- 1Department of Horticulture, College of Agriculture, G.B. Pant University of Agriculture and Technology, Pantnagar, India
- 2School of Agriculture, ITM University, Gwalior, India
- 3College of Science, University of Al-Qadisiyah, Al Diwaniyah, Iraq
- 4Civil Environmental and Natural Resources Engineering, Lulea University of Technology, Lulea, Sweden
- 5Department of Irrigation and Drainage Engineering College of Technology, G.B. Pant University of Agriculture and Technology, Pantnagar, India
- 6Department of Zoology, College of Science, King Saud University, Riyadh, Saudi Arabia
- 7Department of Plant Protection, College of Horticulture and Forestry, Agriculture University, Kota, India
Drip irrigation and mulching are often used to alleviate the problem of poor water management in many crops; however, these technologies have not yet been tested for applying water at critical stages of guava orchard growth in subtropical humid Tarai regions of India to improve the yield and quality. A field experiment was conducted over 2020 and 2021 which included three irrigation strategies: severe deficit irrigation (DI50), moderate deficit irrigation (DI75), and full irrigation (FI100), as well as four mulching methods: silver-black mulch (MSB), black mulch (MB), organic mulch (MOM), and a control without mulch (MWM). The results showed that both the relative leaf water content (RLWC) and the proline content exhibited an increasing trend with a decrease in the irrigation regime, resulting in a 123% increase in the proline content under DI50 conditions compared with FI100, while greater plant growth was recorded in fully irrigated plants and using silver-black mulch. Leaf nutrient analysis showed that FI100 and MOM produced significantly higher concentrations of all nutrients. However, moderate deficit irrigation (DI75) along with silver-black mulch (MSB) produced higher numbers of fruits per plant, higher average fruit weights, higher fruit yields, and maximum ascorbic acid contents. The irrigation water productivity (IWP) decreased with an increase in the irrigation regime; from severe water deficit to full irrigation, resulting in a 33.79% improvement in IWP under DI50 conditions as compared with FI100. Regression analysis outperforms principal component regression analysis for fruit yield prediction, with adjusted R2 = 89.80%, RMSE = 1.91, MAE = 1.52, and MAPE = 3.83. The most important traits affecting the fruit yield of guava, based on stepwise regression, were leaf proline, leaf Cu, fruit weight, and IWP.
1 Introduction
Guava (Psidium guajava L.) is one of the most important fruit crops and is widely cultivated in tropical and sub-tropical regions of the world. Guava is known as the “Apple of the tropics” because it is the only tropical fruit which is as nutritionally beneficial as the apple (Khan et al., 2013; Nimisha et al., 2013; Takeda et al., 2022). Over the past two decades, guava land areas and their production have increased at a tremendous pace as the demand for fruits has increased due to their nutritional superiority and affordable prices (Preet et al., 2021). Guava land areas, production, and productivity have increased from 1.55 Mha, 17.15 MT, and 11.10 t/ha, respectively, in 2001 to 2.92 Mha, 43.61 MT, and 14.93 t/ha in 2019–20 (Indiastat-focused on facts). This indicates the mounting importance of this fruit crop as the land area has just less than doubled, with 2.5 times higher production (Anonymous, 2019). In the Uttarakhand plains, its land area has also increased, corresponding to a large area of India; however, the productivity (5.67 t/ha) is still very low as compared with the national average (Department of horticulture and food processing, 2018). The main factor behind the low productivity is poor orchard management practices, resulting in biotic and abiotic stresses (Joshi et al., 2012). Water stress during the critical stages of fruit growth and development is the main reason for poor productivity of guava (Usman et al., 2022). Water management, especially during the period of fruit maturation, plays an important role in improving the yield as well as the quality.
Water shortage is a major barrier in crop production in almost every region of the world (Shao et al., 2009; Bartlett et al., 2019; Kogan et al., 2019). The scarcity of fresh water resources has stimulated research into water-saving strategies in agriculture, with the aim to produce more crop per drop (Stefanelli et al., 2010). India accounts for approximately 18% of the world’s population and contains 4% of the world’s fresh water, out of which 80% is used in agriculture. According to international criteria, a country is categorized as water-stressed and water-scarce if the per capita water availability falls below 1700 m3 and 1000 m3 respectively. India is already a water-stressed country, with 1544 m3 per capita water availability and is approaching the water-scarce category (Dhawan, 2017). Thus, the main challenge confronting both rain-fed and irrigated agriculture is to improve water use efficiency (WUE) and sustainable water use for agriculture (Berihun, 2011). The use of micro-irrigation systems was found to result in 30–70% water savings in various orchard crops, along with 10–60% increases in yields as compared with conventional methods of irrigation. It is prudent to make efficient use of water and to irrigate larger land areas using the available water resources. This can be achieved by introducing advanced methods of irrigation and improved water management practices (Zaman et al., 2001). In recent years, the implementation of deficit irrigation in various fruit crops has gained popularity due to its excellent influence on water savings, productivity, and produce quality (Galindo et al., 2018).
In order to maximize economic returns while using limited water, deficit irrigation (DI) is used and is the practice of providing irrigation below crop evapotranspiration (ETc) requirements (Fereres and Soriano, 2007). During various growth stages of the crop, reducing water delivery to the right requirements improves the water use efficiency and produce quality without significantly impacting the yield (Panigrahi et al., 2014). Certain studies have shown that crops can adapt to water shortages, and that a modest water shortage may not have a substantial impact on agricultural productivity (García-Tejero et al., 2010b; Zhong et al., 2019). Scholars have conducted extensive studies to assess the response of fruit tree development to deficit irrigation, most notably on apples (Zhong et al., 2019), almonds (López-López et al., 2018), oranges (Zapata-Sierra and Manzano-Agugliaro, 2017), grapes (Faci et al., 2014), and pear-jujubes (Cui et al., 2008). El Jaouhari et al. (2018) discovered that a moderate deficit irrigation (75% ETc) can increase the WUE and the quality parameters without sacrificing the yield; however, a severe deficit (50% ETc) was insufficient to maintain an acceptable fruit size.
Drip irrigation in combination with mulch is one of the best management practices to significantly improve the WUE. Mulching creates an isolating layer between the soil surface and the atmosphere, reducing water vapor interaction between the soil surface and the atmosphere (Zribi et al., 2015). Consequently, water evaporation from mulched soil is reduced compared with bare soil, resulting in more available water for beneficial crop transpiration (Sarkar and Singh, 2007; Hou et al., 2010). Surface mulches have been used to reduce the soil temperature and the wind velocity at the soil surface (Kay, 1978; Jalota and Prihar, 1990).
From a commercial standpoint, VNR Bihi is the dominant guava variety in India, since its larger-sized fruits fetch a decent price in both domestic and foreign markets. This variety’s cultivation is mostly limited to central and northern India, where 90% of the annual rainfall occurs in three to 4 months (June–September). Irrigation is mostly used during the fruiting season (September to January) to boost the water productivity and to increase the yield of larger fruits. VNR Bihi guava is, however, well-suited to drip irrigation. One of the key factors for sustaining guava production in this location is to schedule deficit watering with mulching.
Deficit irrigation and mulching have been examined in various field tests as water conservation and water-saving strategies. Furthermore, according to the current literature, there is no information comparing the influence of deficit irrigation with mulching on leaf physiological parameters, nutrient uptake, yield, fruit quality, and irrigation water productivity in guava. Therefore, this study was conducted to determine the best irrigation schedule for VNR Bihi guava, in terms of yield, leaf nutrient content, and irrigation water productivity.
2 Materials and methods
2.1 Experimental site
The field experiment was performed at the G. B. Pant University of Agriculture and Technology’s Horticulture Research Centre in Uttarakhand, India. The experimental site is located in the Himalayas (29.0 N, 79.5 E). The experiment was conducted on five-year-old guava trees cv. VNR Bihi (wedge grafted) planted at a spacing of 5 m × 3 m under a medium-to high-density planting scheme for two consecutive years (2019–20 and 2020–21). From the first year of planting, the trees were drip irrigated.
The climate of the experimental site has been categorized as sub-humid and sub-tropical with a hot and dry summer and an extremely cold winter. The details of the weather parameters recorded during the crop growth period are depicted in Figures 1A,B. The mean annual rainfall in this region is 1450 mm, out of which 70% occurs during the rainy season (July–September). The total rainfall was 1296.6 and 1252.8 mm during the years 2019–20 and 2020–21, respectively. The soil of the experimental site has been classified as Mollisol. The texture of the experimental soil was silt loam, with a neutral pH (7.1) and EC (0.38 ds/m), medium organic carbon content (0.67%), low available nitrogen (185.95 kg ha−1), and both medium available phosphorus (28.92 kg ha−1) and potassium (220.34 kg ha−1).
2.2 Treatments and layout
The experiment was carried out under natural field conditions in a Factorial Randomized Block Design (FRBD), with three replications comprised of two factors (deficit irrigation and mulching). A total of three irrigation regimes were designed, including severe deficit irrigation (DI50), moderate deficit irrigation (DI75), and full irrigation (FI100). The irrigation levels were applied on the basis of the crop-evapotranspiration requirement (ETc): DI50—deficit irrigation at 50% ETc, DI75—deficit irrigation at 75% Etc, and FI100—full irrigation at 100% ETc. Irrigation was applied mainly during the fruit growth period using a drip system. The water supply was stopped during the monsoon season (July–September) due to adequate rainfall fulfilling the crop water need during this period. Four mulching treatments were employed: silver-black mulch (MSB), black mulch (MB), organic mulch (MOM), and a control without mulch (MWM). Silver-black and black colored polyethylene mulches 100 microns thick and 1.2 m width were used as inorganic mulches (Figure 2A). A 10 cm thick organic mulch (rice straw) was applied uniformly in each replication in the experiment (Figure 2B). Various mulching treatments were given the same quantity of irrigation under the same water deficit schemes.
2.3 Irrigation scheduling and crop management practices
Every other day, four on-line 6 l h−1 pressure-compensated drip emitters per plant were installed on two 16 mm diameter lateral pipes to provide irrigation. The emitters were set at a distance of 1 m from the plant stem. Based on a 100% class-A pan evaporation rate, the water quantity applied during full irrigation (FI, 100% ETc) was estimated using the following formula:
where ETc is the crop evapotranspiration (mm/day), Kp is the pan coefficient (0.7), Ep is the 2-day cumulative pan evaporation (mm), and Kc is the crop coefficient (0.8 for no mulching and 0.56 for mulches). The Kc values decrease by an average of 10–30% due to the 50–80% reduction in soil evaporation under mulching (Allen et al., 1998). The volume of water applied by the drip irrigation system was estimated using the following relationship:
where V = the total amount of water applied (L/day/plant), Ep = the open pan evaporation (mm/day), Kp = the pan coefficient, Kc = the crop coefficient, Sp = the plant to plant spacing, Sr = the row to row spacing, Wp = the wetting factor, and ER = the effective rainfall. The irrigation efficiency of the drip was considered to be 90%. The effective rainfall was calculated monthly, based on the USDA, S.C.S. method (United States Department of Agriculture, Soil Conservation Services):
where ER = the effective rainfall (mm) and Pt = the total rainfall (mm).
The crop coefficient (Kc), rainfall, and irrigation applied during the irrigation season, under different irrigation treatments, are shown in Table 1. One pair leaf pruning was practiced in the last week of April to regulate rainy season flush and to optimize winter season flowering. Standard recommended doses of fertilizer, i.e., N:P2O5:K2O at 375:325:250 g/tree/year were applied during both years.
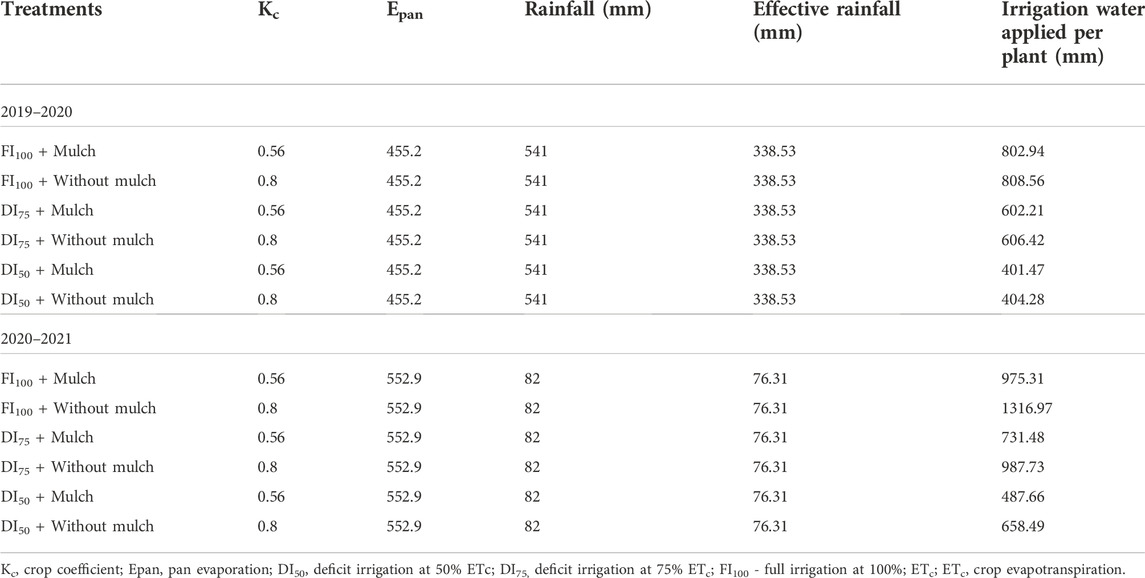
TABLE 1. The crop coefficient (Kc), rainfall and irrigation applied during irrigation season under different irrigation and mulching treatments.
2.4 Measurement and analysis
2.4.1 Leaf physiological parameters
For determining the relative leaf water content (RLWC), two leaves per plant in a similar position were cut from each shoot at midday. The RLWC was calculated using the formula given by Bowman (1989):
The proline content of the guava leaves was estimated according to the procedure of Bates et al. (1973). The total chlorophyll content was estimated in fresh guava leaves using the method described by Hiscox and Israelstam (1979). The total chlorophyll content was then calculated by using following formula:
where, A = the absorbance of chlorophyll extract at a specific indicated wavelength, V = the final volume of the sample, and W = the fresh weight of tissue extracted.
Towards the end of each irrigation season, fully expanded, mature leaves (without petioles) were collected from the plant canopy for each treatment and analyzed for macronutrients (N, P, and K) and micronutrients (Fe, Mn, Cu, and Zn). Two leaves displaying opposite phyllotaxy and emerging simultaneously were considered as a single leaf. As the majority of the shoots (95%) contained six leaf pairs, leaves from six different positions were sampled and indicated as leaf position I-VI from the base to the top. The leaves were spread in all four directions and were located at a height of 0.5–2 m above the soil level. The sample sizes consisted of 20 leaves per sample per replication. The leaf samples were thoroughly washed and dried at 65°C for 48 h. The dried samples were homogenously powdered and digested in a tri-acid mixture made up of two parts HClO4 + five parts HNO3 + one part H2SO4. Leaf acid extracts were analyzed for N using the modified micro-Kjeldahl method, P using the vanadomolybdo-phosphoric acid method, K using flame photometry, and micronutrients (Fe, Mn, Cu, and Zn) using an atomic absorption spectrophotometer (Model-908, GBC Scientific equipment, Australia).
The plant height, average plant spread (mean diameter of the canopy spread in E–W and N–S directions), and stem girth diameter (stem diameter measured 50 cm above the ground surface) were recorded annually. The plant canopy volume was calculated using the following formulae and was expressed in cubic meters (m3):
where, r = the radius of the plant canopy (m), h = the total height of the plant, and π = 3.14.
The numbers and weights of complete fruits collected from each experimental plant were recorded, and the mean yield per plant was computed by multiplying the number of fruits per plant by the average fruit weight undergoing the various treatments (Figure 4). The fruit lengths and fruit diameters were measured using a Digital Vernier’s Caliper for ten fruits/replication, randomly selected from tagged guava fruits grown under different treatments. The fruit yield per unit quantity of irrigation water applied was calculated to determine the irrigation water productivity (IWP). The ascorbic acid content of the guava fruits was determined by using a 2,6-dicholorophenol-indophenol visual titration method described by Ranganna (1986). Ten grams of pulp was weighed and crushed using a mortar and pestle. The juice was filtered from the crushed pulp into a 100 ml volumetric flask and the final volume was made up to 100 ml by adding 3% metaphosphoric acid (HPO3) solution. A 10 ml aliquot was taken from the obtained solution and was used for titration against the dye (2,6-dicholorophenol-indophenol) until a light pink color appeared and persisted for at least for 15 s (end point). The titer value was recorded, and the ascorbic acid content was calculated and expressed as mg/100 g of pulp:
2.5 Statistical analysis
Statistical analysis of two FRBDs was carried out by using the R package (Popat and Banakara, 2020). The means of the levels of significant factors were compared using Duncan’s new multiple range test (Tallarida and Murray, 1987). The graphs presented in this manuscript were prepared using the R package ggbiplot2 (Wickham, 2016). The Pearson correlation was determined for all variables in the study (Obilor and Amadi, 2018). The significance of correlation was tested using a t-test (n = 72). Stepwise regression analysis was performed by using the fruit yield (kg/plant) as the dependent variable, and remaining variables were used as independent variables. Residual analysis of the regression model was performed by using a run test (Siegel, 1988) and a Shapiro–Wilk test (Shapiro and Wilk, 1965) was used to test the assumptions of randomness and the normality of residuals. Goodness of fit statistics such as the RMSE (root mean squared error), MAE (mean absolute error), and MAPE (mean absolute percentage error) were determined for the regression model. All 20 variables were first subjected to principal component regression analysis using the R package prcomp. Among the principal components obtained, those with eigen values greater than 1 were used as independent variables and the yield was used as the dependent variable. All analyses were performed using R software, version 4.0.5 (Shukla et al., 2021; Elbeltagi et al., 2022).
3 Results and discussion
3.1 Leaf physiological parameters
The mean relative leaf water contents (RLWC) under different irrigation treatments were affected significantly (Figure 3A). The highest values for the RLWCs (81.35% and 81.10%) were observed for fully irrigated plants, whereas the lowest values were observed for plants under DI50 (67.75% and 66.58%), in 2019–20 and 2020–21, respectively. However, the lower values of the RLWC in 2020 compared with those in 2019 reflect the higher plant water status in the former year. The lower atmospheric evaporative demand, coupled with the better rainfall distribution in 2019 are probably responsible for the higher plant water potential in 2019.
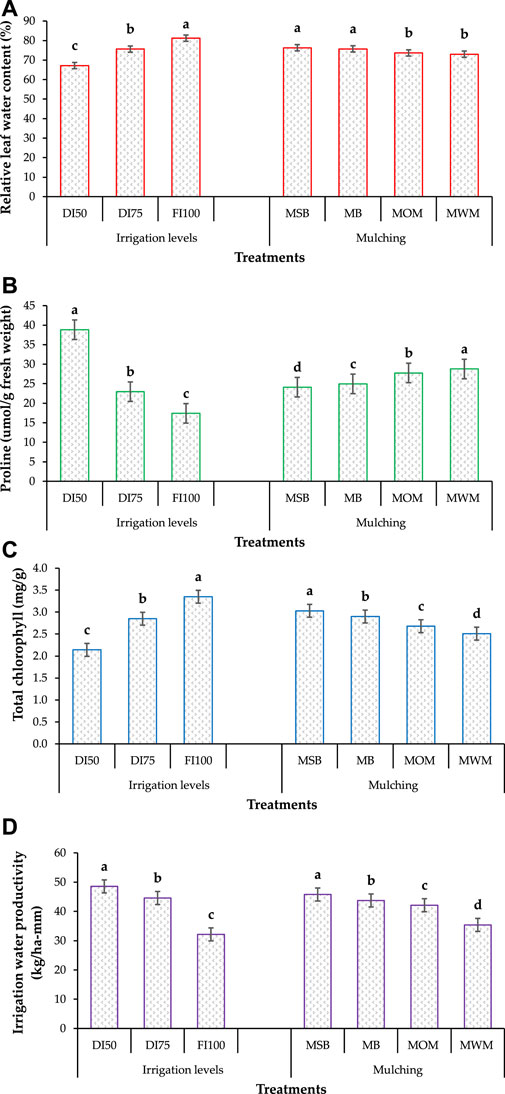
FIGURE 3. (A) Relative leaf water content (RLWC), (B) proline content, (C) total chlorophyll content and (D) irrigation water productivity influenced by irrigation levels and mulching. Different letters (A–D) indicate significantly different values at p ≤ 0.05 according to Duncan’s multiple range test. The vertical bar at each data point represents the standard error of mean.
The increased values of the RLWC indicates that the plant received its required water level to accomplish different plant physiological functions. The RLWC is an integrative index of the plant water status, and is used to evaluate the plant’s tolerance to water stress. A reduction in the RLWC under water stress leads to stomatal closure, resulting in decreased CO2 assimilation (Gindaba et al., 2004). Similar results were also reported by Abdel-Razik (2013) and Khalifa (2013) in mangos, and by Hamdy et al. (2016) in pomegranates; the authors reported that the plants produced low values of relative leaf water content in deficit irrigation conditions.
Different mulches also substantially influenced the RLWC of guava plants. The highest values for the RLWCs were observed with MSB, which was statistically at par with MB, whereas the lowest value was observed for MWM plants during both years of experimentation (Figure 3A). Moreover, interactive effects between deficit irrigation and mulching, with respect to the relative leaf water content, were significant during both years. Pooled data for the 2 years revealed that the highest value for the RLWC (82.31%) was observed with FI100MB (full irrigation at 100% ETc and with black mulch), whereas the lowest value of the RLWC (63.09%) was observed with plants under DI50MWM conditions (deficit irrigation at 50% ETc without mulch). These findings are in accordance with the findings of Pratima (Pratima, 2014), who reported significantly higher relative leaf water content in kiwifruits under deficit irrigation conditions coupled with black polyethylene mulching as compared with un-mulched conditions.
The proline accumulation in leaves significantly increased when plants were irrigated at DI50 in comparison with fully irrigated plants, over both years of the experiment (Figure 3B). During 2019–20, the average leaf proline content increased from 17.29 μmol/g fresh weight under FI100 conditions to 38.80 μmol/g fresh weight when plants were irrigated at DI50 conditions.
Similarly, in 2020–21, the leaf proline content increased from 17.52 μmol/g fresh weight in FI100 conditions to 38.88 μmol/g fresh weight under DI50 conditions. The proline content of leaves increased as the irrigation water decreased, implying that proline production is a common response of plants that are under water stress, as proline regulates cell osmotic equilibrium and protects against the harmful effects of water stress (Sampathkumar et al., 2014). Similar findings were also reported by Srikasetsarakul et al. (2011) in mangos and by Teixeira and Pereira (2007) in potatoes. Moreover, the minimum leaf proline content (24.05 μmol/g fresh weight) was recorded in MSB (silver-black) mulch, whereas the maximum (28.68 μmol/g fresh weight) in MWM (without mulch) plants. Similarly, in 2020–21, the minimum leaf proline content (24.17 μmol/g fresh weight) was recorded in MSB (silver-black) mulch, whereas the maximum (28.86 μmol/g fresh weight) in MWM (without mulch) plants. The interaction effect of deficit irrigation and mulching on the leaf proline contents was non-significant during both years.
Leaf chlorophyll content is a vital component for photosynthesis, and indicates the amount of photosynthates present in the plant system, which help to regulate plant growth. The total chlorophyll content was significantly affected by deficit irrigation and mulching treatments during both years of experimentation (Figure 3C). Pooled data indicated that plants irrigated at 100% ETc exhibited the highest chlorophyll content: 3.35 mg g−1, whereas the minimum (2.14 mg g−1) was recorded for DI50 conditions. The reduction in chlorophyll content with respect to water deficit may be due to the fact that photosynthetic pigments are very sensitive to drought stress, resulting in the destruction of chlorophyll. Under stressful conditions, glutamate, which is a fundamental source for both chlorophyll and proline formation, is thought to be primarily utilized for proline production, as a protectant suitable solute (Jaleel et al., 2009). Furthermore, during shortage irrigation, activation of the chlorophyllase enzyme can produce a drop in chlorophyll concentrations (Farooq et al., 2009).
Plants mulched with silver-black (MSB) registered significantly higher total leaf chlorophyll contents as compared with black mulch and MWM (without mulch) during both years (Figure 3C). The increased total chlorophyll might be due to the fact that the plastic mulching increased the soil microbial population along with the nitrogen absorption, consequently increasing the chlorophyll content of the plant leaves, as mentioned by Eissa (2002). The greater chlorophyll concentration in plants growing on polyethylene mulch could be due to a difference in chlorophyll synthesis and breakdown. Furthermore, the greater activity of the enzyme chlorophyllase could be related to the lower chlorophyll contents in non-mulched leaves. Differences in chlorophyll levels could also be attributable to differences in the degree of light reflection by the mulches (Pandey et al., 2016; Farooq et al., 2021, 2022; Kumar et al., 2022). Similar results were also reported by Deb et al. (2014), who recorded maximum total chlorophyll contents in polyethylene mulches in strawberries.
3.2 Leaf nutrient composition
The macronutrient (N, P, and K) and micronutrient (Cu, Mn, Zn, and Fe) concentrations in leaves responded differently to various irrigation regimes, according to leaf nutrient analyses (Tables 2, 3). The FI100 regime produced significantly higher leaf N, P, and K contents, which were statistically comparable to the DI75 condition, but significantly higher than the DI50 regime. The increased availability of such nutrients in the soil under FI100 resulted in higher N, P, and K contents in leaves of completely irrigated plants. The decrease in leaf nitrogen content under deficit irrigation conditions could be due to a decrease in the nitrogen solubility caused by soil water stress: the plant does not absorb enough nitrogen (Tahir et al., 2003). Similar results were also reported by Khattab et al. (2011); the leaf nitrogen percentage of pomegranates increased with increasing irrigation water levels compared with drought stress conditions, and the available N in the soil (NO3− and NH4+), nitrogen fixation, uptake, and nitrogen use efficiency were significantly reduced, leading to lower nitrogen accumulation in plants. These findings agree with the earlier studies of Panigrahi et al. (2012) on Kinnow mandarins, Gupta, (2019) on litchi, and Preet et al. (2021) on the response of guava to integrated nutrient and water management. Leaf N, P, and K contents also significantly differed when guava plants were mulched using silver-black, black, and organic mulch (rice straw) for two consecutive years. Furthermore, mulching with different types of mulches significantly influenced the leaf N, P, and K contents; a maximum was observed for MOM, followed by MSB, and a minimum for MWM over two consecutive years. The interaction effects of deficit irrigation and mulching had non-significant influences on the N, P, and K contents of leaves during both years of experimentation.
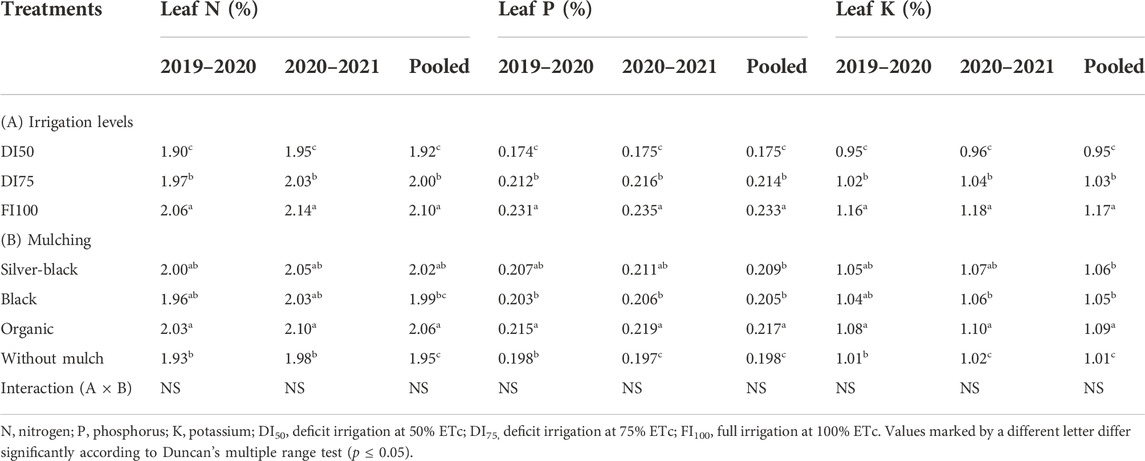
TABLE 2. Macronutrient (N, P and K) content in leaves of VNR Bihi guava influenced by irrigation levels and mulching.
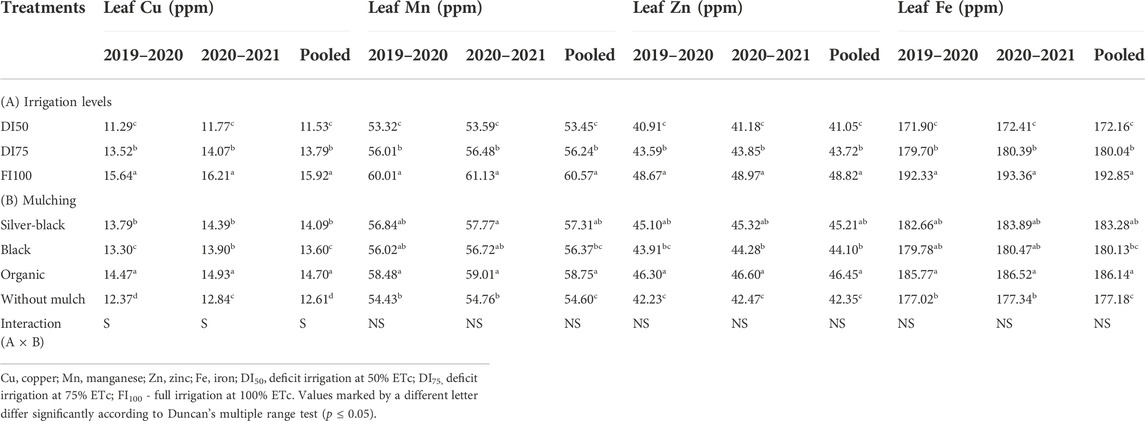
TABLE 3. Micronutrients (Cu, Mn, Zn and Fe) content in leaves of VNR Bihi guava influenced by irrigation levels and mulching.
Moreover, the highest concentrations of micronutrients were registered for FI100 conditions (Cu, 15.64–16.21 ppm; Mn, 60.01–61.13 ppm; Zn, 48.67–48.97 ppm; and Fe, 192.33–193.36 ppm), followed by DI75 conditions (Cu, 13.52–14.07 ppm; Mn, 56.01–56.48 ppm; Zn, 43.59–43.85 ppm; and Fe, 179.70–180.39 ppm), and the minimum were recorded for DI50 conditions (Cu, 11.29–11.77 ppm; Mn, 53.32–53.59 ppm; Zn, 40.91–41.18 ppm; and Fe, 171.90–172.41 ppm), presented in Table 3. The increased availability of micronutrients under full irrigation conditions might be attributed to a low redox potential due to a low oxygen content, the increased solubility of the reduced form of iron (Fe3+ to Fe2+), and other micronutrients in soil (Marathe et al., 2009). Increased micronutrient concentrations in leaves under the full irrigation regime were also reported by Khan et al. (2013) for guava, Panigrahi et al. (2014) for Kinnow mandarins, and Nadu (2018) for pomegranates. Various mulches also significantly influenced the leaf micronutrient concentrations (Cu, Mn, Zn, and Fe) during 2019–20 and 2020–21. The highest concentrations of the micronutrients were registered for MWM, followed by MSB, while the minimum concentrations of micronutrients was recorded in un-mulched plants. Barman et al. (2017), for guava cv. Lalit, also reported increased concentrations of micronutrients under mulched conditions.
3.3 Plant vegetative growth
Different growth parameters such as plant height, average plant spread, stem girth diameter, canopy volume, and leaf area were significantly influenced by different irrigation treatments and mulch types (Table 4). Among the irrigation regimes, FI100 treatment registered significantly higher plant heights, average plant spreads, stem girth diameters, canopy volumes, and leaf areas, whereas minimum values were observed for DI50 conditions during both years. The increased ABA biosynthesis in the roots and the reduction of cytokinin synthesis in the roots, branches, and buds in deficit irrigation conditions affects the vegetative growth of plants (Dodd, 2005). Earlier studies by Panigrahi et al. (2014) on Kinnow mandarins also showed similar findings; a decrease in vegetative growth upon deficit irrigation treatment.
The maximum plant height was recorded for MSB, and the minimum under MWM conditions. Moreover, MSB, MB, and MOM were statistically at par with one another during both years of experimentation. On the basis of pooled data, the canopy volume was 27.59% higher for MSB compared with MWM. The leaf area was also significantly higher for MSB: 8.56% higher than that of MWM. The increased plant growth parameters due to mulching might be caused by higher plant physiological processes as congenial moisture and a range of temperatures were available over the experimental period (Khan et al., 2013). Moreover, optimum moisture availability in silver-black mulch maintains proper turgor pressure, required for stomatal opening for gaseous exchange, which eventually led to a higher photosynthetic rate (Ayotamuno et al., 2007). The above findings are in agreement with the results of Singh et al. (2020) and Preet et al. (2021); the authors reported maximum canopy volumes with silver-black mulch as compared with no mulch in guava cv. VNR Bihi.
3.4 Yield parameters
Table 5 presents the numbers of fruits per plant, average fruit weights, fruit yields, fruit lengths, and fruit diameters produced under various irrigation regimes and mulches. The number of fruits harvested per plant and the mean fruit weights increased with increasing irrigation regime, i.e., from 50% ETc to 75% ETc under DI conditions, and were slightly lower in FI100 (full irrigation at 100% ETc) as compared with DI75 (deficit irrigation at 75% ETc) during both years. However, regarding the fruit weights, FI100 and DI75 treatments were statistically at par with each other during both years of the study. Moreover, the number of fruits harvested per plant was higher in 2019 than in 2020, due to better flowering, higher average rainfall, and lower fruit cracking in 2019 than in 2020. In contrast, the mean fruit weights were higher in 2020 than in 2019, due to a lower number of fruits per plant in 2020 than in 2019. Intrigliolo et al. (2013) reported a significant increase in the fruit numbers and fruit weights for pomegranates under deficit irrigation conditions; the authors concluded that mitigated competition between vegetative growth and reproductive organs, caused by mild water stress, reduced the abscission of reproductive organs. Drip irrigation provides a consistent soil moisture regime in which roots remain active throughout the season, resulting in an optimum availability of nutrients and proper translocation of food materials, which accelerates fruit growth and development in guava. The authors recorded a maximum fruit weight at 80% irrigation using plastic mulching in guava cv. Allahabad Safeda (Singh et al., 2015). Panigrahi et al. (2012) also reported similar decreased mean fruit weights as the irrigation regime decreased from 80% Ecp to 40% Ecp under DI conditions, in Nagpur mandarins.
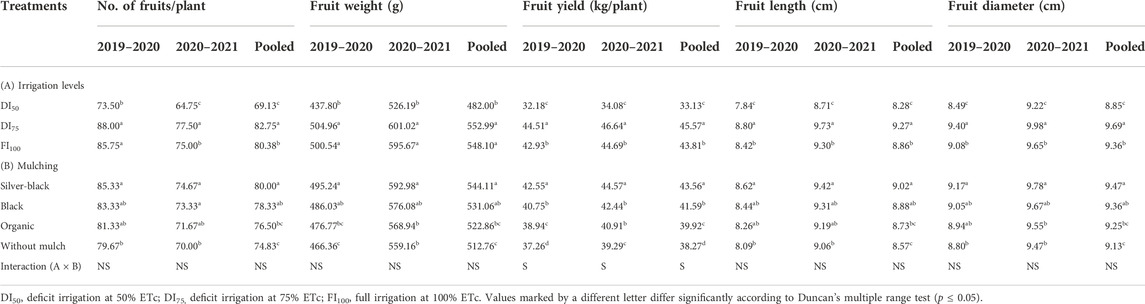
TABLE 5. Number of fruits harvested, average fruit weight, fruit yield, fruit length and fruit diameter of VNR Bihi guava influenced by irrigation levels and mulching.
The highest fruit yields were recorded for DI75 (44.51 and 42.93 kg/plant), followed by FI100 (46.64 and 44.69 kg/plant) during 2019–20 and 2020–21, respectively. The fruit yields under different irrigation treatments were higher in 2020 than in 2019, due to higher fruit weights in the former year. On the basis of pooled data, the fruit yields increased by almost 37.54% upon increasing the irrigation level from 50% ETc to 75% ETc under DI. The possible reasons for higher fruit yields under DI75 might be that the water deficit (20–25% available soil water depletion) in the root zone under this treatment suppressed vegetative growth of the plants without much effect on the leaf photosynthesis rate; plants invested higher quantities of photosynthates towards reproductive growth (fruiting) than vegetative growth (Panigrahi et al., 2012). As guava is a hardy plant and can be grown in semi-arid and arid zones under water stress conditions, this might be a reason why plants irrigated with moderate water deficits (deficit irrigation at 75% ETc) performed better as compared with full irrigation. Similar results were reported by Kaushik et al. (2013), Singh et al. (2015), and Preet et al. (2021) in guava.
Mulching significantly influenced the guava plant yields during both years of study. MSB exhibited almost 14.19% and 16.46% higher fruit yields during 2019–20 and 2020–21, respectively, as compared with un-mulched plants (Table 5). The positive impact on yield parameters due to various mulches might be attributed to an alteration of the microclimate in favor of the guava plants viz., temperature regulation, maintenance of appropriate soil moisture status, as well as reduced weed competition, soil compaction, and erosion, generating enhanced moisture and nutrient availability in the fruit plants. These favorable factors undoubtedly improved the guava plant yields (Singh et al., 2020). The interactive relationship between deficit irrigation and mulch, i.e., DIxM, and its effect on the fruit yield/plant varied and were statistically significant in both consecutive years (Table 6). On the basis of pooled data for the 2 years, it is pertinent to mention that the fruit yield/plant improved by 36.81% in guava plants irrigated under DI75MSB as compared with DI50MWM. Singh et al. (2015) also revealed higher fruit yields in guava cv. Allahabad Safeda., treated with drip irrigation at 80% Ecp and using plastic mulching.
The average fruit lengths and diameters were significantly lower in 2019–20 compared with 2020–21. In both years, these parameters were highest for DI75 treatment and were significantly different from the FI100 and the DI50 treatments. It is also clear from the pooled data for two consecutive years that fruit lengths and diameters improved by 10.67% and 8.66%, respectively, and were improved under DI75 as compared with DI50 conditions. The increased fruit lengths and diameters under moderate water deficit conditions might be due to balanced vegetative growth and maximum interception of light, as elucidated by Kumawat et al. (2017) for guava. Similar findings for guava were also reported by Kaushik et al. (2013) and Preet et al. (2021). Likewise, different types of plant mulches also significantly influenced the fruit lengths and widths during both years of investigation. Pooled data indicated that plants mulched with MSB exhibited substantially higher fruit lengths (9.02 cm) and fruit diameters (9.47 cm), followed by MB, and minimum values observed for un-mulched plants. The positive impact of various mulches on the fruit length might be due to the fact that the mulches provided consistently improved available soil moisture in the plant basin, in which the plant roots remained active throughout the season, resulting in optimum availability of nutrients and proper translocation of food materials, which accelerated fruit growth and development (Joshi et al., 2011). Higher fruit lengths and diameters caused by silver-black mulch were also reported by Singh (2020) in VNR Bihi guava and by Beelagi (2020) in pomegranates.
3.5 Irrigation water productivity
The irrigation water productivity was significantly affected by various deficit irrigation levels during both years of experimentation (Figure 3D). The irrigation water productivity decreased as the irrigation regime increased from DI50 to FI100. The irrigation water productivity improved by 33.27% and 34.43% under DI50 as compared with FI100 during 2019–20 and 2020–21, respectively. The rate of water loss through evaporation from the soil surface was much lower under deficit irrigation regimes. Hence, the irrigation water productivity was higher in this regime as compared with the full irrigation conditions. The increased yields and irrigation water productivity achieved under deficit irrigation might be due to the excellent soil water relationship, with higher concentrations of oxygen present in the root zone and efficient utilization of water and nutrients. A deficit of or excessive water stress leads to stomatal closure, thereby improving the irrigation water productivity in water-stressed plants. An improvement in the irrigation water productivity in response to deficit irrigation compared with full irrigation was also reported in citrus (Pérez-Pérez et al., 2008; García-Tejero et al., 2010a), pomegranates (Dinc et al., 2018), mangos (Upreti et al., 2018), and guava (Preet et al., 2021).
The irrigation water productivity was influenced by different types of mulches, with plants mulched using MSB exhibiting significantly higher irrigation water productivities, followed by MB, with the lowest productivity observed in MWM over both years of study (Figure 3D). Sakariya et al. (2018) reported a higher irrigation water productivity using silver-black mulch in the papaya variety Madhu Bindu in Taiwan. Consistent with the above findings, da Silva et al. (2009) reported similar findings in mangos and Tiwari et al. (2014) in sapota. The interactive relationship between deficit irrigation and mulch, i.e., DIxM, and its effect on the irrigation water productivity varied significantly in both consecutive years. Pooled data for the 2 years indicated that the irrigation water productivity improved by 47.28% in guava plants irrigated under DI50MSB as compared with FI100MWM.
3.6 Ascorbic acid (mg/100 g)
The data presented in Table 7 reveal that various irrigation levels significantly influenced the ascorbic acid content of guava during 2019–20 and 2020–21. Plants irrigated at the DI75 (deficit irrigation at 75% ETc) level exhibited a maximum ascorbic acid content of 112.87 mg/100 g, followed by 102.85 mg/100 g for DI50 (deficit irrigation at 50% ETc), with a minimum recorded (90.41 mg/100 g) for FI conditions (full irrigation at 100% ETc). Similarly, during 2020–21, the maximum ascorbic acid concentration (115.44 mg/100 g) was recorded for DI75 (deficit irrigation at 75% ETc), and the minimum (93.30 mg/100 g) for FI (full irrigation at 100% ETc). In our study, the water deficit increased the vitamin C content of the fruit as compared with full irrigation. The tolerance to water deficit is correlated with ascorbic acid accumulation, which plays an important role in ROS (reactive oxygen species) detoxification (Wang et al., 2012). However, vitamin C is a major antioxidant in plants, capable of neutralizing active forms of oxygen. These results were similar to those of Ripoll et al. (2016), who found that water stress during the maturation phase increased the vitamin C levels. This effect could be related to the overall attempt made by the tree to combat water stress via the de novo synthesis of ascorbic acid (Navarro et al., 2010). Kowitcharoen et al. (2018) also suggested that increases in ascorbic acid contents in water deficit trees bearing sugar apple fruits may have been caused by abiotic stress conditions. Normally, stress conditions can induce ABA biosynthesis, which can promote hydrogen peroxide production; hydrogen peroxide is classified as a type of stress signal that may induce the antioxidant system in the plant to maintain or increase the ascorbic acid content. Singh et al. (2015) and Gupta (2019) also reported similar findings for guava and litchi, respectively, with higher ascorbic acid contents observed at a mild water deficit condition as compared with full irrigation.
In the year 2019–20, the highest ascorbic acid content was 105.74 mg/100 g, recorded in plants mulched using silver-black, followed by plants mulched with black (103.61 mg/100 g), and the lowest value (98.23 mg/100 g) observed for MWM (without mulch). During the second year of the study, the highest ascorbic acid content (109.03 mg/100 g) was also observed for MSB (silver-black) mulch, and the lowest (100.40 mg/100 g) for MWM (without mulch). However, MSB and MB were statistically at par with each other in both years of the study (Table 7). The increase of the ascorbic acid contents in guava fruits under different mulches might be due to optimum soil moisture content, providing excellent fruit quality parameters and soil nutrient status throughout the experimentation period (Tiwari et al., 2014). Prakash et al. (2011) and Singh (2020) also reported significant increases in the ascorbic acid contents under various mulches, for mango and guava, respectively. Furthermore, the interactive effect of deficit irrigation and mulching (DIxM) indicated that the ascorbic acid content of guava fruit was statistically significant, as mentioned in Table 7. A maximum pooled value for the ascorbic acid content was obtained: 116.22 mg/100 g for DI75MSB (deficit irrigation at 75% ETc and using silver-black mulch), which was significantly higher than other treatments. Similar findings were also observed by Joshi et al. (2012), who reported that drip irrigation coupled with mulch application significantly improved the ascorbic acid content in litchi fruits.
3.7 Correlation matrix
The degree of linear association of the fruit yield with all other variables (plant height, canopy spread, canopy volume, stem girth, leaf area, number of fruits, fruit weight, fruit length, fruit diameter, RLWC, proline content, chlorophyll, leaf macronutrients and micronutrients, and IWP) is presented in a correlation matrix in Table 8 and in Figure 4. The yield positively correlated with the total chlorophyll content, leaf area, leaf P content, canopy volume, canopy spread, fruit diameter, plant height, RLWC, fruit weight, leaf Cu content, fruit length, number of fruits, and the leaf K and N contents, while significant negative correlations were observed with the IWP and the leaf proline content.
3.8 Stepwise regression analysis
Stepwise regression analysis was performed by using the fruit yield (kg/plant) as a dependent variable and the remaining variables as independent variables. The correlation matrix (Table 8) showed a significant correlation among independent variables, which generates a multicollinearity problem. Stepwise regression analysis overcomes the problem of multicollinearity. The results revealed that out of the 20 independent variables, four (leaf proline content, fruit weight, irrigation water productivity, and leaf Cu content) were considered to explain the variable fruit yield. The regression model was found to be highly significant, with F calculated to be 157.9 (p-value = 2.2 × 10–16). The regression coefficients for all variables are shown in Table 9. The four variables leaf proline content, fruit weight, IWP, and leaf Cu content were found to be significant, and can be used to predict the fruit yield. The regression model is as follows:
The R2 value was 0.904, which means that 90.40% of the variation in the dependent variable (fruit yield) is explained by the model. The adjusted R2 value was 0.898. The low values for goodness of fit statistics such as the RMSE (1.91), MAE (1.52), and MAPE (3.83) indicate that there is a small deviation between the actual values and the predicted values, as shown in Figure 5A. The run test statistic of the residuals was 37 with a p-value of 0.99, indicating that the residuals are random and do not follow any pattern. The Shapiro–Wilk test statistic was 0.99, with a p-value of 0.88, indicating that the residuals follow a normal distribution. Thus, residual analysis indicates that the regression model does not violate the assumption of normality or the randomness of the residuals.
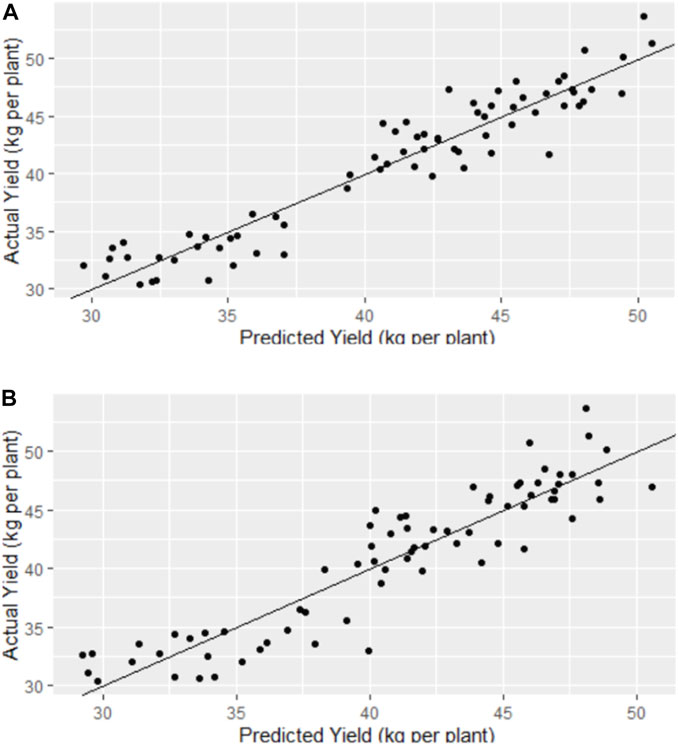
FIGURE 5. Actual yield vs. predicted yield of VNR Bihi guava plants by (A) step wise regression and (B) principal component regression.
3.9 Principal component regression analysis
PCA was performed for the 20 variables, and three principal components showing eigen values over one were used to predict the fruit yield. The results showed that the first three principal components captured 83.25% of the variation of the data set. The eigen values and the percentage of variance, explained by the principal component, is shown in Table 10. The multiple linear regression model was fitted using the three principal components as dependent variables and the yield as the independent variable.
The principal component regression model was found to be highly significant, with F calculated to be 125.1 (p-value = 2.2 × 10–16). The regression coefficients for all variables are shown in Table 11. Among the three principal components, PC1 and PC3 exhibited significant regression coefficients. The regression model is as follows:
The R2 and adjusted R2 values were 0.846 and 0.839, respectively. The lower values of goodness of fit statistics such as the RMSE (2.41), MAE (1.93), and MAPE (4.87) indicate that there is a small deviation between the actual values and the predicted values, as shown in Figure 5B. The run test statistic of the residuals was 24 with a p-value of 0.002, indicating that the residuals are not random. The Shapiro–Wilk test statistic was 0.98, with a p-value of 0.78, indicating that the residuals follow normal distributions. Thus, residual analysis indicates that the regression model does not violate the assumption of normality and does violate the randomness of the residuals.
4 Conclusion
Fully-irrigated plants and silver-black mulch produced the highest vegetative growths and leaf nutrient contents. However, deficit irrigation at 75% Etc, along with silver-black mulch used during the fruit growth period produced higher numbers of fruits per plant, higher average fruit weights, and higher fruit yields. Moreover, the irrigation water productivity also improved substantially in deficit irrigated plants. Out of both the techniques used for the prediction of fruit yield, the stepwise regression model presented a higher value of the adjusted R2 and lower values of the RMSE, MAE, and MAPE. Furthermore, the stepwise regression model also follows the assumptions of normality and randomness of the residuals. Thus, we conclude that the stepwise regression model is preferred over the principal component regression model to predict the fruit yield in this study, using variables viz., the leaf proline content, fruit weight, IWP, and leaf Cu content. Based on the present findings, it can be inferred that application of deficit irrigation at 75% ETc using silver-black mulch imposed desirable levels of water stress on guava plants. This improved their irrigation water productivities, yields, and the fruits quality, and could be a superior option for guava cultivation in the subtropical, humid Tarai conditions of Uttarakhand, India, as well as in regions with similar agro-climatic conditions.
Data availability statement
The original contributions presented in the study are included in the article/supplementary material, further inquiries can be directed to the corresponding authors.
Author contributions
Conceptualization, RJ, VS, and PS; methodology, DV; AC; software, RP; validation, RJ, VS, and PS; formal analysis, RP; investigation, SJ; resources, VS; data curation, RP; writing—original draft preparation, RJ, VS; writing—review and editing, NA-A and SA; visualization, RJ; supervision, VS; project administration, AC; funding acquisition, MA-S. All authors have read and agreed to the published version of the manuscript.
Funding
This work was Supported by Researchers Supporting Project Number (RSP 2022R410), King Saud University, Riyadh, Saudi Arabia.
Acknowledgments
We would like to thank the Head of the Department of Horticulture, College of Agricultural, G.B. Pant University of Agriculture and Technology, Pantnagar 263145, Uttarakhand for providing the resources and the laboratory. The authors would like to extend their sincere appreciation to the Researchers Supporting Project Number (RSP 2022R410), King Saud University, Riyadh, Saudi Arabia.
Conflict of interest
The authors declare that the research was conducted in the absence of any commercial or financial relationships that could be construed as a potential conflict of interest.
Publisher’s note
All claims expressed in this article are solely those of the authors and do not necessarily represent those of their affiliated organizations, or those of the publisher, the editors and the reviewers. Any product that may be evaluated in this article, or claim that may be made by its manufacturer, is not guaranteed or endorsed by the publisher.
References
Abdel-Razik, A. M. (2013). Effect of water deficit on growth of some mango (mangifera indica L.) rootstocks. Nat. Sci. 11, 136–142.
Allen, R. G., Pereira, L. S., Raes, D., and Smith, M. (1998). Crop evapotranspiration-Guidelines for computing crop water requirements-FAO Irrigation and drainage paper 56. Rome: Fao, D05109.
Anonymous, (2019). Hortic. Stat. Div. Dep. Agric. Coop. Farmers welfare, minist. Agric. Farmers welf. Gov. India. New Delhi, India: Oxford Univ. Press, 437.
Ayotamuno, J. M., Zuofa, K., Ofori, S. A., and Kogbara, R. B. (2007). Response of maize and cucumber intercrop to soil moisture control through irrigation and mulching during the dry season in Nigeria. Afr. J. Biotechnol. 6, 509–515. doi:10.4314/ajb.v6i5.56826
Barman, P., Srivastava, K. ., and Adak, T. (2017). Response of guava cv. Lalit to plastic mulch, fertigation and micro-nutrient spray under raised bed. Indian J. Agric. Allied Sci. 3, 1–8.
Bartlett, M. K., Detto, M., and Pacala, S. W. (2019). Predicting shifts in the functional composition of tropical forests under increased drought and CO2 from trade-offs among plant hydraulic traits. Ecol. Lett. 22, 67–77. doi:10.1111/ele.13168
Bates, L. S., Waldren, R. P., and Teare, I. D. (1973). Rapid determination of free proline for water-stress studies. Plant Soil 39, 205–207. doi:10.1007/BF00018060
Beelagi, R. (2020). Effect of mulching and drip irrigation on growth, yield and fruit quality of Pomegranate cv. Bhagwa. MSc Thesis. Pantnagar, Uttarkhand: G. B. Pant University of Agriculture and Technology.
Berihun, B. (2011). Effect of mulching and amount of water on the yield of tomato under drip irrigation. J. Hortic. For. 3, 200–206.
Bowman, W. D. (1989). The relationship between leaf water status, gas exchange, and spectral reflectance in cotton leaves. Remote Sens. Environ. 30, 249–255. doi:10.1016/0034-4257(89)90066-7
Cui, N., Du, T., Kang, S., Li, F., Zhang, J., Wang, M., et al. (2008). Regulated deficit irrigation improved fruit quality and water use efficiency of pear-jujube trees. Agric. Water Manag. 95, 489–497. doi:10.1016/j.agwat.2007.11.007
da Silva, V. de P. R., da Cunha Campos, J. H. B., and de Azevedo, P. V. (2009). Water-use efficiency and evapotranspiration of mango orchard grown in northeastern region of Brazil. Sci. Hortic. Amst. 120, 467–472. doi:10.1016/j.scienta.2008.12.005
Deb, P., Sangma, D. K., Prasad, B. V. G., Bhowmick, N., and Dey, K. (2014). Effect of different mulches on vegetative growth of strawberry ( cv . Tioga ) under red and lateritic zone of West Bengal. Int. J. Basic Appl. Biol. 2, 77–80.
Department of horticulture and food processing (2018). Horticulture production data: Horticulture production data 2017-18. State horticulture mission. Available at: https://shm.uk.gov.in/.
Dhawan, V. (2017). “Water and agriculture in India,” in Background paper for the South asia expert panel during the global forum for food and agriculture.
Dinc, N., Aydinsakir, K., Isik, M., Bastug, R., Ari, N., Sahin, A., et al. (2018). Assessment of different irrigation strategies on yield and quality characteristics of drip irrigated pomegranate under mediterranean conditions. Irrig. Sci. 36, 87–96. doi:10.1007/s00271-017-0565-5
Dodd, I. C. (2005). Root-to-shoot signalling: Assessing the roles of ‘up’ in the up and down world of long-distance signalling in planta. Plant Soil 274, 251–270.
Eissa, N. M. H. (2002). Effect of some plasticulture and fertigation treatments on productivity and fruit quality of strawberry.
El Jaouhari, N., Abouabdillah, A., Bouabid, R., Bourioug, M., Aleya, L., and Chaoui, M. (2018). Assessment of sustainable deficit irrigation in a Moroccan apple orchard as a climate change adaptation strategy. Sci. Total Environ. 642, 574–581. doi:10.1016/j.scitotenv.2018.06.108
Elbeltagi, A., Raza, A., Hu, Y., Al-Ansari, N., Kushwaha, N. L., Srivastava, A., et al. (2022). Data intelligence and hybrid metaheuristic algorithms-based estimation of reference evapotranspiration. Appl. Water Sci. 12, 152. doi:10.1007/s13201-022-01667-7
Faci, J. M., Blanco, O., Medina, E. T., and Martínez-Cob, A. (2014). Effect of post veraison regulated deficit irrigation in production and berry quality of Autumn Royal and Crimson table grape cultivars. Agric. Water Manag. 134, 73–83. doi:10.1016/j.agwat.2013.11.009
Farooq, M., Wahid, A., Kobayashi, N., Fujita, D., and Basra, S. M. A. (2009). “Plant drought stress: Effects, mechanisms and management BT - sustainable agriculture,”Editors E. Lichtfouse, M. Navarrete, P. Debaeke, S. Véronique, and C. Alberola (Dordrecht: Springer Netherlands), 153–188. doi:10.1007/978-90-481-2666-8_12
Farooq, T. H., Kumar, U., Shakoor, A., Albasher, G., Alkahtani, S., Rizwana, H., et al. (2021). Influence of intraspecific competition stress on soil fungal diversity and composition in relation to tree growth and soil fertility in sub-tropical soils under Chinese fir monoculture. Sustainability 13, 10688. doi:10.3390/su131910688
Farooq, T. H., Kumar, U., Yan, Y., Arif, M. S., Shakoor, A., Tayyab, M., et al. (2022). Receptiveness of soil bacterial diversity in relation to soil nutrient transformation and canopy growth in Chinese fir monoculture influenced by varying stand density. Trees 36, 1149–1160. doi:10.1007/s00468-022-02278-0
Fereres, E., and Soriano, M. A. (2007). Deficit irrigation for reducing agricultural water use. J. Exp. Bot. 58, 147–159. doi:10.1093/jxb/erl165
Galindo, A., Collado-González, J., Griñán, I., Corell, M., Centeno, A., Martín-Palomo, M. J., et al. (2018). Deficit irrigation and emerging fruit crops as a strategy to save water in Mediterranean semiarid agrosystems. Agric. Water Manag. 202, 311–324. doi:10.1016/j.agwat.2017.08.015
García-Tejero, I., Romero-Vicente, R., Jiménez-Bocanegra, J. A., Martínez-García, G., Durán-Zuazo, V. H., and Muriel-Fernández, J. L. (2010b). Response of citrus trees to deficit irrigation during different phenological periods in relation to yield, fruit quality, and water productivity. Agric. Water Manag. 97, 689–699. doi:10.1016/j.agwat.2009.12.012
García-Tejero, I., Jiménez-Bocanegra, J. A., Martínez, G., Romero, R., Durán-Zuazo, V. H., and Muriel-Fernández, J. L. (2010a). Positive impact of regulated deficit irrigation on yield and fruit quality in a commercial citrus orchard [Citrus sinensis (L.) Osbeck, cv. salustiano]. Agric. Water Manag. 97, 614–622. doi:10.1016/j.agwat.2009.12.005
Gindaba, J., Rozanov, A., and Negash, L. (2004). Response of seedlings of two Eucalyptus and three deciduous tree species from Ethiopia to severe water stress. For. Ecol. Manage. 201, 119–129. doi:10.1016/j.foreco.2004.07.009
Gupta, N. (2019). Effect of drip irrigation and mulching on plant growth, leaf nutrients status, fruit yield and quality of litchi.
Hamdy, A. E., Khalifa, S. M., Shawer, S. S., and Mancy, A. A. G. (2016). Effect of water stress on the growth, nutritional and biochemical status of two varieties of pomegranate seedlings. J. Plant Prod. 7, 1321–1329. doi:10.21608/jpp.2016.47030
Hiscox, J. D., and Israelstam, G. F. (1979). A method for the extraction of chlorophyll from leaf tissue without maceration. Can. J. Bot. 57, 1332–1334. doi:10.1139/b79-163
Hou, X.-Y., Wang, F.-X., Han, J.-J., Kang, S.-Z., and Feng, S.-Y. (2010). Duration of plastic mulch for potato growth under drip irrigation in an arid region of Northwest China. Agric. For. Meteorol. 150, 115–121. doi:10.1016/j.agrformet.2009.09.007
Intrigliolo, D. S., Bonet, L., Nortes, P. A., Puerto, H., Nicolas, E., and Bartual, J. (2013). Pomegranate trees performance under sustained and regulated deficit irrigation. Irrig. Sci. 31, 959–970. doi:10.1007/s00271-012-0372-y
Jaleel, C. A., Manivannan, P., Wahid, A., Farooq, M., Al-Juburi, H. J., Somasundaram, R., et al. (2009). Drought stress in plants: A review on morphological characteristics and pigments composition. Int. J. Agric. Biol. 11, 100–105.
Jalota, S. K., and Prihar, S. S. (1990). Effect of straw mulch on evaporation reduction in relation to rates of. J. Indian Soc. Soil Sci. 38, 728–730.
Joshi, G., Singh, P. K., Singh, S. K., and Srivastava, P. C. (2011). Effect of drip fertigation and mulching on water requirement, yield and economics of high density litchi. Prog. Hortic. 43, 237–242.
Joshi, G., Singh, P. K., Srivastava, P. C., and Singh, S. K. (2012). Effect of mulching, drip irrigation scheduling and fertilizer levels on plant growth, fruit yield and quality of litchi (Litchi chinensis Sonn.). Indian J. Soil Conserv. 40, 46–51.
Kaushik, R. A., Pareek, S., Sarolia, D. K., and Singh, V. (2013). Effect of drip fertigation scheduling on fertilizer use efficiency, leaf nutrient status, yield and quality of ‘Shweta’guava (Psidium guajava L.) under meadow orcharding. Natl. Acad. Sci. Lett. 36, 483–488.
Kay, B. L. (1978). “Mulch and chemical stabilizers for land reclamation in dry regions,” in Reclamation of drastically disturbed lands. Editors F. W. Schaller, and P. Sutten (American Society of Agronomy), 467–483.
Khan, J. N., Jain, A. K., Sharda, R., Singh, N. P., Gill, P. P. S., and Kaur, S. (2013). Growth, yield and nutrient uptake of guava (Psidium Guavaja L.) affected by soil matric potential, fertigation and mulching under drip irrigation. Agric. Eng. Int. CIGR J. 15, 17–28.
Khattab, M. M., Shaban, A. E., El-shrief, A. H., and Mohamed, A. S. E. (2011). Growth and productivity of pomegranate trees under different irrigation levels. III: Leaf pigments, proline and mineral content. J. Hortic. Sci. Ornam. Plants 3, 265–269.
Kogan, F., Guo, W., and Yang, W. (2019). Drought and food security prediction from NOAA new generation of operational satellites. Geomatics, Nat. Hazards Risk 10, 651–666. doi:10.1080/19475705.2018.1541257
Kowitcharoen, L., Wongs-Aree, C., Setha, S., Komkhuntod, R., Kondo, S., and Srilaong, V. (2018). Pre-harvest drought stress treatment improves antioxidant activity and sugar accumulation of sugar apple at harvest and during storage. Agric. Nat. Resour. 52, 146–154. doi:10.1016/j.anres.2018.06.003
Kumar, U., Saqib, H. S. A., Islam, W., Prashant, P., Patel, N., Chen, W., et al. (2022). Landscape composition and soil physical–chemical properties drive the assemblages of bacteria and fungi in conventional vegetable fields. Microorganisms 10, 1202. doi:10.3390/microorganisms10061202
Kumawat, K. L., Sarolia, D. K., Kaushik, R. A., and Jodha, A. S. (2017). Effect of irrigation and fertigation scheduling on growth, flowering, yield and economics of guava cv. Lalit under ultra high density planting system. Indian J. Hortic. 74, 362–368. doi:10.5958/0974-0112.2017.00072.X
López-López, M., Espadafor, M., Testi, L., Lorite, I. J., Orgaz, F., and Fereres, E. (2018). Water use of irrigated almond trees when subjected to water deficits. Agric. Water Manag. 195, 84–93. doi:10.1016/j.agwat.2017.10.001
Marathe, R. A., Bharambe, P. R., Sharma, R., and Sharma, U. C. (2009). Soil properties of vertisol and yield of sweet orange (Citrus sinensis) as influenced by integrated use of organic manures, inorganic and bio-fertilizers. Indian J. Agric. Sci. 79, 3–7.
Nadu, T. (2018). Nutrient uptake, growth and yield of pomegranate as influenced by irrigation frequencies under light textured soils. J. Environ. Biol. 39, 228–236. doi:10.22438/jeb/39/2/MRN-511
Navarro, J. M., Pérez-Pérez, J. G., Romero, P., and Botía, P. (2010). Analysis of the changes in quality in Mandarin fruit, produced by deficit irrigation treatments. Food Chem. 119, 1591–1596. doi:10.1016/j.foodchem.2009.09.048
Nimisha, S., Kherwar, D., Ajay, K. M., Singh, B., and Usha, K. (2013). Molecular breeding to improve guava (Psidium guajava L.): Current status and future prospective. Sci. Hortic. Amst. 164, 578–588. doi:10.1016/j.scienta.2013.10.017
Obilor, E. I., and Amadi, E. C. (2018). Test for significance of Pearson’s correlation coefficient. Int. J. Innov. Math. Stat. Energy Policies 6, 11–23.
Pandey, S., Tewari, G. S., Singh, J., Rajpurohit, D., and Kumar, G. (2016). Efficacy of mulches on soil modifications, growth, production and quality of strawberry (Fragaria x ananassa Duch.). Int. J. Sci. Nat. 7, 813–820.
Panigrahi, P., Sharma, R. K., Hasan, M., and Parihar, S. S. (2014). Deficit irrigation scheduling and yield prediction of ‘Kinnow’ Mandarin (Citrus reticulate Blanco) in a semiarid region. Agric. Water Manag. 140, 48–60. doi:10.1016/j.agwat.2014.03.018
Panigrahi, P., Srivastava, A. K., and Huchche, A. D. (2012). Effects of drip irrigation regimes and basin irrigation on Nagpur Mandarin agronomical and physiological performance. Agric. Water Manag. 104, 79–88. doi:10.1016/j.agwat.2011.11.018
Pérez-Pérez, J. G., Romero, P., Navarro, J. M., and Botía, P. (2008). Response of sweet orange cv ‘Lane late’ to deficit-irrigation strategy in two rootstocks. II: Flowering, fruit growth, yield and fruit quality. Irrig. Sci. 26, 519. doi:10.1007/s00271-008-0113-4
Popat, R., and Banakara, K. (2020). Doebioresearch: Analysis of Design of experiments for biological research.
Prakash, K., Vijayakumar, R. M., Balamohan, T. N., and Sundhar Singh, S. D. (2011). Effect of drip irrigation regimes and fertigation levels on yield and quality of mango cultivar’alphonso’under ultra high density planting. Glob. Conf. Augmenting Prod. Util. Of Mango Biotic Abiotic Stresses 1066, 147–150. doi:10.17660/ActaHortic.2015.1066.17
Pratima, P. (2014). Studies on water relations and deficit irrigation in kiwifruit (Actinidia deliciosa Chev.). Ph. D. Thesis.
Preet, M. S., Kumar, R., Singh, V. P., NehaDongariyal, A., and Srivastava, R. (2021). Response of guava to integrated nutrient and water management. Indian J. Hortic. 78, 189–197. doi:10.5958/0974-0112.2021.00027.X
Ranganna, S. (1986). Handbook of analysis and quality control for fruits and vegetable products (Tata McGraw Hills Publishing Co. Ltd: New Delhi), 102–103.
Ripoll, J., Urban, L., Brunel, B., and Bertin, N. (2016). Water deficit effects on tomato quality depend on fruit developmental stage and genotype. J. Plant Physiol. 190, 26–35. doi:10.1016/j.jplph.2015.10.006
Sakariya, K. K., Satasiya, R. M., Satasiya, V. D., and Sapariya, P. S. (2018). Performance of plastic mulch on papaya crop. Int. J. Curr. Microbiol. Appl. Sci. 7, 3243–3251. doi:10.20546/ijcmas.2018.703.375
Sampathkumar, T., Pandian, B. J., Jeyakumar, P., and Manickasundaram, P. (2014). Effect of deficit irrigation on yield, relative leaf water content, leaf proline accumulation and chlorophyll stability index of cotton–maize cropping sequence. Exp. Agric. 50, 407–425. doi:10.1017/S0014479713000598
Sarkar, S., and Singh, S. R. (2007). Interactive effect of tillage depth and mulch on soil temperature, productivity and water use pattern of rainfed barley (Hordium vulgare L.). Soil Tillage Res. 92, 79–86. doi:10.1016/j.still.2006.01.014
Shao, H.-B., Chu, L.-Y., Jaleel, C. A., Manivannan, P., Panneerselvam, R., and Shao, M.-A. (2009). Understanding water deficit stress-induced changes in the basic metabolism of higher plants – biotechnologically and sustainably improving agriculture and the ecoenvironment in arid regions of the globe. Crit. Rev. Biotechnol. 29, 131–151. doi:10.1080/07388550902869792
Shapiro, S. S., and Wilk, M. B. (1965). An analysis of variance test for normality (complete samples). Biometrika 52, 591–611. doi:10.2307/2333709
Shukla, R., Kumar, P., Vishwakarma, D. K., Ali, R., Kumar, R., and Kuriqi, A. (2021). Modeling of stage-discharge using back propagation ANN-, ANFIS-, and WANN-based computing techniques. Theor. Appl. Climatol. doi:10.1007/s00704-021-03863-y
Singh, R. (2020). Effect of different mulches on soil characteristics, yield and quality of winter season guava.
Singh, V. K., Soni, M. K., and Singh, A. (2015). Effect of drip irrigation and polyethylene mulching on fruit yield and quality of guava cv. Allahabad Safeda under meadow orcharding. Indian J. Hortic. 72, 479. doi:10.5958/0974-0112.2016.00006.2
Singh, V. P., Jat, R., Kumar, V., and Singh, R. (2020). Mulches and their impact on floor management and performance of fruit crops: A review. Curr. J. Appl. Sci. Technol. 39, 62–78. doi:10.9734/cjast/2020/v39i3631074
Srikasetsarakul, U., Sringarm, K., Sruamsiri, P., Ongprasert, S., Spreer, W., Schulze, K., et al. (2011). Effects of partial rootzone drying irrigation on proline content and yield of mango in a commercial orchard. Glob. Conf. Augmenting Prod. Util. Of Mango Biotic Abiotic Stresses 1066, 85–94. doi:10.17660/ActaHortic.2015.1066.8
Stefanelli, D., Goodwin, I., and Jones, R. (2010). Minimal nitrogen and water use in horticulture: Effects on quality and content of selected nutrients. Food Res. Int. 43, 1833–1843. doi:10.1016/j.foodres.2010.04.022
Tahir, F. M., Ibrahim, M., and Hamid, K. (2003). Effect of drought stress on vegetative and reproductive growth behaviour of mango (Mangifera indica L.). Asian J. Plant Sci. 2, 116–118.
Takeda, L. N., Laurindo, L. F., Guiguer, E. L., Bishayee, A., Araújo, A. C., Ubeda, L. C. C., et al. (2022). Psidium guajava L. A systematic review of the multifaceted health benefits and economic importance. Food Rev. Int., 1–31. doi:10.1080/87559129.2021.2023819
Tallarida, R. J., and Murray, R. B. (1987). “Duncan multiple range test BT - manual of pharmacologic calculations: With computer programs,” Editors R. J. Tallarida, and R. B. Murray (New York, NY: Springer New York), 125–127. doi:10.1007/978-1-4612-4974-0_38
Teixeira, J., and Pereira, S. (2007). High salinity and drought act on an organ-dependent manner on potato glutamine synthetase expression and accumulation. Environ. Exp. Bot. 60, 121–126. doi:10.1016/j.envexpbot.2006.09.003
Tiwari, K. N., Kumar, M., Santosh, D. T., Singh, V. ., Maji, M. K., and Karan, A. K. (2014). Influence of drip irrigation and plastic mulch on yield of sapota (achraszapota) and soil nutrients. Irrig. Drain. Syst. Eng. 03, 2–8. doi:10.4172/2168-9768.1000116
Upreti, R., Singh, V. P., and Singh, P. K. (2018). Economic feasibility of drip irrigation regimes with mulching in mango cv. Pant sinduri. Int. J. Curr. Microbiol. App. Sci. 7, 1496–1501. doi:10.20546/ijcmas.2018.711.172
Usman, M., Bokhari, S. A. M., Fatima, B., Rashid, B., Nadeem, F., Sarwar, M. B., et al. (2022). Drought stress mitigating morphological, physiological, biochemical, and molecular responses of guava (psidium guajava L.) cultivars. Front. Plant Sci. 13. doi:10.3389/fpls.2022.878616
Wang, L., Wang, Y., Meng, X., and Meng, Q. (2012). Overexpression of tomato GDP-L-galactose phosphorylase gene enhanced tolerance of transgenic tobacco to methyl viologen-mediated oxidative stress. Plant Physiol. Commun. 48, 689–698.
Zaman, W. U., Arshad, M., and Saleem, A. (2001). Distribution of nitrate-nitrogen in the soil profile under different irrigation methods. Int. J. Agric. Biol. 2, 208–209.
Zapata-Sierra, A. J., and Manzano-Agugliaro, F. (2017). Controlled deficit irrigation for orange trees in Mediterranean countries. J. Clean. Prod. 162, 130–140. doi:10.1016/j.jclepro.2017.05.208
Zhong, Y., Fei, L., Li, Y., Zeng, J., and Dai, Z. (2019). Response of fruit yield, fruit quality, and water use efficiency to water deficits for apple trees under surge-root irrigation in the Loess Plateau of China. Agric. Water Manag. 222, 221–230. doi:10.1016/j.agwat.2019.05.035
Keywords: guava, deficit irrigation, mulching, plant water relations, irrigation water productivity
Citation: Jat R, Singh VP, Ali Abed S, Al-Ansari N, Singh PK, Vishwakarma DK, Choudhary A, Al-Sadoon MK, Popat RC and Jat SK (2022) Deficit irrigation scheduling with mulching and yield prediction of guava (Psidium guajava L.) in a subtropical humid region. Front. Environ. Sci. 10:1044886. doi: 10.3389/fenvs.2022.1044886
Received: 15 September 2022; Accepted: 25 October 2022;
Published: 23 November 2022.
Edited by:
Taimoor Hassan Farooq, Bangor College China, a joint unit of Bangor University and Central South University of Forestry and Technology, ChinaReviewed by:
Vivek Yadav, Northwest A&F University, ChinaVijay Rakesh Reddy Sanikommu, Indian Institute of Horticultural Research (ICAR), India
Varucha Misra, Indian Institute of Sugarcane Research (ICAR), India
Copyright © 2022 Jat, Singh, Ali Abed, Al-Ansari, Singh, Vishwakarma, Choudhary, Al-Sadoon, Popat and Jat. This is an open-access article distributed under the terms of the Creative Commons Attribution License (CC BY). The use, distribution or reproduction in other forums is permitted, provided the original author(s) and the copyright owner(s) are credited and that the original publication in this journal is cited, in accordance with accepted academic practice. No use, distribution or reproduction is permitted which does not comply with these terms.
*Correspondence: Rajkumar Jat, rajrulez95@gmail.com; Nadhir Al-Ansari, nadhir.alansari@ltu.se
†ORCID: Dinesh Kumar Vishwakarma, orcid.org/0000-0002-2421-6995