Corrigendum: Fertile island effect by three typical woody plants on wetlands of Ebinur Lake, northwestern China
- 1Key Laboratory of Oasis Ecology, College of the Ecology and Environment, Ministry of Education, Xinjiang University, Urumqi, China
- 2Student Work Department, Tarim University, Aral, China
Desertification poses a permanent threat to the security of arid ecosystems. Perennial arid vegetation plays a crucial role in maintaining the structure and function of arid ecosystems and slowing the process of desertification by forming “fertile islands” under the tree canopy. However, the process of formation and development of these fertile islands remains uncertain. Here, we explored how three typical woody plants (i.e., Populus euphratica, Haloxylon ammodendron, and Nitraria tangutorum) in the Ebinur Lake Basin of northwestern China differed in their soil nitrogen and phosphorus. 1) Significant differences of organic carbon and total and available nitrogen/phosphorus were observed in the soil among the three typical woody plant-dominated ecosystems. Populus euphratica had significant differences of N and P contents between the canopy and bare soils, except for ammonium nitrogen. 2) Our RDA analysis revealed that the major factors that influenced the soil nutrient differences among the three vegetations were plant crown width, soil water content, salinity, and pH. 3) The organic carbon content of bare soil was significantly correlated with N and P in all the three vegetations. This study contributes to our understanding of the factors that influence the fertile island effect in arid ecosystems, which may contribute to soil conservation in arid areas.
Introduction
Soil and plants are important components of terrestrial ecosystems. The physicochemical properties of soil affect the growth and development of plants (Zhang et al., 2019). Plants improve the physicochemical properties of soil through the rhizosphere (Chen et al., 2022) and litter decomposition (Jiang et al., 2020), both of which affect each other through various feedbacks. The rhizosphere/litter decomposition-influence mechanism is more obvious in arid regions (Mora & Lazaro. 2013; Yang et al., 2015). Around perennial plants, due to the combined action of biological and abiotic processes, soil nutrients gradually flow into the canopy, wheretheir contents are significantly higher than those outside thecanopy, which is evidence of a nutrient-accumulation phenomenon in the spatial distribution that is called the ‘fertile island’ effect (Chen et al., 2004). Formation of the fertile island effect can promote the growth of plant roots (Zhao et al., 2002) and increase the contents of nutrients and water around plants, thereby improving their survival rates, which is important for renewal and diffusion of plants (Fuhlendorf et al., 2001). The fertile island effect is also the main mechanism by which plants use nutrients and adapt to arid and barren environments.
Scholars in China and abroad have conducted many studies on the effects of plant fertilizer island. The formation of global dry land fertile islands depends, to a large extent, on the local climate, topography, soil characteristics, structure, and properties of plant communities and soil microbial communities (Ochoa-Hues et al., 2017). Researchers used biological and abiotic data on a wide range of drought gradients in eastern Australia to show that the ‘fertile island’ effect increases with increasing drought levels(Ding and Eldridge, 2020). In a study on soil properties at three different altitudes in the Qilian Mountains, researchers showed that with the increase in altitude, the soil nutrition and microbial-biomass parameters mostly decreased, and the ‘fertile island’ effect increased(Zhao and An, 2021). In woody plants and shrub communities, such as Haloxylon ammodendron, Tamarix ramosissima, and Populus euphratica, the nutrient enrichment in the topsoil under the canopies was obvious, and the ‘fertile island’ effect in the upper soil layer was greater than that in the lower soil layer (Reynolds et al., 1999; Chen et al., 2018; Guo et al., 2020; Bomans et al., 2021). Researchers investigated the soil moisture, nutrient content, and fine root density, and they found that the interaction between plants and soil was important for the formation and development of fertile islands and that its influence was not only related to the soil surface but also extended to the depth of the root contact (Cao et al., 2021). Researchers studied the soil nutrient content and microbial community under the canopy of a plant community in the southeast of the Tengger Desert in northwestern China, and they found that the bacterial community was significantly related to the spatial heterogeneity of some of the physical and chemical properties of the soil. The establishment of fertile islands in a desert ecosystem formed soil bacterial communities, which affected the soil characteristics, and the soil was then fed back to the plants, which affected plant growth and development (Li et al., 2021).
However, the focus of these studies is the difference in ‘fertile island’ effect in different soil depths or different scales or differences in the fat island effect between plants. Few researchers have focused on the effects of plants themselves or soil conditions on the ‘fertile island’ effect. Therefore, in this study, we analyzed the soil nutrient contents of typical woody plants in arid areas around the Aqikesu River in the Ebinur Lake Basin. The soil nutrient contents under the canopy and bare soil were compared and analyzed, and the following problems were proposed: 1) does the soil under a typical woody plant canopy in the arid area have a ‘fertile island’ effect? 2) Are there significant differences in different plant nutrient ‘fertile island’ effect? 3) What factors affect the ‘fertile island’ effect?
Methods
Overview of the study area
The Ebinur Lake Wetland National Nature Reserve (79°53 ′-85°02 ′ E and 43°38 ′-45°52 ′ N) is located in the Xinjiang Uygur Autonomous Region. The basin has the continental climate of the northern temperate zone. The climate varies substantially over the four seasons. Rainfall in summer is scarce, and winter is cold and dry. The annual average temperature is 6.6–7.8°C. The annual maximum temperature can reach 44°C, and the minimum temperature can reach 33°C below zero. The annual rainfall is less than 100 mm, and the annual gale weather is 165 days (winds greater than 17 m/s). The typical soil types in the study area are mainly gray desert soil, aeolian sandy soil, gray–brown desert soil, meadow soil, and marsh soil, and the soil in the hidden area is mainly saline soil (Wang et al., 2019; Wang. 2020). The main plants in the study area are Populus euphratica, Haloxylon ammodendron, Suaeda glauca, and Kalidium foliatum (Yang et al., 2009).
Sample setting and sample collection
This study is drawn on the results of soil moisture and salinity surveys in previous studies, and we set a transect vertically along the banks of the Achixu River. The transect is about 500 m from the river channel. In the sample belt, the study arranged 30 × 30 m quadrats at 30 m intervals, with a total of 60 quadrats. In addition, the study arranged sample plots about 1 km away from the sample belt, and the study divided the 480 × 600 m sample plots into 320 quadrats of 30 × 30 m. From these 320 quadrats, the study selected the quadrats according to the chessboard interval, for a total of 80 quadrats of 30 × 30 m. The study included a total of 140 quadrats in the transect and plot. Plant quadrat surveys and soil sampling were carried out for each selected quadrat. In the determined standard quadrat, the study selected the soil sampling points by the “diagonal” sampling method, and the study took three portions of soil (three replicates) of 0–10 cm for each quadrat. The study used aluminum boxes to collect the soil samples (the study weighed the quality of the aluminum boxes in advance). After the study completed the aluminum-box collection of the soil, the study numbered the numbers, immediately weighed the fresh soil, and then brought it back to the laboratory. The study sampled each quadrat by species for crown soil sampling (sampling as close as possible to the root) and randomly selected three plants for each plant (less than three plants, similar plants with the same growth in adjacent quadrats). The study selected three to five sampling points under the canopy and gently shoveled the uppermost layer of litter with a small shovel when sampling. Then, soil samples were collected at 0–10 cm depth at the identified sample sites, and three plants were selected for each species. Finally, the researchers mixed the soil collected under the crowns of the three plants. After collecting the soil under the crown, the researcher collected the bare soil of the corresponding quadrat and put the number into a self-sealing bag. After taking the soil samples back to the laboratory, they were naturally air-dried and pretreated, and the corresponding indexes were measured in the later stage.
Determination items and methods
To measure the ‘fertile island’ effect, the study measured seven indicators of the soil function related to C, N, and P cycles: organic carbon, total nitrogen, nitrate nitrogen, ammonium nitrogen, alkali-hydrolyzable nitrogen, total phosphorus, and available phosphorus. The study outlines the experimental method as Table 1.
Data processing in the study
The patch distribution pattern of the arid desert ecosystem is strongly affected by vegetation coverage, so the study defined the nutrient-accumulation effect as the relative difference between the plant canopy and the bare area without plant growth (Allington and Valone. 2014). Armas used the relative interaction index (RII) to estimate the accumulation-effect value of each soil index (Armas et al., 2004). The formula of the relative interaction index (RII) is as follows:
In the aforementioned formula, X is the target variable and Xp and Xn are the soil index values of the canopy soil and the bare soil, respectively.
The study used the average method to calculate the nutrient accumulation effect value of each plant (Bowker et al., 2013). The average method of calculating the formula is as follows:
In the aforementioned formula, MRII represents the nutrient accumulation effect, fi represents the accumulation effect value of different nutrients, ri is a mathematical function that converts fi into a positive value, g represents the standardization of all measured values, and F represents the number of measured nutrients.
The study used a paired t-test to analyze the differences in soil nutrient properties between the canopy soil and bare soil of Populus euphratica, Haloxylon ammodendron, and Nitraria tangutorum and the analysis of variance to analyze these differences. If the results were obvious, then the study used the least squares difference (LSD) method to conduct the difference test for pairwise comparison of the soil nutrient contents in different habitats. The study used SPSS25 (Statistical Product and Service Solutions) (IBM, New York, NY, United States ) to complete the entire statistical analysis and used Origin2022 and Canoco5 (Copyright Petr Šmilauer 2012-2021) to complete the drawing.
Results and analysis
‘Fertile island’ effect of typical woody plants
The study selected typical desert woody plants from all the plants in the survey area: Populus euphratica, Haloxylon ammodendron, and Nitraria tangutorum and analyzed their ‘fertile island’ effect. In Table 2, we can see that the nutrient contents of the soil under the Populus euphratica canopy were significantly different from those of the bare soil, except for ammonium nitrogen. The nutrient contents of TN, TP, AP, and NO3−-N in the soil under the Haloxylon ammodendron canopy were significantly different from those in the bare soil, while the other nutrient contents had no significant differences. The nutrient contents of the soil organic carbon, TN, AP, and NO3−-N under the Nitraria tangutorum canopy were significantly different from those in the bare soil, and there was no significant difference in the nutrient contents. The contents of the soil organic carbon, TN, and TP under Populus euphratica were significantly different from those under Haloxylon ammodendron, and the contents of the soil SOC, TN, and TP under Haloxylon ammodendron were significantly different from those under Nitraria tangutorum. The soil AP content under the Haloxylon ammodendron canopy was significantly different from that under Nitraria tangutorum. Populus euphratica had ‘fertile island’ effect on nutrients other than NH4+-N.
Factor analysis of fertile island effect in typical woody plants
As shown in Figure 1, the ‘TP-fertile island’ effect of Populus euphratica was significantly negatively correlated with the soil salt content of the Populus euphratica canopy (r = -0.37 and -0.38). The ‘AP-fertile island’ effect was significantly negatively correlated with the soil water content, pH value, and salt content (r = -0.41, -0.43, and -0.48), and the ‘NO3−-N-fertile island’ effect was significantly negatively correlated with the soil pH value and salt content (r = -0.46 and -0.49). There was no significant correlation between the ‘fertile island’ effect and the plant crown width. The ‘fertile island’ effect of SOC and TN was significantly positively correlated (r = 0.83), indicating a highly consistent accumulation process and SOC and TN in Populus euphratica. The ‘fertile island’ effect of SOC was significantly positively correlated with the ‘fertile island’ effect of TP, AP, and alkali-hydrolyzable nitrogen (r = 0.43, 0.40, and 0.41), which indicates that the accumulation of SOC affected the accumulation processes of phosphorus and nitrogen to a certain extent. In addition to DBH and soil salt content, the plant crown width, height, DBH, soil water content, pH, and salt content of Populus euphratica were significantly positively correlated.
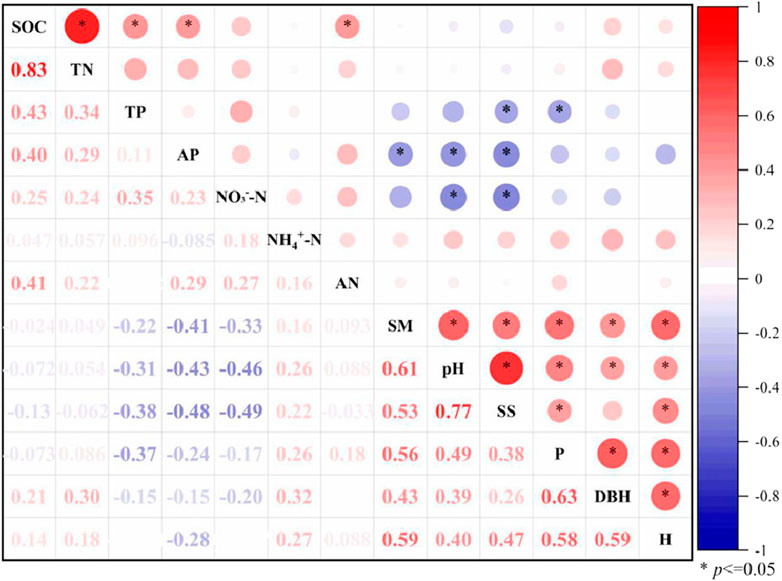
FIGURE 1. Correlation analysis of factors that influence the ‘fertile island’ effect of different nutrient contents in Populus euphratica.
The ‘TP-fertile island effect’ of Haloxylon ammodendron was significantly positively correlated with the plant height of Haloxylon ammodendron (r = 0.39), the ‘AP-fertile island’ effect was significantly negatively correlated with the soil pH and salinity (r = -0.45 and -0.44), and the ‘alkali-hydrolyzable-nitrogen fertile island’ effect was significantly negatively correlated with the soil pH (r = -0.53). There were no significant correlations between the ‘fertile island’ effect and the plant crown width or other influencing factors. The ‘fertile island’ effect of the SOC and TN was significantly positively correlated (r = 0.90). The SOC, TN, TP, and AP-fertile island effect of the ‘NO3−-N-fertile island’ effect are significantly positively correlated (r = 0.40, 0.39, 0.40, and 0.46). The Haloxylon ammodendron crown width was significantly positively correlated with soil pH and salinity. The height of Haloxylon ammodendron is significantly correlated with soil salinity. There was a significant positive correlation between the soil water content, salt content, and pH (Figure 2).
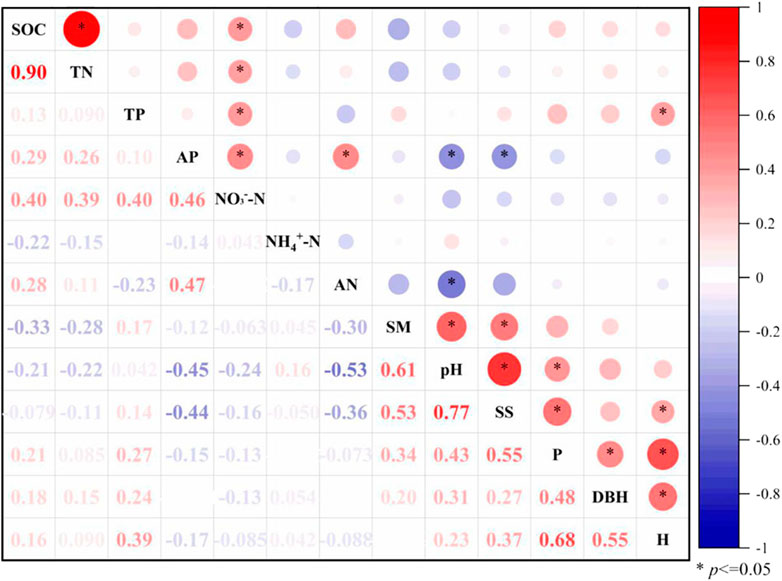
FIGURE 2. Correlation analysis of influencing factors of the ‘fertile island’ effect of different nutrient contents in Haloxylon ammodendron.
The ‘TP-fertile island’ effect of Nitraria tangutorum was significantly positively correlated with the crown width and basal diameter of Nitraria tangutorum (r = 0.43 and 0.47), the ‘AP-fertile island’ effect was significantly negatively correlated with the crown width of Nitraria tangutorum (r = -0.39), and the ‘NO3−-N-fertile island’ effect was significantly negatively correlated with the soil water content and pH (r = -0.44 and -0.47). No significant correlation was observed between the nutrient-fertile island effect and the crown width. The ‘fertile island’ effect of SOC and TN was significantly positively correlated (r = 0.91). The ‘NH4+-N-fertile island’ effect was significantly negatively correlated with the ‘TP-fertile island’ effect (r = -0.51). The crown width was positively correlated with the soil water content, pH, and salinity. There was a significant positive correlation between the soil water content, salt content, and pH (Figure 3).
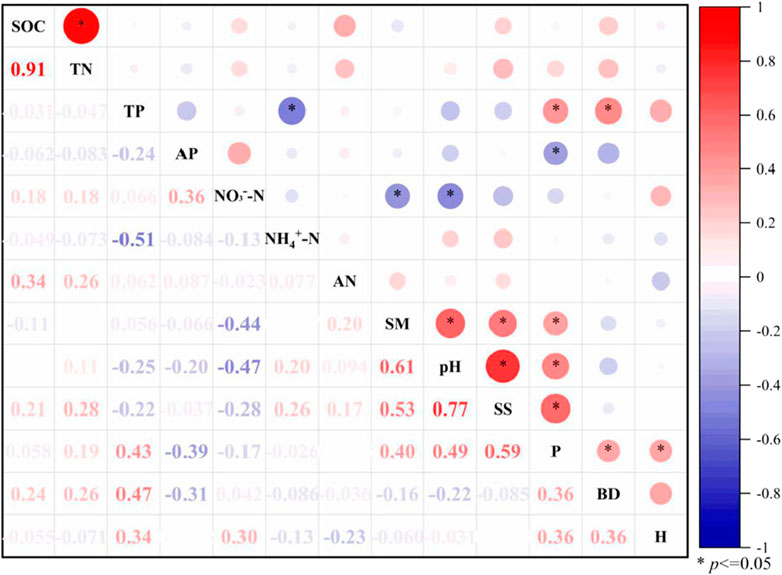
FIGURE 3. Correlation analysis of influencing factors of the ‘fertile island’ effect of different nutrient contents in Nitraria tangutorum.
Relationship between overall plant nutrient fertile island effect and soil physicochemical properties of bare soil
The analysis of RDA results is shown in Figure 4. The first axis explained 53.84% of the relationship between soil physical and chemical factors and the overall nutrient fertile island effect of plants, and the second axis explained 84.78%. The highest correlation in the first ordination axis was mainly soil NO3−-N and soil water content, and the highest correlation in the second axis was soil salt content and AN.
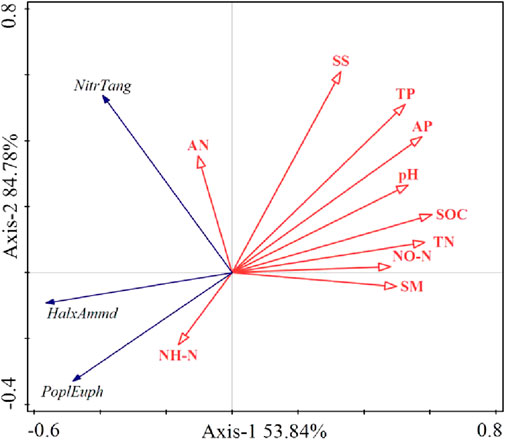
FIGURE 4. Redundancy analysis based on the correlation between soil physico-chemical properties and the overall plant fertile island effect.
This shows that for different species, the first axis mainly reflects the changes in the overall nutrient ‘fertile island’ effect of plants in soil nitrate nitrogen, water content, and other factors, while the second axis mainly reflects the changes in salt content, alkali-hydrolyzable nitrogen, and other factors. Combining the first two axes, nitrate nitrogen, water content, salt content, and alkali-hydrolyzable nitrogen in bare soil are all soil physical and chemical factors that affect the overall nutrient fertile island effect of plants. In addition, as shown in Supplementary Table S1, the SOC content of bare soil is significantly correlated with the overall nutrient fertile island effect of the three species (p = 0.014). This shows that this environmental factor is the main factor affecting the overall nutrient fertile island effect of species.
Discussion
Differences in the fertile island effect of typical woody plants and their possible influencing mechanisms
In this study, in addition to the TP, we found significant differences in the contents of the SOC, TN, AP, and NO3−-N in the soil under the canopies and in the bare soil of typical woody plants in arid areas, which was evidence of the ‘fertile island’ effect in the canopy, which is consistent with the related research (Liu et al., 2016; Cha et al., 2017). Populus euphratica had a ‘fertile island’ effect for each nutrient, and the contents of the soil SOC and TN under the canopy were higher than those of Haloxylon ammodendron and Nitraria tangutorum, which is consistent with the research results of Guo et al. (Guo et al., 2020). However, for the other nutrients, we found the highest soil nutrient contents under the crown. On one hand, different plants have different utilizations of soil nutrients in the growth process (Chen et al., 2013; Wang et al., 2020). On the other hand, the biomass and decomposition rates of different plant litters were different (Yang et al., 2012), so the effects of different plants on the soil nutrients were different.
In this study, the plant biological factors and soil physical factors affected the ‘fertile island’ effect of the TP and AP in the three plants. The reason might be that phosphorus was a limiting factor for plant growth in the study area (Zhang et al., 2019). The main factors that affected the ‘fertile island’ effect of Populus euphratica and Nitraria tangutorum were crown width, soil water content, salinity, and pH. The factors that affected the ‘fertile island’ effect of Haloxylon ammodendron were plant height, soil water content, salinity, and pH. The reason for the effect of the canopy width on the ‘fertile island’ effect might be that the growth of plants under larger canopies and woody canopies increases the threshold wind speed. Therefore, the wind deposits atmospheric dust and windblown sediments from the gaps around plants, which further strengthens the ‘fertile island’ effect (Yan et al., 2019). Soil water content affects the ‘fertile island’ effect due to the amount of water content in the soil affecting the content of hydrolase in the soil, which thereby affects the soil nutrient-cycling process, which results in differences in the soil nutrient contents (Zhang et al., 2022). Soil salinization is a common phenomenon in arid areas. Salinity affects the ‘fertile island’ effect due to salinity and alkaline stress affecting the contents and types of microorganisms and enzymes in the soil, which results in differences in the soil nutrient contents (Cao et al., 2015; Luo et al., 2017).
The main factors that affect the ‘fertile island’ effect of plants and their possible influencing modes
According to the results, biological factors, such as plant crown width and height, were significantly correlated with soil water content, pH, and salinity. Therefore, we can infer that the formation of the fertile island effect is because of the continuous increase in the biomass during plant growth affecting the animal action around plants, which results in the concentrated distribution of animal action near plants, which is convenient for feeding and avoiding light. These action processes increase the litter, soil microorganisms, and surface residues in the soil under the canopies of plants and affect the physical and chemical properties (Zhong et al., 2017; Li et al., 2018). Researchers have found highly consistent processes and effects of the accumulation of SOC and TN in Populus euphratica, Haloxylon ammodendron, and Nitraria tangutorum. The accumulation of SOC in Populus euphratica and Haloxylon ammodendron affected the accumulation of phosphorus and nitrogen to a certain extent, which was consistent with the research of Cao et al. (Cao et al., 2016).
Analysis of influencing factors of plant overall nutrient fertile island effect
This study showed that the bare soil SOC was the main factor that affected the overall fertile island effect. Previous studies have shown that the main reason for the accumulation of nutrients under the canopy of plants was the transport of surface soil materials from close distances (C, N, P, and K) (Qu et al., 2015).
However, due to the insufficient collection of influencing factors in this study, we could only predict a part of the fertile island effect. The effects of abiotic factors on the fertile island effect in plants are yet to be studied, especially the effects of biological factors on the fertile island effect. Abiotic processes were insufficient to promote the continuous development of the fertile island, so plant biological processes have more important effects on the fertile island effect (Li et al., 2017). Plant biological processes mainly include animal and microbial processes. The rhizosphere region contains highly diversified microbial communities, and the number of genes is far greater than that of plant genes, which is one of the most complex ecological regions (Mendes et al., 2013). Researchers have found many microorganisms in the rhizosphere, such as bacteria, fungi, oomycetes, nematodes, protozoa, algae, viruses, and archaea (Bonkowski et al., 2009; Buée et al., 2009). In the rhizosphere region, on one hand, plant root exudates attract all kinds of microorganisms to construct rhizosphere microbial groups, and on the other hand, microorganisms regulate the interaction between plants and the environment through various activities, promote plant growth, accelerate the process of plant restoration, and thus improve the ecological environment (Ying, 2019). Therefore, studying microbial and animal processes, and quantifying them into indicators, is important for a more accurate prediction of the plant fertile island effect.
Conclusion
The results showed that soil nutrients under the canopy of Populus euphratica, Haloxylon ammodendron, and Nitraria tangutorum were significantly higher than those under the bare soil, which proved a fertile island effect exists in the canopy of plants.
Among them, Populus euphratica had a higher fertile island effect than Haloxylon ammodendron and Nitraria tangutorum in terms of nutrient content and enrichment rate. Soil water content, salt content, soil pH, and plant crown width were the main factors affecting the nutrient fertile island effect. The influencing factor of the overall nutrient fertile island effect of the three plants is the SOC content in the bare soil. Therefore, in the process of ecological environment protection in arid areas, maintaining the habitat of trees and shrubs can effectively improve soil fertility, slow down desertification in arid areas, and maintain the stability of the ecosystem.
Data availability statement
The original contributions presented in the study are included in the article/Supplementary Material. Further inquiries can be directed to the corresponding authors.
Author contributions
YZ was responsible for article writing and data analysis. QY, YC, and HW were responsible for field investigation and indoor experiment. GL and HW revised the article later.
Conflict of interest
The authors declare that the research was conducted in the absence of any commercial or financial relationships that could be construed as a potential conflict of interest.
Publisher’s note
All claims expressed in this article are solely those of the authors and do not necessarily represent those of their affiliated organizations, or those of the publisher, the editors, and the reviewers. Any product that may be evaluated in this article, or claim that may be made by its manufacturer, is not guaranteed or endorsed by the publisher.
Supplementary material
The Supplementary Material for this article can be found online at: https://www.frontiersin.org/articles/10.3389/fenvs.2022.1034077/full#supplementary-material
References
Allington, G., and Valone, T. (2014). Islands of fertility: A byproduct of grazing? Ecosystems 17 (1), 127–141. doi:10.1007/s10021-013-9711-y
Armas, C., Ordiales, R., and Pugnaire, F. I. (2004). Measuring plant interactions: A new comparative index. Ecology 85 (10), 2682–2686. doi:10.1890/03-0650
Bomans, A., Cook, A., and Hartley, S., (2021). Bioacoustic monitoring of lower North Island bird communities before and after aerial application of 1080 N. Z. J. Ecol. 45. 3435. doi:10.20417/NZJECOL.45.2
Bonkowski, V, Villenave, C., and Griffiths, B. (2009). Rhizosphere fauna: The functional and structural diversity of intimate interactions of soil fauna with plant roots. Plant Soil 321 (1-2), 213–233. doi:10.1007/s11104-009-0013-2
Bowker, M. A., Maestre, F. T., and Mau, R. L. (2013). Diversity and patch-size distributions of biological soil crusts regulate dryland ecosystem multifunctionality. Ecosystems 16 (6), 923–933. doi:10.1007/s10021-013-9644-5
Buée, M., Boer, W. D., Overbeek, L. V., et al. (2009). The rhizosphere zoo: An overview of plant-associated communities of microorganisms, including phages, bacteria, archaea, and fungi, and some of their structuring factors. Plant & Soil 321 (1-2), 189–212. doi:10.1007/s11104-009-9991-3
Cao, Q., Cao, H. S., Wei, X. L., Karmous, C., and Amara, H. S. (2015). Effects of salt stress on soil microbial biomass carbon and nitrogen and enzyme activity. J. Soil Water Conservation 9 (04), 300–304. doi:10.13870/j.cnki.stbcxb.2015.04.054
Cao, Y. F., Ding, J. X., Yu, Y. J., and Shi, J. (2016). Preliminary study on desert shrub Haloxylon ammodendron 'Feidao' in different texture soils. Soil Sci. 53 (1), 261–270. doi:10.11766/trxb201508130326
Cao, Yf, Gang, H. B., Yan, L. C., and Li, Y. (2021). Soil texture modulates the intensity of fertile islands through affecting the distribution of shrub fine roots. J. Arid Environ. 189, 104503. doi:10.1016/J.JARIDENV.2021.104503
Cha, X. H., Lin, N., Wang, J., and Chen, I. (2017). Nutrient characteristics analysis of ‘Feidao’ in the oasis-desert transitional zone of southern Xinjiang. J. Southwest Agric. 30 (7), 4. doi:10.16213/j.cnki.scjas.2017.7.027
Chen, G. S., Zeng, D. H., Chen, F. S., Fan, Z., and Geng, H. (2004). [A research review on "fertile islands" of soils under shrub canopy in arid and semi-arid regions]. Appl. Ecol. 14 (12), 2295–2300.
Chen, S. Y., Yu, H., Feng, Q. S., Xiao, K., and Wang, H. (2013). Study on spatial heterogeneity of soil N/P ratio in Gannan Plateau. Grassl. J. 21 (1), 30–36. doi:10.11733/j.issn.1007-0435.2013.01.005
Chen, Y., Yao, Z., Sun, Y., Wang, E., Tian, C., Sun, Y., et al. (2022). Current studies of the effects of drought stress on root exudates and rhizosphere microbiomes of crop plant species. Int. J. Mol. Sci. 23 (4), 2374. doi:10.3390/IJMS23042374
Chen, Y. J., Liu, J. Z., Jing, S. H., and Fang, Y. (2018). Research on the phenomenon of 'feidao/gu' under the crown of Tamarix chinensis in the yellow river delta Wetland. J. Liaocheng Univ. Nat. Sci. Ed. 031 (1), 56–64. doi:10.19728/j.issn.1672-6634.2018.01.009
Ding, J., and Eldridge, D. J. (2020). The fertile island effect varies with aridity and plant patch type across an extensive continental gradient. Plant Soil 254, 173–183. doi:10.1007/s11104-020-04731-w
Fuhlendorf, S. D., and Engle, D. M. (2001). Restoring heterogeneity on rangelands: Ecosystem management based on evolutionary grazing patterns. Bioscience 2, 625. doi:10.1641/0006-3568(2001)051[0625:rhorem]2.0.co;2
Guo, X. L., Wang, M. L., Zhao, L. P., and Wang, M. (2020). Effect of Haloxylon ammodendron and Populus euphratica on ‘Feidao’ in arid areas. J. Southwest Agric. 33 (5), 7. doi:10.16213/j.cnki.scjas.2020.5.020
Jiang, Q., He, S. Q., and Gong, Y. B. (2020). Litter nutrient characteristics and potential return ability of different vegetation types. Resour. Environ. arid areas 4, 6. doi:10.13448/j.cnki.jalre.2020.108
Li, J., Gilhooly, W. P., Okin, G. S., and Blackwell, J. (2017). Abiotic processes are insufficient for fertile island development: A 10-year artificial shrub experiment in a desert grassland. Geophys. Res. Lett. 44 (5), 2245–2253. doi:10.1002/2016GL072068
Li, S., Chen, W., Li, Z., Bu, L., Jin, Z., Wei, G., et al. (2021). Fertile islands lead to more conspicuous spatial heterogeneity of bacteria than soil physicochemical properties in a desert ecosystem. Catena 206, 105526. doi:10.1016/J.CATENA.2021.105526
Li, X. F., Zhong, Z. W., Dirk, S., Smit, C., Wang, D., Nummi, P., et al. (2018). Reciprocal facilitation between large herbivores and ants in a semi-arid grassland. Proc. R. Soc. B 285 (1888), 20181665. doi:10.1098/rspb.2018.1665
Liu, X. D., Chen, L., Yang, X. G., and Dency, O. (2016). The 'fertilizer island' effect of two kinds of Caragana korshinskii and artemisia lordosis shrub soil nutrients in a desert steppe. J. Northwest For. Univ. 31 (4), 8. doi:10.3969/j.issn.1001-7461.2016.04.05
Luo, Q., Wang, Y. G., Deng, C. Y., and Li, S. (2017). Distribution of inorganic carbon in the soil profile of the arid area and its relationship with salinity and alkalinity. J. Soil Water Conservation 31 (05), 240–246. doi:10.13870/j.cnki.stbcxb.2017.05.37
Mendes, R., Garbeva, P., and Raaijmakers, J. M. (2013). The rhizosphere microbiome: Significance of plant beneficial, plant pathogenic, and human pathogenic microorganisms. FEMS Microbiol. Rev. 37, 634–663. doi:10.1111/1574-6976.12028
Mora, J. L., and Lazaro, R. (2013). Evidence of a threshold in soil erodibility generating differences in vegetation development and resilience between two semiarid grasslands. J. Arid Environ. 89 (FEB), 57–66. doi:10.1016/j.jaridenv.2012.10.005
Ochoa-Hues, R., Eldridg, D. J., Delgado-B, M., Soliveres, S., Bowker, M. A., Gross, N., et al. (2017). Soil fungal abundance and plant functional traits drive fertile island formation in global drylands. J. Ecol. 106, 242–253. doi:10.1111/1365-2745.12871
Qu, W., Yang, X., Zhang, C., and Chang, P. (2015). Selecting specific PCR primers with MFEprimer. Methods Mol. Biol. 24 (04), 201–213. doi:10.1007/978-1-4939-2365-6_15
Reynolds, J. F., Virginia, R. A., Kemp, P. R., de Soyza, A. G., and Tremmel, D. C. (1999). Impact of drought on desert shrubs: Effects of seasonality and degree of resource island development. Ecol. Monogr. 69 (1), 69–106. doi:10.1890/0012-9615(1999)069[0069:iodods]2.0.co;2
Wang, H. F. (2020). Study on plant diversity and ecosystem function in Ebinur Lake Basin. China: Xinjiang University. doi:10.27429/d.cnki.gxjdu.2020.001436
Wang, J., Ding, J., Li, G., Liang, J., Yu, D., Aishan, T., et al. (2019). Dynamic detection of water surface area of Ebinur Lake using multi-source satellite data (Landsat and Sentinel-1A) and its responses to changing environment. Catena 177, 189–201. doi:10.1016/j.catena.2019.02.020
Wang, J., Shi, T., Yu, D., Teng, D., Ge, X., Zhang, Z., et al. (2020). Ensemble machine-learning-based framework for estimating total nitrogen concentration in water using drone-borne hyperspectral imagery of emergent plants: A case study in an arid oasis, NW China. Environ. Pollut. 266, 115412. doi:10.1016/j.envpol.2020.115412
Yan, Y., Xu, D., Wang, D., Cai, Y., and Jing, B. (2019). Shrub patches capture tumble plants: Potential evidence for a self-reinforcing pattern in a semiarid shrub encroached grassland. Plant Soil 442 (1-2), 311–321. doi:10.1007/s11104-019-04189-5
Yang, X., and Williams, M. (2015). Landforms and processes in arid and semi-arid environments. Catena 134, 1–3.
Yang, X. D., Lv, G. H., Tian, Y. H., and Chi, N. (2009). Ecological grouping of plants in Ebinur Lake Wetland nature Reserve, Xinjiang. J. Ecol. 12, 2489–2494. doi:10.13292/j.1000-4890.2009.0407
Yang, Y., Ha, S., Sun, B. P., and Xiao, X. (2012). Soil nutrient effects of different vegetation restoration types in the southern margin of Mu Us Sandy Land. Agric. Sci. Technol. 28 (8), 1708–1712. doi:10.3969/j.issn.1000-6850.2012.10.007
Ying, M. (2019). Editorial: Biotechnological potential of plant-microbe interactions in environmental decontamination. Front. Plant Sci. 10, 1519. doi:10.3389/fpls.2019.01519
Zhang, L., Lv, G. H., and Jiang, L. M. (2019). Analysis of soil driving forces affecting desert plant biomass distribution in Ebinur Lake Basin. J. Plant Resour. Environ. 2019(3), 12–18. doi:10.3969/j.issn.1674-7895.2019.03.02
Zhang, X. L., Zhou, J. H., Lai, L. M., and Xia, M. (2019). Leaf traits and ecological stoichiometric characteristics of dominant species in oasis-transition zone-Gobidesert community in the lower reaches of Heihe River. Appl. Environ. Biol. 25 (6), 7.
Zhang, Y. L., Lu, Y. X., Yin, B. F., and Chen, R. (2022). Effects of simulated rainfall changes on soil nutrients and enzyme activities in Gurbantunggut Desert. Ecology 42 (05), 1739–1749. doi:10.5846/stxb202102170450
Zhao, H. L., Su, Y. Z., and Zhang, T. H. (2002). [Influencing mechanism of several shrubs and subshrubs on soil fertility in Keerqin sandy land]. Appl. Ecol. 13 (7), 802–806.
Zhao, R., and An, L. (2021). Plant size of the alpine cushion thylacospermum caespitosum affects soil amelioration at different elevations. Plant Ecol. 23, 323–335. doi:10.1007/S11258-020-01108-Y
Keywords: woody plants, fertile island effect, influencing factors, arid area, Ebinur lake basin
Citation: Zhang Y, Wang H, Cai Y, Yang Q and Lv G (2022) Fertile Island Effect by Three Typical Woody Plants on Wetlands of Ebinur Lake, northwestern China. Front. Environ. Sci. 10:1034077. doi: 10.3389/fenvs.2022.1034077
Received: 01 September 2022; Accepted: 14 October 2022;
Published: 03 November 2022.
Edited by:
Z. Y. Yuan, Institute of Soil and Water Conservation (CAS), ChinaReviewed by:
Junqiang Yao, China Meteorological Administration, ChinaJingzhe Wang, Shenzhen Polytechnic, China
Copyright © 2022 Zhang, Wang, Cai, Yang and Lv. This is an open-access article distributed under the terms of the Creative Commons Attribution License (CC BY). The use, distribution or reproduction in other forums is permitted, provided the original author(s) and the copyright owner(s) are credited and that the original publication in this journal is cited, in accordance with accepted academic practice. No use, distribution or reproduction is permitted which does not comply with these terms.
*Correspondence: Hengfang Wang, d2FuZ2hmQHhqdS5lZHUuY24=; Guanghui Lv, bGVyQHhqdS5lZHUuY24=