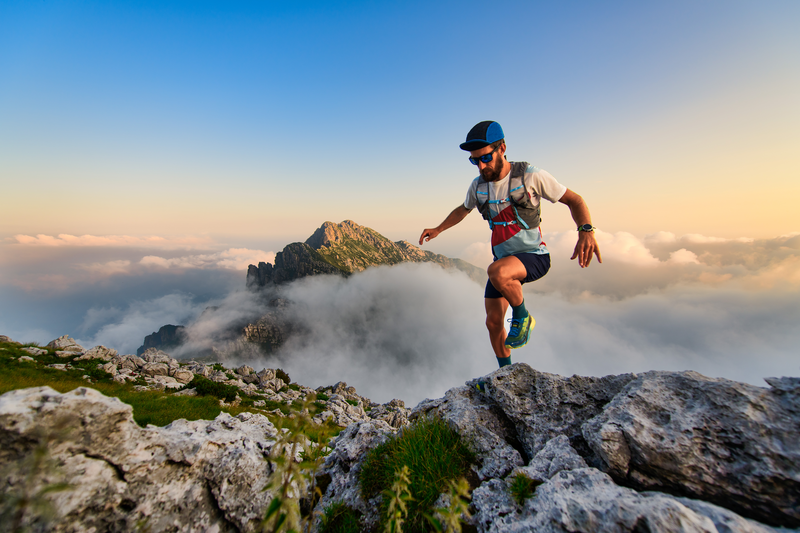
95% of researchers rate our articles as excellent or good
Learn more about the work of our research integrity team to safeguard the quality of each article we publish.
Find out more
ORIGINAL RESEARCH article
Front. Environ. Sci. , 09 September 2022
Sec. Environmental Informatics and Remote Sensing
Volume 10 - 2022 | https://doi.org/10.3389/fenvs.2022.1001064
This article is part of the Research Topic Meta-Scenario Computation for Social-Geographical Sustainability View all 60 articles
This study used both temperature-humidity and wind efficiency indices at three time-scale resolutions (year, season, and month) for the first time, to analyze the spatio–temporal evolution of urban climate comfort in the Guangdong–Hong Kong–Macau Greater Bay Area (GBA). The main factors affecting human-settlement climate comfort were elucidated and the annual changes in both indices used in the study area exhibited fluctuating growth from 2005 to 2020. Moreover, the annual growth of the temperature-humidity and wind efficiency indices in the southern cities of the GBA was relatively fast. In contrast, the annual growth of these indices in the northern cities of the GBA was relatively slow. Overall, the climate of the human-settlement environments in the GBA was the most comfortable in spring and autumn, and summer and winter were characterized by hot and cold climate conditions, respectively. We did not identify any prominent change in the climate comfort of spring and autumn from 2005 to 2020; however, the climate comfort degree deteriorated in summer and ameliorated in winter. On a monthly scale, the human-settlement environments in the GBA were the coldest in December and the hottest in July. The urban human settlements were cold in January and February, hot in May, June, August, and September, and the most comfortable in March, April, October, and November in 2020. We analyzed the factors affecting the climate comfort of human-settlement environments in the study area and found that elevation, gross industrial production, population scale, and construction land area were the most influential parameters. Notably, the impact of natural factors on the climate comfort of human-settlement environments was more significant than that of anthropogenic factors. Moreover, the related factors affected the temperature-humidity index more strongly than the wind efficiency index. Overall, our results provide data-driven guidelines for improving the climate comfort of urban human settlements in the GBA.
The “Outline Development Plan for the Guangdong–Hong Kong–Macau Greater Bay Area (GBA)” is becoming a national strategy in China; thus, it is increasingly important to understand how a vibrant and internationally competitive first-class bay area and world-class city agglomeration can be developed for the society, industry, and government. The objective “to develop a high-quality living circle for living, working, and traveling,” mentioned among the five key aspects of this endeavor not only reflects the governance philosophy of “the people center” but also facilitates the attraction of innovative talents. In this context, climate comfort is one of the most important factors to study for the development of the bay area toward more comfortable ways of living, working, and traveling. In general, urban human-settlement environments are residences in which people live and engage in production, thereby reflecting the economic development level of a region and the quality of life of residents (Li et al., 2021). Structurally, a human-settlement environment is a complex system, which mainly consists of natural, human, living, support, and social systems (Tian et al., 2022; Yang et al., 2020). Of these, the natural system is the foundation, and its overall development and state largely determine the quality of the human-settlement environment (Yang et al., 2022). In this context, climate change is an important element in the natural system and plays a crucial role in controlling the climate comfort of human-settlement environments. Climate comfort generally reflects the level of comfort experienced by healthy human beings without the aid of temperature control devices and is determined by meteorological factors, such as temperature, humidity, wind speed, and sunshine. Fundamentally, it represents a bio-meteorological index, which can be used to evaluate the comfort state of a human body under different climate conditions. In the context of global warming, the recently accelerated urbanization has substantially affected regional climates. Moreover, many people seriously consider the livability standards of a location before choosing their place of residence (Dulal and Akbar, 2013; Wang et al., 2021). Thus, understanding and improving the comfort level of human-settlement climates can improve the quality of human settlements, while uniquely unraveling the fundamental needs of social sustainable development (Zhang et al., 2022).
To date, the research on the climate comfort of human-settlement environments has mainly focused on a narrow scope of topics. First, some studies have examined the spatial characteristics of tourism climate comfort, assessing its impact on tourism potential and development. Specifically, scholars have explored spatial layout characteristics of tourism climate comfort using the tourism climate index (TCI) (Masoudi, 2021). Moreover, a geographic information system (GIS) can be applied to partition and evaluate the ecotourism potential of climate comfort areas (Nasirihendkhaleh et al., 2014). In this way, the impact of climate comfort on health tourism has been elucidated (Amininia et al., 2020). Furthermore, some other studies used the TCI, temperature-humidity, and holiday climate indices to evaluate the influence of climate comfort on tourist visitation patterns for world heritage sites (Hasanah et al., 2020). These studies utilized daily climate data to quantify the comfort climate of national 5A scenic spots (Lei et al., 2020). A comprehensive TCI can be used to evaluate the spatio–temporal evolution of climate comfort for tourists and predict its future development trend (Zhao and Wang., 2021). A previous study used the Grand Shangri-La region as a case study and investigated the change in tourism climate from 1980 to 2016 under intensifying global warming (Cheng and Zhong., 2019). As a result, the spatial distribution of the TCI was analyzed and quantified, thereby covering the areas with different TCI classes in Iran (Bakhtiari et al., 2018). Another study applied the Mediterranean outdoor comfort index and the predicted the mean vote for international tourism to examine the climatic conditions and outdoor thermal comfort in Venice, Rome, and Palermo, located in the north, center, and south of Italy, respectively (Salata et al., 2017).
The second aspect of climate comfort has been addressed by other studies, which examined the influence of natural factors on the climate comfort of the human-settlement environments. These studies explored the impact of natural elements such as elevation (Li and Chi, 2014), meteorology (Tseliou et al., 2010; Eludoyin et al., 2014; Müller et al., 2014; Orosa et al., 2014), rivers (Amorosi et al., 2013; Giannakis et al., 2016), vegetation (Celuppi et al., 2019), air movement acceptability (Cândido et al., 2010), and CO2 concentration (Yüksel et al., 2020), on the climate comfort of human-settlement environments. The third aspect was addressed by some scholars who investigated the influence of human factors on the climate comfort of human-settlement environments; they elucidated the influence of human factors, such as architectural design (Gonzalez-Longo and Sahabuddin, 2019) and land use in an urban area (Cetin, 2020), on the climate comfort of human-settlement environments. The fourth aspect of the climate comfort of human settlements was covered by studies that reported the spatio–temporal characteristics of the climate comfort of human-settlement environments. Such studies quantified the climate comfort of urban human-settlement environments using the climate comfort index and by examining the spatial characteristics of climate comfort (Cao et al., 2019). As a result, the spatio–temporal and seasonal evolutions of the climate comfort of human-settlement environments in China were explored using data from the ground-based meteorological observation station (Yin et al., 2018).
Currently, most studies focusing on the climate comfort of the human-settlement environments address the spatio–temporal distribution of climate comfort, tourism climate comfort, and the influence of individual natural and human factors on climate comfort. However, our knowledge on the climate comfort of human-settlement environments is incomplete at country (e.g., China) (Kong et al., 2019; Kambezidis et al., 2021; Katavoutas et al., 2021; Zhang et al., 2022), economic corridor (e.g., the China-Pakistan Economic Corridor) (Zeng et al., 2020), regional (e.g., northwest China) (Yao et al., 2021), urban agglomeration (e.g., Beijing-Tianjin-Hebei Area) (Liu et al., 2020), provincial (e.g., Liaoning) (Cheung and Hart, 2014; Cao et al., 2016), city (Urumqi) (Li et al., 2014), county (district) (e.g. Changsha county) (Liu et al., 2013), and township scales (e.g., Qiankou town) (Yu et al., 2021). Moreover, studies on the spatio–temporal changes in the climate comfort of human-settlement environments at the bay area scale are very scarce. The Guangdong–Hong Kong–Macau GBA is located at the frontier coastal opening of China, with the Pan-Pearl River Delta region as its vast development hinterland. Guangdong, Hong Kong, and Macau all stand out with prominent development in science and technology, thereby attracting residents, businesses, and investments to the area. By elucidating the climate comfort of the human-settlement environments in the GBA, the quality of life of residents can be improved, while the attraction of talents can lead to the formation of an international science and technology innovation center in the GBA.
To this end, our study used the GBA as the study region, where we combined the temperature-humidity and wind efficiency indices to evaluate the climate comfort of the human-settlement environment from 2005 to 2020. The objectives of this study are 1) to conduct a spatio–temporal analysis of the climate comfort of human-settlement environments using GIS technology and 2) explore the factors that impact the climate comfort of human-settlement environments by multiple logistic regression methods. By elucidating the climate comfort of the human-settlement environments in the GBA, this study provides a scientific reference for attracting innovative talents and improving the quality of life of residents, in addition to providing a useful reference for enabling rational urban regional planning, such as the formation of an international science and technology innovation center in the GBA.
The GBA (China) consists of the Hong Kong and Macau special administrative regions, and nine cities in Guangdong Province. The total area of GBA is 5.6 km2. This area has the highest degree of openness and strongest economic vitality compared to that of other regions in China and also has an important strategic position (Figure 1).
FIGURE 1. The average annual residential climate comfort chart in China from 2005 to 2020 (Map Content Approval Number: GS (2019)1822).
This study used data from near-ground-based climatology databases in China. We used a daily-scale value dataset (v3.0 processing and generation), statistical yearbook data, PM2.5 data, land cover data, vegetation coverage data, digital elevation model (DEM) data, and administrative division data. The China ground climate dataset mainly includes the daily data from 2005 to 2020, containing the following parameters: wind speed, humidity, precipitation, temperature, sunshine, station number, and coordinates (longitude and latitude). The dataset was originally composed based on observations from 824 benchmark meteorological stations in China and provided by the China Meteorological Data Service Center (http://data.cma.cn/).
From a data processing perspective, the data were first collected and sorted, then projected at specific points to overlap with the base map, and daily data were spatially interpolated by the inverse-distance weighting method. Finally, the data were divided and split according to the administrative divisions. By doing this we obtained the final daily data for provinces and cities, as well as the average daily data of meteorological elements in different years and months as the basis for the yearly and monthly data for the different regions.
We used the following socioeconomic data: the permanent population, gross domestic product (GDP), gross industrial production (%), and construction land area. These data were obtained from the China City Statistical Yearbook (http://www.stats.gov.cn/tjsj/), Guangdong Statistical Yearbook (http://stats.gd.gov.cn/), Hong Kong Annual Digest of Statistics (https://www.censtatd.gov.hk/sc/), and Anuário Estatístico Yearbook of Statistics (https://www.dsec.gov.mo/zh-MO/) from 2005 to 2021. The PM2.5 concentrations of cities in the GBA were obtained from the Atmospheric Composition Analysis Group of Dalhousie University (http://fizz.phys.dal.ca/∼atmos/martin/?page_id=140). The PM2.5 data were first rasterized and spatially optimized to the boundaries of the analyzed cities to derive the PM2.5 concentration annual mean data. The land cover data included estimates of arable land, forests, grassland, and artificial ground. The land cover-related data were obtained from the National Catalogue Service for Geographic information (https://www.webmap.cn/main.do?method=index). The GBA DEM elevation, vegetation coverage, and administrative division data were acquired from the Resources and Environment Sciences and Data Center of the Chinese Academy of Sciences (http://www.resdc.cn/data.aspx; DATA ID = 333) (Table 1).
The temperature-humidity index refers to an index of the comprehensive perception of ambient temperature and humidity of the human body, calculated using Eq. 1:
where I is the temperature-humidity index, retaining 1 decimal place; T is the average temperature of a certain evaluation period (°C); and RH is the average air relative humidity during an evaluation period (%).
This refers to an index of comprehensively perceiving wind, temperature, and sunshine, calculated using Eq. 2:
where K is the wind efficiency index, taken as an integer; T is the average temperature of a certain evaluation period (°C); V is the average wind speed (m/s); and S is the average hours of sunshine during an evaluation period, measured in hours per day (h/d).
Generally, an evaluation method combining the temperature-humidity and wind efficiency indices is used to evaluate climate comfort. When the two indices are inconsistent, the wind efficiency index is used in the cold (winter) half-year, and the temperature-humidity index in the hot (summer) half-year. The wind efficiency index is used when the average wind speed exceeds 3 m/s during the evaluation period. The March–May period is regarded as spring, June–August as summer, September–November as autumn, and December–February as winter. High temperature-humidity and wind efficiency indices values indicate a relatively sultry human-settlement climate, whereas low values indicate an extremely cold human settlement climate. The only comfortable human-settlement climate is a moderate temperature-humidity and wind efficiency indices value (Table 2).
We analyzed the temporal evolution characteristics of the temperature-humidity index and found that the index values of the 11 cities in the GBA increased with various intermittent fluctuations from 2005 to 2020 (Figure 2). From a spatial perspective, the temperature-humidity index values of the GBA exhibited gradual increases from north to south in 2020. We found that Macau was among the regions with the highest temperature-humidity index values, while the lowest value was found in Zhaoqing. We further ranked the regions according to the descending order of their temperature-humidity indices values. This analysis revealed the spatial evolution of the northern cities in the GBA (Zhaoqing, Guangzhou, and Huizhou) and most of the southern cities in the GBA (Jiangmen, Dongguan, Shenzhen, Zhuhai, Hong Kong, and Macau), which generally showed an overall growth trend. However, the spatial evolution of the central cities in the GBA (Foshan and Dongguan) and a single southern city in the GBA (Zhongshan) experienced decreases at first but then increased (exhibited growth). We also analyzed the growth range of the temperature-humidity index. It was found that the southern cities in the GBA with relatively large areas exhibited growth of the temperature-humidity index values by 0.8–1. The growth range of the temperature-humidity index values of the northern cities in the GBA was lower (0.6–0.7) (Figure 3).
FIGURE 3. The annual change of temperature-humidity index in the Guangdong–Hong Kong–Macau Greater Bay Area in (A) 2005, (B) 2010, (C) 2015, and (D) 2020.
Furthermore, we analyzed the temporal evolution characteristics of the wind efficiency index. It was found that the rank of the wind index interval range values in the GBA from 2005 to 2020 generally exhibited a decreasing trend first, and then changed to an increasing trend (Figure 4). From a spatial perspective, the wind efficiency index values of the GBA decreased from the central areas in the GBA to the periphery in 2020. Hong Kong exhibited the highest wind efficiency index value, while Zhaoqing exhibited the lowest. Compared with the temperature-humidity index, the spatial differences in wind efficiency in the different cities were more prominent. Moreover, the wind efficiency index values of the southern cities in the GBA demonstrated a growth range of 20–28, indicating relatively strong growth. However, the wind efficiency index values of the northern cities in the GBA exhibited weaker increases, with a growth range of 2–15 (Figure 5).
FIGURE 5. Annual change of wind efficiency index in Guangdong–Hong Kong–Macau Greater Bay Area in 2005, 2010, 2015, and 2020. Seasonal and monthly evolution characteristics of climate comfort of human-settlement environments.
The seasonal evolution characteristics of the climate comfort of human-settlement environments in the GBA were analyzed from 2005 to 2020. No prominent change in the climate comfort of human-settlement environments was discerned in spring and autumn, but the climate comfort in summer and winter exhibited significant changes. We identified an increase in regional temperature in July–August, compared with that in previous years (2005, 2010, and 2015). However, the regional precipitation decreased compared with that in previous years, and precipitation increased in the eastern regions in the GBA because of typhoons (Zhang et al., 2020). Moreover, the temperature in November–December increased and precipitation decreased (Li et al., 2021), compared with that in previous years. Thus, the climate comfort of human-settlement environments in the western cities in the GBA changed from “heat” to “sultry” gradually in the summer, while no prominent change was found in the summer for other cities. The climate comfort of human-settlement environments for peripheral cities in the GBA changed from “extremely cold” to “cold” in winter, while the climate comfort for the central cities in the GBA exhibited no significant change. Note that gas emissions, driven by the industrial transformation of different cities and anthropogenic activities during the process of urbanization also theoretically affect the climate comfort of human-settlement environments. The detected change in the climate comfort of human-settlement environments (from “heat” to “sultry”) worsened the livability in the GBA in the summer, but the change in the climate comfort from “extremely cold” to “cold” ameliorated the livability in the GBA in winter.
The spatial evolution of the seasonal climate comfort of human-settlement environments was also analyzed in the GBA. It was found that all cities were somewhat comfortable in spring in 2020 (Figure 9). As the temperature increased in the summer, the climate comfort in the cities gradually worsened. Moreover, spatial heterogeneity of the climate comfort was discerned in the GBA. Overall, the western cities (Zhuhai, Jiangmen, Foshan, Zhongshan, and Macau) in the GBA were found to have a sultry climate, while the eastern cities (Guangzhou, Dongguan, Shenzhen, Huizhou, and Hong Kong) and a single northern city (Zhaoqing) had comparatively better climate comfort. However, the climate comfort of human-settlement environments was still relatively hot. The human-settlement environments in different cities in the GBA were found to be comfortable in autumn, while the climate of all cities was reportedly relatively cold in winter. In 2005, the climate comfort of human-settlement environments in all cities was found to be comfortable in spring and autumn. However, the cities were found to be relatively hot in summer, with the peripheral cities in the GBA experiencing an extremely cold climate in winter (Figure 6). In 2010, the climate comfort of human settlement environments in spring, summer, and autumn was found to be the same as that in 2005, while the climate comfort of human-settlement environments for all cities was deemed cold in winter (Figure 7). In 2015, the climate comfort of human settlement environments for all cities in the GBA was somewhat high in spring and autumn, but the comfort for west-central cities in the bay (Zhongshan, Zhuhai, and Macao) was deemed “sultry” (Figures 8, 9).
FIGURE 6. Seasonal change of climate comfort of human settlements in the Guangdong–Hong Kong–Macau Greater Bay Area in 2005.
FIGURE 7. Seasonal change of climate comfort of human settlements in the Guangdong–Hong Kong–Macau Greater Bay Area in 2010.
FIGURE 8. Seasonal change of climate comfort of human settlements in the Guangdong–Hong Kong–Macau Greater Bay Area in 2015.
FIGURE 9. Seasonal change of climate comfort of human settlements in the Guangdong–Hong Kong–Macau Greater Bay Area in 2020.
All cities in the Beijing–Tianjin–Hebei region during the March–June and September–October periods were the most comfortable from a climate perspective (Cao and Wang, 2017). The comprehensive synthesis comfort period of the Hohhot–Baotou–Ordos region was mainly April–October (Zhao, 2022), while the months with a higher comfort level in the three northeastern provinces (Liaoning, Jilin, and Heilongjiang) were June–August (Bai, 2020). Although the GBA is hot and the climate comfort is worse than that in northern China in summer, the region is comfortable in both spring and autumn, compared with northern China. Moreover, the climate of human-settlement environments was cold in winter, but compared with that in northern China, which is extremely cold in winter, the GBA was evidently more comfortable.
The monthly changes in the climate comfort of human-settlement environments in the GBA were analyzed. As January and February represent winter months, the climate comfort of human-settlement environments is correspondingly cold in the GBA. Our results showed that the climate comfort changes from “cold” to “comfortable” in March and April, when the GBA experiences spring. May and June represent the transitional season for the GBA (from spring to summer), when the climate comfort gradually changes from “comfortable” to “hot.” Overall, the climate comfort of human-settlement environments in the GBA can be characterized as “hot” in May, and the southwestern cities (Jiangmen, Zhongshan, Zhuhai, and Hong Kong) start experiencing extremely high temperatures in June. Thus, the climate comfort of human-settlement environments shifts to “sultry” in these cities, while the climate comfort of other cities in the area becomes “hot.” In July, the GBA typically enters midsummer, and the climate comfort of human-settlement environments in all cities becomes “sultry.” In August and September, the GBA experiences a transition from summer to autumn. In August, the climate comfort of human-settlement environments in all cities in the GBA becomes “hot.” In September, besides the “comfortable” level of the climate comfort in Zhaoqing, the climate comfort of other cities was “hot.” In October and November, the GBA enters the late autumn period, and the climate comfort of human-settlement environments in all cities can be deemed as “comfortable.” In December, the GBA experiences a seasonal change from autumn to winter, when the climate comfort of human-settlement environments in all cities tends to cool down; overall, the climate comfort level of human-settlement environments in Zhaoqing and Guangzhou prominently changed to “extremely cold,” whereas the climate comfort level of human-settlement environments for all other cities was “cold,” with their climate comfort experiencing a minor change (Figure 10). Notably, elucidating the seasonal and monthly evolution characteristics of climate comfort of human-settlement environments allows us to unravel the spatial characteristics of the “migratory” elderly population and it also allows us to determine the most comfortable period for tourism. Finally, our findings could provide data-driven guidelines for planning the migration of the elderly, while also establishing an innovative and livable highland.
FIGURE 10. The monthly variation of the climate comfort of human settlements in the Guangdong–Hong Kong–Macau Greater Bay Area in 2020.
Human settlements represent a relationship between the five basic elements of ekistics: nature, human, society, buildings, and networks. Of these elements, natural environments play a basic function in human settlement. From the perspective of metropolises, urban groups, and large urban agglomerations, an urban area scale can be used to explore the relationship between various elements in human settlements for building a good livable environment. As global warming is intensifying, the GBA is experiencing extreme temperatures more often (Huang et al., 2012). Thus, climate change crucially affects the climate comfort of human settlements in the area. Given the novelty of this research domain and the data availability, we selected six key indicators that could potentially affect the climate comfort of urban settlements: elevation (Wei and Wang, 2022), vegetation coverage (Hu et al., 2011), haze (Cai et al., 2017), population scale (Nwankwo et al., 2021), gross industrial production (%) (Streimikiene et al., 2012), and construction land area (Wang et al., 2020). Based on this, we performed a diagnosis of multicollinearity to select the required indices. Overall, the elevation, construction land area, population scale, and gross industrial production (%) passed the collinearity test. The correlation of the four elements with the temperature-humidity and wind efficiency indices was quantified using multiple logistic regression. The correlation analysis revealed some related factors, exhibiting a relationship with the temperature-humidity and wind efficiency indices in 2005, 2010, 2015, and 2020. However, there were significant differences in the indices as the influence of related factors on the temperature-humidity index was more important than that on the wind efficiency index. Additionally, the influence of natural factors on climate comfort was greater than that of anthropogenic activities (Table 3). Overall, this study elucidated that the factors influencing climate comfort were elevation, construction land, population scale, and the percentage of gross industrial production.
TABLE 3. Correlation of factors affecting climate comfort of human-settlement in 2005, 2010, 2015, and 2020.
The topography of the GBA is mainly characterized by low mountains and hills. In general, different elevation levels fundamentally control regional meteorological elements such as temperature and wind speed, thereby affecting the climate comfort of human-settlement environments (Yan et al., 2022). Specifically, higher elevations lead to lower temperatures. The lower elevation (310 m) site is warmer (28.5°C average monthly maximum air temperature) and drier (224 mm yr−1) than the upper elevation (844 m) site (23.5°C average monthly maximum air temperature; 272 mm yr−1) (Link et al., 2003). Areas with high elevation are mainly distributed in the northern regions in the GBA, while areas in the southern regions have relatively low elevation (Figure 11). Thus, in the summer, the northern cities in the GBA enter the sultry period later than the southern cities. However, the shift to the comfortable period occurs earlier in the southern cities in the GBA in autumn, and this phenomenon is also affected by elevation. Moreover, the climate comfort of human-settlement environments in the northern cities in the GBA is deemed extremely cold in the winter, while that of the southern cities is cold, which is also affected by the elevation.
The construction land area reflects the carrying and storage capacity of natural elements and the degree of land use change in a region of interest. This is an important factor that affects regional urban construction and development (Zhao et al., 2021). The various types of urban land have different contributions to the urban thermal environment. Among the various types of urban land, public service-facility land contributed the most to the urban thermal environment with an impact of 21.65%, followed by residential, industrial, and commercial service-facility lands, with impacts of 19.89, 18.44, and 17.58%, respectively. Additionally, the impact of road traffic land was 14.92%, whereas that of green square land was the lowest at 7.51%, 14.14% lower than that of public-service facility land (Chen et al., 2022). In general, cities with widespread construction land areas are characterized by higher development intensity, high pollution, and low vegetation coverage and, thus, experience a substantial heat island effect. Continuous urban development (building up) promotes the urban heat island effect. The magnitude of the urban heat island ranged from 0.2 to 4.7°C, subject to seasonal variations and weather conditions (Weng and Yang., 2004). In contrast, cities with low construction land areas have less development intensity, less pollution, relatively high vegetation coverage, and thus, a relatively cooler climate (Ren et al., 2022). Urban land is mainly concentrated in the central cities in the GBA, while forests, grassland, and cultivated land are mainly concentrated in the peripheral cities. The concentration of urban land considerably exacerbates the heat island effect. Thus, the temperature of central cities in the GBA is generally higher than that of the peripheral cities (Figure 12). In addition, the southern areas of the GBA are adjacent to the ocean. The ocean has a large specific heat capacity, which affects the climate factors such as humidity and wind speed; it changes the annual evolution of climate comfort of human-settlement environments slightly or greatly, depending on the season. The average water-cooling island intensity ranges from 1.19 °C to 5.02°C in the four seasons, and the WCI (Wind Chill Index) intensity of all water bodies exhibits the largest fluctuation in summer (Wu et al., 2020).
Population growth can potentially promote the progress of scientific and technological innovation (Chen et al., 2018), thereby moving the region toward economic growth. However, economic development can trigger a sizeable growth in energy consumption, inducing a sharp increase in carbon emissions (Wang et al., 2021). Therefore, population growth and carbon emissions exhibit a positive correlation. In addition, population density and migration are also strongly correlated with carbon emissions. An increase in population density is unfavorable to the reduction of carbon emissions (Minx et al., 2013), and population migration from rural to urban areas will increase the carbon footprint; the apparent carbon emission difference between rural residents and migrants is about 1.5-ton CO2 per capita (Zhang et al., 2016). Anthropogenic CO2 emissions, driven by fossil fuel use, are the main driver of global warming. Thus, an increase in population can considerably alter the regional climate temperature, further affecting the climate comfort of human settlement environments.
A reduction in gross industrial production can help reduce energy consumption, ameliorate energy utilization efficiency, and facilitate the optimization of the energy structure, thereby enhancing land carbon sinks. Such phenomena, in turn, alter the climate comfort of human-settlement environments. Furthermore, intensive gross industrial production can potentially attract high-polluting industries; thus, inducing a significant increase in energy consumption and greenhouse gas emissions and as an indirect effect, the urban heat island effect would be exacerbated in the case of increased production.
This study elucidated unique aspects of climate comfort in the GBA. We estimated the climate comfort at unprecedented scales (national, urban agglomeration, provincial, and municipal scales) by analyzing the spatio–temporal evolution characteristics of climate comfort, on which few studies have been done. Thus, our study deepened the knowledge on the evolution of the climate comfort of human-settlement environments in the GBA. Moreover, we elucidated the factors influencing the studied phenomenon for the first time. In particular, we explored the influence of the related factors on the climate comfort of human-settlement environments by analyzing elevation, population scale, gross industrial production, and construction land area.
Despite these promising findings, our study had the following limitations: 1) because the statistical measurements between Guangdong, Hong Kong, and Macau were inconsistent, the interpretation of the impact of greenhouse gases and other related indicators on the climate comfort of human-settlement environments were critically hindered; 2) when the temperature-humidity and wind efficiency indices were inconsistent, the wind efficiency index was used to analyze the cold (winter) season, and the temperature-humidity index was used to analyze the hot (summer) season. Thus, the evaluation of the climate comfort of human-settlement environments was slightly asymmetric. In particular, the evaluation did not consider the intensity of interactions between these two indices and the comfortable threshold. Future studies should further refine the value range of the climate comfort of human-settlement environments. Moreover, the complex interplay between the two indices (temperature-humidity and wind efficiency indices) used for analysis should be quantified in future studies to improve the evaluation accuracy of the climate comfort of human-settlement environments.
This study used multiple datasets including ground-based climate (mean daily-value data) and land cover data in China, and the temperature-humidity and wind efficiency indices. These data were used to explore the spatio–temporal evolution of the climate comfort of human-settlement environments in the GBA. Furthermore, we elucidated the main factors affecting climate comfort and the following conclusions were drawn: 1) The annual changes in the climate comfort of human settlement environments were analyzed and this revealed that the values of the temperature-humidity index of the GBA decreased from north to south, and that of the wind efficiency index decreased from the center to the periphery in 2020. From 2005 to 2020, the temperature-humidity index values of the northern cities and most southern cities in the GBA exhibited increases, while the central cities and some southern cities first exhibited a decrease and then an increase. Moreover, the wind efficiency index values of the GBA first exhibited a decreasing trend, but then an increasing trend from 2005 to 2020. Overall, the climate comfort of human-settlement environments in the GBA experienced a significant change in spring and summer; the summer conditions changed from “hot” to “sultry” and the winter conditions changed from “extremely cold” to “cold.”
2) The spatio–temporal heterogeneity of the climate comfort of human-settlement environments in the GBA was mainly affected by the elevation, gross industrial production, population scale, and construction land area. Of these factors, elevation had the most significant effect on climate comfort. The impact of the population scale on climate comfort was secondary, followed by gross industrial production and construction land area.
3) The spatial characteristics of the climate comfort of human-settlement environments in the GBA were characterized by an asymmetric pattern. Moreover, the area experiences a comfortable climate in spring, autumn, and winter, thereby agreeing with existing studies (Li et al., 2016). Additionally, we compared the influence of natural factors with that of anthropogenic activities on the climate comfort of human settlement environments. This comparison demonstrated that the correlation between natural factors and the climate comfort of human-settlement environments was more important than that of anthropogenic activities.
The original contributions presented in the study are included in the article/Supplementary Material, further inquiries can be directed to the corresponding author.
Conceptualization, CZ, and DZ; methodology, DZ, and YC; software, YC, and YW; Validation, CZ, and GZ; formal analysis, CZ, and GZ; data curation, DZ, and YC; writing-original draft, GZ, and DZ; writing-review and editing, CZ, and GZ. All authors have read and agreed to the published version of the manuscript.
This research was supported by the Major Program of the National Social Science Foundation of China (Grant No. 21&ZD107).
The authors declare that the research was conducted in the absence of any commercial or financial relationships that could be construed as a potential conflict of interest.
All claims expressed in this article are solely those of the authors and do not necessarily represent those of their affiliated organizations, or those of the publisher, the editors and the reviewers. Any product that may be evaluated in this article, or claim that may be made by its manufacturer, is not guaranteed or endorsed by the publisher.
The Supplementary Material for this article can be found online at: https://www.frontiersin.org/articles/10.3389/fenvs.2022.1001064/full#supplementary-material
Amininia, K., Abad, B., Safarianzengir, V., GhaffariGilandeh, A., and Sobhani, B. (2020). Investigation and analysis of climate comfort on people health tourism in Ardabil province, Iran. Air Qual. Atmos. Health 13, 1293–1303. doi:10.1007/s11869-020-00883-x
Amorosi, A., Bini, M., Giacomelli, S., Pappalardo, M., Ribecai, C., Rossi, V., et al. (2013). Middle to late Holocene environmental evolution of the Pisa coastal plain(Tuscany, Italy)and early human settlements. Quat. Int. 303, 93–106. doi:10.1016/j.quaint.2013.03.030
Bai, Z. (2020). Spatio-temporal differences in climate comfort of human settlements in the three provinces of Northeast China and influencing factor. Dalian: Liaoning Normal University.
Bakhtiari, B., Bakhtiari, A., and Gorouh, Z. (2018). Investigation of climate change impacts on tourism climate comfort in Iran. Glob. Nest J. 20 (2), 291–303. doi:10.30955/gnj.002435
Cai, W., Li, K., Liao, H., Wang, H., and Wu, L. (2017). Weather conditions conducive to Beijing severe haze more frequent under climate change. Nat. Clim. Chang. 7, 257–262. doi:10.1038/NCLIMATE3249
Cândido, C., de Dear, R., Lamberts, R., and Bittencourt, L. (2010). Air movement acceptability limits and thermal comfort in Brazil’s hot humid climate zone. Build. Environ. 45, 222–229. doi:10.1016/j.buildenv.2009.06.005
Cao, W., and Wang, S. (2017). Evaluation of climate suitability for urban human settlement in Beijing-Tianjin-Hebei region. J. Glaciol. Geocryol. 39 (2), 435–442. doi:10.7522/j.issn.1000-0240.2017.0050
Cao, Y., Gao, L., and Wang, X. (2016). Regional characteristics of climate comfort in summer in Liaoning during past 30 years. Sci. Geogr. Sin. 36 (8), 1205–1211. doi:10.13249/j.cnki.sgs.2016.08.011
Cao, Y., Sun, Y., and Wu, M. (2019). Spatial and temporal characteristics of the periods of climate comfort in the Beijing-Tianjin-Hebei region from 1966 to 2015. Acta Ecol. Sin. 39 (20), 7567–7582. doi:10.105846/stxb201805181096
Celuppi, M., Meirelles, C., Cymrot, R., Borst, B., and Gobo, J. (2019). Preliminary approach to the analysis of climate perception and human thermal comfort for riverside dwellings the Brazilian Amazon. J. Build. Eng. 23, 77–89. doi:10.1016/j.jobe.2019.01.019
Cetin, M. (2020). Climate comfort depending on different altitudes and land use in the urban areas in Kahramanmaras City. Air Qual. Atmos. Health 13, 991–999. doi:10.1007/s11869-020-00858-y
Chen, L., Li, X., Yao, Y., and Chen, D. (2018). Effect of population agglomeration on urban economic growth in China. Acta Geogr. Sin. 73 (6), 1107–1120. doi:10.11821/dlxb201806009
Chen, Y., Yang, J., Yang, R., Xiao, X., and Xia, J. (2022). Contribution of urban functional zones to the spatial distribution of urban thermal environment. Build. Environ. 216, 109000. doi:10.1016/j.buildenv.2022.109000
Cheng, Q., and Zhong, F. (2019). Evaluation of tourism climate comfort in the Grand Shangri-La region. J. Mt. Sci. 16 (6), 1452–1469. doi:10.1007/s11629-018-5081-4
Cheung, C., and Hart, M. (2014). Climate change and thermal comfort in Hong Kong. Int. J. Biometeorol. 58, 137–148. doi:10.1007/s00484-012-0608-9
Dulal, H., and Akbar, S. (2013). Greenhouse gas emission reduction options for cities: Finding the “Coincidence of Agendas” between local priorities and climate change mitigation objectives. Habitat Int. 38, 100–105. doi:10.1016/j.habitatint.2012.05.001
Eludoyin, O., Adelekan, I., Webster, R., and Eludoyin, A. (2014). Air temperature, relative humidity, climate regionalization and thermal comfort of Nigeria. Int. J. Climatol. 34, 2000–2018. doi:10.1002/joc.3817
Giannakis, E., Bruggeman, A., Poulou, D., Zoumides, C., and Eliades, M. (2016). Linear parks along urban rivers: Perceptions of thermal comfort and climate change adaptation in Cyprus. Sustainability 8, 1023. doi:10.3390/su8101023
Gonzalez-Longo, C., and Sahabuddin, M. (2019). High-rise social housing in hot-humid climates: Towards an ‘airhouse’ standard for comfort. Appl. Sci. (Basel). 9, 4985. doi:10.3390/app9234985
Hasanah, N., Maryetnowati, D., Edelweis, F., Indriyani, F., and Nugrahayu, Q. (2020). The climate comfort assessment for tourism purposes in Borobudur Temple Indonesia. Heliyon 6, e05828. doi:10.1016/j.heliyon.2020.e05828
Hu, M., Mao, F., Sun, H., and Hou, Y. (2011). Study of normalized difference vegetation index variation and its correlation with climate factors in the three-river-source region. Int. J. Appl. Earth Observation Geoinformation 13, 24–33. doi:10.1016/j.jag.2010.06.003
Huang, Q., Chen, Z., and Liu, Z. (2012). Temporal and spatial variations of extreme temperature in Guangdong over the last 60 years in the background of global warming. Trop. Geogr. 32 (3), 246–251. doi:10.13284/j.cnki.rddl.001618
Kambezidis, H., Psiloglou, B., Varotsos, K., and Giannakopoulos, C. (2021). Climate change and thermal comfort in Greece. Climate 9, 10. doi:10.3390/cli9010010
Katavoutas, G., Founda, D., Kitsara, G., and Giannakopoulos, C. (2021). Climate change and thermal comfort in top tourist destinations—the case of santorini(Greece). Sustainability 13, 9107. doi:10.3390/su13169107
Kong, Q., Zheng, J., Fowler, H., Ge, Q., and Xi, J. (2019). Climate change and summer thermal comfort in China. Theor. Appl. Climatol. 137, 1077–1088. doi:10.1007/s00704-018-2648-5
Lei, H., Zhang, H., Liu, X., Yang, X., Duan, H., Wu, T., et al. (2020). Climate comfort evaluation of national 5A tourist attractions in the mainland of China based on universal thermal climate index. Adv. Meteorology, 1. 4256164. doi:10.1155/2020/4256164
Li, D., Yang, Z., Shi, H., Luan, F., and Wang, Z. (2014). Tourism climate and its comfort degree in Urumqi. Arid Zone Res. 31 (3), 404–409. doi:10.13866/j.azr.2014.03.008
Li, R., and Chi, X. (2014). Thermal comfort and tourism climate changes in the Qinghai-Tibet Plateau in the last 50 years. Theor. Appl. Climatol. 117, 613–624. doi:10.1007/s00704-013-1027-5
Li, S., Sun, M., Zhang, W., Tan, L., Zhu, N., and Wang, Y. (2016). Spatial patterns and evolving characteristics of climate comfortable period in the mainland of China:1961-2010. Geogr. Res. 35 (11), 2053–2070. doi:10.11821/dlyj201611005
Li, W., Du, Y., Liu, W., and Pang, G. (2021). Comments on the characteristics and its impact of weather and climate in Guangdong province from November to December in 2020. Guangdong meteorol. Inside front cover, inside back cover, 43. doi:10.3969/j.issn.1007-6190.2021.01.020
Li, X., Li, S., Tian, S., Guan, Y., and Liu, H. (2021) Air quality and the spatial-temporal differentiation of mechanisms underlying Chinese urban human settlements. Land, 10:1207. doi:10.3390/land10111207
Link, S., Smith, J., Halvorson, J., and Bolton, H. (2003). A reciprocal transplant experiment within a climatic gradient in a semiarid shrub-steppe ecosystem: Effects on bunchgrass growth and reproduction, soil carbon, and soil nitrogen. Glob. Chang. Biol. 9, 1097–1105. doi:10.1046/j.1365-2486.2003.00647.x
Liu, P., Liao, L., and Liu, C. (2013). Urban residential amenity index and its composition factors: The case of Changsha County in Hunan Province. Prog. Geogr. 32 (5), 769–776. doi:10.11820/dlkxjz.2013.05.008
Liu, S., Long, B., Pan, Z., Lun, F., Song, Y., Yuan, W., et al. (2020). Evaluation of climatic comfort of living environment based on age differentials in Beijing-Tianjin-Hebei Area. Ecosyst. Health Sustain. 6 (1), 1843371. doi:10.1080/20964129.2020.1843371
Masoudi, M. (2021). Estimation of the spatial climate comfort distribution using tourism climate index(TCI)and inverse distance weighting(IDW) (case study:Fars Province, Iran). Arab. J. Geosci. 14, 363. doi:10.1007/s12517-021-06605-6
Minx, J., Baiocchi, G., Wiedmann, T., Barrett, J., Creutzig, F., Feng, K. S., et al. (2013). Carbon footprints of cities and other human settlements in the UK. Environ. Res. Lett. 8, 035039. doi:10.1088/1748-9326/8/3/035039
Müller, N., Wilhelm, K., and Barlag, A. (2014). Counteracting urban climate change: Adaptation measures and their effect on thermal comfort. Theor. Appl. Climatol. 115, 243–257. doi:10.1007/s00704-013-0890-4
Nasirihendkhaleh, E., Zahraei, A., Ghadiri, H., and Komasi, H. (2014). Zoning evaluation of Eco-Touristic potentials of bio-climatic comfortability in the province of Fars, using Geographical information system(GIS). Int. J. Adv. Res. 2 (4), 768–776.
Nwankwo, C., Ossai, O., Ayadiuno, R., and Ikeogu, C. (2021). Spatial dimension of climate change vulnerability and urbanization relationship in Nigeria. Int. J. Urban Sci., 1–22. doi:10.1080/12265934.2021.1956365
Orosa, J., Costa, A., Rodríguez-Fernández, A., and Roshan, G. (2014). Effect of climate change on outdoor thermal comfort in humid climates. J. Environ. Health Sci. Eng. 12, 46. doi:10.1186/2052-336X-12-46
Ren, J., Yang, J., Zhang, Y., Xiao, X., Xia, J., Li, X., et al. (2022). Exploring thermal comfort of urban buildings based on local climate zones. J. Clean. Prod. 340, 130744. doi:10.1016/j.jclepro.2022.130744
Salata, F., Golasi, I., Proietti, R., and Vollaro, A. (2017). Implications of climate and outdoor thermal comfort on tourism: The case of Italy. Int. J. Biometeorol. 61, 2229–2244. doi:10.1007/s00484-017-1430-1
Streimikiene, D., Balezentis, T., and Krisciukaitiene, I. (2012). Promoting interactions between local climate change mitigation, sustainable energy development, and rural development policies in Lithuania. Energy policy 50, 699–710. doi:10.1016/j.enpol.2012.08.015
Tian, S., Qi, A., Li, Z., Pan, X., Liu, Y., and Li, X. (2022). Urban “three states” human settlements high-quality coordinated development. Buildings 12, 178. doi:10.3390/buildings12020178
Tseliou, A., Tsiros, I., Lykoudis, S., and Nikolopoulou, M. (2010). An evaluation of three biometeorological indices for human thermal comfort in urban outdoor areas under real climatic conditions. Build. Environ. 45, 1346–1352. doi:10.1016/j.buildenv.2009.11.009
Wang, R., Zhang, H., Qiang, W., Li, F., and Peng, J. (2021). Spatial characteristics and influencing factors of carbon emissions in county-level cities of China based on urbanization. Prog. Geogr. 40 (12), 1999–2010. doi:10.18306/dlkxjz.2021.12.002
Wang, S., Liu, Y., Gao, Q., Li, H., Yu, Y., and Yang, L. (2021). Applicability of passive design strategies in China promoted under global warming in past half century. Build. Environ. 195, 107777. doi:10.1016/j.buildenv.2021.107777
Wang, Y., Song, L., Han, Z., Liao, Y., Xu, H., Zhai, J., et al. (2020). Climate-related risks in the construction of xiongan new area, China. Theor. Appl. Climatol. 141, 1301–1311. doi:10.1007/s00704-020-03277-2
Wei, X., and Wang, X. (2022). Analyzing the spatial distribution of LST and its relationship with underlying surfaces in different months by classification and intersection. Front. Environ. Sci. 10, 872282. doi:10.3389/fenvs.2022.872282
Weng, Q., and Yang, S. (2004). Managing the adverse thermal effects of urban development in a densely populated Chinese city. J. Environ. Manag. 70, 145–156. doi:10.1016/j.jenvman.2003.11.006
Wu, J., Li, C., Zhang, X., Zhao, Y., Liang, J., and Wang, Z. (2020). Seasonal variations and main influencing factors of the water cooling islands effect in Shenzhen. Ecol. Indic. 117, 106699. doi:10.1016/j.ecolind.2020.106699
Yan, W., He, Y., and Qu, X. (2022). Elevation gradient dependence of extreme climate indices on Yunnan Plateau, China. Int. J. Climatol., 1–20. doi:10.1002/joc.7578
Yang, J., Wang, Y., Xiu, C., Xiao, X., Xia, J., and Jin, C. (2020). Optimizing local climate zones to mitigate urban heat island effect in human settlements. J. Clean. Prod. 275, 123767. doi:10.1016/j.jclepro.2020.123767
Yang, J., Xin, J., Zhang, Y., Xiao, X., and Xia, J. (2022). Contributions of sea-land breeze and local climate zones to daytime and nighttime heat island intensity. npj Urban Sustain. 2, 12. doi:10.1038/s42949-022-00055-z
Yao, X., Zhang, M., Zhang, Y., Xiao, H., and Wang, J. (2021). Research on evaluation of climate comfort in northwest China under climate change. Sustainability 13, 10111. doi:10.3390/su131810111
Yin, W., Pan, Z., Pan, Y., Han, G., Wang, J., Huang, N., et al. (2018). The temporal and spatial characteristics of climatic comfortability on human settlement in mainland China. China Popul. Resour. Environ. 28 (7), 5–8.
Yu, Q., Shen, T., Wang, S., and Zhang, Y. (2021). Natural suitability evaluation of human settlement environment in the town of Huangshan City based on GIS. Environ. Pollut. Control 43 (7), 880–885. doi:10.15985/j.cnki.1001-3865.2021.07.015
Yüksel, A., Arici, M., Krajčík, M., and Karabay, H. (2020). Experimental investigation of thermal comfort and CO(2) concentration in mosques: A case study in warm temperate climate of yalova, Turkey. Sustain. Cities Soc. 52, 101809. doi:10.1016/j.scs.2019.101809
Zeng, D., Wu, J., Mu, Y., Li, H., Deng, M., Wei, Y., et al. (2020). An assessment of tourism climate comfort in the China-Pakistan economic corridor. Sustainability 12, 6981. doi:10.3390/su12176981
Zhang, C., Cao, X., and Ramaswami, A. (2016). A novel analysis of consumption-based carbon footprints in China: Unpacking the effects of urban settlement and rural-to-urban migration. Glob. Environ. Change 39, 285–293. doi:10.1016/j.gloenvcha.2016.06.003
Zhang, D., Zhou, C., Sun, D., and Qian, Y. (2022). The influence of the spatial pattern of urban road networks on the quality of business environments: The case of dalian city. Environ. Dev. Sustain. 24, 9429–9446. doi:10.1007/s10668-021-01832-z
Zhang, D., Zhou, C., Zhou, Y., and Zikirya, B. (2022). Spatiotemporal relationship characteristic of climate comfort of urban human settlement environment and population density in China. Front. Ecol. Evol. 10, 953725. doi:10.3389/fevo.2022.953725
Zhang, L., Guo, X ., Du, Y., and Li, W. (2020). Comments on the characteristics and its impact of weather and climate in Guangdong province from July to August in 2020. Guangdong meteorol. Inside front cover, inside back cover, 42. doi:10.3969/j.issn.1007-6190.2020.05.0205
Zhao, F., Lan, X., Li, W., Zhu, W., and Li, T. (2021). Influence of land use change on the Surface albedo and climate change in the qinling-daba mountains. Sustainability 13, 10153. doi:10.3390/su131810153
Zhao, J., and Wang, S. (2021). Spatio-temporal evolution and prediction of tourism comprehensive climate comfort in henan province, China. Atmosphere 12, 823. doi:10.3390/atmos12070823
Keywords: urban human settlement environment, climate comfort, temporal and spatial evolution, influencing factor, Guangdong-Hong Kong-Macau Greater Bay Area
Citation: Zhou C, Zhang D, Cao Y, Wang Y and Zhang G (2022) Spatio–temporal evolution and factors of climate comfort for urban human settlements in the Guangdong–Hong Kong–Macau Greater Bay Area. Front. Environ. Sci. 10:1001064. doi: 10.3389/fenvs.2022.1001064
Received: 22 July 2022; Accepted: 15 August 2022;
Published: 09 September 2022.
Edited by:
Bing Xue, Institute of Applied Ecology, (CAS), ChinaReviewed by:
Xiao Liu, South China University of Technology, ChinaCopyright © 2022 Zhou, Zhang, Cao, Wang and Zhang. This is an open-access article distributed under the terms of the Creative Commons Attribution License (CC BY). The use, distribution or reproduction in other forums is permitted, provided the original author(s) and the copyright owner(s) are credited and that the original publication in this journal is cited, in accordance with accepted academic practice. No use, distribution or reproduction is permitted which does not comply with these terms.
*Correspondence: Guojun Zhang, Z3VvanVuekBnZHVmZS5lZHUuY24=
Disclaimer: All claims expressed in this article are solely those of the authors and do not necessarily represent those of their affiliated organizations, or those of the publisher, the editors and the reviewers. Any product that may be evaluated in this article or claim that may be made by its manufacturer is not guaranteed or endorsed by the publisher.
Research integrity at Frontiers
Learn more about the work of our research integrity team to safeguard the quality of each article we publish.