- 1Department of Civil Engineering, College of Ocean Science, Jeju National University, Jeju, South Korea
- 2Department of Geography, University of Gujrat, Gujrat, Pakistan
- 3Department of Civil, Environmental and Natural Resources Engineering, Lulea University of Technology, Lulea, Sweden
Freshwater resources present in the Upper Indus Basin (UIB) supply water to Pakistan’s irrigation. Half of the annual water discharge in the UIB is contributed from the glacier and snow-fed basins in the Hindu Kush, Karakoram, and Himalaya (HKH) region, and it is currently under the threat of climate change. Therefore, it is very necessary to study and monitor the spatiotemporal changes in the snow cover area (SCA) and its response to climate variables to efficiently manage water reservoirs. Thus, keeping this problem in mind, we conducted this study using the Google Earth Engine Application Programming Interface (GEE API) for Astore and Shigar. We used mean annual Landsat data between 1991 and 2021 to derive the SCA using the normalized difference snow index (NDSI). ASTER GDEM data was used to extract the elevation and analyze the distribution of SCA on different elevation zones. Eventually, we used the climate research unit (CRU) data (rainfall and temperature) to analyze them with SCA. The results revealed that SCA in Astore and Shigar has an increasing trend with a rate of 11.16 km2/year and 4.27 km2/year, respectively. Mean annual precipitation and temperature also confirmed the increasing trend of SCA because mean annual precipitation is increasing and temperature is decreasing in both regions. SCA and elevation analysis revealed that SCA is decreasing on foothills while increasing at the valley top. This is because temperature is increasing and precipitation is decreasing from zone 1 to zone 3 and vice versa for zone 4. It is found that Astore and Shigar in UIB have an increasing trend of SCA and are not affected by global warming. Therefore, it is necessary to conduct studies on large scale to efficiently evaluate the impact of climate change on SCA.
Introduction
Hindu Kush, Karakoram, and Himalaya (HKH) regions are the longest mountainous regions and are spread over various countries including Afghanistan, Bhutan, China, India, Myanmar, Nepal, and Pakistan. The HKH region stores a huge amount of freshwater resources outside the polar region; therefore, it is also known as the Third Pole or Water Tower of Asia (Kehrwald et al., 2008). Many largest rivers in these countries originate from the HKH region and are fed through snow and glacier meltwater (Banerjee et al., 2021). Therefore, this region supports the livelihood of people living downstream through water supply (Sharma et al., 2013, Sahu and Gupta, 2020) and fulfills their essential needs in terms of agriculture production, hydropower generation, drinking, and industrialization (Jain et al., 2009; Hori et al., 2017). However, due to climate change, glaciers and snow-covered areas are under serious threat and consistently change with time (Wester et al., 2019). Pakistan is an agro-economic country and largely dependent on water supply from the Indus River System (IRS) (SIHP, 1990), and the inflow of water in the Indus River is mostly contributed by glaciers and snow meltwater (Bookhagen and Burbank, 2010; Immerzeel et al., 2012). Snow-covered mountains are not directly influenced by the human population, but they are vulnerable to climate change, and subsequently, the rising trend of temperature, decreasing trend of snow cover duration, and changing pattern of snowfall result in early melting of snow cover and influence river runoff in the summer season (Krishnan et al., 2019; Notarnicola, 2020; Yi et al., 2021). Eventually, it will result in scarcity of water, landslide, drought, floods, and glacial lake outburst flood (GLOF) (Tahir et al., 2019). Snow cover research on this region usually revealed a decreasing trend of SCA; however, Tahir et al. (2016), Shafiq et al. (2019), and Azizi and Akhtar, 2021) reported an increasing trend of SCA due to an increasing trend of winter precipitation and decreasing trend of summer temperature. Another study also projected an increasing trend of precipitation in the HKH region during 1901–2099, while temperature indices show an inter-annual decreasing trend (Panday et al., 2015). Moazzam et al. (2022) recently studied the precipitation projection of Gilgit-Baltistan for the 21st century and revealed a significant increasing trend in the midcentury (2021–2060) under representative concentration pathway (RCP) 4.5 and RCP 8.5. Negi and Kanda, (2020) also reported an increasing trend of SCA in the Northwest Himalayan region due to the slowdown warming trend. Therefore, these climate variabilities imply differences in SCA. Hence, it is necessary to monitor the changing phenomena of snow cover. However, a large area is at risk in the HKH region, and knowledge of spatial variability of SCA is limited on a large scale.
In the past, it was very difficult to map the SCA because of high altitude and tough terrains (Aniya et al., 1996; Jacobsen and Theakstone, 1997), but, since the early 1960s, remote sensing sensors have been designed to easily map the SCA with various techniques and methodologies (Abid and Zia, 2019). Now researchers are using various indices and methods for SCA mapping, i.e., normalized difference snow index (NDSI), normalized difference snow thermal index (NDSTI), normalized difference water index (NDWI), normalized difference principal component index (NDPSCI), normalized difference debris index (NDDI), machine learning algorithms [support vector machine (SVM), artificial neural network (ANN), and random forest (RF)], and image classification techniques. In this study, we used NDSI, which is one of the widely used indices globally because of its satisfactory results in mountain shadows.
It is a very challenging task to study the large snow-covered area and manually filter the images with less amount of clouds, download, make composite, and stitch the image which consumes immense time. Therefore, we used a cloud computing (Google Earth Engine) platform, which makes it easy to perform all these tasks using JavaScript or Python APIs. This study aimed to investigate the snow cover area of Astore and Shigar using the normalized difference snow index (NDSI) between 1991 and 2021. The snow cover area was correlated with climate variables (temperature and precipitation) and topographic parameters (elevation). The significance of this study was to understand the SCA relationship with the temperature and rainfall which is useful for mountainous and low-lying glacier-dependent communities.
We chose Astore and Shigar in this study because previous studies have been conducted on the comparison of Astore with Hunza and Gilgit (Tahir et al., 2016); hence, there is a need for a comparative study between other sub-basins of UIB. Therefore, in this study, we evaluated Astore (West Himalayas; snow-fed) and Shigar (Karakoram; glacier-fed) based on meteorological and topographical indicators. Astore and Shigar have distinct geographical locations due to some basic features, i.e., both districts are influenced by the same climate (westerlies) but in a different way. Astore is largely dependent on winter rainfall at low elevation, while Shigar depends on westerly circulated solid precipitation. Shigar is located in the rain shadow of the Western Himalayan region; therefore, it receives a very small amount of monsoon precipitation (Ali et al., 2009), while Astore is located on a north-facing slope in lower latitude and mid-altitude. Therefore, we conducted this study based on these contrasting features.
Study area
The Upper Indus Basin is located between 72o 03′–77 o 44′E and 34o 16′–37 o 06′N. It splits the Karakoram from the Greater Himalayan region (Bishop et al., 2010). The Indus River starts from the Tibetan Plateau and flows toward the northern region of Pakistan and supplies inflow to the Tarbela Dam (Farhan et al., 2015; Farhan et al., 2020). For this study we have chosen two districts, namely, Astore and Shigar that lie in the Western Himalayas and Karakoram region, respectively.
Astore is located in the extreme Western Himalayan region of Northern Pakistan (Figure 1A). Astore covers an area of 5,233 km2 with 4.8% area covered with glaciers from Randolph Glacier Inventory version 6.0 (RGI, 2017). The elevation of Astore ranges from the valley floor to the highest peaks, i.e., Nanga Parbat (1,150–8,056 m) (Figure 1B). The mean annual temperature of Astore ranges between −2.9 and 9.9, while precipitation ranges between 500–870 mm from higher to lower altitude met stations (Farhan et al., 2015). Almost 75% of the precipitation falls in winter and spring seasons due to westerly circulation, while 25% falls in summer and autumn seasons from monsoon and local jet streams (Farhan et al., 2020). The Astore River discharge is influenced by winter rainfall together with solid precipitation forced by westerlies (Tahir et al., 2015).
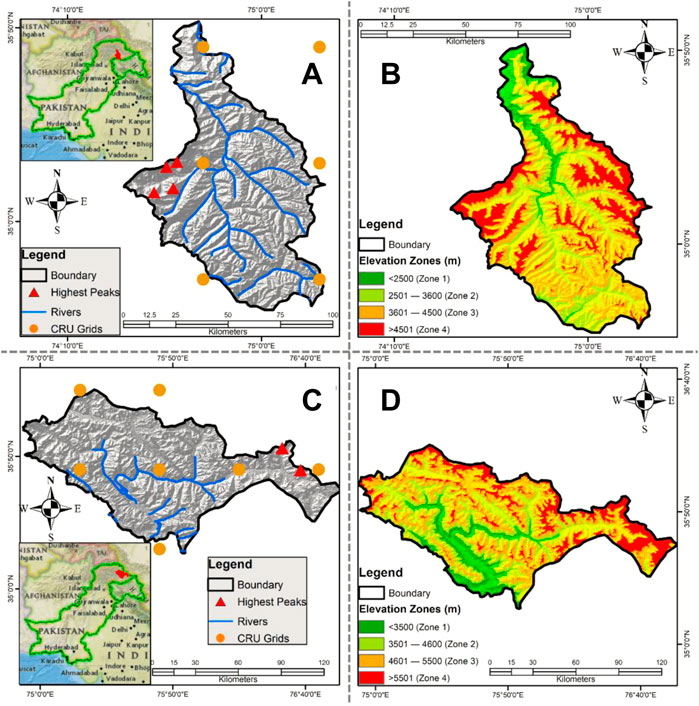
FIGURE 1. (A) Location map of Astore, (B) elevation zones of Astore, (C) location map of Shigar, and (D) elevation zones of Shigar.
Shigar is located in the Karakoram region of Northern Pakistan (Figure 1C). Shigar covers an area of 8,913. The elevation of Shigar ranges between 1,967 and 8,611 m from valley to high mountains (Figure 1D). According to Koppen–Geiger’s climate classification, Shigar falls under the cold desert region, and Shigar receives a little amount of precipitation. Large glacier mountains make a barrier for monsoon storms and enforce a modest impact of the storm on the region (Khan et al., 2014). A considerable amount of winter precipitation and temperature increased; however, the summer season revealed a cooling pattern for the Skardu region (Fowler and Archer, 2005; Hakeem et al., 2014). The Shigar River discharge is dependent on solid precipitation forced by westerlies that melts in the summer season and generates high discharge (ICIMOD, 2005; Fowler and Archer, 2006; Hakeem et al., 2014).
Materials and methods
In this section, we discussed the detailed methodology of the research. The methodological framework of this study was designed following the objective of the study. The study is based on a cloud computing platform (Google Earth Engine) because it provides easy access to global remote sensing datasets in a matter of no time using JavaScript or Python APIs (Gorelick et al., 2017). The Landsat images used in this study were atmospherically corrected, orthorectified, top of atmospheric reflectance (TOA) calibrated, and georeferenced scenes with an accuracy of 0.4 pixels (Zanter, 2016), available in the dataset catalog of GEE. We acquired the Landsat data using the Google Earth Engine (GEE) platform with JavaScript. A total of 5 paths/rows and around 10 scenes were required to cover the study area (Figure 2). We filtered the Landsat Tier-1 data by applying the multiple queries [e.g., date (”2021-01-01”, “2021-12-31”), cloud cover (<10%), and region of interest (Astore, Shigar)] using the GEE image collection catalog.
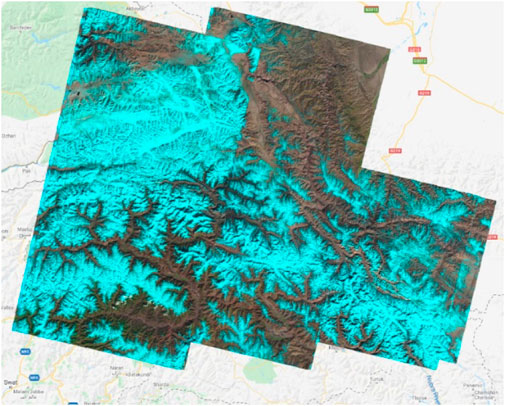
FIGURE 2. Mosaiced image with 10 scenes in Google Earth Engine (GEE) with false color composite and less than 10% cloud cover over the study area.
The images selected in this study do not have a cloud above the region of interest (ROI). We selected the mean annual images to evaluate the yearly SCA variations. Next, we pulled the shapefile of Astore and Shigar which was used to mask the satellite images. Eventually, using the JavaScript code in GEE, we performed NDSI analysis on the masked images from 1991 to 2021, to extract the snow cover area for Astore and Shigar. To evaluate the spatiotemporal variation in SCA, snow and non-snow pixels were segregated using NDSI; usually, a threshold of 0.4 was used which is suitable for classifying the snow and non-snow pixels even in mountain-shadowed areas (Burns and Nolin, 2014). Then, we reclassified the satellite image into the snow and non-snow pixels, and eventually the year-wise SCA was calculated using snow pixels as follows:
We acquired the rainfall and temperature data from the climate research unit (CRU) for the study area with a spatial resolution of 0.5o for the period of 1991–2021. We correlated the SCA with rainfall and temperature on an annual basis to evaluate the impact of climate change on SCA.
We also utilized the Advanced Spaceborne Thermal Emission and Reflection Radiometer (ASTER) GDEM (30 m) to extract the topographic parameters, i.e., elevation in GEE. NDSI classified images, and the topographical parameter was exported for the region of interest (Astore and Shigar) for further analysis in ArcGIS software. In ArcGIS software, we reclassified the NDSI-classified images and calculated the area covered by snow for the study.
Afterward, the elevation was compared with NDSI-reclassified images of Astore and Shigar to calculate the area covered by snow on each elevation zone. Eventually, we prepared the tables, maps, and graphs. The detailed methodological framework of this study is presented in Figure 3.
Landsat data
In this study, Landsat data were acquired from 1991 to 2021 (mean annual images) for the study area because Landsat data are freely available (Patel et al., 2019). Landsat has the longest time series data with a multi-spectral and spatial resolution to evaluate the snow cover (Rastner et al., 2019). All Landsat satellites complete the circle around the Earth in 16 days; however, their characteristics vary (Table 1). In this study, we used Landsat 5 TM, Landsat 7 ETM, and Landsat 8 OLI data.
ASTER data
To evaluate the SCA with topographic parameters, it is necessary to use the digital elevation model (DEM) because elevation, slope, and aspect can be calculated from DEM. Therefore, we used ASTER GDEM v3 data to calculate the elevation and slope for further evaluation of SCA in this study. ASTER DEM has a 30-m spatial resolution. The version 3 data of ASTER has 1.8 million stereo pairs with improved horizontal and vertical accuracy in comparison with the previous version (Meetei et al., 2022). The elevation of Astore and Shigar was divided into four zones (Table 2) to calculate the elevation-wise SCA using the zonal statistics tool in the model builder of ArcGIS.
Climate research unit data (rainfall and temperature)
In this study, we also used climatic data (rainfall and temperature). Due to the scarcity of meteorological station data in this region, therefore, alternatively, we used the CRU v4.05 dataset which was released on 17th March 2021 (Harris et al., 2021) because the CRU dataset achieved better accuracy in the HKH region and it can accurately acquire the data in this region (Kanda et al., 2020). Another study also suggested that CRU and ERA-I datasets perform better, and both datasets precisely capture the spatial distribution of temperature and precipitation in the Greater Himalayas, Northwest Himalayas, and Karakoram region (Negi and Kanda, 2020). CRU data was developed by the climate research unit of the University of East Anglia at the NERC collaborative center using the angular distance weighting method at 0.5o resolution. In this study, we acquired the CRU data [(https://crudata.uea.ac.uk/cru/data/hrg/cru_ts_4.05/ge/) (accessed on 15 May 2022)] between 1991 and 2021 (Harris et al., 2020; Harris et al., 2021). The climatic data were used to estimate the influence of changing climate on SCA.
Results and discussion
Annual snow cover area
In this study, the SCA for Astore and Shigar was computed through Landsat data between 1991 and 2021 in GEE. The mean annual SCA was calculated for both regions as presented in Figures 4A,B. It was observed that SCA varies greatly in both regions due to high variability in precipitation and temperature (Khan et al., 2014; Tahir et al., 2015). Astore SCA varies between 36.7% (1998) and 92.7% (1991) (Supplementary Figures S1,S2), while in Shigar it varies between 47.4% (1997) and 89.18% (2019) (Supplementary Figures S3,S4). A huge variation of SCA can be reflected through the high discharge of Astore River and Shigar River basins. Hakeem et al. (2014) revealed that Shigar River basin runoff is mainly contributed by seasonal and annual SCA. It was observed that Astore and Shigar have an increasing trend of SCA with a rate of 11.16 km2/year and 4.27 km2/year, respectively. Tahir et al. (2015) and Tahir et al. (2016) also revealed an increasing/stable trend of SCA in the Western Himalayas and Central Karakoram region. River basins in the UIB are highly dependent on SCA and glaciers; therefore, this increasing trend of SCA can highly contribute to the Astore River and Shigar River in the future. The increasing trend of precipitation and decreasing trend of temperature feed the mountainous region and reduce the snow melt resulting in a stable/increasing trend of SCA. The results of this study are similar to Gilgit River and Hunza River basins.
It was also observed that the annual SCA was decreasing during 1991–2005 (−38.04 km2/year) in Astore because the temperature was increasing and precipitation was decreasing, while, in the latter part of the study (2006–2021), the results revealed that SCA was increasing (25.76 km2/year) because the temperature was decreasing and precipitation was increasing. It was observed in Shigar that in both periods annual SCA was increasing because the precipitation has an increasing trend and the temperature has a decreasing trend. A similar increasing trend in precipitation and decreasing trend in temperature was observed by Tahir et al. (2015) and Tahir et al. (2016).
Topographic parameter and snow cover area
Topographic parameters, i.e., elevation, slope, and aspect play a significant role in snow cover changes (Li and Li, 2014; Bhambri et al., 2017) because the process of accumulation and melting can be controlled by topography and atmospheric conditions. The air and surface temperatures are increasing at higher altitudes which can significantly affect the SCA (Huang et al., 2017). Many researchers studied elevation-dependent warming and concluded that it will have a significant impact on hydrological cycles due to the huge and early snow melt process (Pepin et al., 2015; Thakuri et al., 2019). Therefore, in this study, we correlated elevation and SCA. It was observed that elevation zone 1 covers 7.83% of the total area of Astore, and usually, in this zone, the precipitation falls in the form of rainfall; hence, on average, this zone covers 0.9% of SCA. The temperature of this zone varies from −8.07 C in January to 12.50 C in August. The snow accumulation period of this zone is comparatively shorter; consequently, this zone occupies only a small portion of SCA (Figure 5A); on contrary, zone 4 of the elevation class covers 21.06% of the total area which is mainly covered with glaciers above the 5,000 mean sea level (m.s.l) (Figure 5C). The areal extent of permanent SCA is mostly situated at zone 4 because at higher altitudes the precipitation falls in the form of snowfall and the temperature of this zone varies from −10.70 C in January to 11.50 C in August. In this zone, the below-freezing temperature remains until April, and the snow melt period becomes shorter. Therefore, zone 4 has weaker snow cover variations (S = 0.03%/year, tau’s = 0.97) (Figure 6A) (Tahir et al., 2015; Misra et al., 2020). Zone 4 of the elevation class covered 27.4% of SCA (Figure 5A). Zone 2 and zone 3 of the elevation class cover a large portion in Astore; similarly, they cover 21% and 50.7% of SCA, but we observed high year-to-year variation in these zones because these zones receive a large amount of precipitation in the form of snow during the accumulation season, but in the melt season the precipitation falls in the form of rain and heavy rainfall in the monsoon season (rain on snow), leading to rapid melting of snow cover. Another reason for the decreasing trend of SCA is that SCA is highly exposed to high temperatures (S = −0.83 and -0.71%/year; tau’s = 0.86 and 0.77). Particularly, in this study, we observed that zone 2 and zone 3 have an increasing trend of temperature and decreasing trend of rainfall, which is the reason for decreasing trend of SCA (Supplementary Table S4). Annual SCA at mid-altitude zones decreases but insignificantly which is closely related to the elevation-dependent temperature change as compared to precipitation (Misra et al., 2020; Shen et al., 2021) (Supplementary Table S4).
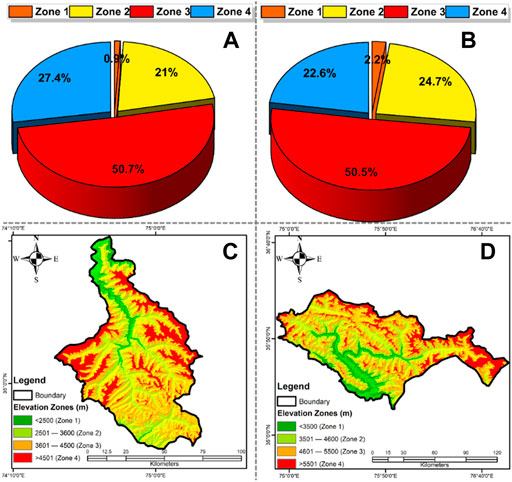
FIGURE 5. Elevation-zone wise mean SCA (1991–2021) of (A) Astore, (B) Shigar, (C) Astore elevation zones, (D) Shigar elevation zones.
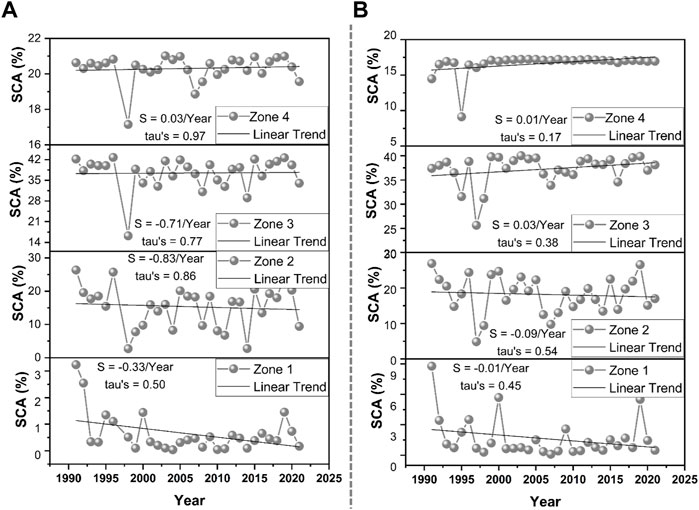
FIGURE 6. Mann–Kendall trend of annual SCA derived from Landsat data in four elevation zones of (A) Astore and (B) Shigar over a 31-year period (1991–2021). Kendall tau, “tau’s”; Sen’s slope, “S.”
Many previous research studies suggested that snow accumulation and snow melt were significantly based on the topography, latitude, and weather conditions of the area (Woo and Thorne, 2006; Jain et al., 2009; Kour et al., 2016). Therefore, the elevation of Shigar was correlated with SCA in this research to examine its impact. The valley floor (zone 1) and high altitude (zone 4) of Shigar cover an area of 12.39% and 17.23%, respectively. At high altitudes, snow is the main form of precipitation and represents a glaciated area, while the valley floor receives precipitation in the form of rainfall. The temperature of zone 1 remains below the freezing point for 5 months in Shigar, while at high-altitude zone 4 the freezing temperature remains for 7 months, which represents the snow accumulation and snow melting period and variations of SCA in zone 1 and zone 4. At high altitudes, less temperature can be examined with a high amount of precipitation which can turn it into snow accumulation (Supplementary Table S4). Subsequently, elevation with climate variables greatly affects the snow cover distribution (Jain et al., 2009). The mid-altitude zones cover 70% of the total area and occupy 75.2% of SCA. This huge portion of SCA at mid-altitude is sensitive and exposed to high temperature in the melt season resulting in high discharge in the river and also presents high SCA variations (Hasson et al., 2014a; Hakeem et al., 2014) (S = −0.09 and 0.03%/year; tau’s 0.54 and 0.38) (Figure 6B).
Trends in annual SCA were computed for a period of 31 years in four elevation zones of Astore and Shigar (Table 2; Figure 6). Low-altitude zones of both regions revealed a decreasing trend, but the trend was insignificant, while, at high-altitude zones of Astore and Shigar, the trend was insignificantly positive (Figure 6; Supplementary Tables S4,S5). The tau values at high elevation for Astore and Shigar were 0.97 and 0.17, while S-values were 0.03% and 0.01%/year, respectively (Figures 6A,B). On contrary, the tau values for mid-altitude zones of Astore were 0.86 and 0.77, while S-values were −0.83% and −0.71%/year (Figure 6A; Supplementary Table S4). Similarly, the tau values of Shigar at mid-altitude were 0.54 and 0.38, while S-values were −0.09% and 0.03%/year. (Figure 6B; Supplementary Table S5).
Climate variables and snow cover area
For a better interpretation of the snow cover area, we compared SCA with climate variables (temperature and precipitation) for the period of 1991–2021. The rainfall and temperature patterns were examined to see the response of SCA to the changing climate. The mean annual temperature and rainfall of Astore and Shigar were computed, and it was revealed that the mean annual temperature of Astore and Shigar was decreasing, while precipitation was increasing in both regions (Figures 7, 8). A linear trend value for Astore (Shigar) was 0.025 mm/year (0.028 mm/year) for precipitation and −0.003 °C/year (−0.017 °C/year) for temperature was found over the studied period (1991–2021) (Figures 7, 8). The increasing and decreasing trends of precipitation and temperature indicate the reason for the stable/increasing trend of SCA because the increasing trend of precipitation feeds the mountainous area and influences the SCA.
In this study, we analyzed the seasonal trend of temperature and precipitation to understand which climatic variable led to an increasing or stable trend of SCA in Astore and Shigar. Long-term (1991–2021) precipitation and temperature data were used to compute the trend. The results revealed that winter precipitation in Astore have an insignificant positive trend (tau’s = 0.84; S = 0.04 mm/year); however, the summer season has an insignificant negative trend of precipitation (tau’s = 0.61; −0.097 mm/year) (Table 3). While it was noticed that the summer season temperature has an insignificant decreasing trend, the winter season temperature has an increasing trend. The increasing and decreasing trends of precipitation and temperature in both seasons can be a possible reason for the stable/increasing trend of SCA in Astore because winter precipitation is increasing which can bring more snowfall in the region and summer season temperature is decreasing which restricts snow melt (Archer and Fowler, 2004; Hewitt, 2007). The increasing trend of precipitation in Asia was also reported by Hartmann et al. (2013). Recently, Moazzam et al. (2022) also revealed that precipitation will increase in the northern region of Pakistan (Gilgit-Baltistan) under representative concentration pathway (RCP) 4.5 and RCP 8.5 (Moazzam et al., 2022).

TABLE 3. MK trend analysis of climate variables of Astore for summer and winter seasons for the period of 1991–2021.
In contrast, it was observed that, in Shigar, precipitation is increasing in winter (tau’s = 0.86; S = 0.034 mm/year) and summer seasons (tau’s = 0.66; S = 0.019 mm/year), while temperature is also increasing in both seasons (Table 4). The increasing trend of SCA in Shigar may be influenced by the increased winter precipitation due to the western disturbance (Ahmad et al., 2019). A recent book published by ICIMOD also reported an increasing trend in winter precipitation (Krishnan et al., 2019). The increasing probability of western disturbance-induced rainfall and snowfall in the winter season will impact an increase in SCA (Midhuna et al., 2020).

TABLE 4. MK trend analysis of climate variables of Shigar for summer and winter seasons for the period of 1991–2021.
Discussion
In this study, we analyzed the snow cover changes of Astore and Shigar using Landsat data in the Google Earth Engine (GEE) platform for the period of 1991–2021. The normalized difference snow index (NDSI) was used in this study for the extraction of snow cover because in previous studies (Burns and Nolin, 2014; Shafique et al., 2018; Tong et al., 2020) NDSI revealed a better accuracy in shadowed and mountainous regions. In this study, it was found that the snow cover area is increasing in Astore and Shigar which is in line with some previous studies (Tahir et al., 2015; Tahir et al., 2016; Anjum et al., 2019; Shafiq et al., 2019). The increasing trend of SCA nourishes the glaciers and makes a positive contribution to the mass balance of glaciers in the Karakoram region which was reported previously (Scherler et al., 2011; Nüsser and Schmidt, 2021; Bhambri et al., 2022), not like other mountainous regions around the globe because the temperature is decreasing and precipitation is increasing in this region. Similarly, IPCC Fifth Assessment Report indicated an increase in SCA due to a positive trend of precipitation (Hartmann et al., 2013) which is also supported by another study that the frequency and occurrence of westerlies-induced precipitation will increase up to 2,100 (Ridley et al., 2013). Moazzam et al. (2022) also revealed an increasing trend of precipitation until mid of this century. The cooling phenomena of the summer season are also a reason for the increasing trend of SCA (Fowler and Archer, 2006). The increasing trend of SCA in the Astore region is unexpected because previous studies reported a decreasing trend of glacier mass balance in the Himalayan region (Ali et al., 2015; Bajracharya et al., 2015; Hayat et al., 2019). Astore is located in the extreme Western Himalayan region and has a different climate from the Eastern Himalayan region and receives westerlies-induced precipitation due to the vicinity of the southern Karakoram region; hence, the winter precipitation of Astore is somewhat similar to Shigar precipitation (Tables 3, 4). Therefore, the climate variability trend plays a significant role in the increasing or decreasing trend of SCA in the Central Karakoram and Western Himalayas region (Tahir et al., 2015). A seasonal analysis was performed for the winter and summer seasons’ precipitation and temperature of Astore and Shigar (Figure 7). The precipitation and temperature (winter) of Astore have an increasing trend but not significantly, while in the summer season both temperature and precipitation have a decreasing trend. The results of this study support a study conducted by Archer and Fowler, (2004) who explained an insignificant decreasing trend in the summer season, while in Shigar it was noted that precipitation and temperature in winter and summer seasons have an insignificant increasing trend. The increasing trend of winter temperature still persists negatively in high-elevation zones (Tahir et al., 2016). Archer and Fowler, (2004) stated an increasing trend of summer and winter precipitation in the Upper Indus Basin. The increasing trend of SCA in Astore is clearer than that in Shigar (Tables 3, 4) because summer temperature in Astore has a decreasing trend [ tau’s = 0.79; S = −0.033 (oC/year)]. Fowler and Archer, (2006) stated that the summer season’s temperature plays a key role in SCA and glacier melt; another study also stated that the pre-monsoon and monsoon temperature of the high mountain area is decreasing (Hussain et al., 2005). The elevation and SCA were also analyzed, and it was found that zone 1 of both regions has a decreasing trend (Qureshi et al., 2017; Ali et al., 2021), while zone 4 has a slightly increasing trend of SCA because the high-altitude region is less exposed to solar radiation as compared to foothills (Saydi and Ding, 2020). The higher altitude regions have consistently below-freezing temperatures throughout the year; therefore, increasing precipitation can possibly feed the high-altitude region, and hence SCA expands (Tahir et al., 2011; Hasson et al., 2014b). Worldwide glaciers are decreasing due to an ongoing climate change prognosis, whereas in some valleys of Karakoram and Himalayan regions its increase is a good sign which has been previously noted in a number of studies under the title of the Karakoram anomaly (Hewitt, 2005; Farinotti et al., 2020; Dimri, 2021). The glacier of the study region feeds the flow in the Indus River, which is a resource and sometimes becomes a hazard for 220 million people in low-lying areas. The increase in glacial mass has also increased river flow in Shigar and Astore rivers (Khalida et al., 2015) which is also a positive sign for the low-lying agricultural land.
Conclusion
Spatial and temporal SCA changes have been calculated using Landsat data with the GEE platform and analyzed with climate variables (rainfall and temperature) and elevation for the Astore and Shigar regions. The results of this study have been evaluated, and it is concluded that SCA in both regions has an increasing trend due to the increasing trend of precipitation and decreasing trend of temperature in the studied period. The results also indicated that the SCA was decreasing (−38.04 km2/year) during 1991–2005 in the Astore region, while in the same period Shigar had an increasing trend. In the latter part of the study (2006–2021), the results revealed an increasing trend of SCA in both regions. The annual SCA varies greatly due to the influence of rainfall and temperature. The minimum annual SCA was observed in the years 1998 (36.7%) and 1997 (47.4%) in Astore and Shigar, while maximum SCA was observed in the years 1991 (92.7%) and 2019 (89.18%) in Astore and Shigar, respectively. A thorough investigation of SCA and elevation revealed that the lowest and highest altitudes have insignificant decreasing and increasing trends of SCA in both regions. SCA and climate variables indicated that precipitation is increasing and temperature is decreasing with time which is a possible reason for the increasing trend of SCA in both regions. The increasing SCA is a positive sign of water resources for the future and agricultural activities in the study region as well as the region relying on water resources from Astore and Shigar rivers. The increasing precipitation, especially decreasing temperature in the study region is in contrast to the current global climate warming, and this needs to be further investigated at the microlevel. This study can help improve the knowledge and understanding of water resource management.
Data availability statement
The original contributions presented in the study are included in the article/Supplementary Material; further inquiries can be directed to the corresponding author.
Author contributions
MM and BL: conceptualization; MM: acquired the data, data curation, formal analysis, and investigation; MM and GR: methodology, validation, visualization, and writing—original draft; BL: supervision, project administration, resources, and writing—review and editing; and NA: acquired funding and writing—review and editing.
Acknowledgments
The author thanks the United States Geological Survey (USGS) and the National Aeronautics and Space Administration (NASA) for providing the Landsat data. The authors are thankful to NASA and the Ministry of Economy, Trade, and Industry (METI) for providing ASTER DEM free of cost for this study.
Conflict of interest
The authors declare that the research was conducted in the absence of any commercial or financial relationships that could be construed as a potential conflict of interest.
Publisher’s note
All claims expressed in this article are solely those of the authors and do not necessarily represent those of their affiliated organizations, or those of the publisher, the editors, and the reviewers. Any product that may be evaluated in this article, or claim that may be made by its manufacturer, is not guaranteed or endorsed by the publisher.
Supplementary material
The Supplementary Material for this article can be found online at: https://www.frontiersin.org/articles/10.3389/fenvs.2022.1006399/full#supplementary-material
References
Abid, Z., and Zia, S. (2019). Mapping and analysis of Baltoto glacier and Shigar river. Pak. Geogr. Rev. 74, 20–32.
Ahmad, M., Alam, K., Tariq, S., and Blaschke, T. (2019). Contrasting changes in snow cover and its sensitivity to aerosol optical properties in Hindukush-Karakoram-Himalaya region. Sci. Total Environ. 699, 134356. doi:10.1016/j.scitotenv.2019.134356
Ali, G., Hasson, S., and Khan, A. M. (2009). Global change impact studies centre (GCISC). Islamabad, Pakistan.Climate change: Implications and adaptation of water resources in Pakistan
Ali, S., Khan, G., Hassan, W., Qureshi, J. A., and Bano, I. (2021). Assessment of glacier status and its controlling parameters from 1990 to 2018 of Hunza basin, western karakorum. Heidelberg: Environmental Science and Pollution Research, 1–13.
Ali, S., Li, D., Congbin, F., and Khan, F. (2015). Twenty first century climatic and hydrological changes over Upper Indus Basin of Himalayan region of Pakistan. Environ. Res. Lett. 10, 014007. doi:10.1088/1748-9326/10/1/014007
Aniya, M., Sato, H., Naruse, R., Skvarca, P., and Casassa, G. (1996). Patagonia lcefield, south America. Photogrammetric Eng. Remote Sens. 62, 1361–1369.
Anjum, M. N., Ding, Y., Shangguan, D., Liu, J., Ahmad, I., Ijaz, M. W., et al. (2019). Quantification of spatial temporal variability of snow cover and hydro-climatic variables based on multi-source remote sensing data in the Swat watershed, Hindukush Mountains, Pakistan. Meteorol. Atmos. Phys. 131, 467–486. doi:10.1007/s00703-018-0584-7
Archer, D. R., and Fowler, H. J. (2004). Spatial and temporal variations in precipitation in the Upper Indus Basin, global teleconnections and hydrological implications. Hydrol. Earth Syst. Sci. 8, 47–61. doi:10.5194/hess-8-47-2004
Azizi, A. H., and Akhtar, F. (2021). Analysis of spatiotemporal variation in the snow cover in Western Hindukush-Himalaya region. Geocarto Int., 1–23. doi:10.1080/10106049.2021.1939442
Bajracharya, S. R., Maharjan, S. B., Shrestha, F., Guo, W., Liu, S., Immerzeel, W., et al. (2015). The glaciers of the Hindu Kush Himalayas: Current status and observed changes from the 1980s to 2010. Int. J. Water Resour. Dev. 31, 161–173. doi:10.1080/07900627.2015.1005731
Banerjee, A., Chen, R., Meadows, M. E., Sengupta, D., Pathak, S., Xia, Z., et al. (2021). Tracking 21st century climate dynamics of the Third Pole: An analysis of topo-climate impacts on snow cover in the central Himalaya using Google Earth Engine. Int. J. Appl. Earth Observation Geoinformation 103, 102490. doi:10.1016/j.jag.2021.102490
Bhambri, R., Bolch, T., Chaujar, R. K., and Kulshreshtha, S. C. (2017). Glacier changes in the Garhwal Himalaya, India, from 1968 to 2006 based on remote sensing. J. Glaciol. 57, 543–556. doi:10.3189/002214311796905604
Bhambri, R., Hewitt, K., Haritashya, U. K., Chand, P., Kumar, A., Verma, A., et al. (2022). Characteristics of surge-type tributary glaciers, Karakoram. Geomorphology 403, 108161. doi:10.1016/j.geomorph.2022.108161
Bishop, M. P., Bush, A. B., Copland, L., Kamp, U., Owen, L. A., Seong, Y. B., et al. (2010). Climate change and mountain topographic evolution in the central Karakoram, Pakistan. Ann. Assoc. Am. Geogr. 100, 772–793. doi:10.1080/00045608.2010.500521
Bookhagen, B., and Burbank, D. W. (2010). Toward a complete Himalayan hydrological budget: Spatiotemporal distribution of snowmelt and rainfall and their impact on river discharge. J. Geophys. Res. 115, F03019. doi:10.1029/2009jf001426
Burns, P., and Nolin, A. (2014). Using atmospherically-corrected Landsat imagery to measure glacier area change in the Cordillera Blanca, Peru from 1987 to 2010. Remote Sens. Environ. 140, 165–178. doi:10.1016/j.rse.2013.08.026
Dimri, A. (2021). Decoding the Karakoram anomaly. Sci. Total Environ. 788, 1–13. doi:10.1016/j.scitotenv.2021.147864
Farhan, S. B., Zhang, Y., Aziz, A., Gao, H., Ma, Y., Kazmi, J., et al. (2020). Assessing the impacts of climate change on the high altitude snow-and glacier-fed hydrological regimes of Astore and Hunza, the sub-catchments of Upper Indus Basin. J. Water Clim. Change 11, 479–490. doi:10.2166/wcc.2018.107
Farhan, S. B., Zhang, Y., Ma, Y., Guo, Y., and Ma, N. (2015). Hydrological regimes under the conjunction of westerly and monsoon climates: A case investigation in the Astore basin, northwestern Himalaya. Clim. Dyn. 44, 3015–3032. doi:10.1007/s00382-014-2409-9
Farinotti, D., Immerzeel, W. W., De Kok, R. J., Quincey, D. J., and Dehecq, A. (2020). Manifestations and mechanisms of the Karakoram glacier anomaly. Nat. Geosci. 13, 8–16. doi:10.1038/s41561-019-0513-5
Fowler, H., and Archer, D. (2006). Conflicting signals of climatic change in the upper Indus Basin. J. Clim. 19, 4276–4293. doi:10.1175/jcli3860.1
Fowler, H., and Archer, D. (2005). Hydro-climatological variability in the Upper Indus Basin and implications for water resources. Regional Hydrological Impacts Clim. Change—Impact Assess. Decis. Mak. 295, 131–138.
Gorelick, N., Hancher, M., Dixon, M., Ilyushchenko, S., Thau, D., and Moore, R. (2017). Google earth engine: Planetary-scale geospatial analysis for everyone. Remote Sens. Environ. 202, 18–27. doi:10.1016/j.rse.2017.06.031
Hakeem, S. A., Bilal, M., Pervez, A., and Tahir, A. A. (2014). Remote sensing data application to monitor snow cover variation and hydrological regime in a poorly gauged river catchment—northern Pakistan. Int. J. Geosciences 5, 27–37. doi:10.4236/ijg.2014.51005
Harris, I., Jones, P., and Osborn, T. (2021). in Cru TS4.05: Climatic Research Unit (CRU) Time-Series (TS) version 4.05 of high-resolution gridded data of month-by-month variation in climate (Jan. 1901- Dec. 2020). Editors N. E. C. F. E. D. ANALYSIS, and C. R. U. CRU).
Harris, I., Osborn, T. J., Jones, P., and Lister, D. (2020). Version 4 of the CRU TS monthly high-resolution gridded multivariate climate dataset. Sci. Data 7, 109. doi:10.1038/s41597-020-0453-3
Hartmann, D. L., Tank, A. M. K., Rusticucci, M., Alexander, L. V., Brönnimann, S., Charabi, Y. A. R., et al. (2013). Climate change 2013 the physical science basis: Working group I contribution to the fifth assessment report of the intergovernmental panel on climate change. Cambridge University Press.Observations: Atmosphere and surface
Hasson, S., Lucarini, V., Khan, M. R., Petitta, M., Bolch, T., and Gioli, G. (2014a). Early 21st century snow cover state over the Western river basins of the Indus River system. Hydrol. Earth Syst. Sci. 18, 4077–4100. doi:10.5194/hess-18-4077-2014
Hasson, S., Lucarini, V., Pascale, S., and Böhner, J. (2014b). Seasonality of the hydrological cycle in major South and Southeast Asian river basins as simulated by PCMDI/CMIP3 experiments. Earth Syst. Dyn. 5, 67–87. doi:10.5194/esd-5-67-2014
Hayat, H., Akbar, T. A., Tahir, A. A., Hassan, Q. K., Dewan, A., and Irshad, M. (2019). Simulating current and future river-flows in the Karakoram and Himalayan regions of Pakistan using snowmelt-runoff model and RCP scenarios. Water 11, 761. doi:10.3390/w11040761
Hewitt, K. (2005). The Karakoram anomaly? Glacier expansion and the ‘elevation effect, ’Karakoram Himalaya. Mt. Res. Dev. 25, 332–340. doi:10.1659/0276-4741(2005)025[0332:tkagea]2.0.co;2
Hewitt, K. (2007). Tributary glacier surges: An exceptional concentration at panmah glacier, Karakoram Himalaya. J. Glaciol. 53, 181–188. doi:10.3189/172756507782202829
Hori, M., Sugiura, K., Kobayashi, K., Aoki, T., Tanikawa, T., Kuchiki, K., et al. (2017). A 38-year (1978–2015) Northern Hemisphere daily snow cover extent product derived using consistent objective criteria from satellite-borne optical sensors. Remote Sens. Environ. 191, 402–418. doi:10.1016/j.rse.2017.01.023
Huang, X., Deng, J., Wang, W., Feng, Q., and Liang, T. (2017). Impact of climate and elevation on snow cover using integrated remote sensing snow products in Tibetan Plateau. Remote Sens. Environ. 190, 274–288. doi:10.1016/j.rse.2016.12.028
Hussain, S. S., Mudasser, M., Sheikh, M., and Manzoor, N. (2005). Climate change and variability in mountain regions of Pakistan implications for water and agriculture. Pak. J. Meteorology 2.
ICIMOD (2005). Inventory of glaciers and glaciers lakes andIdentification of potential glacial LakeOutburst floods (GLOFs) affected by GlobalWarming in the mountains of HimalayanRegion Pakistan.
Immerzeel, W. W., Van Beek, L., Konz, M., Shrestha, A., and Bierkens, M. (2012). Hydrological response to climate change in a glacierized catchment in the Himalayas. Clim. change 110, 721–736. doi:10.1007/s10584-011-0143-4
Jacobsen, F. M., and Theakstone, W. H. (1997). Monitoring glacier changes using a global positioning system in differential mode. Ann. Glaciol. 24, 314–319. doi:10.1017/s0260305500012374
Jain, S. K., Goswami, A., and Saraf, A. K. (2009). Role of elevation and aspect in snow distribution in western Himalaya. Water Resour. manage. 23, 71–83. doi:10.1007/s11269-008-9265-5
Kanda, N., Negi, H. S., Rishi, M. S., and Kumar, A. (2020). Performance of various gridded temperature and precipitation datasets over Northwest Himalayan Region. Environ. Res. Commun. 2, 085002. doi:10.1088/2515-7620/ab9991
Kehrwald, N. M., Thompson, L. G., Tandong, Y., Mosley‐Thompson, E., Schotterer, U., Alfimov, V., et al. (2008). Mass loss on Himalayan glacier endangers water resources. Geophys. Res. Lett. 35, L22503. doi:10.1029/2008gl035556
Khalida, K., Muhammad, Y., Yasir, L., and Ghulam, N. (2015). Detection of river flow trends and variability analysis of Upper Indus Basin, Pakistan. Sci. Int. (Lahore) 27, 1261–1270.
Khan, S., Ashiq, M., and Gabriel, H. (2014). Assessment of flows in a glaciated region-Shigar river basin, Pakistan. Taxila: Technical Journal, University of Engineering and Technology Taxila, 19.
Kour, R., Patel, N., and Krishna, A. P. (2016). Effects of terrain attributes on snow-cover dynamics in parts of Chenab basin, Western Himalayas. Hydrological Sci. J. 61, 1859–1876. doi:10.1080/02626667.2015.1052815
Krishnan, R., Shrestha, A. B., Ren, G., Rajbhandari, R., Saeed, S., Sanjay, J., et al. (2019). “Unravelling climate change in the Hindu Kush Himalaya: Rapid warming in the mountains and increasing extremes,” in The Hindu Kush Himalaya assessment: Mountains, climate change, sustainability and people. Editors P. WESTER, A. MISHRA, A. MUKHERJI, and A. B. SHRESTHA (Cham: Springer International Publishing).
Li, Y., and Li, Y. (2014). Topographic and geometric controls on glacier changes in the central Tien Shan, China, since the Little Ice Age. Ann. Glaciol. 55, 177–186. doi:10.3189/2014aog66a031
Meetei, P. N., Ahluwalia, R. S., Rai, S., Khobragade, S., Sarangi, S., Goel, M., et al. (2022). Spatio-temporal analysis of snow cover and effect of terrain attributes in the Upper Ganga River Basin, central Himalaya. Geocarto Int. 37, 1139–1159. doi:10.1080/10106049.2020.1762764
Midhuna, T. M., Kumar, P., and Dimri, A. P. (2020). A new Western Disturbance Index for the Indian winter monsoon. J. Earth Syst. Sci. 129, 59. doi:10.1007/s12040-019-1324-1
Misra, A., Kumar, A., Bhambri, R., Haritashya, U. K., Verma, A., Dobhal, D. P., et al. (2020). Topographic and climatic influence on seasonal snow cover: Implications for the hydrology of ungauged Himalayan basins, India. J. Hydrology 585, 124716. doi:10.1016/j.jhydrol.2020.124716
Moazzam, M. F. U., Rahman, G., Munawar, S., Tariq, A., Safdar, Q., and Lee, B.-G. (2022). Trends of rainfall variability and drought monitoring using standardized precipitation index in a scarcely gauged basin of northern Pakistan. Water 14, 1132. doi:10.3390/w14071132
Negi, H., and Kanda, N. (2020). Climate change and the white world. Springer.An appraisal of spatio-temporal characteristics of temperature and precipitation using gridded datasets over NW-Himalaya
Notarnicola, C. (2020). Hotspots of snow cover changes in global mountain regions over 2000–2018. Remote Sens. Environ. 243, 111781. doi:10.1016/j.rse.2020.111781
Nüsser, M., and Schmidt, S. (2021). Glacier changes on the Nanga Parbat 1856–2020: A multi-source retrospective analysis. Sci. Total Environ. 785, 147321. doi:10.1016/j.scitotenv.2021.147321
Panday, P. K., Thibeault, J., and Frey, K. E. (2015). Changing temperature and precipitation extremes in the Hindu Kush‐Himalayan region: An analysis of CMIP3 and CMIP5 simulations and projections. Int. J. Climatol. 35, 3058–3077. doi:10.1002/joc.4192
Patel, A., Prajapati, R., Dharpure, J. K., Mani, S., and Chauhan, D. (2019). Mapping and monitoring of glacier areal changes using multispectral and elevation data: A case study over chhota-shigri glacier. Earth Sci. Inf. 12, 489–499. doi:10.1007/s12145-019-00388-x
Pepin, N., Bradley, R. S., Diaz, H., Baraër, M., Caceres, E., Forsythe, N., et al. (2015). Elevation-dependent warming in mountain regions of the world. Nat. Clim. Chang. 5, 424–430. doi:10.1038/nclimate2563
Qureshi, M. A., Yi, C., Xu, X., and Li, Y. (2017). Glacier status during the period 1973–2014 in the Hunza basin, western Karakoram. Quat. Int. 444, 125–136. doi:10.1016/j.quaint.2016.08.029
Rastner, P., Prinz, R., Notarnicola, C., Nicholson, L., Sailer, R., Schwaizer, G., et al. (2019). On the automated mapping of snow cover on glaciers and calculation of snow line altitudes from multi-temporal landsat data. Remote Sens. 11, 1410. doi:10.3390/rs11121410
RGI (2017). A dataset of global glacier outlines: Version 6.0: Technical report, global land ice measurements from Space. Colorado, USA.
Ridley, J., Wiltshire, A., and Mathison, C. (2013). More frequent occurrence of westerly disturbances in Karakoram up to 2100. Sci. Total Environ. 468, S31–S35. doi:10.1016/j.scitotenv.2013.03.074
Sahu, R., and Gupta, R. (2020). Snow cover area analysis and its relation with climate variability in Chandra basin, Western Himalaya, during 2001–2017 using MODIS and ERA5 data. Environ. Monit. Assess. 192, 1–26. doi:10.1007/s10661-020-08442-8
Saydi, M., and Ding, J.-L. (2020). Impacts of topographic factors on regional snow cover characteristics. Water Sci. Eng. 13, 171–180. doi:10.1016/j.wse.2020.09.002
Scherler, D., Bookhagen, B., and Strecker, M. R. (2011). Spatially variable response of Himalayan glaciers to climate change affected by debris cover. Nat. Geosci. 4, 156–159. doi:10.1038/ngeo1068
Shafiq, M. U., Ahmed, P., Islam, Z. U., Joshi, P. K., and Bhat, W. A. (2019). Snow cover area change and its relations with climatic variability in Kashmir Himalayas, India. Geocarto Int. 34, 688–702. doi:10.1080/10106049.2018.1469675
Shafique, M., Faiz, B., Bacha, A. S., and Ullah, S. (2018). Evaluating glacier dynamics using temporal remote sensing images: A case study of Hunza valley, northern Pakistan. Environ. Earth Sci. 77, 162–211. doi:10.1007/s12665-018-7365-y
Sharma, V., Mishra, V. D., and Joshi, P. K. (2013). Implications of climate change on streamflow of a snow-fed river system of the Northwest Himalaya. J. Mt. Sci. 10, 574–587. doi:10.1007/s11629-013-2667-8
Shen, L., Zhang, Y., Ullah, S., Pepin, N., and Ma, Q. (2021). Changes in snow depth under elevation‐dependent warming over the Tibetan Plateau. Atmos. Sci. Lett. 22, e1041. doi:10.1002/asl.1041
SIHP (1990). Snow and ice hydrology project, upper Indus river basin. Lahore: WAPDA-IDRC-Wilfrid Laurier University.
Tahir, A. A., Adamowski, J. F., Chevallier, P., Haq, A. U., and Terzago, S. (2016). Comparative assessment of spatiotemporal snow cover changes and hydrological behavior of the Gilgit, Astore and Hunza River basins (Hindukush–Karakoram–Himalaya region, Pakistan). Meteorol. Atmos. Phys. 128, 793–811. doi:10.1007/s00703-016-0440-6
Tahir, A. A., Chevallier, P., Arnaud, Y., and Ahmad, B. (2011). Snow cover dynamics and hydrological regime of the Hunza river basin, Karakoram range, northern Pakistan. Hydrol. Earth Syst. Sci. 15, 2275–2290. doi:10.5194/hess-15-2275-2011
Tahir, A. A., Chevallier, P., Arnaud, Y., Ashraf, M., and Bhatti, M. T. (2015). Snow cover trend and hydrological characteristics of the Astore River basin (Western Himalayas) and its comparison to the Hunza basin (Karakoram region). Sci. total Environ. 505, 748–761. doi:10.1016/j.scitotenv.2014.10.065
Tahir, A. A., Hakeem, S. A., Hu, T., Hayat, H., and Yasir, M. (2019). Simulation of snowmelt-runoff under climate change scenarios in a data-scarce mountain environment. Int. J. Digital Earth 12, 910–930. doi:10.1080/17538947.2017.1371254
Thakuri, S., Dahal, S., Shrestha, D., Guyennon, N., Romano, E., Colombo, N., et al. (2019). Elevation-dependent warming of maximum air temperature in Nepal during 1976–2015. Atmos. Res. 228, 261–269. doi:10.1016/j.atmosres.2019.06.006
Tong, R., Parajka, J., Komma, J., and Blöschl, G. (2020). Mapping snow cover from daily Collection 6 MODIS products over Austria. J. Hydrology 590, 125548. doi:10.1016/j.jhydrol.2020.125548
Wester, P., Mishra, A., Mukherji, A., and Shrestha, A. (2019). The Hindi Kush Himalaya assessment-mountians, climate change, sustainability and people. Springer Nature Switzerland.
Woo, M. K., and Thorne, R. (2006). Snowmelt contribution to discharge from a large mountainous catchment in subarctic Canada. Hydrol. Process. 20, 2129–2139. doi:10.1002/hyp.6205
Yi, Y., Liu, S., Zhu, Y., Wu, K., Xie, F., and Saifullah, M. (2021). Spatiotemporal heterogeneity of snow cover in the central and Western Karakoram Mountains based on a refined MODIS product during 2002–2018. Atmos. Res. 250, 105402. doi:10.1016/j.atmosres.2020.105402
Keywords: GEE API, Landsat, NDSI, CRU, topographic parameters
Citation: Moazzam MFU, Rahman G, Lee BG and Ansari NA (2022) Trend of snow cover under the influence of climate change using Google Earth Engine platform: A case study of Astore (Western Himalayas) and Shigar (Karakoram region). Front. Environ. Sci. 10:1006399. doi: 10.3389/fenvs.2022.1006399
Received: 29 July 2022; Accepted: 08 September 2022;
Published: 28 September 2022.
Edited by:
Xiuping Li, Institute of Tibetan Plateau Research, Chinese Academy of Sciences (CAS), ChinaReviewed by:
Zhongfan Zhu, Beijing Normal University, ChinaMuhammad Shahid, National University of Sciences and Technology (NUST), Pakistan
Copyright © 2022 Moazzam, Rahman, Lee and Ansari. This is an open-access article distributed under the terms of the Creative Commons Attribution License (CC BY). The use, distribution or reproduction in other forums is permitted, provided the original author(s) and the copyright owner(s) are credited and that the original publication in this journal is cited, in accordance with accepted academic practice. No use, distribution or reproduction is permitted which does not comply with these terms.
*Correspondence: Nadhir Al Ansari, nadhir.alansari@ltu.se