- 1Institute of Ecology and Biodiversity, School of Life Sciences, Shandong University, Qingdao, China
- 2State Key Laboratory of Freshwater Ecology and Biotechnology, Institute of Hydrobiology, Chinese Academy of Sciences, Wuhan, China
- 3Institute for Ecological Research and Pollution Control of Plateau Lakes, School of Ecology and Environmental Science, Yunnan University, Kunming, China
- 4College of Fisheries and Life Science, Dalian Ocean University, Dalian, China
- 5Department of Bioscience and Arctic Research Centre, Aarhus University, Aarhus, Denmark
- 6Sino-Danish Centre for Education and Research, Beijing, China
- 7Limnology Laboratory, Department of Biological Sciences, Middle East Technical University, Ankara, Turkey
- 8Centre for Ecosystem Research and Implementation (EKOSAM), Middle East Technical University, Ankara, Turkey
- 9Institute of Marine Sciences, Middle East Technical University, Mersin, Turkey
Benthivorous fish disturbance and nitrogen loading are two important factors that influence the community structure of submersed macrophytes, but their interactive effect is not well elucidated. We conducted an outdoor mesocosm experiment to examine the individual and combined effects of these two factors on the growth of two submersed macrophytes of different growth forms, i.e., the rosette-forming Vallisneria natans and the canopy-forming Myriophyllum spicatum. The treatments involved two levels of fish (Carassius auratus) disturbance crossed with two levels (0 and 12 g NH4Cl per month) of ammonium (NH4+-N) loading. For M. spicatum, we found that maximum height (MH) was reduced by 30.7%, 26.4%, and 51.0% in fish addition alone (F) and nitrogen addition treatments (N, F + N), respectively, compared with the control (C) treatment. The density of M. spicatum declined by 20%, 62% and 68.8% in the F, N and F + N treatment. The above-ground biomass (AGB) of M. spicatum respectively reduced by 56.7%, 94%, and 96.5% in the F, N and F + N treatments, and the roots/shoots ratio (R/S) increased by 114%, and 176% in N and N + F treatments, respectively. Regarding V. natans, only the MH in the N treatment was reduced (71.9%), and the density exhibited a reduction of 59.1% and 64.5% in the N and F + N treatments, respectively. The AGB of V. natans was significantly lower in the N (90.3%) and N + F (78.4%) treatments compared with the C treatment, while increased by 60.3% in F treatment. The R/S of V. natans increased by 227%, and 74.4% in the N and F + N treatments compared with the C treatment. The interactive effect of fish disturbance and high N on MH and AGB of V. natans and density of M. spicatum were antagonistic. However, the interactive effect on density and BGB of V. natans and AGB of M. spicatum were negatively synergistic. Moreover, fish activity significantly increased the concentration of total suspended solids (TSS) in the water, while total nitrogen (TN), ammonium, total phosphorus (TP), light, pH and salinity were unaffected. When fish and nitrogen were combined, TN, TP, TSS and salinity increased significantly, while pH decreased. Our study reveals that the interactive effects of fish disturbance and high N are synergistic and/or antagonistic, suggesting that the same stressor interaction may vary from synergistic to antagonistic depending on the response variables and growth forms of the macrophytes examined. Our study contributes to the understanding of how different factors can interact with each other and affect submersed macrophytes in aquatic ecosystems. This is timely and relevant knowledge, considering the range of multiple stressors involved in the decline of aquatic ecosystems worldwide at present.
1 Introduction
Due to anthropogenic activities, nitrogen deposition and pollution exert strong stress on aquatic ecosystems worldwide (Greaver et al., 2016), and have adversely affected water quality and human health over the recent decades (Erisman et al., 2013; Hamilton et al., 2016; Ti et al., 2018; Yu et al., 2019). Moreover, the rapid development of agriculture and industrialization have also resulted in massive inputs of nutrient, especially nitrogen (N) to aquatic environments (Ballard et al., 2019; Yu et al., 2019; Ti et al., 2019), with detrimental consequences to the aquatic ecosystem structure, biodiversity and ecological functioning of shallow lakes (Baron et al., 2013; Greaver et al., 2016; Bhagowati and Ahamad, 2019). Submersed macrophytes are key components in freshwater ecosystems and play an important role in maintaining the health of ecosystem structure and function, and they help to maintain clear-water conditions (Jeppesen et al., 1998; Ali et al., 2019; Su et al., 2019a). However, as a result of high nutrient loading and eutrophication, submersed macrophytes in lakes are declining, causing global degradation of aquatic ecosystems (Kosten et al., 2009; Moss et al., 2013; Zhang et al., 2017). Therefore, it is important to explore the role of nitrogen stress on submersed macrophytes for this decline to help guide aquatic ecosystem protection managers.
Presently, several multi-scale experimental studies have revealed the mechanisms of nitrogen (mainly refers to NH4+-N) stress on submersed macrophytes, including 1) physiological toxicity, such as forming oxidative stress (Nimptsch and Pflugmacher, 2007), causing imbalance of nitrogen and carbon metabolism (Zhang et al., 2011) and inhibits photosynthesis (Shi et al., 2020); 2) morphological changes, mainly manifested as decline in shoot height (or leaf length) (Saunkaew et al., 2011), biomass and relative growth rate (Olsen et al., 2015; Zhu et al., 2018; Tan et al., 2019); 3) population decline, negatively affected by longer exposure to high NH4+-N loading (Yu et al., 2022); 4) combined effect, for example, low light may enhance the toxic effect of NH4+-N (Cao et al., 2011). In addition to excessive nitrogen (N) loading, fish has also been suggested to contribute to the recession of macrophytes (Dorenbosch and Bakker, 2012; Nieoczym and Kloskowski, 2014; Badiou and Goldsborough, 2015). For example, fish may 1) cause mechanical damages to submersed macrophytes (e.g., shoot breakage and/or uprooting) by searching for macroinvertebrates (Goldsborough and Wrubleski, 2001); 2) induce low light stress on submersed macrophytes by enhancing water turbidity via sediment resuspension (Badiou et al., 2011; Kloskowski, 2011); 3) increase water column nutrient by disturbance and/or prey on zooplankton, promoting the growth of phytoplankton which may result in low-light stress on the submersed macrophytes (Van de Bund and Van Donk, 2004; Badiou and Goldsborough, 2015); 4) directly decrease macrophyte abundance through herbivory (Nurminen et al., 2003). Though the knowledge of nitrogen or fish stressor on water quality and submersed macrophytes is growing, the understanding of the interaction between nitrogen and fish is lacking.
Submersed macrophytes with different growth forms respond differently to stress induced by high ammonium (NH4-N) loading and low light conditions. For example, canopy-forming species are more tolerant to high NH4-N (<7 mg L−1) than rosette-forming species (Yu et al., 2022), because the former can form a canopy on the water surface, whereas rosette-forming species disperse under water through stolons (Xiao et al., 2006; Li, 2014; Angove et al., 2020). The canopy-forming species can, therefore, capture sufficient light, and plenty of light may relieve plants from NH4-related physiological stress, as suggested in previous mesocosm experiments (Cao et al., 2011; Yuan et al., 2016). Moreover, it has been found that fish disturbance did not affect the relative growth rate (RGR) of canopy-forming H. verticillate (Chen et al., 2020a) but hindered the growth of rosette-forming V. natans (Gu et al., 2018). Therefore, we hypothesised that 1) low light conditions caused by fish disturbance might aggravate NH4-related stress on the population growth of submersed macrophytes, in other words create a negative synergistic effect; 2) the strategies of different growth forms of submersed macrophyte might have different tolerance levels to the combined effect of high NH4-N stress and fish disturbance.
For our experiment, we selected the canopy-forming species Myriophyllum spicatum and the rosette-forming species Vallisneria natans, two common submersed macrophytes worldwide representing plants with contrasting growth forms. We then evaluated the effect of Carassius auratus (fish) and ammonium (NH4+-N) loading on water quality and the growth of these two submersed macrophytes. The objectives of this study were 1) to examine the interactive effect between fish disturbance and NH4-N loading on two growth forms of submersed macrophytes, 2) to explore the strategies of two growth forms of submersed macrophytes to combined effects of fish disturbance and NH4-N loading.
2 Materials and methods
2.1 Research area and experimental materials
The duration of the experiment was from 15 August to 25 December 2017 (132 days) in cement tanks located to the northeast of Lake Bao’an (N 30°17′17″, E 114°43′45″) (Figure 1) on the south bank of the middle Yangtze River. Vallisneria natans and Myriophyllum spicatum were cultured in 24 outdoor cement tanks (729 L, length 90 cm, width 90 cm, height 90 cm height). A 10 cm layer of lake sediment and 70 cm of lake water were added to each tank. The sediment (original contents: total nitrogen 1.83 mg g−1, total phosphorus 0.69 mg g−1, organic matter 34.8 mg g−1) was collected from a nearby pond and fully mixed before the experiment to ensure homogeneity. The seedlings/shoots of two submersed macrophytes were collected from the Lake Xi’liang in Xianning, Hubei Province. They were similar in size and healthy in appearance. For M. spicatum, the apical shoots were 15 cm in length and had a fresh weight of 5.09 ± 3.49 g (mean ± SD), with no flower. For V. natans, intact seedlings (containing roots) were 15 cm in length, with a fresh weight of 4.08 ± 1.73 g leaves and 0.57 ± 0.23 roots. The seedlings/shoots were carefully washed to remove attachments before plantation in tanks.
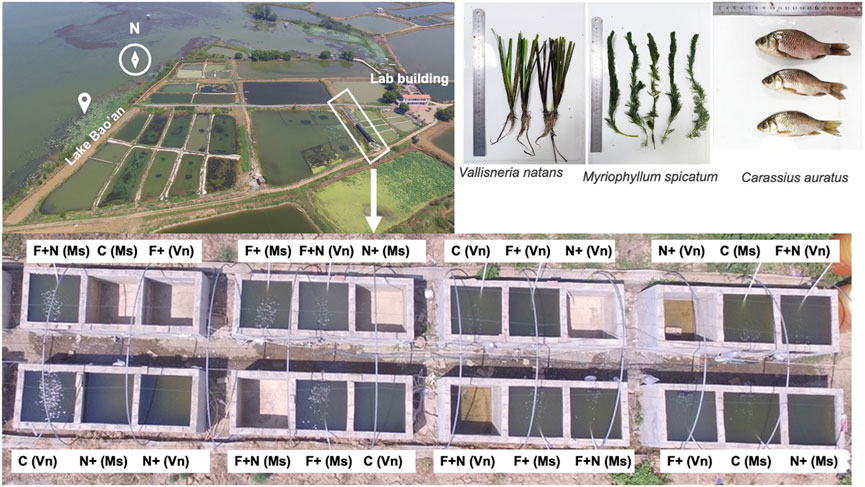
FIGURE 1. The mesocosm experimental systems. C, F+, N+, and F + N represent the following treatments: control, fish introduction alone, ammonium loading alone and both fish introduction and ammonium loading, respectively. Vn, Vallisneria natans; Ms, Myriophyllum spicatum.
Individuals of crucian carp Carassius auratus, a common benthivorous fish widely found in the mid-lower Yangtze lakes (Gao et al., 2014; Yu et al., 2016). Being an omni-benthivorous fish, crucian carp can affect the light intensity by sediment disturbance leading to resuspension and more phytoplankton due to sediment nutrient release, which in turn may result in light limitation of the submersed macrophyte growth (Richardson et al., 1995; Bernes et al., 2015). Therefore, crucian carp is suitable for revealing fish disturbance effects on water quality and submersed macrophytes and it is, moreover, a common fish species in eutrophic shallow Chinese lakes (Liu et al., 2007). The crucian carp Carassius auratus in the experiment were captured from the water bodies around Lake Bao’an by a fisherman. The individuals selected were of similar size (14.3 ± 6.2 cm in length and 79.9 ± 20.8 g in weight) and cultured in tap water for a week before the experiment.
2.2 Experimental design
At the beginning of the experiment, 25 shoots of M. spicatum and 25 seedlings of V. natans were planted in 12 tanks respectively, and there were 24 tanks in totally. Then lake water was added to all tanks to a depth of 70 cm. Seven days after the planting, fish were added to the fish treatment tanks. The tanks were randomly assigned to two fish levels and two NH4+-N loading levels, and each treatment was replicated three times: (C) controls (no fish and no NH4+-N addition); (F) three fish per treatment; (N) 12 g NH4Cl each treatment; (F + N) three fish and 12 g NH4Cl addition in treatment. The NH4Cl addition of 12 g per month was to maintain the target NH4+ concentration of 7 mg/L, because this NH4+ concentration was the threshold of NH4+ toxicity according to our earlier pond experiments (Yu et al., 2017, Yu et al., 2018, Yu et al., 2022). Tap water was added weekly to maintain a constant water level of 70 cm. During the experiment, the water temperature ranged from 10.3°C to 28.1°C, and the average temperature in all tanks was 16.4°C.
2.3 Sampling and measurement
Environmental variables were measured once every month, from 10:00 to 11:00 a.m. Water temperature, dissolved oxygen (DO), pH and conductivity (Cond) were measured in situ at half water depth with a YSI ProPlus (Yellow spring Inc. OH, Yellow Springs, United States). Photosynthetic photon flux density (PPFD) was measured by an illuminometer (KONICA MINOLTA, T-10, China) at the air-water interface (just above the water surface) and 10 cm and 50 cm below the water surface. Approximately 1 L of overlying water just below the water surface was collected once a month at 10:00–10:30 a.m. to determine total nitrogen (TN), ammonium (NH4-N), total phosphorus (TP), total suspended solids (TSS) and phytoplankton biomass (Chl a). TN was determined following an alkaline potassium persulphate digestion-UV spectrophotometric method (PERSEE, TU-1810, Beijing, China) and TP following an ammonium molybdate-ultraviolet spectrophotometric method after digestion with K2S2O8 solution (Huang et al., 1999). For analysis of ammonium (NH4-N), water was filtered with an acetate fiber microporous membrane (0.45 μm pore diameter, Shanghai Xingya Purification Material Factory, Shanghai, China) and then NH4-N was measured with an automatic chemical analyzer (SYSTEA S.p.A, Easychem plus, Italy). TSS was measured by passing a known volume of water through pre-dried and pre-weighed Whatman GF/C filter paper, dried for 24 h at 70°C and then weighed. Phytoplankton chlorophyll a (Chl a) was extracted using 90% acetone (at 4°C for 24 h) after filtration through GF/C filters (Whatman, GE Healthcare United Kingdom Limited, Buckinghamshire, United Kingdom), and absorbance was then read at 665 nm and 750 nm both before and after acidification with 10% HCl using a spectrophotometer.
At the end of the experiment, all plants were harvested and washed carefully with tap water for further measurement. In each tank, the total number of ramets were counted for each species. A shoot that rose from the base was considered a ramet for M. spicatum. The maximum shoot length for M. spicatum and the maximum leaf length for V. natans were considered as the plant maximum height. M. spicatum is a typical canopy macrophyte, the branch number and the height of the lowest branch are vital for canopy formation and influence their adaptation to the environment. Thus, we counted the total branch number and measured the height of the lowest branch for each M. spicatum individual. All V. natans were divided into above-ground and below-ground parts, dried with bibulous paper and weighed, then oven-dried at 75°C to constant weight and weighed again to determine the dry weight. For M. spicatum, 4–7 individuals were randomly selected to determine the dry weight of their leaves and stems, and the rest values were calculated according to their relative proportions. The total biomass of each species in each tank was the sum of the dry weight of all plant organs.
The relative growth rates of the above-ground biomass (RGR-AGB, g g−1 d−1) in each tank was calculated by the following formula:
where DWt and DW0 are the plant dry weight at the end and beginning of the experiment, respectively, and T is the duration of the experimental period. To assess the stressor interaction type, the interaction statistic ρ was calculated following the equation in Turschwell et al. (2022):
The interaction is synergistic when ρ is positive, additive when ρ is zero and antagonistic when ρ is negative. YF, YN, YC, and YFN are biomass of the treatment with stressor fish (F) only, stressor nitrogen (N) only, no stressors (control—C), and both stressors (F and N), respectively. The metric ρ was used to assess whether the change rate of the response is greater with both stressors present than the multiplication with individual stressors present.
2.4 Statistical analyses
Origin 2021, StataSE 15 and Microsoft Excel 2019 were used for all statistical analyses. Values are expressed as the mean ± standard deviation (SD). Shapiro-Wilk test was used to test the model residual normality. Levene homogeneity test of variance was used to test the equality of variance. One way analysis of variance (ANOVA) was used to test the statistical significance of plant growth, morphological and water quality indices. All results were considered significant at the p < 0.05 level, and post hoc pairwise comparisons of means to determine significance were performed using the LSD test (at the 0.05 significance level). Effect sizes allowed us to compare the observed effects of individual and combined stressors. Effect sizes for individual stressors were calculated based on pairwise comparisons between each experimental condition and the control (n = 3). Cohen’s d effect sizes and 95% confidence intervals (CI) were calculated by StataSE 15.0. Given the fact that the effect sizes were large (>0.8; Cohen, 2013) and their CIs did not touch the zero line, they were considered meaningful.
3 Results
3.1 Growing environments
TN concentrations were significantly higher in the 2 N addition treatments (N and F + N) (ca. 0.6 mg/L) than in those without N addition (C and F) for both macrophyte species (Figure 2A). The differences were not significant between N and F + N, nor were they between C and F. During the experiment, TN showed increasing trends in N and F + N and stayed relative stable in C and F for both species (Supplementary Figures S1A,B). The result of NH4+ concentration was consistent with that of TN (Supplementary Figure S2).
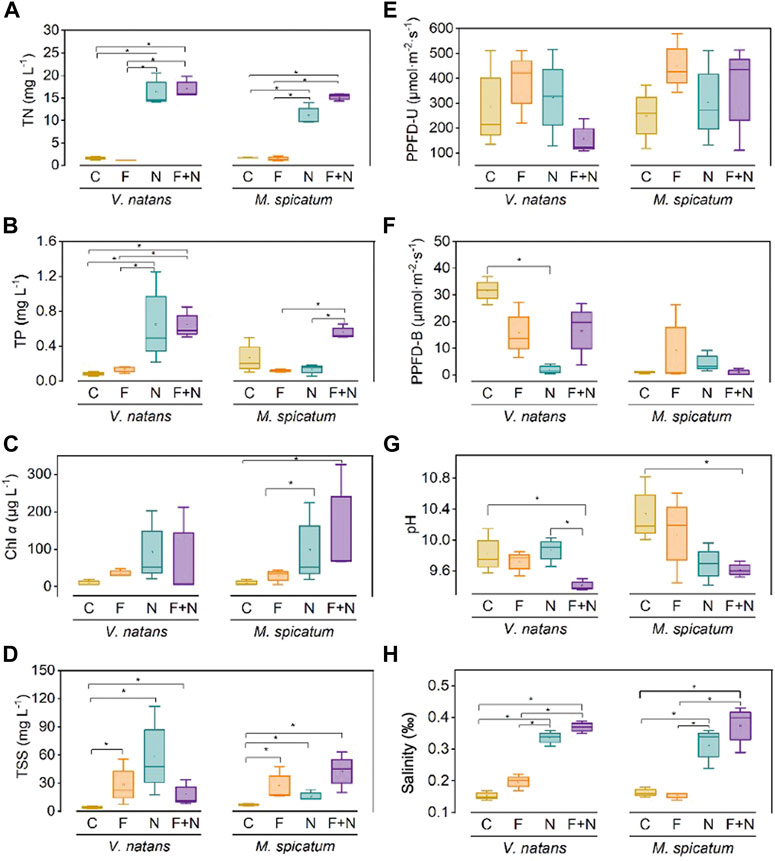
FIGURE 2. Physico-chemical water variables (mean ± SD) including total nitrogen (TN, mg L−1) (A), total phosphorus (mg L−1) (B), phytoplankton chlorophyll a (Chl a, μg L−1) (C), total suspended solids (SS, mg L−1) (D), photosynthetic photon flux density in the upper layer of water with 10 cm water depth (PPFD, μmol· m−2·s−1) (E), photosynthetic photon flux density in the bottom layer of water with 60 cm water depth (PPFD-B, μmol· m−2·s−1) (F), pH (G), and salinity (%) (H) in the water column at the end of the experiment. See Figure 1 for the meaning of the various treatments. “*” indicates significant differences at the 0.05 significance level determined by least significance difference test (LSD).
TP concentrations were significantly higher in the combined F addition and N addition treatments (F + N) (ca. 0.2–0.6 mg/L) than in the treatments without N addition (C and F) (ca. 0.08–0.27 mg/L) for both macrophyte species (Figure 2B). In the N treatment, the TP concentration with V. natans was significantly higher in the C treatment, while this not for M. spicatum. During the experiment, TP showed a declining trend in C, F and N for both species but remained relatively stable in C and F for both species (Supplementary Figures S1C,D).
Chl a concentration in the F and N addition treatments (F, N, F + N) were not significantly different from the C treatment for V. natans species (Figure 2C). However, for M. spicatum, Chl a level in the 2 N addition treatments (N and F + N) were significantly higher than in those without N addition. During the experiment, Chl a in the two N-addition treatments (N and F + N) with V. natans showed a tendency to rise at first and then decrease, while it decreased at first and then increased for M. spicatum (Supplementary Figures S1E,F).
There were significant differences in TSS concentrations in both the F and N addition treatments (F, N and F + N) compared with the C treatment (Figure 2D). For both species, the TSS concentration showed a declining trend during the experiment, except in the N treatment with V. natans and the F + N treatment with M. spicatum (Supplementary Figures S1G,H). The concentration of TSS ranged between ca. 24.8 and 54.1 g L−1 among all treatments.
The PPFD in the upper layer of water (PPFD-U) showed no difference among most treatments (Figure 2E). However, the PPFD at 50 cm depth (PPFD-B) was lower in both the F and N addition treatments (F, N and F + N) with V. natans, while no differences appeared among all treatments with M. spicatum. (Figure 2F).
Other variables in the water column changed with combined F and N addition. pH was significantly lower in the N + F treatment than in the C treatment (Figure 2G). During the experiment, pH was lower in the 2 N addition treatments (N and F + N) for both species (Supplementary Figures S1I,J). The salinity in the 2 N addition treatments (N and F + N) were significantly higher than that in the two treatments without N addition (C and F) for both species (Figure 2H). During the experiment, salinity in the 2 N addition treatments showed an increasing trend, while it remained relatively stable in C and F for both species (Supplementary Figures S1K,L).
3.2 Growth of submersed macrophytes
For V. natans, the maximum height (MH) in the N treatment was significantly lower than in the C treatment (Figure 3A) where it was reduced by 71.9%. However, in the 2 F addition (F and F + N) treatments, MH was not significantly different from that in the C treatment. Regarding M. spicatum, MH in the F addition and N addition treatments (F, N and F + N) were all significantly lower (Figure 3A), and reduced by 30.7%, 26.4%, and 51.0% in F, N, and F + N compared with the C treatment. In all treatments, M. spicatum exhibited significantly higher MH than V. natans.
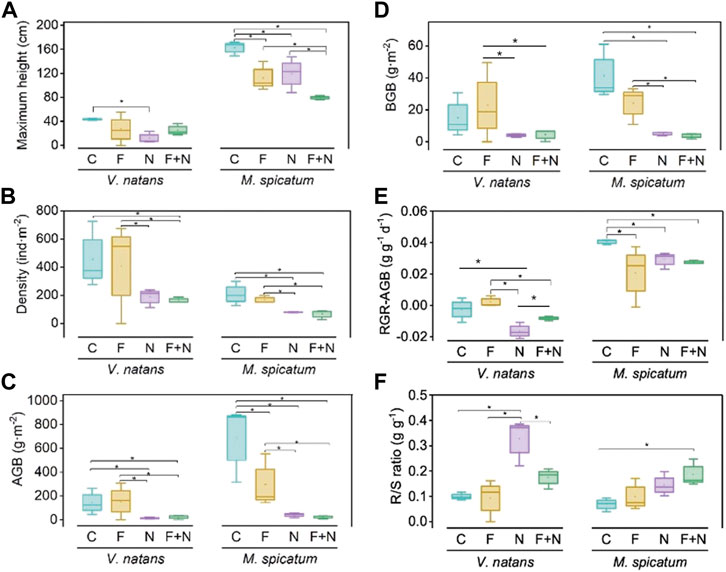
FIGURE 3. The maximum height (A), density (B), above-ground biomass (AGB) (C), below-ground biomass (BGB) (D), relative growth rate of above-ground biomass (RGR-AGB) (E) and root biomass to shoot biomass ratio (R/S ratio) (F) of Vallisneria natans and Myriophyllum spicatum in each treatment at the end of experiment. See Figure 1 for the meaning of various treatments. “*” indicates significant differences at the 0.05 significance level determined by least significance difference test (LSD).
V. natans density was significantly lower in the 2 N addition treatments (F + N and N) compared with the C treatment (Figure 3B), exhibiting a reduction of 59.1% and 64.5% in the N and F + N treatments respectively. For M. spicatum, the density declined by 20%, 62%, and 68.8% in the F, N and F + N treatment compared to the C treatment (Figure 3B). Though the density of both species demonstrated a decreasing trend with fish and/or nitrogen addition, the density of V. natans was higher than that of M. spicatum in all treatments.
The above-ground biomass (AGB) of V. natans was significantly lower in the N (90.3%) and N + F (78.4%) treatments compared with the C treatment (Figure 3C). The results for M. spicatum AGB were similar to those for V. natans in the N and F + N treatments (Figure 3C), showing a reduction of, respectively, 94% and 96.5% in the N and F + N treatments compared to the C treatment. However, the AGB of V. natans and M. spicatum contrasted each other in the F treatment. Thus, the AGB of V. natans increased by 60.3%, while it declined by 56.7% for M. spicatum compared with the C treatment. The below-ground biomass (BGB) of both species exhibited similar fluctuations as with AGB (Figure 3D).
For V. natans, the relative growth rate of the above-ground biomass (RGR-AGB) was significantly lower in the two N-addition treatments (N and F + N) than in the fish-alone treatments (F) (Figure 3E). Regarding M. spicatum, RGR-AGB was significantly lower in the F, N and F + N treatments than in the C treatment. (Figure 3E). The RGR-AGB of M. spicatum was higher than that of V. natans in the C, N and F + N treatments.
The root to shoot biomass ratio (R/S ratio) of V. natans increased by 227% and 74.4% in the N and F + N treatments compared with the C treatment (Figure 3F). For M. spicatum, R/S was significantly higher in the N and N + F treatments than in the C treatment, exhibiting an increase of 114% and 176%, respectively (Figure 3F).
3.3 Integrative assessment of the stress effects
The individual effect of fish on V. natans was not significant for the growth variables studied, and the overall effect of fish was positive (Figure 4A). The individual effect of N was significantly negative on MH and RGR-AGB, while positive on R/S, with a significantly negative overall effect (p = 0.01). Though the combined effect of fish and N was significantly positive on the R/S ratio, the combined effect on the other growth variables of V. natans was negative. The overall effect of fish and N on V. natans. Was negative for all growth variables.
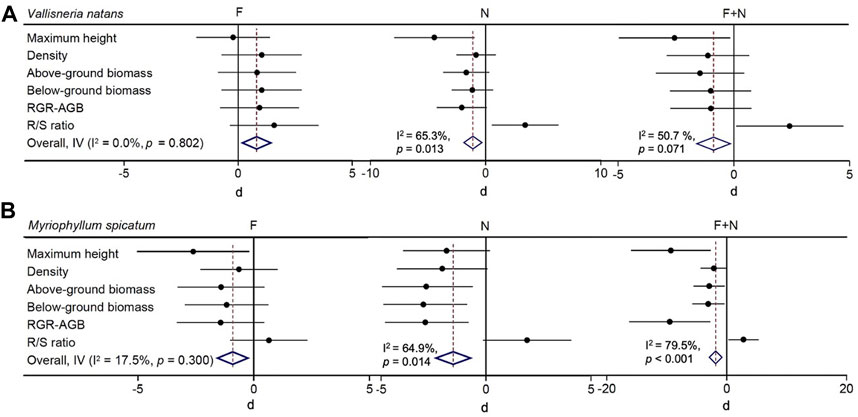
FIGURE 4. Effect sizes (Cohen’s d ± 95% confidence interval) of individual and combined stressor effects on the growth of Vallisneria natans (A) and Myriophyllum spicatum (B) at the end of the experiment. No significant effects are inferred when confidence intervals cross the zero line. Diamond indicates the overall effect. The diamond on the left-hand side of the zero line indicates a negative effect and a positive effect on the right-hand side. See Figure 1 for the meaning of the various treatments.
The individual effect of fish on the MH of M. spicatum was significantly negative. Unlike V. natans, all variables of M. spicatum were negatively affected by fish (Figure 4B). Also, the individual effect of N on the AGB, BGB and RGR-AGB of M. spicatum was significantly negative, and the overall effect of N was significantly negative for all variables of M. spicatum (p = 0.014). The observed combined effects of fish and N on MH, density, AGB, BGB and RGR-AGB were negative, while the combined effect on R/S was positive. The overall effect of fish and N was significantly negative for all variables of M. spicatum (p < 0.001).
According to the results of the interaction metric ρ, the combined fish and N has antagonistic effect on MH, AGB of V. natans and BGB of M. spicatum (ρ < 0), while synergistic on D, BGB of V. natans and AGB of M. spicatum (ρ > 0) (Figure 5). However, the interaction metric ρ of MH (ρ = 0 0.04) and D (ρ = −0.02) of M. spicatum were close to zero, implying an addictive effect.
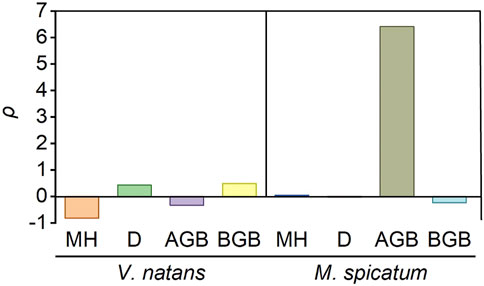
FIGURE 5. The interaction statistic ρ of two submersed macrophytes. MH, maximum height, cm; D, density, ind. m−2; AGB, above-ground biomass, g m−2. BGB, below-ground biomass, g m−2. YF, YN, YC and YFN are biomass of the treatment with stressor fish (F) only, stressor nitrogen (N) only, no stressors (control -C), and both stressors (F and N), respectively. ρ > 0 indicates synergistic effect, ρ = 0 indicates additive effect, and ρ < 0 indicates antagonistic effect.
4 Discussion
4.1 Effects of fish disturbance and ammonium loading on water quality
We found no effect of fish alone on the nutrient concentrations. These contrasts the findings in several other studies (e.g., Badiou and Goldsborough, 2015; Chen et al., 2020a) but it may reflect the high biomass of macrophytes, buffering the effect of fish disturbance and nutrient excretion (Kaldy, 2014). Previous studies have showed that fish disturbance has positive (He et al., 2017; Gu et al., 2018), neutral (Parkos et al., 2003; Chen et al., 2020b) and negative effects (Wahl et al., 2011; Badiou and Goldsborough, 2015) on the content of Chl a in the water column. In our study, the content of Chl a was not affected by fish disturbance, which may be explained by the following mechanisms: 1) the rather dense submersed macrophytes may control phytoplankton by producing allelochemicals that suppress phytoplankton growth rates (Vanderstukken, et al., 2014), and 2) submersed macrophytes may control phytoplankton by competing for nutrients (Jeppesen et al., 1998; Declerck et al., 2007). Benthivorous fish disturbance did increase the content of TSS, consistent with previous findings that fish disturbance causes resuspension of sediment (Badiou and Goldsborough, 2015). However, the resuspension of fish alone had only a weak effect on other water physical and chemical parameters, such as PPFD, salinity and pH.
We found that NH4-N alone or the combined effect of nitrogen and fish significantly changed and the physical-chemical variables in the water and led to higher biomass of phytoplankton, likely as a result of the increasing N loading (Badiou and Goldsborough, 2015; Olsen et al., 2015) combined with N-induced sediment phosphorus release (Ma et al., 2018). The significantly higher salinity in the mesocosms of N treatment can be explained by the Cl− being added with the NH4Cl caused higher salinity.
4.2 Effects of fish disturbance and ammonium loading on submersed macrophytes
The responses of V. natans and M. spicatum to nitrogen and fish, both individually and in combination, were tested in the 5-month mesocosm experiment. Overall, the results showed that fish alone had no effects on the growth of V. natans but negatively affected the height and biomass of M. spicatum. To some extent, nitrogen alone or combined with fish disturbance negatively influenced the growth of both species.
Both macrophyte species survived in fish alone treatment (F) during the experiment, although the height and biomass of M. spicatum were negatively affected by fish disturbance. V. natans produced more ramets than M. spicatum as indicated by the high density of V. natans. However, adult plants of M. spicatum could maintain a higher RGR-AGB and MH than V. natans in the fish treatment. A possible explanation for this is that the clonal growth of V. natans is interconnected by stolons to help stabilise the sediment. Moreover, the striped leaves of V. natans may provide sufficient substrate for the suspended solids and have buffering effect. In addition, the large number of ramets (high density) produced by V. natans may be an advantage for further population reproduction and expansion. Our results are different from those of previous studies showing that fish disturbance inhibited the growth of V. natans (Gu et al., 2018). However, as a canopy-forming species, the leaves of M. spicatum are more abundant in the upper layer of the water column and sparse in the lower part. Therefore, the ability of M. spicatum to compensate for the light reduction due to resuspension is weak, and this species is therefore more vulnerable to fish disturbance.
Although both macrophyte species survived in ammonium loading alone treatment (N) in the 5-month experiment, their growth was negatively affected, as indicated by the changes in maximum height, density, biomass and RGR-AGB of both populations. We found that M. spicatum could maintain higher RGR-AGB and MH than V. natans in N treatment. The higher density of V. natans at high N is an advantage for further population reproduction and expansion. The growth strategies of M. spicatum and V. natans may be an advantage when facing long-term stress, as also indicated by the higher R/S of the two species than in the C treatment. The maximum height and RGR-AGB of V. natans were significantly higher in the N treatment than for M. spicatum, which might reflect that rosette-forming macrophytes 1) usually exhibit a weaker ability to increase plant height in N stress environments, as demonstrated for V. natans by Yu et al. (2017; 2022), and 2) mainly grow by making physiological adjustments to tolerate stress conditions, such as adjusting the metabolisms of carbon and nitrogen (Yuan et al., 2016; Chen et al., 2020b), rather than by increasing plant height. Canopy-forming macrophytes, by contrast, usually elongate their shoots towards the water surface to capture light for growing (Yu et al., 2018; Angove et al., 2020). Consistent with previous studies, the high maximum height in our experiment enabled the shoots of M. spicatum to easily reach the water surface where they formed a canopy allowing them to maintain a competitive advantage in the presence of F and N stressors. In addition, the fact that the MH of M. spicatum were higher than V. natans in all treatments indicated that M. spicatum better tolerate stressors by shoot elongation.
Ecological responses to multiple stressors have been reported, and the interaction types include additive effect, antagonism and synergism which can vary between specific stressor as well as intraspecifically and interspecifically (Birk et al., 2020; Simmons et al., 2021; Turschwell et al., 2022). In our study, the joint effects of fish disturbance and high N on AGB of M. spicatum were negatively synergistic, probably with fish disturbance aggravating high ammonium stress. The joint effects on MH and density of M. spicatum were additive. This is probably because plants under high N stress become more vulnerable when at low light conditions (Gao et al., 2020), which was induced by fish disturbance in this study. The extra energy and carbohydrates are key components for the detoxication process (Netten et al., 2013; Gao et al., 2020), while photosynthesis as a key production process of energy and carbohydrate is inhibited under low light (Cao et al., 2009, 2011; Yuan et al., 2013, 2016). Differently, the joint effect was antagonistic to BGB of M. spicatum, suggesting that though their joint effects may form stress on submersed macrophytes as showed in Figures 3, 4, the consequence is opposite on the below-ground biomass. This implies that fish disturbance may, to some extent, weaken the effect of high N on the below-ground biomass. The above indicated that joint effects from the same stressors may vary from antagonistic, to additive and synergistic depending on the response variables examined. For rosette-forming V. natans, the interactive effects on MH and AGB was antagonistic while synergistic on density and BGB. On average, both macrophytes exhibited reduced growth in response to fish disturbance and N stress, but the interactive effect type was highly species-specific and response variable dependent.
Plant biomass storage and its allocation reflect ecosystem productivity and adaption to different environments (Poorter et al., 2012). We found that R/S increased with N alone and the combination of N and F, indicating a biomass allocation favouring root growth rather than stolon and leaf growth. Our results indicate that M. spicatum and V. natans responded to combined fish and nitrogen stress by changing their allocation patterns. Moreover, R/S of V. natans was the highest in the N treatment. V. natans thus allocates more biomass to the below-ground part than to the above-ground part when facing high N stress compared with M. spicatum. This supports our hypothesis that the strategies of different growth forms of submersed macrophyte might have different tolerance levels to the combined effect of high NH4-N stress and fish disturbance. Previous studies have shown the R/S ratio to be markedly reduced by N addition, as also seen for most herbaceous terrestrial plants (Müller et al., 2000; Poorter et al., 2012; Li et al., 2015; Jing et al., 2020). For aquatic plants, the allocation pattern of above-ground biomass and under-ground biomass depends on the type of resource limitation, when sediment nutrients are limiting, plants allocate more resources to the growth of roots, resulting in higher R/S ratio (Wang et al., 2008; Xie et al., 2013). Our results are inconsistent with the terrestrial plants but supports allocation pattern found for aquatic plants. It reveals that biomass allocation of aquatic plants (high R/S ratio under stress) is not only related to resource limitation, but also a strategy adopted by submersed macrophytes under adverse conditions.
5 Conclusion
Our study demonstrates a combination of fish disturbance and ammonium loading may have negative but variable effects on submersed macrophytes with different growth forms. The interactive effect on height and above-ground biomass of rosette-forming V. natans was antagonistic while being negatively synergistic to density and below-ground biomass. However, the interactive effect on above-ground biomass, density and height of canopy-forming M. spicatum was negatively synergistic and has antagonistic effect on below-ground biomass. These results suggest that the interactive effect type may vary from antagonism to synergism depending on the response variables and growth forms of macrophytes which are examined. This emphasizes the necessity of conducting experiments that include the multiple response indicators to both individual and combined stressors. In contrast to most other studies, we found no negative effect of the fish on the water quality, which likely reflect a high macrophyte coverage in our experiment. We also found that the plants allocated more biomass to roots under multiple stress. Our study contributes to the understanding of how different environmental factors can interact with each other and affect submersed macrophytes in aquatic ecosystems. This is timely and relevant knowledge, considering the range of multiple stressors involved in the decline of aquatic ecosystems worldwide. Further research that explores the interactive mechanisms among different growth traits and growth forms may provide a greater understanding for the ecological responses to multiple stressors.
Data availability statement
The original contributions presented in the study are included in the article/Supplementary Material, further inquiries can be directed to the corresponding authors.
Author contributions
QY: Validation, conceptualization, investigation, methodology, formal analysis, data curation, writing—original draft, writing—review and editing. HW: Conceptualization, methodology, writing—review, supervision, resources, project administration, funding acquisition. ML: Validation, investigation, data curation. CX: Validation, investigation. YM: Validation, investigation. WG: Writing—review and editing, conceptualization, methodology, supervision, and project administration. EJ: Writing—review and editing.
Funding
This work was supported by Major Science and Technology Program for Water Pollution Control and Treatment of China (2017ZX07302-002), National Natural Science Foundation of China (31970347), National Key Research and Development Program of China (2018YFD0900805), State Key Laboratory of Freshwater Ecology and Biotechnology (2019FBZ01), Natural Science Foundation of Shandong Province (ZR2021QC081), and Yunnan Provincial Department of Science and Technology (202001BB050078; 202103AC100001). HW was supported by the Youth Innovation Association of Chinese Academy of Sciences as an excellent member (Y201859). EJ was supported by the TÜBITAK program BIDEB2232 (project 118C250) and ANAEE-Denmark.
Acknowledgments
We thank Anne Mette Poulsen for editing the manuscript.
Conflict of interest
The authors declare that the research was conducted in the absence of any commercial or financial relationships that could be construed as a potential conflict of interest.
Publisher’s note
All claims expressed in this article are solely those of the authors and do not necessarily represent those of their affiliated organizations, or those of the publisher, the editors and the reviewers. Any product that may be evaluated in this article, or claim that may be made by its manufacturer, is not guaranteed or endorsed by the publisher.
Supplementary material
The Supplementary Material for this article can be found online at: https://www.frontiersin.org/articles/10.3389/fenvs.2022.1024524/full#supplementary-material
References
Ali, F., Jilani, G., Fahim, R., Bai, L., Wang, C., Tian, L., et al. (2019). Functional and structural roles of wiry and sturdy rooted emerged macrophytes root functional traits in the abatement of nutrients and metals. J. Environ. Manag. 249, 109330. doi:10.1016/j.jenvman.2019.109330
Angove, C., Norkko, A., and Gustafsson, C. (2020). The fight to capture light: Functional diversity is related to aquatic plant community productivity likely by enhancing light capture. Front. Mar. Sci. 7, 140. doi:10.3389/fmars.2020.00140
Badiou, P., Goldsborough, L. G., and Wrubleski, D. (2011). “Impacts of the common carp (Cyprinus carpio) on freshwater ecosystems: A review (chapter 4),” in Carp: Habitat management and diseases. Editors J. D. Sanders, and S. B. Peterson (New York: Nova Science Publishers), 121–146.
Badiou, P. H. J., and Goldsborough, L. G. (2015). Ecological impacts of an exotic benthivorous fish, the common carp (Cyprinus carpio L.), on water quality, sedimentation, and submerged macrophyte biomass in wetland mesocosms. Hydrobiologia 755 (1), 107–121. doi:10.1007/s10750-015-2220-6
Ballard, T. C., Sinha, E., and Michalak, A. M. (2019). Long-term changes in precipitation and temperature have already impacted nitrogen loading. Environ. Sci. Technol. 53, 5080–5090. doi:10.1021/acs.est.8b06898
Baron, J. S., Hall, E. K., Nolan, B. T., Finlay, J. C., Bernhardt, E. S., Harrison, J. A., et al. (2013). The interactive effects of excess reactive nitrogen and climate change on aquatic ecosystems and water resources of the United States. Biogeochemistry 114 (1-3), 71–92. doi:10.1007/s10533-012-9788-y
Bernes, C., Carpenter, S. R., Gårdmark, A., Larsson, P., Persson, L., Skov, C., et al. (2015). What is the influence of a reduction of planktivorous and benthivorous fish on water quality in temperate eutrophic lakes? A systematic review. Environ. Evid. 4, 7–28. doi:10.1186/s13750-015-0032-9
Bhagowati, B., and Ahamad, K. U. (2019). A review on lake eutrophication dynamics and recent developments in lake modeling. Ecohydrol. Hydrobiology 19 (1), 155–166. doi:10.1016/j.ecohyd.2018.03.002
Birk, S., Chapman, D., Carvalho, L., Spears, B. M., Andersen, H. E., Argillier, C., et al. (2020). Impacts of multiple stressors on freshwater biota across spatial scales and ecosystems. Nat. Ecol. Evol. 4, 1060–1068. doi:10.1038/s41559-020-1216-4
Cao, T., Ni, L. Y., Xie, P., Xu, J., and Zhang, M. (2011). Effects of moderate ammonium enrichment on three submersed macrophytes under contrasting light availability. Freshw. Biol. 56, 1620–1629. doi:10.1111/j.1365-2427.2011.02601.x
Cao, T., Xie, P., Li, Z. Q., Ni, L. Y., Zhang, M., and Xu, J. (2009). Physiological stress of high NH4+ concentration in water column on the submersed macrophyte Vallisneria natans L. Bull. Environ. Contam. Toxicol. 82, 296–299. doi:10.1007/s00128-008-9531-5
Chen, J., Liu, Z., Xiao, S., Chen, R., Luo, C., Zhu, T., et al. (2020b). Effects of benthivorous fish disturbance on chlorophyll a contents in water and the growth of two submersed macrophytes with different growth forms under two light regimes. Sci. Total Environ. 704, 135269. doi:10.1016/j.scitotenv.2019.135269
Chen, J., Su, H., Zhou, G., Dai, Y., Hu, J., Zhao, Y., et al. (2020a). Effects of benthivorous fish disturbance and snail herbivory on water quality and two submersed macrophytes. Sci. Total Environ. 713, 136734. doi:10.1016/j.scitotenv.2020.136734
Declerck, S., Vanderstukken, M., Pals, A., Muylaert, K., and De Meester, L. (2007). Plankton biodiversity along a gradient of productivity and its mediation by macrophytes. Ecology 88, 2199–2210. doi:10.1890/07-0048.1
Dorenbosch, M., and Bakker, E. S. (2012). Effects of contrasting omnivorous fish on submerged macrophyte biomass in temperate lakes: A mesocosm experiment. Freshw. Biol. 57 (7), 1360–1372. doi:10.1111/j.1365-2427.2012.02790.x
Erisman, J. W., Galloway, J. N., Seitzinger, S., Bleeker, A., Dise, N. B., Petrescu, A. M., et al. (2013). Consequences of human modification of the global nitrogen cycle. Phil. Trans. R. Soc. B 368 (1621), 20130116. doi:10.1098/rstb.2013.0116
Gao, J., Liu, L., Ma, N., Yang, J., Dong, Z., Zhang, J., et al. (2020). Effect of ammonia stress on carbon metabolism in tolerant aquatic plant-Myriophyllum aquaticum. Environ. Pollut. 263, 114412. doi:10.1016/j.envpol.2020.114412
Gao, J., Liu, Z. W., and Jeppesen, E. (2014). Fish community assemblages changed but biomass remained similar after lake restoration by biomanipulation in a Chinese tropical eutrophic lake. Hydrobiologia 724 (1), 127–140. doi:10.1007/s10750-013-1729-9
Goldsborough, L. G., and Wrubleski, D. A. (2001). “The decline of Delta Marsh, an internationally significant wetland in southcentral Manitoba,” in 6th prairie conservation and endangered species conference (Winnipeg, MB (CD-ROM).
Greaver, T. L., Clark, C. M., Compton, J. E., Vallano, D., Talhelm, A. F., Weaver, C. P., et al. (2016). Key ecological responses to nitrogen are altered by climate change. Nat. Clim. Chang. 6 (9), 836–843. doi:10.1038/nclimate3088
Gu, J., He, H., Jin, H., Yu, J., Jeppesen, E., Nairn, R. W., et al. (2018). Synergistic negative effects of small-sized benthivorous fish and nitrogen loading on the growth of submerged macrophytes – relevance for shallow lake restoration. Sci. Total Environ. 610-611, 1572–1580. doi:10.1016/j.scitotenv.2017.06.119
Hamilton, D. P., Salmaso, N., and Paerl, H. W. (2016). Mitigating harmful cyanobacterial blooms: Strategies for control of nitrogen and phosphorus loads. Aquat. Ecol. 50, 351–366. doi:10.1007/s10452-016-9594-z
He, H., Hu, E., Yu, J., Luo, X., Li, K., Jeppesen, E., et al. (2017). Does turbidity induced by Carassius carassius limit phytoplankton growth? A mesocosm study. Environ. Sci. Pollut. Res. 24, 5012–5018. doi:10.1007/s11356-016-8247-z
Huang, X. F., Chen, W. M., and Cai, Q. M. (1999). Standard methods for observation and analysis in Chinese ecosystem research network-survey, Observation and Analysis of Lake Ecology. Beijing: Standards Press of China.
Jeppesen, E., Brucet, S., Naselli-Flores, L., Papastergiadou, E., Stefanidis, K., Nõges, T., et al. (2015). Ecological impacts of global warming and water abstraction on lakes and reservoirs due to changes in water level and related changes in salinity. Hydrobiologia 750 (1), 201–227. doi:10.1007/s10750-014-2169-x
Jeppesen, E., Søndergaard, Ma., Søndergaard, Mo., and Christoffersen, K. (1998). “The structuring role of submerged macrophytes in lakes,” in Ecological studies (New York: Springer-Verlag).
Jing, M., Jia, X., Zhang, Y., Cao, J., Zhou, W., Wang, J., et al. (2020). Effects of long-term nitrogen addition on community aboveground and belowground biomass and their ratio in a typical steppe of Inner Mongolia. Chin. J. Ecol. 39, 3185–3193. (in Chinese).
Kaldy, J. E. (2014). Effect of temperature and nutrient manipulations on eelgrass Zostera marina L. from the Pacific Northwest, USA. J. Exp. Mar. Biol. Ecol. 453, 108–115. doi:10.1016/j.jembe.2013.12.020
Kloskowski, J. (2011). Impact of common carp Cyprinus carpio on aquatic communities: Direct trophic effects versus habitat deterioration. Fundam. Appl. Limnol. 178, 245–255. doi:10.1127/1863-9135/2011/0178-0245
Kosten, S., Kamarainen, A. M. Y., Jeppesen, E., Van Nes, E. H., Peeters, E. T. H. M., Mazzeo, N., et al. (2009). Climate-related differences in the dominance of submerged macrophytes in shallow lakes. Glob. Change Biol. 15 (10), 2503–2517. doi:10.1111/j.1365-2486.2009.01969.x
Li, W. (2014). Environmental opportunities and constraints in the reproduction and dispersal of aquatic plants. Aquat. Bot. 118, 62–70. doi:10.1016/j.aquabot.2014.07.008
Li, W., Jin, C., Guan, D., Wang, Q., Wang, A., Yuan, F., et al. (2015). The effects of simulated nitrogen deposition on plant root traits: A meta-analysis. Soil Biol. Biochem. 82, 112–118. doi:10.1016/j.soilbio.2015.01.001
Liu, E. S., Liu, Z. W., and Bao, C. H. (2007). The changes of catches of Carassius auratus and relationship with environment in Lake Taihu, China. J. Lake Sci. 19 (3), 345–350. (in Chhinese). doi:10.18307/2007.0318
Ma, S. N., Wang, H. J., Wang, H. Z., Li, Y., Liu, M., Liang, X. M., et al. (2018). High ammonium loading can increase alkaline phosphatase activity and promote sediment phosphorus release: A two-month mesocosm experiment. Water Res. 145, 388–397. doi:10.1016/j.watres.2018.08.043
Moss, B., Jeppesen, E., Søndergaard, M., Lauridsen, T. L., and Liu, Z. W. (2013). Nitrogen, macrophytes, shallow lakes and nutrient limitation: Resolution of a current controversy? Hydrobiologia 710 (1), 3–21. doi:10.1007/s10750-012-1033-0
Müller, I., Schmid, B., and Weiner, J. (2000). The effect of nutrient availability on biomass allocation patterns in 27 species of herbaceous plants. Perspect. Plant Ecol. Evol. Syst. 3, 115–127. doi:10.1078/1433-8319-00007
Netten, J. J. C., van der Heide, T., and Smolders, A. J. P. (2013). Interactive effects of pH, temperature and light during ammonia toxicity events in Elodea canadensis. Chem. Ecol. 29, 448–458. doi:10.1080/02757540.2013.769971
Nieoczym, M., and Kloskowski, J. (2014). The role of body size in the impact of common carp Cyprinus carpio on water quality, zooplankton, and macrobenthos in ponds. Int. Rev. Hydrobiol. 99, 212–221. doi:10.1002/iroh.201301644
Nimptsch, J., and Pflugmacher, S. (2007). Ammonia triggers the promotion of oxidative stress in the aquatic macrophyte Myriophyllum mattogrossense. Chemosphere 66, 708–714. doi:10.1016/j.chemosphere.2006.07.064
Nurminen, L., Horppila, J., Lappalainen, J., and Malinen, T. (2003). Implications of Rudd (Scardinius erythrophthalmus) herbivory on submerged macrophytes in a shallow eutrophic lake. Hydrobiologia 506, 511–518. doi:10.1023/b:hydr.0000008577.16934.a9
Olsen, S., Chan, F., Li, W., Zhao, S., Søndergaard, M., and Jeppesen, E. (2015). Strong impact of nitrogen loading on submerged macrophytes and algae: A long-term mesocosm experiment in a shallow Chinese lake. Freshw. Biol. 60, 1525–1536. doi:10.1111/fwb.12585
Parkos, J., Santucci, V. J., and Wahl, D. H. (2003). Effects of adult common carp (Cyprinus carpio) on multiple trophic levels in shallow mesocosms. Can. J. Fish. Aquat. Sci. 60, 182–192. doi:10.1139/f03-011
Poorter, H., Niklas, K. J., Reich, P. B., Oleksyn, J., Poot, P., and Mommer, L. (2012). Biomass allocation to leaves, stems and roots: meta-analyses of interspecific variation and environmental control. New Phytol. 193 (1), 30–50. doi:10.1111/j.1469-8137.2011.03952.x
Richardson, M. J., Whoriskey, F. G., and Roy, L. H. (1995). Turbidity generation and biological impacts of an exotic fish Carassius auratus, introduced into shallow seasonally anoxic ponds. J. Fish Biol. 47 (4), 576–585. doi:10.1111/j.1095-8649.1995.tb01924.x
Saunkaew, P., Wangpakapattanawong, P., and Jampeetong, A. (2011). Growth, morphology, ammonium uptake and nutrient allocation of Myriophyllum brasiliense Cambess. under high NH4+ concentrations. Ecotoxicology 20 (8), 2011–2018. doi:10.1007/s10646-011-0744-8
Shi, D., Zhuang, K., Chen, Y., Xu, F., Hu, Z., and Shen, Z. (2020). Effects of excess ammoniacal nitrogen (NH4+-N) on pigments, photosynthetic rates, chloroplast ultrastructure, proteomics, formation of reactive oxygen species and enzymatic activity in submerged plant Hydrilla verticillata (L.f.) Royle. Aquat. Toxicol. 226, 105585. doi:10.1016/j.aquatox.2020.105585
Simmons, B. I., Blyth, P. S. A., Blanchard, J. L., Clegg, T., Delmas, E., Garnier, A., et al. (2021). Refocusing multiple stressor research around the targets and scales of ecological impacts. Nat. Ecol. Evol. 5, 1478–1489. doi:10.1038/s41559-021-01547-4
Su, H., Chen, J., Wu, Y., Chen, J., Guo, X., Yan, Z., et al. (2019a). Morphological traits of submerged macrophytes reveal specific positive feedbacks to water clarity in freshwater ecosystems. Sci. Total Environ. 684, 578–586. doi:10.1016/j.scitotenv.2019.05.267
Su, H., Wu, Y., Xia, W., Yang, L., Chen, J., Han, W., et al. (2019b). Stoichiometric mechanisms of regime shifts in freshwater ecosystem. Water Res. 149, 302–310. doi:10.1016/j.watres.2018.11.024
Tan, X., Yuan, G., Fu, H., Peng, H., Ge, D., Lou, Q., et al. (2019). Effects of ammonium pulse on the growth of three submerged macrophytes. PLoS One 14, e0219161. doi:10.1371/journal.pone.0219161
Ti, C., Gao, B., Luo, Y., Wang, S., Chang, S. X., and Yan, X. (2018). Dry deposition of N has a major impact on surface water quality in the Taihu Lake region in southeast China. Atmos. Environ. 190, 1–9. doi:10.1016/j.atmosenv.2018.07.017
Ti, C., Xia, L., Chang, S. X., and Yan, X. (2019). Potential for mitigating global agricultural ammonia emission: A meta-analysis. Environ. Pollut. 245, 141–148. doi:10.1016/j.envpol.2018.10.124
Turschwell, M. P., Connolly, S. R., Schäfer, R. B., De Laender, F., Campbell, M. D., Mantyka-Pringle, C., et al. (2022). Interactive effects of multiple stressors vary with consumer interactions, stressor dynamics and magnitude. Ecol. Lett. 25, 1483–1496. doi:10.1111/ele.14013
Van de Bund, W. J., and Van Donk, E. (2004). Effects of fish and nutrient additions on food-web stability in a Charophyte-dominated lake. Freshw. Biol. 49, 1565–1573. doi:10.1111/j.1365-2427.2004.01301.x
Vanderstukken, M., Declerck, S. A. J., Decaestecker, E., and Muylaert, K. (2014). Long-term allelopathic control of phytoplankton by the submerged macrophyte Elodea nuttallii. Freshw. Biol. 59 (5), 930–941. doi:10.1111/fwb.12316
Wahl, D. H., Wolfe, M. D., Santucci, V. J., and Freedman, J. A. (2011). Invasive carp and prey community composition disrupt trophic cascades in eutrophic ponds. Hydrobiologia 678, 49–63. doi:10.1007/s10750-011-0820-3
Wang, J., Yu, D., and Wang, Q. (2008). Growth, biomass allocation, and autofragmentation responses to root and shoot competition in Myriophyllum spicatum as a function of sediment nutrient supply. Aquat. Bot. 89, 357–364. doi:10.1016/j.aquabot.2008.04.001
Xiao, K. Y., Yu, D., and Wang, J. W. (2006). Habitat selection in spatially heterogeneous environments: A test of foraging behaviour in the clonal submerged macrophyte Vallisneria spiralis. Freshw. Biol. 51, 1552–1559. doi:10.1111/j.1365-2427.2006.01590.x
Xie, D., Yu, D., You, W., and Wang, L. (2013). Morphological and physiological responses to sediment nutrients in the submerged macrophyte Myriophyllum spicatum. Wetlands 33, 1095–1102. doi:10.1007/s13157-013-0465-2
Yu, C., Huang, X., Chen, H., Godfray, H. C. J., Wright, J. S., Hall, J. W., et al. (2019a). Managing nitrogen to restore water quality in China. Nature 567 (7749), 516–520. doi:10.1038/s41586-019-1001-1
Yu, G., Jia, Y., He, N., Zhu, J., Chen, Z., Wang, Q., et al. (2019b). Stabilization of atmospheric nitrogen deposition in China over the past decade. Nat. Geosci. 12 (6), 424–429. doi:10.1038/s41561-019-0352-4
Yu, J. L., Liu, Z. W., He, H., Zhen, W., Guan, B., Chen, F., et al. (2016). Submerged macrophytes facilitate dominance of omnivorous fish in a subtropical shallow lake: Implications for lake restoration. Hydrobiologia 775 (1), 97–107. doi:10.1007/s10750-016-2717-7
Yu, Q., Wang, H. J., Wang, H. Z., Li, Y., Liang, X. M., Xu, C., et al. (2017). Does the responses of Vallisneria natans (Lour.) Hara to high nitrogen loading differ between the summer high-growth season and the low-growth season? Sci. Total Environ. 601-602, 1513–1521. doi:10.1016/j.scitotenv.2017.05.268
Yu, Q., Wang, H., Wang, H., Xu, C., Liu, M., Ma, Y., et al. (2022). Effects of High ammonium loading on two submersed macrophytes of different growth form based on an 18-month pond experiment. Front. Plant Sci. 13, 939589. doi:10.3389/fpls.2022.939589
Yu, Q., Wang, H. Z., Xu, C., Li, Y., Ma, S. N., Liang, X. M., et al. (2018). Higher tolerance of canopy-forming Potamogeton crispus than rosette-forming Vallisneria natans thigh nitrogen concentration as evidenced from experiments in 10 ponds with contrasting nitrogen Levels. Front. Plant Sci. 9, 1845. doi:10.3389/fpls.2018.01845
Yuan, G., Fu, H., Zhong, J., Qian, L., Ni, L., and Cao, T. (2016). Growth and C/N metabolism of three submersed macrophytes in response to water depths. Environ. Exp. Bot. 122, 94–99. doi:10.1016/j.envexpbot.2015.09.009
Yuan, G. X., Cao, T., Fu, H., Ni, L. Y., Zhang, X. L., Li, W., et al. (2013). Linking carbon and nitrogen metabolism to depth distribution of submersed macrophytes using high ammonium dosing tests and a lake survey. Freshw. Biol. 58, 2532–2540. doi:10.1111/fwb.12230
Zhang, M., Wang, Z., Xu, J., Liu, Y., Ni, L. Y., Cao, T., et al. (2011). Ammonium, microcystins, and hypoxia of blooms in eutrophic water cause oxidative stress and C–N imbalance in submersed and floating-leaved aquatic plants in Lake Taihu, China. Chemosphere 82, 329–339. doi:10.1016/j.chemosphere.2010.10.038
Zhang, Y., Jeppesen, E., Liu, X., Qin, B., Shi, K., Zhou, Y., et al. (2017). Global loss of aquatic vegetation in lakes. Earth. Sci. Rev. 173, 259–265. doi:10.1016/j.earscirev.2017.08.013
Keywords: growth form, submersed macrophytes, nitrogen loading, fish disturbance, interactive effects
Citation: Yu Q, Wang H, Liu M, Xu C, Ma Y, Guo W and Jeppesen E (2022) Interactive effects of benthivorous fish disturbance and ammonium loading on two submersed macrophytes of contrasting growth forms based on a mesocosm study. Front. Environ. Sci. 10:1024524. doi: 10.3389/fenvs.2022.1024524
Received: 21 August 2022; Accepted: 23 September 2022;
Published: 10 October 2022.
Edited by:
Doru Stelian Bănăduc, Lucian Blaga University of Sibiu, RomaniaReviewed by:
Xinhou Zhang, Nanjing Normal University, ChinaJian Cui, Institute of Botany, Jiangsu Province and Chinese Academy of Sciences, China
Copyright © 2022 Yu, Wang, Liu, Xu, Ma, Guo and Jeppesen. This is an open-access article distributed under the terms of the Creative Commons Attribution License (CC BY). The use, distribution or reproduction in other forums is permitted, provided the original author(s) and the copyright owner(s) are credited and that the original publication in this journal is cited, in accordance with accepted academic practice. No use, distribution or reproduction is permitted which does not comply with these terms.
*Correspondence: Haijun Wang, d2FuZ2hhaWp1bkB5bnUuZWR1LmNu; Weihua Guo, d2hndW9Ac2R1LmVkdS5jbg==