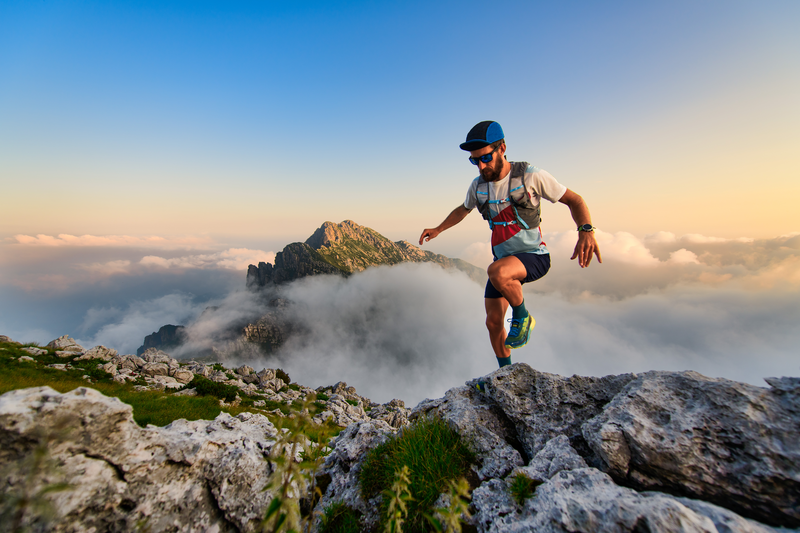
94% of researchers rate our articles as excellent or good
Learn more about the work of our research integrity team to safeguard the quality of each article we publish.
Find out more
ORIGINAL RESEARCH article
Front. Environ. Sci. , 30 November 2022
Sec. Freshwater Science
Volume 10 - 2022 | https://doi.org/10.3389/fenvs.2022.1022776
This article is part of the Research Topic Freshwater Science in the Tropical Anthropocene View all 8 articles
Land use modification is a notable cause of biodiversity loss in streams. However, the impacts of anthropogenic forest conversion on β-diversity are difficult to forecast, especially in Afrotropical stream ecosystem, mainly because: 1) empirical research is scant, and; 2) the few available studies provide conflicting findings. In our study, we used techniques of decomposing β-diversity to evaluate the influence of land use changes on macroinvertebrates β-diversity of 66 stream sites in an Afrotropical Anthropocene. We also evaluated the potential exclusive and shared contributions of ecological drivers of community composition and β-diversity. Our total β-diversity for both forested and modified streams was driven mainly by the turnover component, while the nestedness-resultant component was negligible. The dominance of turnover in both forested and modified streams in our systems stresses the urgency to protect many sites in order to conserve γ-diversity. While β-diversity diminished in our urban + agric streams in relation to our forest streams, leading to a process of biotic homogenization, β-diversity of our forest streams was similar to that of the urban streams, implying that land use does not necessarily lead to a process of biotic homogenization. The contrasting findings about relationships between land use and β-diversity in our study showed that the effects of land use on β-diversity can be variable and context-dependent. Larger parts of variation in community composition and β-diversity were accounted for by the local environmental conditions (through environmental filtering) and land use, while geographical spatial factors (through dispersal limitation) explained little part of variation in our system–highlighting the importance of species sorting (environmental filtering) over dispersal-related processes in metacommunity organization.
The most significant signature of the Anthropocene world is the anthropogenic modification of the ecosystem (McGill et al., 2015; Petsch et al., 2021a), leading to negative impacts such as biodiversity loss (Watson et al., 2016) and biotic homogenization (Dornelas et al., 2015; Magurran et al., 2015), especially in developing parts of the world (Newbold et al., 2015). These have resulted in a global biodiversity condition that is equivalent to earlier worldwide mass extinction incidents (Barnosky et al., 2011, Ceballos et al., 2015), indicating that we are already in a critical state of biodiversity globally (Feio et al., 2022). In particular, freshwater ecosystems (for example, rivers and streams) are among the mainly affected systems by anthropogenic pressures (Keke et al., 2017; Brittain et al., 2020; Keke et al., 2021a). Increased human settlements around the catchments of freshwater systems substantially alter the neighboring landscape and undermine the ecosystem integrity (Fugère et al., 2016; Keke et al., 2020b). The dominance of human settlements around rivers and streams transforms natural forests into forms of human-induced land uses such as agriculture and urbanization.
Although some biodiversity aspects, like species diversity, can be separated into alpha (α), beta (β), and gamma or regional (γ) components (Whittaker 1960), the measurements of β-diversity are specifically important given they constitute variability in community composition among sites (Costa and Melo, 2008; Anderson et al., 2011; Brittain et al., 2020). β-diversity is fundamental in uncovering the mechanisms that drive biological assemblage and helps in creating effective roadmaps for biodiversity conservation (Anderson et al., 2011; Al-Shami et al., 2013). β-diversity is an important component of biodiversity, because it provides a direct linkage between α-diversity and γ-diversity (Whittaker 1960; Anderson et al., 2011). The partitioning of total β-diversity into its turnover and nestedness components gives more understanding of the perspective of spatial differences in community composition (Baselga 2010; Legendre 2014). For instance, a higher contribution of the turnover component to total β-diversity gives an indication that majority of differences in community composition is as a result of different species occupying different sites (Legendre 2014). The conservation implication of dominance of turnover is that many sites would require immediate protection in order to conserve γ-diversity (Fugère et al., 2016). In opposite, a higher contribution of the nestedness component to total β-diversity indicates that differences in community composition is as a result of species-gain or species-loss (i.e., number of species of a site is a strict subset of richer sites) caused by variations in species richness among the sites (Legendre 2014). This implies that a few species-rich sites require to be protected in order to conserve γ-diversity (Fugère et al., 2016). Despite the fact that variation in turnover and nestednes difference components does not only supply useful insights into the spatial variation of community composition but also provides biodiversity evaluation and conservation basis, it has received far less attention in recent freshwater ecological studies (Anderson et al., 2011; Legendre 2014; Rocha et al., 2018; Brittain et al., 2020).
While biotic homogenization is widely known to occur when there is an increase in among-site similarity (i.e., a decrease in β-diversity) created by human-induced disturbances (e.g., habitat alteration, introductions of non-natives), biotic differentiation occurs when there is an increase in among-site dissimilarity (or variation: an increase in β-diversity; Maloney et al., 2011). Land use modification is a notable cause of biodiversity loss in freshwater ecological systems (Siqueira et al., 2015; Arimoro and Keke 2017; Petsch et al., 2021a). However, the impacts of anthropogenic forest conversion on stream β-diversity are difficult to forecast, especially in Afrotropical stream ecosystem, mainly because: 1) empirical research is scant, and; 2) the few available studies provide conflicting findings (Fugère et al., 2016). For instance, on the one hand, forest conversion into agriculture could induce cultural eutrophication, resulting into an increase in β-diversity (biotic differentiation) by intensifying stochastic processes in community assemblage (Bini et al., 2014). Ideally, the persistence of a greater percentage of the regional species pool in higher productive sites than lower productive sites could drive stochasticity in colonization record to result to diverse stability of community assemblage, consequently leading to an increase in β-diversity (Chase 2010). Further, a heterogeneous land use regimen (i.e., having forest, urban, agriculture land use types within the same catchment locality) could lead to elevated β-diversity if different taxa are driven by the environmental states that are related to each land use type (Siqueira et al., 2015). On the other hand, forest conversion leads to habitat deterioration which adversely influences a broad suite of pollution-sensitive species, enabling only a minor percentage of the regional species pool to sustain viable community at deforested localities (Petsch et al., 2021a)—thereby diminishing β-diversity (biotic homogenization). In addition, some physical effects of deforestation such as silt deposition on the substratum, paucity of woody debris, flow velocity changes, reduced addition of coarse organic matter, elevated loads of sediments and pollutants from allochthonous sources, and alteration of channel morphology could diminish habitat heterogeneity (Edegbene et al., 2021a; 2021b), leading to diminished β-diversity (Passy and Blanchet 2007; Donohue et al., 2009; Leal et al., 2016; Castro et al., 2018). Lastly, forest conversion can alter dispersal rates of riverine macroinvertebrates species with terrestrial life stages, and consequently affects β-diversity (Heino et al., 2015; Petsch et al., 2021a). Hence, the influence of land use changes could ultimately be context-dependent, thereby either diminishing β-diversity (biotic homogenization; Passy and Blanchet 2007; Maloney et al., 2011; Siqueira et al., 2015), elevating β-diversity (biotic differentiation; Hawkins et al., 2015; Liborio et al., 2016; Fugère et al., 2016; Roa-Fuentes et al., 2019), or not causing any clear change in β-diversity (Larsen and Ormerod 2014; Heino et al., 2015; Petsch et al., 2021a; Keke et al., 2021b).
The explanatory approach of variance partitioning of ecological predictors emphasizes that communities are jointly driven by local environmental condition through environmental filtering (species sorting) and spatial processes (dispersal-related; e.g., mass effects, dispersal limitation and patch dynamics), although their corresponding importance might be dependent on spatial magnitude of the study extent and dispersal rates of communities (Leibold et al., 2004; Heino 2011; Al-Shami et al., 2013; Arimoro et al., 2021; Li et al., 2021). Among these, mass effects usually happens between sites that are closely-located to each other in proportionately small spatial extent or in conditions marked by increased connectivity (Cottenie et al., 2003; Declerck et al., 2011). As for mass effect model, high dispersal rates may homogenize communities and decrease beta diversity (Mouquet & Loreau 2003), which might possibly hamper the role of environmental filtering on community assemblage (Li et al., 2021). Nonetheless, bioassessment initiatives are generally required at comparatively wide spatial extents, where dispersal limitation (hampering a great percentage of individual species from accessing desirable localities) could influence the effects of local environmental variables on community assemblage (Heino 2013). Environmental filtering is anticipated to be most influential at intermediate extents, where average dispersal degree enables taxa to trail environmental gradients (i.e., species sorting; Soininen 2014). Dispersal-related processes (influenced by reduced dispersal rates and enormous geographical area) assume importance following increase in spatial extent, resulting to elevation in compositional variations between assemblages (Jamoneau et al., 2018; Li et al., 2021). Although studies on geographical variables (longitude, latitude, and altitude) have provided important insights into freshwater studies globally, most of these efforts have been focused on alpha diversity (see Vinson and Hawkins 2003; Heino 2011; Hansen et al., 2013). To our understanding, no study has evaluated the relationship dynamics of β-diversity with geographical variables in the Afrotropic. Similarly, the overall insights into how β-diversity is jointly driven by local environmental variables, spatial factors, and land use associated changes in lotic systems still remain scant globally.
The Afrotropical streams, despite being hugely diverse ecological systems and biodiversity hotspots (Boyero et al., 2011; Collen et al., 2014; Tonkin et al., 2016; Keke et al., 2020a; Arimoro and Keke, 2021), have remained understudied. The Afrotropical region is currently affected by massive forest conversion into anthropogenic uses (Feio et al., 2022), and the expected rapid growth of this region will further deteriorate the current state (Laurance et al., 2014; Keke et al., 2021a). In the light of this, the region has an urgent need to evaluate the effects of forest conversion in the bid to conserve the biodiversity of the tropical ecosystem. In our study, we used techniques of decomposing β-diversity to evaluate the influence of land use changes on β-diversity of streams in an Afrotropical Anthropocene. We also evaluated the ecological drivers of β-diversity. First, we analysed β-diversity for each land use type, with the hypothesis that total β-diversity will be driven mostly by its turnover component. Second, we tested whether land use associated changes could either increase β-diversity (biotic differentiation), decrease β-diversity (biotic homogenization) or do not influence β-diversity in this region. We refrained from setting up a hypothesis for this objective, because of both the paucity of study on effects of land use on β-diversity, and the contradictory accounts provided by empirical studies of land use effects on β-diversity globally. Third, we explored the exclusive and shared contributions of local environmental conditions, spatial factors (geography), land use classes, and region identity (drainage basin) in driving β-diversity. Here, we hypothesized that both local environmental conditions and geographical variables (spatial variables) would be important drivers of β-diversity. Specifically, we expected local environmental variables to dominate over spatial variables given clear variability in the severity of anthropogenic influence and forest conversion in this region (Fugère et al., 2016).
We sampled sixty-six sites located between 5°27′ and 6°50′North, and 5°35′ and 6°41′East of southern Nigeria (Edo and Delta regions) (Figure 1). Edo region represents the upper western Niger Delta while the Delta region represents the lower western Niger Delta. The study area consists of the typical tropical climate of two prominent wet (May to October) and dry (November to April) seasons, with average yearly relative humidity of 85% and average yearly temperature of ca. 28°C (Tonkin et al., 2016). Land use in this area includes rainforest, natural vegetation, urbanization, and agriculture. Aquatic flora comprises mostly of macrophytes (submerged and floating; emergent), Pandanus spp., Mitragyna ciliate, and dense trees [such as palm trees and Indian bamboo (Bambusia spp.)].
We aimed at sites covering the three major land types in the sampled area, derived from the riparian and catchment characteristics: forest, urban, and a combination of both urban and agriculture (used afterwards in this article as urban + agric). The riparian and catchment characterization were based on sites from each land use class having >60% of either forest, urban, or urban + agric (see Supplementary Table S1). The magnitude of forest, urban, and agric + urban in the riparian and catchments of chosen sites was ascertained by the usage of land cover satellite imagery generated by data from Shuttle 152, within a 1500-m buffer size of our studied streams. This approach resulted into 29 forest sites, 20 urban sites, and 17 urban + agric sites.
Macroinvertebrates were collected from each site alongside broad suites of environmental variables. We took into account the possible temporal effects by initially analyzing the data temporally, but we observed that temporality did not affect our analyses. Macroinvertebrates were collected within a 25-m approximation of the wadeable section of each site using a D-frame net of 500 µm mesh size, according to the methods of Jeffries and Mills (1990). At each sampling site, four 3-min samples were collected on each sampling occasion to account for all substrata (stones, sand, silt, mud, and vegetation) and flow regimes (riffles, run, and pool). The four 3-min samples were pooled to represent a unit sample for each site. The collected samples were preserved in 70% ethanol and taken to the laboratory. In the laboratory, samples were processed by washing and sieving through a 500 µm mesh size. Processed samples were then sorted and counted using a dissecting microscope. Using a stereoscopic microscope (910 magnification), sorted individuals were identified to the lowest possible taxonomic level, mostly genus and species, by the use of regional keys (e.g., de Moor et al., 2003), keys adapted from elsewhere (e.g., Merritt and Cummins, 1996) as well as the assistance of regional specialists. This integrated approach ensured a mostly genus or species level identification with species level identification accounting for over 90% representation. Thereafter, we deposited the voucher specimens at Albany Museum, Grahamstown, South Africa.
Environmental variables were sampled at each site alongside with macroinvertebrates collection. We measured a broad group of environmental variables, ranging from physical, chemical, to riparian variables (indicated as ENV hereafter). Habitat features for an individual site were determined over a 100-m reach. A calibrated rod was used for the determination of depth in each site. Flow velocity was determined following the method of Gordon et al. (1994). Canopy cover was determined by the visual percentage estimation along the sampled extent. Substratum particles composition was determined by the visual estimation of percentage of sand, clay, loam, stones, and mud in 100-m sampled extent. The following physical and chemical variables were determined in-situ from each sampling site: temperature, pH, conductivity, total dissolved solids (TDS), using portable Hanna HI 991300/1; turbidity, using portable turbidity meter HI 93102, and; dissolved oxygen, using YSI 55 dissolved oxygen meter. After the in-situ measurements, water samples were taken to the laboratory for the determination of nitrates, phosphates, and biochemical oxygen demand (BOD; APHA, 1995). Nitrates and phosphates were determined spectrophotometrically after reduction with appropriate solutions (APHA 1995). Geographical variables consisted of longitude, latitude, and altitude, indicated as GEO subsequently. We included two dummy variables indicating land use types (i.e., forest, urban, and urban + agric, indicated as LUT subsequently) and regional identity (i.e., Edo and Delta regions where samples were collected from, indicated as REG hereafter). Our approach did not utilize other sophisticated variables, such as variables obtained from the usage of Moran eigenvector maps (Dray et al., 2012; Legendre & Legendre 2012), given that we were simply focused on the linear geographical and altitudinal gradients.
We computed β-diversity for the land use types (forest, urban, and urban + agric). We computed β-diversities by calculating β-diversity components (or indices), namely Sørensen (total β-diversity), turnover (Simpson), and nestedness-resultant. This approach involves the decomposition of total β-diversity into turnover and nestedness components. While turnover is defined as species replacement of species in a system, nestedness typifies the condition where number of species is subset of richer sites. Presence-absence data (of Sørensen index) is driven by variations in site-by-site species composition, while abundance data (of Bray-Curtis) is driven by variations in site-by-site abundance. In all our analyses involving β-diversity, we used presence-absence data in our effort to remove bias due to possible variations in sampling endeavor which can typically influence the abundance data (Brittain et al., 2020). First, we calculated β-diversity dissimilarity matrices using the function beta.pair in the R package betapart (Baselga et al., 2017). We employed the monotonic transformation of a Sørensen index where the turnover and nestedness components are decoupled (Baselga 2010, 2012), leading to the generation of three multiple-site dissimilarity matrices, namely: 1) Sørensen (total) dissimilarity which computes the total spatial turnover in species composition; 2) Simpson (turnover) dissimilarity which computes turnover that minimizes or reduces the differences in species richness; and 3) nestedness which is derived from variations in species richness between sites (Baselga 2012). This analytical approach produced the three dissimilarity (or distance) matrices that were employed as response variable (or data) in the following analyses: permutational multivariate analysis of variance (PERMANOVA; Anderson, 2001), multivariate homogeneity of group dispersions (PERMDISP; Anderson et al., 2006) and distance-based redundancy analysis (dbRDA; Legendre and Anderson 1999).
We used PERMDISP to evaluate the variations in average distances of macroinvertebrates communities to group multivariate centroids (median) among the land use types (forest, urban, and urban + agric). This evaluates variations in β-diversity among the three land use types (forest, urban, and urban + agric). PERMDISP was run using the function betadisper (Anderson et al., 2009) in the R package vegan (Oksanen et al., 2017). We also drew Principal coordinates analysis (PCoA) to evaluate further variations in β-diversity among the land use types (Rocha et al., 2017). We employed PERMANOVA to evaluate the variations in community composition among land use types. We ran PERMANOVA tests using the adonis function in the R package vegan (Oksanen et al., 2017). In cases where we found a significant difference, we ran pairwise comparisons for both PERMDISP and PERMANOVA.
We employed distance-based analyses (dbRDA; Legendre and Anderson 1999) in evaluating the differences in each component of β-diversity in relation to the land use types. We used dbRDA-based forward selection method to select the final environmental variables (ENV), geographical variables (GEO), dummy variable regional identity (REG), and dummy variable land use types (LUT) for the models of each biological dissimilarity (distant) matrix using the functions capscale and ordiR2step in the R package vegan. We conducted variable selection technique for ENV based on the two stopping rules described by Blanchet et al. (2008), while GEO (with three variables), REG (with one variable, two levels), and LUT (with one variable, three levels) were forced into the analytical models. In essence, we did not conduct variable selection for variable groups GEO, REG, and LUT, but only performed variable selection for ENV variable group (with broad suites of variables).
After selecting the final sets of variables for each predictor variable group, we continued with variation partitioning of each dissimilarity matrix (Y) by utilizing ENV, GEO, LUT, and REG as predictor groups, following a partitioning technique that has been universally employed (Borcard et al., 1992; Legendre and Legendre 2012; Heino and Alahuhta 2015). We precisely used dbRDA in variation partitioning to evaluate the pure and/or shared contributions of ENV, GEO, LUT, and REG to β-diversity. Variation partitioning was run using the function varpart in the R package vegan (Oksanen et al., 2013). For all our analyses involving dbRDA, a Lingoes correction for negative eigenvalues was added in our data (Oksanen et al., 2017). Partitioning variations of macroinvertebrates data (Y) among the four groups of predictor variables produced: 1) pure fractions of ENV, GEO, LUT, and REG; 2) shared fractions between any two of ENV, GEO, LUT and REG or among sets of ENV, GEO, LUT, and REG, and; 3) values of unexplained variation (U). We further tested the significance of the generated pure fractions using the anova function in the R package vegan. We recorded the adjusted R2 values because they generally represent unbiased estimates of explained variations since they are corrected for the number of explanatory variables (Miles 2014). Our explanations of predictor accounts were based on adjusted R2, instead of just the p-values, since our attention was mainly on the magnitude of effects and not just significance alone.
A total number of 33,901 macroinvertebrate individuals from 173 species and 55 families were found in the dataset for forest land use type, while a total of 20,012 individuals from 91 species and 54 families were present in urban land use type, and a total of 21,160 individuals from 111 species and 55 families were available in urban + agric land use type (Figure 2). Forest streams comprised more different genera (i.e., were more heterogeneous) compared to urban and urban + agric streams which comprised more similar genera.
FIGURE 2. Venn diagram representing the number of shared and exclusive species among Forest, Urban and Urban + Agric sites.
For total β-diversity, the multiple site dissimilarity for all land use types (forest, urban, and urban + agric; Supplementary Figure S1) showed that the turnover (Simpson) component of the total beta diversity (Sørensen) was more important than the nestedness-resultant component.
We found significant differences in β-diversity among the three land use types (p < 0.05), with forest streams differing significantly (p < 0.05) from urban + agric streams but not significantly different (p > 0.05) from urban streams based on total (Sørensen) and turnover (Simpson) dissimilarities (PERMDISP for total dissimilarity: F = 5.580; p = 0.005; average distance to median = 0.377, 0.348, and 0.303 for forest, urban, and urban + agric, respectively Figures 3, 4. PERMDISP for turnover dissimilarity: F = 3.299; p = 0.043; average distance to median = 0.313, 0.293, and 0.249 for forest, urban, and urban + agric, respectively Figures 3, 4). PERMDISP pairwise comparisons also showed similarity (p > 0.05) between urban and urban + agric streams. The nestedness-resultant component dissimilarity did not vary significantly among the three land use types (PERMDISP: F = 0.809; p = 0.450; average distance to median = 0.077, 0.065, and 0.057 for forest, urban, and urban + agric, respectively Figures 3, 4). Community composition was significantly different among the land use types for total β-diversity (PERMANOVA, p = 0.001; Table 1) and the turnover component (PERMANOVA, p = 0.001), with forest streams differing significantly (p < 0.05) from both urban and urban + agric streams. The significant differences in community composition among land use types were related to turnover, as the nestedness component did not vary significantly among the land use types (PERMANOVA, p = 0.999).
FIGURE 3. Boxplots of median distance to centroid of the land use types (forested, urban, and urban + agric): (A) total β-diversity (Sørensen), (B) the turnover component (Simpson), and (C) Nestedness-resultant component for each land use in the macroinvertebrate data. The line within the box indicates the median; the ends of the box indicates the upper and lower quartiles, the extreme line indicates the highest and lowest value (excluding outliers), while the dots indicates possible outliers.
FIGURE 4. PCoA plots of variation in beta diversity of the land use types (forested, urban, and urban + agric) based on the different distance matrices.
TABLE 1. Permutational Multivariate Analysis of variance (PERMANOVA) result showing differences in community composition among the three land use types (i.e., forest, urban, and urban + agric).
Constrained ordination through dbRDA revealed that slightly different sets of ENV were selected in the models for all dissimilarity matrices used as response. For total β-diversity, the ENV selected in their ranks of significance were: temperature, dissolved oxygen, and biological oxygen demand. For the turnover component, only pH was selected for the model. For the nestedness-resultant component, biological oxygen demand, temperature, nitrates, and phosphates entered the model. Variation partitioning indicated slightly different accounts from the dissimilarity matrices employed by the model (Figure 5). However, generally, most of the variations in community compositions in the model were almost jointly accounted by the local environmental variables (ENV) and land use types (LUT) as predictors, followed by the geographical variables (GEO). The regional identity (REG) did not account for community composition variation as no pure fraction was associated with it. The range of pure fractions of ENV, LUT, REG, and GEO for all dissimilarity matrices used as response were 0.0%–4%, 1%–4%, 0%–0%, and 1%–2%, respectively. There were no shared fractions of variation between any two or among the four predictor variable groups for all dissimilarity matrices used as response. For total β-diversity, variations were mostly shared equally between the ENV (4%) and GEO (4%). For the turnover component, variations in community composition were slightly explained by the ENV (1%), LUT (1%) and GEO (1%). In contrast, only ENV could explain variations among the four predictor variable groups of nestedness-resultant component, as LUT, REG and GEO were not important drivers.
FIGURE 5. Venn diagrams of variation partitioning of macroinvertebrates community composition/structure based on: total dissimilarity as response (A), turnover component as response (B), and nestedness-resultant component as response (C). ENV, local environmental variables; LUT, land use types; REG, Regional identity; GEO, latitude, longitude, and altitude. Numbers shown inside Venn diagrams represent the values of adjusted r2.
Although focus is increasingly shifting to the utility of β-diversity for providing biodiversity insights globally, there is still considerable paucity of insights into β-diversity processes in different regions (for example, the tropics), ecological systems (for example, freshwater bodies), and organism type (for example, macroinvertebrates). Most of the early studies centered on α-diversity (local scale), while site-by-site differences in community composition (β-diversity) and its relationship with local environmental condition as well as geographical variables has been critically understudied, especially in the tropical stream ecosystems (Jiang et al., 2021). While α-diversity is important in assessing the influence of human-induced perturbations on local stream biodiversity, β-diversity is helpful in tracking homogenization with corresponding possible recovery from anthropogenic impairment (Passy and Blanchet 2007).
In support of our hypothesis, our total β-diversity was driven by the turnover component, while the nestedness-resultant component was negligible. It is common that the turnover component of total β-diversity is higher than the nestedness component, especially when using Baselga approach (Soininen et al., 2018). A higher contribution of the turnover component to total β-diversity and species composition gives an indication that majority of variations in community composition is as a result of different species occupying different sites (Legendre 2014). The conservation implication of dominance of turnover is that many sites would require immediate protection in order to conserve γ-diversity (Fugère et al., 2016).
Ecological studies of effects of land use on stream β-diversity have either documented a decrease in β-diversity (biotic homogenization; Passy and Blanchet 2007; Maloney et al., 2011; Siqueira et al., 2015), an increase in β-diversity (biotic differentiation; Hawkins et al., 2015; Liborio et al., 2016; Fugère et al., 2016; Roa-Fuentes et al., 2019) or a weak relationship with no clear trend (Larsen and Ormerod 2014; Heino et al., 2015; Petsch et al., 2021a; Keke et al., 2021b; Petsch et al., 2021b). We found a negative relationship between land use and β-diversity of urban + agric streams, where land use diminished β-diversity–a process widely known as biotic homogenization. In our study, forest streams were more heterogenous with a broad suites of variable sets of most abundant species, whereas urban + agric streams showed strong homogeneity with the occurrence of similar sets of species. We, therefore, put forward that forest conversion and modification can diminish β-diversity by possibly constraining different taxonomic colonization. On this perspective of constrained colonization, only taxa with environmental specificity attributes can show resilience and persistence in colonizing modified freshwater ecosystems, thereby causing modified streams to exhibit strong similarity among them (Roque et al., 2010; Siqueira et al., 2015). This phenomenon may be as a result of impaired environmental condition of modified streams. Impaired and eroded environmental conditions of streams magnify the relevance of environmental filtering which subsequently governs community assemblage, and has minor equilibrium condition (Chase 2007). Nonetheless, forested streams are typically more heterogeneous in relation to refuge, and this enhances predatory evasion (Padial et al., 2009; Keke et al., 2021a). Within the bounds of a heterogeneous environmental setting, firm preference consequences influenced by different dispersal capacities are able to create variability in among-stream biotic assemblage, resulting to an increase in β-diversity (Weiher and Keddy, 1995; Chase 2003). The negative relationship between land use and β-diversity of urban + agric streams in our study supports this assertion given our forest streams had heterogeneous filtering of variable sets of taxa leading to the creation of heterogeneous assemblage, while urban + agric streams with homogenous filtering of similar sets of taxa led to the creation of a more homogenous assemblage. Reductions in stream β-diversity are generally caused by human-induced perturbations, including forest land-use conversion (Siqueira et al., 2015) and biotic invasions caused by the introduction of non-natives (Solar et al., 2015). Homogenization is therefore caused by an increase in biotic similarities among sites (a decrease in β-diversity), created by human-influenced disturbances. Elevation in the dispersal rates of unstable reaches caused by constant substratum erosion and biofilm transport into the drift may hugely be responsible for the diminished β-diversity in our urban + agric sites, although we lack requisite information on dispersal rates to underpin this assertion.
We did not find evidence of biotic homogenization between land use and β-diversity in our urban stream system, implying that land use does not necessarily lead to a process of biotic homogenization. Instead, we observed a weak relationship between land use and β-diversity of urban streams where β-diversity of forested streams was similar to β-diversity of urban streams, although more studies would be needed to unravel why β-diversity of forested streams showed contrasting relationships with different modified land use types. These contrasting results demonstrating the relationships between land use and β-diversity are indicative of the fact that land use effects on β-diversity have no general definition as they still remain context-dependent and controversial, needing further studies. Another possible inference drawn from the contrasting findings about relationships between land use and β-diversity is that β-diversity is unlikely to be modulated by a singular predictor and the differentiation among these possible predictors could elicit different context-specific effects on β-diversity. The contrasting relationship in β-diversity between forested and modified streams in our system might also be plausibly due to the fact that the different modified land uses selected distinct tolerant taxa resulting from increased environmental disparity among land use types, although we did not particularly evaluate this possibility. Modified streams exhibit high environmental heterogeneity resulting from high environmental differentiation among land uses (Barboza et al., 2015; Petsch et al., 2021b), leading to marked community colonization by taxa acclimatized to the prevailing local conditions (Siqueira et al., 2015; Petsch et al., 2021b). It is therefore possible that land use differentiation in other local environmental parameters we did not measure in our study may likely regulate the relationship.
Forest conversion and modification in both tropical and temperate streams have been reported to create differences in community composition between forested and modified streams, where modified sites presented degraded habitat conditions that consequently decreased community colonization (Wantzen 2006; Kasangaki et al., 2008; Lorion and Kennedy 2009; FAO, 2010; Larsen and Ormerod 2014; Masese et al., 2014; Fugère et al., 2016). This might explain the difference in community composition between our forest streams and modified streams (urban and urban + agric). The occurrence of many taxa in our forest streams revealed that they are key components of biodiversity, but the growing urbanization and agriculture are rapidly modifying forested sites. In our bid to conserve the biodiversity and ecological integrity of the tropical region, the forested streams need to be urgently protected.
Our dbRDA-based constrained ordination model indicated that just a relatively small percentage of community variation could be explained by our predictor variable groups. Such low or even critically lower percentage of explained variations are largely typical of community composition studies that employed similar methods of constrained ordinations based on adjusted R2 values (see for example, Beisner et al., 2006; Peres- Neto et al., 2006; Gothe et al., 2013; Heino et al., 2013, 2015; Souffreau et al., 2015; Tonkin et al., 2016; Rocha et al., 2017; Alahuhta et al., 2018; Li et al., 2019, 2020, 2021; Brittain et al., 2020; Keke et al., 2021b). Nonetheless, the inability to explain large percentage of variance does not undermine evaluation of important processes of community assemblage, as they meaningfully reveal that elucidating variations in assemblage structure is hugely difficult (see for example, Low-Décarie et al., 2014; Li et al., 2020). However, we observed that the percentage of explained variation in our study was critically lower than the usual percentages of explained variations presented by most works, but equally as low as our previous work in this Afrotropical region (Keke et al., 2021a). Afrotropical freshwater bodies are prone to massive anthropogenic disturbances which endanger their biodiversity integrity (Fugère et al., 2016; Keke et al., 2021b), so this may also plausibly provide explanation to our critically lower unexplained variance–as they are capable of transforming ecosystem processes and cause taxonomic extinction. The relatively lower spatial extent covered in our study in comparison to other studies that reported much higher percentages of explained variation might be another possible reason we recorded much lower percentage of explained variation. Higher percentages of unexplained variations are often associated with a broad suite of factors such as insufficient modelling of spatial factors, stochastic mechanisms, and missing predictor variables (Heino et al., 2015; Rocha et al., 2017). Although we measured a wide range of predictor variables, we cannot rule out the significance of stochastic mechanisms and missing environmental variables in modulating much higher percentage of explained variance in our study. We, therefore, emphasize that more precise studies are needed to test the aforementioned possibilities, especially in the Afrotropical region.
Like we expected, the environmental variables (ENV) and land use types (LUT), related to local environmental conditions, jointly accounted for the largest proportion of variation in community composition and β-diversity, reinforcing the hypothesis that species sorting mechanisms (environmental filtering) usually dominate above spatial mechanisms (e.g., dispersal limitation) in driving community structures when using variation partitioning and explanatory techniques (Jamoneau et al., 2018; Nicacio and Juen 2018; Brittain et al., 2020; Li et al., 2020; López-Delgado et al., 2020; Murray-Stoker and Murray-Stoker 2020; Keke et al., 2021a). Overall, the variation contributory importance of environmental filtering in comparison to spatial dynamics is hugely dependent on the magnitude of environmental gradients. Human-induced disturbances such as farming activities, forest modifications, dredging activities, oil production activities, urbanization, among others, have been documented as important elements of environmental gradients in present studied area, which are capable of supplying huge possibilities for species sorting on aquatic biotic fauna (Boyero et al., 2011; Tonkin et al., 2016). Environmental variables associated with land use changes must have provided heterogeneous conditions for environmental filtering, mirrored into differences in community composition and β-diversity among land use types in our study. Geographical predictor group (spatial factor; GEO) explained little part of variation, suggesting that spatial factors were not important in community assemblage. The fact that our study did not encompass considerably large spatial scale might be why spatial factor could not explain large part of variation. Despite the obvious fact that species sorting usually seems to be the main driver of community structure, earlier studies have also highlighted the co-importance or dominance of spatial processes in modelling assemblage structure (see Heino et al., 2015; Tonkin et al., 2016; Cai et al., 2017; Castilloescriva et al., 2017; Nicacio and Juen 2018; Cai et al., 2019; Li et al., 2019, 2020; Brittain et al., 2020), which stresses the need to consider spatial processes in biodiversity studies. Astonishingly, fractional (pure and shared) variation contribution from regional identity (REG) was entirely non-existent in our study. This could mostly be because geographical influences exert importance in community structure only when the spatial extent of the study space is considerably large (Rocha et al., 2017; Yongjiu et al., 2017; Li et al., 2020).
To synthesize the major aspects of our result, we have shown that the relationship between land use and stream β-diversity can be variable and context-dependent. Larger parts of variation in community composition and β-diversity were accounted for by the local environmental condition (ENV) while the geographical predictor group (spatial factor; GEO) explained little part of variation, negating the importance of spatial factors in our system. We emphasize the necessity of utilizing approaches of testing the multivariate homogeneity of group dispersions based on deviations from the group centroid (PERMDISP) and permutational multivariate analysis of variance based on location of group centroids (PERMANOVA) in evaluating effects of anthropogenic land use modifications on biodiversity. We also emphasize the importance of considering spatial factors in evaluating how assemblage composition responds to abiotic factors, given the influence of species sorting caused by anthropogenic disturbances may be misconstrued without taking into account other ecological factors (Cai et al., 2019; Li et al., 2019, 2020). Second, biotic assemblage structure and overall biodiversity of our studied area have critically been impaired by anthropogenic impacts, such that riverine biodiversity assessment in relation to environmental condition is pressingly required here and elsewhere.
The original contributions presented in the study are included in the article/Supplementary Material, further inquiries can be directed to the corresponding author.
UK: Conceptualization, methodology, investigation, formal analysis, writing–original draft, writing–review and editing. FOA: Supervision, methodology, writing–original draft. FCA: Writing–review and editing. OO: Methodology, writing–review and editing. AE: Land use delineation, writing–review and editing. FAA: Writing–review and editing. All authors read and approved this manuscript.
This work was supported by British Ecological Society (BES: EA21/1280) in a grant given to the first author. No funds were received for open access publication fees from anyway, as funding from BES did not include open access publication fees.
Professor Jani Heino of Biodiversity Unit, Finnish Environment Institute (SYKE), Natural Environment Centre, Oulu, Finland was helpful in providing advice and feedback with aspects of beta-diversity in R. We are happy to acknowledge the substantial time and effort to reviewing, and the constructive criticism of editors and anonymous referees/reviewers. This experiment complied with the current laws of the country, Nigeria in which it was performed.
The authors declare that the research was conducted in the absence of any commercial or financial relationships that could be construed as a potential conflict of interest.
All claims expressed in this article are solely those of the authors and do not necessarily represent those of their affiliated organizations, or those of the publisher, the editors and the reviewers. Any product that may be evaluated in this article, or claim that may be made by its manufacturer, is not guaranteed or endorsed by the publisher.
The Supplementary Material for this article can be found online at: https://www.frontiersin.org/articles/10.3389/fenvs.2022.1022776/full#supplementary-material
Al-Shami, S. A., Heino, J., Che, Salmah, Suhaila, A. H., and Madrus, M. R. (2013). Drivers of beta diversity of macroinvertebrate communities in tropical forest streams. Freshw. Biol. 58, 1126–1137. doi:10.1111/fwb.12113
Alahuhta, J., Lindholm, M., Bove, C. P., Chappuis, E., Clayton, J., de Winton., M., et al. (2018). Global patterns in the metacommunity structuring of lake macrophytes: Regional variations and driving factors. Oecologia 188, 1167–1182. doi:10.1007/s00442-018-4294-0
Anderson, M. J., Gorley, R. N., and Clark, K. L. (2009). Primer 6 & PERMANOVA+. 6.1.13 & 1.0. 3 ed. Plymouth, UK: PRIMER-E Ltd.
Anderson, M. J. (2001). A new method for nonparametric multivariate analysis of variance. Austral Ecol. 26, 32–46. doi:10.1046/j.1442-9993.2001.01070.x
Anderson, M. J., Crist, T. O., Chase, J. M., Vellend., M., Inouye., B. D., Freestone, A. L., et al. (2011). Navigating the multiple meanings of β diversity: A roadmap for the practicing ecologist. Ecol. Lett. 14, 19–28. doi:10.1111/j.1461-0248.2010.01552.x
Anderson, M. J., Ellingsen, K. E., and McArdle, B. H. (2006). Multivariate dispersion as a measure of beta diversity. Ecol. Lett. 9, 683–693. doi:10.1111/j.1461-0248.2006.00926.x
APHA (1995). Standard methods for the examination of water and wastewater. Washington, DC: American Public Health Association.
Arimoro, F. O., Abubakar, M. D., Obi-Iyeke, G. E., and Keke, U. N. (2021). Achieving sustainable river water quality for rural dwellers by prioritizing the conservation of macroinvertebrates biodiversity in two Afrotropical streams. Environ. Sustain. Indic. 10, 100103. doi:10.1016/j.indic.2021.100103
Arimoro, F. O., and Keke, U. N. (2021). Stream biodiversity and monitoring in north central, Nigeria: The use of macroinvertebrate indicator species as surrogates. Environ. Sci. Pollut. Res. 28, 31003–31012. doi:10.1007/s11356-021-12922-w
Arimoro, F. O., and Keke, U. N. (2017). The intensity of human-induced impacts on the distribution and diversity of macroinvertebrates and water quality of Gbako River, North Central, Nigeria. Energy Ecol. Environ. 2 (2), 143–154. doi:10.1007/s40974-016-0025-8
Barboza, L. G. A., Mormul, R. P., and Higuti, J. (2015). Beta diversity as a tool for determining priority streams for management actions. Water Sci. Technol. 71, 1429–1435. doi:10.2166/wst.2015.112
Barnosky, A. D., Matzke, N., Tomiya al., S., Wogan, G. O. U., Swartz, B., Quental, T. B., et al. (2011). Has the Earth’s sixth mass extinction already arrived? Nature 471, 51–57. doi:10.1038/nature09678
Baselga, A., Orme, C. D. L., Villeger, S., De Bortoli, J., and Leprieur, F. (2017). Betapart: Partitioning beta diversity into turnover and nestedness components. Available at: http://CRAN.R-project.org/package=betapart.
Baselga, A. (2010). Partitioning the turnover and nestedness components of beta diversity. Glob. Ecol. Biogeogr. 19, 134–143. doi:10.1111/j.1466-8238.2009.00490.x
Baselga, A. (2012). The relationship between species replacement, dissimilarity derived from nestedness, and nestedness. Glob. Ecol. Biogeogr. 21, 1223–1232. doi:10.1111/j.1466-8238.2011.00756.x
Beisner, B. E., Peres-Neto, P. R., Lindström, E., Barnett, A., and Longhi, M. L. (2006). The role of environmental and spatial processes in structuring lake communities from bacteria to fish. Ecology 87, 2985–2991. doi:10.1890/0012-9658(2006)87[2985:TROEAS]2.0.CO;2
Bini, L. M., Landeiro, V. L., Padial, A. A., Siqueira, T., and Heino, J. (2014). Nutrient enrichment is related to two facets of beta diversity for stream invertebrates across the United States. Ecology 95, 1569–1578. doi:10.1890/13-0656.1
Blanchet, F. G., Legendre, P., and Borcard, D. (2008). Forward selection of explanatory variables. Ecology 89, 2623–2632. doi:10.1890/07-0986.1
Borcard, D., Legendre, P., and Drapeau, P. (1992). Partialling out the spatial component of ecological variation. Ecology 73, 1045–1055. doi:10.2307/1940179
Boyero, L., Pearson, R. G., Dudgeon, D., Graca, M. A. S., Gessner, M. O., Albarino, R. J., et al. (2011). Global distribution of a key trophic guild contrasts with common latitudinal diversity patterns. Ecology 92, 1839–1848. doi:10.1890/10-2244.1
Brittain, J. E., Heino, J., Friberg, N., Aroviita, J., Kahlert, M., Karjalainen, S., et al. (2020). Ecological correlates of riverine diatom and macroinvertebrate alpha and beta diversity across Arctic Fennoscandia. Freshw. Biol. 67, 49–63. doi:10.1111/fwb.13616
Cai, Y., Xu, H., Vilmi, A., Tolonen, K. T., Tang, X., Qin, B., et al. (2017). Relative roles of spatial processes, natural factors and anthropogenic stressors in structuring a Lake macroinvertebrate metacommunity. Sci. Total Environ. 601e602, 1702.
Cai, Y., Zhang, Y., Hu, Z., Deng, J., Qin, B., Yin, H., et al. (2019). Metacommunity ecology meets bioassessment: Assessing spatio-temporal variation in multiplefacets of macroinvertebrate diversity in human-influenced large lakes. Ecol. Indic. 103, 713–721. doi:10.1016/j.ecolind.2019.03.016
Castilloescriva, A., Aguilaralberola, J. A., and Mesquitajoanes, F. (2017). Spatial and environmental effects on a rock-pool metacommunity depend on landscape setting and dispersal mode. Freshw. Biol. 62, 1004–1011. doi:10.1111/fwb.12920
Castro, D. M. P., Dolédec, S., and Callisto, M. (2018). Land cover disturbance homogenizes aquatic insect functional structure in neotropical savanna streams. Ecol. Indic. 84, 573–582. doi:10.1016/j.ecolind.2017.09.030
Ceballos, G., Ehrlich, P. R., Barnosky, A. D., Garcia, A., Pringle, R. M., and Palmer, T. M. (2015). Accelerated modern human-induced species losses: Entering the sixth mass extinction. Sci. Adv. 1, e1400253. doi:10.1126/sciadv.1400253
Chase, J. M. (2007). Drought mediates the importance of stochastic community assembly. Proc. Natl. Acad. Sci. U. S. A. 104, 17430–17434. doi:10.1073/pnas.0704350104
Chase, J. M. (2003). Experimental evidence for alternative stable equilibria in a benthic pond food web. Ecol. Lett. 6, 733–741. doi:10.1046/j.1461-0248.2003.00482.x
Chase, J. M. (2010). Stochastic community assembly causes higher biodiversity in more productive environments. Science 328, 1388–1391. doi:10.1126/science.1187820
Collen, B., Whitton, F., Dyer, E. E., Baillie, J. E., Cumberlidge, N., Darwall, W. R., et al. (2014). Global patterns of freshwater species diversity, threat and endemism. Glob. Ecol. Biogeogr. 23, 40–51. doi:10.1111/geb.12096
Costa, S. S., and Melo, A. S. (2008). Beta diversity in stream macroinvertebrate assemblages: Among-site and among-microhabitat components. Hydrobiologia 598, 131–138. doi:10.1007/s10750-007-9145-7
Cottenie, K., Michels, E., Nuytten, N., and De Meester, L. (2003). Zooplankton metacommunity structure: Regional vs. local processes in highly interconnected ponds. Ecology 84, 991–1000. doi:10.1890/0012-9658(2003)084[0991:zmsrvl]2.0.co;2
de Moor, I., Day, J., and de Moor, F. (2003). “Guides to the freshwater invertebrates of southern Africa,” in Volume 7: Insecta I. Ephemeroptera, odonata and plecoptera. WRC report No. TT 207/03 (Pretoria, South Africa: Water Research Commission).
Declerck, S. A., Coronel, J. S., Legendre, P., and Brendonck, L. (2011). Scale dependency of processes structuring metacommunities of cladocerans in temporary pools of High-Andes wetlands. Ecography 34, 296–305. doi:10.1111/j.1600-0587.2010.06462.x
Donohue, I., Jackson, A. L., Pusch, M. T., and Irvine, K. (2009). Nutrient enrichment homogenizes lake benthic assemblages at local and regional scales. Ecology 90, 3470–3477. doi:10.1890/09-0415.1
Dornelas, M., Gotelli, N. J., McGill, B., Shimadzu, H., Moyes, F., Sievers, C., et al. (2015). Assemblage time series reveal biodiversity change but not systematic loss. Science 344, 296–299. doi:10.1126/science.1248484
Dray, S., Pélissier, R., Couteron, P., Legendre, M. J. Fortin P., PeresNeto., P. R., Peres-Neto, P. R., et al. (2012). Community ecology in the age of multivariate multiscale spatial analysis. Ecol. Monogr. 82, 257–275. doi:10.1890/11-1183.1
Edegbene, A. O., Arimoro, F. O., Keke, U. N., and Odume, O. N. (2021a). Identifying and classifying macroinvertebrate indicator signature traits and ecological preferences along urban pollution gradient in the Niger Delta. Environ. Pollut. 281, 117076. doi:10.1016/j.envpol.2021.117076
Edegbene, A. O., Arimoro, F. O., Odume, O. N., Ogidiaka, E., and Keke, U. N. (2021b). Can macroinvertebrate traits Be explored and applied in biomonitoring riverine systems draining forested catchments? Front. Water 3, 607556. doi:10.3389/frwa.2021.607556
FAO (2010). Global forest resources assessment 2010. Rome, Italy: Food and Agriculture Organization of the United Nations.
Feio, M. J., Hughes, R. M., Serra, S. R. Q., Nichols, S. J., Kefford, B. J., Lintermans, M., et al. (2022). Fish and macroinvertebrate assemblages reveal extensive degradation of the world's rivers. Glob. Chang. Biol. doi:10.1111/gcb.16439
Fugère, V., Kasangaki, A., and Chapman, L. J. (2016). Land use changes in an afrotropical biodiversity hotspot affect stream alpha and beta diversity. Ecosphere 7, e01355. doi:10.1002/ecs2.1355
Gordon, N. D., Mc Mahon, T. A., and Finlayson, B. L. (1994). Stream hydrology, an introduction forecologists. New York, NY: John Wiley and Sons.
Gothe, E., Angeler, D. G., and Sandin, L. (2013). Metacommunity structure in a small boreal stream network. J. Anim. Ecol. 82, 449–458. doi:10.1111/1365-2656.12004
Hansen, M. C., Potapov, P. V., Moore, R., Hancher, M., Turubanova, S. A., Tyukavina, A., et al. (2013). High-resolution global maps of 21st-century forest cover change. Science 342, 850–853. doi:10.1126/science.1244693
Hawkins, C. P., Mykrä, H., Oksanen, J., and Vander Laan, J. J. (2015). Environmental disturbance can increase beta diversity of stream macroinvertebrate assemblages. Glob. Ecol. Biogeogr. 24, 483–494. doi:10.1111/geb.12254
Heino, J. (2011). A macroecological perspective of diversity patterns in the freshwater realm. Freshw. Biol. 56, 1703–1722. doi:10.1111/j.1365-2427.2011.02610.x
Heino, J., and Alahuhta, J. (2015). Elements of regional beetle faunas: Faunal variation and compositional breakpoints along climate, land cover and geographical gradients. J. Anim. Ecol. 84, 427–441. doi:10.1111/1365-2656.12287
Heino, J., Gronroos, M., Ilmonen, J., Karhu, T., Niva, M., and Paasivirta, L. (2013). Environmental heterogeneity and β diversity of stream macroinvertebrate communities at intermediate spatial scales. Freshw. Sci. 32 (1), 142–154. doi:10.1899/12-083.1
Heino, J., Melo, A., Bini, L., Altermatt, F., Al-Shami, S., Angeler, D., et al. (2015). A comparative analysis reveals weak relationships between ecological factors and beta diversity of stream insect metacommunities at two spatial levels. Ecol. Evol. 5, 1235–1248. doi:10.1002/ece3.1439
Heino, J., Melo, A. S., Siqueira, T., Soininen, J., Valanko, S., and Bini, L. M. (2015). Metacommunity organisation, spatial extent and dispersal in aquatic systems: Patterns, processes and prospects. Freshw. Biol. 60, 845–869. doi:10.1111/fwb.12533
Heino, J. (2013). The importance of metacommunity ecology for environmental assessment research in the freshwater realm. Biol. Rev. 88, 166–178. doi:10.1111/j.1469-185x.2012.00244.x
Jamoneau, A., Passy, S. I., Soininen, J., Leboucher, T., and Tison-Rosebery, J. (2018). Beta diversity of diatom species and ecological guilds: Response to environmental and spatial mechanisms along the stream watercourse. Freshw. Biol. 63 (1), 62–73. doi:10.1111/fwb.12980
Jeffries, M., and Mills, D. (1990). Freshwater ecology principles and applications. London: Belhaven Press.
Jiang, X., Pan, B., Jiang, W., Hou, Y., Yang, H., Zhu, P., et al. (2021). The role of environmental conditions, climatic factors and spatial processes in driving multiple facets of stream macroinvertebrate beta diversity in a climatically heterogeneous mountain region. Ecol. Indic. 124, 107407. doi:10.1016/j.ecolind.2021.107407
Kasangaki, A., Chapman, L. J., and Balirwa, J. (2008). Land use and the ecology of benthic macroinvertebrate assemblages of high-altitude rainforest streams in Uganda. Freshw. Biol. 53, 681–697. doi:10.1111/j.1365-2427.2007.01925.x
Keke, U. N., Arimoro, F. O., Auta, Y. I., and Ayanwale, A. V. (2017). Temporal and Spatial variability in Macroinvertebrate community structure in relation to environmental variables in Gbako River, Niger State, Nigeria. Trop. Ecol. 58 (2), 229–240.
Keke, U. N., Arimoro, F. O., Ayanwale, A. V., Odume, O. N., and Edegbene, A. O. (2020b). Biodiversity patterns along seasonality and environmental factors of stream macroinvertebrate communities of North-Central Nigeria. Egypt. J. Aquat. Biol. Fish. 24 (4), 521–534. doi:10.21608/ejabf.2020.102102
Keke, U. N., Arimoro, F. O., Ayanwale, A. V., and Odume, A. O. Edegbene (2021a). Weak Relationships among macroinvertebrates beta diversity (β), river status, and environmental correlates in a tropical biodiversity hotspot. Ecol. Indic. 129, 202 107868. doi:10.1016/j.ecolind.2021.107868
Keke, U. N., Mgbemena, A. S., Arimoro, F. O., and Omalu, I. C. J. (2020a). Biomonitoring of effects and accumulations of heavy metals insults using some helminth parasites of fish as bio-indicators in an afrotropical stream. Front. Environ. Sci. 8, 576080. doi:10.3389/fenvs.2020.576080
Keke, U. N., Omoigberale, M. O., Ezenwa, I., Yusuf, A., Biose, E., Nweke, N., et al. (2021b). Macroinvertebrate communities and physicochemical characteristics along an anthropogenic stress gradient in a southern Nigeria stream: Implications for ecological restoration. Environ. Sustain. Indic. 12, 100157. doi:10.1016/j.indic.2021.100157
Larsen, S., and Ormerod, S. J. (2014). Anthropogenic modification disrupts species co-occurrence in stream invertebrates. Glob. Chang. Biol. 20, 51–60. doi:10.1111/gcb.12355
Laurance, W. F., Sayer, J., and Cassman, K. G. (2014). Agricultural expansion and its impacts on tropical nature. Trends Ecol. Evol. 29, 107–116. doi:10.1016/j.tree.2013.12.001
Leal, C. G., Pompeu, P. S., Gardner, T. A., Leitão, R. P., Hughes, R. M., Kaufmann, P. R., et al. (2016). Multiscale assessment of human-induced changes to Amazonian instream habitats. Landsc. Ecol. 31, 1725–1745. doi:10.1007/s10980-016-0358-x
Legendre, P., and Anderson, M. J. (1999). Distance-based redundancy analysis: Testing multispecies responses in multifactorial ecological experiments. Ecol. Monogr. 69, 1–24. doi:10.1890/0012-9615(1999)069[0001:dbratm]2.0.co;2
Legendre, P. (2014). Interpreting the replacement and richness difference components of beta diversity. Glob. Ecol. Biogeogr. 23, 1324–1334. doi:10.1111/geb.12207
Leibold, M. A., Holyoak, M., Mouquet, N., Amarasekare, P., Chase, J. M., Hoopes, M. F., et al. (2004). The metacommunity concept: A framework for multi-scale community ecology. Ecol. Lett. 7, 601–613. doi:10.1111/j.1461-0248.2004.00608.x
Li, Z., Chen, X., Jiang, X., Tonkin, J. D., Xie, Z., and Heino, J. (2021). Distance decay of benthic macroinvertebrate communities in a mountain river network: Do dispersal routes and dispersal ability matter? Sci. Total Environ. 758, 143630. doi:10.1016/j.scito.tenv.2020.143630
Li, Z., Jiang, X., Wang, J., Meng, X., Heino, J., and Xie, Z. (2019). Multiple facets of stream macroinvertebrate alpha diversity are driven by different ecological factors across an extensive altitudinal gradient. Ecol. Evol. 9, 1306–1322. doi:10.1002/ece3.4841
Li, Z., Liu, Z., Heino, J., Jiang, X., Wange, J., Tang, T., et al. (2020). Discriminating the effects of local stressors from climatic factors and dispersal processes on multiple biodiversity dimensions of macroinvertebrate communities across subtropical drainage basins. doi:10.1016/j.scitotenv.2019.134750
Libório, R. A., Tanaka, M. O., Velasco, J., Vaughan, I. P., and Ormerod, S. J. (2016). Contrasting effects of natural and anthropogenic stressors on beta diversity in river organisms. Glob. Ecol. Biogeogr. 22, 796–805. doi:10.1111/geb.12060
López-Delgado, E. O., Winemiller, K. O., and Villa-Navarro, F. A. (2020). Local environmental factors influence beta-diversity patterns of tropical fish assemblages more than spatial factors. Ecology 101 (2), e02940. doi:10.1002/ecy.2940
Lorion, C. M., and Kennedy, B. P. (2009). Relationships between deforestation, riparian forest buffers and benthic macroinvertebrates in neotropical headwater streams. Freshw. Biol. 54, 165–180. doi:10.1111/j.1365-2427.2008.02092.x
Low-Décarie, L., Chivers, C., and Granados, M. (2014). Rising complexity and falling explanatory power in ecology. Front. Ecol. Environ. 12, 412–418. doi:10.1890/130230
Magurran, A. E., Dornelas, M., Moyes, F., Gotelli, N. J., and McGill, B. (2015). Rapid biotic homogenization of marine fish assemblages. Nat. Commun. 6, 8405. doi:10.1038/ncomms9405
Maloney, K., Munguia, P., and Mitchell, R. (2011). Anthropogenic disturbance and landscape patterns affect diversity patterns of aquatic benthic macroinvertebrates. J. North Am. Benthol. Soc. 30, 284–295. doi:10.1899/09-112.1
Masese, F. O., Kitaka, N., Kipkemboi, J., Gettel, G. M., Irvine, K., and McClain, M. E. (2014). Macroinvertebrate functional feeding groups in Kenyan highland streams: Evidence for a diverse shredder guild. Freshw. Sci. 33, 435–450. doi:10.1086/675681
McGill, B. J., Dornelas, M., Gotelli, N. J., and Magurran, A. E. (2015). Fifteen forms of biodiversity trend in the Anthropocene. Trends Ecol. Evol. 30, 104–113. doi:10.1016/j.tree.2014.11.006
Merritt, R. W., and Cummins, K. W. (1996). An introduction to the aquatic insects of North America. 3rd Edn. Dubuque, IA: Kendall-Hunt.
Mouquet, N., and Loreau, M. (2003). Community patterns in source-sink metacommunities. Am. Nat. 162 (5), 544–557. doi:10.1086/378857
Murray-Stocker, D., and Murray-Stocker, K. M. (2020). Consistent metacommunity structure despite inconsistent drivers of assembly at the continental scale. J. Anim. Ecol. 89 (7), 1678–1689. doi:10.1111/1365-2656.13220
Newbold, T., Hudson, L. N., Hill, S. L. L., Contu, S., Lysenko, I., Senior, R. A., et al. (2015). Global effects of land use on local terrestrial biodiversity. Nature 520, 45–50. doi:10.1038/nature14324
Nicacio, G., and Juen, L. (2018). Relative roles of environmental and spatial constraints in assemblages of Chironomidae (Diptera) in Amazonian floodplain streams. Hydrobiologia 820, 201–213. doi:10.1007/s10750-018-3657-1
Oksanen, J., Blanchet, F. G., Friendly, M., Kindt, R., Legendre, P., McGlinn, D., et al. (2017). Vegan: Community ecology package. R package version 2.4-3. Available at: http://CRAN.R-project.org/package=vegan.
Oksanen, J., Blanchet, F. G., Kindt, R., Legendre, P., Minchin, P. R., and O’Hara Rb, R. B. (2013). vegan: Community Ecology Package. R package version 2.0-10. Available at: http://CRAN.R-project.org/package=vegan.
Padial, A. A., Thomaz, S. M., and Agostinho, A. A. (2009). Effects of structural heterogeneity provided by the floating macrophyte Eichhornia azurea on the predation efficiency and habitat use of the small Neotropical fish Moenkhausia sanctaefilomenae. Hydrobiologia 624, 161–170. doi:10.1007/s10750-008-9690-8
Passy, S. I., and Blanchet, F. G. (2007). Algal communities in human-impacted stream ecosystems suffer beta-diversity decline. Divers. Distributions 13, 670–679. doi:10.1111/j.1472-4642.2007.00361.x
Peres-Neto, P. R., Legendre, P., Dray, S., and Borcard, D. (2006). Variation partitioning of species data matrices: Estimation and comparison of fractions. Ecology 87, 2614–2625. doi:10.1890/0012-9658(2006)87[2614:vposdm]2.0.co;2
Petsch, D. K., Blowes, S. A., Melo, A. S., and Chase, J. M. (2021b). A synthesis of land use impacts on stream biodiversity across metrics and scales. Ecology 00 (00), e03498. doi:10.1002/ecy.3498
Petsch, D. K., Saito, V. S., Landeiro, V. L., Silva, T. S. L., Bini, L. M., Heino, J., et al. (2021a). Beta diversity of stream insects differs between boreal and subtropical regions, but land use does not generally cause biotic homogenization. Freshw. Sci. 40 (1), 53–64. doi:10.1086/712565
Poff, N. L. (1997). Landscape filters and species traits: Towards mechanistic understanding and prediction in stream ecology. J. north Am. Benthol. Soc. 16 (2), 391–409. doi:10.2307/1468026
Roa-Fuentes, C. A., Heino, J., Cianciaruso, M. V., Ferraz, S., Zeni, J. O., and Casatti, L. (2019). Taxonomic, functional, and phylogenetic β-diversity patterns of stream fish assemblages in tropical agroecosystems. Freshw. Biol. 64, 447–460. doi:10.1111/fwb.13233
Rocha, M. P., Bini, L. M., Domisch, S., Tolonen, K. T., Jyrkänkallio-Mikkola, J., Soininen, J., et al. (2018). Local environment and space drive multiple facets of stream macroinvertebrate beta diversity. J. Biogeogr. 45, 2744–2754. doi:10.1111/jbi.13457
Rocha, M. P., Heino, J., Machado-Velho, L. F., Lansac-Tôha, F. M., and Lansac-Tôha, F. (2017). Fine spatial grain, large spatial extent and biogeography of macrophyte-associated cladoceran communities across Neotropical floodplains. Freshw. Biol. 62 (3), 559–569. doi:10.1111/fwb.12885
Roque, F. O., Siqueira, T., Bini, L. M., Ribeiro, M. C., Tambosi, L. R., Ciocheti, G., et al. (2010). Untangling associations between chironomid taxa in Neotropical streams using local and landscape filters. Freshw. Biol. 55, 847–865. doi:10.1111/j.1365-2427.2009.02314.x
Siqueira, T., Lacerda, C. G. T., and Saito, V. S. (2015). How does landscape modification induce biological homogenization in tropical stream metacommunities? Biotropica 47, 509–516. doi:10.1111/btp.12224
Soininen, J. (2014). A quantitative analysis of species sorting across organisms and ecosystems. Ecology 95 (12), 3284–3292. doi:10.1890/13-2228.1
Soininen, J., Heino, J., and Wang, J. (2018). A meta-analysis of nestedness and turnover components of beta diversity across organisms and ecosystems. Glob. Ecol. Biogeogr. 27 (1), 96–109. doi:10.1111/geb.12660
Solar, R. R. D. C., Barlow, J., Ferreira, J., Berenguer, E., Lees, A. C., Thomson, J. R., et al. (2015). How pervasive is biotic homogenization in human-modified tropical forest landscapes? Ecol. Lett. 18 (10), 1108–1118. doi:10.1111/ele.12494
Souffreau, C., Van der Gucht, K., van Gremberghe, I., Kosten, S., Lacerot, G., Lobão, L. M., et al. (2015). Environmental rather than spatial factors structure bacterioplankton communities in shallow lakes along a > 6000 km latitudinal gradient in South America. Environ. Microbiol. 17 (7), 2336–2351. doi:10.1111/1462-2920.12692
Tonkin, J. D., Arimoro, F. O., and Haase, P. (2016). Exploring stream communities in a tropical biodiversity hotspot: Biodiversity, regional occupancy, niche characteristics and environmental correlates. Biodivers. Conserv. 25, 975–993. doi:10.1007/s10531-016-1101-2
Vinson, M. R., and Hawkins, C. P. (2003). Broad-scale geographical patterns in local stream insect genera richness. Ecography 26, 751–767. doi:10.1111/j.0906-7590.2003.03397.x
Wantzen, K. M. (2006). Physical pollution: Effects of gully erosion on benthic invertebrates in a tropical clear-water stream. Aquat. Conserv. 16, 733–749. doi:10.1002/aqc.813
Watson, J. E. M., Shanahan, D. F., Di Marco, M., Allan, J., Laurance, W. F., Sanderson, E. W., et al. (2016). Catastrophic declines in wilderness areas undermine global environment targets. Curr. Biol. 26, 2929–2934. doi:10.1016/j.cub.2016.08.049
Weiher, E., and Keddy, P. A. (1995). Assembly rules, null models, and trait dispersion: New questions from old patterns. Oikos 7, 159–164. doi:10.2307/3545686
Whittaker, R. H. (1960). Vegetation of the siskiyou mountains, Oregon and California. Ecol. Monogr. 30, 279–338. doi:10.2307/1943563
Keywords: beta diversity, biodiversity, ecological predictors, forest modification, anthropogenic disturbances
Citation: Keke UN, Arimoro FO, Edegbene AO, Akamagwuna FC, Assie FAGJ and Odume ON (2022) Biotic homogenization of stream macroinvertebrates in an Afrotropical Anthropocene: Land use and ecological correlates. Front. Environ. Sci. 10:1022776. doi: 10.3389/fenvs.2022.1022776
Received: 18 August 2022; Accepted: 17 November 2022;
Published: 30 November 2022.
Edited by:
José Francisco Gonçalves Júnior, University of Brasilia, BrazilReviewed by:
Danielle Petsch, Federal University of Rio Grande do Norte, BrazilCopyright © 2022 Keke, Arimoro, Edegbene, Akamagwuna, Assie and Odume. This is an open-access article distributed under the terms of the Creative Commons Attribution License (CC BY). The use, distribution or reproduction in other forums is permitted, provided the original author(s) and the copyright owner(s) are credited and that the original publication in this journal is cited, in accordance with accepted academic practice. No use, distribution or reproduction is permitted which does not comply with these terms.
*Correspondence: Unique N. Keke, bi5rZWtlQGZ1dG1pbm5hLmVkdS5uZw==, dW5pcXVla2tuQGdtYWlsLmNvbQ==
†ORCID: Unique N. Keke, orcid.org/0000-0002-9528-0599; Francis O. Arimoro, orcid.org/0000-0001-6100-4011; Augustine O. Edegbene: orcid.org/0000-0003-4032-3923; Oghenekaro N. Odume, orcid.org/0000-0001-5220-3254
Disclaimer: All claims expressed in this article are solely those of the authors and do not necessarily represent those of their affiliated organizations, or those of the publisher, the editors and the reviewers. Any product that may be evaluated in this article or claim that may be made by its manufacturer is not guaranteed or endorsed by the publisher.
Research integrity at Frontiers
Learn more about the work of our research integrity team to safeguard the quality of each article we publish.