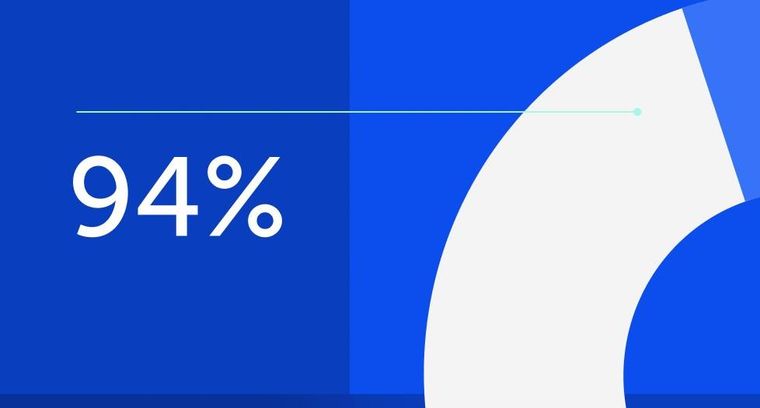
94% of researchers rate our articles as excellent or good
Learn more about the work of our research integrity team to safeguard the quality of each article we publish.
Find out more
ORIGINAL RESEARCH article
Front. Environ. Sci., 02 November 2022
Sec. Soil Processes
Volume 10 - 2022 | https://doi.org/10.3389/fenvs.2022.1036837
Soil salinization, an important type of soil degradation, has become a problem restricting crop production and food quality. The remediation technologies by using compost and biochar were considered sustainable and environment friendly, but the sole application of compost or biochar hardly gets the satisfactory remediation effects. Until now, information about the effects of cocomposted biochar on soils is limited, especially in the coastal soil. This study investigated the impact and potential underlying mechanism of corn straw biochar (BC), seaweed compost (SC), and cocomposted BC and SC (BCSC) on the growth and yield of sorghum (Sorghum bicolor (L.) Moench) in the coastal soil of China in a pot experiment. BC and BCSC treatments increased the dry biomass and yield of the sorghum by 44.0–52.4% and 132.9–192.3%, respectively. Similarly, the root morphologies of sorghum, including surface area and average diameter, were also increased with BC and BCSC addition. Meanwhile, BCSC treatment showed a better performance than what the others did. The enhanced growth and yield of sorghum primarily resulted from the improvement of soil properties (WHC, SOM, and EC) and nutrient availability (Olsen-P and AK content). In addition, the increased diversity and shifted composition of soil bacteria with BC and BCSC addition might also account for the increased growth and yield of sorghum. Furthermore, the enhanced relative abundances of beneficial bacteria Vicinamibacteraceae (39.0%) and Sphingomonadaceae (41.5%) in the rhizosphere soil were positively correlated with the content of available nutrients (NH4+, Olsen-P, and available K) in the coastal soil, which might reveal the mechanism of enhancing growth under the established collaborative interactions of them. Our study provides the potential of using biochar-compost to ameliorate the degradation of coastal soils and improve crop yield.
The Yellow River Delta is one of the most vulnerable and important ecosystems in China (You et al., 2021). Due to strong alkalinity, high salt concentration, and lack of nutrients, the soil often has a negative effect on the growth of plants in this area (Liu et al., 2019a; Liang et al., 2021). Moreover, salinity restricts soil microbial growth and biochemical function (Lu et al., 2015). Consequently, soil remediation is of great importance for maintaining crop yield and food quality in this area (Cai et al., 2021). There are many reported technologies for saline–alkali soil amendment, such as water leaching (Li, 2018), chemical remediation (Wang et al., 2014), and phytoremediation (Hamideh et al., 2017). However, practical applications of these methods are limited due to low efficiency, high costs, or secondary pollution (Zhou et al., 2021). Therefore, a more efficient and environment-friendly method is needed for increasing plant growth, enhancing soil properties, and decreasing salt stress in the coastal saline soil.
Biochar is a polyporus carbon-rich material prepared from various organic waste feedstock under certain thermal combustion with limited oxygen (Yang et al., 2021a). Due to the large porosity, high adsorption capacity, abundant surface functional groups, and rich carbon content, biochar could bring multiple benefits to agricultural sustainability such as enhancement of soil nutrient availability and water holding capacity and improvement of soil structure (Verheijen et al., 2019; Suo et al., 2021). Recent studies had reported a great potential of biochar in improving composting performance, accelerating the humification of feedstock, enhancing soil microbial diversity and activity, mitigating the emission of greenhouse gas (GHG), and removing soil contaminants (Guo et al., 2020; Antonangelo et al., 2021). In addition, a previous study had shown that biochar application at 5–20 t ha−1 increased the NUE and grain yield of wheat by 5.2–37.9% and 2.9–19.4%, respectively, ascribing to the positive role of bacterial interactions in the rhizosphere bacterial community (Sun et al., 2019). Sun et al. (2016) found that the yield of Suaeda salsa increased by 11.7–115.0% with wheat straw biochar addition at the rate of 5–10 g kg−1 due to the significantly reduced soil pH, enhanced total organic matter, and available nutrients in the saline soil. However, a previous study reported little or even negative effects of biochar addition on plant growth and yield (Huang and Gu, 2019). These inconsistent results were mainly due to the differences in species of plant, characteristics of soil and biochar, and the application rate of biochar (Zhang et al., 2020). Moreover, the individual application of biochar might not get satisfactory outcomes in saline soil; thus, the combined application of biochar and other additives was encouraged.
Composting is one of the most efficient technologies to improve soil properties and enhance crop yield because of the disposal of organic wastes and the production of compost that is suitable to be a fertilizer or soil amendment (Qayyum et al., 2017; Kumar et al., 2018; Zahra et al., 2021a). Mostafa et al. (2016) found that compost application at 20 t ha−1 could be an alternative to 50% of the recommended dose of mineral-NPK fertilizers, which improved the soil characteristics and increased the pod and seed of Phaseolus vulgaris. Rehman et al. (2016) reported that the shoot dry weight of hybrid maize increased by 8.7% with the compost addition compared to the control treatment. There were also studies which reported that biochar-compost might be more suitable for soil remediation to enhance plant growth and soil properties (Agegnehu et al., 2017; Teodoro et al., 2020). The interaction between the biochar and compost, which might alter the properties of each other, eventually influenced the remediation efficiency in soils (Tang et al., 2020). Pandit et al. (2020) found that the application of the mixture of Eupatorium adenophorum biochar-compost at the rate of 60 t ha −1 had significantly increased the growth of maize by 12.7% compared to the individual biochar application. However, a study by Schmidt et al. (2014) showed that biochar and biochar-compost treatments had mostly nonsignificant effects on the vine and green cover growth and grape quality over 3 years compared to unamended control soil. The influence of biochar-compost might correlate with the soil types and plant species. So far, few studies were focused on the influence of biochar-compost on crop growth and yield in coastal soils. A recent study had shown that corn straw biochar-compost application at 1.5% could increase the total biomass of seashore mallow and sesbania by 70.8 and 309.0%, respectively, due to the increase of soil properties and the decrease of exchangeable sodium (Luo et al., 2017). In addition, Liang et al. (2021) found that biochar-compost application at 1.5% significantly increased the biomass of Phragmites australis by 35.5%. Based on the abovementioned results, Zahra et al. (2021b, c)believed that biochar-compost should have a great potential in the mitigation of coastal soil degradation and the improvement of crop productivity. However, until now, most of the studies only focused on evaluating the effects of single or different biochars on the coastal soil amendment, and information on the effects of compost of different materials on the characteristics of microbial interactions in the coastal saline soil was limited. In addition, few attempts have been made to investigate the mechanism of the effect of biochar-compost addition on plant growth. Furthermore, the effects of biochar-compost on the amendment of coastal soils, compared with different biochar types, still remain poorly evaluated. Therefore, in this study, we hypothesized that the selected biochar could amend the saline soil to different degrees by altering the soil microenvironments. The purposes of this study were to 1) evaluate the effects of corn straw biochar (BC), seaweed compost (SC), and cocomposted BC and seaweed (BCSC) on the growth and yield of sorghum in the coastal saline soil; 2) investigate the effects of BC, SC, and BCSC on the nutrients and physicochemical properties of saline soil; and 3) explore the responses of soil bacteria to different types of biochar addition in the saline soil.
The surface saline soil (0–20 cm) samples were collected from Dongying city, Shandong Province, China (37.26°N, 118.69°E). After air-drying, the saline soil samples were mixed uniformly and screened through a 2 mm sieve. Data of soil organic matter (SOM), pH, electrical conductivity (EC), cation exchange capacity (CEC), NO3−-N, NH4+-N, Olsen-P, and available K (AK) are shown in Table 1. The determination methods are presented in the supporting information (SI).
The selected biochar, which was prepared from corn straw at 400–450°C for 2 h, was prepared by Liaoning Golden Future Agriculture Technology Co., Ltd. (Anshan, Liaoning Province, China). BC was screened through a 2 mm sieve before utilization. Composting was conducted in a rotary drum composter with the cylindrical shape according to Huang et al. (2021). Organic material for the compost comprised 35% seaweeds, 35% wheat straw, and 30% cow manure to ensure the C/N ratio of the mixture between 25 and 30. Prior to the composting process, seaweeds were crushed into small pieces with a length less than 1 cm, mixed thoroughly with wheat straw at the ratio of 1:1 based on dry weight, and then, mixed with cow manure. Thereafter, BC was added into the mixture and mixed thoroughly at the ratio of 0 and 5% (w/w) based on dry weight. The composts with 0 and 5% BC were labeled as SC and BCSC, respectively. The moisture content was maintained at 60–70% by adding water throughout the composting. The compost samples were collected after being fermented for 80 days and then screened through a 2 mm sieve after air-drying. The properties of BC and BCSC are shown in Table 1. The determination methods are presented in SI.
A flowchart of the research methodology is shown in Figure 1. The prepared BC, SC, and BCSC were incorporated into the selected soil at a rate of 1.0% (w/w), hereafter referred to as BC, SC, and BCSC, respectively. The treatment without amendment was labeled as CK. Each plastic pot (29 × 25 cm; diameter × depth) was filled with 8 kg soil with or without amendment. A four-leaf stage sorghum seedling was transplanted into each pot. Each treatment contained three replicates, and thus, there were 12 pots in total. During the growth period, all pots were moistened to 60% maximum water holding capacity (WHC), which was balanced with tap water. The pots were maintained in a greenhouse under 16 h of supplementary light per day and a daytime temperature in a range of 20–30°C during the whole growing period. The pots were rotated every week until harvest to ensure the homogeneity of the treatments. The soil and sorghum samples were collected for further analysis after 90 days of growth. The soil samples were divided into rhizosphere (Rh) and non-rhizosphere (NRh) soils following the hand shaking method (Wang et al., 2017). The sorghums were divided into shoot, root, and panicle. Epson Scanning (Expression 11000XL, REGENT INSTRUMENTS) and Win Rhizo Pro.2005 were used to scan and analyze the morphological parameters of sorghum roots (Luo et al., 2017).
After deactivating the enzymes at 105°C for 30 min and then drying at 65°C for 24 h, the sorghum samples were weighed and the grains were counted. The soils from Rh and NRh were divided into two parts, respectively; one part was stored at −80°C for bacterial analysis, and another part was characterized after being air-dried. The soil properties including soil pH, EC, NH4+-N, NO3−-N, Olsen-P, AK, SOM, and CEC were determined according to the studies reported previously (Wu et al., 2017; Zheng et al., 2018; Hallam and Hodson, 2020). More details about soil pH, EC, NH4+-N, NO3−-N, Olsen-P, AK, SOM, and CEC are presented in the SI.
Total microbial genomic DNA were extracted and purified by using E.Z.N.A.® soil DNA kits (Omega Bio-tek, Norcross, GA, United States) according to the manufacturer’s instructions. Sub-soil samples were collected from each treatment in triplicates. The bacterial V3–V4 hypervariable region of 16S rRNA was amplified using primers 338F and 806R by an ABI Gene Amp® 9700 PCR thermocycler (ABI, CA, United States). The PCR amplification of the 16S rRNA gene was performed using the following cycle: initial denaturation at 95°C/3 min, followed by 27 cycles of denaturing at 95°C/30 s, 55°C/30 s, and 72°C/45 s, a final extension at 72°C/10 min, and end at 4°C. After purification and being pooled at equal concentrations, the PCR products were paired-end sequenced on an Illumina MiSeq PE300 platform/NovaSeq PE250 platform (Illumina, San Diego, United States). The sequences were grouped into operational taxonomic units (OTUs; 97% similarity cut-off) and clustered using UPARSE (version 7.1). A representative sequence from each OTU was picked and aligned by RDP Classifier version 2.2 against the 16S rRNA database (e.g., Silva v138) using a confidence threshold of 0.7.
The figures and tables were processed using Origin Pro 9.0 (Origin Lab Corp.) and Microsoft Office Excel 2010, respectively. One-way analysis of variance (ANOVA) was used to analyze the difference of the abovementioned parameters between different treatments with SPSS 20.0. An independent-sample t-test (p < 0.05) was used to explore and compare the significant differences between the Rh and NRh soil with SPSS 20.0.
The effects of BC, SC, and BCSC on the growth and yield of sorghum are presented in Figure 2. The total fresh biomass and dry biomass of sorghum plant were significantly increased by BC (21.6–30.3%) and BCSC (44.0–53.1%), respectively. However, SC decreased the total fresh biomass and dry biomass of sorghum by 21.2 and 35.6%, respectively (Figures 2A,B). The plant height of sorghum also showed an increasing trend by BC and BCSC treatments, despite the nonsignificant increase in BCSC treatment (Figure 2C). In addition, the leaf numbers of sorghum were not significantly affected by the addition of amendments. Compared with CK treatment, BC and BCSC treatments increased the grain number by 224.6 and 390.4%, respectively (Figure 2D). However, SC addition had no significant effect on it. The grain fresh biomass significantly increased by 201.1, 57.3, and 256.7%, respectively, after the BC, SC, and BCSC amendment, among which the BCSC treatment induced the biggest increase (Supplementary Figure S1A). Consistently, BC and BCSC treatments increased the dry biomass of grain significantly by 132.9 and 196.4%, respectively (Figure 2D). Compared to CK treatment, BC and SC treatments decreased the root–shoot ratio by 28.3 and 23.3%, respectively. BCSC treatment increased the root–shoot ratio by 30.8%. However, the effect was not significant (Supplementary Figure S1B).
FIGURE 2. Effect of biochar, compost, and biochar-compost addition on sorghum growth and yield in coastal soil. (A) Sorghum fresh biomass, (B) sorghum dry biomass; (C) sorghum height, and (D) sorghum grain dry biomass and grain number. CK: soil without amendment; BC: soil amended with corn straw biochar at 1.0% (w/w); SC: soil amended with seaweed compost at 1.0% (w/w); and BCSC: soil amended with cocomposted biochar and seaweed at 1.0% (w/w). The error bars represent the standard error of the mean (n = 3). Different small letters indicate the significant difference among the different treatments (Duncan’s multiple-comparison test, n = 3, p < 0.05).
Table 2 shows the effects of biochar-compost additions on sorghum root morphology. BC and BCSC addition significantly enhanced the total root area and root diameter of sorghum by 23.1–23.2% and 17.8–73.3%, respectively, compared to CK treatment. However, SC decreased the total root area and root diameter by 33.3 and 24.2%, respectively. In addition, BCSC and SC application increased and decreased the root total volume of sorghum by 86.4 and 51.5%, respectively. BC and BCSC treatments showed nonsignificant effects on the root tips, but SC significantly increased it by 122.3%.
Soil pH was nonsignificantly affected by the amendments except BCSC, which significantly increased the pH value from 8.5 to 8.8 in Rh soil (Figure 3A). EC was decreased by 19.0–54.3% and 44.7–53.1% with the amendment of SC and BCSC in the soil of Rh and NRh, respectively. (Figure 3B). BC and BCSC treatments significantly increased the SOM content in the Rh soil by 71.8 and 61.3%, respectively (Figure 3C). For Rh soil, BC increased WHC by 10.5%. However, SC and BCSC showed little influence on it (Figure 3D). For the NRh soil, WHC increased by 9.5–15.0% with BC, SC, and BCSC amendment. These three amendments also remarkably increased the CEC by 28.0–60.3% and 18.5–27.5% in Rh and NRh soil, respectively (Figure 3E).
FIGURE 3. Effect of biochar, compost, and biochar-compost addition on soil properties. (A) pH, (B) EC, (C) SOM, (D) WHC, and (E) CEC. CK: soil without amendment; BC: soil amended with corn straw biochar at 1.0% (w/w); SC: soil amended with seaweed compost at 1.0% (w/w); and BCSC: soil amended with cocomposted biochar and seaweed at 1.0% (w/w). The error bars represent the standard error of the mean (n = 3). Different small letters indicate the significant difference between the soil treatments, and asterisks indicate the significant difference between the rhizosphere and non-rhizosphere soils (p < 0.05).
For the Rh soil, BC and BCSC treatments increased the content of NH4+-N by 25.7 and 16.0% compared to CK, respectively, while SC showed little influence (Figure 4A). The biochar and compost treatments showed nonsignificant influence on the NH4+-N content in the NRh soil. BC and SC had opposite effects on the NO3−-N content in the Rh soil, which significantly decreased and increased by 80.9 and 124.5%, respectively, while BCSC addition showed no remarkable effect (Figure 4B). For the NRh soil, BC and BCSC decreased the NO3−-N content by 85.8 and 42.4%, respectively, while SC increased it by 81.6%. The NO3−-N content in the NRh soil was significantly higher than that in the Rh soil under SC treatment, but exhibited a nonsignificant difference between the NRh and Rh soil under the other three treatments. For the Rh soil, BC treatment significantly increased the Olsen-P content by 17.4%, while it showed little effect in the NRh soil. SC and BCSC significantly increased the Olsen-P content by 12.3–55.2% and 27.8–50.5%, respectively, in the Rh and NRh soil (Figure 4C). BC, SC, and BCSC additions significantly increased the AK content by 48.8–79.0% and 54.0–74.1% in the Rh and NRh soil, respectively (Figure 4D).
FIGURE 4. Effect of biochar, compost, and biochar-compost addition on N, P and K availability in the soil with sorghum. (A) NH4+–N, (B) NO3-–N, (C) Olsen-P, and (D) AK. CK: soil without amendment; BC: soil amended with corn straw biochar at 1.0% (w/w); SC: soil amended with seaweed compost at 1.0% (w/w); and BCSC: soil amended with cocomposted biochar and seaweed at 1.0% (w/w). The error bars represent the standard error of the mean (n = 3). Different small letters indicate the significant difference between the soil treatments, and asterisks indicate the significant difference between the rhizosphere and non-rhizosphere soils (p < 0.05).
In this study, the diversity and response of the bacterial community in the Rh soil were investigated. The SC and BCSC treatments significantly enhanced the bacterial richness (expressed as Chao1 index, Figure 5A) by 4.8 and 8.2%, respectively. Consistently, BC, SC, and BCSC treatments improved the Shannon indexes, but SC addition showed a subtler effect (Figure 5B). There were 233, 231, 326, and 198 unique OTUs observed, respectively, in the BC, SC, BCSC, and CK treatments in the Venn diagram (Figure 5C). According to the results of principal component analysis (PCA) of Bray–Curtis distances, the first two principal components explained 45.74% of the variation in the total soil bacterial community, and BC, SC and BCSC treatments showed different clustering features compared to CK treatment (Figure 5D). Figure 4E shows that the nine most abundant phyla were Actinobacteriota, Proteobacteria, Chloroflexi, Bacteroidetes, Acidobacteria, Gemmatimonadetes, Myxococcota, Firmicutes, Bacteroidota, and Methylomirabilota, which together accounted for 93.2–94.1% of the total bacterial taxa. BC treatment increased the relative abundances of Chloroflexi, Acidobacteriota, Myxococcota, Bacteroidota, and Firmicutes by 2.3–55.1%, but decreased the relative abundances of Actinobacteriota, Proteobacteria, Gemmatimonadota and Methylomirabilota. SC amendment increased the relative abundances of Chloroflexi and Acidobacteriota by 9.7 and 15.1%, respectively, while decreased the relative abundances of Actinobacteriota, Proteobacteria, Gemmatimonadota, Myxococcota, Firmicutes, and Bacteroidota. BCSC amendment increased the relative abundances of Proteobacteria, Chloroflexi, Acidobacteriota, and Bacteroidota by 0.7–13.8%, but decreased the relative abundances of Actinobacteriota, Gemmatimonadota, Myxococcota, and Firmicutes. At the family level, BC amendments increased the relative abundances of Vicinamibacteraceae by 39% (Figure 5F), but decreased the abundances of Geodermatophilaceae by 20.7%. SC treatment increased the abundances of A4b and Pyrinomonadaceae by 46.4 and 23.1%, respectively, but decreased the abundances of Geminicoccaceae, norank_o_Gaiellales, and Geodermatophilaceae by 16.7–19.2%. BCSC treatment increased the relative abundances of Sphingomonadaceae by 41.5%, but decreased the abundance of Gemmatimonadaceae, Gaiellales, and Bacillaceae by 22.2–32.0%. The relationships between the soil properties and bacterial community under different treatments are shown by the RDA analysis (Figure 6). The first two axes explained 84.3% of the total variance. Soil pH, TP, SOM, CEC, NO3−-N, NH4+-N, and AK were the key factors related to the composition and diversity of the bacterial communities.
FIGURE 5. Effect of biochar, compost, and biochar-compost on the microbial community in coastal soils planted with sorghum. (A) Chao1 index; (B) Shannon index; (C) Venn diagram showing the shared bacterial OTUs; (D) principal component analysis (PCA) of bacterial community composition based on Bray–Curtis similarity; (E) the relative abundance of bacteria at the phylum level; and (F) heatmap of the top 15 bacteria at the family level using the Bray–Curtis distance and complete clustering method in the coastal soil with biochar amendment. CK: soil without amendment; BC: soil amended with corn straw biochar at 1.0% (w/w); SC: soil amended with seaweed compost at 1.0% (w/w); and BCSC: soil amended with cocomposted biochar and seaweed at 1.0% (w/w).
FIGURE 6. Redundancy analysis (RDA) of the relationship between the bacterial family (blue arrows) and soil properties (red arrows) for coastal soil. CK: soil without amendment; BC: soil amended with corn straw biochar at 1.0% (w/w); SC: soil amended with seaweed compost without biochar at 1.0% (w/w); and BCSC: soil amended with cocomposted biochar and seaweed at 1.0% (w/w).
In this study, both BC and BCSC significantly increased the growth and yield of sorghum (Figure 2; Table 2), indicating that BC and BCSC amendments had the potential to improve soil conditions and increase plant productivity in the coastal soil (Zheng et al., 2018; Cai et al., 2021). In addition, compared to BC amendment, a higher fresh biomass of root and shoot (Figure 2A) and a higher grain dry biomass (Figure 2D) of sorghum in BCSC amendment also indicated a greater potential of BCSC to enhance plant growth. Similar results were also found in a previous study, which showed that the addition of the mixture of 75% farm manure and 25% biochar at 2% improved the wheat grain yield by 26–28%, compared to the only compost of farm manure in an alkaline soil (Qayyum et al., 2017). Liang et al. (2021) reported that 1.5% biochar-compost treatment increased the total biomass of Phragmites australis by 35.5% in coastal soil, ascribing to the increase of the nutrient utilization by plants, improvement of soil properties, enhancement of soil nutrient, decrease of soil EC, and alleviation of salinity stress, which was consistent with our results (Figures 2, 3). Similarly, the positive effect of BC and BCSC amendments on sorghum growth might be due to the improvement of soil properties (Agegnehu et al., 2016), enhancement of nutrient availability (Hannet et al., 2021), and shift of the soil bacterial community (Bello et al., 2021). For example, Liu et al. (2019b) found no significant effects on crown daisy and leaf lettuce yields when peanut shell-derived biochar (PBC) was added at 3% in an infertile soil, while 3% PBC-compost addition increased the yield by 15.8–107%, due to the improved soil nutrient availability and nutrient uptake in coastal saline soil. Consequently, the soil properties and nutrients were closely related to the growth and yield of sorghum, which should be further analyzed. In addition, the inhibition of sorghum growth and yield with SC addition in our study might be attributed to the phytotoxins produced by SC (Siles-Castellano et al., 2020). Likewise, Purwaningsih et al. (2021) also reported that wood biochar–seaweed compost of 60 t ha−1 could increase the growth of soybean by 32.5% compared to the compost without biochar. The increased root morphologies (Table 2) of sorghum by BC and BCSC amendment might result from the improved soil porosity, which was beneficial for water infiltration (Chang et al., 2021).
The results of this study supported our hypothesis that soil properties could be improved by BCSC addition in coastal soil (Figure 3). The increased SOM and WHC with BC and BCSC addition (Figures 3C,D) might be attributed to the high carbon content of BC and BCSC (Table 1), which could improve soil quality and fertility (Arif et al., 2017) and prevent nutrient leaching. In accordance with a previous study, biochar-compost could enhance the WHC and SOM content in the agricultural soil and then increase the growth of apple trees (Safaei Khorram et al., 2019). In this study, different amendments showed little influence on soil pH, which is mainly ascribed to the similar pH of the soil (8.35) and additives (7.30–9.75) (Table 1, Naeem et al., 2018). EC indicates the soil salt concentration, which would affect plant total mass through root growth (Xia et al., 2019). Generally, salinity stress could have negative effects on the morphology of root systems, further influence the nutrient utilization, and lead to an inhibition of plant growth eventually (Zhao et al., 2020). In this study, biochar and compost additions significantly decreased the EC of Rh and NRh soil (Figure 2B), which might be attributed to the increased growth and yield of sorghum by enhancing soil nutrient utilization.
In addition, biochar could alter nutrient availability for plant growth in soils (Agegnehu et al., 2017; Zhang et al., 2020). Previous studies reported the inconsistent influence of biochar on the content of available soil nitrogen. For example, Yoo et al. (2014) reported that 10% rice chaff biochar decreased the NH4+-N content and increased the NO3−-N content compared with the treatment without biochar. However, Gao et al. (2019) reported that biochar application decreased the NH4+-N content by 11% and the NO3−-N content by 12% in soil. Zheng et al. (2018) also found that peanut shell biochar addition to the soil at 1.5–10% could decrease the NO3−-N content by 62.7%–89.3% in the Rh and NRh soils. In this study, both BC and BCSC amendments increased the content of NH4+-N and decreased the content of NO3−-N of coastal soil. This might be due to the importance of biochar in stimulating NH4+ immobilization into organic N and decreasing NO3− production potential in soil (Xie et al., 2020). However, Nelson et al. (2016) found that biochar could influence the process of N fixation pathways from NO3− to NH4+, which might weaken the nitrification process (Wang et al., 2014) and increase the content of NO3−-N in the soils.
Additionally, the increased soluble P contents (Figure 4C) after BC and BCSC addition might be related to the P directly released from the amendments and the high adsorption capacity of them, which could inhibit the leaching of P in the soil (Manolikaki and Diamadopoulos, 2019; Yang et al., 2021b). Similar results were also found in the available soil K that the increased soil K content could restrain the Na uptake by plants, which would be beneficial for alleviating soil salt stress and promoting plant growth (Yu et al., 2019). Therefore, soil properties and nutrient availability could be improved by BC and BCSC addition in coastal soils. The inconsistent effects of different amendments on soil physicochemical properties, nutrient states, and plant growth might be because of the differences in soil microbial diversities and community structures, which will be discussed as follows.
In this study, both BC and BCSC amendments significantly induced the shift of the soil bacterial community structure and diversity (Figure 5). For example, BCSC amendment significantly improved the richness and diversity of soil microbes compared with CK (Figures 5A,B), indicating an increased bacterial growth. This was consistent with the results of previous studies that biochar, rich in polyporus and having large surface area, could provide not only a good habitat but also nutrients and C source for soil bacterial growth (Wu et al., 2017; João et al., 2021). Nonetheless, the higher increase in unique OTUs in BC, SC, and BCSC treatments compared to CK treatment (Figure 5C) and the different clustering features of the PCA (Figure 5D) also suggested an enhanced bacterial growth. Moreover, many studies evidenced that biochar could shift microbial communities (Zheng et al., 2018; Yu et al., 2019). In our study, BCSC treatment enhanced the abundance of Proteobacteria, Chloroflexi, and Acidobacteriota compared to CK, but decreased the abundance of Actinobacteriota (Figure 5E). It was reported that Proteobacteria had plant growth-promoting effects by nitrogen fixation and alleviating abiotic stresses (Bruto et al., 2014). Chloroflexi and Acidobacteriota were also reported to have positive influences on enhancing plant growth by promoting nitrogen uptake (Emmett et al., 2020; Hua et al., 2021). These bacteria that benefit plant growth under BCSC treatment might be contributing to the growth and yield of sorghum in coastal soil.
At the family level, BC increased the relative abundance of Vicinamibacteraceae (Figure 5F). It was reported that Vicinamibacteraceae was positively correlated to soil available N content (Yi et al., 2022). Therefore, we speculated that the increased soil NH4+-N content in coastal soil might be due to the enhanced relative abundance of Vicinamibacteraceae under BC treatment. In addition, BCSC treatment increased the abundance of Sphingomonadaceae and decreased the abundance of Gemmatimonadaceae compared to CK treatment (Figure 5F). Previous studies had reported that Sphingomonadaceae was a degrader of organic material (Fei et al., 2020) and could be frequently involved in N and P transformations (Yang et al., 2014; Wang et al., 2020), which revealed that the organic N and P in the soil could be transformed into the available phases and become more easily absorbed by sorghum. This result was consistent with the increased NH4+-N and Olsen-P content in the soil. Li et al. (2021) reported that Gemmatimonadaceae was significantly negatively correlated with the content of available N and P, indicating that the bacteria was adapted to the soil with insufficient P. The result was consistent with the decreased relative abundance of Gemmatimonadaceae in the coastal soil with more available N and P in BCSC treatment compared to CK treatment.
Furthermore, the relative abundance of Sphingomonadaceae was positively correlated with the content of Olsen-P in the soil. The abundance of Gemmatimonadaceae and Vicinamibacteraceae was positively correlated with NO3−-N, NH4+-N, and AK in the soil. These results revealed that the changes of microbial community in the soils might contribute to improving soil properties and eventually enhancing the growth and yield of plants. Overall, the enhanced soil nutrient availability and physicochemical properties, the increased soil bacterial diversity and activity, and the altered soil bacterial community, especially the abundance of bacteria such as Proteobacteria, Chloroflexi and Acidobacteriota under CSC treatment, together enhanced the growth and yield of sorghum in coastal soil (Figure 2).
This study demonstrated that BC and BCSC additions could significantly improve the growth and productivity of sorghum and BCSC had a better performance. The promotion effect of BC and BCSC was mainly attributed to increased soil properties (WHC, SOM, Olsen-P, and AK content), decreased soil EC, and changed soil microbial community. The increase of the abundance of Sphingomonadaceae and Vicinamibacteraceae in the soil was positively correlated with the content of available nutrients in soil, which eventually enhanced the growth and yield of sorghum in costal soil. The results of our study indicated that biochar-compost has the potential to be an amendment for improving soil properties and enhancing plant productivity in the coastal soil. The production technologies of biochars with different feedstocks, the relationships between the properties of biochars, and the soil physiochemical properties should be further explored. Moreover, the technologies of inoculating functional bacteria that could promote plant growth onto biochars were worth developing and applying in coastal soils in the future.
The original contributions presented in the study are included in the article/supplementary material. Further inquiries can be directed to the corresponding authors.
SY: investigation, methodology, formal analysis, and writing—original draft; FS: investigation, methodology, and writing—review and editing; YZ: investigation and methodology; XY: conceptualization, funding acquisition, data curation, formal analysis, and writing—review and editing; HL: linguistic assistance; JW: investigation and methodology; CZ: validation and writing—review and editing; YL: supervision and funding acquisition; and YC: project administration and resources.
This work was financially funded by the Demonstration and Guidance Program for Technology People-Benefit in Qingdao (20-3-4-7-nsh), the Science and Technology Demonstration Project of “Bohai Granary” of Shandong Province (2019BHLC002), and the Agricultural Science and Technology Innovation Program of China (ASTIP-TRIC06).
The authors declare that the research was conducted in the absence of any commercial or financial relationships that could be construed as a potential conflict of interest.
All claims expressed in this article are solely those of the authors and do not necessarily represent those of their affiliated organizations, or those of the publisher, the editors, and the reviewers. Any product that may be evaluated in this article, or claim that may be made by its manufacturer, is not guaranteed or endorsed by the publisher.
The Supplementary Material for this article can be found online at: https://www.frontiersin.org/articles/10.3389/fenvs.2022.1036837/full#supplementary-material
ANOVA, one-way analysis of variance; AK, available potassium; BC, biochar; BCSC, cocomposted BC and SC; CEC, cation exchange capacity; EC, electrical conductivity; NRh, non-rhizosphere; OTUs, operational taxonomic units; PBC, peanut shell-derived biochar; PCA, principal component analysis; RDA, redundancy analysis; Rh, rhizosphere; SC, seaweed compost; SOM, soil organic matter; and WHC, water holding capacity.
Agegnehu, G., Bass, A. M., Nelson, P. N., and andand Bird, M. I. (2016). Benefits of biochar, compost and biochar–compost for soil quality, maize yield and greenhouse gas emissions in a tropical agricultural soil. Sci. Total Environ. 543, 295–306. doi:10.1016/j.scitotenv.2015.11.054
Agegnehu, G., Srivastava, A. K., and Bird, M. I. (2017). The role of biochar and biochar-compost in improving soil quality and crop performance: A review. Appl. soil Ecol. 119, 156–170. doi:10.1016/j.apsoil.2017.06.008
Antonangelo, J. A., Sun, X., and Zhang, H. (2021). The roles of co-composted biochar (COMBI) in improving soil quality, crop productivity, and toxic metal amelioration. J. Environ. Manag. 277, 111443. doi:10.1016/j.jenvman.2020.111443
Arif, M., Ilyas, M., Riaz, M., Shah, K., and Ul Haq, I. (2017). Biochar improves phosphorus use efficiency of organic-inorganic fertilizers, maize-wheat productivity and soil quality in a low fertility alkaline soil. Field crops Res. 214, 25–37. doi:10.1016/j.fcr.2017.08.018
Bello, A., Wang, B., Zhao, Y., Yang, W., Ogundeji, A., Deng, L., et al. (2021). Composted biochar affects structural dynamics, function and co-occurrence network patterns of fungi community. Sci. Total Environ. 775, 145672. doi:10.1016/j.scitotenv.2021.145672
Bruto, M., Prigent-Combaret, C., Muller, D., and Moenne-Loccoz, Y. (2014). Analysis of genes contributing to plant-beneficial functions in plant growth-promoting rhizobacteria and related Proteobacteria. Sci. Rep. 4 (1), 6261–6310. doi:10.1038/srep06261
Cai, J. F., Jiang, F., Liu, X. S., Sun, K., Wang, W., Zhang, M. X., et al. (2021). Biochar-amended coastal wetland soil enhances growth of Suaeda salsa and alters rhizosphere soil nutrients and microbial communities. Sci. Total Environ. 788, 147707. doi:10.1016/j.scitotenv.2021.147707
Chang, Y., Rossi, L., Zotarelli, L., Gao, B., Shahid, M. A., and Sarkhosh, A. (2021). Biochar improves soil physical characteristics and strengthens root architecture in Muscadine grape (Vitis rotundifolia L.). Chem. Biol. Technol. Agric. 8 (1), 7–11. doi:10.1186/s40538-020-00204-5
Emmett, B. D., Buckley, D. H., and Drinkwater, L. E. (2020). Plant growth rate and nitrogen uptake shape rhizosphere bacterial community composition and activity in an agricultural field. New Phytol. 225 (2), 960–973. doi:10.1111/nph.16171
Fei, Y., Huang, S., Zhang, H., Tong, Y., Wen, D., Xia, X., et al. (2020). Response of soil enzyme activities and bacterial communities to the accumulation of microplastics in an acid cropped soil. Sci. Total Environ. 707, 135634. doi:10.1016/j.scitotenv.2019.135634
Gao, S., DeLuca, T. H., and Cleveland, C. C. (2019). Biochar additions alter phosphorus and nitrogen availability in agricultural ecosystems: A meta-analysis. Sci. Total Environ. 654, 463–472. doi:10.1016/j.scitotenv.2018.11.124
Guo, X., Liu, H., and Zhang, J. (2020). The role of biochar in organic waste composting and soil improvement: A review. Waste Manag. 102, 884–899. doi:10.1016/j.wasman.2019.12.003
Hallam, J., and Hodson, M. E. (2020). Impact of different earthworm ecotypes on water stable aggregates and soil water holding capacity. Biol. Fertil. Soils 56, 607–617. doi:10.1007/s00374-020-01432-5
Hannet, G., Singh, K., Farrar, M. B., Muqaddas, B., and Bai, S. H. (2021). Effects of biochar, compost, and biochar-compost on soil total nitrogen and available phosphorus concentrations in a corn field in Papua New Guinea. Environ. Sci. Pollut. Res. 28 (21), 27411–27419. doi:10.1007/s11356-021-12477-w
Hua, B., Li, Z., Gao, W., Feng, H., Chen, N., Li, J., et al. (2021). Soil amendment in plastic greenhouse using modified biochar: Soil bacterial diversity responses and microbial biomass carbon and nitrogen. Biotechnol. Lett. 43 (3), 655–666. doi:10.1007/s10529-020-03046-1
Huang, B., Jia, H., Han, X., Gou, J., Huang, C., Wang, J., et al. (2021). Effects of biocontrol Bacillus and fermentation bacteria additions on the microbial community, functions and antibiotic resistance genes of prickly ash seed oil meal-biochar compost. Bioresour. Technol. 340, 125668. doi:10.1016/j.biortech.2021.125668
Huang, L., and Gu, M. (2019). Effects of biochar on container substrate properties and growth of plants—a review. Horticulturae 5 (1), 14. doi:10.3390/horticulturae5010014
Kumar, A., Prakash, C. B., Brar, N. S., and Kumar, B. (2018). Potential of vermicompost for sustainable crop production and soil health improvement in different cropping systems. Int. J. Curr. Microbiol. Appl. Sci. 7 (10), 1042–1055. doi:10.20546/ijcmas.2018.710.116
Li, J. H., Cheng, B. H., Zhang, R., Li, W. J., Shi, X. M., Han, Y. W., et al. (2021). Nitrogen and phosphorus additions accelerate decomposition of slow carbon pool and lower total soil organic carbon pool in alpine meadows. Land Degrad. Dev. 32 (4), 1761–1772. doi:10.1002/ldr.3824
Li, X. (2018). Salt leaching and Iris germanica L. growth in two coastal saline soils under drip irrigation with saline water. Sci. Hortic. 237, 164–168. doi:10.1016/j.scienta.2018.04.002
Liang, J. F., Li, Q. W., Gao, J., Feng, J. G., Zhang, X. Y., Wu, Y. Q., et al. (2021). Biochar rhizosphere addition promoted Phragmites australis growth and changed soil properties in the Yellow River Delta. Sci. Total Environ. 761, 143291. doi:10.1016/j.scitotenv.2020.143291
Liu, B., Cai, Z., Zhang, Y., Liu, G., Luo, X., and Zheng, H. (2019a). Comparison of efficacies of peanut shell biochar and biochar-based compost on two leafy vegetable productivity in an infertile land. Chemosphere 224, 151–161. doi:10.1016/j.chemosphere.2019.02.100
Liu, M., Wang, C., Wang, F., and Xie, Y. (2019b). Maize (Zea mays) growth and nutrient uptake following integrated improvement of vermicompost and humic acid fertilizer on coastal saline soil. Appl. Soil Ecol. 142, 147–154. doi:10.1016/j.apsoil.2019.04.024
Lu, H., Lashari, M. S., Liu, X., Ji, H., Li, L., Zheng, J., et al. (2015). Changes in soil microbial community structure and enzyme activity with amendment of biochar-manure compost and pyroligneous solution in a saline soil from Central China. Eur. J. Soil Biol. 70, 67–76. doi:10.1016/j.ejsobi.2015.07.005
Luo, X., Liu, G., Xia, Y., Chen, L., Jiang, Z., Zheng, H., et al. (2017). Use of biochar-compost to improve properties and productivity of the degraded coastal soil in the Yellow River Delta, China. J. Soils Sediments 17 (3), 780–789. doi:10.1007/s11368-016-1361-1
Manolikaki, I., and Diamadopoulos, E. (2019). Positive effects of biochar and biochar-compost on maize growth and nutrient availability in two agricultural soils. Commun. Soil Sci. Plant Anal. 50 (5), 512–526. doi:10.1080/00103624.2019.1566468
Naeem, M. A., Khalid, M., Aon, M., Abbas, G., Amjad, M., Murtaza, B., et al. (2018). Combined application of biochar with compost and fertilizer improves soil properties and grain yield of maize. J. Plant Nutr. 41 (1), 112–122. doi:10.1080/01904167.2017.1381734
Nelson, M. B., Martiny, A. C., and Martiny, J. B. (2016). Global biogeography of microbial nitrogen-cycling traits in soil. Proc. Natl. Acad. Sci. 113 (29), 8033–8040. doi:10.1073/pnas.1601070113
Pandit, N. R., Schmidt, H. P., Mulder, J., Hale, S. E., Husson, O., and Cornelissen, G. (2020). Nutrient effect of various composting methods with and without biochar on soil fertility and maize growth. Archives Agron. Soil Sci. 66 (2), 250–265. doi:10.1080/03650340.2019.1610168
Purwaningsih, O., Pamungkas, P. B., Beny, D., and Oktavia, M. (2021). Response of soybean growth in sandy coastal soil to seaweed compost and biochar application. J. Phys. Conf. Ser. 1823 (16), 012028. doi:10.1088/1742-6596/1823/1/012028
Qayyum, M. F., Liaquat, F., Rehman, R. A., Gul, M., ul Hye, M. Z., Rizwan, M., et al. (2017). Effects of co-composting of farm manure and biochar on plant growth and carbon mineralization in an alkaline soil. Environ. Sci. Pollut. Res. 24 (33), 26060–26068. doi:10.1007/s11356-017-0227-4
Rehman, M. Z. U., Rizwan, M., Ali, S., Fatima, N., Yousaf, B., Naeem, A., et al. (2016). Contrasting effects of biochar, compost and farm manure on alleviation of nickel toxicity in maize (Zea mays L.) in relation to plant growth, photosynthesis and metal uptake. Ecotoxicol. Environ. Saf. 133, 218–225. doi:10.1016/j.ecoenv.2016.07.023
Safaei Khorram, M., Zhang, G., Fatemi, A., Kiefer, R., Maddah, K., Baqar, M., et al. (2019). Impact of biochar and compost amendment on soil quality, growth and yield of a replanted apple orchard in a 4‐year field study. J. Sci. Food Agric. 99 (4), 1862–1869. doi:10.1002/jsfa.9380
Schmidt, H. P., Kammann, C., Niggli, C., Evangelou, M. W., Mackie, K. A., and Abiven, S. (2014). Biochar and biochar-compost as soil amendments to a vineyard soil: Influences on plant growth, nutrient uptake, plant health and grape quality. Agric. Ecosyst. Environ. 191, 117–123. doi:10.1016/j.agee.2014.04.001
Siles-Castellano, A. B., López, M. J., López-González, J. A., Suarez-Estrella, F., Jurado, M. M., Estrella-Gonzalez, M. J., et al. (2020). Comparative analysis of phytotoxicity and compost quality in industrial composting facilities processing different organic wastes. J. Clean. Prod. 252, 119820. doi:10.1016/j.jclepro.2019.119820
Sun, H., Shi, W., Zhou, M., Ma, X., and Zhang, H. (2019). Effect of biochar on nitrogen use efficiency, grain yield and amino acid content of wheat cultivated on saline soil. Plant Soil Environ. 65 (2), 83–89. doi:10.17221/525/2018-PSE
Sun, J., He, F., Shao, H., Zhang, Z., and Xu, G. (2016). Effects of biochar application on Suaeda salsa growth and saline soil properties. Environ. Earth Sci. 75 (8), 630–636. doi:10.1007/s12665-016-5440-9
Suo, F., You, X., Yin, S., Wu, H., Zhang, C., Yu, X., et al. (2021). Preparation and characterization of biochar derived from co-pyrolysis of Enteromorpha prolifera and corn straw and its potential as a soil amendment. Sci. Total Environ. 798, 149167. doi:10.1016/j.scitotenv.2021.149167
Tang, J., Zhang, L., Zhang, J., Ren, L., Zhou, Y., Zheng, Y., et al. (2020). Physicochemical features, metal availability and enzyme activity in heavy metal-polluted soil remediated by biochar and compost. Sci. Total Environ. 701, 134751. doi:10.1016/j.scitotenv.2019.134751
Teodoro, M., Trakal, L., Gallagher, B. N., Simek, P., Soudek, P., Pohorely, M., et al. (2020). Application of co-composted biochar significantly improved plant-growth relevant physical/chemical properties of a metal contaminated soil. Chemosphere 242, 125255. doi:10.1016/j.chemosphere.2019.125255
Verheijen, F. G., Zhuravel, A., Silva, F. C., Amaro, A., Ben-Hur, M., and Keizer, J. J. (2019). The influence of biochar particle size and concentration on bulk density and maximum water holding capacity of sandy vs sandy loam soil in a column experiment. Geoderma 347, 194–202. doi:10.1016/j.geoderma.2019.03.044
Wang, H., Zheng, H., Jiang, Z., Dai, Y., Liu, G., Chen, L., et al. (2017). Efficacies of biochar and biochar-based amendment on vegetable yield and nitrogen utilization in four consecutive planting seasons. Sci. Total Environ. 593, 124–133. doi:10.1016/j.scitotenv.2017.03.096
Wang, L., Sun, X., Li, S., Zhang, T., Zhang, W., and Zhai, P. (2014). Application of organic amendments to a coastal saline soil in North China: Effects on soil physical and chemical properties and tree growth. PloS one 9 (2), e89185. doi:10.1371/journal.pone.0089185
Wang, M., Xie, X., Wang, M., Wu, J., Zhou, Q., and Sun, Y. (2020). The bacterial microbiota in florfenicol contaminated soils: The antibiotic resistome and the nitrogen cycle. Environ. Pollut. 259, 113901. doi:10.1016/j.envpol.2019.113901
Wu, S., He, H., Inthapanya, X., Yang, C., Zeng, G., et al. (2017). Role of biochar on composting of organic wastes and remediation of contaminated soils—A review. Environ. Sci. Pollut. Res. 24 (20), 16560–16577. doi:10.1007/s11356-017-9168-1
Xia, W. Y., Du, Y. J., Li, F. S., Li, C. P., Yan, X. L., Arulrajah, A., et al. (2019). In-situ solidification/stabilization of heavy metals contaminated site soil using a dry jet mixing method and new hydroxyapatite based binder. J. Hazard. Mater. 369, 353–361. doi:10.1016/j.jhazmat.2019.02.031
Xie, Y., Yang, C., Ma, E., Tan, H., Zhu, T., and Muller, C. (2020). Biochar stimulates NH4+ turnover while decreasing NO3− production and N2O emissions in soils under long-term vegetable cultivation. Sci. Total Environ. 737, 140266. doi:10.1016/j.scitotenv.2020.140266
Yang, F., Sui, L., Tang, C., Li, J., Cheng, K., and Xue, Q. (2021b). Sustainable advances on phosphorus utilization in soil via addition of biochar and humic substances. Sci. Total Environ. 768, 145106. doi:10.1016/j.scitotenv.2021.145106
Yang, S., Zhang, X., Cao, Z., Zhao, K., Wang, S., Chen, M., et al. (2014). Growth‐promoting S phingomonas paucimobilis ZJSH 1 associated with D endrobium officinale through phytohormone production and nitrogen fixation. Microb. Biotechnol. 7 (6), 611–620. doi:10.1111/1751-7915.12148
Yang, Y., Ye, S., Zhang, C., Zeng, G., Tan, X., Song, B., et al. (2021a). Application of biochar for the remediation of polluted sediments. J. Hazard. Mater. 404, 124052. doi:10.1016/j.jhazmat.2020.124052
Yi, M., Zhang, L., Qin, C., Lu, P., Bai, H., Han, X., et al. (2022). Temporal changes of microbial community structure and nitrogen cycling processes during the aerobic degradation of phenanthrene. Chemosphere 286, 131709. doi:10.1016/j.chemosphere.2021.131709
Yoo, G., Kim, H., Chen, J., and Kim, Y. (2014). Effects of biochar addition on nitrogen leaching and soil structure following fertilizer application to rice paddy soil. Soil Sci. Soc. Am. J. 78 (3), 852–860. doi:10.2136/sssaj2013.05.0160
You, X., Yin, S., Suo, F., Xu, Z., Chu, D., Kong, Q., et al. (2021). Biochar and fertilizer improved the growth and quality of the ice plant (Mesembryanthemum crystallinum L.) shoots in a coastal soil of Yellow River Delta, China. Sci. Total Environ. 775, 144893. doi:10.1016/j.scitotenv.2020.144893
Yu, H., Zou, W., Chen, J., Chen, H., Yu, Z., Huang, J., et al. (2019). Biochar amendment improves crop production in problem soils: A review. J. Environ. Manag. 232, 8–21. doi:10.1016/j.jenvman.2018.10.117
Zahra, M. B., Aftab, Z. E. H., Akhter, A., and Haider, M. S. (2021c). Cumulative effect of biochar and compost on nutritional profile of soil and maize productivity. J. Plant Nutr. 44 (11), 1–13. doi:10.1080/01904167.2021.1871743
Zahra, M. B., Aftab, Z. E. H., and Haider, M. S. (2021a). Water productivity, yield and agronomic attributes of maize crop in response to varied irrigation levels and biochar–compost application. J. Sci. Food Agric. 101 (11), 4591–4604. doi:10.1002/jsfa.11102
Zahra, M. B., Fayyaz, B., Aftab, Z. E., and Haider, M. S. (2021b). Mitigation of degraded soils by using biochar and compost: A systematic review. J. Soil Sci. Plant Nutr. 21 (4), 2718–2738. doi:10.1007/s42729-021-00558-1
Zhang, Y., Wang, X., Liu, B., Liu, Q., Zheng, H., You, X., et al. (2020). Comparative study of individual and co-application of biochar and wood vinegar on blueberry fruit yield and nutritional quality. Chemosphere 246, 125699. doi:10.1016/j.chemosphere.2019.125699
Zhao, W., Zhou, Q., Tian, Z., Cui, Y., Liang, Y., and Wang, H. (2020). Apply biochar to ameliorate soda saline-alkali land, improve soil function and increase corn nutrient availability in the Songnen Plain. Sci. Total Environ. 722, 137428. doi:10.1016/j.scitotenv.2020.137428
Zheng, H., Wang, X., Chen, L., Wang, Z., Xia, Y., Zhang, Y., et al. (2018). Enhanced growth of halophyte plants in biochar‐amended coastal soil: Roles of nutrient availability and rhizosphere microbial modulation. Plant Cell. Environ. 41 (3), 517–532. doi:10.1111/pce.12944
Keywords: biochar, compost, saline soil, soil amendment, sorghum yield, soil physicochemical properties, soil bacteria
Citation: Yin S, Suo F, Zheng Y, You X, Li H, Wang J, Zhang C, Li Y and Cheng Y (2022) Biochar-compost amendment enhanced sorghum growth and yield by improving soil physicochemical properties and shifting soil bacterial community in a coastal soil. Front. Environ. Sci. 10:1036837. doi: 10.3389/fenvs.2022.1036837
Received: 05 September 2022; Accepted: 14 October 2022;
Published: 02 November 2022.
Edited by:
Saif Ullah, Guangxi University, ChinaReviewed by:
Muhammad Naeem, Hebei Normal University, ChinaCopyright © 2022 Yin, Suo, Zheng, You, Li, Wang, Zhang, Li and Cheng. This is an open-access article distributed under the terms of the Creative Commons Attribution License (CC BY). The use, distribution or reproduction in other forums is permitted, provided the original author(s) and the copyright owner(s) are credited and that the original publication in this journal is cited, in accordance with accepted academic practice. No use, distribution or reproduction is permitted which does not comply with these terms.
*Correspondence: Xiangwei You, eW91eGlhbmd3ZWlAY2Fhcy5jbg==; Yadong Cheng, Y2hlbmd5YWRvbmdAY2Fhcy5jbg==
†These authors have contributed equally to this work
Disclaimer: All claims expressed in this article are solely those of the authors and do not necessarily represent those of their affiliated organizations, or those of the publisher, the editors and the reviewers. Any product that may be evaluated in this article or claim that may be made by its manufacturer is not guaranteed or endorsed by the publisher.
Research integrity at Frontiers
Learn more about the work of our research integrity team to safeguard the quality of each article we publish.