- 1State Key Labortary of Biogeology and Environmental Geology, School of Environmental Studies, China University of Geosciences, Wuhan, China
- 2Department of Environmental Sciences, Kohat University of Science and Technalogy, Kohat, Pakistan
- 3Department of Botany, Abdul Wali Khan University Mardan, Mardan, Pakistan
- 4Natural and Medical Sciences Research Center, University of Nizwa, Nizwa, Oman
- 5Department of Zoology, College of Science, King Saud University, Riyadh, Saudi Arabia
- 6Department of Medicine and Infectious Diseases, Faculty of Veterinary Medicine, Cairo University, Giza, Egypt
- 7Chrono-Environnement Laboratory, UMR CNRS 6249, Bourgogne, Franche-Comté University, Besançon, France
- 8Pharmacology Department, Faculty of Veterinary Medicine, Suez Canal University, Ismailia, Egypt
Groundwater contamination through potentially harmful metals (PHMs) is an environmental hazard in Pakistan with significant human health risk reports. The current research was conducted in Sheikhupura District, which is a major industrial site in Punjab, Pakistan. According to the Punjab Directorate of Industries in Pakistan, there are a total of 748 industries in this area. These industries produce a lot of waste and effluent, which contaminate the environment with harmful and toxic materials. Continuous irrigation with industrial effluent and sewage sludge may make groundwater sources vulnerable. Therefore, we collected 243 groundwater samples from community tube wells to investigate the groundwater quality cconcerning PHM contaminations in the study area. This research presents the values of pH, total dissolved solids (TDS), electrical conductivity (EC), and potentially harmful metals (PHMs) like arsenic (As), manganese (Mn), lead (Pb), zinc (Zn), copper (Cu), nickel (Ni), and iron (Fe). PHMs such as As (91%), Mn (14%), Pb (97%), Fe (45%), Zn (15%), in these samples were beyond the permitted limit recommended by the world health organization (WHO). Principal component analysis (PCA) results with total variability of (60%) reveal that the groundwater sources of the study area are contaminated about 30.9, 31.3, and 37.6% of contaminations of groundwater sources of this study are resulted from geogenic sources, anthropogenic sources, or both geogenic and anthropogenic sources, respectively. Such sources may include rock-water interaction, mining actions, agricultural practices, domestic sewage, and industrial effluent in the study area. Saturation indices show that the aquifers of the study area are saturated with lead hydroxide, zinc hydroxide, and goethite minerals, indicating that these minerals have a vital role in the contamination of groundwater. Health risk assessment results predicted that the non-carcinogenic risk (HQ) values of PHMs were found within the permissible limit (<1), except As (1.58E+00) for children, while carcinogenic risk (CR) values of all selected PHMs were lower than the maximum threshold CR value (1 × 10−4).
Introduction
Potentially harmful metals (PHMs) in drinking groundwater sources are commonly reported environmental issue (Nawab et al., 2018a). Most of the PHMs contain arsenic (As), zinc (Zn), lead (Pb), mercury (Hg), manganese (Mn), iron (Fe), copper (Cu), and nickel (Ni), (Papazotos, 2021), which are released into groundwater sources as a result of natural and anthropogenic actions (Chen et al., 2020; Nawab et al., 2021). PHMs can negatively affect human health and is the common cause of various health diseases such as cancer, hyperkeratosis, melanosis, peripheral vascular diseases, lung diseases, and hypertension are some of the health outcomes associated with toxic metals ranging from acute to chronic effects (Nawab et al., 2018b; Khalid et al., 2020; Ricolfi et al., 2020). The important physicochemical structures or setups that contribute the mobility of PHMs into the aquifers are either lowering or increasing pH in the watershed (Nawab et al., 2018c; Alsubih et al., 2021). PHMs in groundwater can also rise due to a low hydrological slope, which causes lesser infiltration of water, or an arid climate, which causes evaporating accumulation (Nawab et al., 2018d; Kaur et al., 2020). Furthermore, the elevated concentration of PHMs in groundwater sources is due to mining actions, industrial waste-water recharge, and agricultural pesticides (Masood et al., 2019; Ullah et al., 2022a).
Groundwater is a key source of freshwater for drinking, irrigation, and industries everywhere across the globe, especially in developing countries, but it is regularly challenged by environmental conditions and human activity (Nawab et al., 2017; Ullah et al., 2021). Almost one-third of the population around the world drink from freshwater sources (Sharma et al., 2021). Groundwater is the only source of drinking water in certain dry and semi-arid regions. In recent decades, water supply has suffered an increase in challenges, especially quality control issues and the loss of freshwater sources (Nawab et al., 2016; Ali et al., 2021). Groundwater quality is affected by various factors, including recharge sources, lithology, hydrodynamic setting, mineralization of watersheds, water-rock interaction, such as mineral dissolution, ion exchange, redox, and human impacts (Masood et al., 2019; He et al., 2021).
A wide-ranging appraisal of groundwater eminence is needed to configure the hydro-geochemistry by several approaches. For this purpose, researchers have advantageously implemented numerous methods to examine the hydrochemical features of groundwater chemistry (Tokatli and Ustaoğlu, 2020; Tokatlı et al., 2022). Many geo-statistical methods and multivariate statistical analysis have been implied to broadly comprehend the geochemistry of groundwater, such as heavy metal evaluation index (HEI), Pearson correlation index (PCI), and factor analysis (FA), spatial distribution of contaminants level, water quality index (WQI), mineral phases, and health risk assessment in many developing countries (Tokatli et al., 2021b). When it comes to addressing fundamental human requirements, water quality is as vital as availability because a lack of water and sanitation causes many public health issues (Tokatli et al., 2021a; Ahmad et al., 2021b). The reliability and sustainable alternative characteristics of aquifers within a region are often used to evaluate the consumption and accessibility of local water resources in recent decades (Zhou et al., 2021).
Pakistan is also facing the problem of groundwater contamination and is severely water-stressed in terms of both surface and groundwater supplies, which are decreased significantly in recent decades. In contrast, household groundwater consumption is high (Hussain et al., 2021; Iqbal et al., 2021). Over-exploitation has resulted in a considerable increase in groundwater quality problems in several parts of Pakistan, especially in Punjab Province. Groundwater with high levels of PHMs is a severe health concern, although research on the impacts is still limited (Ismail and Ahmed, 2021). Punjab groundwater vulnerability has increased in recent decades due to a variety of factors. In detained extremely contaminated groundwater, total dissolved solids (TDS) levels of 400–20,000 mg/L, As 0.5–200 μg/L have been reported (Ullah et al., 2021).
Previous information concerning PHMs pollution in drinking groundwater sources of Punjab Province, Pakistan, has addressed minor regions. To investigate a realistic situation of groundwater sources polluted with PHMs, conducting a detailed survey of drinking groundwater sources seems compulsory. Therefore, we chose district Sheikhupura, of Punjab, Pakistan, for the current research work due to the increased populations and industrialization in the study area. Sheikhupura District is a major industrial site in Punjab, Pakistan. Since 1969, the Sheikhupura Industrial Estate has existed. The Punjab Small Industries Corporation (PSIC) was established in 1973. According to the Punjab Directorate of Industries in Pakistan, there are a total of 748 industries in this area. These industries produce a lot of waste and effluent, which contaminates the environment with harmful and toxic elements. Continuous irrigation with industrial effluent and sewage sludge may make groundwater sources vulnerable. There is no plan in the study area by any industrial unit or municipality to treat wastewater and effluent before dumping it down into the drains (Aslam et al., 2020). In this context, it is necessary to investigate the drinking water sources concerning PHMs to protect the health risk of the local inhabitants. Based on the above consideration, this research aimed to (i) to investigate the levels of PHMs such as As, Mn, Pb, Zn, Cu, Ni, and Fe, in drinking groundwater sources of the study area: (ii) to calculate health risk index of PHMs via oral ingestion of groundwater: (iii) to find the mineral phases of the selected PHMs in groundwater through PHREEQC interactive software: and (iv) to understand the source provenance of selected PHMs released into the aquifers of the study area.
Study area
The research was carried out in the Sheikhupura district of Punjab, Pakistan. The study area is located at 31° 27' 48′ N, latitude and 74°' 6′ 0′ E, longitude, as shown in (Figure 1), and is the semi-arid zone of Punjab, Province, Pakistan. The study area’s population is 3,321,029, of which 25% is urban. District Sheikhupura undergoes intense weather changes from winter to summer. The weather is chilly and foggy from mid-December to mid-February, with mild precipitation. The temperature rises in April and the 3 months of May, June, and July are quite hot, although monsoon season begins in mid-June and lasts until August. The district’s average rainfall is 630 mm (Shaheen et al., 2019). The main growing crops in the study area are wheat, rice, sugarcane, and vegetables (Abbas and Cheema, 2015).
Geological setting
The ground slope in the southwest is around 0.3–0.4 m per kilometer in length. The surrounding flooding plains in the center of the plain are elevated to a height of 15.24 (m) by terraces or bars. Exploratory drilling revealed that a 1,500 (m) thick quaternary alluvium with scattered bedrock hills had overlain Precambrian basement rocks, breaking the plain’s flat relief. The area is covered in meander-belt deposits, stream-belt deposits, and floodplain deposits (Aslam et al., 2020). The soils consist of the alluvial deposits of the river Indus and its tributaries. Individual strata’s vertical and horizontal continuity is limited in diverse alluvial deposits (Ehsan et al., 2020). The soil is generally medium-textured reddish-brown to grayish-brown, with a high percentage of acceptable to very fines and tiny amounts of clay and gravel. Fine to medium sand, silt, and clay make up most of the alluvial complex. Pebbles of siltstone and mudstone are found embedded in silty or clayey sand. Despite their variety, the alluvial sediments constitute a coherent highly transmissive no artesian aquifer. The strata above 91.44 (m) are compacted but extremely productive. In the research region, the hydraulic head ranges from 129 (m) to 171(m) (with an average of 150 m) (Siddique et al., 2020).
Chemical and statistical analysis
The groundwater samples were collected from community tube wells in Sheikhupura, Punjab, Pakistan, following standard protocol adopted by (Bux et al., 2022a). The total of (n = 243) drinking groundwater samples were collected for heavy metals detection and other physicochemical parameters. The sampling survey was performed in the summer 2021. The groundwater samples were collected in contamination-free polyethylene bottles having a capacity of 1.5L. Afore sampling, the groundwater sources were pumped for several minutes to avoid the effect of stagnant water. The basic water quality parameters like pH, total dissolved solids (TDS), and electrical conductivity (EC) were measured in the field by using a pH meter and electrochemical analyzer (Hac-44600–00, Loveland, United States). The samples for PHMs detection like, Fe, Mn, Zn, Pb, and Cu, were acidified with 3% HNO3 to adjust the pH of the samples to less than 2. The samples were also filtered through Whatman filter paper (No.0.42 µm) to protect the atomic absorption spectrophotometer (APHA, 1998) and confirm its accuracy (Ashraf et al., 2020). The groundwater samples were kept in an ice-box then instantly shifted to the laboratory for further analysis. The concentration of PHMs was measured by Inductively Coupled Plasma Mass Spectrometry (ICPMS Agilent 7500-United States).
Regular quality control checks, standards operating protocols, reagent blanks, standard calibration, and duplicate analyses were used to achieve accuracy and precision in the analytical data results following (Ahmad et al., 2021a). The reagents used for the analysis were bought from Germany (Merck Company). All glassware was carefully cleaned with deionized water and a 30 percent HCl solution to eliminate the contaminants. Glassware was oven-dried after being washed. After six samples, reagent blanks were used to monitor and examine contamination, with the concentration of the blank being subtracted from the groundwater concentration. Following the standard protocol for detection of PHMs adopted by (Abbas and Cheema, 2015) and (Aragay et al., 2011): Ni 0.07 μg L−1, Pb 0.04 μg L−1, Cu 0.014 μg L−1, Mn 0.002 μg L−1, Fe 0.02 μg L−1, As 1 μg L−1, on (ICPMS Agilent 7500-United States).
Statistical analysis was performed using (IBM-SPSS software version 25) to understand the data set by representing various operations (Muhammad et al., 2021). Descriptive statistics, such as mean, minimum, maximum, Principal component analysis, Pearson correlation matrix, and Quantile-Quantile (Q-Q) plotting, were calculated over SPSS software (IBM-SPSS software version 25, Armonk, NY, United States). Mineral phases of groundwater sources were calculated through the geochemical modeling program PHREEQC (version 3.1) to determine the tendency of groundwater dissolved minerals. The study area map was built over ARC-GIS software (version 9.3).
Face to face interview
In the study area, questionnaire survey was conducted with the help of environmental experts and health workers following (Tiwari et al., 2020). The research team visited various groups of people in the study area, including youngsters (1–16 years old) and adults (17–55 years old). The majority of local respondents specified that the study area’s industrial setup and commercial operations are to blame for the groundwater contamination and health status. The head of the expert team asked several questions concerning their age, profession, ingestion of drinking groundwater sources, education, source of income, and health status. After detailed interviews from inhabitants in the study area, it was concluded that most of them belong to low-income families and can't afford to buy mineral water and used groundwater sources for ingestion motive. Most people were affected by the ingestion of polluted groundwater with PHMs. The commonly reported ailments in the study area were hair loss, lung cancer, bladder, liver, and neuronal damage. To record the toxicity level of PHMs in drinking groundwater human health risk assessment was performed, as shown in Table 3.
Human health risk assessment
Humans were exposed through three different routes: oral consumption, skin contact, and inhalation. Oral intake is still the most vulnerable among these three pathways. This study calculated PHMs exposure through oral ingestion using chronic daily intake (CDI Ingestion), Hazard Quotient (HQ Ingestion), and Hazard Index (HI Ingestion) formulas. These formulas aid in calculating and assessing the exposure level and ingestion rate (IR) of PHMs through groundwater consumption. Human health risk assessment was calculated using equations developed by the USEPA followed by (Usman et al., 2021).
here in equations, CPHMs represents the concentration of potentially harmful metals (mg/L), IR = ingestion rate (2L/day for adult and 1 L/day for children), EF = exposure frequency (365 days/year), ED = exposure duration 350 days, BW = body weight (72 kg for adult and 32 kg for children), AT = averaging time (72 × 365 days). Here CR represents cancer risk, and CSF represents the cancer slope factor for each metal following (Rashid et al., 2019).
Geochemical composition of groundwater
Table 1 shows the geochemistry of groundwater variables of samples (n = 243) collected from the study area. The pH value in groundwater samples varied from 7.6–8.3 with an average value of 7.86 and was found in WHO recommended values, indicating a slightly alkaline nature of groundwater sources. Being a crucial water quality parameter pH determination is compulsory due to its vital role in water chemistry, alkalinity, and solubility of groundwater variables (Rashid et al., 2021). The value of total dissolved solids (TDS) was recorded, ranging from 209–3,116 mg/L with a mean value of 500 mg/L and found within the acceptable limit recommended by WHO (2021). The elevated value of TDS in groundwater sources is due to ion dissolution, which might be credited to progressively depleting salts and minerals over time (Rashid et al., 2019). The electrical conductivity (EC) value in groundwater samples ranged from 249 to 865 μs/cm, with an average value of 511.23 μs/cm. The high (EC) water samples indicate aquifer constituent leakage or suspension and other sources such as saline water bodies (Sharma et al., 2021; Ghani et al., 2022).
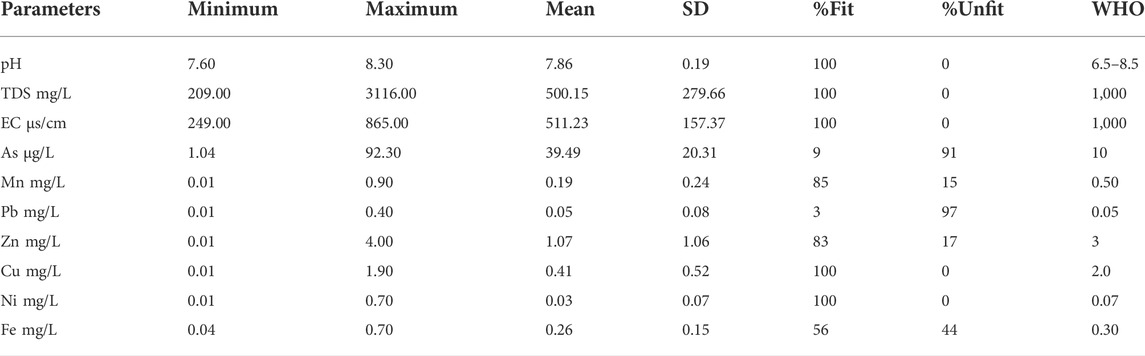
TABLE 1. Descriptive statistics of selected parameters in groundwater samples (n = 243) collected from the study area.
As concentration in groundwater samples varied from 1.04–92.30 μg/L with an average value of 39.49 μg/L, 91% of samples were recorded beyond the recommended value of 10 μg/L recommended by WHO. The presence of high arsenic in drinking groundwater is a direct result of anthropogenic and geogenic sources. Such sources may include waste-water recharge, agricultural practices, mining actions, rock-water interaction, weathering, which has been identified as a severe environmental concern by many researchers such as documented by (Farooqi et al., 2007; Abbas et al., 2021; Rashid et al., 2022b). As can also be released into groundwater due to high salinity, alkalinity, and anoxic conditions (Eslami et al., 2022). Geogenic As pollution of groundwater is more widespread in alluvial aquifers. Gravel, sandstone, silt, and sand that have been in a river canal or flood plain for a long time make up these aquifers (Mallongi et al., 2022). In comparison to other areas of Punjab, Pakistan, the study area has a high concentration of arsenic, which could have negative health consequences for its residents.
The concentration of Mn in groundwater samples ranged from 0.01–0.90 mg/L with an average value of 0.19 mg/L; 15% of samples were beyond the permitted limit recommended by WHO. Mn is a naturally occurring mineral and is one of the most numerous metals on the planet’s surface, in air, water, and soil. It can be found in natural sources of groundwater and surface water and human activity such as mining and industrial wastes (Vinnarasi et al., 2022). Mn is also utilized in various sectors, including iron and steel alloys, batteries, glass, fireworks, cleaning supplies, fertilizers, varnish, fungicides, cosmetics, and livestock feed additions (Jabbo et al., 2022).
The Pb concentration in groundwater samples was in the range of 0.01–0.40 mg/L, with an average value of 0.05 mg/L. Among (n = 243) groundwater samples, 97% samples were beyond WHO’s permitted limit. Lead (Pb) can be found at varying degrees of solubility in rocks and mineral deposits. The elevated (Pb) concentrations in groundwater can result from the leaching of such rocks and minerals (Mallongi et al., 2022). The concentration of Zn in groundwater samples ranges from 0.01–4 mg/L having a mean value of 1.07 mg/L; 15% of samples were beyond the recommended value of WHO. Mostly (Zn) contamination take place in groundwater due to artificial channels, such as by-products of steel manufacture or coal-fired power plants, or waste material combustion. Some fertilizers include zinc, which can seep into groundwater (Bux et al., 2022b). The concentration of Cu varied from 0.01–1.9 mg/L with an average value of 0.41 mg/L and all of the sample were in acceptable guidelines of WHO. The factors which may lead to the elevated concentration of Cu in groundwater sources are corrosion of residential plumbing, faucets, and water fixtures. Copper leaches from plumbing materials, including pipes, fittings, and brass faucets, which is absorbed by water (Ahmad et al., 2021a; Nawab et al., 2022).
The concentration of Ni in groundwater samples were recorded, ranging from 0.01to 0.7 with an average value of 0.03 mg/L, and all of the samples were in WHO recommended guideline value. However, its anthropogenic causes may include steel alloy, electroplating, automobiles, batteries, electronic components, jewelry, sinks, utensils, surgical clips, and kitchen appliances as documented by (Rashid et al., 2021). On the other hand, (Ni) may be present in some groundwater sources due to dissolution from nickel ore-bearing rocks (Jehan et al., 2020; Bhatti et al., 2021). The Fe concentration in groundwater samples ranged from 0.04–0.70 mg/L with an average value of 0.26 mg/L; among (n = 243), 45% of samples were beyond the permitted limit recommended by WHO. Iron is the second most abundant metallic element in the Earth’s crust, while it has a low concentration in water. The most prevalent sources of iron in groundwater are naturally occurring, such as weathering of iron-containing minerals in rocks (Ghosh et al., 2020; Ullah et al., 2022b). The results of this research were compared with study of (Khalid et al., 2020) conducted in Vehari, Punjab, Pakistan, and the comparison shows similarity with the above mentioned research. Similarly this study results was also compared with the study of (Tokatli, 2021) conducted in industrial and agriculture zone of Turkey, who has precieved high concentration of such selected PHMs in groundwater. Likely another research which was conducted by (Varol and Tokatlı, 2022) and (Tokatli et al., 2022), in Turkey and comparison of the current research work shows that the concentratons of selected PHMs was higher the these research work.
Comparison of the current research with previous studies in Pakistan
Pakistan’s environmental issues are mostly linked to the country’s current economic and social imbalances. Due to population migration from rural to urban areas, Pakistan’s main cities are seeing haphazard, uncontrolled expansion, worsening the problem (Abbas and Cheema, 2015). Numerous previous researchers have also found high concentrations of metals in groundwater than acceptable limits in several regions of Pakistan. The research conducted by (Afzal et al., 2014) in the industrial area of Kasur, Pakistan, exposed that the PHMs concentration like Ni (0.11), Cr (1.32), Fe (0.56), Pb (0.14), Zn (0.14) mg/L, respectively, mainly were higher than the permitted limit recommended by WHO for drinking purposes. Moreover, the research conducted in Sialkot city of Punjab, Pakistan, by (Ullah et al., 2009) has also reported high levels of PHMs in drinking groundwater sources: Ni (0.10), Zn (0.16), Cu (0.06), Cr (0.03), Pb (0.49), Mn (0.03), Fe (0.30) mg/L, respectively. The research conducted by (Ul Haq et al., 2011) in 18 districts in Pakistan has also reported that the Pb concentration (0.146) mg/L, in drinking water, was beyond WHO’s permitted limit. Likely another research work on groundwater concerning PHMs in Vehari, Pakistan, conducted by (Khalid et al., 2020), also reported the toxic heavy metals in drinking groundwater: Pb (0.18), Cd (0.01), Ni (0.13), Cu (0.10), Zn (0.55), Fe (1.34) mg/L respectively. The comparison shows that the current research work was almost similar to the previous studies. As a result, the heavy metal pollution situation in Pakistan is of major concern in order to minimize adverse human health effects from various exposure pathways.
Principal component analysis multilinear regression
Principal component analysis multilinear regression (PCA-MLR) is a valuable method used to comprehend the pollution bases of PHMs in groundwater in terms of % age contribution. The pollution index (PI) was calculated for overall groundwater parameters. Then, PCA was measured through the Varimax rotation reduction dimension method. The R2 values were taken from the model summary, then the R2 of individual factors PC1, PC2, PC3, PC4, and PC5 were calculated by removing one component and leaving all the other components as independent values. After the R2 calculation, the R2 difference was estimated by subtracting the R2 value of each element from the overall R2 values. The percentage contribution was calculated by summing the R2 differences.
A total of five principal components were achieved for (n = 243) samples, such as PC1, PC2, PC3, PC4, and PC5, respectively, with eigenvalues of 1.483, 1.321, 1.186, 1.028, and 0.998, respectively, as shown in Table 2. The varience for each factor was calculated to be 14.81%, 13.22%, 11.86%, 10.285%, and 9.977%, respectively, with a total variance of 60.54%. In contrast, the total loading factors were estimated to be 10, as shown in Figure 2. However, PC1 and PC2 were built in a biplot, and their cumulative variability was 28.03% (see Figure 2B).
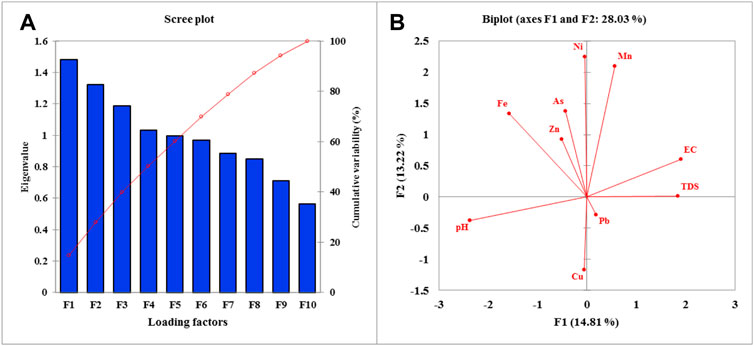
FIGURE 2. (A) Overall loading factors of PCA results, (B) Contribution of the first two loading factors F1 and F2 after varimax rotation.
PC1 was counted with 14.81% variability having an eigenvalue of 1.483 and showing strong and moderate loading for pH, TDS, EC, and Fe, and their numerical values were calculated to be 0.723, 0.564, and 0.577, respectively. PC1 suggested that geogenic input significantly impacts groundwater physicochemical variables, pH and TDS play a vital role in the saturation of water variables in the study area. PC2 was calculated with a variability of 13.22%, having an eigenvalue of 1.321, which shows strong loading for Mn and Ni; their numerical values are 0.608 and 0.645, respectively. Manganese (Mn) is an element that can be found naturally in rocks, soil and subsurface pollution sources. Manganese is rarely found in a water source. It's common in iron-bearing streams, but it's less common than iron (Ahmad et al., 2020).
Moreover, the Mn pollution in the study area is also due to many Steel industries in the study area, such as Liaquat, steel industries, Aziz Steel Industries, Al Fatah steel industries, Agha, steel Industries, they often use Mn for deoxidizing and desulfurizing additives and as an alloying constituent. It can improve the rolling and forging qualities and the strength, toughness, stiffness, hardness, and hardenability of steel. The wastewater release of mentioned industries affects the groundwater quality of the study area. The primary source of nickel (Ni) is ore-bearing rocks, which can contribute to the pollution of Ni in groundwater sources, while the anthropogenic source of Ni in the study area is metal mining, municipal and industrial waste, and automobile emissions. Nickel’s industrial applications include electroplating, steel production, chemical, and metallurgical industries of Sheikhupura, which may pollute the groundwater quality (Jabbar). PC2 is also reflected for geogenic and anthropogenic sources of contamination in groundwater. PC3 was counted with the variability of 11.86% with an eigenvalue of 1.186 and shows strong negative loading for As and Pb having a loading value of −0.683 and −0.515, respectively. The negative values of these two variables show that they have no direct effect on each other. Arsenic (As) can enter groundwater and drinking water from a variety of natural sources as well as manmade acts. Geologic formations (e.g., sedimentary deposits/rocks, volcanic rocks and soils), geothermal, coal, and volcanic activities are key natural sources. Anthropogenic activities such as mining, burning of fossil fuels, using arsenical fungicides, herbicides, and insecticides in agriculture, and using wood preservatives are the primary anthropogenic sources of As pollution of groundwater. Coal combustion has a significant impact on As pollution in the environment. The origin of Pb in shallow groundwater sources may contain naturally occurring ores rich in various pollutants, which seep into water bodies and pollute them. Groundwater contamination with high Pb levels has been related to these ores. Moreover, mining and smelting of ore, manufacturing lead containing products, burning coal and oil, and waste incineration are all anthropogenic sources of lead (Deeba et al., 2019).
PC3 shows the mixed type of contaminant which may contaminate groundwater sources. PC4 shows the variability of 10.285% with an eigenvalue of 1.028 and shows strong loading for TDS and Pb with values of 0.511 and 0.640, respectively. Natural sources, sewage, urban and agricultural run-off, and industries of lead manufacturing products and wastewater all contribute to TDS and Pb in water systems (Masood et al., 2019). PC4 shows the mixed type of source that the aquifers of the study area are contaminated due to geogenic and anthropogenic actions. PC5 was counted with variability of 9.997% with eigenvalue 0.998, which shows strong negative loading for EC and positive for Cu and their values are −0.522, 0.555, respectively, suggesting that these two variables had no direct correlation effect on each other. The high electrical conductivity (EC) in groundwater sources is due to conductive ions coming from dissolved salts and inorganic substances such as sulfides, chloride, and carbonate compounds, while copper (Cu) levels in surface and groundwater are typically relatively low. Copper contamination can occur in the environment due to mining, farming, manufacturing, and municipal or industrial effluent. PC5 reflect mixed type due to geogenic and anthropogenic source of contamination. As shown in Figure 3, principal component analysis (PCA) results with total variability of (60.154%) reveal that the groundwater sources of the study area are contaminated due to (30.9%) geogenic sources, (31.3%) anthropogenic sources, and (37.6%) of mixed type including both geogenic and anthropogenic source of contamination.
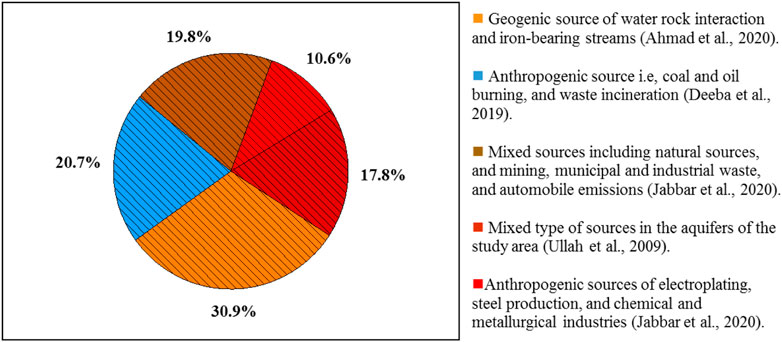
FIGURE 3. Shows percentage contribution of groundwater pollution sources: water-rock interaction, industrial and municipal waste, agricultural practices, and mining activities in the study area.
Mineral phases of potentially harmful metals in groundwater
The presence of various solutes derived from the atmosphere or by the weathering and erosion of rocks affects the natural groundwater quality. The interaction between rock and water causes mineral species to dissolve (Saleh et al., 1999). Under equilibrium conditions, groundwater is saturated with a specific mineral species; although, it may continue to dissolve more minerals, which may eventually precipitate, and the water becomes excessively saturated with that mineral. Chemical equilibrium for a particular mineral species can be observed by calculating the saturation index (SI) (Pande et al., 2020). Saturation data is being used to estimate subsurface minerals. As shown in (Figure 4), the results of mineral phases for groundwater samples, such minerals include hausmannite, zinc hydroxide, pyrochroite, lead hydroxide, goethite, and pyrolusite, respectively. The SI value for hausmannite, zinc hydroxide, and pyrochroite were found in the range of (−7.6941−3.5589), (−1.3947−2.3741), and (−5.1547–−1.8704), and their mean values were recorded to be (−2.856880), (0.713146), and (−3.718981), respectively. The SI value for lead hydroxide, pyrolusite, and goethite were found in the range of (1.7007−3.8798), (−8.1348–−3.4504), and (9.2632–10.1506), and their mean values were recorded to be (2.446345), (−6.168955), and (9.3142), respectively. The saturation indices result of mineral phases shows that the groundwater sources of the study area were saturated for goethite, lead hydroxide, and zinc hydroxide minerals, which indicates that these minerals had a significant role in the contamination of groundwater sources of the study area.
Quantile-quantile plotting
Quantile-Quantile plotting was plotted for PHMs in groundwater sources samples to determine normal distribution of selected PHMs in the study area. To obtain the outcome of Q-Q plotting, groundwater data for selected elemental composition were plotted in the (IBM-SPSS software version 25). It's a graphical method for showing data from the first data set quantiles against the second data set. The expected normal values were shown in the first data set, whereas the observed values were shown in the second data set. At a 45° reference line, both values are sorted up and down in the form of a scatter plot. The standard distribution outline is revealed by the values of quantiles, which can be found in a straight diagonal line. Figure 5 shows the Q-Q plotting result for selected groundwater variables. The quantiles R2 values for As, Mn, Zn, Cu, Ni, and Fe in the normal Q-Q box plots were found to be (0.971), (1), (0.989), (0.991), (1), and (1), respectively.
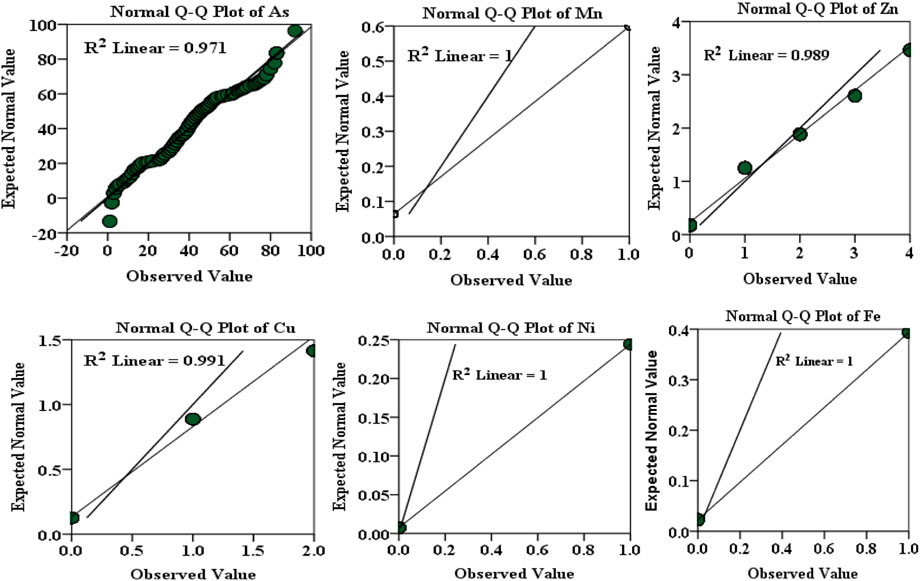
FIGURE 5. Quantile-Quantile (Q-Q) plotting shows distribution of the groundwater variables in the study area.
Human health risk exposure assessment
The health risk assessment is a valuable numerical technique used to calculate the association among toxic elements in drinking water and human health. The health risk estimation model is an internationally recognized method recommended by the USEPA (2005), including hazard identification, dose-effect analysis, exposure assessment, and risk characterization (Ahmad et al., 2021a; Rashid et al., 2022a). Therefore, we also conduct a survey in the study area to estimate risk exposure. In the study area, the research team visited several groups of people, including youngsters (1–16 years old) and adults (17–55 years old). The majority of the population, or 70% of local respondents, believe that the study area’s industrial and commercial activities are to blame for the contamination of the groundwater and the study area’s health situation. In the study area, the commonly reported disorders were irritability, constipation, sleep disorders, hearing loss, exhaustion, cramps, gastrointestinal disease, neurological damage, learning difficulties, low appetite, stunted growth, organ failure, flu, vomiting, coma, and convulsions were among the most prevalent diseases. The effects of PHMs in groundwater were examined in terms of chronic daily intake (CDI), Hazard Quotient (HQ), and Cancer Risk (CR), to better comprehend the exact environmental guidelines and increased health frustration for the local people. In this investigation, CDI, HQ, and CR via oral ingestion route for two groups of populations, children, and adults, were computed following USEPA criteria, and the findings are shown in Table 3. As a result of groundwater consumption, most persons in the research region were highly exposed to PHMs.
As shown in Table 3 the average CDI value of As, Mn, Pb, Zn, Cu, Ni, and Fe was calculated to be 4.73E-04, 2.35E-06, 5.76E-07, 1.29E-05, 4.96E-06, 3.59E-07, and 3.10E-06 respectively. Similarly, the average CDI value of As, Mn, Pb, Zn, Cu, Ni, and Fe, for adults were counted to be 6.01E-05, 2.98E-07, 7.31E-08, 1.63E-06, 6.3E-07, 4.55E-08, and 3.94E-07 respectively. For children, the average non-carcinogenic risk of As, Mn, Pb, Zn, Cu, and Ni was calculated to be 1.58E+00, 1.68E-05, 3.61E-14 4.28E-08, 1.34E-04, and 1.79E-05 respectively. Likewise, the non-carcinogenic value of the abovementioned PHMs for adults were calculated and their values are 2.00E-01, 2.13E-06, 2.09E-05, 5.44E-09, 1.70E-05, and 2.28E-06, respectively. The average carcinogenic risk of As, Pb, and Ni for children was calculated, and their values were found to be 3.16E-04, 1.48E-14, and 2.11E-07, respectively. Similarly, the average carcinogenic risk of As, Pb, and Ni, for adults were found to be 4.01E-05, 8.61E-06, and 2.68E-08, respectively, as shown in Table 3. Thus, it was noticed from the result that PHMs in groundwater sources of the study area shows that the non-carcinogenic risk (HQ) values of PHMs were found within the permissible limit (<1), except As (1.58E+00) for children, while carcinogenic risk (CR) values of all selected PHMs were lower than the maximum threshold CR value (1 × 10−4). The result of this study was compared with the study conducted by (Ashraf et al., 2021) and (Mohmand et al., 2015), the comparison showed that the results of this research was similar to the above mentioned research work.
Implications for the sustainable management of groundwater resources
Groundwater contains various essential elements for human health and growth, including Ni, Cu, Mn, Fe, and Zn. The majority of these necessary elements must be consumed in a certain amount as part of our regular food nutrition. However, due to their persistent, genotoxic, and bioavailable character, excessive intake of the heavy metals commonly utilized in daily life have negative health consequences. Furthermore, PHMs can easily infiltrate the food chain, interacting with marine and terrestrial animals, which most likely move and transmit water contaminants to the ecosystem. Therefore, pollutants can easily migrate from one biological system to another, posing serious health concerns to individuals and the environment. Excessive consumption of PHMs, is deemed hazardous to human health. Lower to moderate PTEs ingestion causes hearing loss, lower intelligence, flu, growth retardation, and gastrointestinal problems in humans, whereas excessive PHMs exposure causes irritability, sleep disturbances, vomiting, constipation, cramps, loss of appetite, exhaustion, convulsions, neurological impairment, coma, organ failure, and death. This issue is made worse in nations with a shortage of other water supplies, frequent droughts, and an increase in the importance of groundwater for drinking and cooking. To stop further groundwater degradation and harm to people, it is necessary to concentrate on monitoring and careful management of the current groundwater resources. According to our research, water management authorities should develop a groundwater system monitoring network. By doing so, it will be possible to monitor groundwater quality and quantity in real-time and create measures to prevent the issue from getting worse. Local governments should press for more aggressive and stringent efforts to be taken to establish safe drinking water wells. Effective steps should also be done to raise public awareness of the need to use groundwater resources responsibly and safely.
Conclusion
This study was conducted in Sheikhupura, Punjab, Pakistan, concerning the contaminations of PHMs in drinking groundwater sources. The total of 243 samples were collected from various drinking groundwater sources to investigate the levels of PHMs, like As, Mn, Pb, Zn, Cu, Ni, and Fe, along with basic water quality parameters like pH, TDS, and EC. The results show that the mean concentrations of PHMs and other physiochemical parameters of the samples, such as pH (7.86), TDS (500.15 mg/L), EC (511.23 μs/cm), As (39.49 μg/L), Mn (0.19 mg/L), Pb (0.05 mg/L), Zn (1.07 mg/L), Cu (0.41 mg/L), Ni (0.03 mg/L), and Fe (0.26 mg/L) were beyond the permitted limit recommended by world health organization (WHO) for drinking purpose, and among 243 samples, approximately 91%, 14%, 97%, 45%, and 15% of samples have elevated as, Mn, Pb, Fe, and Zn, respectively. Principal component analysis (PCA) results with total variability of (60.154%) reveal that the groundwater sources of the study area are contaminated about 30.9, 31.3 and 37.6% of contaminations of groundwater sources of this study are resulted from geogenic sources, anthropogenic sources, or both geogenic and anthropogenic sources, respectively. Such sources may include rock-water interaction, mining actions, agricultural practices, domestic sewage, and industrial effluent in the study area. Saturation indices results show that the aquifers of the study area are saturated with lead hydroxide, zinc hydroxide, and goethite minerals, indicating that these minerals have a vital role in the contamination of groundwater sources of the study area. Health risk assessment results shows that the non-carcinogenic risk (HQ) values of PHMs were found within the permissible limit (<1), except As (1.58E+00) for children, while carcinogenic risk (CR) values of all selected PHMs were lower than the maximum threshold CR value (1 × 10–4).
Data availability statement
The raw data supporting the conclusions of this article will be made available by the authors, without undue reservation.
Author contributions
ZU: Conceptualization, Methodology, Writing original draft, AR: Methodology, JG: Software and validation, JN: validation, X-CZ: Supervison, Validation and results analysis, MS: Experimental work, AA: Validation, MK and LA: Review and editing, MA-D: Field sampling, JI: Field sampling. All authors have read and agreed to the published version of the manuscript.
Funding
This project was supported by the Researchers Supporting Project number (RSP-2021/218), King Saud University, Riyadh, Saudi Arabia.
Acknowledgments
The authors extend their appreciation to the Researchers Supporting Project 492 number (RSP-2021/218), King Saud University, Riyadh, Saudi Arabia.
Conflict of interest
The authors declare that the research was conducted in the absence of any commercial or financial relationships that could be construed as a potential conflict of interest.
Publisher’s note
All claims expressed in this article are solely those of the authors and do not necessarily represent those of their affiliated organizations, or those of the publisher, the editors and the reviewers. Any product that may be evaluated in this article, or claim that may be made by its manufacturer, is not guaranteed or endorsed by the publisher.
References
Abbas, M., and Cheema, K. (2015). Arsenic levels in drinking water and associated health risk in district Sheikhupura, Pakistan. J. Animal Plant Sci. 25, 719–724.
Abbas, Z., Imran, M., Natasha, N., Murtaza, B., Amjad, M., Shah, N. S., et al. (2021). Distribution and health risk assessment of trace elements in ground/surface water of kot addu, Punjab, Pakistan: A multivariate analysis. Environ. Monit. Assess. 193, 351–362. doi:10.1007/s10661-021-09150-7
Afzal, M., Shabir, G., Iqbal, S., Mustafa, T., Khan, Q. M., and Khalid, Z. M. (2014). Assessment of heavy metal contamination in soil and groundwater at leather industrial area of Kasur, Pakistan. CLEAN–Soil, Air, Water 42, 1133–1139. doi:10.1002/clen.201100715
Ahmad, A., Hussain, S., Rao, S. M., Asghar, A., Irshad, M., Aslam, M., et al. (2020). Comparative studies of lead and heavy metals concentrations in Pakistan soil and its toxic effects. Pol. J. Environ. Stud. 29, 2533–2542. doi:10.15244/pjoes/106028
Ahmad, S., Imran, M., Murtaza, B., Arshad, M., Nawaz, R., Waheed, A., et al. (2021a). Hydrogeochemical and health risk investigation of potentially toxic elements in groundwater along River Sutlej floodplain in Punjab, Pakistan. Environ. Geochem. Health 43, 5195–5209. doi:10.1007/s10653-021-00941-y
Ahmad, S., Imran, M., Murtaza, B., Arshad, M., Nawaz, R., Waheed, A., et al. (2021b). Hydrogeochemical and health risk investigation of potentially toxic elements in groundwater along River Sutlej floodplain in Punjab, Pakistan. Environ. Geochem. Health 43, 5195–5209. doi:10.1007/s10653-021-00941-y
Ali, L., Rashid, A., Khattak, S. A., Gao, X., Jehan, S., and Javed, A. (2021). Geochemical modeling, fate distribution, and risk exposure of potentially toxic metals in the surface sediment of the Shyok suture zone, northern Pakistan. Int. J. Sediment Res. 36, 656–667. doi:10.1016/j.ijsrc.2021.02.006
Alsubih, M., El Morabet, R., Khan, R. A., Khan, N. A., Ahmed, S., Qadir, A., et al. (2021). Occurrence and health risk assessment of arsenic and heavy metals in groundwater of three industrial areas in Delhi, India. Environ. Sci. Pollut. Res. 28, 63017–63031. doi:10.1007/s11356-021-15062-3
Aragay, G., Pons, J., and Merkoçi, A. (2011). Recent trends in macro-micro-and nanomaterial-based tools and strategies for heavy-metal detection. Chem. Rev. 111, 3433–3458. doi:10.1021/cr100383r
Ashraf, A., Chen, X., and Ramamurthy, R. (2021). Modelling heavy metals contamination in groundwater of Southern Punjab, Pakistan. Int. J. Environ. Sci. Technol. 18, 2221–2236. doi:10.1007/s13762-020-02965-w
Ashraf, S., Rizvi, N. B., Rasool, A., Mahmud, T., Huang, G. G., and Zulfajri, M. (2020). Evaluation of heavy metal ions in the groundwater samples from selected automobile workshop areas in northern Pakistan. Groundw. Sustain. Dev. 11, 100428. doi:10.1016/j.gsd.2020.100428
Aslam, B., Ismail, S., and Ali, I. (2020). A GIS-based DRASTIC model for assessing aquifer susceptibility of safdarabad tehsil, Sheikhupura district, Punjab province, Pakistan. Model. Earth Syst. Environ. 6, 995–1005. doi:10.1007/s40808-020-00735-8
Bhatti, Z., Ishtiaq, M., Khan, S., Baig, S., Muhammad, I., Din, Z., et al. (2021). Contamination level, source identification and health risk assessment of potentially toxic elements in drinking water sources of mining and. Pakistan: Non-Mining Areas of Khyber Pakhtunkhwa.
Bux, R. K., Haider, S. I., Mallah, A., Solangi, A. R., Moradi, O., Karimi-Maleh, H., et al. (2022a). Spatial analysis and human health risk assessment of elements in ground water of District Hyderabad, Pakistan using ArcGIS and multivariate statistical analysis. Environ. Res. 210, 112915. doi:10.1016/j.envres.2022.112915
Bux, R. K., Haider, S. I., Mallah, A., Solangi, A. R., Moradi, O., and Karimi-Maleh, H. (2022b11291). Spatial analysis and human health risk assessment of elements in ground water of District Hyderabad, Pakistan using ArcGIS and multivariate statistical analysis. Environmental Research.
Chen, X., Zeng, X.-C., Kawa, Y. K., Wu, W., Zhu, X., Ullah, Z., et al. (2020). Microbial reactions and environmental factors affecting the dissolution and release of arsenic in the severely contaminated soils under anaerobic or aerobic conditions. Ecotoxicol. Environ. Saf. 189, 109946. doi:10.1016/j.ecoenv.2019.109946
Deeba, F., Abbas, N., Butt, M., and Irfan, M. (2019). Ground water quality of selected areas of Punjab and Sind Provinces, Pakistan. Chem. Int. 5, 241–246. doi:10.5281/zenodo.2573563
Ehsan, N., Shan, A., Riaz, S., Uz Zaman, Q., Javied, S., and Jabeen, M. (2020). Health risk assessment due to exposure of arsenic contamination in drinking water of district Shiekhupura, Punjab, Pakistan. Hum. Ecol. Risk Assess. An Int. J. 26, 162–176. doi:10.1080/10807039.2018.1498292
Eslami, H., Esmaeili, A., Razaeian, M., Salari, M., Hosseini, A. N., Mobini, M., et al. (2022). Potentially toxic metal concentration, spatial distribution, and health risk assessment in drinking groundwater resources of southeast Iran. Geosci. Front. 13, 101276. doi:10.1016/j.gsf.2021.101276
Farooqi, A., Masuda, H., Kusakabe, M., Naseem, M., and Firdous, N. (2007). Distribution of highly arsenic and fluoride contaminated groundwater from east Punjab, Pakistan, and the controlling role of anthropogenic pollutants in the natural hydrological cycle. Geochem. J. 41, 213–234. doi:10.2343/geochemj.41.213
Ghani, J., Ullah, Z., Nawab, J., Iqbal, J., Waqas, M., Ali, A., et al. (2022). Hydrogeochemical characterization, and suitability assessment of drinking groundwater: Application of geostatistical approach and geographic information system. Front. Environ. Sci. 10, 874464. doi:10.3389/fenvs.2022.874464
Ghosh, G. C., Khan, M., Hassan, J., Chakraborty, T. K., Zaman, S., Kabir, A., et al. (2020). Human health risk assessment of elevated and variable iron and manganese intake with arsenic-safe groundwater in Jashore, Bangladesh. Sci. Rep. 10, 5206–5209. doi:10.1038/s41598-020-62187-5
He, X., Li, P., Wu, J., Wei, M., Ren, X., and Wang, D. (2021). Poor groundwater quality and high potential health risks in the datong basin, northern China: Research from published data. Environ. Geochem. Health 43, 791–812. doi:10.1007/s10653-020-00520-7
Hussain, R., Khattak, S. A., Ali, L., Sattar, S., Zeb, M., and Hussain, M. L. (2021). Impacts of the linear flowing industrial wastewater on the groundwater quality and human health in Swabi, Pakistan. Environ. Sci. Pollut. Res. 28, 56741–56757. doi:10.1007/s11356-021-13842-5
Iqbal, J., Su, C., Rashid, A., Yang, N., Baloch, M. Y. J., Talpur, S. A., et al. (2021). Hydrogeochemical assessment of groundwater and suitability analysis for domestic and agricultural utility in southern Punjab, Pakistan. Water 13, 3589. doi:10.3390/w13243589
Ismail, S., and Ahmed, M. F. (2021). GIS-based spatio-temporal and geostatistical analysis of groundwater parameters of Lahore region Pakistan and their source characterization. Environ. Earth Sci. 80, 719–726. doi:10.1007/s12665-021-10034-9
Jabbar, M. Spatial analysis of the factors responsible for waterborne diseases in rural communities located along the Hudiara Drain, Lahore. Pak. Geogr. Rev. 75, 84–94.
Jabbo, J. N., Isa, N. M., Aris, A. Z., Ramli, M. F., and Abubakar, M. B. (2022). Geochemometric approach to groundwater quality and health risk assessment of heavy metals of Yankari Game Reserve and its environs, Northeast Nigeria. J. Clean. Prod. 330, 129916. doi:10.1016/j.jclepro.2021.129916
Jehan, S., Khattak, S. A., Muhammad, S., Ali, L., Rashid, A., and Hussain, M. L. (2020). Human health risks by potentially toxic metals in drinking water along the Hattar Industrial Estate, Pakistan. Environ. Sci. Pollut. Res. 27, 2677–2690. doi:10.1007/s11356-019-07219-y
Kaur, M., Kumar, A., Mehra, R., and Kaur, I. (2020). Quantitative assessment of exposure of heavy metals in groundwater and soil on human health in Reasi district, Jammu and Kashmir. Environ. Geochem. Health 42, 77–94. doi:10.1007/s10653-019-00294-7
Khalid, S., Shahid, M., Shah, A. H., Saeed, F., Ali, M., Qaisrani, S. A., et al. (2020). Heavy metal contamination and exposure risk assessment via drinking groundwater in Vehari, Pakistan. Environ. Sci. Pollut. Res. 27, 39852–39864. doi:10.1007/s11356-020-10106-6
Mallongi, A., Rauf, A. U., Daud, A., Hatta, M., Al-Madhoun, W., Amiruddin, R., et al. (2022). Health risk assessment of potentially toxic elements in maros karst groundwater: A Monte Carlo simulation approach. Geomatics, Nat. Hazards Risk 13, 338–363. doi:10.1080/19475705.2022.2027528
Masood, N., Farooqi, A., and Zafar, M. I. (2019). Health risk assessment of arsenic and other potentially toxic elements in drinking water from an industrial zone of gujrat, Pakistan: A case study. Environ. Monit. Assess. 191, 95–15. doi:10.1007/s10661-019-7223-8
Mohmand, J., Eqani, S. a. M. a. S., Fasola, M., Alamdar, A., Mustafa, I., Ali, N., et al. (2015). Human exposure to toxic metals via contaminated dust: Bio-accumulation trends and their potential risk estimation. Chemosphere 132, 142–151. doi:10.1016/j.chemosphere.2015.03.004
Muhammad, N., Nafees, M., Ge, L., Khan, M. H., Bilal, M., Chan, W. P., et al. (2021). Assessment of industrial wastewater for potentially toxic elements, human health (dermal) risks, and pollution sources: A case study of gadoon amazai industrial estate, swabi, Pakistan. J. Hazard. Mater. 419, 126450. doi:10.1016/j.jhazmat.2021.126450
Nawab, J., Din, Z. U., Ahmad, R., Khan, S., Zafar, M. I., Faisal, S., et al. (2021). Occurrence, distribution, and pollution indices of potentially toxic elements within the bed sediments of the riverine system in Pakistan. Environ. Sci. Pollut. Res. 28, 54986–55002. doi:10.1007/s11356-021-14783-9
Nawab, J., Farooqi, S., Xiaoping, W., Khan, S., and Khan, A. (2018a). Levels, dietary intake, and health risk of potentially toxic metals in vegetables, fruits, and cereal crops in Pakistan. Environ. Sci. Pollut. Res. 25, 5558–5571. doi:10.1007/s11356-017-0764-x
Nawab, J., Ghani, J., Khan, S., and Xiaoping, W. (2018b). Minimizing the risk to human health due to the ingestion of arsenic and toxic metals in vegetables by the application of biochar, farmyard manure and peat moss. J. Environ. Manag. 214, 172–183. doi:10.1016/j.jenvman.2018.02.093
Nawab, J., Khan, S., Ali, S., Sher, H., Rahman, Z., Khan, K., et al. (2016). Health risk assessment of heavy metals and bacterial contamination in drinking water sources: A case study of malakand agency, Pakistan. Environ. Monit. Assess. 188, 286–298. doi:10.1007/s10661-016-5296-1
Nawab, J., Khan, S., Khan, M. A., Sher, H., Rehamn, U. U., Ali, S., et al. (2017). Potentially toxic metals and biological contamination in drinking water sources in chromite mining-impacted areas of Pakistan: A comparative study. Expo. Health 9, 275–287. doi:10.1007/s12403-016-0240-8
Nawab, J., Khan, S., and Xiaoping, W. (2018c). Ecological and health risk assessment of potentially toxic elements in the major rivers of Pakistan: General population vs. Fishermen. Chemosphere 202, 154–164. doi:10.1016/j.chemosphere.2018.03.082
Nawab, J., Khan, S., Xiaoping, W., Rahman, A., Ali, H., Qamar, Z., et al. (2018d). Spatial distribution of toxic metals in drinking water sources and their associated health risk in district buner, Northern Pakistan. Hum. Ecol. Risk Assess. An Int. J. 24, 615–626. doi:10.1080/10807039.2017.1395684
Nawab, J., Rahman, A., Khan, S., Ghani, J., Ullah, Z., Khan, H., et al. (2022). Drinking water quality assessment of government, non-government and self-based schemes in the disaster affected areas of khyber pakhtunkhwa, Pakistan. Expo. Health, 1–17. doi:10.1007/s12403-022-00511-2
Pande, C. B., Moharir, K. N., Singh, S. K., and Dzwairo, B. (2020)., 22. India, 7453–7471. doi:10.1007/s10668-019-00531-0Groundwater evaluation for drinking purposes using statistical index: Study of akola and buldhana districts of Maharashtra, IndiaEnviron. Dev. Sustain.
Papazotos, P. (2021). Potentially toxic elements in groundwater: A hotspot research topic in environmental science and pollution research. Environ. Sci. Pollut. Res. 28, 47825–47837. doi:10.1007/s11356-021-15533-7
Rashid, A., Ayub, M., Javed, A., Khan, S., Gao, X., Li, C., et al. (2021). Potentially harmful metals, and health risk evaluation in groundwater of Mardan, Pakistan: Application of geostatistical approach and geographic information system. Geosci. Front. 12, 101128. doi:10.1016/j.gsf.2020.12.009
Rashid, A., Ayub, M., Khan, S., Ullah, Z., Ali, L., Gao, X., et al. (2022a). Hydrogeochemical assessment of carcinogenic and non-carcinogenic health risks of potentially toxic elements in aquifers of the hindukush ranges, Pakistan: Insights from groundwater pollution indexing, GIS-based, and multivariate statistical approaches. Environ. Sci. Pollut. Res. Int., 1–25. doi:10.1007/s11356-022-21172-3
Rashid, A., Ayub, M., Ullah, Z., Ali, A., Khattak, S. A., Ali, L., et al. (2022b). Geochemical modeling source provenance, public health exposure, and evaluating potentially harmful elements in groundwater: Statistical and human health risk assessment (HHRA). Int. J. Environ. Res. Public Health 19, 6472. doi:10.3390/ijerph19116472
Rashid, A., Khan, S., Ayub, M., Sardar, T., Jehan, S., Zahir, S., et al. (2019). Mapping human health risk from exposure to potential toxic metal contamination in groundwater of lower dir, Pakistan: Application of multivariate and geographical information system. Chemosphere 225, 785–795. doi:10.1016/j.chemosphere.2019.03.066
Ricolfi, L., Barbieri, M., Muteto, P. V., Nigro, A., Sappa, G., and Vitale, S. (2020). Potential toxic elements in groundwater and their health risk assessment in drinking water of Limpopo National Park, Gaza Province, Southern Mozambique. Environ. Geochem. Health 42, 2733–2745. doi:10.1007/s10653-019-00507-z
Saleh, A., Al-Ruwaih, F., and Shehata, M. (1999). Hydrogeochemical processes operating within the main aquifers of Kuwait. J. Arid Environ. 42, 195–209. doi:10.1006/jare.1999.0511
Shaheen, A., Iqbal, J., and Hussain, S. (2019). Adaptive geospatial modeling of soil contamination by selected heavy metals in the industrial area of Sheikhupura, Pakistan. Int. J. Environ. Sci. Technol. (Tehran). 16, 4447–4464. doi:10.1007/s13762-018-1968-4
Sharma, T., Bajwa, B. S., and Kaur, I. (2021). Contamination of groundwater by potentially toxic elements in groundwater and potential risk to groundwater users in the Bathinda and Faridkot districts of Punjab, India. Environ. Earth Sci. 80, 250–265. doi:10.1007/s12665-021-09560-3
Siddique, J., Menggui, J., Shah, M. H., Shahab, A., Rehman, F., and Rasool, U. (2020). Integrated approach to hydrogeochemical appraisal and quality assessment of groundwater from Sargodha District, Pakistan. Geofluids 2020, 1. doi:10.1155/2020/6621038
Tiwari, R., Aulakh, R. S., Bedi, J. S., Gill, J. P. S., and Kumar, A. (2020). Occurrence and spatial distribution of metals and arsenic in groundwater sources of Punjab (India), and their health risk assessment. Toxicol. Environ. Chem. 102, 425–438. doi:10.1080/02772248.2020.1808895
Tokatli, C. (2021). Health risk assessment of toxic metals in surface and groundwater resources of a significant agriculture and industry zone in Turkey. Environ. Earth Sci. 80, 426–438. doi:10.1007/s12665-021-09467-z
Tokatlı, C., Islam, A. R. M. T., Onur, Ş. G., Ustaoğlu, F., Islam, M. S., and Dindar, M. B. (2022). A pioneering study on health risk assessment of fluoride in drinking water in Thrace Region of northwest Türkiye. Groundw. Sustain. Dev. 19, 100836. doi:10.1016/j.gsd.2022.100836
Tokatli, C., Mutlu, E., and Arslan, N. (2021a). Assessment of the potentially toxic element contamination in water of Şehriban Stream (Black Sea Region, Turkey) by using statistical and ecological indicators. Water Environ. Res. 93, 2060–2071. doi:10.1002/wer.1576
Tokatli, C., Titiz, A. M., Uğurluoğlu, A., Islam, M., Ustaoğlu, F., and Islam, A. R. M. (2022). Assessment of the effects of COVID-19 lockdown period on groundwater quality of a significant rice land in an urban area of Türkiye. Environ. Sci. Pollut. Res. 29, 71752–71765. doi:10.1007/s11356-022-20959-8
Tokatli, C., Uğurluoğlu, A., Köse, E., Çiçek, A., Arslan, N., Dayioğlu, H., et al. (2021b). Ecological risk assessment of toxic metal contamination in a significant mining basin in Turkey. Environ. Earth Sci. 80, 17–19. doi:10.1007/s12665-020-09333-4
Tokatli, C., and Ustaoğlu, F. (2020). Health risk assessment of toxicants in Meriç river delta wetland, Thrace region, Turkey. Environ. Earth Sci. 79, 426–437. doi:10.1007/s12665-020-09171-4
Ul Haq, N., Arain, M., Badar, N., Rasheed, M., and Haque, Z. (2011). Drinking water: A major source of lead exposure in karachi, Pakistan. East. Mediterr. Health J. 17 (11), 882–886. doi:10.26719/2011.17.11.882
Ullah, R., Malik, R. N., and Qadir, A. (2009). Assessment of groundwater contamination in an industrial city, Sialkot, Pakistan. Afr. J. Environ. Sci. Technol. 3, 12.
Ullah, Z., Rashid, A., Ghani, J., Talib, M. A., Shahab, A., and Lun, L. (2022a). Arsenic contamination, water toxicity, source apportionment, and potential health risk in groundwater of jhelum basin, Punjab, Pakistan. Biol. Trace Elem. Res. 11, 1. doi:10.1007/s12011-022-03139-0
Ullah, Z., Talib, M. A., Rashid, A., Ghani, J., Shahab, A., Irfan, M., et al. (2021). Hydrogeochemical investigation of elevated arsenic based on entropy modeling, in the aquifers of district sanghar, sindh, Pakistan. Water 13, 3477. doi:10.3390/w13233477
Ullah, Z., Xu, Y., Zeng, X.-C., Rashid, A., Ali, A., Iqbal, J., et al. (2022b). Non-carcinogenic health risk evaluation of elevated fluoride in groundwater and its suitability assessment for drinking purposes based on water quality index. Int. J. Environ. Res. Public Health 19, 9071. doi:10.3390/ijerph19159071
Usman, Q. A., Muhammad, S., Ali, W., Yousaf, S., and Jadoon, I. A. (2021). Spatial distribution and provenance of heavy metal contamination in the sediments of the Indus River and its tributaries, North Pakistan: Evaluation of pollution and potential risks. Environ. Technol. innovation 21, 101184. doi:10.1016/j.eti.2020.101184
Varol, M., and Tokatlı, C. (2022). Seasonal variations of toxic metal (loid) s in groundwater collected from an intensive agricultural area in northwestern Turkey and associated health risk assessment. Environ. Res. 204, 111922. doi:10.1016/j.envres.2021.111922
Vinnarasi, F., Srinivasamoorthy, K., Saravanan, K., Kanna, A. R., Gopinath, S., Prakash, R., et al. (2022). Hydrogeochemical characteristics and risk evaluation of potential toxic elements in groundwater from Shanmughanadhi, Tamilnadu, India. Environ. Res. 204, 112199. doi:10.1016/j.envres.2021.112199
Who (2021). A global overview of national regulations and standards for drinking-water quality. Switzerland: World Health Organization.
Keywords: groundwater, potential harmful metals, mineral phases, source apportionment, health risk indices
Citation: Ullah Z, Rashid A, Ghani J, Nawab J, Zeng X-C, Shah M, Alrefaei AF, Kamel M, Aleya L, Abdel-Daim MM and Iqbal J (2022) Groundwater contamination through potentially harmful metals and its implications in groundwater management. Front. Environ. Sci. 10:1021596. doi: 10.3389/fenvs.2022.1021596
Received: 17 August 2022; Accepted: 10 October 2022;
Published: 07 November 2022.
Edited by:
Guijin Su, Research Center for Eco-environmental Sciences (CAS), ChinaCopyright © 2022 Ullah, Rashid, Ghani, Nawab, Zeng, Shah, Alrefaei, Kamel, Aleya, Abdel-Daim and Iqbal. This is an open-access article distributed under the terms of the Creative Commons Attribution License (CC BY). The use, distribution or reproduction in other forums is permitted, provided the original author(s) and the copyright owner(s) are credited and that the original publication in this journal is cited, in accordance with accepted academic practice. No use, distribution or reproduction is permitted which does not comply with these terms.
*Correspondence: Xian-Chun Zeng, eGlhbmNodW4uemVuZ0BnbWFpbC5jb20=, eGlhbmNodW5femVuZ0Bob3RtYWlsLmNvbQ==