- 1International Joint Research Laboratory for Global Change Ecology, School of Life Sciences, Henan University, Kaifeng, Henan, China
- 2Yellow River Floodplain Ecosystems Research Station, Henan University, Xingyang, China
- 3State Key Laboratory of Subtropical Mountain Ecology (Funded by Ministry of Science and Technology and Fujian Province), Fujian Normal University, Fuzhou, Fujian, China
Variations in soil aggregates and soil organic carbon (SOC) in response to land-use change are important to understanding the carbon cycle in forest ecosystems. However, few studies investigated the effect of forest type on aggregate stability, SOC content, and particulate organic carbon (POC) content. Therefore, we collected soil and fine root samples in two natural forests (Pinus massoniana and Quercus variabilis) and a planted forest (Cunninghamia lanceolata) in a warm temperate–subtropical climate transition zone to analyze the effect of forest type on aggregate stability, SOC content, and POC content. The results showed that the mean weight diameter (MWD) of the soil aggregates was significantly higher in Quercus variabilis and Pinus massoniana forests (62% and 21%, respectively) than in the Cunninghamia lanceolata forest due to higher mycelial length density, mycelial infection rate, and glomalin content. Similarly, the SOC and POC contents were significantly higher in Quercus variabilis and Pinus massoniana forests than in the Cunninghamia lanceolata forest (p < 0.05). The dominant size fraction of aggregate was highly correlated with the carbon fraction content. The SOC and POC contents and fungal traits (mycelial length density, mycelial infection rate, and glomalin content) were significantly positively correlated with the MWD. These results indicated that natural forests had higher aggregate stability than planted forests due to higher SOC content and more favorable fungal traits in the warm temperate–subtropical climate transition zone.
Introduction
Soil aggregates are critical components of soil and a key indicator to evaluating soil quality and carbon sequestration potential (An et al., 2010; Chen et al., 2017). Soils primarily comprise macroaggregates (0.25–2 mm), which reduce water runoff and limit oxygen diffusion (Bonifacio et al., 2006; Bouajila and Gallali, 2010; Huang et al., 2010), and microaggregates (<250 μm), which bind soil organic carbon (SOC) and prevent its removal by erosion. Aggregate stability affects these functions and is influenced by abiotic factors (e.g., texture) (Peng et al., 2017; He et al., 2020; Zhang et al., 2019), vegetation, microbial communities, and their interactions (Yang et al., 2009; Feng et al., 2020). Despite the complex formation mechanism of aggregates, plant roots and their mycelia have a crucial influence on the stability of soil aggregates. Thus, the vegetation type affects soil aggregate stability and carbon sequestration through plant roots and mycelia in forest soils. Therefore, understanding the effect of plant roots and mycelial growth on soil aggregates is crucial to shed light on soil’s carbon sequestration potential.
Since more than 90% of soil carbon (C) is contained in soil aggregates, the formation and disruption of soil aggregates control the turnover rate of SOC (Jastrow et al., 2007). Various physical, chemical, and biological factors can contribute to the formation and stability of soil aggregates, such as fine roots (Merino-Martín et al., 2020) and fungal hyphae (Morris et al., 2019). Numerous studies have demonstrated a strong relationship between glomalin-related soil proteins (GRSPs) and soil aggregate stability (Qin et al., 2017; Holátko et al., 2020; Li et al., 2020). Specifically, plants have many fine roots that produce root exudates to promote the formation of aggregates because macromolecular mucopolysaccharides produced by plant root exudates strongly adhere to soil particles. Similarly, microorganisms stimulate the formation of hyphae, and the cementation of microbial metabolites promotes the formation of aggregates. Fine roots, SOC, and mycorrhizal fungi are involved in the formation of soil aggregates, influencing soil C stability. However, the land-use type affects these factors (Merino-Martín et al., 2020), thereby influencing soil aggregates and C stability.
Land use influences soil aggregates via binding agents in soils, such as soil organic matter and fungal hyphae (Zeng et al., 2018). For instance, a study in Costa Rica showed that SOC, soil total phosphorus, and cation exchange capacity were the main drivers of soil aggregated stability on a large scale. However, the fine root traits, especially root biomass and root length density, had the largest influence on soil aggregate stability at the community level (Bissonnais et al., 2018). Similarly, differences in the SOC content between planted and natural forests were observed and attributed to differences in the root system. A meta-analysis showed that the fine root biomass and fine root production were 36.5% and 36% higher, respectively, in natural forests than in planted forests (Cai et al., 2019). In contrast, Merino-Martín et al. (2020) found that soil aggregate stability was primarily associated with differences in the soil microbial community between natural and planted artificial forests (Merino-Martín et al., 2020). In addition, arbuscular mycorrhizal fungi (AMF) associated with plant roots appear to act as long-term soil binding agents that release stable glycoprotein (glomalin), which can bind soil particles to form aggregates (Wright and Upadhyaya, 1996; Dou et al., 2019), influencing soil structure (Zhang et al., 2019; Bai et al., 2020). These results demonstrated that multiple factors, such as forest type or land-use type, contributed to soil aggregate formation and stabilization. The dynamics of soil aggregates in different forests affect the SOC content due to different binding agents. Therefore, it is critical to investigate the stability and influencing factors of soil aggregates in different forest types and their association with C stability to understand global C cycles. However, knowledge gaps exist on how the interactions between soil aggregates and binding agents (e.g., roots and fungal hyphae) influence soil C stability.
Forests in climate transition zones are crucial to the global carbon balance and the response to ongoing climate change; they are also sensitive to global climate change. Therefore, we selected three forest types (Pinus massoniana Lamb., Quercus variabilis Bl., and Cunninghamia lanceolata (Lamb.) Hook in a warm temperate and subtropical climate transition zone to explore the effects of forest type on soil aggregation and SOC fractions. The following two hypotheses were tested: 1) soil aggregation is higher in natural forest soil than planted forest soil due to more abundant fungal hyphae and 2) natural forest soil captures more C than planted forest soil.
Materials and methods
Site description and experimental design
A natural coniferous forest, natural broadleaved forest, and planted Chinese fir forest were the study sites in the Xian Mountain region (32°06′N, 114°01′E, 204 m a.s.l.) of the Nanwan Forest Service, Xinyang, Henan, China. The area has a subtropical monsoon climate, with an annual average temperature of 15.2°C and average precipitation of 1,063 mm. The forest soil is classified as a Haplic Luvisol (FAO classification). The dominant forest types in this area are Quercus variabilis Bl, Pinus massoniana Lamb, and Cunninghamia lanceolata (Lamb.) Hook. Therefore, we selected two natural forest areas (Quercus variabilis forest (QF) and Pinus massoniana forest (PF)) and one planted forest area (Cunninghamia lanceolata forest (CF)) with three replications as the experimental sites. Other tree species in the QF are Liquidambar formosana Hance, Acer mono Maxim, and Lindera glauca (Sieb. et Zucc.) Bl. Other tree species in the PF are Quercus acutissima Carruth, Lindera glauca (Sieb. et Zucc.) Bl, and Vitex negundo L. Deforestation in this area before the 1960s significantly reduced forest resources. Subsequently, the local government carried out afforestation and closed hillsides to facilitate it, increasing the area of planted and natural secondary forests. The trees have similar ages and are currently 60 years old. Each forest site was larger than 100 × 100 m2 and was surrounded by a 100-m buffer zone.
Soil sampling
The three forest types (QF, PF, and CF) were sampled in July 2019. The stands had similar slope aspects, elevations, and soil types. Forest types with similar slope aspects were selected randomly. Each forest type was divided into three sample plots, with ten sampling points each. In total, 10 soil cores (0–10 cm depth) were collected with a soil auger (5 cm diameter) at each plot and were combined into one sample for analyzing soil chemical properties. We collected another 10 samples with similar methods to analyze the root traits. Undisturbed soil samples were obtained at each forest site using bulk soil sampling for aggregate separation. The samples were placed in a plastic box and transported to the laboratory. We collected a total of 27 soil samples (three forests × three replicates × three types of soil samples) to analyze the chemical properties, fine root traits, and soil aggregates. All fresh samples were placed into a cooler with an ice bag and sent to the laboratory as soon as possible for analysis.
After removing all the visible roots and stones, the soil was passed through a 2-mm sieve. The sample was divided into two subsamples. One subsample was air-dried and passed through a 0.25-mm mesh to measure the chemical composition of the soil, and the other subsamples were kept at 4°C for analysis of the microbial biomass carbon (MBC), microbial biomass nitrogen (MBN), and microbial biomass phosphorus (MBP) contents.
Analysis of soil properties
SOC and total nitrogen (TN) were measured using a Vario Max CNS elemental analyzer (Elementar Analysensysteme GmbH, Hanau, Germany). The available phosphorus (AP) was extracted with 0.5 M of NaHCO3 and analyzed using an ultraviolet (UV) spectrophotometer (UV-1900, Daojin, Japan). The soil pH was determined using a pH meter (Sartorius PT-21, Shanghai, China) and using a soil–water ratio of 1:2.5. The MBC, MBN, and MBP contents were obtained by chloroform fumigation and extraction (Joergensen and Mueller, 1996). The MBC and MBN contents were determined using a C analyzer (Vario TOC, German Elementar Company, Hanau, Germany). The total glomalin-related soil proteins (T-GRSP) and easily extractable glomalin-related soil proteins (EE-GRSP) in the soil were extracted and measured as described by Wright and Upadhyaya (1996). A UV spectrophotometer was used to determine the MBP and GRSP contents. The details of the GRSP assay were described by Chen et al. (2019).
Root sampling and root trait measurements
We carefully washed the soil cores with tap water in a basin to separate the fine roots of the plants. All living roots visible to the naked eye were collected with tweezers. Roots less than 2 mm in diameter were defined as fine roots. We randomly selected a subsample of the roots for morphological measurements. We weighed them and placed them on the scanner without overlap. The roots were scanned using a flatbed scanner (Cannon LiDE 220) at a resolution of 600 dpi (Pierret et al., 2013), followed by oven-drying at 60°C and weighing. The root images (600 dpi) were analyzed with the open-source software IJ_Rhizo (Pierret et al., 2013, http://www.plant-image-analysis.org). The total root length and mean root diameter (MD) were measured with the same software. The specific root length was defined as the ratio between the total root length and dry weight. The total root weight density (RWD) was defined as the ratio between the total dry weight of the root and the volume of the soil from which the roots were extracted. The root length density (RLD) was defined as the total root length divided by the volume.
Soil aggregate separation
The aggregates were separated by wet sieving because the stability index of soil aggregates obtained by wet sieving is more reproducible than that obtained by dry sieving (Ma et al., 2020). In this study, the soil aggregates were divided into five size classes (0.053–8 mm). Briefly, we immersed the air-dried soil in deionized water for 10 min and placed it in the aggregate analyzer (DM200-Ⅲ, China). We sieved the soil sample using sieves with meshes of 4, 2, 1, 0.25, and 0.053 mm and shook the sieves 50 times (3 cm amplitude). After sieving and drying (oven-drying at 60°C for 48 h), the aggregates were separated into five size classes (4–8, 2–4, 1–2, 0.25–1, and 0.053–0.25 mm). The particles in the 2–8 mm size class were defined as large macroaggregates, those in the 0.25–2 mm size class were small macroaggregates, and those in the 0.053–0.25 mm size class were microaggregates.
Soil aggregate stability was determined using the mean weight diameter (MWD) (Bissonnais et al., 2018) as follows:
where wi is the proportion of soil aggregates in the corresponding size class, n is the number of size classes, and Xi is the mean diameter of the ith size class (mm).
Soil organic matter fractionation
Soil particulate organic matter (POM) was separated using size fractionation and the method described by Cambardella and Elliott (1992). We used an electronic balance to weigh 20 g (<2 mm of air-dried soil), which was placed into a conical flask. We added 100 ml of 5 g L−1 sodium hexametaphosphate solution to the conical flask and shook it manually for 10 min, followed by shaking on a reciprocal shaker (180 rpm) for 18 h. The dispersed soil sample was passed through 250-μm and 53-μm sieves, and the remaining material in the sieve was rinsed thoroughly with distilled water. The organic matter with the soil that remained on the 250-μm mesh was defined as coarse particulate organic matter (c-POM), and the material on the 53-μm mesh was the fine particulate organic matter (f-POM). The remaining material (<53 μm) was defined as the soil with mineral-associated organic matter (MAOM) and mineral-associated organic nitrogen (MAON). It was rinsed thoroughly with distilled water to remove the dispersion solution. These fractions were then placed in a 60°C drying oven for 48 h and weighed. The C and N contents in the c-POM, f-POM, MAOM, and MAON were determined by an elemental analyzer (Vario EL III, Elementa, Germany).
Mycorrhizal colonization and total hyphae length
We calculated the mycorrhizal colonization as the root tips per root length under an anatomical microscope and estimated the mycorrhizal infection rate (MIR) of each seedling by calculating the number of mycorrhizal root tips in 200 randomly selected root tips.
We used the membrane filter technique (Wick et al., 2007) and measured the total length of the fungal hyphae under a UV irradiation microscope (Olympus BX61; Olympus Co., Tokyo, Japan) with 9,200 magnification. The details of measuring the hyphal length have been described by Chen et al. (2019) and Bloem et al. (1995).
Statistical analysis
The differences in the soil abiotic and biotic factors and the soil aggregates between the forest types were determined by a one-way ANOVA test with Duncan’s post hoc multiple comparisons. Pearson’s correlation coefficient was used to assess the correlation between the soil abiotic and biotic factors standardized by the Z-score using R 4.1.1 software. We used Akaike’s Information Criterion (AIC) to select the optimum multiple linear regression model describing the relationship between the binding agents and the MWD. The multiple regression analyses were performed using the “glmulti” function in R.
Results
Effects of forest type on soil and fine root factors
The three forest types exhibited significant differences in the soil parameters (SOC, TN, AP, pH, MBC, MBN, and MBP), fine root traits (root C, root N, RWD, and MD), and fungal traits (T-GRSP, EE-GRSP, mycelial length density, and MIR) (Table 1). SOC was significantly higher in the QF and PF than in the CF (p < 0.05). Similarly, the TN content was significantly higher in the QF than in the CF, whereas no difference was found in the TN content between the PF and CF. In contrast, the soil AP content of the CF was significantly higher than that of the QF and PF (p < 0.05). Soil pH significantly differed between the three forest types, with mean pH values ranging from 3.8 in the PF soil to 6.08 in the CF soil. The proportions of MBC and MBN were significantly different between the three forest types. The MBC and MBN contents were significantly higher in the QF and CF than in the PF (p < 0.05).
The root C and N contents of the QF were significantly higher than those of the CF and PF (p < 0.05). The RWD did not differ significantly between the three forests. The RLD of the QF was higher than that of the CF, but the MD of the CF was significantly higher than that of the QF and PF (p < 0.05). Additionally, the forest type influenced the fungal traits. The mycelial length density and MIR of the QF and PF were higher than those of the CF (p < 0.05). Moreover, the proportions of the T-GRSP and EE-GRSP in the QF soil were significantly higher than that of the CF soil (p < 0.05).
Soil aggregate distribution and mean weight diameter
The soil aggregate distribution significantly differed between the three types of forests (Figure 1). The proportion of macroaggregates accounted for more than 50% of the aggregates in all three forests. The highest proportion of aggregates with 4–8 mm size occurred in the QF, whereas the lowest was observed in the CF (p < 0.05). These proportions were 186% and 86% higher in the QF and PF, respectively, than in the CF. In contrast, the proportion of 0.053–1 mm aggregates was significantly higher (22% higher) in the CF than in the QF (p < 0.05). Furthermore, the soil aggregate stability index (MWD) had a range of 1.09–2.08 mm; it was significantly lower in the CF soil (p < 0.05), whereas the highest MWD value occurred in the QF soil (90.8% higher than in the CF).
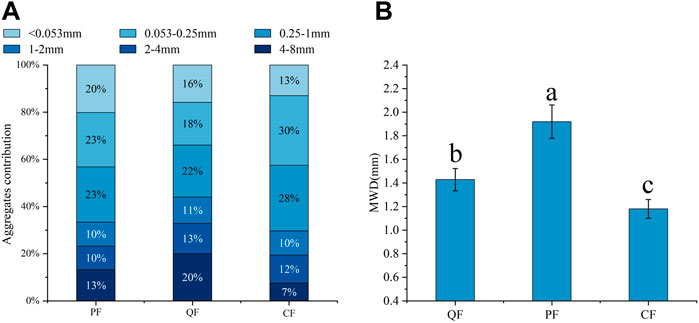
FIGURE 1. Particle size composition of different forest types. Note: lowercase letters indicate a significant difference in the MWD between different forest types. Different blue gradient indicate the number of aggregates with different particle sizes.
Effects of forest type on soil organic carbon/nitrogen fractions
The SOC content ranged from 25.09 to 32.65 g kg−1 at depths of 0–10 cm. The QF and PF had the highest SOC content; it was significantly higher (32.88% and 16.24%, respectively) than in the CF soil. The TN contents (1.28–2.37 g kg−1) showed a similar trend as the SOC in the three forests (Table 1). Similarly, the c-POM content (5.51–14.33 g kg−1) was significantly higher in the QF than in the PF and CF (Figure 1). The c-PON content exhibited the same pattern in the three forests (Figure 1). In contrast, the MAOM and MAON contents showed no significant differences between the three forests (Figure 1). The highest MBC and MBN contents occurred in the QF, followed by the CF and the PF. The soil MBP ranged from 0.60 to 5.12 g kg−1 and was significantly lower in the CF soil than in the PF and QF soils.
Effects of soil factors on soil aggregate stability and organic carbon content
Positive correlations were observed between the MWD and soil particulate organic carbon (POC) fraction for all forest types (Figure 2). Furthermore, the MWD was also correlated with the fungal traits. SOC and POM (c-POM, f-POM) contents were significantly positively correlated with mycelial length density, MIR, and N availability (p < 0.05), whereas no significant correlation was found between the MAOM content and the soil factors (Figure 2). The results of the multiple regression analyses are shown in Supplementary Figure S1. The best fit model was MWD = 1+c-POM (AICc = 16.54, weight = 38.70%), demonstrating that c-POM was the most important factor affecting MWD (>80%). The pH and fungal traits had importance values of 24% and 15%, respectively, whereas the root trait had less importance (approximately 10%). SOC and MBC had equal importance.
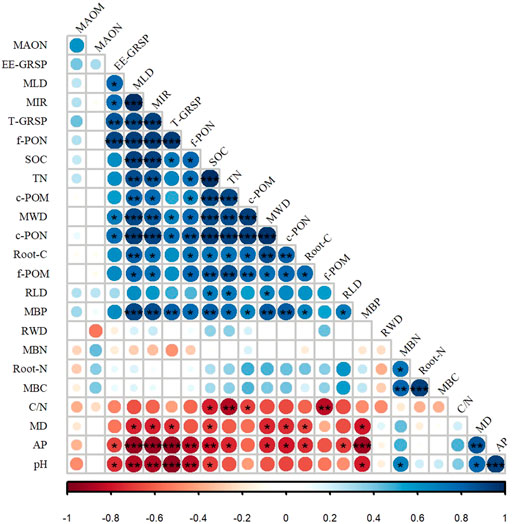
FIGURE 2. Pearson’s correlation coefficients between biological, abiotic factors, and mean weight diameter (MWD). MAOM: mineral-associated organic matter; MAON: mineral-associated organic nitrogen; EE-GRSP: easily extractable glomalin-related soil proteins. MLD: mycelial length density; MIR: mycorrhizal infection rate. T-GRSP: total glomalin-related soil proteins. f-PON: fine particulate organic nitrogen; SOC: soil organic carbon; TN: soil total nitrogen; c-POM: coarse particulate organic matter; c-PON: coarse particulate organic nitrogen; root-C: carbon content in the fine roots; f-POM: fine particulate organic matter; RLD: root length density; MBP: microbial biomass phosphorus; RWD: total root weight density; MBN: microbial biomass nitrogen; root-N: nitrogen content in the fine roots; MBC: microbial biomass carbon; C/N: the ratio of carbon and nitrogen; MD: mean root diameter; and AP: available phosphorus. The asterisks indicate significant correlations. Blue represents a positive correlation, and red represents a negative correlation.
Discussion
The effect of forest type on soil aggregates
The highest MWD occurred in the natural forest (Quercus variabilis and Pinus massoniana forests) because the soil had more aggregate binding agents, especially for the fungal traits, SOC, and POM. This result is consistent with the first hypothesis. The soil in these three forests was dominated by macroaggregates (0.25–8 mm aggregates >50%), and the stability of the soil aggregates (MWD) was in the range of 1.09–2.08 mm. These results were consistent with those of a previous study (Lu et al., 2012; Lan et al., 2022). The ANOVA results revealed significant differences (p < 0.05) in the macroaggregates (4–8 mm) between the natural forest and the planted forest (Figure 1; Supplementary Table S1). The higher proportions of macroaggregates in the natural forest may be attributed to the fungal nutrient preference. Copiotrophs are more abundant in macroaggregates, whereas oligotrophs are more abundant in microaggregates (Trivedi et al., 2017). The species diversity is higher in a secondary forest than in a planted forest, resulting in higher diversity of organic matter in the soil (Dou et al., 2019). The MBP content was significantly higher in the natural forest than in the planted forest. The phosphorus absorption strategy of microorganisms may be one reason for the difference between the fungal hyphae in the natural and planted forests because there was a significant correlation between the MBP content and fungal hyphae. Soil phosphorus is a relatively stable substance. Fungi obtain nutrients from more active unstable organic matter rather than from clay minerals with higher phosphorus content (Keyes et al., 2022). Organic matter, such as litter and fine root exudates, provides nutrients for copiotroph microorganisms. Additionally, the fungal traits, including the T-GRSP content, EE-GRSP content, mycelial length density, and MIR, were important factors affecting the stability of aggregates (Yuan et al., 2022). Microaggregates are formed first, and hyphae and GRSP cluster microaggregates into macroaggregates (Bavel, 1950; Zhang et al., 2019). Natural forests have more abundant organic matter than planted forests; therefore, the values of the fungal traits were significantly higher in natural secondary forests than in planted forests (Xie et al., 2013), resulting in higher contents of macroaggregates in natural secondary forests.
Aggregate stability is also related to soil fragmentation (Zhang et al., 2021; Spohn and Giani, 2010). The higher MD in the CF might have disrupted the macroaggregates and affected aggregate stability, although a positive effect of tree roots on aggregate formation was found in previous research (Li et al., 2020). In addition, the pH had a high importance value in predicting the MWD (Fig. S1) but did not directly affect aggregate stability. The pH was significantly negatively correlated with the fungal traits, including mycelial length density, MIR, and the T-GRSP and EE-GRSP contents, indirectly affecting aggregate stability. In summary, the fungal traits and SOC were important parameters predicting the MWD in different forest types. These findings suggest that SOC and fungal traits have a larger influence than other soil properties based on different thresholds of the aggregate stability, i.e., MWD, in a tropical–warm temperate transition zone.
The effects of forest type on SOC fractions
Many studies have investigated SOC in different forest types and found that natural forests (native or secondary forests) had higher carbon sequestration potential than planted forests (Jin et al., 2014; Hua et al., 2022; Jin et al., 2022). Similarly, in this study, the SOC content was higher in the natural forest (QF and PF) soils than in the planted forest (CF) soil (Table 1), consistent with the second hypothesis. Furthermore, we investigated the SOC components, including c-POM, f-POM, and MAOM, in the three forest types (Figure 3). The result showed no significant difference in the MAOM and f-POM contents between the three forest types (Figure 4). In contrast, the c-POM content was significantly higher in the QF than in the CF, indicating that c-POM comprised the largest proportion of SOC in the QF. Studies have shown that the POC content in the soil is primarily affected by the decomposition of POC and the input of plant residues (Puget et al., 1998). In multispecies stands, the litter species, fine root exudates, and other diverse types of organic carbon supplement the soil (Villarino et al., 2021; Liu et al., 2020). The positive effects of different kinds of organic matter input on soil carbon were mainly the result of interspecific competition.
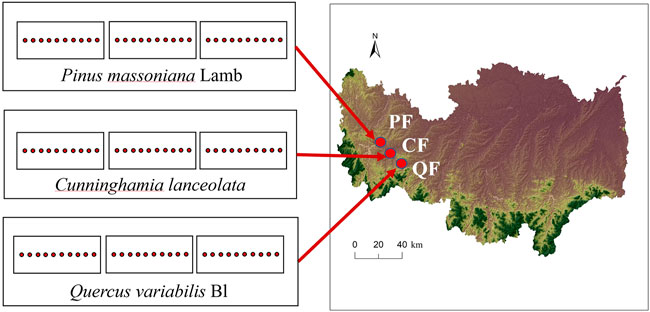
FIGURE 3. Map of the sampling location and the sampling scheme. QF, Quercus variabilis forest; PF, Pinus massoniana forest; and CF, Cunninghamia lanceolata forest. Each site has three random plots with 10 sampling sites in each plot.
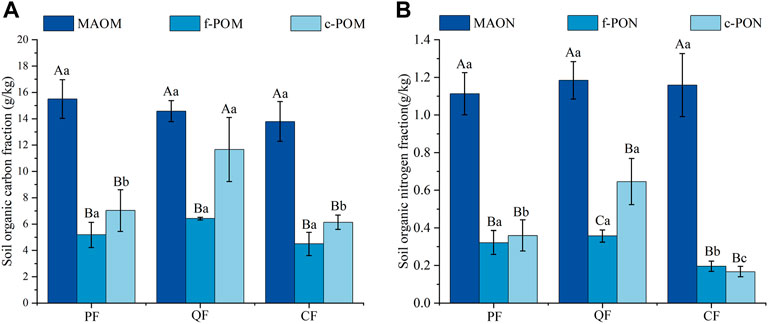
FIGURE 4. Fractions of organic C (A) and N (B) in soils under various types of forests. Note: capital (uppercase) letters indicate significant differences in the particle compositions (components) between the different forest types.
Another reason for the higher SOC in natural forests than in plantations may be a difference in the carbon content in the fine roots and microorganism community. We found a significant positive correlation between the carbon content in the fine roots or fungal hyphae (mycelial length density, MIR) and each of the SOC, c-POM, and f-POM contents (Figure 2). It has become increasingly clear that the amount of SOC inputs from fine roots are significantly larger than those from aboveground litter inputs (Xia et al., 2015) because root litter contains more carbon with a complex structure and is more difficult to decompose than leaf litter. Therefore, it may contribute more to POM. The higher root carbon in the natural forests than in the planted forest suggests that the SOC content was higher in the natural forests than in the planted forests because of the higher carbon content in the fine roots. In addition, the microbiome in forest soils is controlled by the soil environment. Thus, the abundance and activity of microorganisms determine the pathway of C from early-stage plant litter residues to persistent SOC (Witzgall et al., 2021). The POC content in the soil increases when the plant residue input exceeds the decomposition rate (Midwood et al., 2021). Cunninghamia lanceolata forests have less litter than forests with other tree species, such as Quercus variabilis (Gale et al., 2000; Sharma and Acharya, 2000; Leifheit et al., 2014), and have a less favorable environment for mycelial growth than secondary forests (Huang et al., 2010; Zhang et al., 2019; Lan et al., 2021). Unlike the MBC and MBN content, the MBP content was significantly correlated with the SOC content and the fungal hyphae because plants can obtain phosphorus through root epidermal cells and root hairs and through fungal hyphae (Zhang et al., 2022). In this study, natural forests had more fungal hyphae than planted forests, affecting the phosphorus acquisition strategy. These conditions may have led to more fungal hyphae to supplement SOC. In summary, the higher mycelial length density and MIR in the PF resulted in higher c-POM content in the soil, increasing SOC storage. Our results provide new insights into the potential effects of fungal indicators and root traits in the forest topsoil on SOC, soil aggregate stability, and carbon sequestration capacity.
Conclusion
Our study compared the effects of biotic and abiotic indicators of different forest types on soil carbon composition and aggregate stability. The results showed significant differences in SOC content and MWD between the three dominant forest types in the mountainous regions of China’s warm temperate–subtropical climate transition zone. The SOC content and MWD were the highest in the Quercus variabilis forest. The reason for the significantly higher POC content and MWD in the natural secondary forest than in the planted forest was that the fungal traits, including the T-GRSP and EE-GRSP contents, mycelial length density, and MIR, were significantly higher in the natural forest than in the planted forest. This study also highlights the crucial role of arbuscular mycorrhizal fungal hyphae and fine roots in increasing aggregate stability (Spohn and Giani, 2010; Zhang and Xie, 2019; Liu et al., 2020; Witzgall et al., 2021).
Data availability statement
The original contributions presented in the study are included in the article/Supplementary Material; further inquiries can be directed to the corresponding authors.
Author contributions
ZC, YM, and SH conceived and designed the research. XD, KZ, and JW processed the data. XD analyzed the data and wrote the manuscript. HG and YX reviewed the manuscript. All authors contributed to the article and approved the submitted version.
Funding
This research was financially supported by the National Natural Science Foundation of China (Grant numbers 41930643, 42107225, and 41807330), major public welfare projects in Henan Province (201300311300), and the Natural Science Foundation of Henan Province (No. 202300410082).
Acknowledgments
The authors wish to acknowledge Yanfeng Sun and Dong Wang for their help with reviewing, funding the experiment and providing data for this article.
Conflict of interest
The authors declare that the research was conducted in the absence of any commercial or financial relationships that could be construed as a potential conflict of interest.
Publisher’s note
All claims expressed in this article are solely those of the authors and do not necessarily represent those of their affiliated organizations, or those of the publisher, the editors, and the reviewers. Any product that may be evaluated in this article, or claim that may be made by its manufacturer, is not guaranteed or endorsed by the publisher.
Supplementary material
The Supplementary Material for this article can be found online at: https://www.frontiersin.org/articles/10.3389/fenvs.2022.1052175/full#supplementary-material
SUPPLEMENTARY FIGURE S1 | Model-averaged importance of the predictors of MWD. The importance is based on the sum of Akaike weights derived from model selection using the corrected AIC. Cutoff is set at 0.8 (red dashed line) to differentiate among the most important predictors.
References
An, S. S., Mentler, A., Mayer, H., and Blum, W. E. H. (2010). Soil aggregation, aggregate stability, organic carbon and nitrogen in different soil aggregate fractions under forest and shrub vegetation on the Loess Plateau, China. Catena 81, 226–233. doi:10.1016/j.catena.2010.04.002
Bai, Y. X., Zhou, Y. C., and He, H. Z. (2020). Effects of rehabilitation through afforestation on soil aggregate stability and aggregate-associated carbon after forest fires in subtropical China. Geoderma 376, 114548. doi:10.1016/j.geoderma.2020.114548
Bavel, C. (1950). Mean weight-diameter of soil aggregates as a statistical index of aggregation. Soil. Sci. Soc. Am. J. 4, 20–23. doi:10.2136/sssaj1950.036159950014000C0005x
Bissonnais, Y. L., Prieto, I., Roumet, C., Nespoulous, J., Metayer, J., Huon, S., et al. (2018). Soil aggregate stability in mediterranean and tropical agro-ecosystems: Effect of plant roots and soil characteristics. Plant Soil 424, 303–317. doi:10.1007/s11104-017-3423-6
Bloem, J., Veninda, M., and Shepherd, J. (1995). Fully automatic determination of soil bacterium numbers, cell volumes, and frequencies of dividing cells by confocal laser scanning microscopy and image analysis. Appl. Environ. Microb. 61, 926–936. doi:10.1128/Aem.61.3.926-936.1995
Bonifacio, E., Santoni, S., Falsone, G., and Zanini, E. (2006). Wet aggregate stability of some Botswana soil profiles. Arid. Land. Res. Manag. 20, 15–28. doi:10.1080/15324980500369418
Bouajila, A., and Gallali, T. (2010). Land use effect on soil and particulate organic carbon, and aggregate stability in some soils in Tunisia. Afr. J. Agr. Res. 5, 764–774. doi:10.5897/AJAR10.183
Cai, H. Y., Li, F. R., and Jin, G. Z. (2019). Fine root biomass, production and turnover rates in plantations versus natural forests: Effects of stand characteristics and soil properties. Plant Soil 436, 463–474. doi:10.1007/s11104-019-03948-8
Cambardella, C. A., and Elliott, E. T. (1992). Particulate soil organic-matter changes across a grassland cultivation sequence. Soil. Sci. Soc. Am. J. 56, 777–783. doi:10.2136/sssaj1992.03615995005600030017x
Chen, G. P., Gao, Z. Y., Zu, L. H., Tang, L. L., Feng, X. M., Zhao, T. G., et al. (2017). Soil aggregate characteristics and stability of soil carbon stocks in a Pinus tabulaeformis plantation. New For. 48, 837–853. doi:10.1007/s11056-017-9600-x
Chen, Y., Liu, X., Hou, Y. H., Zhou, S. R., and Zhu, B. (2019). Particulate organic carbon is more vulnerable to nitrogen addition than mineral-associated organic carbon in soil of an alpine meadow. Plant Soil 458, 93–103. doi:10.1007/s11104-019-04279-4
Dou, Y. X., Yang, Y., An, S. S., and Zhu, Z. L. (2019). Effects of different vegetation restoration measures on soil aggregate stability and erodibility on the Loess Plateau, China. Catena 185, 104294. doi:10.1016/j.catena.2019.104294
Feng, Y., Han, S. J., Wei, Y. Q., Xiao, J. G., Geng, S. C., Gu, Y., et al. (2020). Comparative study of lignin stabilizing mechanisms in soil aggregates at virgin mixed broadleaf-pine forest and secondary broadleaf forest at Changbai Mountain Nature Reserve, Northeast China. Ecol. Indic. 117, 106665. doi:10.1016/j.ecolind.2020.106665
Gale, W. J., Cambardella, C. A., and Bailey, T. B. (2000). Root-derived carbon and the formation and stabilization of aggregates. Soil. Sci. Soc. Am. J. 64, 201–207. doi:10.2136/sssaj2000.641201x
He, Y. H., Cheng, W. X., Zhou, L. Y., Shao, J. J., Liu, H. Y., Zhou, H. M., et al. (2020). Soil DOC release and aggregate disruption mediate rhizosphere priming effect on soil C decomposition. Soil. Biol. biochem. 144, 107787. doi:10.1016/j.soilbio.2020.107787
Holátko, J., Brtnick, M., Kuerík, J., Kotianova, M., Elbl, J., Kintl, A., et al. (2020). Glomalin-Truths, myths, and the future of this elusive soil glycoprotein. Soil. Biol. biochem. 153, 108116. doi:10.1016/j.soilbio.2020.108116
Hua, F. Y., Bruijnzeel, L. A., Meli, P., Martin, P. A., Zhang, J., Nakagawa, S., et al. (2022). The biodiversity and ecosystem service contributions and trade-offs of forest restoration approaches. Science 376, 839–844. doi:10.1126/science.abl4649
Huang, L., Wang, C. Y., Tan, W. F., Hu, H. Q., Cai, C. F., and Wang, M. K. (2010). Distribution of organic matter in aggregates of eroded Ultisols, Central China. Soil. till. Res. 108, 59–67. doi:10.1016/j.still.2010.03.003
Jastrow, J. D., Amonette, J. E., and Bailey, V. L. (2007). Mechanisms controlling soil carbon turnover and their potential application for enhancing carbon sequestration. Clim. Change 80, 5–23. doi:10.1007/s10584-006-9178-3
Jin, J., Xiang, W. H., Ouyang, S., Zhou, X. L., Hu, Y. T., Zhao, Z. H., et al. (2022). Stand carbon storage and net primary production in China’s subtropical secondary forests are predicted to increase by 2060. Carbon. bal. manage. 17, 6–14. doi:10.1186/s13021-022-00204-y
Jin, Z., Dong, Y. S., Wang, Y., Wei, X. R., Wang, Y. F., Cui, B. L., et al. (2014). Natural vegetation restoration is more beneficial to soil surface organic and inorganic carbon sequestration than tree plantation on the Loess Plateau of China. Sci. Total. Environ. 485, 615–623. doi:10.1016/j.scitotenv.2014.03.105
Joergensen, R. G., and Mueller, T. (1996). The fumigation-extraction method to estimate soil microbial biomass: Calibration of the kEC value. Soil. Biol. biochem. 28, 25–31. doi:10.1016/0038-0717(95)00102-6
Keyes, S., Veelen, A. V., Dan, M., Scotson, C., Koebernick, N., Petroselli, C., et al. (2022). Multimodal correlative imaging and modelling of phosphorus uptake from soil by hyphae of mycorrhizal fungi. New Phytol. 234, 688–703. doi:10.1111/nph.17980
Lan, J. C., Long, Q. X., Huang, M. Z., Jiang, Y. X., and Hu, N. (2022). Afforestation-induced large macroaggregate formation promotes soil organic carbon accumulation in degraded karst area. For. Ecol. Manag. 505, 119884. doi:10.1016/j.foreco.2021.119884
Lan, T. Y., Gu, J. C., and Wen, Z. H. (2021). Spatial distribution characteristics of carbon storage density in typical mixed fir and broadleaf forests. Energy. Rep. 7, 7315–7322. doi:10.1016/j.egyr.2021.10.094
Leifheit, E. F., Veresoglou, S. D., Lehmann, A., Morris, E. K., and Rillig, M. C. (2014). Multiple factors influence the role of arbuscular mycorrhizal fungi in soil aggregation-a meta-analysis. Plant Soil 374, 523–537. doi:10.1007/s11104-013-1899-2
Li, J., Yuan, X., Ge, L., Li, Q., Li, Z. G., Wang, L., et al. (2020). Rhizosphere effects promote soil aggregate stability and associated organic carbon sequestration in rocky areas of desertification. Agr. Ecosyst. Environ. 304, 107126. doi:10.1016/j.agee.2020.107126
Liu, K., Xu, Y. Z., Feng, W. T., Zhang, X. F., Yao, S. H., Zhang, B., et al. (2020). Modeling the dynamics of protected and primed organic carbon in soil and aggregates under constant soil moisture following litter incorporation. Soil. Biol. biochem. 151, 108039. doi:10.1016/j.soilbio.2020.108039
Lu, L. X., Song, T. Q., Peng, W. X., Zeng, F. P., Wang, K. L., Xu, Y. L., et al. (2012). Profile distribution of soil aggregates organic carbon in primary forests in Karst cluster-peak depression region. Chin. J. Appl. Ecol. 23 (5), 1167–1174. (in Chinese). doi:10.13287/j.1001-9332.2012.0161
Ma, L., Wang, Q., and Shen, S. T. (2020). Response of soil aggregate stability and distribution of organic carbon to alpine grassland degradation in Northwest Sichuan. Geoderma. Reg. 22, e00309. doi:10.1016/j.geodrs.2020.e00309
Merino-Martín, L., Stokes, A., Gweon, H. S., Moragues-Saitua, L., Staunton, S., Plassard, C., et al. (2020). Interacting effects of land use type, soil microbes and plant traits on aggregate stability. Soil. Biol. biochem. 154, 1–19. doi:10.1016/j.soilbio.2020.108072
Midwood, A. J., Hannam, K. D., Gebretsadikan, T., Emde, D., and Jones, M. D. (2021). Storage of soil carbon as particulate and mineral associated organic matter in irrigated woody perennial crops. Geoderma 403, 115185. doi:10.1016/j.geoderma.2021.115185
Morris, E. K., Morris, D. J. P., Voget, S. C., Gleber, S. C., Bigalke, M., Wilcke, W., et al. (2019). Visualizing the dynamics of soil aggregation as affected by arbuscular mycorrhizal fungi. Isme. J. 13, 1639–1646. doi:10.1038/s41396-019-0369-0
Peng, X. H., Zhu, Q. H., Zhang, Z. B., and Hallett, P. D. (2017). Combined turnover of carbon and soil aggregates using rare Earth oxides and isotopically labelled carbon as tracers. Soil. Biol. biochem. 109, 81–94. doi:10.1016/j.soilbio.2017.02.002
Pierret, A., Gonkhamdee, S., Jourdan, C., and Maeght, J. L. (2013). IJ_Rhizo: An open-source software to measure scanned images of root samples. Plant Soil 373, 531–539. doi:10.1007/s11104-013-1795-9
Puget, P., Angers, D. A., and Chenu, C. (1998). Nature of carbohydrates associated with water-stable aggregates of two cultivated soils. Soil. Biol. biochem. 31, 55–63. doi:10.1016/S0038-0717(98)00103-5
Qin, H., Chen, J. H., Wu, Q. F., Niu, L. M., Li, Y. C., Liang, C. F., et al. (2017). Intensive management decreases soil aggregation and changes the abundance and community compositions of arbuscular mycorrhizal fungi in Moso bamboo (Phyllostachys pubescens) forests. For. Ecol. Manag. 400, 246–255. doi:10.1016/j.foreco.2017.06.003
Sharma, P. K., and Acharya, C. L. (2000). Carry-over of residual soil moisture with mulching and conservation tillage practices for sowing of rainfed wheat (Triticum aestivum L.) in north-west India. Soil. till. Res. 57, 43–52. doi:10.1016/S0167-1987(00)00141-0
Spohn, M., and Giani, L. (2010). Water-stable aggregates, glomalin-related soil protein, and carbohydrates in a chrono sequence of sandy hydromorphic soils. Soil. Biol. biochem. 42, 1505–1511. doi:10.1016/j.soilbio.2010.05.015
Trivedi, P., Delgado-Baquerizo, M., Jeffries, T. C., Trivedi, C., Anderson, I. C., Lai, K., et al. (2017). Soil aggregation and associated microbial communities modify the impact of agricultural management on carbon content. Environ. Microbiol. 19, 3070–3086. doi:10.1111/1462-2920.13779
Villarino, S. H., Pinto, P., Jackson, R. B., and Piñeiro, G. (2021). Plant rhizodeposition: A key factor for soil organic matter formation in stable fractions. Sci. Adv. 7, eabd3176. doi:10.1126/sciadv.abd3176
Wick, L. Y., Remer, R., Wurz, B., Reichenbach, J., Braun, S., Scharfer, F., et al. (2007). Effect of fungal hyphae on the access of bacteria to phenanthrene in soil. Environ. Sci. Technol. 41, 500–505. doi:10.1021/es061407s
Witzgall, K., Vidal, A., Schubert, D. I., Hoschen, C., Schweizer, S. A., Buegger, F., et al. (2021). Particulate organic matter as a functional soil component for persistent soil organic carbon. Nat. Commun. 12, 4115. doi:10.1038/s41467-021-24192-8
Wright, S. F., and Upadhyaya, A. (1996). Extraction of an abundant and unusual protein from soil and comparison with hyphal protein of arbuscular mycorrhizal fungi. Soil. Sci. 161, 575–586. doi:10.1097/00010694-199609000-00003
Xia, M., Talhelm, A. F., and Pregitzer, K. S. (2015). Fine roots are the dominant source of recalcitrant plant litter in sugar maple-dominated northern hardwood forests. New Phytol. 208, 715–726. doi:10.1111/nph.13494
Xie, J. S., Guo, J. F., Yang, Z. J., Huang, Z. Q., Chen, G. S., and Yang, Y. S. (2013). Rapid accumulation of carbon on severely eroded red soils through afforestation in subtropical China. For. Ecol. Manag. 300, 53–59. doi:10.1016/j.foreco.2012.06.038
Yang, Y. S., Guo, J. F., Chen, G. S., Yin, Y. F., and Lin, C. F. (2009). Effects of forest conversion on soil labile organic carbon fractions and aggregate stability in subtropical China. Plant Soil 323, 153–162. doi:10.1007/s11104-009-9921-4
Yuan, Y., Li, Y., Mou, Z. J., Kuang, L. H., Wu, W. J., Zhang, J., et al. (2022). Phosphorus addition decreases microbial residual contribution to soil organic carbon pool in a tropical coastal forest. Glob. Change. Biol. 27, 454–466. doi:10.1111/gcb.15407
Zeng, Q. C., Darboux, F., Man, C., Zhu, Z. L., and An, S. S. (2018). Soil aggregate stability under different rain conditions for three vegetation types on the Loess Plateau (China). Catena 167, 276–283. doi:10.1016/j.catena.2018.05.009
Zhang, G. H., and Xie, Z. F. (2019). Soil surface roughness decay under different topographic conditions. Soil. till. Res. 187, 92–101. doi:10.1016/j.still.2018.12.003
Zhang, H., Zhou, G. M., Wang, Y. X., Bai, S. B., Sun, Z. B., Berninger, F., et al. (2019). Thinning and species mixing in Chinese fir monocultures improve carbon sequestration in subtropical China. Eur. J. For. Res. 138, 433–443. doi:10.1007/s10342-019-01181-7
Zhang, P. P., Wang, Y. Q., Xu, L., Li, R. J., Sun, H., and Zhou, J. X. (2021). Factors controlling spatial variation in soil aggregate stability in a semi-humid watershed. Soil. till. Res. 214, 105187. doi:10.1016/j.still.2021.105187
Keywords: soil aggregate stability, organic carbon, different forest types, particle organic carbon, fungal traits
Citation: Dong X, Zhao K, Wang J, Gui H, Xiao Y, Chen Z, Miao Y and Han S (2023) Effects of forest types on soil carbon content in aggregate faction under climate transition zone. Front. Environ. Sci. 10:1052175. doi: 10.3389/fenvs.2022.1052175
Received: 23 September 2022; Accepted: 12 December 2022;
Published: 09 January 2023.
Edited by:
Tiequan Zhang, Harrow Research and Development Centre, CanadaReviewed by:
Mengyang You, Northeast Institute of Geography and Agroecology (CAS), ChinaWenbing Tan, Chinese Research Academy of Environmental Sciences, China
Copyright © 2023 Dong, Zhao, Wang, Gui, Xiao, Chen, Miao and Han. This is an open-access article distributed under the terms of the Creative Commons Attribution License (CC BY). The use, distribution or reproduction in other forums is permitted, provided the original author(s) and the copyright owner(s) are credited and that the original publication in this journal is cited, in accordance with accepted academic practice. No use, distribution or reproduction is permitted which does not comply with these terms.
*Correspondence: Zhijie Chen, emhqaWUxOTg4QDEyNi5jb20=; Yuan Miao, bWlhb3l1YW4wOTIxQDEyNi5jb20=; Shijie Han, aGFuc2pAaWFlLmFjLmNu
†These authors have contributed equally to this work