- 1Guangdong Provincial Key Laboratory of Agricultural and Rural Pollution Abatement and Environmental Safety, College of Natural Resources and Environment, South China Agricultural University, Guangzhou, China
- 2Key Laboratory of Tropical Agro-Environment, Ministry of Agriculture, South China Agricultural University, Guangzhou, China
- 3Key Laboratory of the Pearl River Estuarine Dynamics and Associated Process Regulation, Ministry of Water Resources, Pearl River Hydraulic Research Institute, Pearl River Water Resource Commission, Guangzhou, China
- 4National Key Laboratory of Water Environmental Simulation and Pollution Control, South China Institute of Environmental Sciences, Ministry of Environmental Protection of the People’s Republic of China, Guangzhou, China
Recycling nutrients in municipal sewage sludge (MSS) to soil would support sustainable development. In this study, a comprehensive recycling using specific plants able to grow in the fresh MSS and an indirect application technique was developed. Fresh MSS was placed in permeable containers next to Handroanthus chrysanthus plants to provide indirect fertilization. Sludge treatment plants (Alocasia macrorrhiza and Pennisetum hybridum) were grown directly on the Fresh MSS to produce plant biomass and treat MSS. The basal diameters of the H. chrysanthus plants were markedly increased by the treatment. Nutrients were extracted from MSS more readily and more biomass was produced by the P. hybridum than the A. macrorrhiza plants. The heavy metal contents of the soil did not increase significantly and not generate potential ecological risk, but the organic matter, nitrogen, and phosphorus contents increased markedly. The fresh MSS leachate met the relevant fecal coliform and heavy metal irrigation water standards. At the end of the treatment, the MSS mass had markedly decreased and the treated MSS was used as a seedling substrate for two garden plant seedlings. The net carbon emissions from the comprehensive recycling are estimated as -15.79 kg CO2e (CO2 equivalent) per ton fresh sludge, in contrast, the emissions from composting treatment are estimated as 8.15 kg CO2e. The method allows nutrients in MSS to be recycled without causing heavy metal pollution and without net carbon emission, while gives gardening products with commercial value.
1 Introduction
Municipal sewage sludge (MSS) is a solid waste produced during sewage treatment processes and contains large amounts of nutrients that could be utilized. However, MSS also contains relatively large amounts of harmful substances, and appropriate disposal of MSS is challenging but must be achieved to protect human and environmental health. Researchers around the world have been developing methods for treating and disposing of MSS for many years (Parr et al., 1978). Such methods involve phosphorus recovery, co-incineration, building material production, and anaerobic fermentation (Chang et al., 2020; Liang S. et al., 2021; Liang Y. et al., 2021; Iglesias-Iglesias et al., 2021; Zat et al., 2021; Ottosen et al., 2022). There are many excellent techniques for treating and disposing of MSS, but many are too expensive to be used in developing areas. The focus has previously been on disposing of MSS in economically developed areas, but MSS disposal in developing areas should not be ignored.
It is expensive to use advanced MSS treatment methods. Installing a MSS dehydration and incineration plant in China costs US$ 578,000/ (t MSS) before operating costs (Hao et al., 2019). There are additional costs involved in using MSS ash. Yu et al. (2021) calculated the cost of recovering phosphorus from MSS ash and found that, excluding the cost of producing the MSS ash and building a phosphorus recovery system, the reagents required to produce 1 kg of hydroxyapatite (Ca5(PO4)3OH) cost US$ 32.2 whereas producing 1 kg of hydroxyapatite from phosphate ore cost only US$ 1.0 ± 0.3. These methods may be able to be used in large developed cities but cannot be afforded in developing areas. Many areas in China and other developing countries are economically developing. Local economic conditions clearly need to be considered when selecting a MSS disposal technique (Lu et al., 2019).
Applying MSS to land is a cheap disposal technique that has been used for a long time (Guoqing et al., 2019). This returns soil-derived nutrients in MSS to the soil and creates a circular flow of nutrients (Gronman et al., 2016). Sludge applications in forests can be environmentally sound if application rates are matched to site characteristics (Zasoski et al., 1984). Badza et al. (2020) assessed the use of MSS in agricultural processes using the characteristics of MSS from 18 wastewater treatment plants in South Africa and found that applying MSS to land was the best option. However, hazardous substances in MSS pose risks to soil, plants, and humans. In particular, heavy metals in MSS tend to accumulate in soil (Zhang et al., 2021), can enter the food chain through surface water and groundwater, and can be absorbed by crops (Zeng et al., 2019), so direct application of MSS to land is controversial (Hei et al., 2016). Composting MSS does not decrease the total amount of heavy metals in the MSS (Wei Y. et al., 2020), so heavy metals can be released into the soil when MSS compost is applied to soil. Riaz et al. (2018) found Cd concentrations higher than the relevant limits in rice grown in soil to which MSS compost and MSS had been applied at an application rate of 1% of the soil mass.
The heavy metals contamination issue is being attracted much attention in China because the soils in south China are acid and the capacity for heavy metals is low. This was why the MSS compost is being banned since 2021 by the Chinese Ministry of Agriculture to be an “organic fertilizer” to apply to agricultural lands via the organic fertilizer standards (NY/T 525–2021). Accordingly, an indirect application technique (IAT) allowing MSS to be used to fertilize crops while preventing heavy metal contamination of the soil was proposed (Lin et al., 2021b). This technique uses permeable bags to contain the sludge and, after the release of nutrients such as N, p, K via leaching by rainfall or spread irrigation, the most part of heavy metals (less soluble than major nutrients) is recovered and taken away from agricultural lands with the solid residue of sludge. It has previously been found that nutrients leached more readily than heavy metals from MSS (Xu et al., 2015; Lin et al., 2021a). Considerably less heavy metal pollution therefore occurs when the IAT is used than when normal MSS application methods are used. It was found in a nine-year study that the IAT is a cheap and simple method for using MSS to fertilize crops that provides nutrients for plants such as bananas, papayas, and corn and does not cause the soil to become contaminated with heavy metals (Lin et al., 2021b). It has been found that specific plants (later called sludge treatment plants) can be grown on fresh MSS and that the plant products can safely be harvested and used. Samake et al. (2003) found that Alocasia macrorrhiza can be grown on MSS. It has been found that A. macrorrhiza stabilized MSS in ∼5 months, during which time the number of Escherichia coli markedly decreased and the cress seed germination index reached 100%. Hei et al. (2016) found that Pennisetum hybridum seedlings transplanted with substrate into fresh MSS survived and that the harvested P. hybridum was economically valuable. Combining the IAT with sludge treatment plants could allow MSS to be used to cheaply generate economic benefit while disposing of the MSS.
In this study, we developed a comprehensive recycling method to recycle MSS using the IAT and sludge treatment plants. The aim was to develop a low-carbon, cheap, and safe MSS disposal technique that will allow MSS to be treated of in developing areas. The fertilization effect and safety of the IAT were assessed at a high MSS application rate (720 t/ha fresh MSS) using the garden tree species Handroanthus chrysanthus. Sludge treatment plants (A. macrorrhiza and P. hybridum) were concurrently grown in the MSS and the products were harvested to give additional value. The treated MSS was then recycled and used as a substrate in a plant nursery to ensure that the resource was completely utilized. Finally, the carbon emission or the greenhouse gas emissions (GHGE) of the comprehensive recycling of MSS were also estimated.
2 Materials and methods
2.1 Experiment one: comprehensive treatment of MSS on land in a garden
2.1.1 Experimental site and materials
The experimental site, which had mountainous lateritic red soil, was in a forest of one-year-old H. chrysanthus. The site was ∼900 m2 and was far from any heavily populated areas (N 23o19′32.05″, E 113o46′5.79″). The MSS used in the experiment, later called MSS I, was collected at a sewage treatment plant in a residential area near the BYD Auto Co. Plant in Huizhou, Guangdong Province, China. The sewage treatment plant used the A2/O method to treat domestic sewage, and the MSS was dehydrated through mechanical compression. The physicochemical properties of MSS I are shown in Table 1. In southern China, there are less Hg and As in MSS, the main heavy metals with high contamination risk are Cd, Cu, Pb, and Zn (Guo et al., 2014), so this study focuses on these four heavy metals.
The P. hybridum plants were obtained from the Ecological Experiment Base of the South China Agricultural University (Guangzhou, Guangdong Province, China). Old stems of P. hybridum with similar diameters and strengths were selected, and stem segments with two tillering nodes were cut. Seedlings were cultivated in small pots for 2 weeks and then transplanted with the nursery substrate into the MSS used in the experiment. The A. macrorrhiza plants were obtained from the green belt of the South China Agricultural University. Newly grown A. macrorrhiza seedlings were selected and grown in small pots. Two weeks later, the seedlings were transplanted with nursery substrate into fresh MSS.
The MSS utilization method that was used is called an indirect application technique (IAT; Lin et al., 2021b). Briefly, a permeable mesh was laid over the soil, then a cylindrical container was formed on the permeable mesh using a root controlling material that is commonly used in gardens (Peter, 2000). MSS was added to the container to allow the MSS to indirectly fertilize the H. chrysanthus plants. Sludge treatment plants (A. macrorrhiza and P. hybridum) were planted in the MSS to further utilize the nutrients in the MSS (Figure 1).
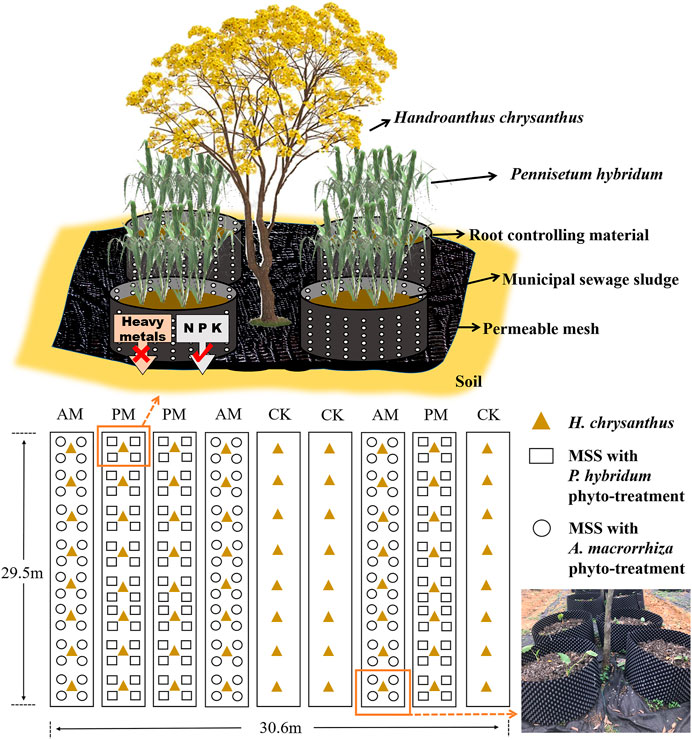
FIGURE 1. Layout of the experimental site and treatments (CK: control without MSS addition; AM: added MSS with A. macrorrhiza phyto-treatment; PM: added MSS with P. hybridum phyto-treatment).
The experimental design is shown in Figure 1. The ∼900 m2 forested land containing H. chrysanthus was divided into nine rows. Treatments were randomly assigned to the rows. Three rows were used for each treatment, and there were three treatments (control (CK), AM (indirect fertilization of H. chrysanthus and planting with A. macrorrhiza on MSS), and PM [(indirect fertilization of H. chrysanthus and planting with P. hybridum on MSS)]. The CK rows received no treatment, meaning natural H. chrysanthus growth occurred. For the AM and PM treatments, a black fiber mesh was laid on the ground and four sludge containers, each with a diameter of 1 m, were placed around each tree. Fresh MSS (250 kg) was added to each container, and 4 A. macrorrhiza or P. hybridum seedlings were planted in the fresh MSS (about five plants per m2 of sludge surface). The surface of the fresh MSS was covered with 1 kg of mature composted MSS after planting to prevent harmful organisms and gases being released to the air. MSS was applied to 48 trees, and 48 t of MSS was used to treat the trees. The basal diameter, diameter at breast height, height, and crown width of each H. chrysanthus tree were determined before and after the experiment to assess growth.
2.1.2 Sample collection and analysis
Surface soil (0–20 cm) and MSS samples were collected from the experimental area before and after the experiment. The N and p contents of the soil and MSS samples were determined using established methods (Lu, 2000). The Cd, Cu, Pb, and Zn contents of the soil and MSS were determined by atomic absorption spectrophotometry after digestion in a microwave according to a Chinese environmental standard method (HJ 832–2017, HJ means the method is certified by Ministry of Environmental Protection of China) and using the standard substance (GBW-07405: GSS-5) for analysis quality control. The germination index (GI) of MSS was determined using a method proposed by the Chinese sludge standards (GB/T 23,486–2009) using the Chinese cabbage seed germination test. This test is similar to the cress seed germination test, which is internationally recognized as a measure of the phytotoxicity of the sludge material (Zucconi et al., 1981), and calculates this parameter using the following equation:
GI = (seed germination rate (%) X root length) of treatment/ (seed germination rate (%) X root length) of water control.
The fecal coliforms in MSS were detected using the fermentation method (GB 7959–2012, GB means the method is National Standard Method of China).
Once the experiment had started, samples of A. macrorrhiza and P. hybridum grown on MSS were collected from random four sludge container under two H. chrysanthus trees per treatment in months 1, 2, 3, and 6 to allow the optimal harvest times for A. macrorrhiza and P. hybridum to be determined. Plant samples collected in month 6, were deactivated at 105°C for 30 min, and then dried at 65°C for 48 h. The dry samples were ground and then the nutrient and heavy metal contents were determined. The plant samples were digested in H2SO4 and H2O2 and then the N and p contents were determined using the methods proposed by Chinese Society of Soil Science (Lin et al., 2021b). The K and heavy metal contents (Cd, Cu, Pb, and Zn) of the plant samples were determined by atomic absorption spectrophotometry (GB/T 5009.15–2014) and using a standard substance (GBW-10013: GSB-4) for quality control.
2.1.3 Evaluation of the potential ecological risk of soil heavy metals
Hakanson potential ecological risk assessment was used to assess the risk of heavy metal pollution in the soil (Hakanson, 1980). The potential ecological risk index for single heavy metal (E) and all heavy metals (RI) were calculated using the following equation:
where Ci is the measured value of soil heavy metal i; Cs is the reference value (the natural background value of soil in Guangzhou city, they are 0.14, 13.6, 42.9, and 58.1 mg/kg for Cd, Cu, Pb, and Zn, respectively); and Ti is the toxicity coefficient of heavy metal i (Cd = 30, Cu = 5, Pb = 5, and Zn = 1).
The heavy metals evaluated in this study were different from the original method, so a correction was required (Li et al., 2015). Among the four heavy metals, Cd has the largest T (30), according to which the limit value of the first grading standard (Low) of E in this study is 30, and the limit value of the grading standard of each grade of E is obtained by multiplying by two in turn. In the original method, the first level limit value of RI (150) is divided by the sum of all heavy metals E (133) to obtain the coefficient 1.13. The sum of four heavy metals E in this study is 41, multiplying 41 by 1.13 and taking tens place, the first level limit value of RI in this study can be obtained (1.13 × 41 = 46.33 ˜ 50), which is multiplied by two in turn to obtain each level of RI. The corrected grading criteria used to define ecological risk of this study are shown in Table 2.
2.2 Experiment 2: simulation of leaching from MSS
MSS I contained a relatively low number of fecal coliforms, so it did not represent the health risks posed by IAT of most MSS. A leaching experiment was therefore performed using a sample called MSS II that contained larger amounts of harmful microorganisms (with a fecal coliform bacteria value of <0.01; Table 1). Fecal coliforms and the heavy metal concentrations in the leachate samples were determined and compared with the Chinese national standard for irrigation water quality (GB 5084–2021) to indirectly determine whether fresh MSS that did not meet sanitary indicator standards could be used in the IAT.
MSS II was collected from the Heshan Sewage Treatment Plant in Jiangmen, Guangdong Province. The experimental site was at the Ecological Farm of the South China Agricultural University in Tianhe District, Guangzhou (N 23o09′57.21″, E 113o21′37.78″). The experimental system (shown in Supplementary Figure S1) consisted of a leachate collection vessel with an upper surface area of 0.12 m2 on which 5 kg of fresh MSS II in a black fiber mesh bag was placed. Three control vessels without MSS bag were also set-up to collect rainwater. The experiment started on 13 April and rainwater was collected for the first time on 16 April. Leachate was collected three times in 30 days. The pH, chemical oxygen demand, fecal coliform concentration, and Cd, Cu, N, p, Pb, and Zn concentrations in the rainwater and leachate samples were determined. The pH was determined using a glass electrode. Fecal coliforms were detected using a rapid paper strip method (HJ 755–2015). The chemical oxygen demand was determined using the dichromate method (HJ 828–2017). The Cd, Cu, Pb, and Zn concentrations were determined by atomic absorption spectrophotometry (GB 7475–1987).
2.3 Experiment 3: investigation of follow-up utilization of MSS
At the end of Experiment one, Jasminum sambac and H. chrysanthus seedlings were planted in the MSS that had been treated by growing plants in it to investigate follow-up recycling uses of MSS. J. sambac seedlings 15 cm tall were purchased from Jianye Jasmine Base in Nanning, Guangxi, China. H. chrysanthus seedlings 50–60 cm tall were obtained from the Garden Company of the South China Agricultural University. The J. sambac plants were planted on Xinwei Road in Zengcheng District, Guangzhou (N23°19′32.05″, E113°46′5.79″), and the H. chrysanthus seedlings were planted at the Ecological Farm of the South China Agricultural University (N23°09′57.21″, E113°21′37.78″).
In the experiment using J. sambac, it was grown in two types of treated MSS (AM and PM) (10 kg/pot), one that had previously been used to grow P. hybridum (i.e., from the Experiment I PM group) and the other that had previously been used to grow A. macrorrhiza (i.e., from the Experiment I AM group). J. sambac was also grown in control soil (CK) (10 kg/pot), which was soil collected from a mountain near the experimental site. For each treatment, 10 replicates were performed. The J. sambac plants were grown for 3 months. At the end of the experiment, J. sambac plant survival and height were recorded.
In the experiment in which H. chrysanthus seedlings were planted, 20 kg of the treated MSS was mixed with 20 kg of soil to investigate whether a mixture of MSS and soil could be used as a seedling substrate. Three treatments were performed: 1) CK: Control, soil (40 kg) from local ordinary farmland without fertilizer or sludge. 2) CF: soil (40 kg) fertilized with chemical fertilizers (4 g N, 4 g P2O5, and 4 g K2O added with a 15–15–15 compound fertilizer). 3) PM: mixture of soil and an equal mass of MSS on which P. hybridum plants had previously been grown (i.e., from the Experiment I PM group). Four replicates of each treatment were performed. The plants were grown for 6 months. The H. chrysanthus plant heights were recorded once each month.
2.4 Net greenhouse gas emissions estimation
Greenhouse gas emissions (GHGE), also called carbon emissions, are generally calculated in terms of carbon dioxide equivalents (CO2e). Net GHGE consist of three main components: direct carbon emission, indirect carbon emission and carbon emission reduction. The comprehensive treatment proposed in this research use green plants and sunlight, might have less carbon emission, and the P. hybridum produced in the treatment process could be used as fuel biomass, which could replace fossil fuels to achieve carbon reduction. Similar to aerobic composting treatment, the comprehensive treated MSS could use as an organic fertilizer. Therefore, comparing the net GHGE of the comprehensive recycling with the aerobic composting treatment can measure the carbon emission level of the integrated method. This study assessed the net GHGE according to the accounting guidelines given in Intergovernmental Panel on Climate Change (IPCC, 2019).
2.4.1 Direct carbon emission
MSS may release CH4 and N2O during the treatment process. Therefore, the direct carbon emissions in the treatment process are calculated as follows:
Where:
2.4.2 Indirect carbon emission
The treatment of MSS consumes fuel and electricity, which indirectly generates carbon emissions are calculated as follows:
Where:
2.4.3 Carbon Reduction
Both treatments are capable of producing fertilizer that can replace the carbon emissions from the production of chemical fertilizers, calculated as follows:
Where:
As an energy plant, the use of P. hybridum as a fuel can offset carbon emissions from fossil fuel combustion, calculated as follows:
Where:
2.5 Statistical analysis
SPSS 22 (SPSS Inc., Chicago, IL, United States) was used to conduct statistical analyses. The normality of distribution and homogeneity of variance were tested before performing ANOVA. Significant differences between the treatments were analyzed using ANOVA and Duncan’s multiple range tests. Significance was accepted at p < 0.05. For the principal component analysis (PCA), Bray-Curtis dissimilarities were calculated using the vegan package (v.2.5.4) in R (v.3.6.3). Barplot was generated using the ggplot2 package (v.3.3.3).
3 Results
3.1 Growth of H. chrysanthus
We measured the basal diameters, diameters at breast height, heights, and crown widths of the H. chrysanthus trees before and after the experiment and then calculated the amounts by which the four measurements had increased. The results are shown in Table 3. After a year, the basal diameters and diameters at breast height had increased by only 2.13 and 1.43 cm, respectively, for the trees in the control (CK), but 3.06 and 2.61 cm for the trees in the AM treatment, 3.26 and 2.83 cm for the trees in the PM treatment. The basal diameters and diameters at breast height for the trees in the three treatments were significantly different (n = 24, p = 0.05). The crown widths and tree heights were also higher for the trees treated with MSS than for the CK trees but the differences were not significant (n = 24, p = 0.05). We concluded that the IAT and sewage plant treatment had a favorable effect on H. chrysanthus growth.
3.2 Effects on the sludge treatment plants
3.2.1 Optimal sludge treatment plants harvest times and yields
The sludge treatment plants needed to be harvested regularly to maximize MSS utilization. The planting times that gave the highest plant yields were identified by harvesting and weighing the sludge treatment plants at different times. The A. macrorrhiza and P. hybridum plants were harvested and weighed in months 1, 2, 3, and 6. The yields in months 1, 2, 3, and 6 were converted into annual yields by multiplying the yields by factors of 12, 6, 4, and 2, respectively, to allow the yields to be compared. P. hybridum harvested in month three gave the highest annual yield (100 ± 25 t/ha). A. macrorrhiza harvested in month 6 gave the highest annual yield (9.0 ± 1.4 t/ha). The annual yields at the same harvesting times were significantly higher for P. hybridum than A. macrorrhiza (n = 4, p = 0.05). For example, the annual yield was 18.5 times higher for P. hybridum than A. macrorrhiza when the plants were harvested in month 3 (Supplementary Figure.S2). This indicated that more biomass could be harvested if P. hybridum was planted than if A. macrorrhiza was planted in the MSS.
3.2.2 Nutrient extraction by the sludge treatment plants
The measured K, N, and p contents (dry weight) of the P. hybridum biomass were 27.12 ± 7.15, 15.11 ± 3.67, and 5.56 ± 1.42 g/kg, respectively, and the K, N, and p contents of the A. macrorrhiza biomass were 45.40 ± 10.37, 27.84 ± 1.77, and 5.31 ± 0.07 g/kg, respectively. Under the optimal harvest time as previously mentioned (annual yield 100 and 9.0 t/ha respectively for P. hybridum and A. macrorrhiza), the P. hybridum plants extracted annually 2712, 1511, and 556 kg/ha of K, N, and p, respectively, from the MSS. The A. macrorrhiza plants extracted 408.6, 250.6, and 47.8 kg/ha of K, N, and p, respectively. More than 6.6, 6.0 and 11.6 times of K, N, and p were extracted when P. hybridum was planted than when A. macrorrhiza was planted in the MSS, meaning P. hybridum is more suitable than A. macrorrhiza for use as a sludge treatment plant to recycle the MSS nutrients into animal feed or organic fertilizer.
3.2.3 Heavy metal contents of the sludge treatment plants
Both A. macrorrhiza and P. hybridum are inedible by humans, so the risks posed to humans by directly planting A. macrorrhiza and P. hybridum in MSS are relatively low. However, we determined the heavy metal contents (dry weight) of the A. macrorrhiza and P. hybridum plants to evaluate possible subsequent uses of the sludge treatment plant biomass. The Cd, Cu, Pb, and Zn contents of the P. hybridum dry biomass were 0.082 ± 0.015, 5.88 ± 0.11, 0.50 ± 0.02, and 38.21 ± 2.22 mg/kg, respectively. The Cd, Cu, Pb, and Zn contents of the A. macrorrhiza dry biomass were 0.568 ± 0.121, 9.26 ± 0.90, 2.32 ± 0.97, and 196.53 ± 4.76 mg/kg, respectively. The Cd, Cu, Pb, and Zn contents were lower for A. macrorrhiza than P. hybridum. The Cd and Pb contents of the P. hybridum and A. macrorrhiza biomass were below the relevant limits for organic fertilizers (Cd ≤ 3 mg/kg, Pb ≤ 50 mg/kg; standard NY 525–2021, Chinese Ministry of Agriculture) and feed (Cd ≤ 1 mg/kg, Pb ≤ 10 mg/kg; standard GB 13078–2017). This indicated that the sludge treatment plant biomass produced on the MSS was safe, in terms of the Cd and Pb contents, for use as animal feed or as raw material for producing organic fertilizer.
3.3 Effects on the soil
3.3.1 Effects on the soil pH and heavy metal contents
After 1 year of the comprehensive treatment, there were no significant differences in the soil pH and the Cd, Cu, Pb, and Zn contents of the soil for the three treatments (n = 3, p = 0.05, Table 4). The Cd, Cu, Pb, and Zn contents of the soil in the AM and PM treatment areas were all below the relevant Chinese soil environmental quality standard limits (GB 15618–2018). As shown in Figure 2A, principal component analysis indicated that there were no differences between the results for the CK, AM, and PM treatments after 1 year, indicating that the IAT and sludge treatment by plants posed little risk of soil pollution.
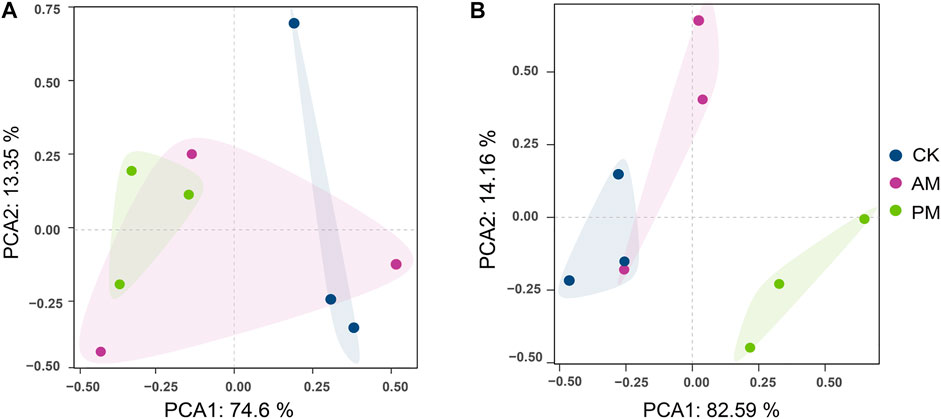
FIGURE 2. The effect of comprehensive treatment on soil, where: (A) shows the principal component analysis of heavy metals (Cd, Cu, Pb, Zn) in soil after the experiment; (B) shows the principal component analysis of soil nutrients (N, P, OM, K) after the experiment.
The assessment of the potential ecological risk of the soil also indicated that the comprehensive treatment poses little risk of soil contamination. According to Eqs 1, 2, ECd, ECu, EPb, and EZn for the AM treatment were 14.14, 4.69, 5.70, and 1.27, respectively, and the RI was 25.80; ECd, ECu, EPb, and EZn for the PM treatment were 16.50, 5.26, 6.00, and 1.37, respectively, and the RI was 29.13. Comparing the corrected grading criteria (Table 2), it can be found that the potential ecological risk to the soil for both treatments is the lowest grade.
3.3.2 Effects on soil nutrients
In the year in which the comprehensive treatment was applied, H. chrysanthus grew well while the N and p contents of the soil also increased significantly in the PM treatment areas than in the CK area, as shown in Table 4. However, the K content did not follow the same trend because the K content was lower for the MSS than the soil (Table 1). The principal component analysis indicated that, in general, the K, N, OM, and p contents of the soil after the experiment were different for the three treatments (Figure 2B) indicating that different amounts of nutrients were leached from the MSS into the soils of the different treatments.
3.4 Changes in MSS properties
3.4.1 Decreases in MSS mass
Each H. chrysanthus plant was treated with 1000 kg of MSS. After 1 year, the total remaining MSS masses for the AM and PM treatments were determined and the decreases in the MSS masses were calculated. The MSS masses in the AM and PM treatments had decreased by 84.42% and 85.37%, respectively, at the end of the year. The IAT and sludge treatment plants treatment transformed the fresh MSS into a soil-like substrate (Supplementary Figure.S3) that still had nutrient value, as shown in Table 4, and could be conveniently transported for further use.
3.4.2 Changes in the MSS pH and heavy metal contents
The pH of the MSS I decreased markedly after it had been applied, as shown in Table 4. The change in pH would have been affected by various factors, including precipitation and OM mineralization and nitrification. Plant root exudates could also have changed the MSS pH (Xu and Coventry, 2003). The heavy metal contents of the MSS increased during the experiment, as shown in Table 4. There were no marked differences between the heavy metal contents of the MSS in the AM and PM treatments. A. macrorrhiza and P. hybridum could have absorbed heavy metals and decreased the heavy metal contents of the MSS. However, A. macrorrhiza and P. hybridum are not hyperaccumulators, so they would not have absorbed very large amounts of heavy metals. Decomposition of OM and leaching of nutrients would have decreased the MSS mass and would have increased the heavy metal contents of the MSS. However, the Cd, Cu, Pb, and Zn contents of the MSS after the plant treatments were lower than the legal limits for MSS to be used in gardens or parks in China (GB/T 2348–2009).
3.4.3 Nutrient and hygiene indicators
Using the recovered MSS as a substrate for growing seedlings required the nutrient and sanitary indicators of the MSS to be assessed. The OM content of the MSS in the AM and PM treatments after 1 year of indirect MSS utilization and plant treatment was ∼400 g/kg (i.e., >250 g/kg; Table 4). The total nutrient (N + P2O5+K2O) content of the treated MSS was ∼94 g/kg (i.e., >30 g/kg). There were no significant differences between the OM and total nutrient contents of the AM- and PM-treated MSS. The nutrient contents of the AM- and PM-treated MSS met the requirements for using the treated MSS in landscaping applications.
The seed germination index (GI) was used to indicate the toxicity of the MSS to plants. The AM and PM treatments strongly improved the GI. The GIs for the AM and PM treatments were 0.87 ± 0.16 and 0.77 ± 0.24, respectively, whereas the GI of the raw MSS was 0.08 ± 0.09, as shown in Table 4. The GIs for the AM- and PM-treated MSS met the requirements for the MSS to be used in gardens or parks in China (GB/T 2348–2009).
The fecal coliform bacteria value (FC) is the minimum sample size required to allow coliforms to be detected. The larger the FC the cleaner the MSS. The Chinese “Quality of Sludge Used in Gardens or Parks” standard (GB/T 2348–2009) stipulates that the FC for MSS must be ≥ 0.01 (equivalent to <100 MPN/g). The FCs for the AM- and PM-treated MSS after 1 year were 0.03 ± 0.00 and 0.11 ± 0.05, respectively. The FC for the PM-treated MSS was significantly different from the FCs for the AM-treated and untreated MSS (n = 3, p = 0.05). This indicated that the PM treatment markedly improved the sanitary level of the MSS.
3.5 Harmful substances in the MSS leachate
It can be seen from Table 1 that the FC for MSS II was above the relevant limit of 0.01 in standard GB/T 2348–2009 and reached 0.0005 ± 0.0003 (equivalent to 2.0×103 MPN/g). However, as shown in Table 5, the fecal coliform concentration in the first leachate was only 160 MPN/L. The fecal coliform concentration limit specified in the Chinese standard for irrigation water (GB 5084–2021) is 4.0×104 MPN/L. This indicated that microorganisms were retained well by the MSS in the mesh package because the fecal coliform concentration was expected to be higher for the first MSS leachate than for later MSS leachates.
The Cu content of the MSS II, 3602 ± 100 mg/kg (Table 1), was higher than the limit specified in Chinese standard GB/T 2348–2009. The Cu concentration in the leachate caused by rainfall was only 0.584 ± 0.285 mg/L (Table 5), which was lower than the Chinese limit of 1 mg/L for irrigation water (standard GB 5084–2021). The Cd, Pb, and Zn concentrations in the leachates were very low. Similar results were found in a previous study. Xu et al. (2015) performed multiple leaching tests lasting up to 9 months (n = 30) using polluted MSS with a Cd content of 4.92 ± 0.09 mg/kg. They found low Cd concentrations of between 0 and 0.00156 mg/L in the leachate.
3.6 Subsequent MSS utilization
3.6.1 Growth of J. sambac planted in treated MSS
The MSS that had been used to grow A. macrorrhiza and P. hybridum in experiment one (see section 2.1) was used to grow J. sambac plants. The J. sambac plants grown in the AM- and PM-treated MSS survived well, the survival rates all being 100% (n = 10). The J. sambac seedlings were ∼15 cm tall at the beginning of the experiment. Three months after being planted, the J. sambac plants grown in the AM- and PM-treated MSS were >70 cm tall (70.11 ± 4.58 and 75.35 ± 13.11 cm, respectively) and the plants grown in soil were only ∼50 cm tall (49.33 ± 6.66 cm). The J. sambac plants grown in the AM- and PM-treated MSS were significantly taller than the J. sambac plants grown in soil (n = 10, p = 0.05).
3.6.2 Growth of H. chrysanthus seedlings fertilized with treated MSS
The heights of the H. chrysanthus plants from the three treatments are shown in Figure 3. The seedling heights were significantly different for the different treatments in month 4 (n = 4, p = 0.05). The plants from the chemical fertilizer treatment and PM (i.e., P. hybridum treated MSS) treatment were significantly taller than the plants from the CK treatment, but the plants from the chemical fertilizer and PM treatments were not significantly different (n = 4, p = 0.05). The PM-treated MSS performed as well as or better than the chemical fertilizer treatment, indicating that P. hybridum treated MSS provided a good degree of fertilization.
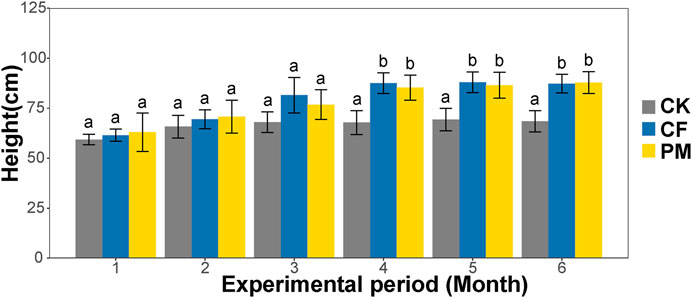
FIGURE 3. Plant heights of H. chrysanthus seedlings grown in soil with or without sludge addition (CK: the seedlings were grown in soil; CF: the seedlings were grown in soil with chemical fertilizers; PM: the seedlings were grown in soil amended by an equal mass of P. hybridum phyto-treated sludge. Different letters indicate that the difference was significant in the height between the treatments according to Duncan’s multiple range test (n = 4, p = 0.05)).
3.7 Carbon emission
The net GHGE is estimated for 1 t fresh MSS. The properties of MSS were referred to MSS I (87.98% water content, 43.56% organic matter, and 4.26% total nitrogen), and the MSS reduction was 85% for the comprehensive recycling and 63% was taken for the composting treatment (Liu et al., 2014). Only the carbon emissions in the treatment processing are considered, excluding the carbon emissions generated during the sludge concentration and dewatering in sewage treatment plant and the subsequent utilization/ application of sludge products.
The emissions of CH4 from aerobic composting treatment can be taken as 0.2 kg/t (Wang et al., 2022), and are negligible in the comprehensive treatment due to the small volume of MSS in each planting unit. The emission of N2O during aerobic composting was taken as 0.043 kg/t (Liu et al., 2014). The denitrification of the comprehensive treatment is weak, and the production of N2O should not exceed that of the composting treatment. The value taken is consistent with that of composting, 0.043 kg/t. Therefore, according to the calculation of Eq. (3), the direct carbon emission from composting treatment is 17.81 kg CO2e, and the comprehensive treatment is 12.81 kg CO2e.
The garden forestry site of the comprehensive treatment is usually farer than that of composting site, so the transport distance for the comprehensive treatment is taken as 50 km, while the composting treatment is taken as 30 km. The composting process consumes electrical energy with a consumption of about 10.06 kWh/t (Liu et al., 2014), while the comprehensive treatment has no electrical energy consumption. Therefore, the indirect carbon emission of composting treatment is 9.67 kg CO2e and the comprehensive treatment is 2.63 kg CO2e, calculated according to Eq. (4).
According to the reduction rate of MSS after treatment, the sludge mass after composting is 370 kg, and the comprehensive recycling is 150 kg. The loss rate of N in composting treatment is about 40% (Wang et al., 2019), then the N content of composting treatment is calculated as 2.56%, and the comprehensively treated MSS is measured as 2.60%. In addition, the comprehensive recycling is capable of producing plant products, for example, 1 t fresh MSS can produce about 16 kg of dry matter in the case of P. hybridum. Therefore, according to the calculation of Eqs 5, 6, the carbon reduction of composting treatment is -19.33 kg CO2e and the comprehensive recycling is -31.22 kg CO2e.
As a result, the carbon emissions of the two treatments are shown in Figure 4. The net GHGE from the composting treatment was 8.15 kg CO2e and the comprehensive recycling was -15.79 kg CO2e. This means that the comprehensive recycling of MSS is carbon-negative and contributes to the reduction of carbon emission.
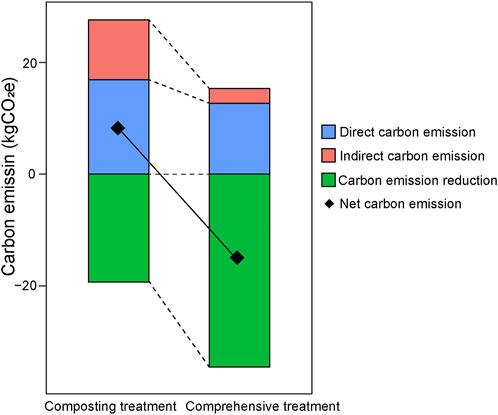
FIGURE 4. Comparison of the carbon emissions of a composting treatment and the comprehensive treatment.
4 Discussion
Comparison between the comprehensive recycling and the conventional land application in environmental benefits.
The comprehensive recycling could fully utilize the nutrients in fresh MSS. A water-permeable material was used to separate the MSS from the soil. Nutrients could be leached from the MSS for use by the H. chrysanthus trees but heavy metals were mainly retained by the MSS. The method effectively decreased the amounts of heavy metals that were released from the MSS into the soil (Lin et al., 2021b). In addition, both A. macrorrhiza and P. hybridum could strongly grow on the MSS, so these species could be used to treat the MSS and therefore offer economic benefits. The conventional method for utilizing MSS on land is the application of composted MSS. Organic pollutants in MSS can be decomposed during the composting process (Zhang et al., 2019) and the bio-availabilities of heavy metals can decrease (Chen et al., 2019). However, composting cannot fundamentally solve the problems caused by heavy metals in MSS. Applying MSS compost can still cause heavy metals to accumulate in soil and plants (Papafilippaki et al., 2015). Repeated applications of MSS compost to soil can markedly increase the amounts of heavy metals leached from the MSS-treated soil and pose the risk of underground water becoming polluted (Fang et al., 2017). The comprehensive treatment could decrease the amounts of heavy metals released into the soil. Our results indicated that the heavy metal contents of the soil did not increase markedly when large amounts of MSS were utilized by comprehensive treatment, as shown in Figure 2A and Table 4.
China has currently proposed two goals of carbon peaking and carbon neutrality, which set a higher standard for how MSS should be treated. The carbon emissions of composting treatment and the comprehensive recycling were also calculated. The emission of N2O of the comprehensive treatment was set to equal to aerobic composting and the emissions of CH4 was omitted, due to the lack of available data. Wang et al. (2022) calculated net carbon emissions from different treatment processes for MSS (40–50% OM) and found that composting treatment had lower net carbon emissions than landfills, incineration, pyrolysis, and anaerobic digestion. This study shows that the net carbon emissions of the comprehensive recycling are even lower than those of composting (Figure 4). The comprehensive recycling achieves carbon reduction and is conducive to achieving the dual carbon goals.
In addition, the comprehensive treatment also facilitated the fixation of CO2 by H. chrysanthus. The carbon stock was estimated to be 50% of the total biomass (IPCC 2005) which consist of above ground biomass (AGB) and below ground biomass (BGB). The BGB was estimated from AGB using a root-shoot ratio of 0.26 and the AGB of the trees can be estimated as Khadanga and Jayakumar (2020) proposed. The formula is as follows:
where
According to Table 3, the diameter at breast height was from 7.05 ± 0.50 cm to 8.41 ± 0.64 cm for the CK treatment, and was from 6.72 ± 0.51 cm to 9.47 ± 0.89 cm for the PM treatment. Therefore, according to Eq. (7), the increased biomass of one H. chrysanthus tree in the CK treatment can be estimated as 20.41 kg and the PM treatment can be estimated as 43.89 kg. The biomass of each H. chrysanthus tree in the PM treatment increased by 23.48 kg over the CK treatment. The planting density of the PM treatment is 800 plants/ha, which means an additional 18.78 t of biomass and 9.39 t of carbon sequestration per hectare of land can be obtained. The comprehensive recycling achieves more carbon fixation, which likewise contributes to the dual carbon goal.
Comparison between the comprehensive recycling and the conventional land application in economic benefits.
The proposed comprehensive recycling does not require a large investment but does require a large area of garden forestry lands (including fruit forestry). However, the garden forestry lands do not affect its productivity by additional sludge phyto-treatment, the garden trees even grow better than the control as shown in this study. In addition, the comprehensive recycling can produce economic benefits in a number of ways: 1) Nutrients for garden plants are provided, meaning smaller amounts of mineral fertilizers need to be purchased; 2) The system markedly improves the nutrient contents of the soil under the indirect application device and improves the soil; 3) Sludge treatment plants growing in the MSS can be used as organic fertilizer or animal feed or plant fuel even for papermaking; 4) After the utilization process is complete, the MSS is fully mature and can be used as organic fertilizer or substrate for garden plants; 5) Carbon emission reductions from MSS treatment can be traded in the carbon trading market.
The main methods to dispose MSS are land application, incineration, disposal in sanitary landfills and making building materials, which accounted for 29.3%, 26.7%, 20.1%, and 15.9%, respectively, of the total amount of MSS disposed of in China in 2019 (Wei L. et al., 2020). The comprehensive recycling used in this study is in the ‘land application’ category. The ultimate goal of any land application system is to make use of the nutrients in MSS, it means less expenditure on chemical fertilizers and more economic value generation. Gouin (1985) reported that composted sewage sludge could replace a complete fertilizer to get comparable growing of Chrysanthemums. The comprehensive recycling reuses directly and comprehensively the nutrients in fresh MSS, and is suitable in gardening developed areas such as Guangdong Province of China.
5 Conclusion
The main conclusions are drawn as follows:
(1) The comprehensive recycling increased the growth of H. chrysanthus. Good levels of biomass production and nutrient extraction were achieved when P. hybridum was planted in fresh sludge and harvested in month 3.
(2) The heavy metal contents of the soil did not markedly increase during the experiments but the N, OM, and P contents increased markedly, the comprehensive recycling has little impact on potential ecological risk to the soil.
(3) The MSS became mature by the end of the process and was suitable for use as a substrate for cultivating flower seedlings.
(4) Even fresh MSS that exceeded the relevant standards gave leachate with fecal coliform and Cu concentrations that met the standards for irrigation water.
(5) The comprehensive recycling, which has lower carbon emissions than composting, is carbon-negative and suitable in gardening developed areas.
Data availability statement
The original contributions presented in the study are included in the article/Supplementary Material, further inquiries can be directed to the corresponding author.
Author contributions
XL: Investigation, Writing – original draft, Formal analysis. CC: Investigation. HL: Methodology. LH: Resources. LZ: Visualization. ZW: Formal analysis. YC: Supervision. Q-TW: Conceptualization, Writing – review and; editing, Funding acquisition.
Funding
This study was supported by the R & D program of Guangdong Provincial Department of Science and Technology (Grant No. 2018B030324003, 2021B1212040008, 20220307); Local Innovation and Entrepreneurship Team Project of Guangdong Special Support Program, China (Grant No. 2019BT02L218); the Water Resources Innovation Project of Guangdong Province, China (Grant No. 2017-29); the National Natural Science Foundation of China (Grant No. 21606092).
Acknowledgments
We thank Gareth Thomas, PhD, from Liwen Bianji, Edanz Group China, for editing the English text of a draft of this manuscript.
Conflict of interest
The authors declare that the research was conducted in the absence of any commercial or financial relationships that could be construed as a potential conflict of interest.
Publisher’s note
All claims expressed in this article are solely those of the authors and do not necessarily represent those of their affiliated organizations, or those of the publisher, the editors and the reviewers. Any product that may be evaluated in this article, or claim that may be made by its manufacturer, is not guaranteed or endorsed by the publisher.
Supplementary material
The Supplementary Material for this article can be found online at: https://www.frontiersin.org/articles/10.3389/fenvs.2022.1023356/full#supplementary-material
References
Badza, T., Tesfamariam, E. H., and Cogger, C. G. (2020). Agricultural use suitability assessment and characterization of municipal liquid sludge: Based on South Africa survey. Sci. Total Environ. 721, 137658. doi:10.1016/j.scitotenv.2020.137658
Castellanos, S., and Ramón, J. (2018). Dynamic characteristics of 22 woods determined by the tranverse vibration method. Rev. Mex. ciencias For. 9 (48), 181–202. doi:10.29298/rmcf.v8i48.150
Chang, Z., Long, G., Zhou, J. L., and Ma, C. (2020). Valorization of sewage sludge in the fabrication of construction and building materials: A review. Resour. Conservation Recycl. 154, 104606. doi:10.1016/j.resconrec.2019.104606
Chen, S., Lu, F., and Wang, X. (2015). Estimation of greenhouse gases emission factors for China's nitrogen, phosphate, and potash fertilizers. Acta Ecol. sin. 35 (19), 6371–6383. doi:10.5846/stxb201402210304
Chen, X., Zhao, Y., Zeng, C., Li, Y., Zhu, L., Wu, J., et al. (2019). Assessment contributions of physicochemical properties and bacterial community to mitigate the bioavailability of heavy metals during composting based on structural equation models. Bioresour. Technol. 289, 121657. doi:10.1016/j.biortech.2019.121657
Fang, W., Delapp, R. C., Kosson, D. S., Van Der Sloot, H. A., and Liu, J. (2017). Release of heavy metals during long-term land application of sewage sludge compost: Percolation leaching tests with repeated additions of compost. Chemosphere 169, 271–280. doi:10.1016/j.chemosphere.2016.11.086
Gouin, F. R. (1985). Growth of Hardy Chrysanthemums in containers of media amended with composted municipal sewage sludge. J. Environ. Hortic. 3, 53–55. doi:10.24266/0738-2898-3.2.53
Gronman, K., Ypya, J., Virtanen, Y., Kurppa, S., Soukka, R., Seuri, P., et al. (2016). Nutrient footprint as a tool to evaluate the nutrient balance of a food chain. J. Clean. Prod. 112, 2429–2440. doi:10.1016/j.jclepro.2015.09.129
Guo, G., Chen, T., Yang, J., Zheng, G., and Gao, D. (2014). Regional distribution characteristics and variation of heavy metals in sewage sludge of China. Acta Sci. Circumstantiae 34 (10), 2455–2461.
Guoqing, X., Xiuqin, C., Liping, B., Hongtao, Q., and Haibo, L. (2019). Absorption, accumulation and distribution of metals and nutrient elements in poplars planted in land amended with composted sewage sludge: A field trial. Ecotoxicol. Environ. Saf. 182, 109360. doi:10.1016/j.ecoenv.2019.06.043
Hakanson, L. (1980). An ecological risk index for aquatic pollution control. A sedimentological approach. Water Res. 14, 975–1001. doi:10.1016/0043-1354(80)90143-8
Hao, X-D., Chen, Q., Li, J., and Jiang, H. (2019). Ultimate approach to handle excess sludge: Incineration and drying. CHINA WATER & WASTEWATER 35 (4), 35–42.
Hei, L., Lee, C. C., Wang, H., Lin, X. Y., Chen, X. H., and Wu, Q. T. (2016). Using a high biomass plant Pennisetum hydridum to phyto-treat fresh municipal sewage sludge. Bioresour. Technol. 217, 252–256. doi:10.1016/j.biortech.2016.02.025
Iglesias-Iglesias, R., Portela-Grandío, A., Treu, L., Campanaro, S., Kennes, C., and Veiga, M. C. (2021). Co-digestion of cheese whey with sewage sludge for caproic acid production: Role of microbiome and polyhydroxyalkanoates potential production. Bioresour. Technol. 337, 125388. doi:10.1016/j.biortech.2021.125388
IPCC (2019). 2019 refinement to the 2006 IPCC guidelines for national greenhouse gas inventories. Available online at https://www.ipcc.ch/report/2019-refinement-to-the-2006-ipcc-guidelines-for-national-greenhouse-gas-inventories/ (accessed June 5, 2022).
IPCC (2005). Carbon dioxide capture and storage carbon dioxide capture and storage. Available online at https://www.ipcc.ch/report/carbon-dioxide-capture-and-storage/ (accessed July 4, 2022).
IPCC (2007). Climate change 2007: Mitigation of climate change. Available online at https://www.ipcc.ch/report/ar4/wg3/ (accessed June 5, 2022).
Khadanga, S. S., and Jayakumar, S. (2020). Tree biomass and carbon stock: Understanding the role of species richness, elevation, and disturbance. Trop. Ecol. 61 (1), 128–141. doi:10.1007/s42965-020-00070-0
Li, Y-M., Ma, J-H., Liu, D-X., Sun, Y-L., and Chen, Y-F. (2015). [Assessment of heavy metal pollution and potential ecological risks of urban soils in Kaifeng City, China]., China. Environ. Sci. 36, 1037–1044. doi:10.13227/j.hjkx.2015.03.037
Liang, S., Yang, L., Chen, H., Yu, W., Tao, S., Yuan, S., et al. (2021a). Phosphorus recovery from incinerated sewage sludge ash (ISSA) and reutilization of residues for sludge pretreated by different conditioners. Resour. Conservation Recycl. 169, 105524. doi:10.1016/j.resconrec.2021.105524
Liang, Y., Xu, D., Feng, P., Hao, B., Guo, Y., and Wang, S. (2021b). Municipal sewage sludge incineration and its air pollution control. J. Clean. Prod. 295, 126456. doi:10.1016/j.jclepro.2021.126456
Lin, X., Chen, X., Li, S., Chen, Y., Wei, Z., and Wu, Q. (2021a). Sewage sludge ditch for recovering heavy metals can improve crop yield and soil environmental quality. Front. Environ. Sci. Eng. 15 (2), 22. doi:10.1007/s11783-020-1314-1
Lin, X., Li, S., Wei, Z., Chen, Y., Hei, L., and Wu, Q-T. (2021b). Indirect application of sludge for recycling in agriculture to minimize heavy metal contamination of soil. Resour. Conservation Recycl. 166, 105358. doi:10.1016/j.resconrec.2020.105358
Liu, H. T., Zheng, H. X., Chen, T. B., Zheng, G. D., and Gao, D. (2014). Reduction in greenhouse gas emissions from sewage sludge aerobic compost in China. Water Sci. Technol. 69 (6), 1129–1135. doi:10.2166/wst.2013.773
Lu, J-Y., Wang, X-M., Liu, H-Q., Yu, H-Q., and Li, W-W. (2019). Optimizing operation of municipal wastewater treatment plants in China: The remaining barriers and future implications. Environ. Int. 129, 273–278. doi:10.1016/j.envint.2019.05.057
MEE (2020). 2019 baseline emission factors for regional power grids in China. Available online at https://www.mee.gov.cn/ywgz/ydqhbh/wsqtkz/202012/t20201229_815386.shtml (accessed May 4, 2022).
Nazli, R. I., Kusvuran, A., Tansi, V., Ozturk, H. H., and Budak, D. B. (2020). Comparison of cool and warm season perennial grasses for biomass yield, quality, and energy balance in two contrasting semiarid environments. Biomass Bioenergy 139, 105627. doi:10.1016/j.biombioe.2020.105627
Ottosen, L. M., Thornberg, D., Cohen, Y., and Stiernström, S. (2022). Utilization of acid-washed sewage sludge ash as sand or cement replacement in concrete. Resour. Conservation Recycl. 176, 105943. doi:10.1016/j.resconrec.2021.105943
Papafilippaki, A., Paranychianakis, N., and Nikolaidis, N. P. (2015). Effects of soil type and municipal solid waste compost as soil amendment on Cichorium spinosum (spiny chicory) growth. Sci. Hortic. 195, 195–205. doi:10.1016/j.scienta.2015.09.030
Parr, J. F., Epstein, E., and Willson, G. B. (1978). Composting sewage sludge for land application. Agric. Environ. 4, 123–137. doi:10.1016/0304-1131(78)90016-4
Peter, L. (2000). Fabricated from cuspated sheet plant growth container. Munich: European Patent Office, EP1241928 A1.
Riaz, U., Murtaza, G., Saifullah, Farooq M., and Farooq, M. (2018). Influence of different sewage sludges and composts on growth, yield, and trace elements accumulation in rice and wheat. Land Degrad. Dev. 29 (5), 1343–1352. doi:10.1002/ldr.2925
Samake, M., Wu, Q. T., Mo, C. H., and Morel, J. L. (2003). Plants grown on sewage sludge in South China and its relevance to sludge stabilization and metal removal. J. Environ. Sci. 15 (5), 622–627.
Wang, L., Li, D., Liu, Z., and Li, H. (2022). Analysis on carbon emission from sludge treatment and disposal. China Environ. Sci. X, 1–10.
Wang, X., Zheng, G., Chen, T., Shi, X., Wang, Y., Nie, E., et al. (2019). Effect of phosphate amendments on improving the fertilizer efficiency and reducing the mobility of heavy metals during sewage sludge composting. J. Environ. Manage. 235, 124–132. doi:10.1016/j.jenvman.2019.01.048
Wei, L., Zhu, F., Li, Q., Xue, C., Xia, X., Yu, H., et al. (2020a). Development, current state and future trends of sludge management in China: Based on exploratory data and CO2-equivaient emissions analysis. Environ. Int. 144, 106093. doi:10.1016/j.envint.2020.106093
Wei, Y., Zhao, Y., Zhao, X., Gao, X., Zheng, Y., Zuo, H., et al. (2020b). Roles of different humin and heavy-metal resistant bacteria from composting on heavy metal removal. Bioresour. Technol. 296, 122375. doi:10.1016/j.biortech.2019.122375
Xu, R. K., and Coventry, D. R. (2003). Soil pH changes associated with lupin and wheat plant materials incorporated in a red–Brown Earth soil. Plant Soil 250 (1), 113–119. doi:10.1023/a:1022882408133
Xu, T., Xie, F., Wei, Z., Zeng, S., and Wu, Q. T. (2015). Phytoremediation of sewage sludge and use of its leachate for crop production. Environ. Technol. 36 (23), 3000–3007. doi:10.1080/09593330.2014.955061
Yu, X., Nakamura, Y., Otsuka, M., Omori, D., and Haruta, S. (2021). Development of a novel phosphorus recovery system using incinerated sewage sludge ash (ISSA) and phosphorus-selective adsorbent. Waste Manag. 120, 41–49. doi:10.1016/j.wasman.2020.11.017
Zasoski, R. J., Edmonds, R. L., Bledsoe, C. S., Henry, C. L., Vogt, D. J., Vogt, K. A., et al. (1984). Municipal sewage sludge use in forests of the Pacific northwest, U.S.A.: Environmental concerns. Waste Manag. Res. 2, 227–246. doi:10.1016/0734-242x(84)90029-6
Zat, T., Bandieira, M., Sattler, N., Segadães, A. M., Cruz, R. C. D., Mohamad, G., et al. (2021). Potential re-use of sewage sludge as a raw material in the production of eco-friendly bricks. J. Environ. Manag. 297, 113238. doi:10.1016/j.jenvman.2021.113238
Zeng, L., Lin, X., Zhou, F., Qin, J., and Li, H. (2019). Biochar and crushed straw additions affect cadmium absorption in cassava-peanut intercropping system. Ecotoxicol. Environ. Saf. 167, 520–530. doi:10.1016/j.ecoenv.2018.10.003
Zhang, J., Bao, Y., Jiang, Y., Liu, H. T., Xi, B. D., and Wang, D. Q. (2019). Removal and dissipation pathway of typical fluoroquinolones in sewage sludge during aerobic composting. Waste Manag. 95, 450–457. doi:10.1016/j.wasman.2019.06.036
Zhang, Q., Zou, D., Zeng, X., Li, L., Wang, A., Liu, F., et al. (2021). Effect of the direct use of biomass in agricultural soil on heavy metals- activation or immobilization? Environ. Pollut. 272, 115989. doi:10.1016/j.envpol.2020.115989
Keywords: municipal sewage sludge, indirect application, garden plant, greenhouse gas emissions, sludge treatment plant
Citation: Lin X, Chen C, Li H, Hei L, Zeng L, Wei Z, Chen Y and Wu Q-T (2022) Comprehensive recycling of fresh municipal sewage sludge to fertilize garden plants and achieve low carbon emission: A pilot study. Front. Environ. Sci. 10:1023356. doi: 10.3389/fenvs.2022.1023356
Received: 19 August 2022; Accepted: 26 September 2022;
Published: 11 October 2022.
Edited by:
shengsen wang, Yangzhou University, ChinaReviewed by:
Guodi Zheng, Institute of Geographic Sciences and Natural Resources Research (CAS), Chinajose navarro pedreño, Miguel Hernández University of Elche, Spain
Copyright © 2022 Lin, Chen, Li, Hei, Zeng, Wei, Chen and Wu. This is an open-access article distributed under the terms of the Creative Commons Attribution License (CC BY). The use, distribution or reproduction in other forums is permitted, provided the original author(s) and the copyright owner(s) are credited and that the original publication in this journal is cited, in accordance with accepted academic practice. No use, distribution or reproduction is permitted which does not comply with these terms.
*Correspondence: Qi-Tang Wu, d3VxaXRhbmdAc2NhdS5lZHUuY24=