- 1Institute of Biology, Plant Ecology, Freie Universität Berlin, Berlin, Germany
- 2Berlin-Brandenburg Institute of Advanced Biodiversity Research (BBIB), Berlin, Germany
Microplastics enter the soil in a variety of shapes and polymer types altering soil properties with known consequences for plant growth. However, the effects of a range of different microplastic shapes and types on seed germination are mostly unknown. Here, we established a glasshouse experiment that included 12 microplastic types representing different shapes (fibers, films, foams and fragments) and polymers, and mixed each of them with soil at a concentration of 0.4% (w/w). Fifty seeds of Daucus carota were sown and monitored for 49 days to evaluate different germination parameters. Our results showed that microplastic films and fibers decrease seed germination velocity as they may affect soil water status, likely interfering with different phases of seed germination: Seeds may imbibe toxic microplastic leachates, and be affected by a physical blockage; testa rupturing may be delayed as this also depends on water uptake. Microplastic toxic leachates may affect activity of enzymes key for seed germination, and delay embryo growth and radicle emergence. Microplastics, irrespective of their shape and polymer type, increase synchrony of seed germination, which might be linked with microplastics exerting a mild stress on seeds. The final percentage of germination was not affected by microplastics in soil, implying that microplastics did not affect seed viability. Our results showed that microplastics affect seed germination mainly as a function of their shape.
Introduction
Microplastics (MPs) in soil are currently considered an important threat to terrestrial ecosystems. These particles may enter the soil through soil amendments, plastic mulching, irrigation, flooding or atmospheric input (Rillig, 2012; Bläsing and Amelung, 2018). As manufacturers seek to produce plastics with specific properties such as fiexibility, roughness, resistance, and durability (Espí et al., 2006), they may appear in a variety of shapes, polymers, and sizes (Rillig et al., 2019; Helmberger et al., 2020), with known consequences on terrestrial ecosystems (Akdogan and Guven 2019; Rillig and Lehmann, 2020; Xu et al., 2020; Lozano et al., 2021a).
Previous research showed that, as a function of their shape and polymer type, microplastics alter soil properties with consequences for plant growth (De Souza Machado et al., 2019; Lozano et al., 2021b; Zhao et al., 2021). For example, fibers may lead to enhanced soil water content (De Souza Machado et al., 2019; Lozano and Rillig 2020), while foams can increase soil macroporosity, contributing to the observed increase in shoot mass by 27% and 45% respectively, in comparison with the control without microplastics (Lozano et al., 2021b). Films may have positive effects on plant biomass (Qi et al., 2018; Lozano et al., 2021b) while fragments that contain highly toxic substances may negatively affect plant growth (van Kleunen et al., 2020). Such effects could persist in the soil, even in the absence of microplastics (Lozano and Rillig, 2022).
Research about microplastic effects on plant performance has been focused on plant growth, largely overlooking the effects that MPs may have on early stages of plant performance such as seed germination, often considered one of the most vulnerable stages in the life cycle of a plant (Baskin and Baskin, 2014). Recent research has shown that microplastics of polystyrene beads of different sizes may have negative effects on seed germination of Lepidium sativum (Bosker et al., 2019), and that polystyrene nanoplastics (still smaller particles than microplastics) may negatively affect spore germination of ferns (Yuan et al., 2019), although they may have negligible effects on germination of wheat seeds (Lian et al., 2020). Certainly, we do not yet know the effects of microplastics on key germination parameters such as synchrony; and we do not know how this and other parameters such as germination velocity or final percentage of germination might depend on microplastic shape and polymer type.
Plants can have synchronized seed germination (i.e., germination of all seeds occurring simultaneously), bringing the advantage that any suitable conditions can favor all seeds in the seed bank. Likewise, germinating faster may bring benefits in terms of early access to resources (or space) and can lead to reduced competition during the initial stage of establishment (Gioria et al., 2018). In any case, germination should be timed to occur only when conditions for seedling growth and development are suitable, avoiding unfavorable abiotic (e.g., low resources), or biotic (e.g., plant pathogens, competition) conditions. Thus, unfavourable conditions brought about by microplastics in soil may interfere with seed germination.
Microplastics in soil change several soil properties (De Souza Machado et al., 2019; Lozano et al., 2021b), and thus potentially alter conditions for seed germination. For instance, fibers may lead to water being held in the soil for longer, films could decrease soil bulk density, while foams and fragments can increase soil aeration and macroporosity (Lozano et al., 2021b). Such soil properties are known to influence seed germination (Baskin and Baskin, 2014). In addition, MPs can also carry a high diversity of fungal and bacterial pathogens (Curren and Leong., 2019; Gkoutselis et al., 2021), and can present additional challenges to the soil environment due to the leaching of potentially toxic additives (Kim et al., 2020; Waldman and Rillig, 2020), also with potential consequences on seed germination. As microplastics enter the soil in a variety of shapes and polymer types, the effect on seed germination parameters such as velocity, synchrony and final percentage of germination may differ as a function of these plastic properties. To test this, we established a glasshouse experiment that included four microplastic shapes (i.e., fibers, films, foams, and fragments), each of them represented by different polymer types including polyethylene terephthalate (PES), polyamide (PA), polypropylene (PP), low density polyethylene (PE), polyethylene terephthalate (PET), polyurethane (PU), polystyrene (PS) and polycarbonate (PC). We evaluated effects on seed germination parameters of Daucus carota during 49 days after sowing.
Material and methods
Microplastics
We selected twelve different secondary microplastics that represent four microplastic shapes: fibers, films, foams and fragments. We also represented eight polymer types: polyester made to at least 80% of polyethylene terephthalate (PES), polyamide (PA), polypropylene (PP), low density polyethylene (PE), polyethylene terephthalate (PET), polyurethane (PU), polystyrene (PS) and polycarbonate (PC). See details of the plastics in Supplementary Table S1. From these plastics, fibers and films were manually cut with scissors to a length < 5.0 mm or 5.0 mm2, respectively. Microplastic fibers had a length of 1.28 ± 0.03 mm and a diameter of 0.030 ± 0.0008 mm; films had a length of 3.6 ± 1.2 mm2. Foams and fragments (large solid plastics) were cut into small pieces by using a Philips HR3655/00 Standmixer (1,400 W, ProBlend 6 3D Technologie, Netherlands), sieved through 4 mm mesh and, if required, cut with scissors in order the obtain microplastics (i.e., plastics particles < 5 mm2). Microplastics were microwaved (2 min at 500 W) to minimize microbial contamination. Melting points were not reached during microwaving.
Soil preparation
Sandy loam soil (Albic Luvisol; 0.07% N, 0.77% C, pH 6.66) from a dry grassland located in Dedelow, Brandenburg, Germany (53° 37′ N, 13° 77’ W) was collected, air-dried, sieved through a 4 mm mesh size, homogenized, and mixed with each of the microplastics at a concentration of 0.4% (w/w). That is, 0.80 g of each microplastic type was mixed into 200 g of soil for each pot (9 cm diameter, 7.2 cm height, 500 ml). Soil preparation was done separately for each pot in order to provide an equal distribution of microplastics throughout the soil. Microplastics were separated manually and mixed with the soil for 1 min in a large container, before placing it into the pot. We followed the same procedure with the control pots without microplastics, to generate the same soil disturbance.
Experimental design
In September 2019, we established the experiment in a glasshouse with a daylight period set at 12 h, 50 klx, and a temperature regime at 22/18°C day/night with a relative humidity of ∼40%. Pots were watered with 60 ml and incubated for 1 week in order to allow the potential leaching of plastic components into the soil. Then, fifty seeds of Daucus carota, a biennial herbaceous typical of German dryland ecosystems (Federal Agency for nature Conservation 2019), were randomly sowed in each pot, and germination defined as the seedling emergence, was recorded at day 7, 14, 21, 28, 35, 42 and 49 after sowing. Seedlings were removed after emergence. Pots were watered twice per week by gently spraying 30 ml of distilled water onto the soil surface. Prior to sowing, seeds were surface-sterilized with 4% sodium hypochlorite for 5 min and 75% ethanol for 2 min, thoroughly rinsed with sterile water and kept at 4°C for 5 days. Seeds were obtained from a commercial supplier in the region (Rieger-Hofmann GmbH, Blaufelden, Germany). Seed viability test indicated germination of ∼70%.
Our experimental design thus included: four microplastic shapes x three polymer types x 10 replicates = 120 pots. Eighteen additional pots were established as control without microplastics. Pots were randomly distributed in the greenhouse chamber, and their position shifted twice during the experiment to homogenize environmental conditions.
Statistical analyses
To determine the effect of microplastic shape and polymer type on seed germination, we calculated the i) index of germination velocity, which denotes how rapid seeds germinate (where the minimum is 0 and the maximum is 100), ii) index of germination synchrony, which indicates synchronization of germination (where the minimum is 0 and the maximum is 1) and iii) the final germination percentage, indicating the potential to complete germination (where the minimum is 0% and the maximum is 100%) (Ranal and Santana, 2006; Aravind et al., 2021). Germination parameters were calculated using the “germinationmetrics” R package (Aravind et al., 2021). Then, we performed linear models to test the effect of microplastic shape and polymer type on germination parameters. Residuals were checked to validate assumptions of normality and homogeneity. When necessary, we implemented the function “varIdent” to account for heterogeneity. After this, in the selected model, we implemented the function “glht” and the “Dunnett” test from the “multcomp” R package (Hothorn et al., 2008; Bretz et al., 2011), in order to compare each microplastic type with the control (without microplastics). Comparisons between polymer types within each microplastic shape were also performed. Curves of cumulative germination and the other figures were made using the “ggplot2” R package. Additional linear models and multiple comparisons were performed for each day of germination measurement. Statistical analyses were done in R 4.1.0 (R Core Team, 2020).
Results
Our results showed that microplastics in soil affected germination-related parameters such as germination velocity and synchrony. First, we found that ranging from 0 to 100, germination velocity was of 2.7 in soils with fibers, 2.9 with films, 3.4 with foams, 3.2 with fragments and 3.4 in control soils without microplastics (Figure 1). Out of these, we only found differences between control soils without microplastics and soils mixed with fibers (p = 0.03), and a trend for films (p = 0.1). Germination velocity decreased by ∼20% in soils with fibers and by ∼15% in soils with films in comparison with the control soils without microplastics (Figure 1; Table 1, Supplementary Table S2). We also found differences between PE and PU foams (p = 0.02), with values of 2.6 and 4.0 for germination velocity (Figure 1).
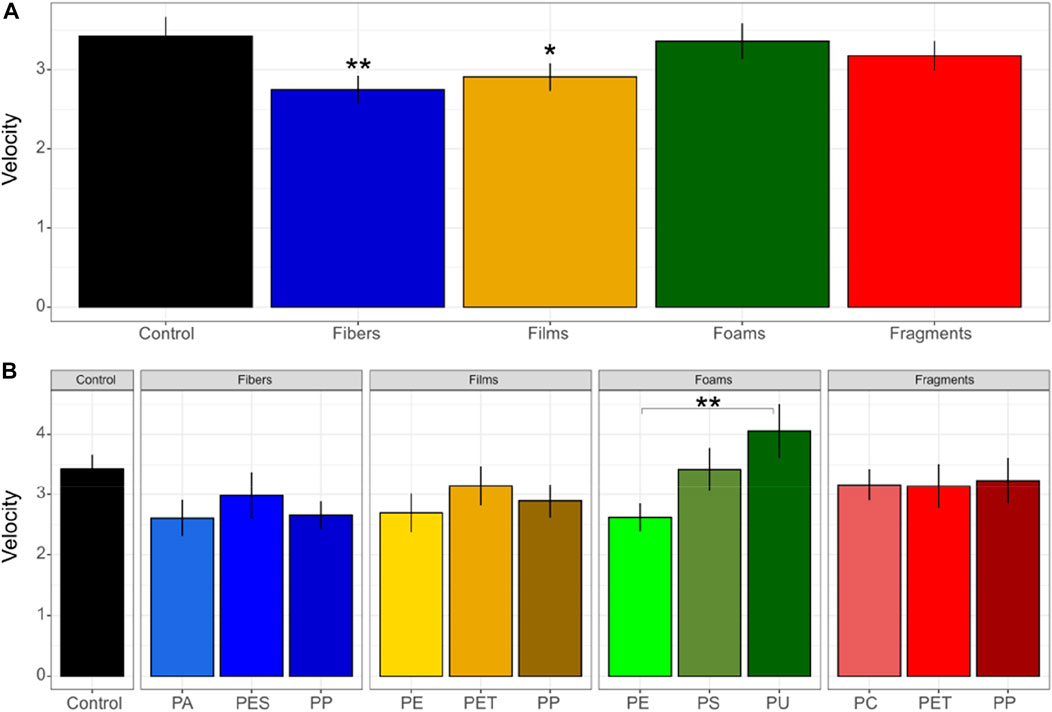
FIGURE 1. Germination velocity of seeds of Daucus carota. Microplastic shape (A) and polymer types effects (B) on germination velocity was evaluated. Differences between microplastic types and the control without microplastics) were tested using Dunnett test. Differences between polymer types within each microplastic shape were tested using Tukey test (**p < 0.05; *p < 0.1). Polymers: PA (polyamide), PES (polyester), PP (polypropylene), LDPE (low density polyethylene), PET (polyethylene terephthalate), PS (polystyrene), PU (polyurethane), and PC (polycarbonate). n = 10 for microplastics, n = 18 for control samples. The index of germination velocity denotes how rapid seeds germinate (where the minimum is 0 and the maximum is 100).
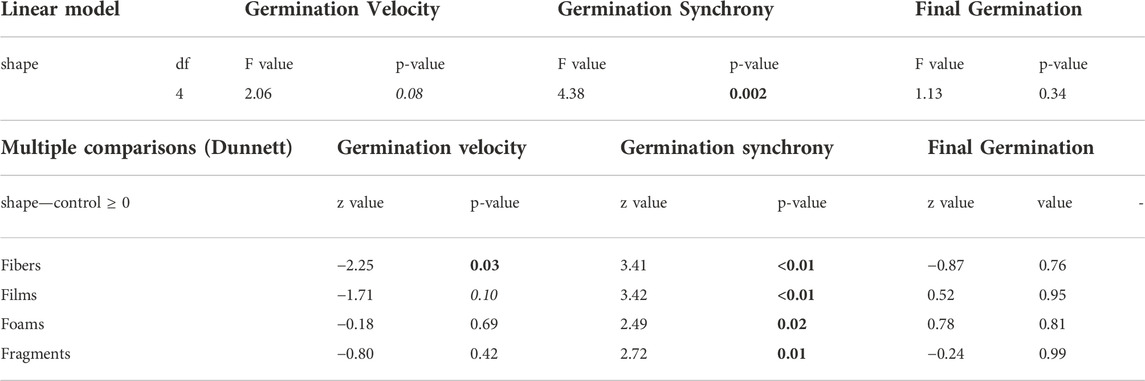
TABLE 1. Microplastic shape effects on seed germination velocity, synchrony and on final germination percentage. Results of linear models and multiple comparisons by using the Dunnett test. Values in bold indicate a strong effect (p < 0.05) and in italics a moderate effect (p < 0.1) of microplastic shape on the dependent variable.
Germination synchrony was of ∼0.37 in soils mixed with fibers and films, ∼0.36 in soils mixed with foams and fragments, and 0.29 in control soils without microplastics (Figure 2), evidencing that germination synchrony increased with each microplastic shape in comparison with the control soil without microplastics (p < 0.05, Figure 2 and Table 1). That is, synchrony increased by ∼24% in soil mixed with fibers, by ∼25% with films, by ∼20% with foams, and by ∼21% in soil mixed with fragments in comparison with the control soil without microplastics. No differences were found among microplastic shapes. Regarding polymer type, we observed that PES and PP fibers, PET and PP films, PU foams and PET fragments were the polymers that strongly increased germination synchrony in Daucus carota (Supplementary Table S3). Differences in germination synchrony were found between PE and PS foams (p = 0.1).
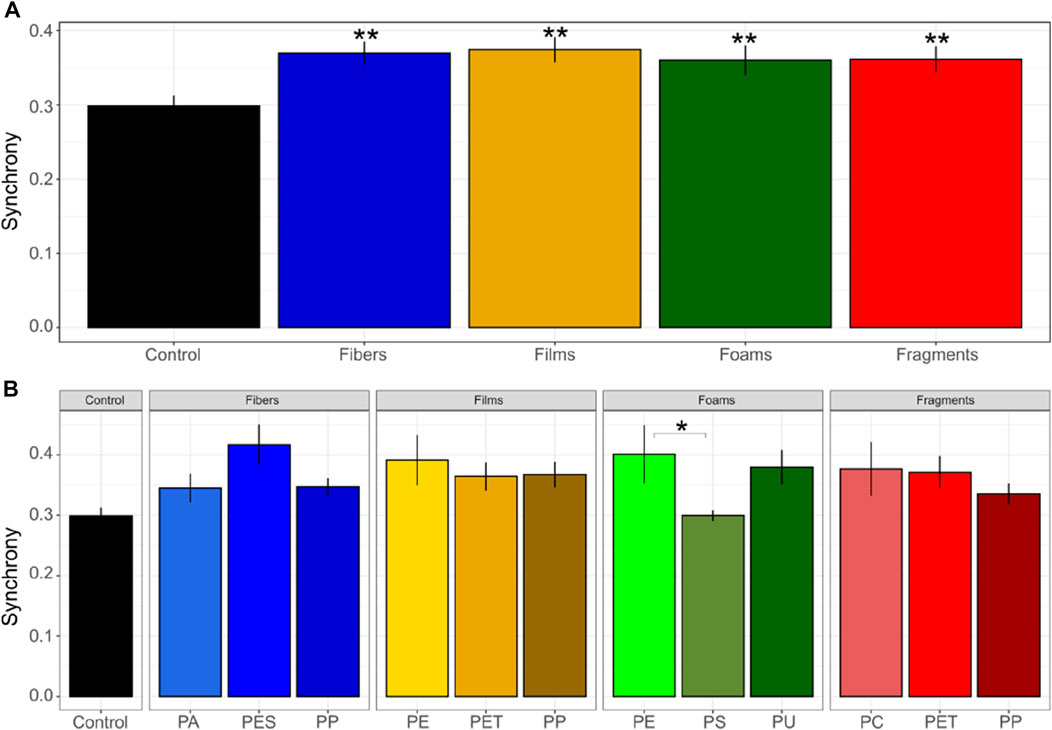
FIGURE 2. Germination synchrony of seeds of Daucus carota. Microplastic shape (A) and polymer types effects (B) on germination synchrony was evaluated. Differences between microplastic types and the control without microplastics) were tested using Dunnett test. Differences between polymer types within each microplastic shape were tested using Tukey test (**p < 0.05; *p < 0.1). Polymers: PA (polyamide), PES (polyester), PP (polypropylene), LDPE (low density polyethylene), PET (polyethylene terephthalate), PS (polystyrene), PU (polyurethane), and PC (polycarbonate). n = 10 for microplastics, n = 18 for control samples. The index of germination synchrony, indicates synchronization of germination (where the minimum is 0 and the maximum is one.
Final percentage of germination was similar among microplastic shapes. It was of ∼39% in soils with fibers, ∼43% with films, ∼44% with foams, ∼41% with fragments and ∼42% in control soils without microplastics (Figure 1). The final percentage of germination was highest with PU foams (∼49%) and lowest with PP fibers (∼37%), however, there were no differences in this germination parameter related to the polymer type (Figure 1).
In addition, our results showed that the final percentage of germination was not evidently affected by microplastics (Figure 3). Nonetheless, the curves of germination showed that on the 7th day germination was lower with microplastics in the soil than in the control soil without microplastics. The germination became asymptotic from the 28th day, and overall, we observed that films and foams showed a tendency to promote germination while fibers and fragments showed an opposite trend (Supplementary Tables S4, S5; Supplementary Figures S1, S2).
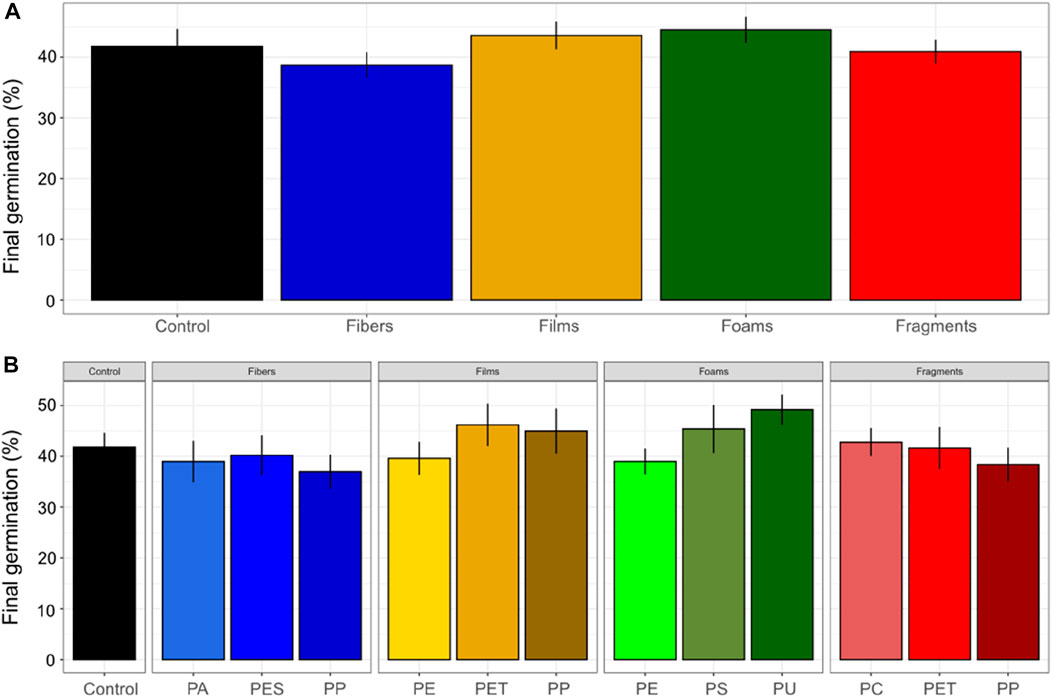
FIGURE 3. Final germination percentage of seeds of Daucus carota. Microplastic shape (A) and polymer types effects (B) on final germination was evaluated. Differences between microplastic types and the control without microplastics) were tested using Dunnett test. Differences between polymer types within each microplastic shape were tested using Tukey test (**p < 0.05; *p < 0.1). Polymers: PA (polyamide), PES (polyester), PP (polypropylene), LDPE (low density polyethylene), PET (polyethylene terephthalate), PS (polystyrene), PU (polyurethane), and PC (polycarbonate). n = 10 for microplastics, n = 18 for control samples. The final germination percentage indicates the potential to complete germination (where the minimum is 0% and the maximum is 100%).
Discussion
Microplastic films and fibers in soil decrease seed germination velocity
Our results showed that microplastic films and fibers in the soil decreased the seed germination velocity of Daucus carota.
Germination is a triphasic proccess that commences with the rapid initial uptake of water by the dry seed (phase I, i.e., imbibition), followed by a plateau phase of stable water absorption and testa ruptures (phase II), and concludes with a burst of water uptake, elongation of the embryonic axis and radical and hypocotyl emergence (phase III) (Finch-Savage and Leubner-Metzger 2006; Srivastava et al., 2021). The presence of MPs in soil may chemically and physically affect seed germination during some or all of these three phases (Figure 4). That is, in the first phase (seed imbibition), seeds are vulnerable to dissolved toxic substances as they imbibe plenty of water from its surroundings through seed pores (Bewley, 1997). Therefore, leached substances from microplastic fibers or films could have been toxic for seeds. Fibers and films can contain hazardous monomers (Lithner et al., 2011), and can carry pathogens and many other pollutants (Wang et al., 2021) that can be leached into the water such as plasticizers, colorants and other harmful agents such as heavy metals (Rochman et al., 2019), which are known to be toxic for seed germination (Balestri et al., 2019; Stefannello et al., 2019). Previous research showed that MP leachates (e.g., from polycarbonate), may negatively affect germination (Bosker et al., 2019; Pflugmacher et al., 2020), but leachates from other MPs (e.g., from polystyrene, polyethylene) have no effects on germination of wheat or soybean seeds (Lian et al., 2020; Li et al., 2021). Added to this “chemical effect”, films and fibers may have a “physical effect” on seeds. That is, MPs adhering to the surface of the seed pores (Bosker et al., 2019) can slow down water uptake and thus delay seed germination. This seems reasonable as Daucus carota seeds are rough and have different channels and appendages, “barbs”, that facilitate, especially for fibers, microplastic adhesion to the coating surface.
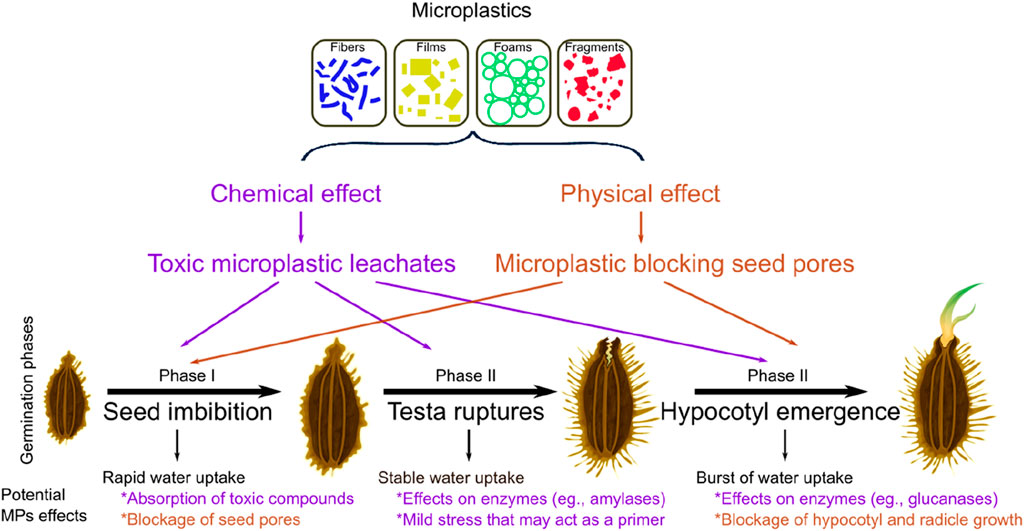
FIGURE 4. Potential effects of microplastics (MPs) on the different phases of seed germination. Microplastics of different shapes may affect seed germination via toxic microplastic leachates (chemical effects) and/or via blockage of seed pores (physical effects). Phase I (seed imbibition) would be affected due to the absorption of toxic chemical compounds, or due to the blockage of seed pores by MPs. Phase II (testa ruptures) can be affected by the negative effect of MPs leachates on enzymes key for seed germination. In this phase, the mild stress caused by MPs may act to some extent as a priming agent that contributes to synchronize seed germination. In phase III (hypocotyl emergence), MPs leachates may negatively affect enzymes needed for hypoctyl and radicle emergence. A physical blockage can also affect hypocotyl and radicle growth.
In the second phase of germination (stable water uptake and rupture of seed testa), both the chemical and physical effects of MPs may delay the testa rupturing as this process depends on water uptake and swelling of the embryo and the endosperm (Finch-Savage and Leubner-Metzger 2006). In this phase, the activation of enzymes and the synthesis of proteins that support germination also occurs (Srivastava et al., 2021) and can be potentially affected by microplastics. This is possible, as different toxic compounds associated with microplastics (Lithner et al., 2011) are known to affect activity of enzymes such as amylase, protease or ribonuclease, which are key for seed germination (Sethy and Ghosh 2013). This entire stress condition due to MPs in soil may potentially increase the abscisic acid (ABA) production, a regulator of dormancy (Finch-Savage and Leubner-Metzger 2006) contributing to a delay in seed germination.
Finally, in the third phase of germination (radicle and hypocotyl emergence), enzymes such as glucanases or mannanases whose production enables the tip of the radicle to penetrate the testa (Srivastava et al., 2021) can be also potentially affected, as discussed above, by MPs in the soil. In addition, toxic compounds may delay embryo growth and radical emergence by decreasing levels of carbohydrate-metabolizing enzymes (Sethy and Ghosh 2013). The physical blockage of MPs on seeds may also delay hypocotyl growth. Delay in seed germination is a potentially serious issue, especially if the seed bank longevity is short and the seeds lose their viability before the stress caused by MPs is removed. In addition, although seeds may maintain their viability, that delay may affect reproductive success as the abundance and diversity of pollinators change depending on the time of germination (Kehrberger and Holzschuh 2019).
Microplastics in soil increase synchrony of seed germination
Our results showed that microplastics irrespective of their shape increased the synchrony of seed germination of Daucus carota. That is, the seeds tended to germinate more at the same time in the microplastic treatments compared to the germination behavior in the control without microplastics. Polymer type also affected seed germination synchrony. Out of all polymer types used, PES and PP fibers, PET and PP films, PU foams and PET fragments, which persist in terrestrial systems (Zhang et al., 2019), were the polymers that most increased this germination parameter.
The presence of MPs in the soil creates a stressful environment mainly driven by the chemical toxicity and the physical blockage in seed pores. As seeds can recognize potential harmful chemicals and defer germination until better establishment conditions occur (Renne et al., 2014), we would expect that with time, the soil substrate acts as a buffer and likely absorbs the leached chemicals from MPs, protecting the seeds and creating again suitable conditions for a synchronous germination. In addition, seeds could have been subjected to a mild stress in the early phases of germination (phases I and II) (sensu Srivastava et al., 2021), where the leachates absorbed by the seeds may have helped to synchronize germination. This type of procedure has been widely used in managed crops. There, seeds imbibe a chemical priming agent such as smoke substances, nitric oxide, among others (Flematti et al., 2004; Finch-Savage and Leubner-Metzger 2006) that synchronizes crop seed germination and enables plants to survive better under adverse environmental conditions (Srivastava et al., 2021).
In natural environments, the fact that MPs increase seed germination synchrony may have negative consequences for the species if unsuitable conditions following germination cause high seedling mortality. By contrast, asynchronous germination may ensure seed presence in the soil seed bank at different times of the year (Venable, 1989) promoting species persistence, despite periods of unfavorable conditions. Moreover, synchronic germination could limit the creation of phenological niches throughout the year (Gioria et al., 2018) affecting plant reproduction (flowering, pollination) with consequences for plant establishment. Finally, the fact that MPs increase seed germination synchrony may affect the timing of plant-plant interactions (facilitation, competition) as well as the timing of associations with soil biota, with consequences for plant community assembly.
Microplastics in soil did not affect the final percentage of germination
Our results showed that MPs affect both velocity and synchrony of seed germination.
However, we did not find differences in the final percentage of germination between treatments with MPs and the control without MPs, probably because to some extent the soil may have absorbed MPs leachates buffering that negative effect making it a transient phenomenon. However, others have observed that even low MPs concentrations in soil (0.001% or 0.2% w/w) may reduce final percentage of seed germination by ∼6% or up to 55% (Boots et al., 2019; Pignatelli et al., 2020), as plastics can contain hazardous monomers and substances of varying toxicity (Lithner et al., 2011). Indeed, in one case final seed germination could not recover with time after being exposed to MPs (Pflugmacher et al., 2020), either because of MP toxicity or because of the continuous blockage of seed pores.
Future research on the effects of MPs on seed germination should be performed under field conditions where the plastic has been leaching substances into the soil over time and whose physical and chemical properties have likely already changed due the continued presence of MP. The effects that microplastics may have on germination of plant species with different life cycles (annual, biennial, perennes) or different strategies (native, invasive) and the consequences that this may have on community assembly needs to be further assessed. Similarly, the role of the soil substrate as a buffer for the negative effects of MPs must be addressed, as this function can be positive for seed germination but potentially negative for plant growth due to MPs effects on soil biota. Our research lays the basis for future reseach into the mechanisms by which microplastic in soil may affect seed germination.
Data availability statement
The datasets presented in this study can be found in online repositories. The names of the repository/repositories and accession number(s) can be found below: https://doi.org/10.6084/m9.figshare.20231277.v3.
Author contributions
YML conceived the ideas and designed methodology with input from MCR; YML, and PUC established and maintained the experiment in the greenhouse; PUC collected the data; YML analyzed the data and wrote the first draft. All authors contributed to the draft and gave final approval for publication. We have no conflict of interest to declare.
Funding
The work was funded by the German Federal Ministry of Education and Research (BMBF) within the collaborative Project “Bridging in Biodiversity Science (BIBS)” (funding number 01LC1501A). MCR additionally acknowledges funding from the EU projects PAPILLONS and MINAGRIS. YML additionally acknowledges funding from the Deutsche Forschungsgemeinschaft (DFG), project number 492483645.
Acknowledgments
We thank Sthefania Quintero-Pineda for her design on Daucus carota seeds used in Figure 4. We acknowledge support by the Open Access Publication Initiative of Freie Universität Berlin.
Conflict of interest
The authors declare that the research was conducted in the absence of any commercial or financial relationships that could be construed as a potential conflict of interest.
Publisher’s note
All claims expressed in this article are solely those of the authors and do not necessarily represent those of their affiliated organizations, or those of the publisher, the editors and the reviewers. Any product that may be evaluated in this article, or claim that may be made by its manufacturer, is not guaranteed or endorsed by the publisher.
Supplementary material
The Supplementary Material for this article can be found online at: https://www.frontiersin.org/articles/10.3389/fenvs.2022.1017349/full#supplementary-material
References
Akdogan, Z., and Guven, B. (2019). Microplastics in the environment: A critical review of current understanding and identification of future research needs. Environ. Pollut. 254, 113011. doi:10.1016/j.envpol.2019.113011
Aravind, J., Vimala, D., Radhamani, J., Jacob, S., and Kalyani, S. (2021). Germinationmetrics: Seed germination indices and curve fitting. Available at: https://github.com/aravind-j/germinationmetricshttps://cran.r-project.org/package=germinationmetrics [Accessed November 9, 2022].
Balestri, E., Menicagli, V., Ligorini, V., Fulignati, S., Raspolli Galletti, A. M., and Lardicci, C. (2019). Phytotoxicity assessment of conventional and biodegradable plastic bags using seed germination test. Ecol. Indic. 102, 569–580. doi:10.1016/j.ecolind.2019.03.005
Baskin, C., and Baskin, J. (2014). Seeds: Ecology, biogeography, and evolution of dormancy and germination. San Diego: Academic Press.
Bewley, J. D. (1997). Seed germination and dormancy. Plant Cell 9, 1055–1066. doi:10.1105/tpc.9.7.1055
Bläsing, M., and Amelung, W. (2018). Plastics in soil: Analytical methods and possible sources. Sci. Total Environ. 612, 422–435. doi:10.1016/j.scitotenv.2017.08.086
Boots, B., Russell, C. W., and Green, D. S. (2019). Effects of microplastics in soil ecosystems: Above and below ground. Environ. Sci. Technol. 53, 11496–11506. doi:10.1021/acs.est.9b03304
Bosker, T., Bouwman, L. J., Brun, N. R., Behrens, P., and Vijver, M. G. (2019). Microplastics accumulate on pores in seed capsule and delay germination and root growth of the terrestrial vascular plant Lepidium sativum. Chemosphere 226, 774–781. doi:10.1016/j.chemosphere.2019.03.163
Bretz, F., Hothorn, T., and Westfall, P. H. (2011). Multiple comparisons using R. Boca Raton, FL: CRC Press.
Curren, E., and Leong, S. C. Y. (2019). Profiles of bacterial assemblages from microplastics of tropical coastal environments. Sci. Total Environ. 655, 313–320. doi:10.1016/j.scitotenv.2018.11.250
de Souza Machado, A. A., Lau, C. W., Kloas, W., Bergmann, J., Bachelier, J. B., Faltin, E., et al. (2019). Microplastics can change soil properties and affect plant performance. Environ. Sci. Technol. 53, 6044–6052. doi:10.1021/acs.est.9b01339
Espí, E., Salmerón, A., Fontecha, A., García, Y., and Real, A. I. (2006). Plastic films for agricultural applications. J. Plastic Film Sheeting 22, 85–102. doi:10.1177/8756087906064220
Finch-Savage, W. E., and Leubner-Metzger, G. (2006). Seed dormancy and the control of germination. New Phytol. 171, 501–523. doi:10.1111/j.1469-8137.2006.01787.x
Flematti, G. R., Ghisalberti, E. L., Dixon, K. W., and Trengove, R. D. (2004). A compound from smoke that promotes seed germination. Science 305, 977. doi:10.1126/science.1099944
Gioria, M., Pyšek, P., and Osborne, B. A. (2018). Timing is everything: Does early and late germination favor invasions by herbaceous alien plants? J. Plant Ecol. 2018, rtw105. doi:10.1093/jpe/rtw105
Gkoutselis, G., Rohrbach, S., Harjes, J., Obst, M., Brachmann, A., Horn, M. A., et al. (2021). Microplastics accumulate fungal pathogens in terrestrial ecosystems. Sci. Rep. 11, 13214. doi:10.1038/s41598-021-92405-7
Helmberger, M. S., Tiemann, L. K., and Grieshop, M. J. (2020). Towards an ecology of soil microplastics. Funct. Ecol. 34, 550–560. doi:10.1111/1365-2435.13495
Hothorn, T., Bretz, F., and Westfall, P. (2008). Simultaneous inference in general parametric models. Biom. J. 50, 346–363. doi:10.1002/bimj.200810425
Kehrberger, S., and Holzschuh, A. (2019). How does timing of flowering affect competition for pollinators, flower visitation and seed set in an early spring grassland plant? Sci. Rep. 9, 15593. doi:10.1038/s41598-019-51916-0
Kim, S. W., Waldman, W. R., Kim, T.-Y., and Rillig, M. C. (2020). Effects of different microplastics on nematodes in the soil environment: Tracking the extractable Additives using an ecotoxicological approach. Environ. Sci. Technol. 54, 13868–13878. doi:10.1021/acs.est.0c04641
Li, B., Huang, S., Wang, H., Liu, M., Xue, S., Tang, D., et al. (2021). Effects of plastic particles on germination and growth of soybean (Glycine max): A pot experiment under field condition. Environ. Pollut. 272, 116418. doi:10.1016/j.envpol.2020.116418
Lian, J., Wu, J., Xiong, H., Zeb, A., Yang, T., Su, X., et al. (2020). Impact of polystyrene nanoplastics (PSNPs) on seed germination and seedling growth of wheat (Triticum aestivum L.). J. Hazard. Mat. 385, 121620. doi:10.1016/j.jhazmat.2019.121620
Lithner, D., Larsson, Å., and Dave, G. (2011). Environmental and health hazard ranking and assessment of plastic polymers based on chemical composition. Sci. Total Environ. 409, 3309–3324. doi:10.1016/j.scitotenv.2011.04.038
Lozano, Y. M., Aguilar-Trigueros, C. A., Onandia, G., Maaß, S., Zhao, T., and Rillig, M. C. (2021a). Effects of microplastics and drought on soil ecosystem functions and multifunctionality. J. Appl. Ecol. 58, 988–996. doi:10.1111/1365-2664.13839
Lozano, Y. M., Lehnert, T., Linck, L. T., Lehmann, A., and Rillig, M. C. (2021b). Microplastic shape, polymer type, and concentration affect soil properties and plant biomass. Front. Plant Sci. 12, 616645. doi:10.3389/fpls.2021.616645
Lozano, Y. M., and Rillig, M. C. (2020). Effects of microplastic fibers and drought on plant communities. Environ. Sci. Technol. 54, 6166–6173. doi:10.1021/acs.est.0c01051
Lozano, Y. M., and Rillig, M. C. (2022). Legacy effect of microplastics on plant-soil feedbacks. Front. Plant Sci. 13, 965576. doi:10.3389/fpls.2022.965576
Pflugmacher, S., Sulek, A., Mader, H., Heo, J., Noh, J. H., Penttinen, O.-P., et al. (2020). The influence of new and artificial aged microplastic and leachates on the germination of Lepidium sativum L. Plants 9, 339. doi:10.3390/plants9030339
Pignattelli, S., Broccoli, A., and Renzi, M. (2020). Physiological responses of garden cress (L. sativum) to different types of microplastics. Sci. Total Environ. 727, 138609. doi:10.1016/j.scitotenv.2020.138609
Qi, Y., Yang, X., Pelaez, A. M., Huerta Lwanga, E., Beriot, N., Gertsen, H., et al. (2018). Macro- and micro- plastics in soil-plant system: Effects of plastic mulch film residues on wheat (Triticum aestivum) growth. Sci. Total Environ. 645, 1048–1056. doi:10.1016/j.scitotenv.2018.07.229
R Core Team (2020). R: A language and environment for statistical computing. Vienna, Austria: R Foundation for Statistical Computing. Available at: https://www.R-project.org/.
Ranal, M., and Santana, D. (2006). How and why to measure the germination process? Rev. Bras. Bot. 29, 1–11. doi:10.1590/s0100-84042006000100002
Renne, I. J., Sinn, B. T., Shook, G. W., Sedlacko, D. M., Dull, J. R., Villarreal, D., et al. (2014). Eavesdropping in plants: Delayed germination via biochemical recognition. J. Ecol. 102, 86–94. doi:10.1111/1365-2745.12189
Rillig, M. C., and Lehmann, A. (2020). Microplastic in terrestrial ecosystems. Science 368, 1430–1431. doi:10.1126/science.abb5979
Rillig, M. C., Lehmann, A., Ryo, M., and Bergmann, J. (2019). Shaping up: Toward considering the shape and form of pollutants. Environ. Sci. Technol. 53, 7925–7926. doi:10.1021/acs.est.9b03520
Rillig, M. C. (2012). Microplastic in terrestrial ecosystems and the soil? Environ. Sci. Technol. 46, 6453–6454. doi:10.1021/es302011r
Rochman, C. M., Brookson, C., Bikker, J., Djuric, N., Earn, A., Bucci, K., et al. (2019). Rethinking microplastics as a diverse contaminant suite. Environ. Toxicol. Chem. 38, 703–711. doi:10.1002/etc.4371
Sethy, S., and Gosh, S. (2013). Effect of heavy metals on germination of seeds. J. Nat. Sci. Biol. Med. 4, 272. doi:10.4103/0976-9668.116964
Srivastava, A. K., Suresh Kumar, J., and Suprasanna, P. (2021). Seed ‘primeomics’: Plants memorize their germination under stress. Biol. Rev. 96, 1723–1743. doi:10.1111/brv.12722
Stefanello, R., Viana, B. B., and Nevesdas, L. A. S. (2019). Cadmium toxicity on seed germination and initial growth of chia. Acta Sci. Biol. Sci. 41, 42174. doi:10.4025/actascibiolsci.v41i1.42174
van Kleunen, M., Brumer, A., Gutbrod, L., and Zhang, Z. (2020). A microplastic used as infill material in artificial sport turfs reduces plant growth. PLANTS PEOPLE PLANET 2, 157–166. doi:10.1002/ppp3.10071
Venable, L. (1989). “Modeling the evolutionary ecology of seed banks,” in Ecology of seed banks (Massachusetts: Academic Press).
Waldman, W. R., and Rillig, M. C. (2020). Microplastic research should embrace the complexity of secondary particles. Environ. Sci. Technol. 54, 7751–7753. doi:10.1021/acs.est.0c02194
Wang, L., Liu, Y., Kaur, M., Yao, Z., Chen, T., and Xu, M. (2021). Phytotoxic effects of polyethylene microplastics on the growth of food crops soybean (Glycine max) and mung bean (vigna radiata). Int. J. Environ. Res. Public Health 18, 10629. doi:10.3390/ijerph182010629
Xu, B., Liu, F., Cryder, Z., Huang, D., Lu, Z., He, Y., et al. (2020). Microplastics in the soil environment: Occurrence, risks, interactions and fate – a review. Crit. Rev. Environ. Sci. Technol. 50, 2175–2222. doi:10.1080/10643389.2019.1694822
Yuan, W., Zhou, Y., Liu, X., and Wang, J. (2019). New perspective on the nanoplastics disrupting the reproduction of an endangered fern in artificial freshwater. Environ. Sci. Technol. 53, 12715–12724. doi:10.1021/acs.est.9b02882
Zhang, S., Wang, J., Liu, X., Qu, F., Wang, X., Wang, X., et al. (2019). Microplastics in the environment: A review of analytical methods, distribution, and biological effects. TrAC Trends Anal. Chem. 111, 62–72. doi:10.1016/j.trac.2018.12.002
Keywords: germination phases, microplastic leachates, microplastic shape, seed pores, testa ruptures, timing, microplastic toxicity, microplastic polymer type
Citation: Lozano YM, Caesaria PU and Rillig MC (2022) Microplastics of different shapes increase seed germination synchrony while only films and fibers affect seed germination velocity. Front. Environ. Sci. 10:1017349. doi: 10.3389/fenvs.2022.1017349
Received: 11 August 2022; Accepted: 18 November 2022;
Published: 30 November 2022.
Edited by:
Gilles Colinet, University of Liège, BelgiumReviewed by:
Olli-Pekka Penttinen, University of Helsinki, FinlandYinglong Su, East China Normal University, China
Copyright © 2022 Lozano, Caesaria and Rillig. This is an open-access article distributed under the terms of the Creative Commons Attribution License (CC BY). The use, distribution or reproduction in other forums is permitted, provided the original author(s) and the copyright owner(s) are credited and that the original publication in this journal is cited, in accordance with accepted academic practice. No use, distribution or reproduction is permitted which does not comply with these terms.
*Correspondence: Yudi M. Lozano, yudyja@gmail.com
†ORCID: Yudi M. Lozano, orcid.org/0000-0002-0967-8219; Matthias C. Rillig, orcid.org/0000-0003-3541-7853