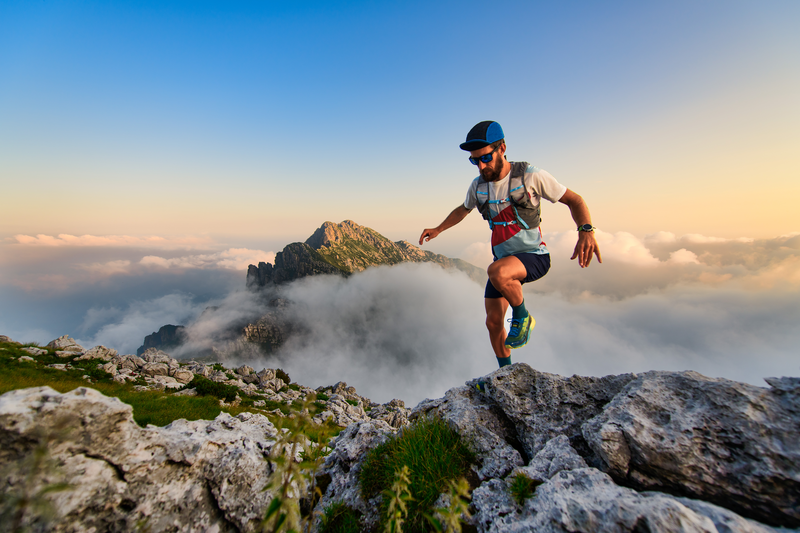
94% of researchers rate our articles as excellent or good
Learn more about the work of our research integrity team to safeguard the quality of each article we publish.
Find out more
ORIGINAL RESEARCH article
Front. Environ. Sci. , 13 January 2023
Sec. Interdisciplinary Climate Studies
Volume 10 - 2022 | https://doi.org/10.3389/fenvs.2022.1028793
This article is part of the Research Topic Energy Materials Based Novel Solar Thermal Applications View all 13 articles
This work aims to reduce the energy consumption of existing buildings through retrofitting and converting them into net zero energy buildings (NZEBs). Various retrofits for an existing academic building were modeled and analyzed using REVIT software. Energy consumption was reduced to approximately 34% through retrofits, and a Solar System of 41.6 kW was integrated to meet the reduced energy demand, consequently converting the building into an net zero energy building (NZEB). Furthermore, environmental and economic analyses were conducted, and the results show a reduction of 32.8 metric tons of CO2 emissions per year as a result of retrofitting the building, converting it into an net zero energy building. The building envelope retrofits and electrical appliances have a payback period of 2.96 and 2.62 years, respectively, whereas the proposed Solar System has a payback period of only 2.3 years. Moreover, the building was rated using the Leadership in Energy and Environmental Design (LEED) rating tool, and it qualifies for silver certification after retrofits and the integration of renewable energy sources (RES). The reduction in energy consumption and integration of renewable energy sources contribute to achieving Sustainable Development Goal (SDG) 7, and reduced CO2 emissions save climate variations, which leads to achieving Sustainable Development Goal 13.
Net zero energy building (NZEB) is an emerging reform in building construction, which addresses the increasing energy demands around the world, especially in developing and energy-deficient countries (Valasai et al., 2017) (Yousuf et al., 2014). Due to the speedy growth of urbanization and infrastructure change, the energy crisis has become a major issue, not only in developing countries but across the globe (Raheem et al., 2016). To meet the ever-increasing energy demands, fossil fuels are depleting at a much higher rate. Moreover, they are also responsible for greenhouse gas emissions (GHG) in the atmosphere, which is a serious environmental concern (Pervez et al., 2021). Since the energy deficit and GHG emissions have become a global concern, the World Health Organization (WHO) has recently issued a set of Sustainable Development Goals (SDGs) and has set a target of 2030 to transform the world into a better place for all (Pradhan et al., 2017). There are 17 SDGs that aim to remove poverty, protect our environment, and make this planet safer and more reliable for humanity (Chirambo, 2018). SDG 7“Affordable and Clean Energy” and SDG 13“Climate Action” are primarily related to the energy reforms required globally that ensure a reduction in energy scarcity and carbon emissions for a better climate (Röck et al., 2020). The retrofitting of an existing structure is one way of contributing to the achievement of SDGs 7 and 13 (Allen et al., 2016). All over the world, construction industries have started to adopt novel technologies to overcome the energy deficit, among which, the NZEB has gained prime importance (McCollum et al., 2018).
Over the past few decades, the concept of the NZEB has been adopted by many countries. Its refined definition is a building that generates an equal or greater amount of emission-free renewable energy than its emission-producing, non-renewable energy consumption (Soni & Bhagat Singh, 2020). Many researchers have emphasized reducing energy usage rather than renewable energy production through different methods, such as passive designing (Hasan & Mohamed, 2015), energy-efficient building services (Torcellini et al., 2006), thermal resistive construction materials (Aized et al., 2017), building envelope insulation strategies to gain maximum daylight and ventilation benefits, and the integration of renewable energy generation systems (Purbantoro & Siregar, 2019). Design-related strategies are, however, to be considered for a new building yet to be constructed where the implementation of other techniques has been considered in the design phase and operation phase retrofits as well.
New buildings have the potential for the application of all energy-efficient strategies, but improvement in the energy performance of existing buildings is also extremely important, along with the need of the hour: to save energy (Maheshwari et al., 2017). For existing buildings, retrofitting (after initial construction and occupation) is the most efficient way to reduce energy consumption, and integrating a renewable energy source fulfills the purpose of enhancing the building’s energy performance (Ma et al., 2012; Farooqui, 2014). Building retrofits have helped to reduce global greenhouse gas emissions significantly (Li et al., 2019). In developing nations, retrofitting existing buildings to increase their energy saving and reduce the environmental burden is now a critical component of modern building sector strategies (Aman et al., 2017). Building energy retrofit measures have shown the potential to reduce energy loads by 30% (Aydin And Mihlayanlar, 2020), and a deeper retrofit can even reach 72% in some developed countries (Xiao, 2014).
Various studies have shown that energy-efficient retrofitting measures include the replacement of conventional lighting fixtures with energy-efficient ones (Kaewunruen et al., 2019), applying window shading (Ahmed & Asif, 2020), the use of glazed glass, and improving airtightness (Urbikain, 2020). The use of an energy-efficient air conditioning system (She et al., 2018) and adding building envelope insulation are also among the eight energy efficiency measures (EEMs) that can reduce energy use by up to 60% (Pohoryles et al., 2020) and CO2 emissions by up to 30% (Kamal et al., 2019). Among these retrofit measures, replacing inefficient equipment with more energy-efficient options can save up to 27.4% of energy consumption (Luddeni et al., 2018).
In recent times, the replacement of conventional lights with energy-efficient LEDs has been proven to be an effective retrofit measure (Hayter Kandt, 2011). Furthermore, reflecting daylight off indoor surfaces inside a building is a way of using renewable energy sources, i.e., using sunlight for lighting purposes (Mughal et al., 2018). As lighting fixtures release heat, minimizing the use of artificial lights and replacing them with daylight or LED lights (Dubois et al., 2015) will not only reduce the impact of lighting on energy consumption (Vogiatzi et al., 2020) but also results in the reduced thermal effect on a building, which consequently reduces cooling loads from the overall energy consumption, thus lowering energy costs (Winkler et al., 2018). However, sunlight may be a good source of renewable energy, but a location with good sunlight reception also faces the challenge of an increase in building temperature in hot climates and thus an increase in cooling loads on energy consumption (Sharaf, 2014). By providing building envelope insulation, using glazed windows for blocking out the heat of sunlight and only allowing useful light to enter, and insulating the roofs and walls with heat repellants like epoxy (Wong, 2021), calcium silicate, or polythene, the cooling loads can also be controlled for the reduction of the overall energy consumption (Annibaldi et al., 2020). It is considered important to assess the extent of achievement in converting any construction to a net zero energy building, and in the past few decades, it has become an essential practice to rate buildings on their energy performance (Doan et al., 2017).
With different scenarios, building types, climatic conditions, and norms of the construction industries, there is vast diversity in retrofitting techniques and their effectiveness. This work represents a step further toward the achievement of SDG 7 and SDG 13 by converting an academic building to a net zero energy building through indigenous retrofitting and integrating renewable energy sources. The building under consideration is rated under a standard building rating tool: Leadership in Energy and Environmental Design (LEED). Furthermore, a comparison is made in terms of energy consumption and CO2 emissions before and after indigenous retrofitting solutions.
Buildings such as residential apartments, academic blocks, hospitals, etc., and most importantly, multi-purpose buildings, which are used throughout the day, are the targeted buildings for this study. This study focuses on the conversion of the academic block of the University of Engineering and Technology (UET), which is predicted to save energy and provide financial support to the institution. Details of the selected academic building are given in Table 1. The architectural plans of the first and second floors of the building are given in Supplementary Figures S1, S2, respectively, in the supplementary information file.
The methodology involves the collection of pre-existing information, like the manual counting of appliances with their power rating, the material used in the building, the building elevation, and full details of all other appliances that contribute to the energy consumption of the building. Table 2 shows the details of existing fixtures. Figure 1A shows the 3D model of the building, and Figure 1B represents the energy model of the building on REVIT.
Different retrofitting measures were adopted to make the building an NZEB. After the collection of the existing data, it was analyzed that the existing appliances were obsolete and not energy efficient and led to energy wastage. Similarly, the building envelope was not thermally resistive, with single-glazed windows and an uninsulated roof and walls, which significantly affected the cooling load of the internal space of the building. Existing appliances were replaced by energy-efficient appliances; for example, conventional fluorescent lights were replaced with energy-efficient lights such as LEDs, old, conventional fans were replaced with new, energy-efficient fans, and conventional air conditioners were replaced with the latest inverter technology-based air conditioners.
The building envelope is a major contributor to raising the cooling requirements of the building due to heat transfer through windows, doors, walls, and the roof. Therefore, to reduce the cooling requirements, different retrofitting measures were used in the building. The retrofitting measures include the replacement of single-glazed windows with double-glazed windows, roof coating with reflective paint, and the addition of a wall insulation layer. All the above cases, including the existing data and after retrofitting measures, were analyzed using REVIT, a building information modeling (BIM) tool.
After performing electrical retrofitting and making improvements in the building envelope, the reduction in energy consumption was calculated through BIM analysis. After minimizing the total energy consumption of the building through various retrofitting measures, solar photovoltaic-based energy was integrated to compensate for the remaining energy needs to make the building an NZEB. Solar panels were integrated because enough space was available on the rooftop of the building for their installation and because of their economic gain and environmentally friendly behavior. PV installation on the rooftop has multiple benefits, including the provision of insulation in the roof to prevent excessive heat penetration into the building. This, in turn, reduces the cooling requirements of the building. The Solar System containing 80 PV panels was applied with an intra-row spacing of 3.3 ft. The Helioscope tool was used for the integration of solar panels with the building system. The power of the system is 41.6 kW, with an annual production of 63.15 MWh.
An analysis of CO2 emissions was conducted using electrical energy consumption (kWh) and the emission factor (EF) as per the available literature (Latif et al., 2022) relevant to the selected location. An economic analysis of all retrofitting measures was conducted based on the payback period. The payback period was based on the energy saving resulting from retrofitting measures and the integration of the solar PV system. Annual energy savings based on the electricity consumption tariff in Pakistan were evaluated and the resulting reduction in CO2 emissions was calculated. Eventually, the completion of all these analyses enabled the achievement of SDG 7, ensuring access to affordable, reliable, sustainable, and modern energy for all, and SDG 13, contributing to combatting climate change.
For electrical retrofitting, the first step is to collect the existing electricity consumption data of the building through an energy audit. It is found that the existing fixture in the building consumes extra energy and needs to be replaced with energy-efficient ones. Based on the electrical audit results, electrical fixtures that consume less energy and deliver the same output were selected. The difference in the power of the existing and proposed fixtures is shown in Table 3.
The comparison of existing and proposed fixtures for each electrical appliance is shown in Figures 2, 3.
Figure 4 shows the reduction in energy loads through retrofitting. The electrical load of the building is reduced by 34% by adopting various retrofitting measures. The lighting load of the building is reduced by 39.60% from the existing loads. Similarly, the fan load and the air conditioning load are reduced by 40.90% and 15.19%, respectively.
FIGURE 4. Difference in energy consumption of existing and proposed electrical fixtures of the selected building.
For the retrofitting of the building envelope, various options were selected, including the addition of insulation in walls, the replacement of windows glass, and the coating of the roof with reflective paint. Energy Analysis of the building for various retrofitting measures was done through a building information modeling (BIM) tool, i.e., REVIT. The main aim of energy analysis of a building is to check for its cost-effective and sustainable alternatives at the design and planning stage. BIM gives many such opportunities, of which, creating insights and energy analysis through REVIT is one of the most effective. To determine the cooling loads of the selected building for different retrofitting measures, it was required to run an energy simulation through REVIT.
For wall insulation, an insulation sheet of 1-inch thickness was applied, which reduced the building temperature by 8–10°. The existing window glass was replaced with double-glazed glass, which impacted the cooling loads. For the roof retrofitting, epoxy paint was applied to reflect most of the incoming solar heat.
The spaces generated in the architectural model are considered for heating and cooling load calculation. After updating the weather details of the selected location, reports of heating and cooling load are generated for the existing and proposed fixtures. For the existing building, the peak cooling load was found to be 124.1 tons at 2:00 p.m. in June, whereas the peak cooling load for all combined retrofit cases was found to be 87.5 tons at 2:00 p.m. in June, a decrease of 30% from existing loads.
The prominent reductions in the cooling loads attained through the implementation of various retrofitting measures are shown in Figure 5, and a maximum reduction of 13% in cooling load was achieved through the use of double-glazed windows. The application of epoxy resins on the roof resulted in an 8% reduction. However, the value for all combined cases indicates a 30% decrease in cooling loads, which ultimately leads to significant energy savings.
Firstly, the building is retrofitted using different measures, and energy consumption is reduced. Then, according to the energy demand, a Solar System is installed to generate energy to meet the requirement of the building. The energy consumption of the building is reduced by applying all measures in the building, as discussed in Section 3.1. By implementing all the retrofitting measures, the annual energy consumption is reduced from 69,356.0 kWh to 46,729.5 kWh, which is approximately a 34% reduction, as shown in Figure 6.
The main objective of this study is the conversion of the selected building to an NZEB, so there was a need to integrate renewable energy to meet the remaining energy demand. A solar energy system was installed, the generation of which would be beneficial in net metering, and ultimately, the building would be converted into an NZEB.
As the building energy usage was reduced by implementing different retrofitting measures, the next step was to design a Solar System to meet the remaining energy demand, which was approximately 46 MWh (46,729 kWh). Therefore, a Solar System was designed using Helioscope software. It consisted of 80 panels with an inter-row spacing of 3.3 ft and was installed on the roof with a tilt angle of 32°. Figure 7 shows the detailed layout of the Solar System design. The system’s installed capacity was 41.6 kW, with an annual production of 63.15 MWh.
Carbon dioxide emissions are harmful gases released into the atmosphere by different human activities. Therefore, it was important to analyze carbon dioxide emissions in the selected building, CERAD, before and after the retrofitting measures. The analysis was conducted by Eq. 1, as reported in (Latif et al., 2022).
where ‘EC’ is the electrical energy consumption (kWh) and ‘EF’ represents the emission factor. The carbon dioxide reduction was calculated based on an emission factor of .405 kg CO2/kWh, as per the IGCEP (Indicative Generation Capacity Expansion Plan) report by NTDC (National Transmission and Dispatch Company) per kWh energy generation (Latif et al., 2022).
Figure 8A illustrates the carbon dioxide emissions of electrical fixtures such as lights, fans, and air conditioners. The energy-based carbon dioxide emissions are further reduced by improving the building envelope with different techniques. The reduction in cooling loads lowers the use of electrical appliances to cool the building. Consequently, the carbon dioxide emissions are reduced from 26.5 metric tons to 17.9 metric tons through the integration of all retrofitting measures. In the retrofitted building, the CO2 emissions are reduced by approximately 33% as compared to the existing building emissions, as shown in Figure 8B. The integration of solar systems also has an environmental impact. Therefore, the installed system of 41.6 kW eliminates 25.2 metric tons of carbon dioxide per year. Table 4 depicts a clear reduction in emissions of retrofitted electrical fixtures that can be observed from the results.
FIGURE 8. (A) Comparison of reduction in CO2 emissions before and after retrofitting measures in electrical fixtures and (B) overall reduction in CO2 emission of the building after retrofitting.
The assessment of SDGs is related to the energy consumption of the building and its influence on the climate. The reduction in energy consumption and the integration of renewable energy resources contribute to the achievement of SDG 7, and carbon dioxide emissions lessen the frequent climate variations that facilitate the attainment of SDG 13. Therefore, this analysis can act as one of the important steps in attaining SDG 7 and SDG 13 through retrofitting.
Economic analysis of all retrofitting measures is determined based on the payback period. The simple payback period was calculated for the implementation of all energy-efficient retrofitting measures using the relation expressed in Eq. 2
Where ‘
The cost estimates of all materials and components used for retrofitting were taken from the local market to evaluate the payback period. For the wall insulation, epoxy roof, and double-glazed windows, the area of application, and cost per square foot were determined. The cost of the energy saved through these retrofitting measures and solar energy integration was evaluated and thus, the payback period was calculated. The payback period of electrical retrofitting is shown in Table 5. It can be observed that the combined amount required for electrical retrofitting is approximately 8,000 USD, with an expected payback period of 2.62 years.
Table 6 enlists the payback periods for each retrofit measure of the building envelope. The combined cost required for the improvement in the building envelope was almost 15,000 USD and their payback period was observed to be 2.96 years. Moreover, the installation of the Solar System on the roof of the building cost approximately 20,000 USD, and the payback period for this system was 2.3 years.
The building was rated according to LEED v4.1 O + M (details are available in Supplementary Table S1 in supplementary information) for the existing building before and after retrofitting. The precondition points are required by the building and have no credit value. They are assessed using a “Yes or No” system. Other points are received for achievement, and the point for “yes” counts for the building rating while “no” indicates the absence of that aspect/factor. Such ratings vary from certified to platinum.
Upon assessment of the building, it was found that the building scored 38 points before retrofitting and lies in the range of non-certified buildings, as per LEED standards. For this study, a minimum score was given to the transportation performance, water performance, waste performance, and indoor environmental quality performance as the focus of this study is only on energy performance (Elsorady & Rizk, 2020) (Khan et al., 2020). The scorecards for existing and improved buildings are attached in Supplementary Figures S3, S4, in the supplementary information file. However, after the electrical and building envelope retrofitting, the energy performance level, which lies under the “energy and atmosphere” credit category, was improved. Along with this, under the same credit category, the building gained a point for “grid harmonization” as, after the installation of solar panels, the building was capable of generating energy. It was found that the building could be a candidate for silver certification as it gained 59 points after electrical and building envelope retrofitting. A prominent difference in the energy and atmosphere aspects can be observed as it improved from 13 points for the existing building to 33 points with the installation of the Solar System of 41.6 kW. Table 7 shows the comparative rating of the building.
This work presents an analysis of the conversion of an existing academic building to a net zero energy building through retrofitting measures and the integration of solar energy to reduce the CO2 emissions. The study concludes that:
1. The electrical retrofitting measures for lighting fixtures reduced energy consumption by 26%. The combined impact of applying all retrofitting measures resulted in a 30% reduction in the cooling load of the building and the results are aligned with that of the existing literature (Al-Mofeez, 2006; Patel et al., 2019).
2. A reduction in the energy load of the retrofitted building resulted in a 32% reduction in carbon dioxide emissions. The integration of solar photovoltaic panels on the rooftop resulted in a further reduction of 25.2 metric tons of CO2 emissions per year.
3. The cost of retrofitting the building envelope with double-glazed windows, epoxy coating, and wall insulation was approximated to be 15,000 USD, with a payback period of 2.96 years. Electrical retrofitting required around 8,000 USD, with an expected payback period of 2.62 years, while the integration of the Solar System required 20,000 USD, with an expected payback of 2.3 years.
4. The energy-efficient building is a way forward in the achievement of SDGs 7 and 13. The building was capable of achieving a silver rating in LEED v4.1 O + M after retrofitting measures, whereas, with the previous conditions, it did not qualify to be certified.
5. Buildings that consume more energy must be converted into NZEBs, and this concept should be included in the rules and regulations of the planning and construction of residential buildings. Besides the integration of the photovoltaic system, the building’s waste-to-energy concept can make the buildings more sustainable.
The original contributions presented in the study are included in the article/Supplementary Material, further inquiries can be directed to the corresponding author.
IR and MAM conceived the concept. IR executed the work and drafted the manuscript in coordination with MAM and AQ. MAM and MAS supervised the work. AQ and KI reviewed and refined the draft with AR and KM. All authors contributed equally to the preparation and revision of this manuscript and have no conflict of interest.
The authors are thankful to the Director, CERAD, and UET Lahore for facilitating and supporting this work.
The authors declare that the research was conducted in the absence of any commercial or financial relationships that could be construed as a potential conflict of interest.
All claims expressed in this article are solely those of the authors and do not necessarily represent those of their affiliated organizations, or those of the publisher, the editors and the reviewers. Any product that may be evaluated in this article, or claim that may be made by its manufacturer, is not guaranteed or endorsed by the publisher.
The Supplementary Material for this article can be found online at: https://www.frontiersin.org/articles/10.3389/fenvs.2022.1028793/full#supplementary-material
Ahmed, W., and Asif, M. (2020). BIM-based techno-economic assessment of energy retrofitting residential buildings in hot humid climate. Energy and Build. 227, 110406. doi:10.1016/j.enbuild.2020.110406
Aized, T., Mehmood, S., and Anwar, Z. (2017). Building energy consumption analysis, energy saving measurements and verification by applying HAP software. Pak. J. Engg. Appl. Sci 21.
Al-Mofeez, I. A. (2006). Predicted vs. long-term electrical energy consumption pre and post energy conservation measures: A case study of one-story house in dhahran, Saudi arabia.Proceedings of the 4th International Energy Conversion Engineering Conference and Exhibit (IECEC) June 2006. San Diego, CA, USA 1 (2), 388–397. doi:10.2514/6.2006-4044
Allen, C., Metternicht, G., and Wiedmann, T. (2016). National pathways to the sustainable development goals (SDGs): A comparative review of scenario modelling tools. Environ. Sci. Policy 66, 199–207. doi:10.1016/j.envsci.2016.09.008
Aman, M. A., Ahmad, S., Munir, M., and Ali, M. (2017). Solutions of current energy crisis for Pakistan. Int. J. Comput. Sci. Inf. Secur. 15 (5), 145–149.
Annibaldi, V., Cucchiella, F., De Berardinis, P., Gastaldi, M., and Rotilio, M. (2020). An integrated sustainable and profitable approach of energy efficiency in heritage buildings. J. Clean. Prod. 251, 119516. doi:10.1016/j.jclepro.2019.119516
Aydin, D., and Mihlayanlar, E. (2020). A case study on the impact of building envelope on energy efficiency in high-rise residential buildings. Archit. Civ. Eng. Environ. 13 (1), 5–18. doi:10.21307/acee-2020-001
Chirambo, D. (2018). Towards the achievement of SDG 7 in sub-Saharan Africa: Creating synergies between Power Africa, Sustainable Energy for All and climate finance in-order to achieve universal energy access before 2030. Renew. Sustain. Energy Rev. 94, 600–608. doi:10.1016/j.rser.2018.06.025
Doan, D. T., Ghaffarianhoseini, A., Naismith, N., Zhang, T., Ghaffarianhoseini, A., and Tookey, J. (2017). A critical comparison of green building rating systems. Build. Environ. 123, 243–260. doi:10.1016/j.buildenv.2017.07.007
Dubois, M., Bisegna, F., Gentile, N., Knoop, M., Matusiak, B., Osterhaus, W., et al. (2015). Retrofitting the electric lighting and daylighting systems to reduce energy use in buildings: A literature review. Energy Res. J. 6 (1), 25–41. doi:10.3844/erjsp.2015.25.41
Elsorady, D. A., and Rizk, S. M. (2020). LEED v4.1 operations and maintenance for existing buildings and compliance assessment: Bayt Al-Suhaymi, Historic Cairo. Alexandria Eng. J. 59 (1), 519–531. doi:10.1016/j.aej.2020.01.027
Farooqui, S. Z. (2014). Prospects of renewables penetration in the energy mix of Pakistan. Renew. Sustain. Energy Rev. 29, 693–700. doi:10.1016/j.rser.2013.08.083
Hasan, A., and Mohamed, A. (2015). “Net- and nearly- zero energy buildings: A review of the definitions and case studies,” in Proceedings of the 6th International Conference on Heating, Ventilation and Air-Conditioning, Tehran, Iran, May 2015.
Hayter, S. J., and Kandt, A. (2011). “Renewable energy applications for existing buildings preprint,”. NREL/CP-7A40-52172 (Colorado, CO, USA: National Renewable Energy Lab).
Kaewunruen, S., Sresakoolchai, J., and Kerinnonta, L. (2019). Potential reconstruction design of an existing townhouse in Washington DC for approaching net zero energy building goal. Sustain. Switz. 11 (23), 6631–6715. doi:10.3390/su11236631
Kamal, A., Al-ghamdi, S. G., and Koç, M. (2019). Role of energy ef fi ciency policies on energy consumption and CO 2 emissions for building stock in Qatar. J. Clean. Prod. 235, 1409–1424. doi:10.1016/j.jclepro.2019.06.296
Khan, A. A., Zaheer, M. U., Asghar, S., and Gardezi, S. S. (2020). Green rating assessment of a residential building in Pakistan using LEED’S. Open J. Sci. 3 (2), 126–139. doi:10.31580/ojst.v3i2.1474
Latif, M. H., Amjad, M., Tahir, Z. ur R., Qamar, A., Asim, M., Mahmood, W., et al. (2022). Nexus implementation of sustainable development goals (SDGs) for sustainable public sector buildings in Pakistan. J. Build. Eng. 52, 104415. doi:10.1016/j.jobe.2022.104415
Li, Y. L., Han, M. Y., Liu, S. Y., and Chen, G. Q. (2019). Energy consumption and greenhouse gas emissions by buildings: A multi-scale perspective. Build. Environ. 151, 240–250. doi:10.1016/j.buildenv.2018.11.003
Luddeni, G., Krarti, M., Pernigotto, G., and Gasparella, A. (2018). An analysis methodology for large-scale deep energy retrofits of existing building stocks: Case study of the Italian office building. Sustain. Cities Soc. 41, 296–311. doi:10.1016/j.scs.2018.05.038
Ma, Z., Cooper, P., Daly, D., and Ledo, L. (2012). Existing building retrofits: Methodology and state-of-the-art. Energy Build. 55, 889–902. doi:10.1016/j.enbuild.2012.08.018
Maheshwari, S., Chauhan, P., Tandon, S., and Sagar, S. (2017). A review study on net zero energy building. Int. Res. J. Eng. Technol. (IRJET) 4, 1567–1570.
McCollum, D. L., Echeverri, L. G., Busch, S., Pachauri, S., Parkinson, S., Rogelj, J., et al. (2018). Connecting the sustainable development goals by their energy inter-linkages. Environ. Res. Lett. 13 (3), 033006. doi:10.1088/1748-9326/aaafe3
Mughal, S., Sood, Y. R., and Jarial, R. K. (2018). A review on solar photovoltaic technology and future trends. Int. J. Sci. Res. Comput. Sci. 4 (1), 227–235.
Patel, A., Ghodasara, D., Bhatt, N., and Kandya, A. (2019). “Energy retrofitting of a commercial building towards a” net zero energy building” by simulation model,” in Creative construction conference (Budapest, Hungary: Budapest University of Technology and Economics), 699–712. doi:10.3311/ccc2019-096
Pervez, H., Ali, Y., and Petrillo, A. (2021). A quantitative assessment of greenhouse gas (GHG) emissions from conventional and modular construction: A case of developing country. J. Clean. Prod. 294, 126210. doi:10.1016/j.jclepro.2021.126210
Pohoryles, D. A., Maduta, C., Bournas, D. A., and Kouris, L. A. (2020). Energy performance of existing residential buildings in europe: A novel approach combining energy with seismic retrofitting. A Nov. approach Comb. energy seismic Retrofit. 223, 110024. doi:10.1016/j.enbuild.2020.110024
Pradhan, P., Costa, L., Rybski, D., Lucht, W., and Kropp, J. P. (2017). A systematic study of sustainable development goal (SDG) interactions. Earth’s Future 5 (11), 1169–1179. doi:10.1002/2017EF000632
Purbantoro, F., and Siregar, M. (2019). Design of net zero energy building (NZEB) for existing building in jakarta. IOP Conf. Ser. Earth Environ. Sci. 399 (1), 012076. doi:10.1088/1755-1315/399/1/012076
Raheem, A., Abbasi, S. A., Memon, A., Samo, S. R., Taufiq-Yap, Y. H., Danquah, M. K., et al. (2016). Renewable energy deployment to combat energy crisis in Pakistan. Energy, Sustain. Soc. 6 (1), 16. doi:10.1186/s13705-016-0082-z
Röck, M., Ruschi, M., Saade, M., Balouktsi, M., Nygaard, F., Birgisdottir, H., et al. (2020). Embodied GHG emissions of buildings – the hidden challenge for e ff ective climate change mitigation. Appl. Energy 258, 114107. doi:10.1016/j.apenergy.2019.114107
Sharaf, F. M. (2014). Daylighting: An alternative approach to lighting buildings. J. Am. Sci. 10 (4), 1–5.
She, X., Cong, L., Nie, B., Leng, G., Peng, H., Chen, Y., et al. (2018). Energy-efficient and -economic technologies for air conditioning with vapor compression refrigeration: A comprehensive review. Appl. Energy 232, 157–186. doi:10.1016/j.apenergy.2018.09.067
Soni, K. M., and Bhagat Singh, P. (2020). First onsite net zero energy green building of India. Int. J. Environ. Sci. Technol. 17 (4), 2197–2204. doi:10.1007/s13762-019-02514-0
Torcellini, P., Pless, S., Deru, M., and Crawley, D. (2006). Zero energy buildings: A critical look at the definition. ACEEE Summer Study Pac. Grove 15.
Urbikain, M. K. (2020). Energy efficient solutions for retrofitting a residential multi-storey building with vacuum insulation panels and low-E windows in two European climates. J. Clean. Prod. 269, 121459. doi:10.1016/j.jclepro.2020.121459
Valasai, G. Das, Uqaili, M. A., Memon, H. U. R., Samoo, S. R., Mirjat, N. H., and Harijan, K. (2017). Overcoming electricity crisis in Pakistan: A review of sustainable electricity options. Renew. Sustain. Energy Rev. 72, 734–745. doi:10.1016/j.rser.2017.01.097
Vogiatzi, C., Gemenetzi, G., Massou, L., Poulopoulos, S., Papaef, S., and Zervas, E. (2018). Energy use and saving in residential sector and occupant behavior: A case study in athens. Energy Build. 181, 1–9. doi:10.1016/j.enbuild.2018.09.039
Winkler, J., Munk, J., and Woods, J. (2018). Effect of occupant behavior and air-conditioner controls on humidity in typical and high-efficiency homes. Energy and Build. 165, 364–378. doi:10.1016/j.enbuild.2018.01.032
Wong, I. L. (2021). A review of daylighting design and implementation in buildings. Renew. Sustain. Energy Rev. 74, 959–968. doi:10.1016/j.rser.2017.03.061
Xiao, N. (2014). Energy-efficiency building envelope technologies Naiyuan. Thesis. Gävle, Sweden: University of Gävle, 1–34.
Yousuf, I., Ghumman, A. R., Hashmi, H. N., and Kamal, M. A. (2014). Carbon emissions from power sector in Pakistan and opportunities to mitigate those. Renew. Sustain. Energy Rev. 34, 71–77. doi:10.1016/j.rser.2014.03.003
BIM Building Information Modeling
BREEAM Building Research Establishment Environmental Assessment Method
BTU British Thermal Unit
CASBEE Comprehensive Assessment System for Built Environment Efficiency
EEM Energy Efficiency Measure
EISA Energy Independence and Security Act
EPA Environmental Protection Agency
GBRT Green Building Rating Tool
GHG Greenhouse Gas
GS Green Star
IGCEP Indicative Generation Capacity Expansion Plan
kWh Kilowatt hour
LBC Living Building Challenge
LEED Leadership in Energy and Environmental
NZEB Net Zero Energy Building
NTDC National Transmission and Dispatch Company
PEC Pakistan Engineering Council
RES Renewable Energy Sources
SDG Sustainable Development Goal
USD United States Dollar
USGBC US Green Building Council
WHO World Health Organization
Keywords: retrofitting, building information modeling (BIM), net zero energy building (NZEB), renewable energy integration, RIVET
Citation: Razzaq I, Amjad M, Qamar A, Asim M, Ishfaq K, Razzaq A and Mawra K (2023) Reduction in energy consumption and CO2 emissions by retrofitting an existing building to a net zero energy building for the implementation of SDGs 7 and 13. Front. Environ. Sci. 10:1028793. doi: 10.3389/fenvs.2022.1028793
Received: 26 August 2022; Accepted: 28 December 2022;
Published: 13 January 2023.
Edited by:
Chuanbao Wu, Shandong University of Science and Technology, ChinaReviewed by:
Muhammad Aftab Akram, Pak-Austria Fachhochschule Institute of Applied Sciences and Technology, PakistanCopyright © 2023 Razzaq, Amjad, Qamar, Asim, Ishfaq, Razzaq and Mawra. This is an open-access article distributed under the terms of the Creative Commons Attribution License (CC BY). The use, distribution or reproduction in other forums is permitted, provided the original author(s) and the copyright owner(s) are credited and that the original publication in this journal is cited, in accordance with accepted academic practice. No use, distribution or reproduction is permitted which does not comply with these terms.
*Correspondence: Muhammad Amjad, YW1qYWQ5MDAyQHVldC5lZHUucGs=
Disclaimer: All claims expressed in this article are solely those of the authors and do not necessarily represent those of their affiliated organizations, or those of the publisher, the editors and the reviewers. Any product that may be evaluated in this article or claim that may be made by its manufacturer is not guaranteed or endorsed by the publisher.
Research integrity at Frontiers
Learn more about the work of our research integrity team to safeguard the quality of each article we publish.