10.1016/j.jece.2020.104296
Zhang, Y., Li, Z., Zhao, Y., Chen, S., and Mahmood, I. B. (2013). Stabilization of source-separated human urine by chemical oxidation. Water Sci. Technol. 67 (9), 1901–1907. doi:10.2166/wst.2013.055
Zhao, Z., Xu, L., Shang, X., and Chen, K. (2013). Water regeneration from human urine by vacuum membrane distillation and analysis of membrane fouling characteristics. Sep. Purif. Technol. 118, 369–376. doi:10.1016/j.seppur.2013.07.021
Zheng, M., Xie, T., Li, J. Y., Xu, K., and Wang, C. W. (2018). Biochar as a carrier of struvite precipitation for nitrogen and phosphorus recovery from urine. J. Environ. Eng. 144 (10). doi:10.1061/(ASCE)EE.1943-7870.0001450
Zhou, X., Li, Y., Li, Z., Xi, Y., Nazim Uddin, S. M., and Zhang, Y. (2017). Investigation on microbial inactivation and urea decomposition in human urine during thermal storage. J. Water, Sanit. Hyg. Dev. 7 (3), 378–386. doi:10.2166/washdev.2017.142
Zhou, X., Li, Y., Li, Z., Xi, Y., Uddin, S. M. N., and Zhang, Y. (2017). Investigation on microbial inactivation and urea decomposition in human urine during thermal storage. J. Water, Sanitation Hyg. Dev. 7 (3), 378–386. doi:10.2166/washdev.2017.142
Zhu, N., Zhang, D., Wang, W., Li, X., Yang, B., Song, J., et al. (2020). A novel Coronavirus from patients with pneumonia in China, 2019. N. Engl. J. Med. 382 (8), 727–733. doi:10.1056/NEJMoa2001017
Zhu, Z., Liu, Y., Xu, L., Guan, W., Zhang, X., Qi, T., et al. (2015). Extra-pulmonary viral shedding in H7N9 Avian Influenza patients. J. Clin. Virology 69, 30–32. doi:10.1016/j.jcv.2015.05.013
Zoellig, H., Fritzsche, C., Morgenroth, E., and Udert, K. M. (2015). Direct electrochemical oxidation of ammonia on graphite as a treatment option for stored source-separated urine. Water Res. 69, 284–294. doi:10.1016/j.watres.2014.11.031
Keywords: urine source separation, nutrient recovery, pathogens inactivation, microbial risk, urine treatment
Citation: Xu K, Lu J, Hu L, Li J, Cheng S, Zheng M and Wang C (2022) Pathogens inactivation in nutrient recovery from urine: A review. Front. Environ. Sci. 10:1056019. doi: 10.3389/fenvs.2022.1056019
Received: 28 September 2022; Accepted: 28 November 2022;
Published: 07 December 2022.
Edited by:
Huiyu Dong, Research Center for Eco-Environmental Sciences (CAS), ChinaReviewed by:
Santiago Septien Stringel, University of KwaZulu-Natal, South AfricaHongbo Liu, University of Shanghai for Science and Technology, China
Copyright © 2022 Xu, Lu, Hu, Li, Cheng, Zheng and Wang. This is an open-access article distributed under the terms of the Creative Commons Attribution License (CC BY). The use, distribution or reproduction in other forums is permitted, provided the original author(s) and the copyright owner(s) are credited and that the original publication in this journal is cited, in accordance with accepted academic practice. No use, distribution or reproduction is permitted which does not comply with these terms.
*Correspondence: Kangning Xu, eHVrYW5nbmluZ0BiamZ1LmVkdS5jbg==; Jiyun Li, bGlqaXl1bm5AMTYzLmNvbQ==