- 1Department of Agroecology and Environment, Soil Quality and Soil Use Research Group, Agroscope, Zürich, Switzerland
- 2Program Area Next-Generation Horticultural Systems, Open-Field Horticultural Systems Research Group, Leibniz Institute of Vegetable and Ornamental Crops (IGZ) e.V, Großbeeren, Germany
- 3Research Area Landscape Functioning, Isotope Biogeochemistry and Gas Fluxes Working Group, Leibniz Centre for Agricultural Landscape Research (ZALF) e.V, Müncheberg, Germany
Recycling nutrients is essential for closing nutrient loops within a circular economy. Using locally available resources such as human excreta to produce bio-based recycling fertilizers can substitute mineral fertilizers and thereby promote environmentally friendly food production. To better understand the fertilizer potential and nitrogen value of human excreta, three novel and safe recycling products were evaluated in a field experiment. Two nitrified urine fertilizers (NUFs) and one fecal compost were applied alone or in combination, and compared against the commercial organic fertilizer vinasse. In addition, the uptake of pharmaceuticals was assessed for treatments with compost application. White cabbage (Brassica oleracea var. capitata f. alba) was cultivated in plots in three different soil types (sand, loam or silt) treated with the fertilizers according to plant needs and mineral soil nitrogen content. The two NUFs resulted in marketable yields similar to those of vinasse in all soil types. Combining fecal compost with a NUF led to increased marketable yield compared to compost alone. The highest yield was recorded from the sandy soil, where vinasse and NUF treatments led to comparable yields, as expected in organic productions systems (up to 72 t ha−1). The cabbage yield and total aboveground fresh biomass followed the following trend in all soils: NUFs ∼ vinasse ≥ compost + NUF ≥ compost. Nitrogen uptake in the cabbage heads and total biomass was significantly higher in sand (69.5–144 kg ha−1) than loam (71.4–95.8 kg ha−1). All compost treatments alleviated the effect of soil type and resulted in comparable nitrogen uptake and yield in all soil types. Plant uptake of pharmaceuticals (Carbamazepin) was higher in sand than in loam, and concentration in the edible part was lower than in the outer leaves. In conclusion, NUF alone appears to be a promising successful fertilizer substitute in horticultural food production. The combined application of NUF and compost led to slightly lower crop yields, but may increase soil carbon content in the long term, promoting climate-friendly food production.
1 Introduction
In view of a growing world population and the human alteration of nutrient cycles, including nitrogen (N) and phosphorus (P) (Rockström et al., 2009), transforming food production is a major challenge of this century (Springmann et al., 2018). Both N and P are essential nutrients for healthy plant growth in crop production; however, their addition to synthetic fertilizers is currently organized in a linear economy. The Haber-Bosch process, used to generate plant-available N from its airborne unreactive form, is energy intensive, depending on fossil fuels such as natural gas, and associated with high greenhouse gas emissions (Wang et al., 2021). P is obtained from finite, depleting phosphate rock resources and its mining is increasingly more expensive and polluting (Desmidt et al., 2015). This background emphasize the need for significant improvements of nutrient management in agriculture and for alternative, circular N and P sources to achieve global food security (Gerten et al., 2020). Therefore, circular economy is a key strategy emphasized in policies and laws, e.g., by the circular economy action plan under the Green Deal of the European Commission (2020).
Current practices include the recycling of nutrients from livestock farming, biogas production or composting of agricultural and domestic residues. In recent years, novel recycling fertilizers (RFs) have become increasingly established–these are produced from biological or chemical processing of organic waste stream to recover nutrients (Mehta et al., 2015). Practical examples of RFs include vinasse, an organic RF produced by fermentation of biomass residues from bio-ethanol production (Silva and Gollo, 2014), or struvite (NH4MgPO4.6H2O), an organo-mineral RF derived from wastewater or sewage sludge ash P precipitation (Kumar and Pal, 2015). While struvite production allows for partly recycling of P from wastewater, N is not captured, but removed from wastewater through a combination of nitrification and denitrification. However, these treatment steps require 50–80% of the energy consumed by wastewater treatment plants (Fricke, 2009), lead to significant N losses due to emissions of the potent greenhouse gas N2O (Vasilaki et al., 2019), and are often still insufficient. Notably, despite nitrification treatment, sewer systems contribute significantly (estimations lie between 25 and >50%) to the total N input to aquatic ecosystems in Germany (umweltbundesamt UBA, 2020) and worldwide (Tuholske et al., 2021). Moreover, most nutrients (e.g., P, K, S, Ca) remain in the sewage sludge, where cross-contamination occurs with pharmaceuticals, heavy metals and microplastics from sources other than toilets, which impedes field fertilization with sewage sludge.
Nevertheless, human excreta can be a key source for ‘urban mining’ of N and P (Mihelcic et al., 2011; Chowdhury et al., 2014; Esculier et al., 2019). Because human urine and feces contribute significantly to the nutrient load to a central sewage system, i.e. up to 80% of N and 60% of P in wastewater (Herrmann and Klaus, 1997; Simha and Ganesapillai, 2017). Research over the last decade has demonstrated that source separation of human excreta is a viable path for the integrated recycling of plan nutrients, including N and P (e.g., Larsen et al., 2013; Harder et al., 2019). Most approaches for recycling-oriented source separation involve the use of waterless/dry toilets that either collect urine and feces together or separately (Larsen et al., 2013; Londong, 2016). Besides the potential to significantly increase nutrient recovery, the benefits of a separate collection of human excreta comprise inter alia the decreased use of freshwater and the reduced harm to aquatic environments through high nutrient loads or dilution of trace substances (Morgan, 2007; Remy and Jekel, 2008; Bisinella de Faria et al., 2015; Bradford-Hartke et al., 2015; Ishii and Boyer, 2015; Kjerstadius et al., 2017; Hilton et al., 2021; Larsen et al., 2021; McCartney et al., 2021).
Following the collection and separation of excreta, there are various options of processes for their treatment and recycling (cf. Harder et al., 2019). RFs produced from source-separated human excreta include, among others, nitrified urine or fecal compost. During the technical nitrification of urine, N in (temporarily stored) urine, is present in the form of NH3 or NH4+ (which are in equilibrium) and is biologically oxidized to NO3− (Udert and Wächter, 2012; Fumasoli et al., 2016). The NH4+/NO3− ratio can be adjusted by adding a base (increasing pH) (Bornemann et al., 2018), resulting in RFs suitable for different types of plant production. Nitrified urine is a RF with a high content of mineral N (Nmin) and, therefore, produces a good short-term fertilizing effect (Krause et al., 2021). For the treatment of solid contents from dry toilets, composting is an effective sanitation option (Vinnerås, 2007; Ogwang et al., 2012; Tilley et al., 2014; Krause et al., 2015; Sangar et al., 2015). Fecal compost contains a significant proportion of organically bound N, a higher carbon (C) to N ratio compared to urine-based RF, and an availability of nutrients to plants similar to that in composts derived from plant or animal materials (Krause et al., 2021). In compost, nutrients are bound mainly in the organic matrix and can be mineralized–they therefore produce a long-term fertilizing effect, further contributing to humus reproduction, as well as C binding and storage in the soil (Heinonen-Tanski and Van Wijk-Sijbesma, 2005; Krause et al., 2016).
A positive fertilizing effect, based on content and availability of plant nutrients, has been demonstrated for nitrified urine as well as fecal compost. Empirically, urine-based RFs are comparable to mineral fertilizers containing urea, NH4+ or NO3− or PO43- (Arnold and Schmidt, 2012; Meyer et al., 2018) and have been proven to be suitable RF in the cultivation of inter alia maize, beans, wheat, barley or miscanthus (e.g., Andersson, 2015; Andreev et al., 2016; Viskari et al., 2018). Studies on fecal composts conducted in African countries revealed that crop productivity can improve when fecal compost is used as a soil amendment (e.g., Niwagaba et al., 2009; Ogwang et al., 2012; Krause et al., 2016). Previous experiments with lettuce showed that fecal compost can support plant growth to a certain extent, but does not provide sufficient N for horticultural production (Schröder et al., 2021).
Other important considerations for human excreta-derived RFs are the associated risks of transmissible human pathogens and of pharmaceutical residues as pollutants (Krause et al., 2021). The majority of treatment approaches for dry toilet contents integrate processes of pathogen inactivation (“hygienization”) and pollutant elimination, especially pharmaceuticals, to ultimately recycle the remaining nutrients safely (ibid.). Hygienization can be achieved by heat exposure and distillation of urine (Udert and Wächter, 2012) and pasteurization or thermophilic composting of the fecal matter (Feachem et al., 1983; Bundesrepublik Deutschland, 2015). With regards to pollutant elimination, only a small proportion of pharmaceuticals is likely to be removed during storage and biological treatment, i.e., nitrification, of urine (Özel Duygan et al., 2021). Further removal to below the limit of detection (LOD) can be achieved with activated C filtration of urine (Köpping et al., 2020; Özel Duygan et al., 2021). Pharmaceuticals contained in the solid contents of excreta can be largely eliminated by means of thermal composting (Heinzelmann, 2020; Eberhardt, 2021). However, little is yet known about the distribution or concentrations of pharmaceuticals in the environment (cf. Krause et al., 2021). The distribution of antibiotics in soil and their uptake by edible crops has been studied for wastewater applications and animal manure (Carter et al., 2014; Pan and Chu, 2017), or stored urine (Winker et al., 2010; Arnold and Schmidt, 2012; Christou et al., 2019), but has not yet been investigated for RFs such as nitrified urine of fecal compost.
In summary, research shows a strong potential for nutrient cycling from human excreta collected in source-separating toilets and treated appropriately in novel recycling-oriented sanitation approaches. However, more studies applying these novel RFs in modern food production systems are required. Furthermore, the combination of nitrified urine with fecal compost could take advantage of the aforementioned properties of both RFs. Therefore, the present study was designed as a proof-of-concept for the use of RFs from dry and separately collected human excreta in open-field horticulture. The RFs were evaluated against a conventional organic fertilizer and studied in three different soil types. The main objective was to assess the RFs regarding biomass production, N efficiency and pharmaceutical risk potential due to plant uptake. Moreover, we discuss the benefits and risks of applying the recycling products as fertilizers, e.g. hygienic requirements, quality as soil amendment, and potential salinity problems by addition of NaCl via human urine.
We addressed the following research questions: i) How do RFs perform against the conventional product vinasse in cabbage cultivation? ii) How does the N-fertilizer effect of the combined application of nitrified urine and fecal compost compare against the application of either RF alone? iii) How does the soil type affect the N-fertilizer value? iv) Which pharmaceuticals are taken up by the crop, at what concentrations, and in which compartments (leaves, head)? and v) Does the soil type (sand vs. loam) affect uptake of pharmaceutical compounds by the crop?
2 Material and methods
2.1 Experimental set-up
2.1.1 General set-up
The field experiment was conducted 20 km south of Berlin, at the Leibniz Institute of Vegetable and Ornamental Crops (Großbeeren, Germany; 52° 33′ N, 13° 22′ E) between June and October 2019 (Supplementary Figure S1 and S2). An open-field experimental plot system was utilized with three different soil types: i) Arenic-Luvisol with silty sand and 5.5% clay (‘sand’); ii) Gleyic-Fluvisol with heavy sandy loam and 27.5% clay (‘loam’); and iii) Luvic-Phaeozem with medium content of clayey silt and 17.2% clay (‘silt’) (Rühlmann and Ruppel, 2005). Soil physical characteristics and organic C (Corg) concentrations of comparable plots nearby, with similar soils are listed in Supplementary Table S1 as approximate guide value. The twenty-four 4-m2 (2 × 2 m) plots were originally set up in 1972. They are 0.75 m deep with an upper 0.5 m layer containing the soil material and a 0.5–0.75 m layer consisting of a coarse-sandy drainage layer. The plots were arranged in a 12 × 2 box plot formation for each soil type separated by embedded stone walls and a concrete base including a drainage opening. The crops previously grown on these plots between 2010 and 2018 included 1 year marigold, than 6 years nasturtium, and lastly 2 years melon. For the present study, the model plant selected was white cabbage organic cultivar ‘Dottenfelder Dauer’ (Brassica oleracea var. capitata f. alba).
Before fertilization and planting, soil samples were taken from a depth of 0–30 and 30–45 cm in each plot. For each soil type, samples from the two depths were combined to measure pH, Nmin, P, K and Mg (for methodology see Section 2.3.2).
For each type of soil, six fertilizer treatments were set up, including compost amendments, liquid organo-mineral fertilization with recycling products, and the combination of novel organic and organo-mineral fertilizers (see Section 2.2). For each treatment and soil type, four replicate plots were established (n = 4) and arranged in a randomized two block approach. The box plot of each soil type was separated in the middle (6 × 2 plots) from west to east into the two blocks, with two replicates placed randomly per block. Sixteen cabbage plants were planted in each plot, in a 4 × 4 arrangement, with within-row and intra-row distances of 40 and 60 cm, respectively. Fertilizer requirement for each soil type was calculated according to the German fertilizer ordinance (Bundesrepublik Deutschland, 2019) (cf. Supplementary Table S2). With the exception of the sole compost treatment, the fertilizer requirement was split into two applications: the first, supplying 30% of the N demand, before transplanting seedlings to the field, and the second, supplying 70% of the N demand, during the plants’ vegetative growth period (see section 2.2.2).
2.1.2 Growth conditions
The cabbage plants were grown under greenhouse conditions until the two-to three-leaf stage, transferred in pots to outside climate conditions for 2 weeks, and were then planted in the field at the three-to four-leaf stage in mid-June 2019. The total duration of cultivation in the open field was 140 days.
During the growth period, all plots were covered by a net for protection against the white fly and other pests. Yet, around 8 weeks before harvest, first caterpillars (Pieris brassicae) were noted. When their number continued to grow, an ecological crop-protecting agent (NeemAzal®; BayWa AG, Germany) was applied (3 L ha−1) 6 weeks before harvest. Overhead irrigation was implemented according to the irrigation software BEREST (Gutezeit et al., 1993). BEREST models irrigation by estimating soil water content, expected evapotranspiration and precipitation over 5 days, using the daily soil water content in the rooted soil layer, the soil water-holding capacity, the plant growth stage, and the potential evapotranspiration as input variables. The temperature (reflectometer PT100b1/3 DIN; Messtechnik Geraberg GmbH, Geratal, Germany) and matric potential (tensiometer T22968, transmitter ES 1075; bambach GbR Tensio-Technik, Geisenheim, Germany) were recorded for each soil type at 10-cm soil depth during the field experiment in four replicates. Precipitation, irrigation, as well as air and soil temperature during the growth period are depicted in Supplementary Figures S3 and S4.
2.2 Fertilizer treatments
2.2.1 Tested RFs
Four RFs were tested and used in the study: Two nitrified urine fertilizers (NUFs)—Aurin and C.R.O.P.® (CROP), a compost made from solid contents collected from dry toilets (fecal compost), and a certified vinasse.
The NUF Aurin is a certified liquid RF from human urine, permitted for horticultural applications in Switzerland, Liechtenstein and Austria (VunaNexus, 2021). The so-called VUNA process (from “Valorization of Urine Nutrients in Africa” (Eawag Engineering, 2015)) was developed at the Swiss Federal Institute of Aquatic Science and Technology (Eawag), and commercialized by VUNA GmbH (Dübendorf, Switzerland). For Aurin production, stored urine is derived from source-separating toilets (save! LAUFEN Bathrooms AG, Laufen, Switzerland) installed at the main research facility of Eawag. The source-separated urine is collected in the basement of the building, and processed in a VUNA demonstration plant. Through the VUNA process, urine is first biologically nitrified to a NH4+/NO3- ratio of 1:1, then filtrated through activated C to remove trace substances and pollutants, such as pharmaceuticals and hormones, and finally concentrated by distillation to reach a 95–97% volume reduction (Fumasoli et al., 2016; Köpping et al., 2020).
CROP, the other NUF product used in this study, is also produced via nitrification, and by using a trickling filter comprised of a biofilm with lava particles as colonization media (Bornemann et al., 2018). Compared to the VUNA process, nitrification in the CROP production process is prolonged to a NH4+/NO3− ratio of >1:3 by adding shell limestone to increase pH. The CROP batch used in this study was produced from synthetic urine, synthesized as described in Feng and Wu (2006), mimicking the composition of stored human urine.
The fecal compost originated from mobile dry toilets put up at various festivals in Northern Germany. In these toilets, human excreta are collected without water, and without separation of urine and feces; thus, the liquid and solid excreta are collected together, mixed with sawdust and toilet paper. However, the urine runs through the solid matter, and this leakage is collected in a tank and disposed via the sewage system. Hence, the collected fecal matter is enriched by urine and the N it contained. Sawdust is added after each use of the toilet, during storage, and before delivery to the composting plant, to reduce odor. Generally, residue from dry toilets comprises a mix of human feces (∼30% Vol.), toilet paper (∼13% Vol.), sawdust (∼50% Vol.), and straw used as drainage material in the collection bins (∼7% Vol.). Composting was carried out by a professional compost-producing company (Birkenhof Kompostier-und Lohnunternehmen Schulze-Kahley GmbH, Lindendorf, Germany). Overall, ∼40 t (∼30 m³) residues from dry toilets were mixed with 4.25 t (∼10.2 m³) green cuttings and 0.7 t straw (∼3 m³) as structural material, and arranged into two compost windrows. During the 6-month composting process, the temperature was constantly monitored in three locations inside the compost windrow and three on the surface; this remained at >55 °C for the first 42 days, and >40 °C for the remaining duration of the process. The final product was mixed, sieved and stored under a plastic cover until further usage. The bulk density of the fecal compost was determined to be 472.5 kg m−³.
The fourth fertilizer used–vinasse–is a by-product of the biomass distillation from sugar, starch crops or cellulosic material. This is normally composed of 93% water and 7% solids with high levels of salts and organic compounds (Carrilho et al., 2016). The product used in the present study (Aminofert®, BayWa AG) is certified for organic farming and is a commonly used organic fertilizer product; as a conventional, commercial organo-mineral fertilizer, this served as the control treatment in our experiments.
2.2.2 Treatments
Using the aforementioned four RFs, six fertilizer treatments were applied: i) Aurin alone, ii) CROP alone, iii) fecal compost alone, iv) vinasse alone, v) fecal compost + Aurin, and vi) fecal compost + CROP.
The N demand of the white cabbage crop was estimated to be 200 kg N ha−1 (Feller et al., 2011), or 0.08 kg N per 4-m2 plot. Fertilizer requirement was subsequently calculated according to the German fertilizer ordinance (DüV, 2017), taking into consideration soil Nmin, the previous crop, and a markdown for lower expected yields due to additional N supply from crop residues in the plots (cf. Table 4, appendix four in DüV (2017); also see Supplementary Table S2), resulting in 100 kg N ha−1 or 0.04 kg N per plot (Supplementary Table S3). Based on the Nmin content of the soil, the three different soils slightly varied in application rate (Supplementary Table S4).
The dosing of the fertilizers was calculated based on crop N demand and assuming N availability of 100% for the two NUFs (equivalent to synthetic mineral fertilization), 30% for the fecal compost (equivalent availability to pig manure after DüV), and 55% for vinasse (according to Feller et al., 2017). All compost-treated soil received the whole compost dosage at once. Therefore, the fecal compost was incorporated in the top 10-cm soil layer by hand hoe 1 week prior to planting. For all other fertilizers, the application was split into two doses, as often done in horticultural practice: 30% of the estimated N demand was applied to the plots 1 week before planting, and the remaining 70% within the beginning of head-building phase, approximately 6 weeks after planting and when crop nutrient demand is at its highest. The NUFs and vinasse were diluted accordingly to ensure the same water supply for each plot. Liquid fertilizer-water mixes were applied using a watering can. For the first application before planting, the liquid products were poured homogeneously on the soil, whereas the second dose was applied close to the soil surface underneath the leaves of each cabbage plant (Supplementary Figure S5). The total amounts of nutrients applied are listed in Supplementary Table S4.
2.3 Harvesting, sampling and analysis
2.3.1 Harvesting and sampling
All cabbage plants were visually assessed before harvest. Each plant was rated on the basis head size and color of the outer leaves. The nutritional N status was evaluated according to the color of the old leaves (green, yellow-green, yellow, yellow-purple, purple). Score data were normalized to 100% to reflect the proportion of plants with nutritional deficiency and the overall severity within the plot.
The cabbage was harvested 140 days after planting in the field. Ten out of the 16 plants per plot were selected as representative specimens, excluding outliers such as those with abnormal growth or visible damage (Supplementary Figure S5). The plants were sectioned into the head, leaves and stem. Each plant part was weighed to determine mass of fresh matter (FM), then chopped into compound samples in the size of about 2 by 2 cm. Three subsamples of the plant compartments were assessed: One was dried at 60 °C until constant weight and ground to < 0.25 mm to analyze the N concentration; another was dried at 105 °C until constant weight for dry matter (DM) analysis; the third was frozen and stored at −20 °C to stop any microbial or enzymatic activity, for analysis for pharmaceutical compounds. The remaining six plants in the plot were harvested, split into head and leaves, and their weight was included in the calculation of the complete FM yield per plot.
After harvesting the plots, soil samples were taken. Six soil samples per plot were taken randomly from the top 45-cm layer, and mixed. Three ∼200-g subsamples were taken: One was dried at 105 °C until constant weight, with initial and final weight recorded and used for humidity calculations; another was dried at 37 °C until constant weight and stored for total N analysis; the third was frozen at −4°C to stop any microbial or enzymatic activity, until Nmin analysis.
2.3.2 Fertilizer, soil and plant analysis
For quality assurance of the fecal compost, thorough analyses were carried out at an external laboratory (AWV - Dr. Busse GmbH, Plauen, Germany) prior to treatments, according to DIN SPEC 91421 (DIN, 2020). The components analyzed included DM content, pH, organic substance content, nutrient content (NH4+, Ntot, P2O5, Ptot, CaO, Fe), heavy metal content (As, Pb, Cd, Cr, Cr VI, Cu, Ni, Hg, Tl, Zn) and potential pollutants such as organic compounds [adsorbable organic halogens, polychlorinated biphenyls (PCBs), polychlorinated dibenzodioxins and -furanes, dioxin-like PCBs, perfluorinated tensides, and polycyclic aromatic hydrocarbons](see Supplementary Table S5). The fecal compost was further subjected to microbiological analyses–hygiene testing–at an external laboratory (LUFA Nord-West, Oldenburg, Germany), including measurements of total aerobic bacteria and colonies of fecal coliforms Escherichia coli, fecal enterococci and Salmonella spp. (according to methods C1 to C4 in BGK Kap. IV; 2006-09, see Supplementary Table S5).
The Aurin and CROP fertilizers were analyzed to outline their nutrient composition profiles. Nmin measurements (NH4-N and NO3-N) were obtained by flow injection analysis (FIAmodula; MLE GmbH, Dresden, Germany) according to the official method VDLUFA A 6.1.4.1 (Verband deutscher landwirtschaftlicher Untersuchungs-und Forschungsanstalten (VDLUFA), 2012). For the analysis of the other nutritional elements (P, K, Ca, Mg, Na, S, B, Fe, Mn, Zn, and Cu), the samples were digested in HCl 0.025M, diluted 1:10 (if needed) and analyzed by ICP-OES (iCAP™ 7,400 Duo; Thermo Fisher Scientific GmbH, Dreieich, Germany) according to DIN EN ISO 11885. For chloride (Cl), samples were analyzed by IC (Dionex DX 120; Thermo Fisher Scientific GmbH, Dreieich, Germany) at the Institute of Ecology, Department of Soil Sciences, Technische Universität Berlin. The nutrient composition of vinasse was provided by the manufacturer.
Soil Nmin (NO3−-N + NH4+-N) was measured photometrically by flow injection analysis in a 1:4 dilution with 12.5 mmol. Parameters CaCl2, K, P, Mg and pH were conducted by an external laboratory (Eurofins Agraranalytik Deutschland GmbH), according to methods A 6.1.4.1 (CaCl2), A 6.2.1.2 (K, P, Mg) and A 5.1.1 (pH) (Verband deutscher landwirtschaftlicher Untersuchungs-und Forschungsanstalten, 2012).
Basic soil characteristics are presented in Table 1 and the nutrient composition profiles of the RF tested in this study are presented in Table 2.
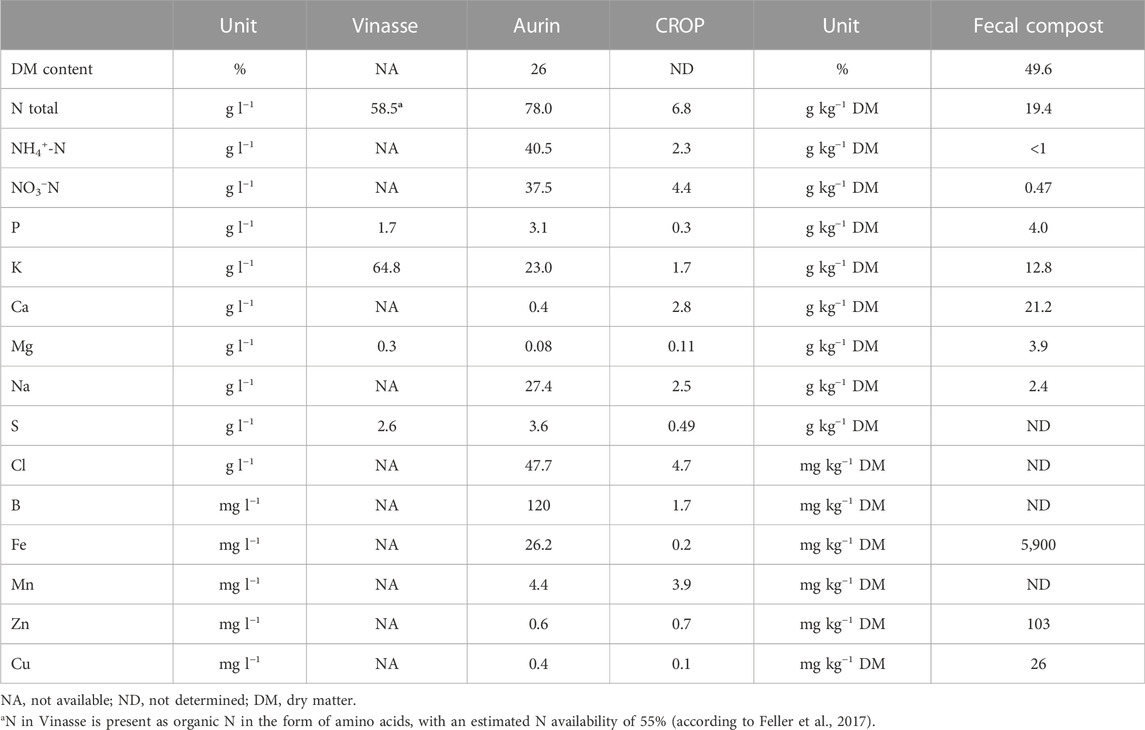
TABLE 2. Composition of the fertilizers tested in this study. The nutrient composition of vinasse was provided by the manufacturer. The Aurin and CROP fertilizers were analyzed as described in section. The fecal compost at an external laboratory for thorough quality assurance according to DIN SPEC 91421 (DIN, 2020); more data shown for the fecal compost in Supplementary Table S5.
Analysis was carried out only on plant samples from the sand and loam soil plots, not silt, due to capacity issues. Loam and sand were chosen to allow for the evaluation of the N fertilizer potential in two distinct soil textures. C:N ratio in the dry plant tissue samples was assessed by elemental analysis (Vario EL III Element Analyzer; Elementar Analysensysteme GmbH; Langenselbold, Germany) at the Institute of Ecology, Department of Soil Sciences, Technische Universität Berlin according to method MB1-A 2.2.5 (Verband deutscher landwirtschaftlicher Untersuchungs-und Forschungsanstalten, 2012).
2.3.3 Analyses of pharmaceutical compounds
Initially, target screening was conducted on a mixed sample of the fecal compost at the Helmholtz Centre for Environmental Research (Leipzig, Germany) to assay for a wide range of compounds. The sample was frozen at -20 °C for transport. Extraction of 1 g compost sample was done in duplicate, according to (Machate et al., 2021) and measured via LC-HRMS target screening, searching for 433 compounds. For the LC-HRMS measurement a Thermo Ultimate 3000 LC system coupled to a quadrupole-Orbitrap instrument (Thermo Q Exactive™ Plus) with electrospray ionization, was used, as described in detail by AOAC International (2012), Nanusha et al. (2020).
The initial target screening provided the baseline for a second level of targeted analysis, performed at the Laboratory for Organic Trace Analysis and Natural Substances (Institute for Ecology, Technische Universität Berlin). This included an adapted solid-phase extraction method for specific pharmaceutical compounds, with a lower LOD and targeted extraction solvents. Based on the first-line screening report, substances with concentrations >2 μg kg−1 were selected for second-line analysis (and if a standard for the targeted analysis was affordable or available). In addition, substances not detected in the first-line screening, but which are commonly found in sewage sludge, were also included in the second-line analysis of freeze-dried compost samples. An adapted solid-phase extraction method for the select pharmaceutical compounds (method AOAC 2007.01 of Lehotay et al. (2007)) was employed to determine whether the substances were absent from the compost, or whether they were present in concentrations below the detection limit of the initial target screening. Compost as a substrate is difficult to analyze; however, with this adapted extraction method matrix effects can be avoided, and even low concentrations can be detected and measured with LOD of 0.0703 μg kg−1 DM.
For the targeted solid-phase extraction, fresh plant and compost samples (in duplicated) were ground with a mortar and liquid N. Once the samples were milled, they were stored at -18 °C in a clean labeled Falcon tube. The detailed sample extraction is described in the Supplementary Material and Method (Supplementary section S3.1) Analysis of selected compounds (cf. Table 8) was carried out by means of HPLC-MS/MS (Agilent 1,260 Infinity—6,460 Triple Quad LCMS; Agilent) with a core-shell reversed phase column (Kinetex™ 2.6 µm C18 100 Å; Phenomenex Inc.).
2.4 Calculations and statistical analysis
N uptake by plant biomass was calculated for each plant compartment (head, leaves, stem) separately using the following formula:
The sum of N uptake in all plant compartments was assigned as N in aboveground biomass, while that in the stem and leaves was summarized as N in harvest residues. Yield and N uptake data were analyzed for each soil type separately using one-way ANOVA with a mixed model procedure (SAS v9.4). Statistically relevant differences (‘honestly significant difference’, HSD) were evaluated by Tukey’s test (α = 0.05).
The experimental design did not allow for a two-way ANOVA of fertilizer and soil type, as the soil type only presents a pseudo replicate. By considering the three different soil types as different experimental units, and hence separate environments, the fertilizer model could be adapted. Recommendations by Moore and Dixon (2015) were followed to account for variability among different environments (soil types), as described by the following model of Dixon, Moore and van Santen (2018):
yijk = the measured response, either yield, total FM or N uptake on the plot with treatment k in block j in soil i. µ = overall mean, Si = effect of soil type i, βij = effect of block j in soil type i, τk = effect of treatment k, Sτik = interaction effect of treatment k in soil type i, and εijk = experimental error for plot jk in soil type i.
Fertilizer treatment, soil type and block were included as fixed effects, whereas block nested within soil type [block (soil type)] were included as random effects. The SAS MIXED procedure (method, type3) was applied to analyze the model, as described by Moore and Dixon, (2015) and Dixon et al. (2018).
Pharmaceutical compound uptake was evaluated as described for yield and N uptake: First, via one-way ANOVA using the mixed model procedure for loam and sand separately, then by two-way ANOVA and the model from Dixon et al. (2018) to account for the effect of soil type and interaction between fertilizer and soil type. Bar plots for the results on pharmaceutical compound uptake were built using R (Core Team, 2021).
3 Results
3.1 Yield and produced biomass
Overall, marketable cabbage yield ranged between 33.9 and 72.3 Mg ha−1 with a total aboveground FM biomass of 71.6–121 Mg ha−1 (Figure 1, Supplementary Table S6). In all soil types, a trend in the resulting yield and biomass was observed for the different fertilizer treatments: Vinasse ∼ Aurin ∼ CROP ≥ compost + NUF ≥ compost. The NUFs resulted in comparable yield and total biomass production, similar to that of the control treatment with vinasse. Fecal compost led to the lowest yield and total biomass, but the combination of compost and NUF led to increased outputs in all soil types. Overall, total biomass, and, to an extent, the head yield was comparable between the vinasse and compost + NUF treatments.
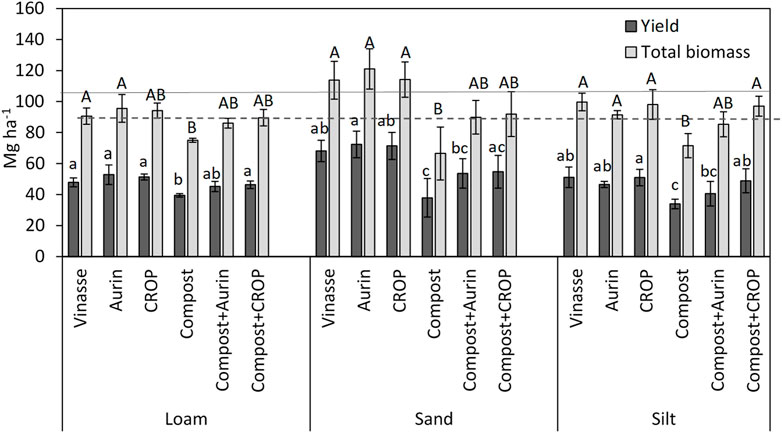
FIGURE 1. Marketable yield and total aboveground fresh matter biomass (Mg ha−1) including the head, leaves and stem of white cabbage plants grown in three different soil types. Error bars indicate standard deviation (n = 4). Different letters reflect means differing significantly from one another (HSD, Tukey test, α = 0.05, n = 4). Horizontal lines depict expected cabbage yields in organic (dashed line, 72 Mg ha−1) and conventional (solid line, 105 Mg ha−1) production (Kuratorium für Technik und Bauwesen in der Landwirtschaft KTBL, 2017). Data are provided as Supplementary Table S6.
3.2 N fertilizer value
N uptake in the cabbage heads ranged between 69.5 and 144 kg ha−1 in sand, and between 71.4 and 95.8 kg ha−1 in loam (Figure 2). Differences among fertilizer treatments revealed a similar overall trend as noted for FM yield, with certain peculiarities. In loam, the NUFs and vinasse resulted in a N uptake comparable to that of compost + CROP. Fecal compost application alone led to the lowest N uptake. In sandy soil, the differences among the outcomes of the treatments were more pronounced: Vinasse and the NUFs resulted in a comparable cabbage head N uptake, which was significantly higher than that observed for the compost and compost + CROP treatments. CROP fertilization alone led to comparable results as the combined application compost + Aurin. Cabbage head N uptake following the Aurin treatment was twice as high as that from compost application alone (Figure 2; Table 3).
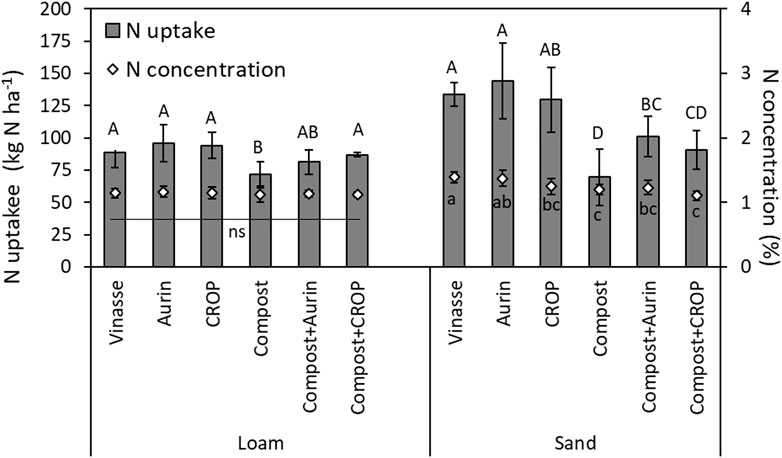
FIGURE 2. N uptake by the cabbage heads (kg ha−1; left y-axis) and proportion of N in the dry matter of the cabbage heads (“concentration”, right y-axis) in the loam and sand soil types. Error bars indicate standard deviation (n = 4). Different letters reflect means differing significantly from one another (HSD, Tukey test, α = 0.05, n = 4). NS = not significant.
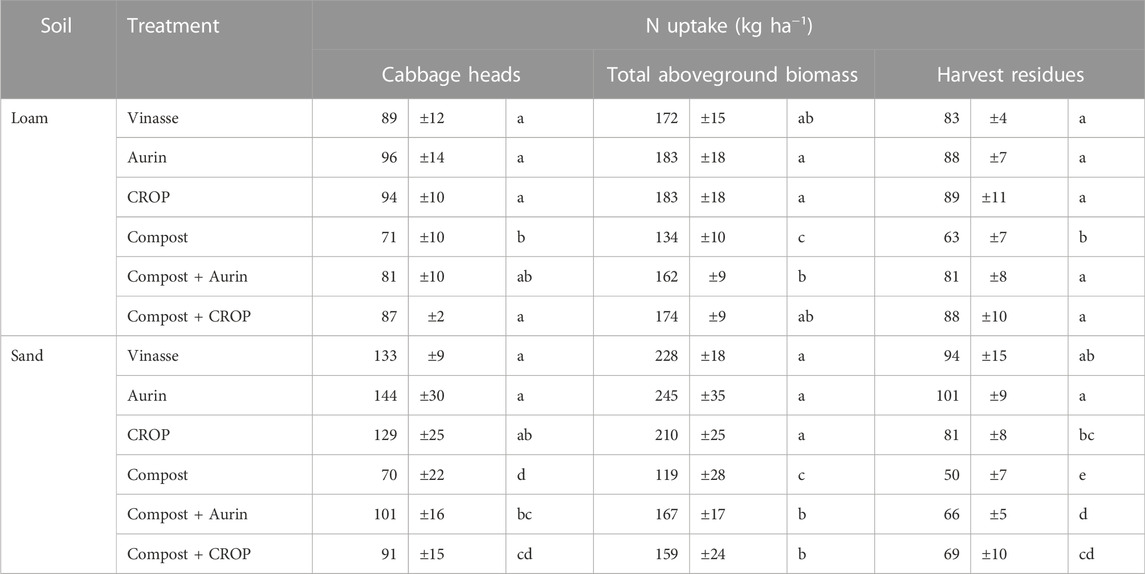
TABLE 3. N uptake in the marketable yield (cabbage head), total aboveground plant biomass and harvest residues (leaves and stem). Data are presented as the mean ± standard deviation (n = 4). Different letters reflect means differing significantly from one another (HSD, Tukey test, α = 0.05, n = 4).
The proportion of N concentration in the cabbage head dry tissue was comparable among all treatments in the loam plots (Figure 2, Supplementary Table S7). In sand, the N concentration tended to follow the pattern of N uptake: The vinasse and NUFs led to comparable outcomes; yet vinasse led to higher N concentrations than compost alone or compost + NUFs. The combination of compost + NUF resulted in similar N concentration as compost alone. Moreover, the Aurin application led to comparable proportions of N in the cabbage head dry tissue compared to Vinasse.
The N uptake by the total aboveground biomass of the plants ranged between 119 and 245 kg ha−1 and was almost double that measured in the marketable yield (cabbage heads alone) (Table 3). Uptake of N was significantly higher in the products of the NUF and vinasse treatments; in loam, the results from the compost + CROP treatment were of a comparable range. The lowest N uptake by the total biomass was observed for the sole compost application in both soil types. The amounts of N potentially remaining in the field in the form of harvest residues were comparable among most of the treatments in loam, but the compost treatment led to lower amounts. In sand, the estimated N in the harvest residues followed a partially similar trend as observed for the cabbage head N uptake: Vinasse, Aurin ≥ CROP ≥ compost + CROP, compost + Aurin > compost.
3.3 Influence of soil texture on fertilizer value
The treatment type and soil texture revealed significant interaction effects for all tested harvest parameters: Marketable yield (head FM), total aboveground biomass (FM and DM), cabbage head N uptake, and total N uptake (Table 4). The soil texture notably affected fertilizer performance, with a tendency for sand to result in better outcomes than loam or silt (Figure 1).
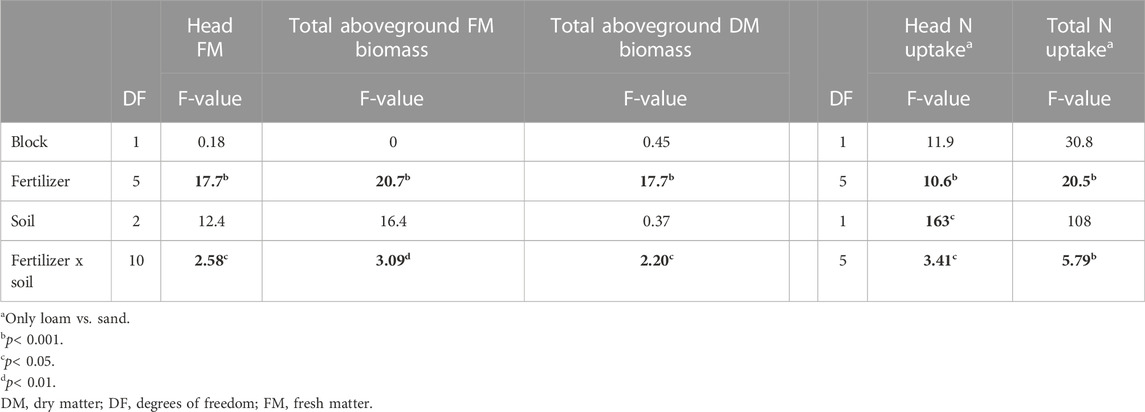
TABLE 4. Effect of soil type on fertilizer treatment, and the interaction effect. Significant F-values are in bold, generated by the type3 method and Kenward-Roger test.
However, all types of soil treated with compost alone resulted in comparable marketable yield and total aboveground fresh biomass (Table 5). This was also reflected by p-values >0.05 for the respective compost treatments and the soil interaction effects. The NUFs and vinasse fertilizer were significantly affected by soil texture, with higher marketable yields, biomass and N uptake in sand rather than in loam or silt (Table 5). The proportion of N concentration in the cabbage head tissue was significantly higher for the plants grown in sand than those in loam, in agreement with the higher N uptake (Figure 2).
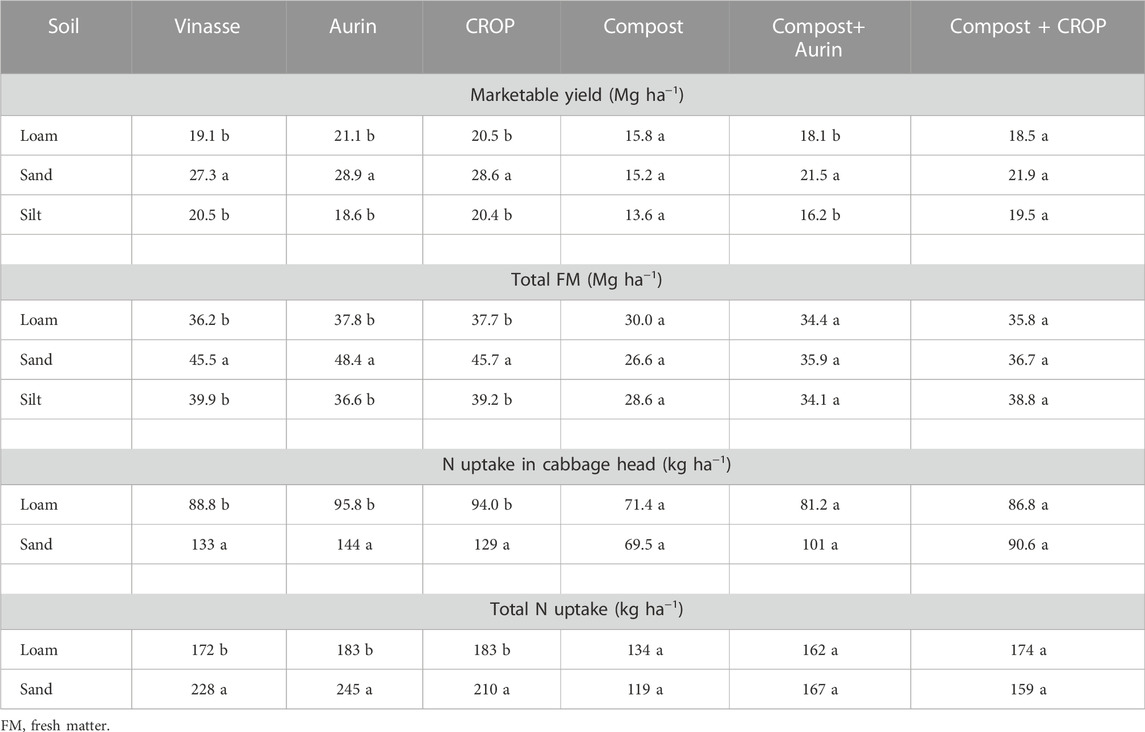
TABLE 5. Effect of soil type on fertilizer treatment. Different letters reflect means differing significantly from one another for soil types (HSD, Tukey test, α = 0.05, n = 4).
The Nmin after harvest was significantly affected by the soil type, with the following pattern being observed: Loam > silt > sand. Mean Nmin contents in the top 0–45 cm layers of soil were 25.8, 10.4 and 19.4 kg ha−1 in loam, sand and silt, respectively (Table 6). Soil NO3−-N made up the largest fraction within Nmin. Fertilizer treatments did not significantly affect the total Nmin content in sand or silt after harvest–differences on soil NH4+-N and NO3−-N according to treatment were observed only in the loam soil. Vinasse led to higher NO3−-N contents than compost + Aurin, while the compost treatment alone resulted in higher NH4+-N content than CROP.
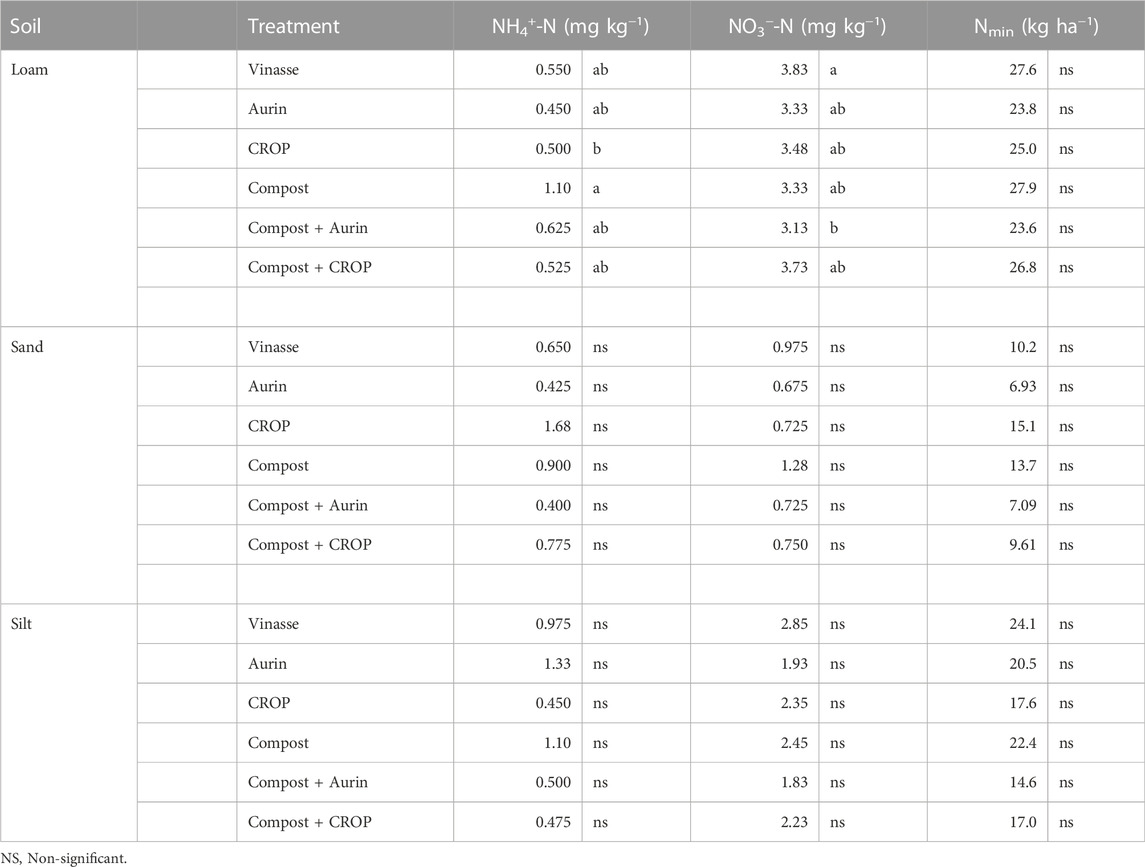
TABLE 6. Nmin content in the soil (0–45 cm depth) after harvest. Different letters among treatments within each soil, reflect means differing significantly from one another for soil types (HSD, Tukey test, α = 0.05, n = 4).
Finally, among the three soil types, sand brought about the least N deficiency, as indicated by more green leaves on the plants (Supplementary Fig. S6). Silt and loam both produced a large share of plants with strong deficiency symptoms in the leaves (purple and yellow-purple color).
3.4 Pharmaceutical compounds
3.4.1 Pharmaceutical content of fecal compost
The first target screening of the fecal compost sample revealed the presence of a wide range of chemical compounds, from pharmaceuticals to rubber additives, flame retardants, UV filters, corrosion inhibitors and insect repellants (Table 7). Out of 310 target compounds screened for, 20 substances were detected, with 11 being pharmaceutical compounds. The substances found in the highest concentrations were diphenhydramine, followed by salicylic acid, gemfibrozil and allopurinol (20.6–108 μg kg−1).
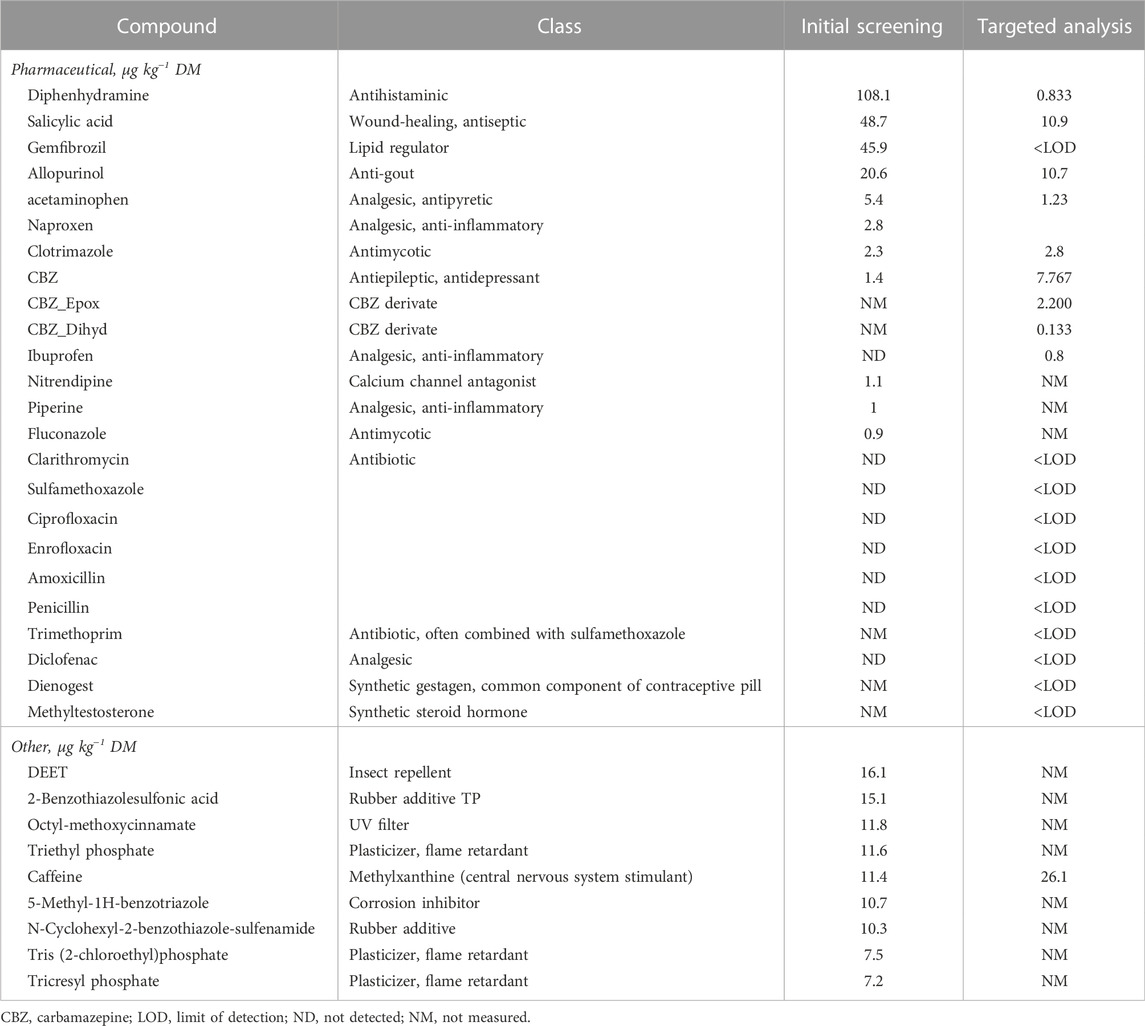
TABLE 7. Pharmaceutical and other chemical compounds detected in fecal compost, by means of an initial screening of 310 compounds, followed by a targeted analysis of specific compounds.
The second screening approach, involving a smaller and adapted set of target compounds, indicated slightly different results compared to the initial screening. For certain substances, the measurements were lower, particularly for diphenhydramine, salicylic acid and allopurinol. Other compounds appeared to be below the LOD (gemfibrozil and naproxen), whereas CBZ concentration was measured to be higher, and multiple forms of CBZ were detected (Table 7). Out of the 21 tested compounds, 11 were below the LOD in the second analysis. Ibuprofen was not detected in the initial screening, yet appeared at a low concentration (0.8 μg kg−1 DM) in the adapted solid phase extraction method.
3.4.2 Crop uptake of CBZ
Of all tested compounds, the cabbage plants appeared to contain only traces of CBZ and ibuprofen. According to personal communication with the lab manager, concentrations of ibuprofen were detected only in even lower trace concentrations compared to CBZ. In the following, we present and discuss results for CBZ.
The highest CBZ concentrations were measured in sand fertilized with compost, with 1.16 and 2.80 μg kg−1 DM detected in the cabbage head and leaves, respectively (Figure 3). In loam, only 0.06–0.21 µg CBZ per kg DM was detected across all treatments and plant segments–no significant differences were observed among CBZ uptake in the head and leaves from the different fertilizer treatments. The localization of CBZ inside the crop differed among plant compartments, with higher concentrations measured in the leaves than in the cabbage heads from both soils (p < 0.001). In the cabbage head tissue, very low CBZ concentrations were detected, with maximum values of 1.16 and 0.07 μg kg−1 leaf DM from sand and loam, respectively.
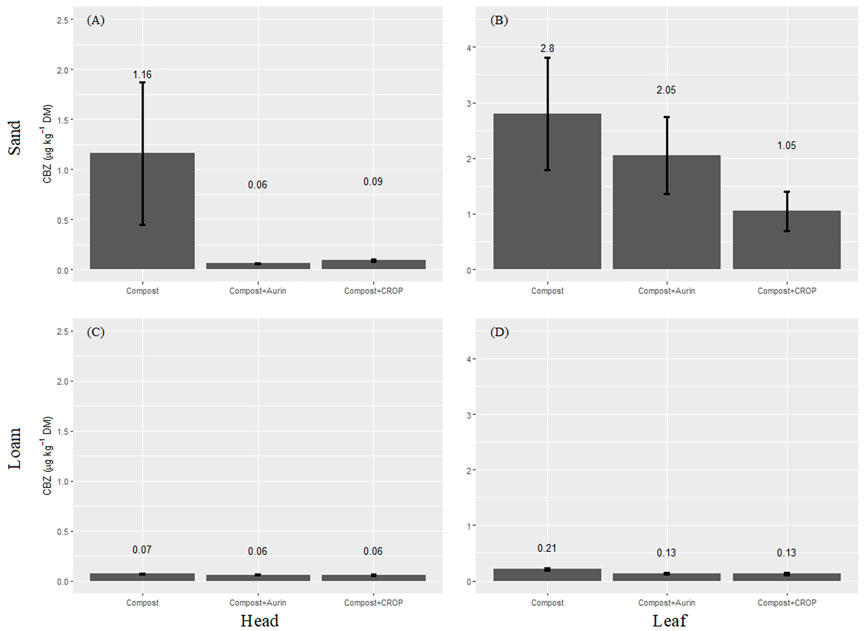
FIGURE 3. CBZ concentrations in cabbage heads (μg kg−1; (A,C)) and leafs (μg kg−1, (B,D) in the dry matter (DM) and for soil types sand (A,B) and loam (C,D). Data provided in Supplementary Table S8.
When testing for soil effects (Table 8), no significant influence of the soil type nor interaction effect was observed for CBZ content in the leaves or head of the cabbage plants. A significant fertilizer–soil interaction was observed for total CBZ uptake. Compost treatment had significantly higher CBZ concentration in the plants grown in sand (5.44 μg kg−1 DM) compared to loam (0.275 μg kg−1 DM). It should be noted, that application rate of compost varied in the different treatments and soils (Supplementary Table S4), which also lead to different amounts of pharmaceuticals being added to the soil. Therefore, the compost treatment resulted in significantly higher total CBZ uptake than the compost + NUF treatments in the sandy soil (Figure 3). A statistical indication for similar fertilizer–soil interaction effects was noted for the content in the cabbage head (p < 0.05), as well as a potential fertilizer–soil effect on leaf uptake (p < 0.01) (Table 8).
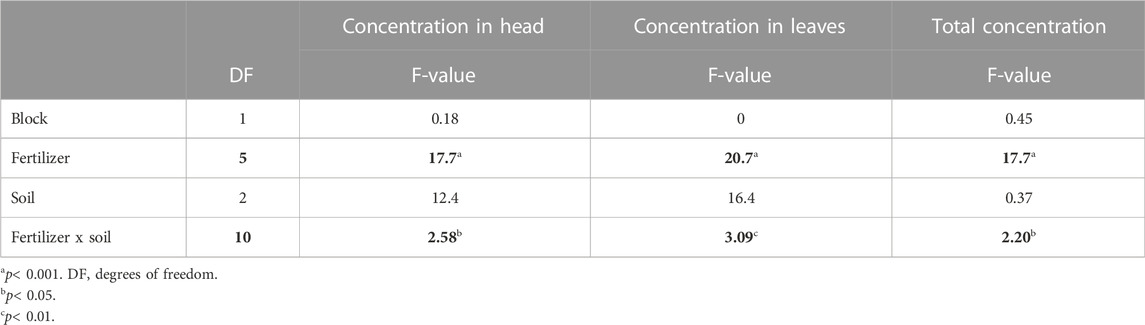
TABLE 8. Effect of soil type and fertilizer treatment on CBZ concentration per plant compartment, and the interaction effect. Significant F-values are in bold, generated by the type3 method and Kenward-Roger test.
4 Discussion
4.1 Fertilizer value of recycling products
The cabbage yields from sand fertilized with NUFs approached the marketable yield expected for organic cabbage production in Germany (72 Mg ha−1) (Kuratorium für Technik und Bauwesen in der Landwirtschaft KTBL, 2017). These were the highest yields achieved in the present study, and were comparable to those from low Nmin application rates (50 kg N ha−1 year) in another long-term study at the Leibniz Institute of Vegetable and Ornamental Crops (Großbeeren, Germany; unpublished data). Everaarts and De Moel (1998) found cabbage yields (cultivar Bently) under optimum N application (132–250 kg N ha−1) in different soils between 74–100 Mg ha−1. The N application rate in present study (∼100 kg N ha−1) seems understated compared to conventional cabbage production and other experiments, which can be explained by the experimental approach. The calculated application rate included all accountable N amounts (Supplementary Table S3) and also tried to approach the principle of organic production.
The two tested NUFs led to comparable yield and biomass production as the control vinasse treatment. In agreement, Pradhan et al. (2007) found that using stored human urine as fertilizer produced cabbage yields comparable to those from a conventional mineral NPK fertilizer (9:6:17). Another study demonstrated decreased yields of okra plants in a pot experiment when soil was fertilized with 100% stored human urine, explained by the acidifying and salinizing effects on the tropical soil that has been used (Sangare et al., 2015).
The N concentration measured in the cabbage heads (1.1–1.4% N) in the present study, irrespective of soil type, was lower than that reported by (Thorup-Kristensen and Sørensen, 1999). The authors found 1.7–2.4% N in DM of above-ground plant parts at harvest, with a total N supply of 160–400 kg ha−1. Although the N supply was much higher than in the present study, the total aboveground plant DM of 13.3–16.0 Mg ha−1 was of a similar range (10.1–17 Mg ha−1).
The differences in N uptake among treatments (e.g., vinasse and NUFs > compost) were expected, as they were related to availability of N and other nutrients provided with the respective fertilizers. In loam, the resulting total N uptake, as well as that in the cabbage heads, was comparable among treatments, except for compost. In sand, the differences among treatments were more pronounced and followed the supply of Nmin by fertilizer addition (Supplementary Table S4). The NUFs, with higher Nmin supply, appeared to be less efficient in loam than in sand. Therefore, it can be presumed that N was immobilized in the loam. Notably, on the topic of N efficiency in loam, the N uptake by the total aboveground biomass from compost + NUF treatments was equivalent to the sum of the respective uptake from compost and NUF treatments alone: when accounting for 30% of the sole compost N uptake plus 70% of the sole NUF uptake, the uptake from the combined treatments came close to this. However, in sand, the N uptake following the combined treatments were slightly lower (-40 to -48 kg ha−1) than the sum of calculated respective N uptake values from the sole applications. Compost addition to the sand may have enhanced microbial activity, and thereby immobilized a certain amount of N, accounting for the lower N recovery in the combined treatments.
Although sole compost application led to the lowest yields and N uptake compared with the NUF and control treatments, Schröder et al. (2021) found that similar fecal compost led to higher N and P availability compared to conventional green waste compost. In this context, the combined application of compost + NUF provides the advantages of both: A slow-release and short-term N fertilizer (Winker et al., 2009). In most cases, compost + NUF treatment increased yield and N uptake compared to compost alone, and yielded plant head and total biomass in quantities comparable to the control vinasse. Hence supplementing fecal composts with Nmin, e.g., via urine, can balance the N demand, as also concluded by Kelova et al. (2021). Sangare et al. (2015) tested different combinations of urine with fecal composts and determined that the optimal ratio for okra yield was 75% of N supplied by urine and 25% by compost. This ratio was comparable to the 30:70 application of compost + NUF in the present study.
The N remaining in the field in the form of harvest residues reached 101 kg ha−1 in sand fertilized with Aurin. A similar amount of N in cabbage crop residues was determined by Everaarts and Booi (2000), measuring values of 113 kg ha−1 on average, but with a much higher N application rate (mineral N fertilizer: 237–270 kg ha−1). Moreover, maximum head DM yield (9-10.5 and 8-8.5 t ha−1) at different sites with varying clay content, as reported by the authors, was in a comparable range as in present study for NUFs and vinasse in sand (8.7-9.5 t ha−1). Thus, it seems that the conditions in present study for sand resulted in higher N efficiency than those described in Everaarts and Booi. However, for the loamy soil, N efficiency among treatments appeared to be lower and indicated a higher N demand.
4.2 Effect of soil texture on fertilizer value
In contrast to our results, a long-term field trial at a nearby site at the Leibniz Institute of Vegetable and Ornamental Crops, employing a similar plot system and soil types, demonstrated higher yields of crops grown in loam and silt compared to sand (Ruehlmann, 2013). Soils with higher clay content generally tend to encourage improved cabbage growth, indicating that additional factors may have affected the yield from loam and silt in the present study.
The experimental N application rate by fertilizers herein was on a level lower than that in conventional production; hence, soil N mineralization was expected to supply additional N throughout the vegetation period. Differences in N uptake in loam and sand were predicted, but the results were unexpected. Loamy soils generally tend to have a higher N mineralization potential, related mainly to their higher content of organic matter (OM) and clay (González-Prieto et al., 1996; Ros et al., 2011). However, the higher yield and N uptake rates were noted in the plots with the sandy soil. Possible reasons for this include N immobilization or unfavorable growth conditions in loam, which is a heavier soil. The plots were regularly irrigated, thus excluding imbalances in the soil water balance. Still, loamy soils have a higher water holding capacity and during hot summer days the sandy top soil dried out very fast. Regarding unfavorable conditions, it could be suggested that the cabbage leaves in loam with higher water content were more attractive for the caterpillars (Pieris brassicae), which were noted around 8 weeks before harvest and later treated. These are only speculations, although first caterpillars were noted in loam and appeared to be in higher numbers in loam than in sand (visual impression). Also Gutbrodt et al. (2011) observed that soil draught increased feeding preference of Pieris brassicae on cabbage. Yet real draught events were excluded in present study.
A visual assessment of the plant nutritional status 2 weeks before harvest indicated a notably higher proportion of plants with yellowish-purple outer leaves in the loam and silt plots compared to those in sand, indicating N deficiency in the cabbage plants (Supplementary Figure S6) (Yara, 2022). Therefore, we suggest the main reason for the lower yield and N uptake in loam to be soil microbial immobilization and a general N limitation in the soil. Immobilized microbial N is expected to have an average turnover time of 1–2 months (Davidson et al., 1992). After harvest, soil Nmin in loam (treatment mean 25.8 kg N ha−1) was on average 15 kg N ha−1 higher than in sand (10.4 kg N ha−1). Although these are only comparably small differences related to total N uptake, it could be an indication of gradual remineralization of organic N in the loam.
The combination of compost + NUF, as well as the treatment with compost alone, resulted in comparable yields and biomass production in the two soil types, despite the differences in texture (Tables 4, 5). Hence, the application of compost negated the effect of soil texture. It is known that the addition of OM improves the soil’s physicochemical properties, particularly for sandy soils (Shepherd et al., 2002; Karami et al., 2012). Specifically, addition of OM in the form of fecal compost has been shown to improve the soil’s water-holding capacity and structure, as well as buffering its pH (Jönsson et al., 2004; Krause et al., 2016). The application of compost to sandy soil is expected to improve mineralization of organic N, as the well aerated soil with low Corg (Supplementary Table S1) and clay content could provide a faster C and N turnover. But this was not observed in the present study, where compost addition lead to comparable yields and N uptake among soils (Table 5).
4.3 Compost pharmaceutical content and crop uptake
The total pharmaceutical load in the compost, according to two analyses (Table 7), appeared to be several magnitudes lower than values reported for sewage sludge or animal slurry (Krause et al., 2021). For instance, in sewage sludge, the maximum concentrations of CBZ approached 680 μg kg−1, and 3,237 μg kg−1 for ibuprofen. The two screening approaches varied in detection of certain pharmaceuticals, as related to their methodology, and is further discussed in the Supplements (Supplementary discussion, Section 4.1).
In general, the low concentrations of drugs and other chemicals are in agreement with findings from Dalahmeh et al. (2022) and other studies (Butkovskyi et al., 2016), in which composting has been shown to significantly decrease the concentrations of pharmaceutical compounds compared to the parent material, and led to full degradation of most substances and hormones. In a 363-day sludge composting experiment, a large reduction of these was observed over time, and CBZ undetectable levels, with a half-life of 28 days (Dalahmeh et al., 2022).
The application rate of the compost and the concentration of pharmaceuticals in it appeared to affect the level of uptake in the plants. The higher CBZ concentrations in the plants following treatment with compost alone versus compost + NUF (in sand), are primarily due to the higher input rate via the compost, thus higher quantities of pharmaceuticals, which were added. Although the CBZ concentration in the compost appeared to be quite low, a trend could still be observed among the treatments. Notably, only CBZ was detected in the plant biomass, but not its metabolites, which were present in the compost (Table 7). In contrast, Goldstein et al. (2014) reported CBZ as well as the metabolites epoxy-CBZ and dihydro-CBZ present in tomato and cucumber leaves and fruits with spiked wastewater. Therefore, it is possible for these forms to be taken up by the plant; however, the lower concentrations of the two metabolites compared to CBZ in the compost might explain why any uptake was below the LOD.
It should be taken into account that white cabbage has a long cultivation duration (140 days), allowing time for microbial degradation in the soil (Dalahmeh et al., 2022). The gradual growth and development of the cabbage crop led to higher concentrations of chemicals in the outer leaves than in the marketable head product, likely because the head develops later than the leaves. It has been shown that translocation of pharmaceuticals and uptake into plants depends on a variety of factors, such as the environmental conditions, the crop type, the physicochemical properties of the compound and the soil amendment, as well as the soil texture and cation-exchange capacity (Leal et al., 2013; Camotti Bastos et al., 2020; Keerthanan et al., 2021). Hence, the type of soil can also affect uptake of pharmaceuticals into plants–as postulated by Christou et al. (2019)—particularly when comparing heavy-against light-textured soils. This was partially corroborated in the present study, by the higher measured CBZ uptake in the plants grown in sandy soil compared to loam with compost treatment (Figure 3 and Supplementary Table S8).
It has to be noted that compost application in sand was generally higher (approx. 1 kg or 30% of compost FM) than in loam, due to the calculated N demand and initial soil Nmin concentration. Yet, these higher amount of compost, as well as pharmaceuticals added in sand cannot alone account for the higher CBZ concentration in sand compared to loam. For example, concentration in the leaves was on average more than ten times higher in sand than in loam (Figure 3), hence cannot simply be related to 30% higher FM addition Therefore, the influence of soil texture still showed a visible impact in this study. Similar results for soil textural differences were reported in a lysimeter study of root vegetables in three different soils, where higher concentrations of pharmaceutical compounds were detected in the roots and leaves of plants grown in sandy versus loamy soil (Malchi et al., 2014). Pharmaceutical substances can bind to clay and OM particles, reducing the likelihood of uptake into the plant (Chefetz et al., 2008; Christou et al., 2019). Therefore, in soils poor in soil OM, the mobility of pharmaceutical compounds such as CBZ can increase significantly, as reported by (Chefetz et al., 2008).
Overall, the low concentrations of pharmaceutical compounds in the compost, as well as the low uptake in the plants, suggests a low risk for horticultural crop production. Dalahmeh et al. (2022) concluded that the remaining low concentrations of pharmaceutical chemicals found in the 363-day sludge compost could be metabolized by the soil microflora, and that composting may be a suitable treatment for degrading pharmaceutical substances and hormones.
4.4 Experimental limitations and short-comings
The experimental plot design shows the advantage to test different fertilizer treatments and different soil types at the same site within a small area, but also holds some limitations. The different soil environments need to be replicated as well to avoid pseudo-replication and to allow for analysis as Two-Way-ANOVA. Moreover, the soil filled into the embedded plots does not reflect a naturally developed soil type and only serves to assess different soil texture classes.
Regarding fertilizer treatments, vinasse could have undergone the same fertilizer analyses as the other recycling treatments to provide more precise data. However, as commercial product with high quality standard, the nutrient concentrations provided on the fertilizer package are expected to be in a reasonable accurate range. In general, a commercial mineral fertilizer treatment would have been advantageous to compare all recycling fertilizers regarding N availability and N efficiency. However, the initial experimental idea was based on evaluating the recycling fertilizers against other commonly used, commercial fertilizers containing recycled nutrients. The plot arrangement restricted the total treatment number, thus vinasse was favored as recycling fertilizer with high N availability.
In addition, soil Nmin analysis within the vegetation period could have improved discussion of the differences in N uptake between loam and sand and avoid speculations. Additional soil analysis of Corg and other nutrients, as well as soil physical parameters could have supported the discussion on the effect of soil texture on fertilizer value but were limited due to financial restrictions. A rough indication for physical parameters from comparable plots by Barkusky (2009) is provided in the Supplements (Supplementary Table S1), but only served as orientation. The Corg concentrations in the soils were already on a very low level with values ranging from 0.55 to 1.5%. Similar concentrations can be expected for the plots in present study, which probably have not greatly improved over the years, as the plots were intensively managed, and only received mineral N fertilizers and harvest residues as soil amendments. As Corg measurements were missing, as well as more detailed soil analyses, further discussion on the yield gap between sand and loam are limited and we would enter the realms of speculation to go beyond our current discussion.
In general, the experiment was conducted as fertilization experiment, with N fertilizer rate related to different soil Nmin levels. But the different application rates of compost among soils limited accurate evaluation of soil textural influence on pharmaceutical uptake by cabbage, although such effect still could be recognized.
4.5 Benefits and risks of the tested RFs
A suitable level of hygiene is a key prerequisite for the use of fecal compost. Once demonstrated, the compost can compete with conventional organic waste-based composts, and carries a lower risk of microplastics (Krause et al., 2021). However, the risk of human pharmaceuticals and hormones being added to soils is a relevant concern. The cabbage plants were therefore assessed for uptake of pharmaceutical substances, as discussed above. Standardized pharmaceutical analyses for composts are currently of limited availability, costly and time-consuming. Hence, a standardized quality assurance for fecal composts regarding pharmaceutical substances and hormones by laboratory analysis is needed (Deutsches Institut für Normung, 2020; Krause et al., 2021).
In general, the risk for human health of pharmaceutical compounds entering the food system by means of fecal compost use, seems low, as determined in our study. Cabbage exhibits a low-risk potential, as only the non-edible plant parts took up CBZ and ibuprofen. Even though measurable concentrations were detected in the biomass, these concentrations were extremely low and corresponded to 0.384 μg in an average cabbage head of 1.08 kg grown from the compost treatment (Supplementary Table S9), implying that >521,000 cabbage heads would be needed to accumulate a dose equivalent to one CBZ pill (200 mg). Such extraordinary high consumption rates were also calculated for wheat, where one would need to eat wheat grain for >100 years to ingest the equivalent of a daily treatment dose of CBZ (>400 mg) (Arnold and Schmidt, 2012).
For the NUFs, the established processing of e.g., Aurin, ensures the removal of pharmaceuticals and pathogens, therefore not providing a risk of pollutants entering the soil. Further research is needed on the long-term accumulation of NaCl by continuous urine application, and the potential mitigation by combined compost application. For instance, in the present study, treatment with the two NUFs led to application of 8.3–9.2 g NaCl per m2 (Supplementary Table S4). Pradhan et al. (2007) found no significant differences in Cl− concentrations in cabbage tissue when fertilized with stored human urine versus mineral NPK fertilizer. By contrast, Sangare et al. (2015) reported that application of human urine alone caused acidification and salinization in the soil, resulting in the lower okra yields. The study of Sangare et al. assessed the application of stored human urine onto Ferralsol filled in closed pots. El hasini et al. (2020) assessed different composts as amendments to alleviate soil salinity, and found composts rich in humic acids with a C/N ratio of 35 were the most suitable, likely due to the chelating ability of compost humic acids, removing Na from the soil solution. In the present study, with the tested soil types under controlled irrigation, salt stress was not considered as a potential threat from a one-time application. This is corroborated by Gómez-Muñoz et al. (2017), who found no negative impact from salt accumulation on crop yield after 11 years of repeated applications of human urine to Cambisol in Denmark. Tropical soils, such as Ferralsols or Andosols are often limited in nutrients or low in pH, hence prone to unbalanced fertilization, as observed in the studies by Sangare et al. (2015) and others (Baijukya et al., 2006). Supplying sufficient nutrients and improving the soil’s chemical and physical properties by addition of OM, is therefore essential for tropical soils. Krause et al. (2016) demonstrated that soil acidity could be better mitigated by the biochar-fecal compost, compared to standard compost application, emphasizing the positive effect of biochar as a soil conditioner.
The application of fecal compost and urine-based products as soil amendments and N fertilizers provides several benefits, as mentioned above. Any associated risk potential can be mitigated by a functioning compost process (Dalahmeh et al., 2022), as well as by the current techniques for urine treatment (Martin et al., 2020).
Despite the shown potential of the tested novel recycling fertilizers, a widespread applicability in open-field horticulture is inhibited due not to the legal situation. Unlike with other source materials, which are already used as fertilizers such as wastewater sludge, liquid animal manure, or bio-waste, there is no regulation for human excreta collected separately from the wastewater system. In order to support practical application of recycling fertilizers from contents from dry toilets there is thus the need for a clear legal framework, including an updated fertilizer regulation that accepts the use of quality-controlled recycled fertilizer from human waste. Precisely, human urine and human feces should be incorporated in a conclusive “approved list” that names the substances from which fertilizer may be produced in German fertilizer law (cf. Table 7, appendix 2, DüMV) or be included in the list of component material categories (CMC) of the European fertilizer law (cf. Annex II, EU Fertilizer Products Regulation).
5 Conclusion
RFs, and particularly NUFs alone or in combination with fecal compost, were revealed to be viable alternatives to commercial fertilizer products. The N fertilizer value of the NUFs was comparable to vinasse, and, in combination with fecal compost, resulted in comparable cabbage yields. The soil texture influenced the performance of the NUFs and vinasse (sand > loam), whereas compost application alleviated the soil effect.
The risk for human health of contamination with pharmaceutical compounds from fecal compost application in the tested production systems appears low, as indicated by the markedly low concentrations of CBZ in the edible parts of the plants. However, the effect of the pharmaceutical substances on the soil systems was not assessed in this study. The consequences of long-term application of fecal compost requires further investigation, particularly regarding the preservation of soil multi-functionality. Even if the crop production may not be affected, any impairment of soil biodiversity and habitat function should be determined. At the same time, it would of scientific interest to evaluate the total net effect of adding fecal compost, e.g., the benefit of added OM and the potential negative effect of the pharmaceutical compounds on the soil microbiome. By combined application of NUFs and fecal compost, the added OM could act as buffer for Na+ accumulation, and thereby sustain soil structure. Therefore, compost + NUF, a combination rarely tested in fields studies, exhibits several benefits that warrant further investigation.
Data availability statement
The original contributions presented in the study are included in the Supplementary Material, further inquiries can be directed to the corresponding author.
Author contributions
FH and AK designed the study and planned the experiments. The field experiment setup and harvesting were carried out by FH, AK, OM, and CS with help from research assistants and gardeners at the Leibniz Institute of Vegetable and Ornamental Crops (Großbeeren, Germany). FH and OM contributed to preparing the samples for analysis. FH, AK, OM, and ST contributed to the interpretation of the results. For the manuscript, FH took the lead in the Results and Discussion, and AK in the Introduction and Material and methods. OM, ST and CS participated in writing the manuscript. All authors provided critical feedback and helped shape the study, analyses and manuscript.
Funding
The study was conducted with the support of the European Union’s Horizon 2020 research and innovation programme under grant agreement No 774233, project Sino-European Innovative Green and Smart Cities (SiEUGreen).
Acknowledgments
The authors want to thank the team of gardeners at the Leibniz Institute of Vegetable and Ornamental Crops (Großbeeren, Germany) for the care they provided to the plants and their support during the experiments, and to the staff at the Leibniz Institute of Vegetable and Ornamental Crops who performed laboratory analyses and provided general support on a number of related matters. We also thank Martin Krauss from Helmholtz Centre for Environmental Research (UFZ, Leipzig, Germany) and Thi Lam Huong Pham from Technische Universität Berlin (Germany) for their advice and support with the analysis of pharmaceutical compounds. Finally, we would like to acknowledge our (former) colleagues from IGZ, Eckhard George and Aladdin Halbert-Howard, who helped in many ways in the process of planning the fertilization strategy, respectively, performing the experiment, applying fertilizers, screening the experiment, collecting data or cutting samples.
Conflict of interest
The authors declare that the research was conducted in the absence of any commercial or financial relationships that could be construed as a potential conflict of interest.
Publisher’s note
All claims expressed in this article are solely those of the authors and do not necessarily represent those of their affiliated organizations, or those of the publisher, the editors and the reviewers. Any product that may be evaluated in this article, or claim that may be made by its manufacturer, is not guaranteed or endorsed by the publisher.
Supplementary material
The Supplementary Material for this article can be found online at: https://www.frontiersin.org/articles/10.3389/fenvs.2022.1038175/full#supplementary-material
References
Andersson, E. (2015). Turning waste into value: Using human urine to enrich soils for sustainable food production in Uganda. J. Clean. Prod. 96, 290–298. doi:10.1016/j.jclepro.2014.01.070
Andreev, N., Ronteltap, M., Lens, P. N., Boincean, B., Bulat, L., and Zubcov, E. (2016). Lacto-fermented mix of faeces and bio-waste supplemented by biochar improves the growth and yield of corn (Zea mays L.). Agric. Ecosyst. Environ. 232, 263–272. doi:10.1016/j.agee.2016.08.012
AOAC International (2012). AOAC official method 2007.01: Pesticide residues in foods by acetonitrile - extraction and partitioning with magnesium sulfate gas chromatography/mass spectrometry and liquid chromatography/tandem mass spectrometry. 19th Ed. Gaithersburg, MD, USA: AOAC INTERNATIONAL.
Arnold, U., and Schmidt, J. (2012). ‘Research Project Sanitary Recycling Eschborn (SANIRESCH) Project component: Agricultural Production/Legal Situation’. Eschborn, Deutschland: GIZ - deutsche Gesellschaft für Internationale Zusammenarbeit. Available at: https://www.susana.org/_resources/documents/default/2-1628-fs-agricultural.pdf.
Baijukya, F. P., de Ridder, N., and Giller, K. E. (2006). Nitrogen release from decomposing residues of leguminous cover crops and their effect on maize yield on depleted soils of bukoba district, Tanzania. Plant Soil 279 (1), 77–93. doi:10.1007/s11104-005-2504-0
Barkusky, D. (2009). in Dauerfeldversuche in brandenburg und Berlin beiträge für eine nachhaltige landwirtschaftliche bodennutzung. Editors M. Brandenburg (U. Zu. Potsdam).
Bisinella de Faria, A. B., Sperandio, M., Ahmadi, A., and Tiruta-Barna, L. (2015). Evaluation of new alternatives in wastewater treatment plants based on dynamic modelling and life cycle assessment (DM-LCA). Water Res. 84, 99–111. doi:10.1016/j.watres.2015.06.048
Bornemann, G., Waßer, K., and Hauslage, J. (2018). The influence of nitrogen concentration and precipitation on fertilizer production from urine using a trickling filter. Life Sci. Space Res. 18, 12–20. doi:10.1016/j.lssr.2018.04.003
Bradford-Hartke, Z., Lane, J., Lant, P., and Leslie, G. (2015). Environmental benefits and burdens of phosphorus recovery from municipal wastewater. Environ. Sci. Technol. 49 (14), 8611–8622. doi:10.1021/es505102v
Bundesrepublik Deutschland, I. (2019). Verordnung über das Inverkehrbringen von Düngemitteln, Bodenhilfsstoffen, Kultursubstraten und Pflanzenhilfsmittels. Bundesanzeiger Verlag GmbH, 1–118. Available at: https://www.gesetze-im-internet.de/bundesrecht/d_mv_2012/gesamt.pdf.
Bundesrepublik Deutschland, I. (2015). “Verordnung über die Verwertung von Bioabfällen auf landwirtschaftlich, forstwirtschaftlich und gärtnerisch genutzten Böden (Bioabfallverordnung - BioAbfV),” in German biowaste ordinance’, international biochar initiative (IBI (Bundesanzeiger Verlag GmbH), 1–58. Available at: https://www.gesetze-im-internet.de/bioabfv/__10.html.
Butkovskyi, A., Ni, G., Hernandez Leal, L., Rijnaarts, H., and Zeeman, G. (2016). Mitigation of micropollutants for black water application in agriculture via composting of anaerobic sludge. J. Hazard. Mater. 303, 41–47. doi:10.1016/J.JHAZMAT.2015.10.016
Camotti Bastos, M., Soubrand, M., Le Guet, T., Le Floch, E., Joussein, E., Baudu, M., et al. (2020). Occurrence, fate and environmental risk assessment of pharmaceutical compounds in soils amended with organic wastes. Geoderma 375, 114498. doi:10.1016/J.GEODERMA.2020.114498
Carrilho, E. N. V. M., Labuto, G., and Kamogawa, M. Y. (2016). Destination of vinasse, a residue from alcohol industry: Resource recovery and prevention of pollution. Environmental Materials and Waste: Resource Recovery and Pollution Prevention, 21–43. doi:10.1016/B978-0-12-803837-6.00002-0
Carter, L. J., Harris, E., Williams, M., Ryan, J. J., Kookana, R. S., and Boxall, A. B. A. (2014). Fate and uptake of pharmaceuticals in soil–plant systems. J. Agric. Food Chem. 62 (4), 816–825. doi:10.1021/jf404282y
Chefetz, B., Mualem, T., and Ben-Ari, J. (2008). Sorption and mobility of pharmaceutical compounds in soil irrigated with reclaimed wastewater. Chemosphere 73 (8), 1335–1343. doi:10.1016/J.CHEMOSPHERE.2008.06.070
Chowdhury, R. B., Moore, G. A., Weatherley, A. J., and Arora, M. (2014). A review of recent substance flow analyses of phosphorus to identify priority management areas at different geographical scales. Resour. Conservation Recycl. 83, 213–228. doi:10.1016/j.resconrec.2013.10.014
Christou, A., Papadavid, G., Dalias, P., Fotopoulos, V., Michael, C., Bayona, J. M., et al. (2019). Ranking of crop plants according to their potential to uptake and accumulate contaminants of emerging concern. Environ. Res. 170, 422–432. doi:10.1016/j.envres.2018.12.048
Dalahmeh, S. S., Thorsén, G., and Jönsson, H. (2022). Open-air storage with and without composting as post-treatment methods to degrade pharmaceutical residues in anaerobically digested and dewatered sewage sludge. Sci. Total Environ. 806, 151271. doi:10.1016/J.SCITOTENV.2021.151271
Davidson, E. A., Hart, S. C., and Firestone, M. K. (1992). Internal cycling of nitrate in soils of a mature Coniferous forest. Ecology 73, 1148–1156. doi:10.2307/1940665
Desmidt, E., Ghyselbrecht, K., Zhang, Y., Pinoy, L., Van der Bruggen, B., Verstraete, W., et al. (2015). Global phosphorus scarcity and full-scale P-recovery techniques: A review. Crit. Rev. Environ. Sci. Technol. 45 (4), 336–384. doi:10.1080/10643389.2013.866531
Deutsches Institut für Normung, D. (2020). DIN (2020) DIN SPEC 91421:2020-12, Quality assurance of recycling products from dry toilets for use in horticulture. 50.
Dixon, P. M., Moore, K. J., and van Santen, E. (2018). “The analysis of combined experiments,” in Applied statistics in agricultural, biological, and environmental Sciences (ASA, CSSA, and SSSA Books), 201–234. doi:10.2134/appliedstatistics.2016.0004.c8
DüV (2017). ‘Verordnung über die Anwendung von Düngemitteln, Bodenhilfsstoffen, Kultursubstraten und Pflanzenhilfsmitteln nach den Grundsätzen der guten fachlichen Praxis beim Düngen (Düngeverordnung - DüV)’, BGBl, I.
Eawag Engineering, D. P. (2015). VUNA project – nutrient recovery from urine. Available at: https://www.eawag.ch/en/department/eng/projects/vuna.
Eberhardt, Y. (2021). Analysis of antibiotics in sewage sludge and compost using LC-MS/MS. Master’s t. University of Applied Sciences and Arts Northwestern Switzerland FHNW.
El hasini, S., De Nobili, M., El. Azzouzi, M., Azim, K., Douaik, A., Laghrour, M., et al. (2020). The influence of compost humic acid quality and its ability to alleviate soil salinity stress. Int. J. Recycl. Org. waste Agric. 9 (1), 21–31. doi:10.30486/ijrowa.2020.671213
Esculier, F., Le Noe, J., Barles, S., Billen, G., Creno, B., Garnier, J., et al. (2019). The biogeochemical imprint of human metabolism in paris megacity: A regionalized analysis of a water-agro-food system. J. Hydrology 573, 1028–1045. doi:10.1016/j.jhydrol.2018.02.043
European Commission (2020). Changing how we produce and consume: New Circular Economy Action Plan shows the way to a climate-neutral, competitive economy of empowered consumers. Press release. Available at: https://ec.europa.eu/commission/presscorner/detail/en/ip_20_420.
Everaarts, A. P., and Booi, R. (2000). The effect of nitrogen application on nitrogen utilization by white cabbage (Brassica oleracea var. capitata) and on nitrogen in the soil at harvest. J. Hortic. Sci. Biotechnol. 75 (6), 705–712. doi:10.1080/14620316.2000.11511311
Everaarts, A. P., and De Moel, C. P. (1998). The effect of nitrogen and the method of application on yield and quality of white cabbage. Eur. J. Agron. 9 (2), 203–211. doi:10.1016/S1161-0301(98)00038-0
Feachem, R. G., Bradley, D. J., and Mara, D. M. (1983). Sanitation and disease - health aspects of excreta and wastewater management. in World Bank studies in water supply and sanitation, 3. Saniitiatiion and Disease
Feller, C., Fink, M., Laber, H., Maync, A., Paschold, P., Scharpf, H. C., et al. (2011). Düngung im Freilandgemüsebau. Schriftenr. Leibniz-Instituts für Gemüse- Zierpflanzenbau (IGZ) 3 (4), 1–265.
Feller, C., Fink, M., and Sradnick, A. (2017). Nährstoffgehalte und N-Mineralisierung organischer Düngemittel. Version 03.05.2017Available at: https://www.igzev.de/publikationen/IGZ_Organische_Duenger_Naehrstoffgehalte_N-Mineralisierung.pdf.
Feng, D.-L., and Wu, Z.-C. (2006). Culture of Spirulina platensis in human urine for biomass production and O(2) evolutionScience. J. Zhejiang Univ. 7, 34–37. doi:10.1631/jzus.2006.B0034
Fricke, K. (2009). Energieeffizienz kommunaler kläranlagen. Umweltbundesamt, Dessau, 10. Available at: www.umweltbundesamt.de.
Fumasoli, A., Etter, B., Sterkele, B., Morgenroth, E., and Udert, K. M. (2016). Operating a pilot-scale nitrification/distillation plant for complete nutrient recovery from urine. Water Sci. Technol. 73 (1), 215–222. doi:10.2166/wst.2015.485
Gerten, D., Heck, V., Jagermeyr, J., Bodirsky, B. L., Fetzer, I., Jalava, M., et al. (2020). Feeding ten billion people is possible within four terrestrial planetary boundaries. Nat. Sustain. 3 (3), 200–208. doi:10.1038/s41893-019-0465-1
Goldstein, M., Shenker, M., and Chefetz, B. (2014). Insights into the uptake processes of wastewater-borne pharmaceuticals by vegetables. Environ. Sci. Technol. 48 (10), 5593–5600. doi:10.1021/es5008615
Gómez-Muñoz, B., Magid, J., and Jensen, L. S. (2017). Nitrogen turnover, crop use efficiency and soil fertility in a long-term field experiment amended with different qualities of urban and agricultural waste. Agric. Ecosyst. Environ. 240, 300–313. doi:10.1016/j.agee.2017.01.030
González-Prieto, S. J., Cabaneiro, A., Villar, M. C., Carballas, T., and Carballas, M. (1996). Effect of soil characteristics on N mineralization capacity in 112 native and agricultural soils from the northwest of Spain. Biol. Fert. Soils 22 (3), 252–260. doi:10.1007/BF00382521
Gutbrodt, B., Mody, K., and Dorn, S. (2011). Drought changes plant chemistry and causes contrasting responses in lepidopteran herbivores. Oikos 120 (11), 1732–1740. doi:10.1111/j.1600-0706.2011.19558.x
Harder, R., Wielemaker, R., Larsen, T. A., Zeeman, G., and Oberg, G. (2019). Recycling nutrients contained in human excreta to agriculture: Pathways, processes, and products. Crit. Rev. Environ. Sci. Technol. 49, 695–743. doi:10.1080/10643389.2018.1558889
Heinonen-Tanski, H., and Van Wijk-Sijbesma, C. (2005). Human excreta for plant production. Bioresour. Technol. 96 (4), 403–411. doi:10.1016/j.biortech.2003.10.036
Heinzelmann, A. (2020). Abbauverhalten pharmazeutischer Rückstände in thermophiler Kompostierung. Gießen, Germany: Justus-Liebig-Universität Gießen.
Herrmann, T., and Klaus, U. (1997). Fluxes of nutrients in urban drainage systems: Assessment of sources, pathways and treatment techniques. Water Sci. Technol. 36 (8–9), 167–172. doi:10.2166/wst.1997.0661
Hilton, S. P., Keoleian, G. A., Daigger, G. T., Zhou, B., and Love, N. G. (2021). Life cycle assessment of urine diversion and conversion to fertilizer products at the city scale. Environ. Sci. Technol. 55 (1), 593–603. doi:10.1021/acs.est.0c04195
Ishii, S. K. L., and Boyer, T. H. (2015). Life cycle comparison of centralized wastewater treatment and urine source separation with struvite precipitation: Focus on urine nutrient management. Water Res. 79, 88–103. doi:10.1016/J.WATRES.2015.04.010
Jönsson, H., Stintzing, A. R., Vinnerås, B., and Salomon, E. (2004). Guidelines on the use of urine and faeces in crop production. Publicatio. Stockholm, Sweden: EcoSanRes Programme, Stockholm Environment Institute.
Karami, A., Homaee, M., Afzalinia, S., Ruhipour, H., and Basirat, S. (2012). Organic resource management: Impacts on soil aggregate stability and other soil physico-chemical properties. Agric. Ecosyst. Environ. 148, 22–28. doi:10.1016/J.AGEE.2011.10.021
Keerthanan, S., Jayasinghe, C., Biswas, J. K., and Vithanage, M. (2021). Pharmaceutical and Personal Care Products (PPCPs) in the environment: Plant uptake, translocation, bioaccumulation, and human health risks. Crit. Rev. Environ. Sci. Technol. 51 (12), 1221–1258. doi:10.1080/10643389.2020.1753634
Kelova, M. E., Eich-Greatorex, S., and Krogstad, T. (2021). Human excreta as a resource in agriculture – evaluating the fertilizer potential of different composting and fermentation-derived products. Resour. Conservation Recycl. 175, 105748. doi:10.1016/J.RESCONREC.2021.105748
Kjerstadius, H., Bernstad Saraiva, A., Spangberg, J., and Davidsson, A. (2017). Carbon footprint of urban source separation for nutrient recovery. J. Environ. Manag. 197, 250–257. doi:10.1016/J.JENVMAN.2017.03.094
Köpping, I., McArdell, C. S., Borowska, E., Bohler, M. A., and Udert, K. M. (2020). Removal of pharmaceuticals from nitrified urine by adsorption on granular activated carbon. Water Res. X X9, 100057. doi:10.1016/j.wroa.2020.100057
Krause, A., Hafner, F., Augustin, F., and Udert, K. M. (2021). Qualitative risk analysis for contents of dry toilets used to produce novel recycling fertilizers. Circ. Econ. Sust. 1 (3), 1107–1146. doi:10.1007/s43615-021-00068-3
Krause, A., M, K., and J, K. (2015). Nutrient recycling from sanitation and energy systems to the agroecosystem- Ecological research on case studies in Karagwe, Tanzania. Afr. J. Agric. Res. 10 (43), 4039–4052. doi:10.5897/ajar2015.10102
Krause, A., Nehls, T., George, E., and Kaupenjohann, M. (2016). Organic wastes from bioenergy and ecological sanitation as a soil fertility improver: A field experiment in a tropical andosol. SOIL 2 (2), 147–162. doi:10.5194/soil-2-147-2016
Kumar, R., and Pal, P. (2015). Assessing the feasibility of N and P recovery by struvite precipitation from nutrient-rich wastewater: A review. Environ. Sci. Pollut. Res. 22 (22), 17453–17464. doi:10.1007/s11356-015-5450-2
Kuratorium für Technik und Bauwesen in der Landwirtschaft KTBL (2017). Gemüsebau: Freiland und Gewächshaus. Darmstadt, Germany: Datensammlung.
Larsen, T. A., Gruendl, H., and Binz, C. (2021). The potential contribution of urine source separation to the SDG agenda – A review of the progress so far and future development options. Environ. Sci. Water Res. Technol. 7 (7), 1161–1176. doi:10.1039/D0EW01064B
Larsen, T., Udert, K. K. M., and Lienert, J. (2013). Source separation and decentralization for wastewater management. London, United Kingdom: IWA Publishing.
Leal, R. M. P., Alleoni, L. R. F., Tornisielo, V. L., and Regitano, J. B. (2013). Sorption of fluoroquinolones and sulfonamides in 13 Brazilian soils. Chemosphere 92 (8), 979–985. doi:10.1016/J.CHEMOSPHERE.2013.03.018
Lehotay, S. J., Neil, M. 0., Tully, J., Valverde, A., Contreras, M., Mol, H. G. J., et al. (2007). Determination of pesticide residues in foods by acetonitrile extraction and partitioning with magnesium sulfate: Collaborative Study, J. Aoac Inter., 90 (2), 485–520. doi:10.1093/jaoac/90.2.485
Londong, J. (2016). “New alternative sanitation systems - NASS. Terminology, material flows, treatment of partial flows, utilisation,” in Weiterbildendes Studium Wasser und Umwelt. German Association for Water Wastewater and Waster. Weimar, Germany: DWA. Available at: 978-3-95773-213-2.
Machate, O., Dellen, J., Schulze, T., Wentzky, V. C., Krauss, M., and Brack, W. (2021). Evidence for antifouling biocides as one of the limiting factors for the recovery of macrophyte communities in lakes of Schleswig-Holstein. Environ. Sci. Eur. 33 (1), 57. doi:10.1186/s12302-021-00500-3
Malchi, T., Maor, Y., Tadmor, G., Shenker, M., and Chefetz, B. (2014). Irrigation of root vegetables with treated wastewater: Evaluating uptake of pharmaceuticals and the associated human health risks. Environ. Sci. Technol. 48 (16), 9325–9333. doi:10.1021/es5017894
Martin, T. M. P., Esculier, F., Levavasseur, F., and Houot, S. (2020). Human urine-based fertilizers: A review. Crit. Rev. Environ. Sci. Technol. 52, 890–936. doi:10.1080/10643389.2020.1838214
McCartney, S. N., Watanabe, N. S., and Yip, N. Y. (2021). Emerging investigator series: Thermodynamic and energy analysis of nitrogen and phosphorous recovery from wastewaters. Environ. Sci. Water Res. Technol. 7 (11), 2075–2088. doi:10.1039/D1EW00554E
Mehta, C. M., Khunjar, W. O., Nguyen, V., Tait, S., and Batstone, D. J. (2015). Technologies to recover nutrients from waste streams: A critical review. Crit. Rev. Environ. Sci. Technol. 45 (4), 385–427. doi:10.1080/10643389.2013.866621
Meyer, G., Frossard, E., Mader, P., Nanzer, S., Randall, D. G., Udert, K. M., et al. (2018). Water soluble phosphate fertilizers for crops grown in calcareous soils – An outdated paradigm for recycled phosphorus fertilizers? Plant Soil 424 (1–2), 367–388. doi:10.1007/s11104-017-3545-x
Mihelcic, J. R., Fry, L. M., and Shaw, R. (2011). Global potential of phosphorus recovery from human urine and feces. Chemosphere 84 (6), 832–839. doi:10.1016/j.chemosphere.2011.02.046
Moore, K. J., and Dixon, P. M. (2015). Analysis of combined experiments revisited. Agron. J. 107 (2), 763–771. doi:10.2134/agronj13.0485
Morgan, P. (2007). Toilets that Make Compost: Low-cost, sanitary toilets that produce valuable compost for crops in an African context. Stockholm, Sweden: EcoSanRes Programme.
Nanusha, M. Y., Krauss, M., Schonsee, C. D., Gunthardt, B. F., Bucheli, T. D., and Brack, W. (2020). Target screening of plant secondary metabolites in river waters by liquid chromatography coupled to high-resolution mass spectrometry (LC–HRMS). Environ. Sci. Eur. 32 (1), 142. doi:10.1186/s12302-020-00399-2
Niwagaba, C., Nalubega, M., Vinneras, B., Sundberg, C., and Jonsson, H. (2009). Bench-scale composting of source-separated human faeces for sanitation. Waste Manag. 29 (2), 585–589. doi:10.1016/j.wasman.2008.06.022
Ogwang, F., Tenywa, J. S., Otabbong, E., Tumuhairwe, J. B., and Amoding-Katusabe, A. (2012). Faecal blending for nutrient enrichment and speedy sanitisation for soil fertility improvement. Kampala, Uganda: ISRN Soil Science, 1–7. doi:10.5402/2012/424171
Özel Duygan, B. D., Udert, K. M., Remmele, A., and McArdell, C. S. (2021). Removal of pharmaceuticals from human urine during storage, aerobic biological treatment, and activated carbon adsorption to produce a safe fertilizer. Resour. Conservation Recycl. 166, 105341. doi:10.1016/j.resconrec.2020.105341
Pan, M., and Chu, L. M. (2017). Fate of antibiotics in soil and their uptake by edible crops. Sci. Total Environ. 599–600, 500–512. doi:10.1016/j.scitotenv.2017.04.214
Pradhan, S. K., Nerg, A. M., Sjoblom, A., Holopainen, J. K., and Heinonen-Tanski, H. (2007). Use of human urine fertilizer in cultivation of cabbage (Brassica oleracea)––Impacts on chemical, microbial, and flavor quality. J. Agric. Food Chem. 55 (21), 8657–8663. doi:10.1021/jf0717891
Remy, C., and Jekel, M. (2008). Sustainable wastewater management: Life cycle assessment of conventional and source-separating urban sanitation systems. Water Sci. Technol. 58 (8), 1555–1562. doi:10.2166/wst.2008.533
Rockström, J., Steffen, W., Noone, K., Persson, A., Chapin, F. S., Lambin, E. F., et al. (2009). A safe operating space for humanity. Nature 461 (7263), 472–475. doi:10.1038/461472a
Ros, G. H., Hanegraaf, M. C., Hoffland, E., and van Riemsdijk, W. H. (2011). Predicting soil N mineralization: Relevance of organic matter fractions and soil properties. Soil Biol. Biochem. 43 (8), 1714–1722. doi:10.1016/J.SOILBIO.2011.04.017
Ruehlmann, J. (2013). The box plot experiment in grossbeeren after eight rotations: Nitrogen, carbon and energy balances. Archives Agron. Soil Sci. 59 (8), 1159–1176. doi:10.1080/03650340.2012.711473
Rühlmann, J., and Ruppel, S. (2005). Effects of organic amendments on soil carbon content and microbial biomass – results of the long-term box plot experiment in Grossbeeren. Archives Agron. Soil Sci. 51 (2), 163–170. doi:10.1080/03650340400026651
Sangare, D., Sou, M., Hijikata, N., Lahmar, R., Yacouba, H., Coulibaly, L., et al. (2015). Toilet compost and human urine used in agriculture: Fertilizer value assessment and effect on cultivated soil properties. Environ. Technol. 36 (9–12), 1291–1298. doi:10.1080/09593330.2014.984774
Schröder, C., Häfner, F., Larsen, O. C., and Krause, A. (2021). Urban organic waste for urban farming: Growing lettuce using vermicompost and thermophilic compost. Agronomy. doi:10.3390/agronomy11061175
Shepherd, M. A., Harrison, R., and Webb, J. (2002). Managing soil organic matter – implications for soil structure on organic farms. Soil Use Manag. 18 (1), 284–292. doi:10.1111/j.1475-2743.2002.tb00270.x
Silva, A. L. L. D., and Gollo, L. (2014). Development of a vinasse culture medium for plant tissue culture. Pak. J. Bot. 46 (6), 2195
Simha, P., and Ganesapillai, M. (2017). Ecological sanitation and nutrient recovery from human urine: How far have we come? A review. Sustain. Environ. Res. 27 (3), 107–116. doi:10.1016/j.serj.2016.12.001
Thorup-Kristensen, K., and Sørensen, J. N. (1999). Soil nitrogen depletion by vegetable crops with variable root growth. Acta Agric. Scand. Sect. B - Soil & Plant Sci. 49 (2), 92–97. doi:10.1080/09064719950135597
Tilley, E., Ulrich, L., Luethi, C., Reymond, P., Zurburegg, C., Lüthi, C., et al. (2014). Compendium of sanitation systems and technologies. Dübendorf, Switzerland: Development.
Tuholske, C., Halpern, B. S., Blasco, G., Villasenor, J. C., Frazier, M., and Caylor, K. (2021). Mapping global inputs and impacts from of human sewage in coastal ecosystems. PLOS ONE 16 (11), e0258898. doi:10.1371/journal.pone.0258898
Udert, K. M., and Wächter, M. (2012). Complete nutrient recovery from source-separated urine by nitrification and distillation. Water Res. 46 (2), 453–464. doi:10.1016/j.watres.2011.11.020
Umweltbundesamt UBA (2020). Einträge von Nähr- und Schadstoffen in die Oberflächengewässer. Available at: https://www.umweltbundesamt.de/daten/wasser/fliessgewaesser/eintraege-von-naehr-schadstoffen-in-die#nahrstoffeintrage-sinken-wieder-langsam.
Vasilaki, V., Massara, T., Stanchev, P., Fatone, F., and Katsou, E. (2019). A decade of nitrous oxide (N(2)O) monitoring in full-scale wastewater treatment processes: A critical review. Water Res. 161, 392–412. doi:10.1016/j.watres.2019.04.022
Verband deutscher landwirtschaftlicher Untersuchungs- und Forschungsanstalten (Vdlufa), I. (2012). Methodenbuch Band I Die Untersuchung von Böden, chapter A 6.2.1.1 Bestimmung von Phosphor und Kalium im Calcium-Acetat-Lactat-Auszug, 6. Teillfg. ’ Darmstadt, Germany: VDLUFA-Verlag.
Vinnerås, B. (2007). Comparison of composting, storage and urea treatment for sanitising of faecal matter and manure. Bioresour. Technol. 98 (17), 3317–3321. doi:10.1016/j.biortech.2006.07.011
Viskari, E. L., Grobler, G., Karimaki, K., Gorbatova, A., Vilpas, R., and Lehtoranta, S. (2018). Nitrogen recovery with source separation of human urine—preliminary results of its fertiliser potential and use in agriculture. Front. Sustain. Food Syst. 2. doi:10.3389/fsufs.2018.00032
VunaNexus (2021). Urine recycling Technology. Available at: https://www.vunanexus.com/urin-recycling-technologie.
Wang, M., Khan, M. A., Mohsin, I., Wicks, J., Ip, A. H., Sumon, K. Z., et al. (2021). Can sustainable ammonia synthesis pathways compete with fossil-fuel based Haber–Bosch processes? Energy Environ. Sci. 14 (5), 2535–2548. doi:10.1039/D0EE03808C
Winker, M., Clemens, J., Reich, M., Gulyas, H., and Otterpohl, R. (2010). Ryegrass uptake of carbamazepine and ibuprofen applied by urine fertilization. Sci. Total Environ. 408 (8), 1902–1908. doi:10.1016/j.scitotenv.2010.01.028
Winker, M., Vinneras, B., Muskolus, A., Arnold, U., and Clemens, J. (2009). Fertiliser products from new sanitation systems: Their potential values and risks. Bioresour. Technol. 100 (18), 4090–4096. doi:10.1016/j.biortech.2009.03.024
Yara (2022). Nitrogen deficiency in cabbages. (chlorotic),additionally on the leaf blades Available at: httpds://www.yara.co.uk/crop-nutrition/cabbage/nutrient-deficiencies-cabbage/nitrogen-deficiency-cabbage/#:∼:text=Cabbage - Chlorosis&text=Symptoms-,With nitrogen deficiency the foliage becomes yellowish-green.
Keywords: dry toilets, resource-oriented sanitation, circular economy, nitrified urine, fecal compost, field experiment, horticulture, open field cabbage cultivation
Citation: Häfner F, Monzon Diaz OR, Tietjen S, Schröder C and Krause A (2023) Recycling fertilizers from human excreta exhibit high nitrogen fertilizer value and result in low uptake of pharmaceutical compounds. Front. Environ. Sci. 10:1038175. doi: 10.3389/fenvs.2022.1038175
Received: 06 September 2022; Accepted: 07 December 2022;
Published: 16 January 2023.
Edited by:
Björn Vinnerås, Swedish University of Agricultural Sciences, SwedenReviewed by:
Susanne Eich-Greatorex, Norwegian University of Life Sciences, NorwayMaría Belén Almendro-Candel, Miguel Hernández University of Elche, Spain
Copyright © 2023 Häfner, Monzon Diaz, Tietjen, Schröder and Krause. This is an open-access article distributed under the terms of the Creative Commons Attribution License (CC BY). The use, distribution or reproduction in other forums is permitted, provided the original author(s) and the copyright owner(s) are credited and that the original publication in this journal is cited, in accordance with accepted academic practice. No use, distribution or reproduction is permitted which does not comply with these terms.
*Correspondence: Ariane Krause, krause@igzev.de
†These authors have contributed equally to this work