- 1Institute of Environment, Resources, Soil and Fertilizer, Zhejiang Academy of Agricultural Sciences, Hangzhou, China
- 2Fort Lauderdale Research and Education Center, Department of Microbiology and Cell Science, University of Florida, Fort Lauderdale, FL, United States
- 3Zhejiang Agricultural Technology Extension Center, Hangzhou, China
- 4Zhuji Agricultural Technology Extension Center, Zhuji, China
Ammonia (NH3) volatilization losses result in low nitrogen use efficiency (NUE) and various environmental impacts in agroecosystems. Machine-transplanted rice with side-deep fertilization (MRSF) has been recommended as an effective alternative to traditional transplantation with manual broadcasting of fertilizer. Controlled-release nitrogen fertilizer (CRF) can enhance rice yield and NUE in paddy fields. However, there is scarce information about combined effects of MRSF and CRF on NH3 volatilization loss and rice grain yield, NUE, net economic benefit (NEB) in a double rice cropping system. In this study, a field experiment was conducted to evaluate the impact of MRSF with CRF on grain yields, NUE and economic returns of early rice and late rice from 2019 to 2021, as well as NH3 emissions in two rice seasons (2019 and 2021). Six treatments were designed as no N fertilizer (N0), compound fertilizer broadcasting (CFB), compound fertilizer side-deep placement (CFD), CRF broadcasting (CRFB), CRF side-deep placement (CRFD1), and single side-deep placement of CRF (CRFD2). The results showed that the CFD and CRFB treatments decreased NH3 volatilization while enhancing or maintaining rice yield and NUE compared to the CFB treatment. MRSF with CRF (CRFD1 and CRFD2) significantly reduced NH3 emissions of early and late rice by 57.6–67.9% and 62.2–80.9% by decreasing the NH4+–N concentrations in the surface water compared to the CFB treatment, respectively. Rice grain yields in the MRSF with CRF treatments increased by 3.9–17.3% in early rice and 5.4–21.6% in late rice relative to the CFB treatment. In addition, MRSF with CRF treatments improved NUE for early and late rice from 32.1 to 36.2% and 21.3–28.4% in the CFB treatment to 48.4–61.2% and 39.7–62.3%, respectively. The yield-scale NH3 volatilization losses were reduced under the MRSF with CRF treatments by 61.2–71.5% in early rice and 67.4–84.3% in late rice. Furthermore, MRSF with single basal application of CRF reduced time-consuming and labor-intensive while increasing rice yields and net economic benefits. Overall, co-application of MRSF and CRF can reduce NH3 emissions, and improve rice yield, NUE and profitability in double rice cropping systems.
1 Introduction
Rice (Oryza sativa L.) is one of the most important staple foods globally and provides food for nearly 50% of the world’s population (Seck et al., 2012). China, the world’s largest rice producer, produces approximately 212 million tons of rice, accounting for more than 34% of the total cereal production, and has recorded an average rice grain yield of 7044 kg ha−1 in 2020 (National Bureau of Statistics of China, 2021). N Fertilizer is a crucial role in enhancing crop growth and productivity in agroecosystems, and contributes to an almost 50% increase in food production (Peng et al., 2006; Yang et al., 2021). However, crop yield does not increase proportionally with increasing N fertilizer application (Zhang et al., 2012). Consequently, excessive N application has resulted in many environmental issues, such as greenhouse gas emissions, soil acidification, groundwater nitrate pollution and surface water eutrophication, ultimately negative affecting human health (Zhu and Chen, 2002; Ju et al., 2009; Zhang et al., 2013). Therefore, there is an urgent need to determine optimal N management strategies for cleaner rice production.
Ammonia volatilization is generally considered as the main pathway of N loss in paddy fields due to high temperature and intensive rainfall during the rice-growing season, accounting for 9–40% (average 17%) of the total N fertilizer applied (Cui et al., 2014; Liu et al., 2015; Yao et al., 2018b). Excess NH3 emissions contribute to various environmental impacts, such as atmospheric haze pollution, aquatic eutrophication, rain acidification and climate change (Liu et al., 2015; Jiang et al., 2017; Wang and Lu, 2020). Furthermore, the NH3 emitted from agricultural soil causes 30% of N deposition in the environment (Wolfe and Patz, 2002; Wang et al., 2021). Therefore, reducing NH3 volatilization losses from soils is urgently needed in the context of increasing food demand due to population growth in China. The Chinese government has developed policies targeting the reduction of NH3 emission from agroecosystem in 2018 (Adalibieke et al., 2021). Recently, many improved N management strategies have been proposed, such as increasing number of N fertilizer application, adjusting the rate and time of N fertilizer application, and application of enhanced efficiency fertilizers (Linquist et al., 2013; Yao et al., 2018b; Rose et al., 2018; Lyu et al., 2021). However, the development of these practices is restricted by a lack of related knowledge, higher cost, or extra labor input (Zhang et al., 2017; Yin et al., 2021). Hence, developing cleaner, simplified and efficient fertilization methods in rice production is paramount to improve rice yield and economic return, and minimize N risk to the environment.
Broadcasting and split application of normal urea are often used in traditional rice production, which usually leads to relatively low NUE and high N loss, further reducing farmers’ income and causing environmental issues (Liu et al., 2020). Additionally, it is difficult for farmers to master the correct N application rate and timing, requiring more time and labor (Ke et al., 2018). In recent decades, controlled-release N fertilizers (CRF) have been shown to reduce the number of N fertilizer application and support crop productivity (Chien et al., 2009; Grant et al., 2012; Chen et al., 2020; Zhang et al., 2022). Many studies have shown that broadcasting of CRF can enhance rice grain yield and NUE, and reduce NH3 volatilization and greenhouse gas emission compared to urea application (Yang et al., 2012; Geng et al., 2015; Guo et al., 2019). Moreover, deep placement of N fertilizer, including point-deep and side-deep placement, is also an efficient fertilization method for improving crop yield and NUE while minimizing environmental pollution in rice-based system (Bautista et al., 2001; Gaihre et al., 2015; Yao et al., 2018a; Zhu et al., 2019). However, manual deep placement of fertilizer is more time-consuming and labor-costing than mechanical deep placement (Liu et al., 2020; Min et al., 2021). In recent decades, the agricultural workforce has been decreasing because of the rapid urbanization in China (Wang et al., 2016). Furthermore, there is a short turn-around period between early rice harvesting and late rice planting in the double rice cropping system.
Mechanized transplantation has gradually become the main rice planting mode in China (Miao et al., 2016). However, traditional mechanized transplantation and fertilization are not synchronous, and which are time-consuming and labor-intensive for rice production (Min et al., 2021). Machine-transplanted rice with side-deep fertilization (MRSF) is an emerging technology wherein rice seedlings are transplanted and fertilizers are applied 3–5 cm in-depth and 5–7 cm away from the rice seedling root simultaneously (Zhu et al., 2019). This practice is an efficient way to achieve synergetic improvement of grain yield and NUE, saving time and labor (Zhao et al., 2021; Zhong et al., 2021a, Zhong et al., 2021b). Previous studies have indicated that MRSF can increase rice yield in a single-cropping rice system relative to the broadcasting of fertilizers (Zhu et al., 2019, Zhu et al., 2021; Min et al., 2021). Zhong et al. (2021a) reported that MRSF enhances the grain yield of early and late rice by 0.5–20.2% and 5.3–15.0%, compared to the surface application of compound fertilizer in South China. A 3-year field experiment conducted by Min et al. (2021) found that MRSF treatments significantly reduced the NH3 emission by 51.0–62.8% compared to surface broadcast of fertilizer in a single rice cropping system. However, little is known about the effect of MRSF with CRF on NH3 emission, yield-scale NH3 emissions, and economic returns in double rice cropping systems.
The double rice cropping system is one of the most important rice-based production, accounting for 35% of rice yield in China (Liang et al., 2019). The average N application rate for early and late rice is 197 and 191 kg ha−1 in China, respectively (Liang et al., 2019). However, Zhong et al. (2021b) recommended a much lower rate, 150 and 165 kg N ha−1, as optimum for early and late rice under MRSF with compound fertilizer, respectively. Whether MRSF with CRF further reduces the required N application rate with supporting rice yield is unclear. Therefore, the objectives of the current study were: 1) to assess how MRSF with CRF affects NH3 volatilization loss and yield-scale NH3 emissions, as well as NH4+–N concentration in floodwater; 2) to clarify the effects of MRSF with CRF on rice yield, NUE, and net economic benefit; and 3) to evaluate whether MRSF with one-time CRF application can replace conventional fertilization in the double rice cropping systems.
2 Materials and methods
2.1 Experimental site description and materials
A 3-years field experiment was conducted from 2019 to 2021 in Jinan Town, Zhuji City, Zhejiang Province, southeast China (29°46ʹ N, 120°22′ E). The experimental site has a typical subtropical monsoon climate with an average annual temperature and rainfall of 16.3°C and 1374 mm, respectively. The mean temperature and rainfall during the early and late rice growing seasons are shown in Figure 1. The double rice cropping system is dominant in this region. According to Chinese Soil Taxonomy, the soil at the experimental site is classified as anthrosol and waterloggogenic paddy soil (Cooperative Research Group on Chinese Soil Taxonomy, 2001). The basic properties of the soil (0–20 cm) were as follows: pH 5.3, soil organic matter (SOM) 38.8 g kg−1, total nitrogen (TN) 2.0 g kg−1, available phosphorus (AP) 10.4 mg kg−1, and available potassium (AK) 106.0 mg kg−1.
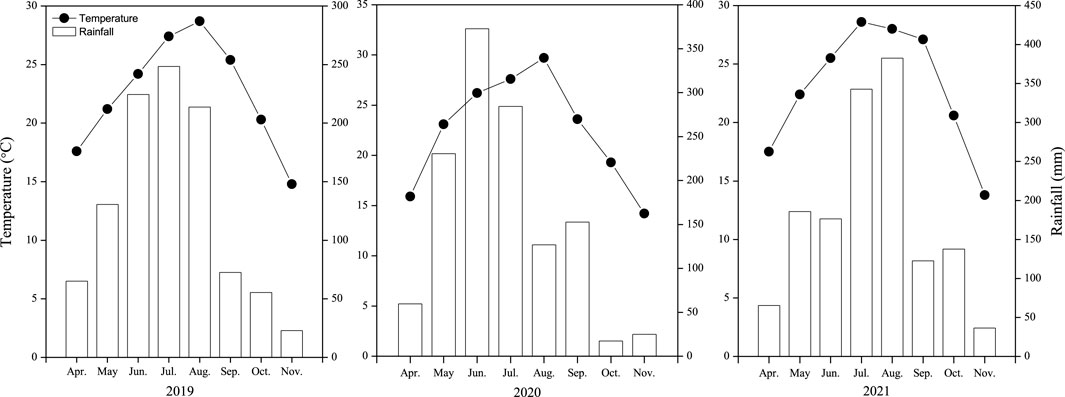
FIGURE 1. The monthly mean rainfall and temperature of early and late rice growing seasons from 2019 to 2021.
The early and late rice varieties used in this study were “Zhongzao 39” and “Yongyou 1540”, respectively. A Yanmar rice transplanter equipped with a side-deep fertilization apparatus (2FC-6, Yanmar Holdings Co., Ltd. Japan) was used for synchronized rice transplanting and side-deep placement of fertilizers. The two types of N fertilizer were compound fertilizer with an N:P2O5:K2O ratio of 21:8:18 (special fertilizer for double season rice production) and CRF with 40% N from common urea and 60% N from polymer-coated urea. Conventional fertilizers, such as urea (46% N), calcium superphosphate (12% P2O5) and potassium chloride (60% K2O) were adopted in this study.
2.2 Experimental design and management
The field experiment was executed from 2019 to 2021 and featured six treatments with three replicates in a complete randomized block design. The area of each plot was 64.8 m2 (3.6 m × 18 m), with a 0.3 m-wide ridge around the plots. The ridges of each plot were covered with plastic film to prevent water and nutrient exchange. The treatments consisted of 1) N0: a control with no N fertilizer; 2) CFB: broadcasting of compound fertilizer, 165 kg N ha−1, split into three fertilizations; 3) CFD: side-deep placement of compound fertilizer, 135 kg N ha−1, split into two fertilizations; 4) CRFB: broadcasting of CRF, 135 kg N ha−1, split into two fertilizations; 5) CRFD1: side-deep placement of CRF, 135 kg N ha−1, split into two fertilizations; 6) CRFD2: side-deep placement of CRF, 135 kg N ha−1, one-time fertilization. The application rates and timing of N fertilizer use for each treatment are shown in Table 1. For the MRSF treatments (CFD, CRFD1 and CRFD2), fertilizers were applied 3–5 cm below the soil and 3–5 cm from the rice seedlings. Under all treatments, P and K fertilizers were applied once as basal fertilizers at rates of 60 kg P2O5 ha−1 and 90 kg K2O ha−1 before the rice was transplanted. The insufficient amount of P and K fertilizers was supplemented with calcium superphosphate (12% P2O5) and potassium chloride (60% K2O).
The seedlings for early and late rice were planted at a density of 555, 556 plants ha−1 and 347, 222 plants ha−1, respectively. Early rice seedling transplantation and basal fertilization were performed on 17 April 2019; 20 April 2020; and 19 April 2021. Late season rice seedling transplantation and basal fertilization occurred on 27 July 2019; 28 July 2020; and 28 July 2021. N fertilizers for early rice at the tillering stage were applied on 24 April 2019; 27 April 2020; and 26 April 2021. The panicle fertilizers were applied on 20 May 2019; 22 May 2020; and 20 May 2021. The urea-N for late rice at the tillering stage was applied on 4 August 2019; 6 August 2020; and 6 August 2021, and the panicle fertilizers were applied on 1 September 2019; 2 September 2020; and 31 August 2021. Plots were irrigated 7 days before rice transplantation and maintained at 3–5 cm until mid-season aeration and the water level was maintained. After aeration, the surface floodwater was continually maintained at 3–5 cm and drained before physiological maturity for the harvest. Pesticides and herbicides were applied according to the practices of local farmers if necessary.
2.3 Sampling and measurements
2.3.1 Ammonia volatilization
The NH3 volatilization flux was determined by the vented-chamber method (Wang et al., 2004) using a polyvinylchloride tube (PVC, 16 cm inner diameter, 30 cm height) in the 2019 and 2021 double-cropping rice seasons (Supplementary Figure S1). Two pieces of sponge (16 cm diameter, 2 cm thickness) soaked with 15 ml of phosphoglycerol were placed in a PVC tube as the absorbent. The NH3 absorbent was mixed with 50 ml phosphoric acid, 40 ml glycerol and 1000 ml deionized water. The samples were collected at 2-days intervals for 1 week after each N fertilization, and then collected at an interval of 7–10 days. The upper sponges were used to absorb NH3 from ambient air. The lower sponges absorbed NH3 from the paddy soil and were extracted with 300 ml of 1.0 mol L−1 KCl solution. The ammonium content in the extracted solutions was analyzed using a continuous flow analyzer (Skalar SAN++, Netherlands).
2.3.2 Surface water
During the measurement of NH3 volatilization, surface floodwater (50 ml) of the paddy field was collected by the multi-point sampling method using a 10 ml syringe. Field surface water was sampled on days 1, 3, 5, and 7 days after each N fertilization. All water samples were immediately delivered to the laboratory and stored at −20°C for further analysis. The NH4+–N concentration of the surface water was determined using a continuous flow analyzer (Skalar SAN++, The Netherland).
2.3.3 Plant sampling and analysis
Mature early and late rice from each plot were harvested and divided into grain and straw. The grain and straw samples were oven-dried at 105°C for 30 min and then at 75°C until a constant weight was reached to determine the rice grain and straw biomass. The dry plant samples were ground into powder using a grinder and passed through a 0.15-mm sieve for N content measurement. The N concentrations of the rice grains and straw were determined using the Kjeldahl method (Lu, 1999).
2.3.4 Net economic benefit
The net economic benefit (NEB) was calculated as the difference between the economic benefit of rice yield (EByield) and the cost of rice production (EBcost). The cost of rice production includes seed, fertilizer, pesticide, labor, and machinery costs.
2.4 Data analysis
The NH3 volatilization rates were calculated using the method described by Xu et al. (2012):
where F is the NH3 volatilization flux (kg N ha−1 d−1), M is the NH3–N collected by the PVC collector (mg), A is the cross-sectional area of the PVC collector (m2), and D is the interval for ammonia volatilization sample collection (d).
The cumulative NH3 volatilization losses were the sum of the NH3 volatilization fluxes on the sampling days.
The yield-scale NH3 emissions were calculated according to Pittelkow et al. (2014):
where CNH3 is the accumulated NH3 volatilization loss (kg N ha−1) and Y is the rice grain yield (kg ha−1).
The NH3–N volatilization factor was calculated using the method described by He et al. (2018):
where CNH3N and CNH3N0 were the cumulative NH3 losses (kg N ha−1) from the treatments with N fertilizer application and the treatment without N application (N0), respectively, and FN is the application rate of N fertilizer (kg ha−1).
The N use efficiency (NUE) was calculated as:
where UN is the N uptake by rice plant (kg ha−1) in the treatments with N fertilizer application, UN0 is the N uptake by plant (kg ha−1)in the N0 treatment, and FN is the application rate of N fertilizer (kg ha−1).
2.5 Statistical analysis
The one-way or two-way analysis of variance (ANOVA) was performed using SPSS 20.0 (SPSS Inc., Chicago, IL, United States). The treatments were compared using the least significant difference (LSD) at p < 0.05. Pearson’s correlation analysis was performed to determine the relationship between NH3 volatilization fluxes and NH4+–N concentrations in the surface water using SPSS 20.0 (SPSS Inc., Chicago, IL, United States).
3 Results
3.1 Ammonia volatilization loss
N fertilization application significantly increased the NH3 volatilization loss in the two rice seasons over 2 years (2019 and 2021) (Figure 2). The NH3 volatilization flux peaked within 3 days after each fertilization and decreased to relatively low levels. After each fertilization, the peak value of NH3 volatilization flux appeared in the CF treatments over the 2 years. One week later after N fertilization, the NH3 volatilization rate in the N application treatments reached that of the N0 treatment.
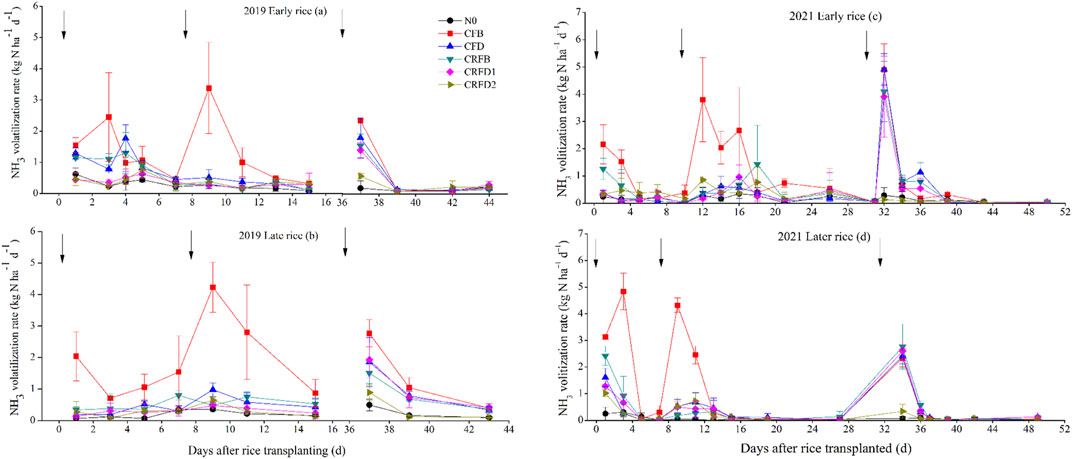
FIGURE 2. The NH3 volatilization fluxes for early (A,C) and late rice (B,D) under different treatments in 2019 (A,B) and 2021 (C,D). Values are presented as the mean ± standard deviation (n = 3). The arrow represents the timing of N fertilizer application, following basal, tillering and panicle fertilizations, respectively. N0: no N fertilizer; CFB: compound fertilizer broadcasting; CFD: compound fertilizer side-deep placement; CRFB: controlled-release N fertilizer broadcasting; CRFD1: controlled-release N fertilizer side-deep placement; CRFD2: controlled-release N fertilizer side-deep placement, one-time fertilization.
The cumulative NH3 volatilization loss in the different treatments showed that the N fertilizer application significantly increased the cumulative loss of NH3 volatilization compared to the N0 treatment (Table 2). The cumulative NH3 volatilization losses were 2.9–7.7 kg N ha−1 under N0 treatments over 2 years. For the early rice season, cumulative NH3 volatilization loads in N fertilization treatments were 4.6–19.6 kg N ha−1 and 7.7–40.0 kg N ha−1, which accounted for 4.8–11.9% and 4.2–17.9% of current application rate of N fertilizer in 2019 and 2021, respectively. Additionally, 3.4–29.6 kg N ha−1 and 2.9–32.1 kg N ha−1 of the total NH3 emissions were observed for late rice under the N fertilization treatments, which accounted for 9.5–24.2% and 4.8–19.5% of the current season N rate in 2019 and 2021, respectively. MRSF treatments (CFD, CRFD1 and CRFD2) decreased the total NH3 volatilization losses for early and late rice by 42.5–67.9% and 58.6–80.9% compared to CFB treatment, respectively. The NH3 volatilization factor decreased significantly under CFB treatment from 4.4 to 10.1% and 8.8–10.1% to 0.2–7.4% and 0.6–5.6% under MRDF treatments for early and late rice over 2 years, respectively.
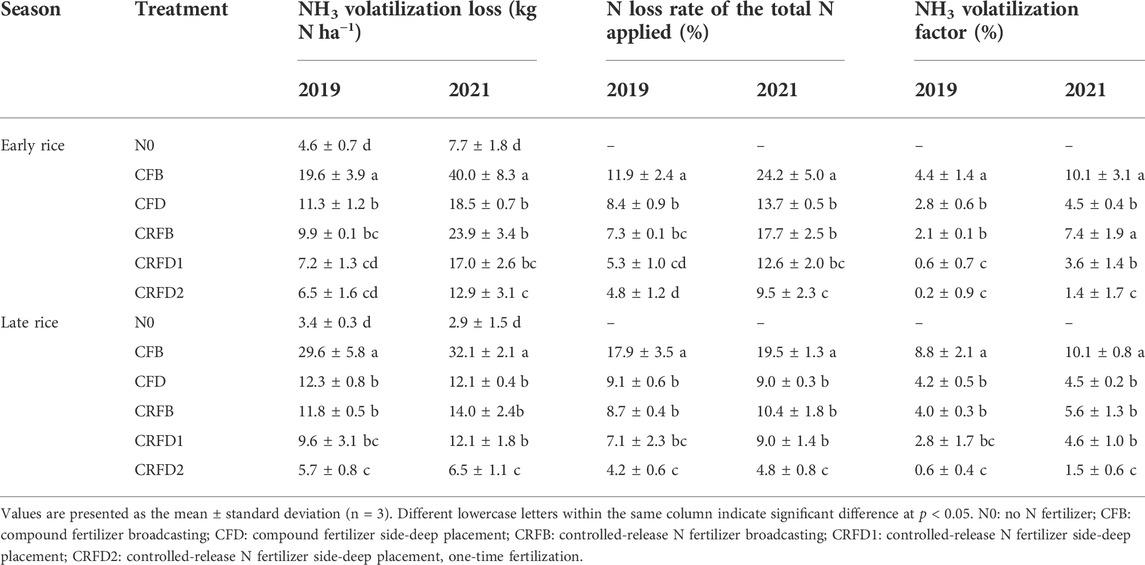
TABLE 2. The cumulative NH3 emissions and NH3 volatilization factor for early rice and late rice under different treatments in 2019 and 2021.
3.2 NH4+–N concentration in surface floodwater
The NH4+–N concentrations in the floodwater for early and late rice across the 2 years are presented in Figure 3. The surface water NH4+–N concentrations in N0 treatment remained low levels with average values ranging from 0.95 to 2.49 mg N L−1 during the rice-growing seasons across 2 years. The concentrations of NH4+–N in surface water increased following N fertilizer application and then decreased near the N0 level, which presented the same pattern as NH3 volatilization fluxes. The average concentrations of NH4+–N in surface floodwater under CFB treatment were 9.6–15.7 mg N L−1 for four rice seasons, which was significantly decreased by 44.1–60.5%, 47.6–74.6% and 53.3–76.4% under CFD, CRFD1 and CRFD2 treatments, respectively. The CRFB treatment significantly reduced the average NH4+–N concentrations for early and late rice seasons by 44.9–56.2% and 48.9–52.9% respectively, compared to CFB treatment. The NH3 fluxes were significantly correlated with the NH4+–N concentrations in floodwater in both seasons in 2019 and 2021 (Figure 4).
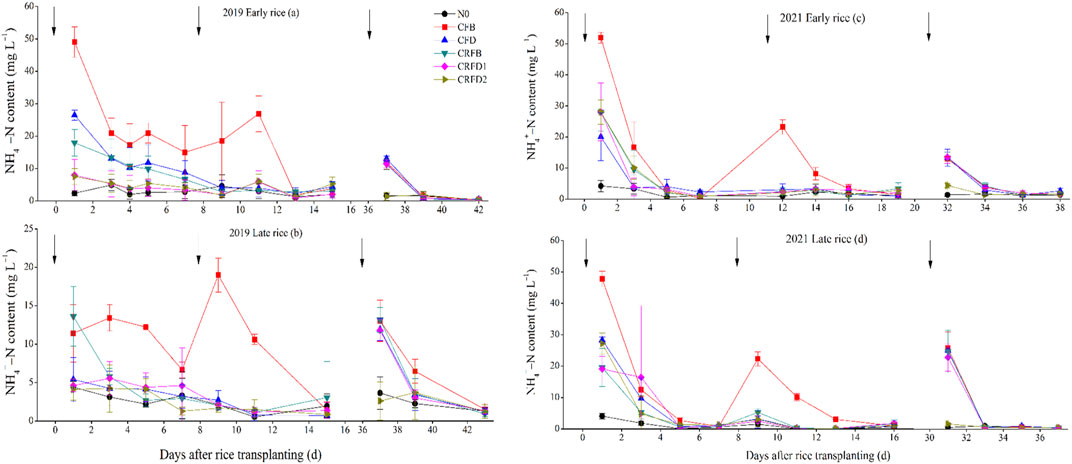
FIGURE 3. The NH4+–N concentrations in surface water for early (A,C) and late rice (B,D) under different treatments in 2019 (A,B) and 2021 (C,D). Values are presented as the mean ± standard deviation (n = 3). The arrow represents the timing of N fertilizer application, following basal, tillering and panicle fertilizations, respectively. N0: no N fertilizer; CFB: compound fertilizer broadcasting; CFD: compound fertilizer side-deep placement; CRFB: controlled-release N fertilizer broadcasting; CRFD1: controlled-release N fertilizer side-deep placement; CRFD2: controlled-release N fertilizer side-deep placement, one-time fertilization.
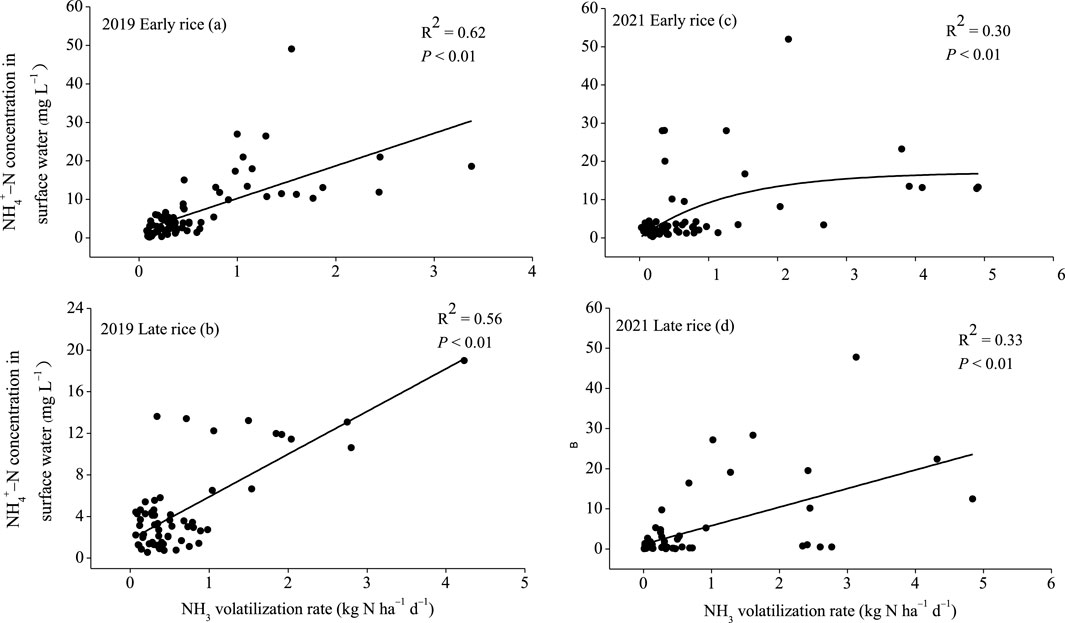
FIGURE 4. The relationship between NH3 volatilization rates and NH4+–N concentrations in surface water for early (A,C) and late rice (B,D) in 2019 (A,B) and 2021 (C,D).
3.3 Grain yield, N use efficiency and yield-scale NH3 volatilization
The grain yields of early and late rice were significantly affected by year (Y), treatment (T) and their interaction (Supplementary Table S1). The application of N fertilizer significantly increased the grain yield of early and late rice compared to the N0 treatment over three rice growing seasons (Figures 5A–C). When N fertilizer rate decreased from 165 kg N ha−1 under CFB treatment to 135 kg N ha−1 under CFD treatment, whereas early and late rice grain yields increased by 5.7–9.1% and 4.2–16.2%, respectively, in 2019. There were no significant differences in rice grain yields between CFB and CRFB treatments, except for early rice in 2021. However, side-deep placement of controlled-release N fertilizer (CRFD1 and CRFD2) significantly increased the grain yield of early rice by 15.7–17.3% in 2021, and late rice yield by 5.4–21.6% over 3 years.
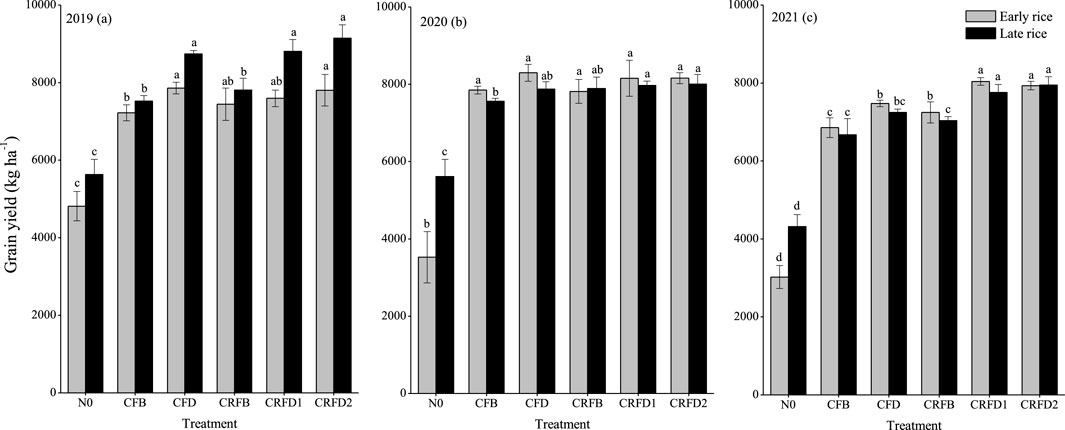
FIGURE 5. The grain yield for early and late rice under different treatments in 2019 (A), 2020 (B) and 2021 (C). Values are presented as the mean ± standard deviation (n = 3). Different lowercase letters above the column indicate significant difference at p < 0.05. N0: no N fertilizer; CFB: compound fertilizer broadcasting; CFD: compound fertilizer side-deep placement; CRFB: controlled-release N fertilizer broadcasting; CRFD1: controlled-release N fertilizer side-deep placement; CRFD2: controlled-release N fertilizer side-deep placement, one-time fertilization.
The N use efficiencies of early and late rice under CFB treatment were 32.1–36.2% and 21.3–28.4% across 3 years, respectively (Figure 6). MRDF treatments (CFD, CRFD1, and CRFD2) increased the NUE by 32.1–82.1% for early rice, and 19.7–192.6% for late rice compared to the CFB treatment over 3 years. The NUE was similar to that of the CRFD1 and CRFD2 treatments based on field observations over 3 years. The CRFB treatment had a significantly higher NUE index than CFB treatment, except for late rice in 2021.
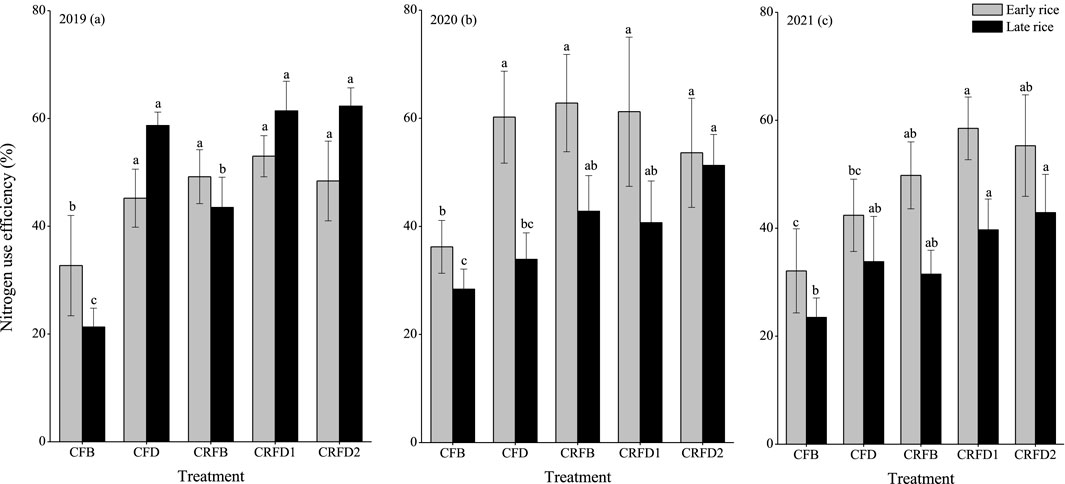
FIGURE 6. The N use efficiency for early and late rice under different treatments in 2019 (A), 2020 (B) and 2021 (C). Values are presented as the mean ± standard deviation (n = 3). Different lowercase letters above the column indicate significant difference at p < 0.05. N0: no N fertilizer; CFB: compound fertilizer broadcasting; CFD: compound fertilizer side-deep placement; CRFB: controlled-release N fertilizer broadcasting; CRFD1: controlled-release N fertilizer side-deep placement; CRFD2: controlled-release N fertilizer side-deep placement, one-time fertilization.
The yield-scale NH3 volatilization in both of early and late rice were significantly affected by year (Y), treatment (T) and their interaction (Supplementary Table S1). The CFB treatment had the highest yield-scale NH3 volatilization during the early and late rice seasons over the 2 years (Figure 7). In contrast, the lowest yield-scale NH3 volatilization was found in the CRFD2 treatment, at approximately 0.86–1.66 g kg−1 and 0.62–0.82 g kg−1 for early and late rice over the years, respectively. Compared to the CFB treatment, the reduction of yield-scale NH3 volatilization for early and late rice in the MRSF treatments ranged from 44.3 to 66.8% and 64.4–84.4% in 2019, and from 57.7 to 71.5% and 65.4–83.1% in 2021, respectively.
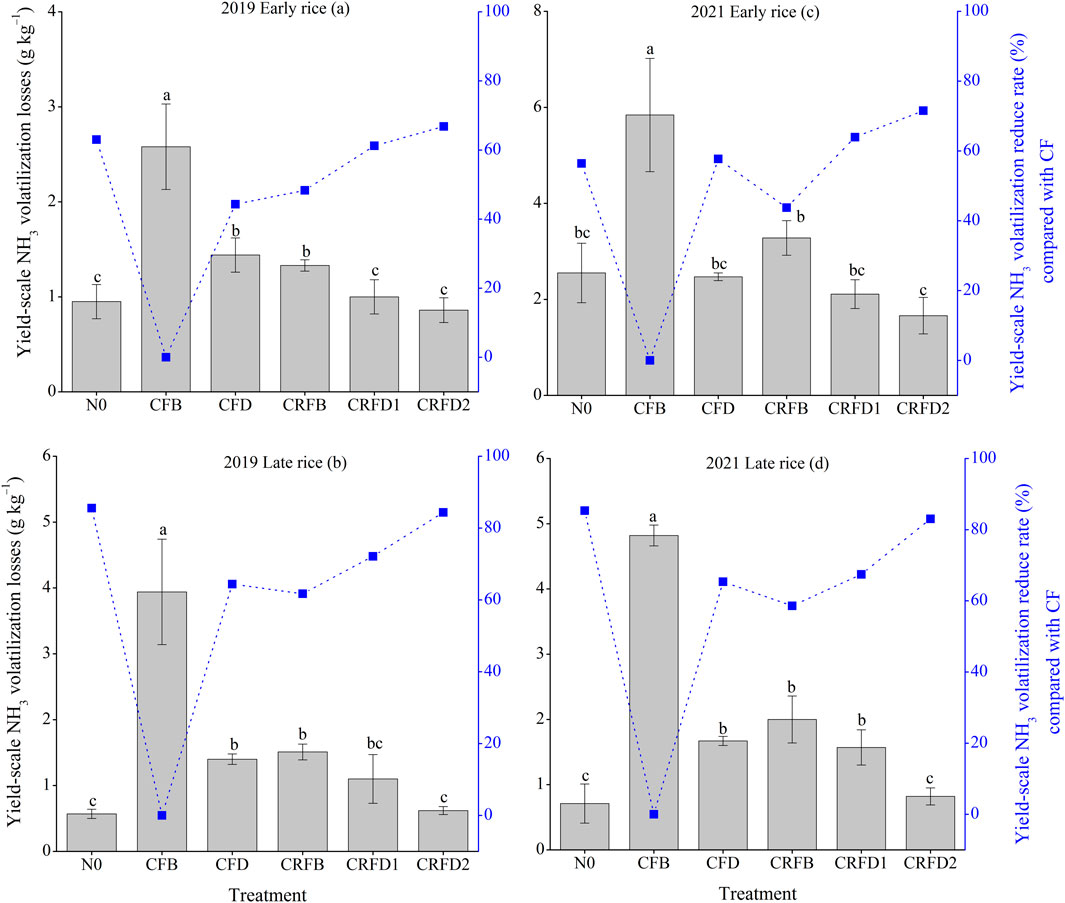
FIGURE 7. The yield-scale NH3 volatilization losses for early (A,C) and late rice (B,D) under different treatments in 2019 (A,B) and 2021 (C,D). Values are presented as the mean ± standard deviation (n = 3). Different lowercase letters above the column indicate significant difference at p < 0.05. N0: no N fertilizer; CFB: compound fertilizer broadcasting; CFD: compound fertilizer side-deep placement; CRFB: controlled-release N fertilizer broadcasting; CRFD1: controlled-release N fertilizer side-deep placement; CRFD2: controlled-release N fertilizer side-deep placement, one-time fertilization.
3.4 Economic return
The cost of rice production ranged from 4.2 × 103–6.7 × 103 yuan ha−1 and 6.7 × 103–9.3 × 103 yuan ha−1 for early and late rice, respectively (Table 3). Compared to the N0 treatment, the application of N fertilizer significantly increased the economic and net economic benefits for early and late rice over 3 years. The economic benefit of rice production (EByield) of the MRSF treatment for early rice and late rice significantly increased over 3 years, respectively, compared to the CFB treatment. However, no significant differences were observed in EByield between the MRSF and CFB treatments in 2020. The CRFB treatment had similar EByield as the CFB treatment in both seasons over 3 years, except for early rice in 2021. The MRSF treatments significantly increased the net economic benefit (NEB) for early and late rice by 15.3–27.5% and 41.7–59.4% in 2019, 11.9–18.5% and 17.2–27.3% in 2020, and 24.6–42.9% and 31.6–65.0% in 2021 compared to the CFB treatment. The profitability of the CRFB treatment for early rice and late rice increased by 5.0–17.1% and 14.9–22.3% across 3 years relative to the CF treatment.
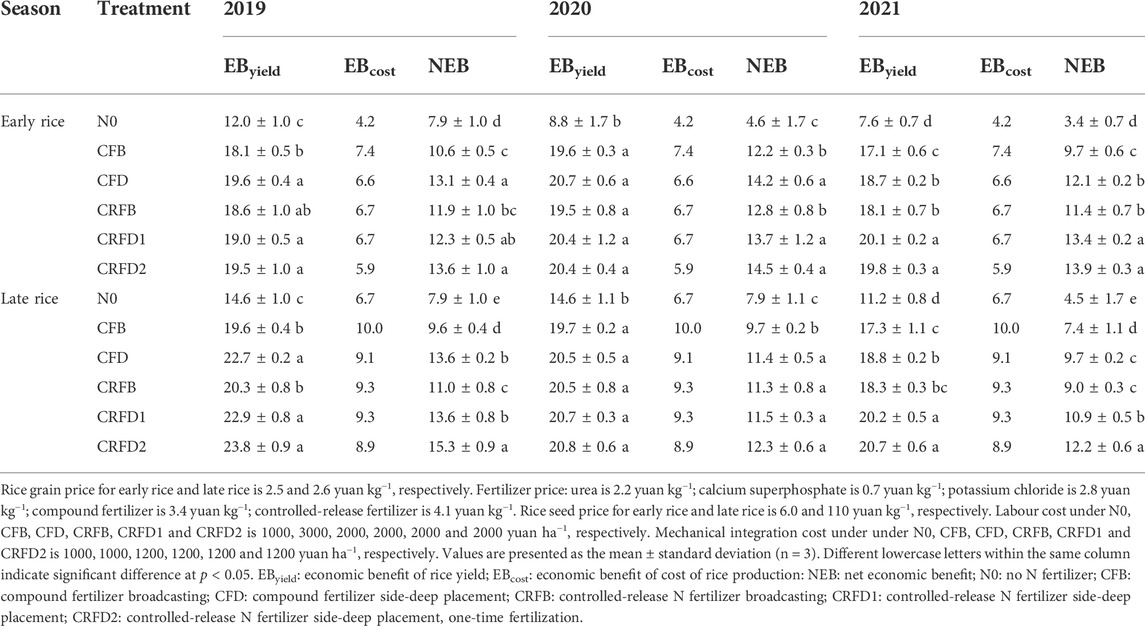
TABLE 3. The economic benefit and net economic benefit for early rice and late rice under different treatments in 2019, 2020 and 2021 (×103 yuan ha−1).
4 Discussion
The NH3 volatilization is regarded as the primary way of N loss in paddy fields, accounting for 9–40% of the total N fertilizer applied (Cui et al., 2014; Liu et al., 2015; Yao et al., 2018b). The broadcasting of N fertilizers is susceptible to N loss via NH3 volatilization in rice paddy fields (Zhang et al., 2017). In the present study, the cumulative NH3 loss from the CFB treatment ranged from 19.6 to 29.6 kg N ha−1 and from 32.1 to 40.0 kg N ha−1 for early and late rice, accounting for 11.9–17.9% and 19.5–24.2% of the total N applied seasonally. This is consistent with the result of Shang et al. (2014), who observed that the cumulative NH3 loss was from 12.8 to 27.3 kg N ha−1 for early rice and from 17.3 to 32.7 kg N ha−1 for late rice in a long-term fertilization experiment, which accounted for 9.2–33.6% and 17.8–32.2%. In contrast, our result is lower than the result from Zhong et al. (2021b), who found that 80.6–89.3 kg N ha−1 and 94.9–96.9 kg N ha−1 were emitted by early and late rice in a double rice cropping system. The NH3 volatilization from paddy fields is influenced by environmental factors, such as rainfall, temperature, soil properties, NH4+–N concentration and pH of surface water (Xu et al., 2012; Wang et al., 2018). Most of the NH3 emissions in the CFB treatment occurred during the basal and tillering fertilization stage (Supplementary Table S2). This is because the N application rate was relatively higher and the N uptake by rice seedlings was much lower at these stages than at the panicle fertilization stage.
Controlled-release N fertilizer application significantly reduces NH3 emissions by 23–62% in early rice and 14–59% in late rice, compared to the broadcasting of urea (Li et al., 2017, Li et al., 2018; Tian et al., 2021). A meta-analysis conducted by Jiang et al. (2022) reported that controlled-release urea increased rice yield while reducing environmental risk. Our results showed that the CRFB treatment had a significantly lower NH3 volatilization loss than the CFB treatment, mainly because of lower NH3 volatilization fluxes under CRFB treatment. Similarly, Tian et al. (2021) found that broadcasting of CRF significantly reduced NH3 volatilization loss from early and late rice by 20–43% and 20–32%, respectively, compared to the broadcasting of urea. The reason is that the N release rate from CRF is slower than that from conventional urea.
In the present study, the MRSF treatments decreased the cumulative NH3 ammonia losses from paddy fields by 42.5–67.9% in early rice and 58.5–80.9% in late rice over a 2-year (2019 and 2021) field observation compared to the CFB treatment (Table 2). Similar to our results, Zhong et al. (2021b) found that NH3 emissions from the double rice cropping system could be dramatically reduced by MRSF treatments relative to conventional N fertilizer. The lower NH4+–N concentration in the surface water explains the lower NH3 volatilization losses under the MRSF treatments (Figure 3). It is well known that the NH4+–N concentration in the surface water is one of the main factors affecting NH3 volatilization in paddy fields (Sun et al., 2019). In our study, the NH4+–N concentration in the surface water significantly correlated with the NH3 volatilization flux (Figure 4). Furthermore, the MRSF treatments decreased NH3 emissions, primarily during basal and tillering fertilization (Supplementary Table S2). Compared to the CFD treatment, the combined side-deep placement and CRF further reduced the cumulative NH3 volatilization loss in 2019 (Table 2). This is because the N released from compound fertilizers is faster than that from CRF, which may result in higher NH4+–N in surface water (Hou et al., 2021). The CRFD2 treatment had a higher NH3 volatilization loss than the N0 treatment, indicating that a small quantity of NH4+–N may diffuse upward to the soil surface and flooded water (Huda et al., 2016; Yao et al., 2018b); which, in turn, increases the NH3 emissions in the CRFD2 treatment.
Conventional broadcasting of N fertilizers is considered an inefficient method for rice production (Nkebiwe et al., 2016). Rice grain yield may be decreased by simply reducing the application rate of N fertilizer without changing the fertilization method (Yin et al., 2022). However, deep placement of N fertilizer is a more effective alternative to broadcast fertilization to increase crop yield and NUE in paddy fields (Nkebiwe et al., 2016; Zhu et al., 2022). In the current field experiment, side-deep placement of fertilizer increased rice grain yield and NUE in both seasons over the 3 years (Figures 5, 6). It is generally recognized that deep placement of N fertilizer can increase rice grain yield by 15–20% and NUE by 50–70% compared to the surface broadcasting of N fertilize (Pan et al., 2017; Yao et al., 2018a, 2018b; Li et al., 2021). Deep placement of N fertilizer results in higher NH4+–N concentrations in and around fertilizer band, and thereby enhances N uptake by rice root and improves grain yield compared to the broadcast fertilization (Yao et al., 2018a; Zhu et al., 2022). For example, Zhong et al. (2021a) demonstrated that grain yields in the MRSF treatments with compound fertilizer increased by 5.3–20.2% in early rice and 1.3–15.0% in late rice compared to the broadcasting of compound fertilizer. Similar results have been also found in upland fields ( ). Deep placement of fertilizer could increase yield and NUE of maize in northwest China (Guo et al., 2016; Wu et al., 2021). In 2021, the rice grain yields in MRSF with CRF were higher than in the MRSF with compound fertilizer in both seasons (Figure 5C). Deep placement of CRF can increase rice yield and N utilization compared to urea deep placement due to continuous supply high level of NH4+–N to rice plant under MRSF with CRF during rice growing season (Hou et al., 2021; Ke et al., 2018).
Previous studies have shown that deep placement of N fertilizer increases the net economic benefit compared to surface broadcasting of N fertilizer in paddy fields (Li et al., 2021; Zhu et al., 2022). The labor cost was lower in MRSF treatment than in CFB treatment due to reduction of topdressing timing. Similarly, Min et al. (2021) demonstrated that MRSF treatments save 1500–2250 yuan ha−1 of labor costs compared to manual broadcasting of fertilizer in a single rice cropping system in southeast China. Use of MRSF reduces labor and machining cost by 700 yuan ha−1 compared to broadcasting of fertilizer in double rice cropping system. In this study, although the fertilizer costs for the CRFD1 and CRFD2 were higher than those for the CFB treatment, MRSF with CRF could increase the rice grain yield and economic benefit and reduce labor costs ultimately MRSF increased the profitability for farmers. This is consistent with the results of Zhong et al. (2021a), who found that MRSF increased the economic benefit for early and late rice by 15.0–31.8% and 9.9–25.7% compared to the CFB treatment. Li et al. (2021) reported that mechanized deep placement of N fertilizer had higher gross economic return and lower input cost in pot-seedling transplanting rice, compared to conventional fertilization.
Compared to maize and wheat, rice production is more labor-intensive, time-consuming and economic input due to manual fertilizer application and pesticide spraying (Yang et al., 2022a; Yang et al., 2022b). In rice production in China, the N fertilization is usually split into multiple applications and broadcasted by hand (Zhang et al., 2013). With rapid urbanization in China, light-simplified fertilization has become an urgent need for rice production, especially for double rice cropping systems with short turn-around periods between early rice harvest and late rice planting (Wang et al., 2016). The light-simplified fertilization is reflected in time and labor savings (Paman et al., 2014). In addition, efficient use of N fertilizer is needed to meet the increasing food demand, minimize negative environmental impacts and maximize farmers’ profits (Mazid Miah et al., 2016). Previous studies have shown that single application of CRF could enhance crop yield and NUE while reducing labor and time required for rice production (Yang et al., 2012; Zhang et al., 2018; Xu et al., 2022; Zhang et al., 2022). However, in this rice production, CRF is also applied by hand before rice transplanting, which is not adapted to the mechanization of rice production.
In the current study, MRSF treatments were shown to positively improve rice yield and NUE and reduce NH3 volatilization loss in double rice cropping systemd. More importantly, the MRSF with one-time CRF application (CRFD2) had the lowest NH3 emissions and highest NEB among the three MRSF treatments. Additionally, CRFD2 treatment is less time- and labor-intensive than CFD and CRFD1 treatment because of the single application as basal fertilizer. These results showed that integrating machine transplantation and single basal fertilization of CRU would result in light-simplified rice production, saving both labor cost and time and improving rice grain yield and profitability. Li et al. (2021) reported that mechanical deep placement with single basal fertilization of compound fertilizer could increase the grain yield and the nutrients uptake of early rice compared to broadcasting of fertilizer. Hou et al. (2021) found that deep placement of CRF was a more efficient strategy for higher grain yield and NUE of rice than deep placement of urea. Considering farmers’ interest, rice yield, environmental impact and economic benefit, MRSF with a one-time application of CRF is a promising fertilizer management method for double-cropping rice production in southeast China.
5 Conclusion
The results of the present study demonstrated that the cumulative NH3 losses from early and late rice paddy fields accounted for 11.9–17.9% and 19.5–24.2% of the total applied N in the CFB treatment. However, the MRSF treatments significantly decreased the N loss via NH3 volatilization by 42.5–67.9% and 58.5–80.9% for early and late rice across 2 years, compared to the CFB treatment. This was primarily due to decreased NH4+–N concentration in flooded water during basal and tillering fertilizations under MRSF treatments. Changing the N fertilizer application method from surface broadcast to side-deep placement increased rice grain yield, NUE and net economic benefit, while reducing yield-scale NH3 volatilization in double rice cropping systems. More importantly, MRSF with a one-time CRF application (CRFD2) significantly increased crop yields and mitigated NH3 emissions in a double rice cropping system. Thus it was identified as a light-simplified fertilization method that could enhance economic profitability and save labor input and topdressing time. Future research should evaluate the responses of greenhouse gases emissions to MRSF treatments in double rice cropping systems.
Data availability statement
The original contributions presented in the study are included in the article/Supplementary Material, further inquiries can be directed to the corresponding author.
Author contributions
ZC: investigation, conceptualization, writing—original draft; QW: conceptualization, writing-review and editing, supervision, funding acquisition; JcM: investigation, formal analysis, data curation; JZ: methodology, data curation, software; YH: software, data curation. JwM: conceptualization, visualization; JY: investigation; QY: data curation; PZ: methodology, formal analysis; WS: data curation, formal analysis; GZ: investigation, formal analysis; YZ: investigation, formal analysis.
Funding
This research was financially supported by the Key Research and Development Program of Zhejiang Province of China (2019C02017, 2021C02035, 2020C02030-06), the Construction Project of Agricultural Green Development Pilot Support System of Huangyan District (HY202001) and the Project of Unification of Water and Soil Science for One Healthy funded by the Zhejiang Academy of Agricultural Sciences.
Conflict of interest
The authors declare that the research was conducted in the absence of any commercial or financial relationships that could be construed as a potential conflict of interest.
Publisher’s note
All claims expressed in this article are solely those of the authors and do not necessarily represent those of their affiliated organizations, or those of the publisher, the editors and the reviewers. Any product that may be evaluated in this article, or claim that may be made by its manufacturer, is not guaranteed or endorsed by the publisher.
Supplementary material
The Supplementary Material for this article can be found online at: https://www.frontiersin.org/articles/10.3389/fenvs.2022.1006606/full#supplementary-material
References
Adalibieke, W., Zhan, X., Cui, X., Reis, S., Winiwarter, W., and Zhou, F. (2021). Decoupling between ammonia emission and crop production in China due to policy interventions. Glob. Chang. Biol. 27, 5877–5888. doi:10.1111/gcb.15847
Bautista, E. U., Koike, M., and Suministrado, D. C. (2001). PM—power and machinery: Mechanical deep placement of nitrogen in wetland rice. J. Agric. Eng. Res. 78, 333–346. doi:10.1006/jaer.2000.0675
Chen, Z., Wang, Q., Ma, J., Zou, P., and Jiang, L. (2020). Impact of controlled-release urea on rice yield, nitrogen use efficiency and soil fertility in A single rice cropping system. Sci. Rep. 10, 10432. doi:10.1038/s41598-020-67110-6
Chien, S. H., Prochnow, L. I., and Cantarella, H. (2009). Chapter 8. Recent developments of fertilizer production and use to improve nutrient efficiency and minimize environmental impacts. Adv. Agron. 102, 267–322.
Cooperative Research Group on Chinese Soil Taxonomy (2001). Chinese soil Taxonomy. Beijing: Science Press. (in Chinese).
Cui, Z., Wang, G., Yue, S., Wu, L., Zhang, W., Zhang, F., et al. (2014). Closing the N-use efficiency gap to achieve food and environmental security. Environ. Sci. Technol. 48, 5780–5787. doi:10.1021/es5007127
Gaihre, Y. K., Singh, U., Islam, S. M. M., Huda, A., Islam, M. R., Satter, M. A., et al. (2015). Impacts of urea deep placement on nitrous oxide and nitric oxide emissions from rice fields in Bangladesh. Geoderma 259–260, 370–379. doi:10.1016/j.geoderma.2015.06.001
Geng, J., Sun, Y., Zhang, M., Li, C., Yang, Y., Liu, Z., et al. (2015). Long-term effects of controlled release urea application on crop yields and soil fertility under rice-oilseed rape rotation system. Field Crops Res. 184, 65–73. doi:10.1016/j.fcr.2015.09.003
Grant, C. A., Wu, R., Selles, F., Harker, K. N., Clayton, G. W., Bittman, S., et al. (2012). Crop yield and nitrogen concentration with controlled release urea and split applications of nitrogen as compared to non-coated urea applied at seeding. Field Crops Res. 127, 170–180. doi:10.1016/j.fcr.2011.11.002
Guo, L., Ning, T., Nie, L., Li, Z., and Lal, R. (2016). Interaction of deep placed controlled-release urea and water retention agent on nitrogen and water use and maize yield. Eur. J. Agron. 75, 118–129. doi:10.1016/j.eja.2016.01.010
Guo, C., Ren, T., Li, P., Wang, B., Zou, J., Hussain, S., et al. (2019). Producing more grain yield of rice with less ammonia volatilization and greenhouse gases emission using slow/controlled-release urea. Environ. Sci. Pollut. Res. 26, 2569–2579. doi:10.1007/s11356-018-3792-2
He, T., Liu, D., Yuan, J., Ni, K., Zaman, M., Luo, J., et al. (2018). A two years study on the combined effects of biochar and inhibitors on ammonia volatilization in an intensively managed rice field. Agric. Ecosyst. Environ. 264, 44–53. doi:10.1016/j.agee.2018.05.010
Hou, P., Yuan, W., Li, G., Petropoulos, E., Xue, L., Feng, Y., et al. (2021). Deep fertilization with controlled-release fertilizer for higher cereal yield and N utilization in paddies: The optimal fertilization depth. Agron. J. 113, 5027–5039. doi:10.1002/agj2.20772
Huda, A., Gaihre, Y. K., Islam, M. R., Singh, U., Islam, M. R., Sanabria, J., et al. (2016). Floodwater ammonium, nitrogen use efficiency and rice yields with fertilizer deep placement and alternate wetting and drying under triple rice cropping systems. Nutr. Cycl. Agroecosyst. 104, 53–66. doi:10.1007/s10705-015-9758-6
Jiang, Y., Deng, A., Bloszies, S., Huang, S., and Zhang, W. (2017). Nonlinear response of soil ammonia emissions to fertilizer nitrogen. Biol. Fertil. Soils 53, 269–274. doi:10.1007/s00374-017-1175-3
Jiang, Z., Yang, S., Chen, X., Pang, Q., Xu, Y., Qi, S., et al. (2022). Controlled release urea improves rice production and reduces environmental pollution: A research based on meta-analysis and machine learning. Environ. Sci. Pollut. Res. 29, 3587–3599. doi:10.1007/s11356-021-15956-2
Ju, X. T., Xing, G. X., Chen, X. P., Zhang, S. L., Zhang, L. J., Liu, X. J., et al. (2009). Reducing environmental risk by improving N management in intensive Chinese agricultural systems. Proc. Natl. Acad. Sci. U. S. A. 106, 3041–3046. doi:10.1073/pnas.0813417106
Ke, J., He, R., Hou, P., Ding, C., Ding, Y., Wang, S., et al. (2018). Combined controlled-released nitrogen fertilizers and deep placement effects of N leaching, rice yield and N recovery in machine-transplanted rice. Agric. Ecosyst. Environ. 265, 402–412. doi:10.1016/j.agee.2018.06.023
Li, L., Zhang, Z., Tian, H., Ashraf, U., Mo, Z., Tang, X., et al. (2021). Productivity and profitability of mechanized deep nitrogen fertilization in mechanical pot-seedling transplanting rice in south China. Agron. J. 113, 1664–1680. doi:10.1002/agj2.20564
Li, P., Lu, J., Hou, W., Pan, Y., Wang, Y., Khan, M. R., et al. (2017). Reducing nitrogen losses through ammonia volatilization and surface runoff to improve apparent nitrogen recovery of double cropping of late rice using controlled release urea. Environ. Sci. Pollut. Res. 24, 11722–11733. doi:10.1007/s11356-017-8825-8
Li, P., Lu, J., Wang, Y., Wang, S., Hussain, S., Ren, T., et al. (2018). Nitrogen losses, use efficiency, and productivity of early rice under controlled-release urea. Agric. Ecosyst. Environ. 251, 78–87. doi:10.1016/j.agee.2017.09.020
Liang, K., Zhong, X., Pan, J., Huang, N., Liu, Y., Peng, B., et al. (2019). Reducing nitrogen surplus and environmental losses by optimized nitrogen and water management in double rice cropping system of South China. Agric. Ecosyst. Environ. 286, 106680. doi:10.1016/j.agee.2019.106680
Linquist, B. A., Liu, L., van Kessel, C., and van Groenigen, K. J. (2013). Enhanced efficiency nitrogen fertilizers for rice systems: Meta-analysis of yield and nitrogen uptake. Field Crops Res. 154, 246–254. doi:10.1016/j.fcr.2013.08.014
Liu, T. Q., Fan, D. J., Zhang, X. X., Chen, J., Li, C. F., and Cao, C. G. (2015). Deep placement of nitrogen fertilizers reduces ammonia volatilization and increases nitrogen utilization efficiency in No-tillage paddy fields in central China. Field Crops Res. 184, 80–90. doi:10.1016/j.fcr.2015.09.011
Liu, T. Q., Li, S. H., Guo, L. G., Cao, C. G., Li, C. F., Zhai, Z. B., et al. (2020). Advantages of nitrogen fertilizer deep placement in greenhouse gas emissions and net ecosystem economic benefits from No-tillage paddy fields. J. Clean. Prod. 263, 121322. doi:10.1016/j.jclepro.2020.121322
Lu, R. K. (1999). Soil and agro-chemistry analytical methods. Beijing: Agriculture Science and Technology Press of China. (in Chinese).
Lyu, Y., Yang, X., Pan, H., Zhang, X., Cao, H., Ulgiati, S., et al. (2021). Impact of fertilization schemes with different ratios of urea to controlled release nitrogen fertilizer on environmental sustainability, nitrogen use efficiency and economic benefit of rice production: A study case from southwest China. J. Clean. Prod. 293, 126198. doi:10.1016/j.jclepro.2021.126198
Mazid Miah, M. A., Gaihre, Y. K., Hunter, G., Singh, U., and Hossain, S. A. (2016). Fertilizer deep placement increases rice production: Evidence from farmers’ fields in southern Bangladesh. Agron. J. 108, 805–812. doi:10.2134/agronj2015.0170
Miao, X., Xing, X., Ding, Y., Ke, J., Liu, Z., Tang, S., et al. (2016). Yield and nitrogen uptake of bowl-seedling machine-transplanted rice with slow-release nitrogen fertilizer. Agron. J. 108, 313–320. doi:10.2134/agronj2015.0101
Min, J., Sun, H., Wang, Y., Pan, Y., Kronzucker, H. J., Zhao, D., et al. (2021). Mechanical side-deep fertilization mitigates ammonia volatilization and nitrogen runoff and increases profitability in rice production independent of fertilizer type and split ratio. J. Clean. Prod. 316, 128370. doi:10.1016/j.jclepro.2021.128370
National Bureau of Statistics of China (NBSC) (2021). China statistical yearbook. Beijing: China Statistics Press. (in Chinese).
Nkebiwe, P. M., Weinmann, M., Bar-Tal, A., and Müller, T. (2016). Fertilizer placement to improve crop nutrient acquisition and yield: A review and meta-analysis. Field Crops Res. 196, 389–401. doi:10.1016/j.fcr.2016.07.018
Paman, U., Inaba, S., and Uchida, S. (2014). The mechanization of small-scale rice farming: Labor requirements and costs. Eng. Agric. Environ. Food 7, 122–126. doi:10.1016/j.eaef.2014.03.001
Pan, S., Wen, X., Wang, Z., Ashraf, U., Tian, H., Duan, M., et al. (2017). Benefits of mechanized deep placement of nitrogen fertilizer in direct-seeded rice in south China. Field Crops Res. 203, 139–149. doi:10.1016/j.fcr.2016.12.011
Peng, S., Buresh, R. J., Huang, J., Yang, J., Zou, Y., Zhong, X., et al. (2006). Strategies for overcoming low agronomic nitrogen use efficiency in irrigated rice systems in China. Field Crops Res. 96, 37–47. doi:10.1016/j.fcr.2005.05.004
Pittelkow, C. M., Adviento-Borbe, M. A., van Kessel, C., Hill, J. E., and Linquist, B. A. (2014). Optimizing rice yields while minimizing yield-scaled global warming potential. Glob. Chang. Biol. 20, 1382–1393. doi:10.1111/gcb.12413
Rose, T. J., Quin, P., Morris, S. G., Kearney, L. J., Kimber, S., Rose, M. T., et al. (2018). No evidence for higher agronomic N use efficiency or lower nitrous oxide emissions from enhanced efficiency fertilisers in aerobic subtropical rice. Field Crops Res. 225, 47–54. doi:10.1016/j.fcr.2018.06.001
Seck, P. A., Diagne, A., Mohanty, S., and Wopereis, M. C. S. (2012). Crops that feed the world 7: Rice. Food Secur. 4, 7–24. doi:10.1007/s12571-012-0168-1
Shang, Q., Gao, C., Yang, X., Wu, P., Ling, N., Shen, Q., et al. (2014). Ammonia volatilization in Chinese double rice-cropping systems: A 3-year field measurement in long-term fertilizer experiments. Biol. Fertil. Soils 50, 715–725. doi:10.1007/s00374-013-0891-6
Sun, X., Zhong, T., Zhang, L., Zhang, K., and Wu, W. (2019). Reducing ammonia volatilization from paddy field with rice straw derived biochar. Sci. Total Environ. 660, 512–518. doi:10.1016/j.scitotenv.2018.12.450
Tian, C., Zhou, X., Ding, Z., Liu, Q., Xie, G., Peng, J., et al. (2021). Controlled-release N fertilizer to mitigate ammonia volatilization from double-cropping rice. Nutr. Cycl. Agroecosyst. 119, 123–137. doi:10.1007/s10705-020-10108-3
Wang, C., Sun, H., Zhang, J., Zhang, X., Lu, L., Shi, L., et al. (2021). Effects of different fertilization methods on ammonia volatilization from rice paddies. J. Clean. Prod. 295, 126299. doi:10.1016/j.jclepro.2021.126299
Wang, H., Zhang, D., Zhang, Y., Zhai, L., Yin, B., Zhou, F., et al. (2018). Ammonia emissions from paddy fields are underestimated in China. Environ. Pollut. 235, 482–488. doi:10.1016/j.envpol.2017.12.103
Wang, X., Yamauchi, F., Otsuka, K., and Huang, J. (2016). Wage growth, landholding, and mechanization in Chinese agriculture. World Dev. 86, 30–45. doi:10.1016/j.worlddev.2016.05.002
Wang, Y., and Lu, Y. (2020). Evaluating the potential health and economic effects of nitrogen fertilizer application in grain production systems of China. J. Clean. Prod. 264, 121635. doi:10.1016/j.jclepro.2020.121635
Wang, Z. H., Liu, X. J., Ju, X. T., Zhang, F. S., and Malhi, S. S. (2004). Ammonia volatilization loss from surface-broadcast urea: Comparison of vented- and closed-chamber methods and loss in winter wheat–summer maize rotation in north China plain. Commun. Soil Sci. Plant Anal. 35, 2917–2939. doi:10.1081/css-200036499
Wolfe, A. H., and Patz, J. A. (2002). Reactive nitrogen and human health: Acute and long-term implications. AMBIO A J. Hum. Environ. 31, 120–125. doi:10.1579/0044-7447-31.2.120
Wu, P., Liu, F., Li, H., Cai, T., Zhang, T., and Jia, Z. (2021). Suitable fertilizer application depth can increase nitrogen use efficiency and maize yield by reducing gaseous nitrogen loss. Sci. Total Environ. 781, 146787. doi:10.1016/j.scitotenv.2021.145787
Xu, J., Peng, S., Yang, S., and Wang, W. (2012). Ammonia volatilization losses from A rice paddy with different irrigation and nitrogen managements. Agric. Water Manag. 104, 184–192. doi:10.1016/j.agwat.2011.12.013
Xu, X., Ma, F., Zhou, J., and Du, C. (2022). Control-released urea improved agricultural production efficiency and reduced the ecological and environmental impact in rice-wheat rotation system: A life-cycle perspective. Field Crops Res. 278, 108445. doi:10.1016/j.fcr.2022.108445
Yang, M., Zhu, X., Bai, Y., Sun, D., Zou, H., Fang, Y., et al. (2021). Coated controlled-release urea creates A win-win scenario for producing more staple grains and resolving N loss dilemma worldwide. J. Clean. Prod. 288, 125660. doi:10.1016/j.jclepro.2020.125660
Yang, Y., Zhang, M., Li, Y. C., Fan, X., and Geng, Y. (2012). Controlled release urea improved nitrogen use efficiency, activities of leaf enzymes, and rice yield. Soil Sci. Soc. Am. J. 76, 2307–2317. doi:10.2136/sssaj2012.0173
Yang, Z., Cheng, Q., Liao, Q., Fu, H., Zhang, J., Zhu, Y., et al. (2022a). Can reduced-input direct seeding improve resource use efficiencies and profitability of hybrid rice in China? Sci. Total Environ. 833, 155186. doi:10.1016/j.scitotenv.2022.155186
Yang, Z., Zhu, Y., Zhang, J., Li, X., Ma, P., Sun, J., et al. (2022b). Comparison of energy use between fully mechanized and semi-mechanized rice production in Southwest China. Energy 245, 123270. doi:10.1016/j.energy.2022.123270
Yao, Y., Zhang, M., Tian, Y., Zhao, M., Zhang, B., Zeng, K., et al. (2018a). Urea deep placement in combination with Azolla for reducing nitrogen loss and improving fertilizer nitrogen recovery in rice field. Field Crops Res. 218, 141–149. doi:10.1016/j.fcr.2018.01.015
Yao, Y., Zhang, M., Tian, Y., Zhao, M., Zhang, B., Zhao, M., et al. (2018b). Urea deep placement for minimizing NH3 loss in an intensive rice cropping system. Field Crops Res. 218, 254–266. doi:10.1016/j.fcr.2017.03.013
Yin, Y., Peng, X., Guo, S., Zhai, L., Hua, L., Wang, H., et al. (2022). How to improve the light-simplified and cleaner production of rice in cold rice areas from the perspective of fertilization. J. Clean. Prod. 361, 131694. doi:10.1016/j.jclepro.2022.131694
Yin, Y., Zhao, R., Yang, Y., Meng, Q., Ying, H., Cassman, K. G., et al. (2021). A steady-state N balance approach for sustainable smallholder farming. Proc. Natl. Acad. Sci. U. S. A. 118, e2106576118. doi:10.1073/pnas.2106576118
Zhang, F., Cui, Z., Chen, X., Ju, X., Shen, J., Chen, Q., et al. (2012). Integrated nutrient management for food security and environmental quality in China. Adv. Agron. 116, 1–40. doi:10.1016/B978-0-12-394277-7.00001-4
Zhang, G., Zhao, D., Liu, S., Liao, Y., and Han, J. (2022). Can controlled-release urea replace the split application of normal urea in China? A meta-analysis based on crop grain yield and nitrogen use efficiency. Field Crops Res. 275, 108343. doi:10.1016/j.fcr.2021.108343
Zhang, M., Yao, Y., Zhao, M., Zhang, B., Tian, Y., Yin, B., et al. (2017). Integration of urea deep placement and organic addition for improving yield and soil properties and decreasing N loss in paddy field. Agric. Ecosyst. Environ. 247, 236–245. doi:10.1016/j.agee.2017.07.001
Zhang, S., Shen, T., Yang, Y., Li, Y. C., Wan, Y., Zhang, M., et al. (2018). Controlled-release urea reduced nitrogen leaching and improved nitrogen use efficiency and yield of direct-seeded rice. J. Environ. Manage. 220, 191–197. doi:10.1016/j.jenvman.2018.05.010
Zhang, W. F., Dou, Z. X., He, P., Ju, X. T., Powlson, D., Chadwick, D., et al. (2013). New technologies reduce greenhouse gas emissions from nitrogenous fertilizer in China. Proc. Natl. Acad. Sci. U. S. A. 110, 8375–8380. doi:10.1073/pnas.1210447110
Zhao, C., Huang, H., Qian, Z. H., Jiang, H. X., Liu, G. M., Xu, K., et al. (2021). Effect of side deep placement of nitrogen on yield and nitrogen use efficiency of single season late japonica rice. J. Integr. Agric. 20, 1487–1502. doi:10.1016/s2095-3119(20)63362-7
Zhong, X., Peng, J., Kang, X., Wu, Y., Luo, G., Hu, W., et al. (2021a). Optimizing agronomic traits and increasing economic returns of machine-transplanted rice with side-deep fertilization of double-cropping rice system in southern China. Field Crops Res. 270, 108191. doi:10.1016/j.fcr.2021.108191
Zhong, X., Zhou, X., Fei, J., Huang, Y., Wang, G., Kang, X., et al. (2021b). Reducing ammonia volatilization and increasing nitrogen use efficiency in machine-transplanted rice with side-deep fertilization in A double-cropping rice system in southern China. Agric. Ecosyst. Environ. 306, 107183. doi:10.1016/j.agee.2020.107183
Zhu, C. H., Ouyang, Y. Y., Diao, Y., Yu, J. Q., Luo, X., Zheng, J. G., et al. (2021). Effects of mechanized deep placement of nitrogen fertilizer rate and type on rice yield and nitrogen use efficiency in chuanxi plain, China. J. Integr. Agric. 20, 581–592. doi:10.1016/s2095-3119(20)63456-6
Zhu, C., Xiang, J., Zhang, Y., Zhang, Y., Zhu, D., and Chen, H. (2019). Mechanized transplanting with side deep fertilization increases yield and nitrogen use efficiency of rice in eastern China. Sci. Rep. 9, 5653. doi:10.1038/s41598-019-42039-7
Zhu, W. B., Zeng, K., Tian, Y. H., and Yin, B. (2022). Coupling side-deep fertilization with Azolla to reduce ammonia volatilization while achieving A higher net economic benefits in rice cropping system. Agric. Ecosyst. Environ. 333, 107976. doi:10.1016/j.agee.2022.107976
Keywords: machine-transplanted rice, side-deep fertilization, ammonia volatilization, nitrogen use efficiency, economic benefit
Citation: Chen Z, Wang Q, Ma J, Zhao J, Huai Y, Ma J, Ye J, Yu Q, Zou P, Sun W, Zhang G and Zhao Y (2022) Combing mechanical side-deep fertilization and controlled-release nitrogen fertilizer to increase nitrogen use efficiency by reducing ammonia volatilization in a double rice cropping system. Front. Environ. Sci. 10:1006606. doi: 10.3389/fenvs.2022.1006606
Received: 29 July 2022; Accepted: 15 August 2022;
Published: 13 September 2022.
Edited by:
Zijun Zhou, Sichuan Academy of Agricultural Sciences, ChinaReviewed by:
Hanlin Zhang, Shanghai Academy of Agricultural Sciences, ChinaChunQuan Zhu, China National Rice Research Institute (CAAS), China
Copyright © 2022 Chen, Wang, Ma, Zhao, Huai, Ma, Ye, Yu, Zou, Sun, Zhang and Zhao. This is an open-access article distributed under the terms of the Creative Commons Attribution License (CC BY). The use, distribution or reproduction in other forums is permitted, provided the original author(s) and the copyright owner(s) are credited and that the original publication in this journal is cited, in accordance with accepted academic practice. No use, distribution or reproduction is permitted which does not comply with these terms.
*Correspondence: Qiang Wang, qwang0571@126.com