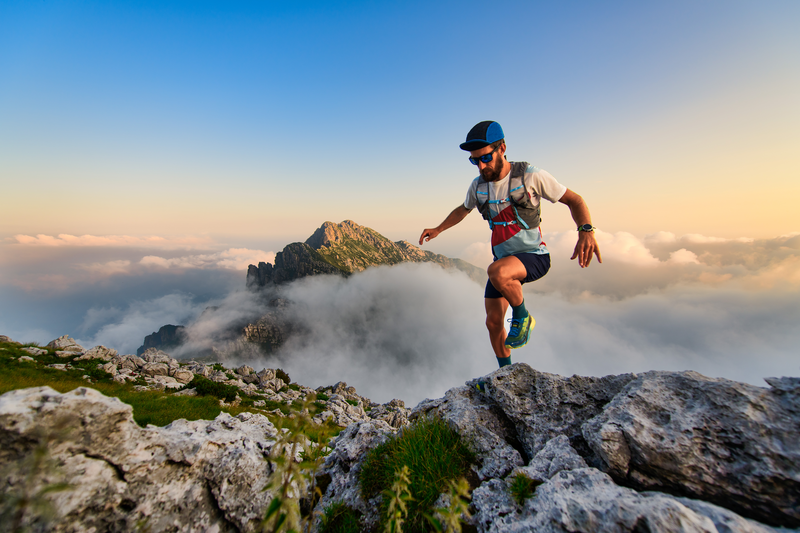
95% of researchers rate our articles as excellent or good
Learn more about the work of our research integrity team to safeguard the quality of each article we publish.
Find out more
EDITORIAL article
Front. Environ. Sci. , 28 October 2022
Sec. Atmosphere and Climate
Volume 10 - 2022 | https://doi.org/10.3389/fenvs.2022.1053941
This article is part of the Research Topic Permafrost Environment Changes in a Warming Climate View all 12 articles
Editorial on the Research Topic
Permafrost degradation affects hydrology, ecology, and carbon cycle
Permafrost regions account for about 22% of the exposed land area in the Northern Hemisphere (Obu et al., 2019). As one of physical characteristics in the cold environment, permafrost is sensitive to climate change. During the past decades, permafrost in high latitude and high-altitude regions shows obvious degradation, which is indicated by increasing ground temperature, deepening active layer, shrinking of permafrost area, and development of thermokarst features (Biskaborn et al., 2019).
Permafrost is distributed beneath the earth’s surface. Permafrost can regulate the regional water cycle and ecology from several mechanisms. First, as a weak impermeable layer, permafrost can prevent water vertical infiltration and increase the surface soil water content. Second, the freeze-thaw cycles of the active layer can store excess water from summer rainfall as ice during winter, and the melting of this ice can supply soil water in the following summer. Third, ground ice melting can provide soil water for plant growth (Sugimoto et al., 2003). Permafrost regions also store a large amount of soil organic carbon, which is almost twice as the carbon currently contained in the atmosphere (Mishra et al., 2021). These carbon pools have been gradually accumulated and preserved during the past thousands of years due to the low-temperature limiting the microbial decomposition of organic matter. The permafrost degradation may remobilize these carbon pools by releasing greenhouse gases into the air. This process contributes one of the great uncertainties in the terrestrial carbon cycle feedback (Schuur et al., 2015). In addition, permafrost regions also store a large number of pollutants and heavy metals (e.g., mercury) which have been sequestrated for a long time. Permafrost degradation poses environmental risks and thawing permafrost may release these biological or chemical substances that can affect human health (Schuster et al., 2018; Miner et al., 2021).
To address the issues on how permafrost environment is changing, to what extent the changing permafrost may affect the hydrology, ecology, carbon cycle, and pollutants, eleven multi-discipline studies are collected in this special topic on permafrost environment changes in a warming climate.
Permafrost regions have been warming at two to three times the global average (Hu et al., 2021). Using the monthly air temperature reanalysis dataset from the Climate Research Unit (CRU, University of East Anglia), it was found that the air freezing index in the Mongolian Plateau decreased by 4.1 C d yr-1, and the air thawing index increased by 2.3 C d yr−1 during 1901–2019. The northern permafrost regions showed large variabilities in freezing and thawing index than the southern non-permafrost regions (Ma et al.). Based on the meteorological station records from 1957 to 2019, the annual mean air temperature has increased by 0.031–0.039°C yr−1 in the hinterland of the Qinghai-Tibet Plateau. The ground temperature within the active layer at 1 m depth increased at an average rate of 0.05°C yr−1 (Zhou et al.). Along with climate warming, frequency of extreme events also changed. On the Qinghai-Tibet Plateau, the warmth indices such as warm days, warm nights, summer days, and tropical nights increased at rates of 1.1, 1.6, 1.4 and 0.3 days per decade from 1960 to 2016. Meanwhile, cold indices including the number of cool days, cool nights, ice days, and frost days decreased significantly (Gong et al.). These results confirmed the rapid warming of the permafrost environment during the past decades and also provide useful data to understand the changing patterns and future projections of permafrost.
Three studies (i.e., Yang et al.; Rossi et al.; Polyakov et al.) examined the detecting permafrost and soil mapping method in permafrost regions. The equivalent anti-flux opposing coils were used to eliminate the blind area for the transient electromagnetic method, and the results showed that this method solved the problem of the shallow detection blind area, eliminated the interference caused by the primary field, and improved the horizontal and vertical resolutions (Yang et al.). In the Russian Arctic, geophysical and geocryological methods including landscape microzonation, borehole drilling, ground temperature measurements, and geoelectric surveys were employed to investigate the active layer thickness. The results showed that the multidisciplinary approach can be also useful for other areas (Rossi et al.). In permafrost regions, soil type is one of the most fundamental properties because it is an important parameter for Earth System Models as well as the carbon stocks estimation. However, due to the harsh natural conditions, field investigation of soil types is usually costly and difficult. Using the unmanned aerial vehicle (UAV) imaging data in the Lena River Delta, classical soil sections, geomorphological observation, and determination of the main chemical parameters of soils are presented. Although accurate mapping of soil types should be based on chemical analysis, this result suggests that the high-resolution soil-geomorphological maps based on the Geographic Information System and UAV data are useful for the mapping of soil types under the high variability of the watershed dan cryogenic landscapes (Polyakov et al.).
Permafrost significantly affects ecology and hydrology (Woo et al., 2008). A review paper in this topic summarizes that soil water potential is widely used to describe the energy state of liquid water. The movement of liquid water in the soil is mainly determined by soil matric potential. The process of ice lenses development in permafrost has been explained by mathematical models, however, existing models might be too simplified (Fu et al.). Therefore, new model development for ice formation for micro landscapes is still largely needed. To investigate the effects of hydrology on peat permafrost and carbon process, a process-based model, i.e., HPM-Arctic, was used the simulate the past and future changes in a peatland ecosystem in the Canadian Arctic. The results showed that the regional hydrology and basin characteristics strongly determined peat accumulation history and its future changes in organic carbon stocks under different climate scenarios (Treat et al.). For the carbon cycle in the Arctic permafrost, a pilot study showed that extensively grazing by large animals can cool the ground temperature by modifying ground cover properties. In addition, the soil organic carbon content is also higher in the extensively grazing sites than that of non-grazing sites, which is likely attributed to the higher carbon input (Windirsch et al.).
Heavy metals are anthropogenic contaminants that can be transported for long distances. Due to the atmospheric circulation and deposition, large heavy metals have been transported to the Arctic, Antarctic, as well as the Qinghai-Tibet Plateau. A review paper in this topic issue pointed out that heavy metals on the Qinghai-Tibet plateau are mainly from surrounding heavily-polluted regions. The shrinkage of the cryosphere may increase the release of these heavy metals in the future. This work highlights the importance of heavy metals in permafrost environments.
This special topic has collected the studies of permafrost regions located in the Arctic, Mongolia, and the Qinghai-Tibet Plateau. The results deepen our understanding of changing trends of climate and permafrost, interactions among permafrost, hydrology, ecology, carbon cycle, and risks of heavy metals. We hope this special topic could provide valuable references to the researchers with relevant interest and play an active role in promoting the research of permafrost changes and their environmental impacts.
All authors listed have made a substantial, direct, and intellectual contribution to the work and approved it for publication.
This work was supported by the National Natural Science Foundation of China (41941015, 32061143032), National Key Research and Development Program of China (2019YFA0607003), the State Key Laboratory of Cryospheric Science (SKLCS-ZZ-2022), and the West Light Foundation of the Chinese Academy of Sciences. WZ acknowledged grants of the Swedish Research Council VR 2020-05338.
The authors declare that the research was conducted in the absence of any commercial or financial relationships that could be construed as a potential conflict of interest.
All claims expressed in this article are solely those of the authors and do not necessarily represent those of their affiliated organizations, or those of the publisher, the editors and the reviewers. Any product that may be evaluated in this article, or claim that may be made by its manufacturer, is not guaranteed or endorsed by the publisher.
Biskaborn, B. K., Smith, S. L., Noetzli, J., Matthes, H., Vieira, G., Streletskiy, D. A., et al. (2019). Permafrost is warming at a global scale. Nat. Commun. 10 (1), 264. doi:10.1038/s41467-018-08240-4
Hu, G., Zhao, L., Wu, T., Wu, X., Park, H., Fedorov, A., et al. (2021). Spatiotemporal variations and regional differences in air temperature in the permafrost regions in the Northern Hemisphere during 1980–2018. Sci. Total Environ. 197, 148358. doi:10.1016/j.scitotenv.2021.148358
Miner, K. R., D’Andrilli, J., Mackelprang, R., Edwards, A., Malaska, M. J., Waldrop, M. P., et al. (2021). Emergent biogeochemical risks from arctic permafrost degradation. Nat. Clim. Chang. 11 (10), 809–819. doi:10.1038/s41558-021-01162-y
Mishra, U., Hugelius, G., Shelef, E., Yang, Y., Strauss, J., Lupachev, A., et al. (2021). Spatial heterogeneity and environmental predictors of permafrost region soil organic carbon stocks. Sci. Adv. 7 (9), eaaz5236. doi:10.1126/sciadv.aaz5236
Obu, J., Westermann, S., Bartsch, A., Berdnikov, N., Christiansen, H. H., Dashtseren, A., et al. (2019). Northern hemisphere permafrost map based on TTOP modelling for 2000–2016 at 1 km2 scale. Earth-Science Rev. 193, 299–316. doi:10.1016/j.earscirev.2019.04.023
Schuster, P. F., Schaefer, K. M., Aiken, G. R., Antweiler, R. C., Dewild, J. F., Gryziec, J. D., et al. (2018). Permafrost stores a globally significant amount of mercury. Geophys. Res. Lett. 45 (3), 1463–1471. doi:10.1002/2017gl075571
Schuur, E., McGuire, A. D., Schadel, C., Grosse, G., Harden, J. W., Hayes, D. J., et al. (2015). Climate change and the permafrost carbon feedback. Nature 520 (7546), 171–179. doi:10.1038/nature14338
Sugimoto, A., Naito, D., Yanagisawa, N., Ichiyanagi, K., Kurita, N., Kubota, J., et al. (2003). Characteristics of soil moisture in permafrost observed in east siberian taiga with stable isotopes of water. Hydrol. Process. 17 (6), 1073–1092. doi:10.1002/hyp.1180
Keywords: permafrost, climate warming, hydrology, ecology, carbon cycle
Citation: Wu X, Zhang W and Mu C (2022) Editorial: Permafrost degradation affects hydrology, ecology, and carbon cycle. Front. Environ. Sci. 10:1053941. doi: 10.3389/fenvs.2022.1053941
Received: 26 September 2022; Accepted: 14 October 2022;
Published: 28 October 2022.
Edited by:
Hong Liao, Nanjing University of Information Science and Technology, ChinaReviewed by:
Nikita Tananaev, Siberian Branch of the Russian Academy of Sciences (RAS), RussiaCopyright © 2022 Wu, Zhang and Mu. This is an open-access article distributed under the terms of the Creative Commons Attribution License (CC BY). The use, distribution or reproduction in other forums is permitted, provided the original author(s) and the copyright owner(s) are credited and that the original publication in this journal is cited, in accordance with accepted academic practice. No use, distribution or reproduction is permitted which does not comply with these terms.
*Correspondence: Xiaodong Wu, d3V4ZEBsemIuYWMuY24=
Disclaimer: All claims expressed in this article are solely those of the authors and do not necessarily represent those of their affiliated organizations, or those of the publisher, the editors and the reviewers. Any product that may be evaluated in this article or claim that may be made by its manufacturer is not guaranteed or endorsed by the publisher.
Research integrity at Frontiers
Learn more about the work of our research integrity team to safeguard the quality of each article we publish.