- 1MOE Key Laboratory of Environment Remediation and Ecological Health, College of Environmental and Resource Science, Zhejiang University, Hangzhou, China
- 2Cultivated Land Quality Monitoring and Protection Center, Ministry of Agriculture and Rural Affairs, Beijing, China
The cadmium (Cd) hyperaccumulator Sedum alfredii has been identified to have great ability to accumulate >100 ppm (dry weight) of Cd in its aboveground biomass. However, little attention has been paid to the possibility that S. alfredii may benefit from this trait. Here, we investigated the effect of Cd accumulation on the performance of the black bean aphid Aphis fabae in S. alfredii. The results showed that 6 weeks of Cd exposure prevented S. alfredii from being infested by aphids. In another experiment, S. alfredii was pretreated with 100 μmol⋅dm⁻³ CdCl2 for 7 days. Prolonged Cd exposure significantly reduced the number of aphids in the Cd-pretreated S. alfredii after 7 days of aphid infestation. The Cd concentration in the phloem exudates of S. alfredii was also high. Micro X-ray fluorescence mapping of aphids collected from Cd-treated plants revealed high levels of Cd in the stylets. In summary, Cd protects S. alfredii from A. fabae through toxicity, but not deterrence, which may be related to the abundance of Cd in the phloem.
1 Introduction
Contamination of arable soil with heavy metals has become a severe environmental issue, especially in China (Zhao et al., 2015; He et al., 2017). Cadmium (Cd), the most severe and prevalent heavy metal contaminant in soil, negatively impacts the human health and ecosystems (Wen-Li et al., 2014; Rubina et al., 2020). Phytoremediation is a feasible and cost-efficient strategy for the remediation of soils contaminated with heavy metals (Clemens et al., 2013). Cadmium-hyperaccumulating plants, which can accumulate metals beyond the critical concentration level (>100 ppm, dry weight) in their shoots, are prerequisites for efficient phytoremediation (Kramer, 2010; Verbruggen et al., 2013). In recent decades, considerable interest has been shown in these particular plant species. Findings have revealed that their metal hyperaccumulation ability mainly depends on active root uptake, efficient xylem loading, powerful leaf sequestration, and efficient phloem remobilization (Navariizzo, 2010; Ent et al., 2013; Tian et al., 2016; Tian et al., 2017; Hu et al., 2019b). Although progress has been made concerning research in metal transport and accumulation (Lu et al., 2008; Hu et al., 2019a), few studies have been devoted to understanding the relationship between heavy metals and biotic stress, particularly in insects (Dai et al., 2020; Shimizu-Inatsugi et al., 2021; Liu et al., 2022). Several hypotheses have been proposed regarding the possible ecological functions of metal hyperaccumulation (Tewes et al., 2018). Among these hypotheses, the elemental defense hypothesis is the most popular (Boyd, 2007; Boyd, 2013), which postulates that high internal metal concentrations could protect plants by reducing the performance of pathogens and herbivores. The deterrent and toxic effects of metals on herbivores have been addressed in several studies (Rascio and Navari-Izzo, 2011; Boyd, 2013). Generally, toxic metals are quite effective in defending against tissue-chewing herbivores (Lin et al., 2020), whereas the results of studies on vascular tissue-feeding insects are controversial or negative (Boyd, 2007; Lin et al., 2020). Therefore, enhancing traits related to insect resistance and promoting metal hyperaccumulation in shoots may contribute to optimizing phytoremediation efficiency.
Aphids are typical phloem feeders that tap phloem tissues to obtain sugar and amino acids from the phloem fluid, and they are important pests of agricultural and horticultural crops worldwide (Will et al., 2013). Generally, aphids prefer water-enriched plants to wooden plants (Will et al., 2013). Several studies have shown that metal accumulation may enhance aphid resistance in plants. For example, it has been reported that Zn and Cd in Arabidopsis helleri (Stolpe et al., 2017; Irfan Naikoo et al., 2021b) and Se in Brassica juncea (Hanson et al., 2004; Shi et al., 2020) were effective in reducing aphid performance in corresponding plants. However, contrasting results have also been shown, such as with the aphids that were unaffected by Ni in the hyperaccumulator Streptanthus polygaloides (Boyd and Martens, 1999; Irfan Naikoo et al., 2021a) and by Cd in Brassica juncea (Konopka et al., 2013; Butt et al., 2018). Sedum alfredii is a Cd-hyperaccumulating plant native to China (Yang et al., 2004; Tian et al., 2010). However, aphids are a severe threat to the growth of S. alfredii and heavy metal phytoremediation. Although the ability of S. alfredii to concentrate Cd in its aboveground parts has been widely studied (Lu et al., 2008; Tian et al., 2016; Hu et al., 2019b), there is no consistent conclusion on whether S. alfredii could benefit from Cd accumulation in terms of resisting aphids. Therefore, in this study, we pretreated S. alfredii with 0 or 100 μmol⋅dm⁻³ Cd under aphid infestation to investigate aphid Cd distribution and feeding behavior. Thus, the objectives of this study were to 1) examine the effect of Cd on aphid resistance in S. alfredii and 2) explore the relationship between biotic stress and metal remediation.
2 Materials and methods
2.1 Plant culture
Seeds of Cd-hyperaccumulating S. alfredii were collected from an old Pb/Zn mine in Zhejiang Province, China (Yang et al., 2004). The seeds were grown in non-contaminated soil for several generations, and healthy and uniform shoots were selected and pre-cultured hydroponically, as previously reported (Lu et al., 2008). The hydroponic solution contained 2 mmol⋅dm⁻³ Ca(NO3)2, 0.1 mmol⋅dm⁻³ KCl, 0.1 mmol⋅dm⁻³ KH2PO4, 0.7 mmol⋅dm⁻³ K2SO4, 0.5 mmol⋅dm⁻³ MgSO4, 10 μmol⋅dm⁻³ H3BO3, 0.5 μmol⋅dm⁻³ MnSO4, 5 μmol⋅dm⁻³ ZnSO4, 0.2 μmol⋅dm⁻³ CuSO4, 0.01 μmol⋅dm⁻³ (NH4)6Mo7O24, and 50 μmol⋅dm⁻³ Fe-EDTA and was replaced every 3 days. The pH was adjusted to 5.8 using 0.1 mol⋅dm⁻³ HCl. The seedlings were cultivated in a natural light greenhouse (350 μmol m−2s−2, 16 h light/8 h dark) with a cooling system, maintaining the temperature at 22–25°C and the humidity at 60%–70%.
2.2 Aphid infestation treatment
The aphid used in the present study was the black bean aphid Aphis fabae Scopoli (Hemiptera, Aphididae), which naturally colonizes S. alfredii. In the greenhouse, an aphid attack occurred naturally before the experiment. During the experiment, sick S. alfredii seedlings were continuously infested with A. fabae, making the greenhouse a susceptible environment. A separate experiment was designed for aphid infestation treatment. First, 4-week-old S. alfredii seedlings were pretreated with 0 or 100 μmol⋅dm⁻³ CdCl2 for 7 days and then exposed to aphids (Figure 1A) for another 7 days of treatment. During this period, plants infested with aphids were continuously treated with 0 or 100 μmol⋅dm⁻³ CdCl2. Each treatment was replicated four times. Four seedlings were placed in a transparent plastic box with a ventilating window covered with an insect net for each replicate. Two out of four seedlings pretreated with 0 or 100 μmol⋅dm⁻³ CdCl2 were randomly chosen. Then, 200 adult aphids collected from Cd-free S. alfredii plants (mainly used for aphid culture) were placed at the bottom of the box in the center, where each seedling was equally accessible. One hour later, for each replicate, one host plant with 0 μmol⋅dm⁻³ CdCl2 and one with 100 μmol⋅dm⁻³ CdCl2 were randomly chosen for determining the number of aphids on each seedling and the concentration of Cd in aphids and plants. This was used to determine the preference of aphids for choosing a host plant.
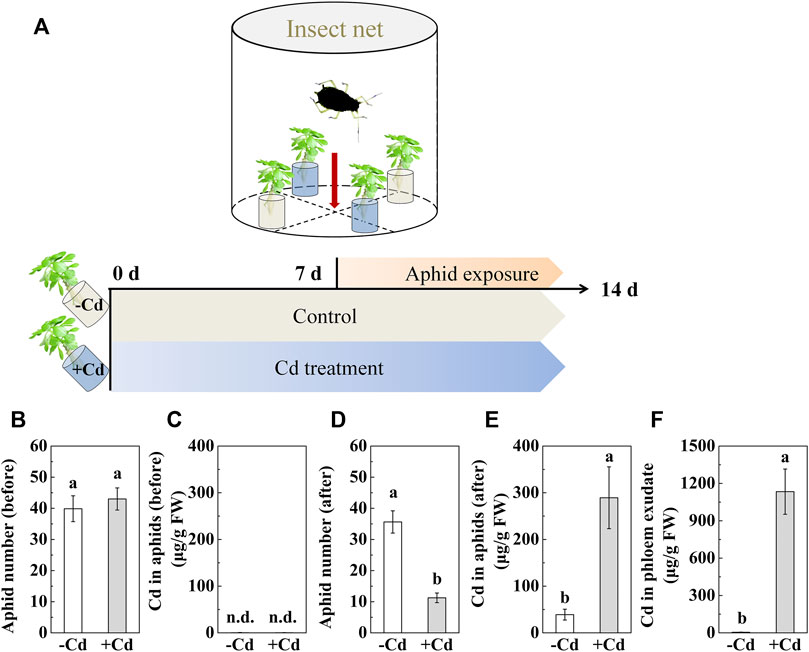
FIGURE 1. Short-term effect of Cd accumulation on aphid (Aphis fabae Scopoli) colonization in the Cd hyperaccumulator Sedum alfredii in a small and closed environment. (A) Experimental procedure. Four-week-old seedlings were pretreated with 0 (-Cd) or 100 μmol⋅dm⁻³ (+Cd) CdCl2 for 7 days and were then exposed to aphids that were collected from Cd-free S. alfredii plants (mainly used for aphid culture) for another 7 days of treatment. In this period, the plants infested with aphids were continuously treated with 0 or 100 μmol⋅dm⁻³ CdCl2. (B) Number of adult aphids on S. alfredii with 0 or 100 μmol⋅dm⁻³ Cd after 1 h of infestation with 200 aphids randomly placed in each box. (C) Cd concentration in aphids before culturing on Cd-pretreated plants. (D) Number of adult aphids on S. alfredii with 0 or 100 μmol⋅dm⁻³ Cd after 7 days of infestation with 200 aphids randomly placed in each box. (E) Cd concentration in surviving aphids after 7 days of culturing in S. alfredii. (F) Cd concentration in phloem exudates collected from leaves of S. alfredii after being treated with 0 or 100 μmol⋅dm⁻³ CdCl2 for 14 days. Data are shown as means ± SD (n = 4). Different letters indicate significant differences between the control and Cd-treated plants (t-test, p < 0.01). n. d. indicates that the Cd content was too low to be detected. FW, fresh weight.
For another experiment, 4-week-old seedlings of S. alfredii were pretreated with nutrient solutions containing 0 or 100 μmol⋅dm⁻³ CdCl2 for 7 days, which were reapplied every 4 days. Plants were then placed in a greenhouse (350 μmol m−2s−2, 16 h light/8 h dark, 22–25°C, 60%–70% humidity) with aphids for another 6 weeks of treatment. During this period, the plants infested with aphids were treated with or without 100 μmol⋅dm⁻³ CdCl2. Each treatment consisted of 12 seedlings (four in one pot per replicate), and the pots were randomized.
2.3 Parameter determination
2.3.1 Element determination
After the aphid infestation treatment, plant growth and Cd concentrations in plant tissues and aphids for both the control and Cd-treated S. alfredii were determined. Briefly, samples were rinsed with deionized water and dried with a paper towel. The samples were then dried in an oven at 75°C until a constant weight was obtained. After the plant tissues and aphids were digested with HNO3-H2O2 (3:1, v/v) at 180°C, the concentrations were determined by inductively coupled plasma mass spectrometry (Agilent 7500a, United States) (Tian et al., 2011).
2.3.2 Elemental mapping by micro-X ray fluorescence
In each treatment (0 or 100 μmol⋅dm⁻³ CdCl2), five aphids were randomly selected for micro-X-ray analysis at 1 h and 7 days after the onset of aphid infestation. As previously described (Tian et al., 2011; Tian et al., 2016), the elemental distribution of the aphids was collected by micro-X-ray fluorescence (XRF) in five replicates. In brief, aphids were directly pasted on sulfur-free tape and freeze-dried, and the distribution of Cd, S, and P in the intact aphids was mapped with a vortex silicon drift detector. The energy of the incident X-rays was 13.5 keV.
2.3.3 Phloem exudation
In a separate experiment, 6-week-old S. alfredii seedlings were treated with nutrient solutions containing 0 or 100 μmol⋅dm⁻³ CdCl2 for 14 days in a growth chamber (LeDian, Shanghai, China) under a 16 h light/8 h dark cycle (25°C/22°C) at 350 μmol m−2 s−2 and 65% relative humidity. Petioles were then cut above the stem, surface sterilized in 50% ethanol and 0.0006% bleach for 10 s, rinsed in sterile 1 mmol⋅dm⁻³ EDTA, and submerged in 2 ml of 1 mmol⋅dm⁻³ EDTA and 50 μg ml−1 ampicillin, as described previously (Chanda et al., 2011; Lim et al., 2020). The purity of the phloem exudate was evaluated based on their glucose (Miller, 1959) and sucrose (Zhang et al., 2009) contents. A subsample of phloem exudates (5.0 ml) was mixed with 2 ml of 2% (w/v) HNO3 to determine Cd, Zn, Ca, and K contents by inductively coupled plasma mass spectrometry.
2.4 Statistical analysis
Data were statistically analyzed using IBM®SPSS®Statistics 25 (IBM, United States). The data on aphid numbers and metal concentrations in plants, aphids, and phloem exudates were analyzed by one-way analysis of variance. Statistical significance was set at p < 0.01. All statistical graphs were plotted using Origin version 2021b (OriginLab, Northampton, MA, United States). XRF mappings were processed using the software package SMAK version 0.34, S-4 (http://www.sams-xrays.com/smak).
3 Results
3.1 The influence of aphids on S. alfredii growth
After the 6-week greenhouse experiment, the Cd-treated S. alfredii plants were still healthy, with very few aphids observed, while the Cd-free S. alfredii were heavily infested by A. fabae and showed low biomass, chlorotic leaves, and wilting plants (Figure 2). In the susceptible open environment, A. fabae in control plants was mainly found on young stems and leaves near the growing tip, as indicated in Figure 2A. Moreover, the sticky honeydew produced by the aphids was visible, along with the resulting black sooty mold that grew on the honeydew and the aphid slough abandoned during its growth (Figure 2B). In contrast, the growing tips of the Cd-treated plants were clean (Figure 2C); however, one or two aphids were sometimes present on the plants. Furthermore, the metal concentrations in the shoots of S. alfredii were as high as 3,211 ± 610 μg g−1 dry weight (Table 1).
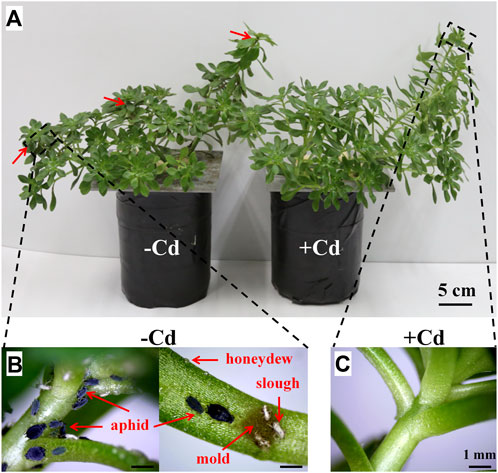
FIGURE 2. Long-term effect of Cd accumulation on the aphid resistance of the Cd hyperaccumulator Sedum alfredii in an open pathogenic environment. (A) Four-week-old seedlings were treated with 0 (-Cd) or 100 μmol⋅dm⁻³ (+Cd) CdCl2 in a greenhouse full of plants infested by Aphis fabae Scopoli (Hemiptera, Aphididae). After 6 weeks, the control (-Cd, left) plants were colonized by aphids, while very few aphids were observed in Cd-treated (+Cd, right) plants. The red arrow indicates the main site of aphid colonies. (B) Symptoms of aphid attack in the growing tip of control plants. (C) Growing tip of Cd-treated plants. Scale bars: A = 5 cm; B and C = 1 mm.
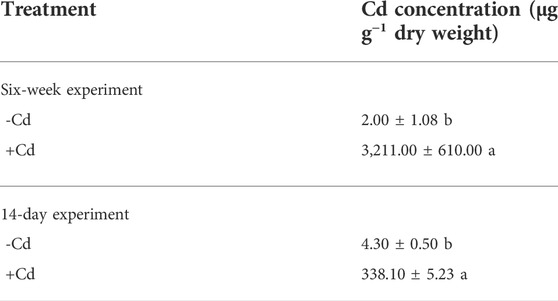
TABLE 1. Cadmium concentration in shoots of S. alfredii seedlings treated with 0 (-Cd) or 100 μmol⋅dm⁻³ (+Cd) CdCl2 at the end of long- (six weeks) and short- (14 days) term aphid infestation experiments. Data represent means ± SD (n = 4). Different letters indicate significant differences between the control and Cd-treated plants (t-test, p < 0.01).
3.2 The effect of Cd on S. alfredii aphid defense
The results showed that S. alfredii pretreated with Cd remained healthy despite being grown in the same closed environment full of plants attacked by aphids (Figure 1). As shown in Figures 1B, D, upon immediate infestation, there was no apparent difference in the number of aphids between S. alfredii with and without Cd treatment. However, the number of aphids in Cd-treated S. alfredii severely decreased to 10 after 7 days of infestation. Furthermore, concomitant with the high level of Cd in the phloem exudate of S. alfredii (Figure 1F), the concentration of Cd in the aphids significantly increased 3-fold (Figures 1C–E). Meanwhile, no glucose (data not shown) but high levels of sucrose were detected in the phloem exudates (Figure 3A). Interestingly, there was no significant difference in the distribution of other elements, such as Ca, K, and Zn, in the phloem exudates of S. alfredii with and without Cd treatment (Figure 3).
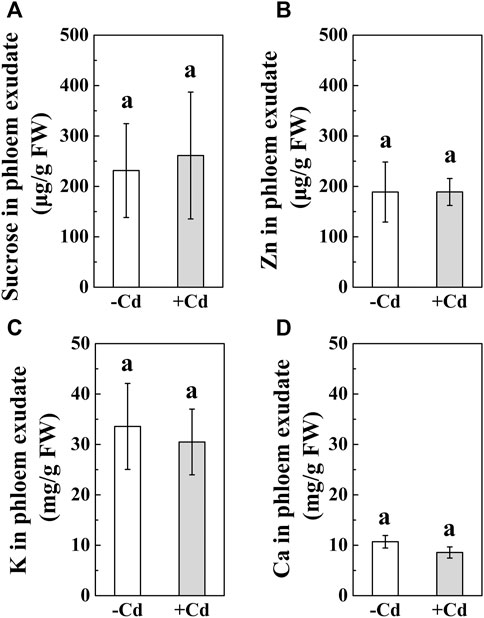
FIGURE 3. Sucrose and elements in the phloem exudates collected from leaves of 6-week-old S. alfredii after being treated with 0 or 100 μmol⋅dm⁻³ CdCl2 for 14 days. (A) Sucrose concentration. (B) Zn concentration. (C) K concentration. (D) Ca concentration. Data are shown as means ± SD (n = 4). Different letters indicate significant differences between the control and Cd-treated plants (t-test, p < 0.01). FW, fresh weight.
3.3 The elemental distribution patterns in the aphid body
Figure 4 shows the micro XRF images with the distribution patterns of Cd in the aphids collected from S. alfredii leaves treated with 100 μmol⋅dm⁻³ Cd. It is noteworthy that although Cd, S, and P were preferentially distributed in the aphids, the distribution pattern for each element was notably different (Figure 4). It is clear that the concentration of Cd in the stylets, the piercing–sucking mouthpart of aphids, was substantially higher than that in other parts of the aphid body. In contrast, S and P were uniformly distributed throughout the aphid body (Figure 4).
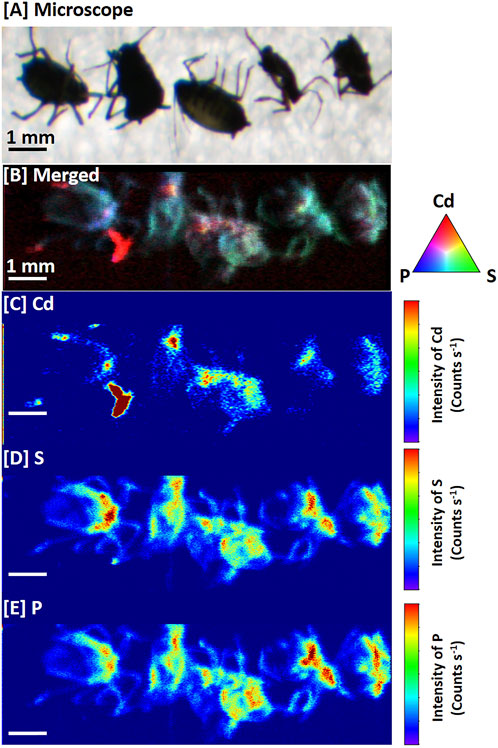
FIGURE 4. Micro X-ray fluorescence images of aphids (Aphis fabae Scopoli). Four-week-old Sedum alfredii seedlings were pretreated with 100 μmol⋅dm⁻³ (+Cd) CdCl2 for 7 days in a small and closed environment. Adult aphids were then introduced into the plants, which were further treated with Cd for another 7 days. (A) Photograph of the typical aphids. (B) Color merge images showing the relative locations of cadmium (Cd; red), phosphorus (P; blue), and sulfur (S; green) in the typical aphids. (C) Cd images in the aphids. (D) S images in the aphids. (E) P images in the aphids.
4 Discussion
4.1 Cd accumulation plays an important role in S. alfredii aphid defense
The protective role of metal hyperaccumulation in plants could be due to the toxicity of metals and their deterrent effects on insect feeding (Morkunas et al., 2018). In this study, we found that the number of aphids that colonized the Cd-pretreated S. alfredii was significantly lower than that in the control plants after 7 days of exposure to 200 aphids (Figure 1D). This is also consistent with the evidence provided in previous studies (Morkunas et al., 2018; Ali et al., 2019; Shi et al., 2020). Interestingly, we still found a few aphids in Cd-treated S. alfredii (Figure 1D), indicating that some insects were unable to immediately adjust their feeding behaviors in response to the food metal composition (Stolpe et al., 2017). This also indicates the bidirectional movement of aphids in detecting Cd (Jhee et al., 2005; Stolpe and Müller, 2016). These results align with the elemental defense hypothesis (Boyd, 2007; Rascio and Navari-Izzo, 2011) since elemental defense is inexpensive and is likely a key mechanism for plant invasion into metal-polluted sites. Heavy metals may change the chemical composition of plants and affect the performance of herbivores (Stolpe et al., 2017). Taking these results together, we conclude that the role of Cd in defending aphids in the hyperaccumulator S. alfredii may not be due to a deterrent effect on aphids.
4.2 Efficient phloem transport significantly enhanced Cd defense ability
In a closed environment experiment, we still found a considerably high Cd concentration in aphids collected from Cd-treated S. alfredii plants (Figure 1E) and many dead aphids below the seedlings (data not shown). We deduced that Cd directly poisoned the aphids and, therefore, prevented their colonization. The connection between the phloem metal concentration and aphid resistance has been proposed for decades (Boyd and Marrten, 1999; Hanson et al., 2004; Stolpe et al., 2017). As Stolpe et al. (2017) showed, Cd in the phloem of A. helleri plays an important role in aphid resistance. However, most heavy metals are stored in the cell vacuoles (Kupper et al., 2000; Rascio and Navari-Izzo, 2011). Previously, we detected a high concentration of Cd in the phloem of S. alfredii by micro XRF (Tian et al., 2016), and Cd was remobilized from mature to developing organs via the phloem (Hu et al., 2019a). In the present study, abundant Cd was also detected in the phloem exudates of S. alfredii (Figure 1F). In contrast to Cd, the concentrations of Ca, K, and Zn were not significantly changed in the phloem. This may partly be due to the characteristics of S. alfredii, which mainly stores a large amount of metals in its cell vacuoles (Tian et al., 2017). In contrast, the non-accumulator species Zea mays (Poaceae) shows reduced K concentration when exposed to high Pb concentrations (Małkowski et al., 2002). Therefore, the high Cd levels found in aphids (Figure 1E) provide strong evidence that high Cd levels in the phloem (or other factors triggered by high Cd) kill the aphids that feed on Cd-treated S. alfredii (Stolpe et al., 2017).
4.3 The unique distribution of Cd in aphids
As phloem-feeding insects, aphids use stylets to obtain nutrition from plant phloem tissues (Will et al., 2013). The stylets are the most direct channel for the plant phloem sap to enter the aphid’s body (Will et al., 2013). There is a highly competitive interaction between Cd and Ca in S. alfredii (Lu et al., 2008; Tian et al., 2011). In our previous research (Tian et al., 2016; Hu et al., 2019a), we found that the S. alfredii phloem has a strong ability to remobilize Cd. The level of Cd in the phloem exudate of S. alfredii leaves was 2- to 50-fold higher than those of other elements (such as P and S), and Ca deficiency triggers phloem remobilization of Cd (Tian et al., 2016). These changes may be strengthened further by aphid saliva (Will et al., 2013; Stolpe et al., 2017). Before ingestion, stylets secrete watery saliva containing proteins that can bind to Ca2+ and regulate the element influx to prevent sieve tube plugging (Will et al., 2007; Carolan et al., 2009; Will et al., 2013). This is strongly supported by our results, as shown in Figure 4, which indicate a large amount of Cd accumulated in the stylets of aphids.
In conclusion, this study reported the effects of Cd accumulation on aphid performance in the Cd hyperaccumulator S. alfredii. The results revealed that Cd or other factors affected by Cd significantly protected S. alfredii plants from aphid infestation, probably by directly poisoning the insects rather than by deterring them. It is likely that the high Cd level in the phloem sap of S. alfredii helps fight against the black bean aphid. Consequently, the current study significantly improves our understanding of the “heavy metal defense” hypothesis to develop strategies for insect resistance and promote heavy metal accumulation in the shoots of these hyperaccumulators, which may contribute to the optimized phytoremediation efficiency.
Data availability statement
The original contributions presented in the study are included in the article/Supplementary Material; further inquiries can be directed to the corresponding author.
Author contributions
LLX, YH, and LLL conceived the ideas and designed the methodology. LLX and YH collected and analyzed the data. LLL and XYL provided supervision, funding, and reagents. LLX and YH analyzed the data and led the writing of the manuscript with comments from LLL and XYL. All authors discussed the results, reviewed the article, and approved the final article.
Funding
This work was supported by projects from the National Natural Science Foundation of China (22276164, 31672235, and 31872167).
Acknowledgments
The authors would also like to thank Editage (www.editage.cn) for English language editing.
Conflict of interest
The authors declare that the research was conducted in the absence of any commercial or financial relationships that could be construed as a potential conflict of interest.
Publisher’s note
All claims expressed in this article are solely those of the authors and do not necessarily represent those of their affiliated organizations, or those of the publisher, the editors, and the reviewers. Any product that may be evaluated in this article, or claim that may be made by its manufacturer, is not guaranteed or endorsed by the publisher.
References
Ali, S., Ullah, M. I., Saeed, M. F., Khalid, S., Saqib, M., Arshad, M., et al. (2019). Heavy metal exposure through artificial diet reduces growth and survival of Spodoptera litura (Lepidoptera: Noctuidae). Environ. Sci. Pollut. Res. 26 (14), 14426–14434. doi:10.1007/s11356-019-04792-0
Boyd, R. S. (2013). Exploring tradeoffs in hyperaccumulator ecology and evolution. New Phytol. 199 (4), 871–872. doi:10.1111/nph.12398
Boyd, R. S., and Martens, S. N. (1999). Aphids are unaffected by the elemental defence of the nickel hyperaccumulator Streptanthus polygaloides (Brassicaceae). Chemoecology 9, 1–7.
Boyd, R. S. (2007). The defense hypothesis of elemental hyperaccumulation: Status, challenges and new directions. Plant Soil 293 (1-2), 153–176. doi:10.1007/s11104-007-9240-6
Butt, A., Qurat ul, A., Rehman, K., Khan, M. X., and Hesselberg, T. (2018). Bioaccumulation of cadmium, lead, and zinc in agriculture-based insect food chains. Environ. Monit. Assess. 190 (12), 698. doi:10.1007/s10661-018-7051-2
Carolan, J. C., Fitzroy, C. I. J., Ashton, P. D., Douglas, A. E., and Wilkinson, T. L. (2009). The secreted salivary proteome of the pea aphid Acyrthosiphon pisum characterised by mass spectrometry. Proteomics 9, 2457–2467. doi:10.1002/pmic.200800692
Chanda, B., Xia, Y., Mandal, M. K., Yu, K., Sekine, K-T., Gao, Q-m., et al. (2011). Glycerol-3-phosphate is a critical mobile inducer of systemic immunity in plants. Nat. Genet. 43 (5), 421–427. doi:10.1038/ng.798
Clemens, S., Aarts, M. G. M., Thomine, S., and Verbruggen, N. (2013). Plant science: The key to preventing slow cadmium poisoning. Trends Plant Sci. 18 (2), 92–99. doi:10.1016/j.tplants.2012.08.003
Dai, Z. C., Cai, H. H., Qi, S. S., Li, J., Zhai, D. L., Wan, J. S. H., et al. (2020). Cadmium hyperaccumulation as an inexpensive metal armor against disease in Crofton weed. Environ. Pollut. 267, 115649. doi:10.1016/j.envpol.2020.115649
Ent, A. V. D., Baker, A. J. M., Reeves, R. D., Pollard, A. J., and Schat, H. (2013). Hyperaccumulators of metal and metalloid trace elements: Facts and fiction. Plant Soil 362, 319–334. doi:10.1007/s11104-012-1287-3
Hanson, B., Lindblom, S. D., Loeffler, M. L., and Eah, P. S. (2004). Selenium protects plants from phloem-feeding aphids due to both deterrence and toxicity. New Phytol. 162, 655–662. doi:10.1111/j.1469-8137.2004.01067.x
He, S., Yang, X., He, Z., and Baligar, V. C. (2017). Morphological and physiological responses of plants to cadmium toxicity: A review. Pedosphere 27 (3), 421–438. doi:10.1016/s1002-0160(17)60339-4
Hu, Y., Tian, S., Foyer, C. H., Hou, D., Wang, H., Zhou, W., et al. (2019a). Efficient phloem transport significantly remobilizes cadmium from old to young organs in a hyperaccumulator Sedum alfredii. J. Hazard. Mater. 365, 421–429. doi:10.1016/j.jhazmat.2018.11.034
Hu, Y., Xu, L., Tian, S., Lu, L., and Lin, X. (2019b). Site-specific regulation of transcriptional responses to cadmium stress in the hyperaccumulator, Sedum alfredii: Based on stem parenchymal and vascular cells. Plant Mol. Biol. 99 (4-5), 347–362. doi:10.1007/s11103-019-00821-1
Irfan Naikoo, M., Ahmad Khan, F., Ahmed, N., Rinklebe, J., Sonne, C., Nishanta, R., et al. (2021a). Biotransfer, bioaccumulation and detoxification of nickel along the soil - faba bean - aphid - ladybird food chain. Sci. Total Environ. 785, 147226. doi:10.1016/j.scitotenv.2021.147226
Irfan Naikoo, M., Fariha, R., Irfan Dar, M., Ahmad Khan, F., Kamel, H., and Parvaiz, A. (2021b). Uptake, accumulation and elimination of cadmium in a soil - faba bean (Vicia faba) - aphid (Aphis fabae) - ladybird (Coccinella transversalis) food chain. Chemosphere 279, 130522. doi:10.1016/j.chemosphere.2021.130522
Jhee, E. M., Boyd, R. S., and Eubanks, M. D. (2005). Nickel hyperaccumulation as an elemental defense of Streptanthus polygaloides (brassicaceae): Influence of herbivore feeding mode. New Phytol. 168 (2), 331–344. doi:10.1111/j.1469-8137.2005.01504.x
Konopka, J. K., Hanyu, K., Macfie, S. M., and Mcneil, J. N. (2013). Does the response of insect herbivores to cadmium depend on their feeding strategy? J. Chem. Ecol. 39, 546–554. doi:10.1007/s10886-013-0273-4
Kraemer, U. (2010). “Metal hyperaccumulation in plants,” in Annual review of plant biology. Editors S. Merchant, W. R. Briggs, and D. Ort, 61, 517–534.
Kupper, H., Lombi, E., Zhao, F. J., and McGrath, S. P. (2000). Cellular compartmentation of cadmium and zinc in relation to other elements in the hyperaccumulator Arabidopsis halleri. Planta 212 (1), 75–84. doi:10.1007/s004250000366
Lim, G-H., Liu, H., Yu, K., Liu, R., Shine, M. B., Fernandez, J., et al. (2020). The plant cuticle regulates apoplastic transport of salicylic acid during systemic acquired resistance. Sci. Adv. 6 (19), eaaz0478. doi:10.1126/sciadv.aaz0478
Lin, T. T., Chen, J. P., Zhou, S. S., Yu, W. H., Chen, G., Chen, L. H., et al. (2020). Testing the elemental defense hypothesis with a woody plant species: Cadmium accumulation protects Populus yunnanensis from leaf herbivory and pathogen infection. Chemosphere 247, 125851. doi:10.1016/j.chemosphere.2020.125851
Liu, Z., Sun, Z. Z., Zeng, C. Z., Dong, X. J., Li, M., Liu, Z. X., et al. (2022). The elemental defense effect of cadmium on Alternaria brassicicola in Brassica juncea. BMC Plant Biol. 22 (1), 17. doi:10.1186/s12870-021-03398-4
Lu, L. L., Tian, S. K., Yang, X. E., Wang, X. C., Brown, P., Li, T. Q., et al. (2008). Enhanced root-to-shoot translocation of cadmium in the hyperaccumulating ecotype of Sedum alfredii. J. Exp. Bot. 59, 3203–3213. doi:10.1093/jxb/ern174
Małkowski, E., Kita, A., Galas, W., Karcz, W., and Kuperberg, J. M. (2002). Lead distribution in corn seedlings (Zea mays L.) and its effect on growth and the concentrations of potassium and calcium. Plant Growth Regul. 37, 69–76. doi:10.1023/a:1020305400324
Miller, G. L. (1959). Use of dinitrosalicylic acid reagent for determination of reducing sugar. Anal. Chem. 31, 426–428. doi:10.1021/ac60147a030
Morkunas, I., Wozniak, A., Van Chung, M., Rucinska-Sobkowiak, R., and Jeandet, P. (2018). The role of heavy metals in plant response to biotic stress. Molecules 23 (9), 2320. doi:10.3390/molecules23092320
Navariizzo, F. (2010). Heavy metal hyperaccumulating plants: How and why do they do it? And what makes them so interesting? Plant Sci. 180, 169–181. doi:10.1016/j.plantsci.2010.08.016
Rascio, N., and Navari-Izzo, F. (2011). Heavy metal hyperaccumulating plants: How and why do they do it? And what makes them so interesting? Plant Sci. 180 (2), 169–181. doi:10.1016/j.plantsci.2010.08.016
Rubina, K., Anjani, K., Nayak, A. K., Shahid, Md, Rahul, T., Vijayakumar, S., et al. (2020). Metal(loid)s (as, Hg, Se, Pb and Cd) in paddy soil: Bioavailability and potential risk to human health. Sci. Total Environ. 699, 134330. doi:10.1016/j.scitotenv.2019.134330
Shi, Z., Wang, S., Pan, B., Liu, Y., Li, Y., Wang, S., et al. (2020). Effects of zinc acquired through the plant-aphid-ladybug food chain on the growth, development and fertility of Harmonia axyridis. Chemosphere 259, 127497. doi:10.1016/j.chemosphere.2020.127497
Shimizu-Inatsugi, R., Milosavljevic, S., Shimizu, K. K., Schaepman-Strub, G., Tanoi, K., and Sato, Y. (2021). Metal accumulation and its effect on leaf herbivory in an allopolyploid species Arabidopsis kamchatica inherited from a diploid hyperaccumulator A. halleri. Plant Species Biol. 36 (2), 208–217. doi:10.1111/1442-1984.12304
Stolpe, C., Krämer, U., and Müller, C. (2017). Heavy metal (hyper)accumulation in leaves of Arabidopsis halleri is accompanied by a reduced performance of herbivores and shifts in leaf glucosinolate and element concentrations. Environ. Exp. Bot. 133, 78–86. doi:10.1016/j.envexpbot.2016.10.003
Stolpe, C., and Müller, C. (2016). Effects of single and combined heavy metals and their chelators on aphid performance and preferences. Environ. Toxicol. Chem. 35, 3023–3030. doi:10.1002/etc.3489
Tewes, L. J., Stolpe, C., Kerim, A., Kraemer, U., and Mueller, C. (2018). Metal hyperaccumulation in the Brassicaceae species Arabidopsis halleri reduces camalexin induction after fungal pathogen attack. Environ. Exp. Bot. 153, 120–126. doi:10.1016/j.envexpbot.2018.05.015
Tian, S. K., Lu, L. L., Yang, X. O., Webb, S. M., Du, Y. H., and Brown, P. H. (2010). Spatial imaging and speciation of lead in the accumulator plant Sedum alfredii by microscopically focused synchrotron X-ray investigation. Environ. Sci. Technol. 44 (15), 5920–5926. doi:10.1021/es903921t
Tian, S. K., Lu, L. L., Labavitch, J., Yang, X. E., He, Z. L., Hu, H. N., et al. (2011). Cellular sequestration of cadmium in the hyperaccumulator plant species Sedum alfredii. Plant Physiol. 157, 1914–1925. doi:10.1104/pp.111.183947
Tian, S. K., Xie, R. H., Wang, H. X., Hu, Y., Ge, J., Liao, X. C., et al. (2016). Calcium deficiency triggers phloem remobilization of cadmium in a hyper-accumulating species. Plant Physiol. 172, 2300–2313. doi:10.1104/pp.16.01348
Tian, S. K., Xie, R. H., Wang, H. X., Hu, Y., Hou, D. D., Liao, X. C., et al. (2017). Uptake, sequestration and tolerance of cadmium at cellular levels in the hyperaccumulator plant species Sedum alfredii. J. Exp. Bot. 68, 2387–2398. doi:10.1093/jxb/erx112
Verbruggen, N., Juraniec, M., Baliardini, C., and Meyer, C-L. (2013). Tolerance to cadmium in plants: The special case of hyperaccumulators. Biometals 26 (4), 633–638. doi:10.1007/s10534-013-9659-6
Wen-Li, Z., Yu, D., Miao-Miao, Z., and Qi, S. (2014). Cadmium exposure and its health effects: A 19-year follow-up study of a polluted area in China. Sci. Total Environ. 470-471, 224–228. doi:10.1016/j.scitotenv.2013.09.070
Will, T., Tjallingii, W. F., Thonnessen, A., and van Bel, A. J. E. (2007). Molecular sabotage of plant defense by aphid saliva. Proc. Natl. Acad. Sci. U. S. A. 104 (25), 10536–10541. doi:10.1073/pnas.0703535104
Will, T., Furch, A. C. U., and Zimmermann, M. R. (2013). How phloem-feeding insects face the challenge of phloem-located defenses. Front. Plant Sci. 4, 336. doi:10.3389/fpls.2013.00336
Yang, X. E., Long, X. X., Ye, H. B., He, Z. L., Calvert, D. V., and Stoffella, P. J. (2004). Cadmium tolerance and hyperaccumulation in a new Zn-hyperaccumulating plant species (Sedum alfredii Hance). Plant Soil 259, 181–189. doi:10.1023/b:plso.0000020956.24027.f2
Zhang, Z. L., Qu, W. J., and Li, X. F. (2009). Plant physiology experimental guide. 4th edition. Beijing, China: China Higher Education Press, 106.
Keywords: hyperaccumulation, insect, Aphis fabae, phloem, elemental defense, heavy metal
Citation: Xu L, Lu L, Lin X and Hu Y (2022) Cadmium armors the Cd hyperaccumulator Sedum alfredii against aphid attack. Front. Environ. Sci. 10:1036708. doi: 10.3389/fenvs.2022.1036708
Received: 05 September 2022; Accepted: 05 October 2022;
Published: 19 October 2022.
Edited by:
Meihua Deng, Zhejiang Academy of Agricultural Sciences, ChinaReviewed by:
Yiquan Ye, Fujian Agriculture and Forestry University, ChinaKai Wang, Ningbo University, China
Copyright © 2022 Xu, Lu, Lin and Hu. This is an open-access article distributed under the terms of the Creative Commons Attribution License (CC BY). The use, distribution or reproduction in other forums is permitted, provided the original author(s) and the copyright owner(s) are credited and that the original publication in this journal is cited, in accordance with accepted academic practice. No use, distribution or reproduction is permitted which does not comply with these terms.
*Correspondence: Yan Hu, aHV5YW5AYWdyaS5nb3YuY24=