- Department of Chemical and Materials Engineering, National Central University, Taoyuan, Taiwan
Management of spent mushroom substrate (SMS) is causing a global environmental concern due to tremendous increase in mushroom production globally. Therefore, in this research, the performance of a two-stage anaerobic co-digestion (TS-AD) of spent mushroom substrate and chicken manure was evaluated in terms of methane and biogas production and process stability with respect to single stage anaerobic digestion (SS-AD). Activation of anaerobic sludge using aeration or heat treatment in the first stage at mesophilic temperature followed by thermophilic co-digestion with chicken manure in the second stage was investigated. TS-AD exhibited better performance and enhanced methane generation over SS-AD. The optimal temperatures were determined as 35°C and 50°C for the first and the second stage of TS-AD, respectively. C/N ratio of 10 was the most suitable for biogas and methane production. TS-AD with C/N ratio of 10 and mesophilic digestion of SMS and sludge for 3 days at 35°C followed by co-digestion of the first stage effluent with chicken manure at 50°C was the optimized state producing 1359 mL of biogas of which 614.42 mL was methane, showing an increment by 59.44% in methane production as compared to SS-AD. TS-AD might be promising approach for utilization of SMS as feed stocks for biogas and methane production.
Introduction
Consumption of edible mushrooms has become very popular nowadays due to their nutritional value. As a result, the global production of mushroom has increased tremendously over the last few decades (Grimm and Wösten, 2018). Nevertheless, commercial production of mushroom has caused serious environmental concern with the generation of large volumes of solid residual left over after harvesting mushroom which is known as spent mushroom substrate (SMS). Correspondingly, 1 kg of mushroom produces 5 kg of SMS (Lam et al., 2019). Management of this waste has become a huge task for farmers and industries involved in mushroom industry as unmanaged SMS can cause toxic foul smell to the surroundings and pollution of air, water, and land (Mahari et al., 2020). So far, SMS has been utilized for composting (Mohd Hanafi et al., 2018), animal feed (Leong et al., 2022), firewood substitute, production of fertilizers (Doan et al., 2017), activated carbon and enzymes (Zhang et al., 2012; Grujić et al., 2015), etc. However, SMS is organic matter rich in semi-digested cellulose, hemicellulose, and low in lignin. Unlike other agricultural feedstock, SMS is available in abundance and throughout the year. Therefore, it appears as promising feedstock for the production of second-generation biofuels (Lin et al., 2017).
Anaerobic digestion (AD) technology is one of the most popular ecofriendly techniques for bioconversion of lignocellulosic waste into sustainable energy as it alleviates pollution by decreasing the amount of solid organic waste as well as assists in recovering energy (Wachemo et al., 2019; Pečar and Goršek, 2020). AD of SMS for production of methane seems an attractive approach. Recent studies show that co-digestion of SMS with animal manure results enhanced methane production as compared to the monodigestion of SMS (Luo et al., 2017). Co-digestion has great advantages over monodigestion especially high nutritional content, better buffering capacity, process stability, and optimal C/N ratio (Mata-Alvarez et al., 2014). Animal manure as co-digestion substrate has been widely regarded to improve the fermentation performances by increasing nutritional content and buffering abilities (Li et al., 2014). Anaerobic co-digestion involves a series of biochemical steps including hydrolysis, acidogenesis, acetogenesis, and methanogenesis (Xi et al., 2021). Usually, all these processes take place in a single bioreactor. However, it is believed that separation of a methanogenesis from acidogenesis and acetogenesis allows acetogenic and methanogenic microbial flora for a better adaptation, lowers VFA accumulation, improves the stability of the reactor, and thereby enhance methane yield (Belluci et al., 2019; Ghanimeh et al., 2019).
Alteration of operation conditions induces change in microbial diversity, composition, and activity in anaerobic digesters. Temperature is a key parameter that affects the performance of anaerobic digestion (Hu et al., 2018). Anaerobic co-digestion involves a diverse group of microbial flora such as hydrolytic, acidogenic, acetogenic, and methanogenic bacteria which require different temperature conditions for their growth and metabolism (Carballa et al., 2011). Therefore, temperature optimization has become an essential factor to make the AD more energy-efficient, productive, and stable (Westerholm et al., 2018). The biochemical composition of the feedstock determines the C/N ratio for the anaerobic digester. C/N ratio is another significant factor which can induce bioreactor efficiency (Dai et al., 2016). Therefore, our study involves optimizing both temperature and C/N ratio.
Despite extensive literature studies, we found limited research involving the application of two-stage anaerobic co-digestion of SMS and chicken manure for methane production. Management of an elevating abundance of SMS is very critical from an environmental point of view. Therefore, the main aim of this study is to study the conversion of SMS and chicken manure to biogas and methane using TS-AD and optimizing the operational parameters.
Materials and Methods
Collection of Feed Stocks and Inoculum
Spent mushroom substrate (SMS) used in this study was taken from Wufeng Dai Culture Farmer in Taichung area. SMS was crushed and ground with a pulverizer, sieved to a size of about 2.0 mm, and stored in a sealed bag at room temperature. SMS and chicken manure were characterized in terms of carbon and nitrogen content, total solids (TS), and volatile solids (VS) according to Standard Methods, APHA 2005 and the results shown in Table 1.
Anaerobic sludge was taken from the pond of the central livestock farm in Linluo District, Pingtung County. After retrieval, the cells were maintained through multiple generations of domestication.
Chicken manure (CM) was taken from Shunfeng Fertilizer Company in Xinwu District, Taoyuan City.
Pretreatment of SMS
Processed SMS was subjected to acid pretreatment using 5% HCl. For this part, 10 g of mushroom-wrapped wood chips were placed in a 1 L fermentation bottle, followed by addition of 50 mL of hydrochloric acid (5 vol%), and subsequently, autoclaved at 121°C for 60 min; 5% HCl hydrolysis of SMS was found to be the most favorable for pretreatment of SMS.
After autoclaving, pretreated SMS in the fermentation bottle was allowed to cool down to room temperature followed by adjusting the pH to 7.0 by addition of 1 M NaOH or 1 M HCl.
Sludge Treatment
For heat treatment, 200 mL of sludge was added in a beaker and allowed for magnetic stirring to slight boiling for 20 min. Some drops of water were added to get rid of bumping due to heating. Subsequently, the sludge was cooled to room temperature for use.
For aeration method, 200 mL of sludge was added in a 1 L fermentation bottle and air was continuously passed at 2 kgf/cm2 for 8 h.
Experimental Set up for Single-Stage Anaerobic Co-Digestion (SS-AD)
5 g of SMS was added to a 1 L fermentation bottle followed by 50 mL of 5% HCl. The mixture was stirred and autoclaved at 121°C for 60 min. After autoclaving, it was cooled to room temperature; 100 mL of activated sludge was poured followed by the addition of 5.34 g of chicken manure. pH of the mixture was adjusted at 7.0 by addition of 1 M NaOH or 1 M HCl. DI water was added to 600 mL working volume. Nitrogen was passed for 5 min to remove oxygen. The bioreactor was placed in an electric thermostatic water bath at 55°C, and fermentation was carried out for 7 days.
Two-Stage Anaerobic Co-Digestion on (TS-AD)
For the first stage, 5 g of SMS was added to a 1 L fermentation bottle followed by 50 mL of 5% HCl. The mixture was stirred and allowed to autoclave at 121°C for 60 min. After autoclaving, it was cooled to room temperature; 100 mL of activated VFA sludge was added and pH was adjusted to 5.5 by addition of 1 M NaOH or 1 M HCl. DI water was added to 600 mL working volume. Nitrogen was passed for 5 min to remove any remains of oxygen in the digester. First stage was operated for 3 days by placing the fermentation bottles in electric thermostatic water bath at 35°C.
For the second stage of fermentation, a peristaltic pump was used to introduce the hydrolysate from the first stage into another digester, which was filled with 9.6 g of chicken manure. Then, 100 mL of anaerobic sludge was poured followed by addition of DI water to maintain 600 mL working volume. Nitrogen was passed for 5 min to remove oxygen thereby creating an anaerobic environment. The bioreactor was placed in an electric thermostatic water bath at 55°C, and fermentation was carried out for 7 days.
Temperature Optimization Experiment
For the first stage, three bioreactors were prepared following the procedure as mentioned above in two-stage experimental designs and placed in electric thermostatic water baths at 25°C, 30°C, and 35°C for 3 days.
For the second stage, reactors were placed in electric thermostatic water baths at five different temperatures of 40°C, 45°C, 50°C, 55°C, and 60°C, and fermentation was carried out for 7 days.
C/N Ratio Optimization
Three different two stage reactors were designed such that the C/N ratio in the reactors were 10, 15, and 20 with the content of SMS and CM as shown in Table 2. TS-AD of all three reactors was performed with optimum temperature which meant the first stage temperature at 35°C and the second stage at 50°C.
Analytical Methods
Standard procedures (APHA, 2005) were used to evaluate carbon, nitrogen, total solids, and volatile solids for SMS. Biogas was considered as the volume of water displaced by gas and was measured daily using a measuring cylinder.
Liquid samples of 3 mL were withdrawn daily from bioreactors using a syringe in a 10 mL centrifuge tube. After measuring pH, the samples were centrifuged at 6000 rpm for 10 min. The supernatant liquid was filtered through a 0.45 µL filter, and 1 mL of liquid was collected for VFA analysis using gas chromatography. A 30-m × 0.25-mm 25-micron capillary column was loaded with a flame ionization detector (FID) of a DB-WAXETR gas chromatograph (China Chromatography, Type 9800). The injection, oven, and detector operating temperatures were set to 200°C, 130°C, and 220°C, respectively. Each sample was run for 8 min. VFA in our study is sum of acetic and propionic acids. Therefore, standard curves of acetic acid and propionic acids were prepared using standard solutions at varied concentrations to calculate VFA in the bioreactors.
Gas samples were withdrawn daily from the bioreactors using 1 mL gas tight syringe and injected into the Gas chromatograph equipped with a 30-m × 0.25-mm 25-micron capillary column and loaded with a flame ionization detector (FID) of a DB-WAXETR gas chromatograph (China Chromatography, Type 9800). The injection, oven, and detector operating temperatures were set to 200°C, 70°C, and 220°C, respectively. Gas samples were analyzed for methane. Standard methane curve was plotted using pure methane gas at various concentrations. Methane in gas samples was estimated with respect to the standard curve.
All the experiments were performed in triplicates and the results were expressed in terms of means ± standard deviation. All the figures in this research were plotted using the Origin Pro 2016 software.
Results and Discussion
Comparison of Methane Yield From Monodigestion of SMS with Co-digestion with Chicken Manure
Monodigestion of SMS and co-digestion of SMS with CM produced 460 and 557 mL of biogas, respectively, on Day 1 which was found as the highest on a single day for 7-day fermentation (Figures 1A,B). Daily biogas production rapidly decreased over the next 6 days in a similar pattern. Meanwhile methane content which started at 33.16% and 36.48%, respectively, on day 1 for mono and co-digesters showed an increasing trend. Monodigestion of SMS and co-digestion of SMS with chicken manure produced the highest methane content as 62.2% and 71.11%, respectively, on Day 4. Also, methane formation remained steady for co-digestion, but there was a sharp decline for the monodigester. Furthermore, both reactors showed a fall in pH for the first 3 days and then steadied at 7–7.3, which is consistent with the results expressed in co-digestion of SMS and corn stover (Zhu et al., 2015). pH change is not too significant for both reactors. Cumulative methane production by co-digestion was 177.35 mL more than monodigestion (Figure 1C).
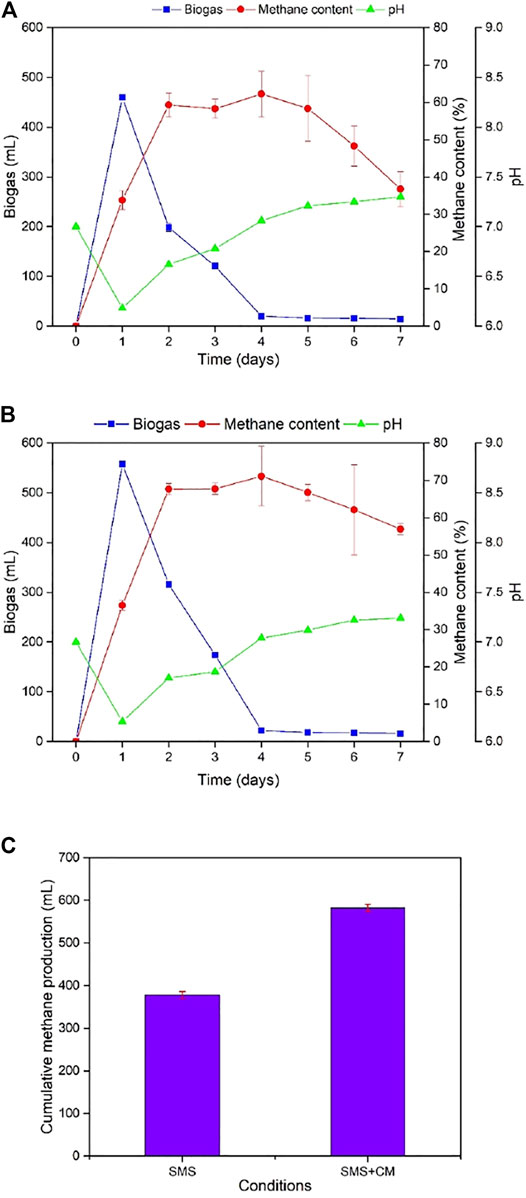
FIGURE 1. Daily biogas production, methane content, and pH for (A) monodigestion and (B) co-digestion. (C) Cumulative methane production for the monodigestion and co-digestion processes.
AD has been widely used to degrade household, agricultural, and industrial waste to produce biogas rich in methane content (Wang et al., 2014). However, monodigestion of SMS might have suffered lower nutritional contents, poor buffering ability, and therefore lesser biogas yield. An effort to boost nutritional content and improve buffering capacity was achieved by co-digestion of SMS with chicken manure, and the results supported the approach. Therefore, anaerobic co-digestion of SMS with chicken manure was employed in the further operations in this study.
Effects of Sludge Treatment on the Anaerobic Co-digesters
Aeration and heat treatment methods were used for sludge treatment in this study, and the results are demonstrated in Figure 2. The treatment methods showed a significant difference in daily biogas production and methane content. Aerated digester and heat-treated digesters produced 515 and 180 mL of biogas, respectively, on Day 1. Although decrease in biogas was found in both digesters, the heat-treated digester had nominal biogas production after Day 3. Moreover, a large amount of methane was detected in the gas samples of the aerated group, and the highest methane content reached 72%, implying that the continuous aeration of the sludge under oxygen failed to remove methanogens. Only a trace amount of methane was detected in the heat treated group, and the highest value was only 5.8%, thereby proving that the method of heat treatment successfully removed the methanogens from the anaerobic fermentation sludge.
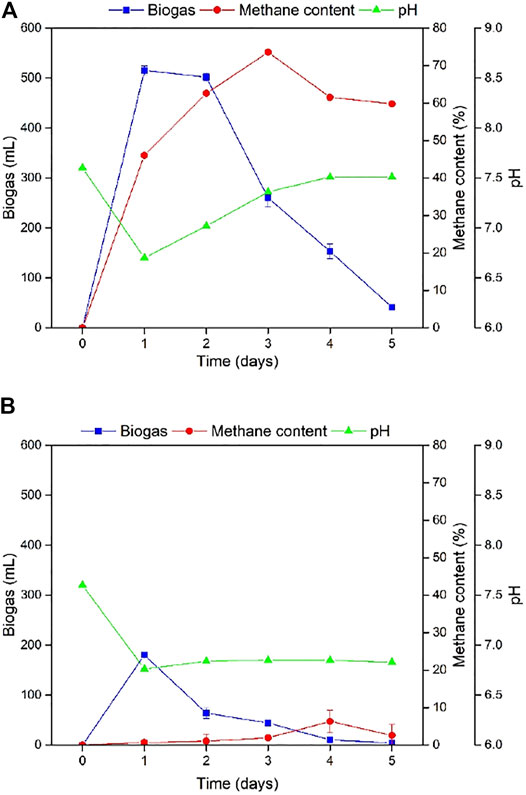
FIGURE 2. Daily biogas production, methane content, and pH for fermenter using (A) heat treated sludge and (B) aerated sludge.
Both aerated and heat-treated sludge digesters showed a significant pH drop on Day 1, from an initial pH value of 7.6 to 6.70 and 6.76, respectively. However, pH continued to increase after day 1 for aerated sludge digester until the end of the experiment with rapid increment on Day 1 and got stable on Days 4 and 5. The heat-treated sludge digester showed stable pH after Day 1. Both groups of experiments showed a clear trend of decreasing pH on day 1, indicating hydrolysis during fermentation. Volatile fatty acids produced by acid-producing bacteria caused pH of the fermentation broth to decrease. Continuous increase in pH exhibited by the aerated group means that volatile fatty acids were consumed for methane conversion while stable pH in the heat-treated group indicates that volatile fatty acids were not utilized for methane conversion. Acidogens can develop spore to survive extreme conditions like heat treatment and this can be used as a strategy to get rid of methanogens (Wainaina et al., 2019). Based on these results, heat-treated sludge was used for further experiments as the strategy was to allow hydrolytic microorganisms to survive and inhibit methane production in the initial stage.
Comparison of Fermentation Performances for SS-AD and TS-AD
Biogas production, methane content, pH value, and volatile fatty acids were used to evaluate the performances of SS-AD and TS-AD. The highest biogas value of 555 and 495 mL for SS-AD and TS-AD, respectively, appeared on day 1 and then continued to decrease (Figure 3). However, TS-AD had better performance in terms of methane content producing 68.65% on the final day. Therefore, it can be implied that TS-AD operation can obtain higher methane concentration in the middle and early stages of fermentation. This might be related with the abundantly available VFAs. Since TS-AD group accumulated more VFAs during the hydrolysis and acid generation stage, it had higher VFAs that were utilized by the methanogens during the methanogenesis stage, thereby increasing methane content rapidly in the first and middle stages. Contrasting pH changes were observed for SS-AD and TS-AD. pH of SS-AD first decreased from 7.6 to 6.86 on Day 1 and then continued to increase until the end of fermentation. pH continued to rise for TS-AD. Conversion of raw materials into VFAs was completed after 3 days of hydrolysis and acid production in the first stage for the TS-AD group. Consumption of VFA continued to rise in the second stage and hence the trend of increasing pH value continued in TS-AD. In the single-stage anaerobic fermentation, the substrates were first converted into volatile fatty acids causing a significant pH drop on Day 1.
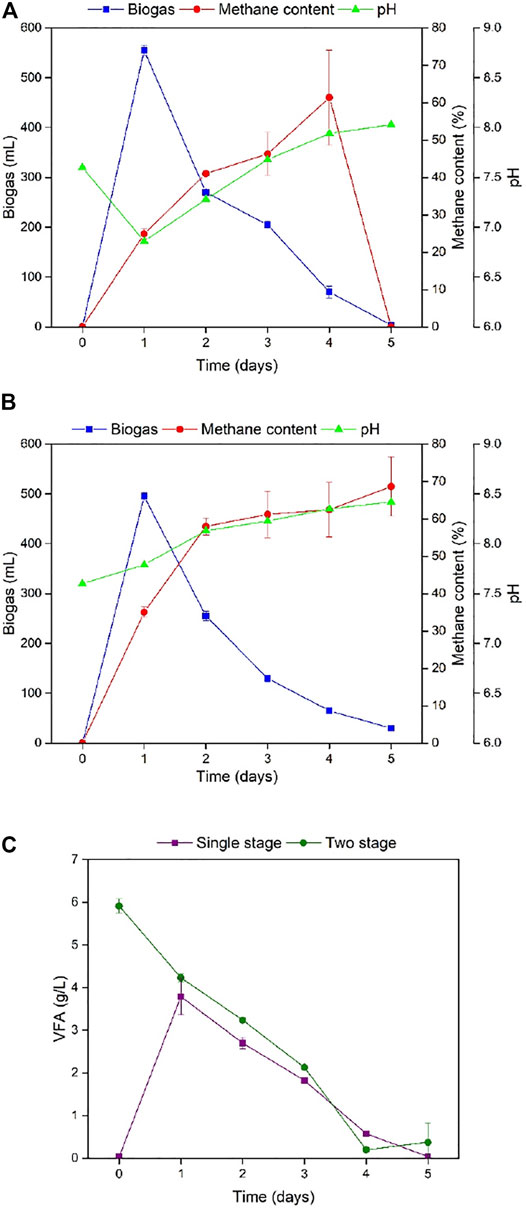
FIGURE 3. Daily biogas production, methane content, and pH for (A) SS-AD and (B) TS-AD; (C) VFA accumulation for SS-AD and TS-AD (SS-AD = Single stage anaerobic digestion, TS-AD = Two stage anaerobic digestion; VFA = Volatile fatty acids).
It can be deciphered from Figure 3 that before the TS-AD group entered the methanogenesis stage, a large amount of VFAs had been accumulated due to the action of hydrolytic and acidogenic bacteria, and VFA concentration reached 5.91 g/L in the first stage. During the second stage, VFAs were continuously consumed by methanogens. In SS-AD, the highest VFAs were accumulated on Day 1 at 3.79 g/L and then decreased continuously due to methanogenesis. In the first 3 days of fermentation, VFAs concentration of the TS-AD group was more than that of SS-AD. Higher VFAs accumulation in TS-AD than SS-AD can be attributed to the separation of the hydrolytic, acidogenic, and acetogenic phases from the methanogenic phase. Separation of methanogens from the acidogenic and acetogenic phases in TS-AD also allowed the microorganism to tolerate more VFA in TS-AD as methanogens are more susceptible to high concentration of VFAs. Therefore, it can be implied that TS-AD is more energy efficient and stable than SS-AD and hence employed for further optimization.
Optimization of Temperature for TS-AD
Temperature is a key operational factor that affects the performance of biogas digesters (Labatut et al., 2014; De Vrieze et al., 2015; Pap et al., 2015). For the first stage of fermentation, the effects of three different temperatures, 25°C, 30°C, and 35°C, on biogas production, VFAs accumulation, and pH were studied. For the second stage, the effects of five different temperatures, 40°C, 45°C, 50°C, 55°C, and 60°C on daily and total biogas production, methane yield, and pH change, were investigated (Figure 4 and Table 3).
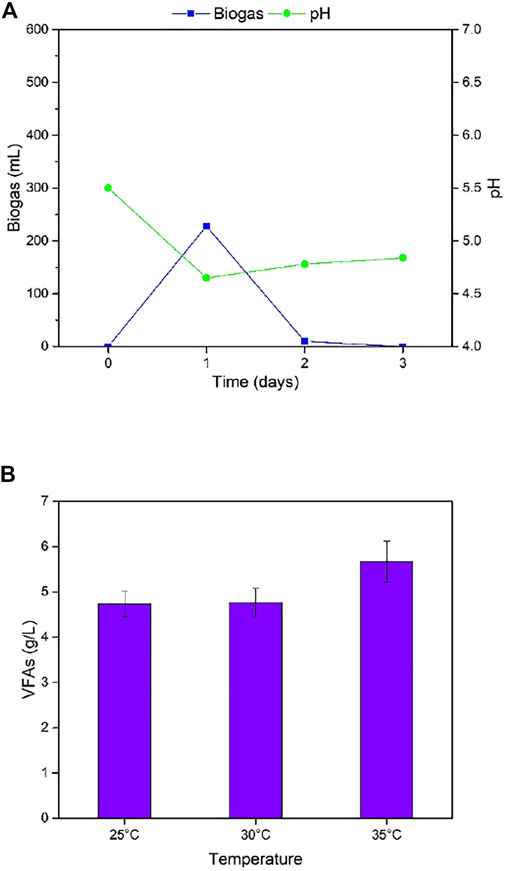
FIGURE 4. (A) Daily biogas production and pH for the first stage of TS-AD at 35°C (B) Total VFA accumulations for the first stage of TS-AD operated at different temperatures (VFA = Volatile fatty acids, TS-AD = Two stage anaerobic digestion).

TABLE 3. Influence of temperature on the performance of the first stage of anaerobic digester of TS-AD
Biogas production in the first stage of TS-AD was highest on Day 1 for all three reactors at different temperatures and then decreased. The digester at 35°C produced 228 mL of biogas which was 149 and 98 mL more than digesters at 25°C and 30°C, respectively. One of the main purpose of TS-AD is the generation of higher content of VFAs in the first stage so that they can be converted to methane in the second stage. VFAs accumulation was highest at 35°C reaching 5.67 g/L. Fall in pH was observed for all three temperatures due to the accumulation of volatile fatty acids in the first stage. pH decreased from 5.5 to 4.76, 4.71, and 4.65 for 25°C, 30°C, and 35°C, respectively, which is consistent with the results for VFAs accumulation. These results highlight the fact that higher temperatures of 30°C and 35°C enhance the activity of hydrolytic acid-producing bacteria, allowing them to complete the conversion of reducing sugars to VFA. Low temperatures affect hydrolytic acid-producing bacteria and the metabolism is relatively slow.
The second stage of TS-AD is basically meant for rapid consumption of VFAs by methanogens to produce methane. It has been widely known that thermophilic anaerobic digestion offers accelerated biochemical reactions and highly efficient organic matter degradation as compared to the mesophilic process (Zábranská et al., 2000; Moset et al., 2015). Since methanogenic bacteria can adapt themselves at different temperatures (Yu et al., 2014), the second stage was operated at thermophilic temperature. Temperature of the second stage affected methanogenic activities for biogas production as shown in Figure 5 and Table 4. Digester at 60°C reached the maximum single-day gas production of 515 mL on Day 1, which was the highest single-day gas production in the five groups of experiments and the earliest group to reach the peak value for biogas production. During the subsequent fermentation, although daily gas production decreased rapidly, the gas production was maintained for 5 days. The highest daily gas production in 55°C digester was 480 mL. Unlike the 60°C digester which showed a sharp decrease in biogas on Day 2, the 55°C digester showed higher daily gas production for Days 1 and 2. The maximum daily gas production of the 50°C digester was 435 mL, which appeared on Day 2. The 50°C digester maintained better performance for the first 3 days, and gas formation continued for 7 days. The maximum gas production for the 45°C digester was 265 mL, which was very low as compared with other groups at high temperatures. However, the 45°C digester did not show a rapid decline after reaching its peak, even rebounded on day 4, and the final biogas production lasted for 7 days. Biogas production trend of the 40°C digester was completely different from the previous high-temperature group. Daily maximum gas production was only 135 mL, but it maintained consistent performance over the entire 7 days. The change was minimal, and biogas production was more evenly distributed.
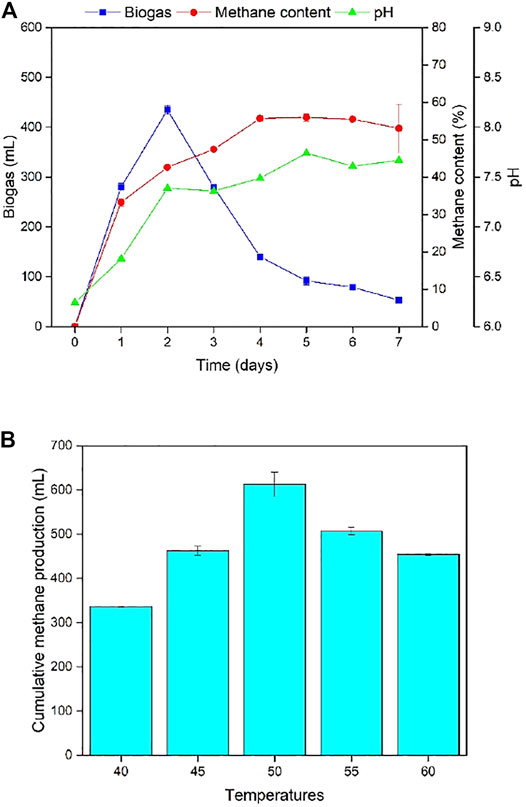
FIGURE 5. (A) Daily biogas production, methane content, and pH for the second stage of TS-AD at 50°C. (B) Cumulative methane production for the second stage of TS-AD operated at different temperatures (TS-AD = Two stage anaerobic digestion).
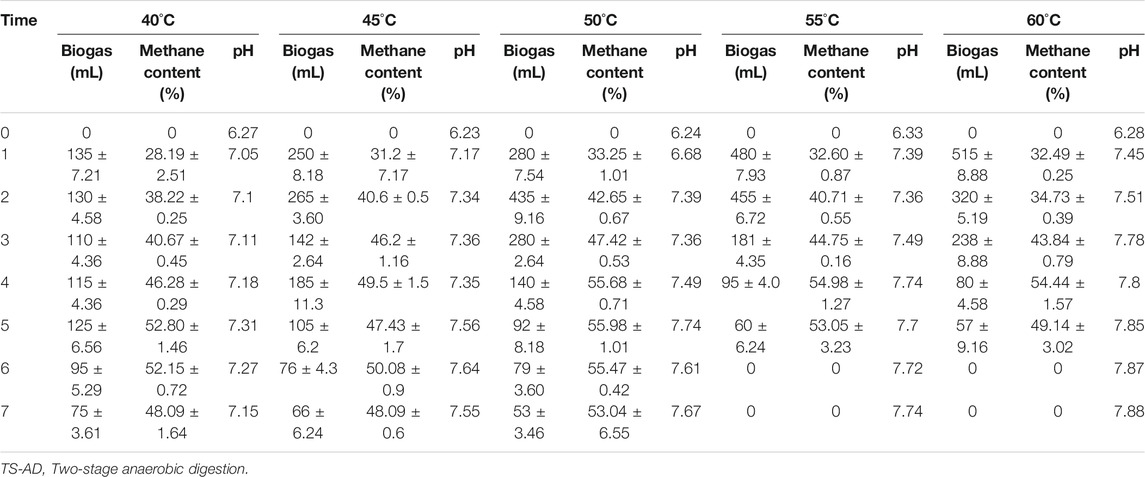
TABLE 4. Influence of temperature on the performance of the second stage of anaerobic digester of TS-AD
Methane production is a very critical parameter for evaluating anaerobic fermentation. Methane content in the five groups of experiments was first increased and then stabilized; however, their final methane content was different. The 50°C group had the highest concentration with 56% methane content producing 613 mL methane which was higher by 275.65, 150.35, 106.24, and 158.75 mL for digesters at 40°C, 45°C, 55°C, and 60°C, respectively. Methanogens are very sensitive to pH, so analysis of pH change was considered very important. pH value in the second stage showed an increasing trend due to bioconversion of VFAs into methane. It was also found that temperature had a correlation with pH value; the higher the temperature was, the higher the final pH value was.
Methanogenic microbial consortia are highly susceptible to temperature. Although higher temperature operation led to increased microbial activity, there was rapid decrement in the performance after reaching the peak. Lower temperature can maintain fermentation operation for a longer time, but the speed will be slower in higher temperature; 50°C was the most suitable temperature for the growth of methanogenic bacteria and the synthesis of biogas and methane. Therefore, the optimized temperatures for the first and second stage were deciphered as 35°C and 50°C, respectively, for TS-AD operation.
Optimization of C/N Ratio in TS-AD
C/N ratio refers to the ratio of carbon source to nitrogen source in the substrate. Maintaining a proper C/N ratio helps to balance the nutrients in the fermentation environment, so that microorganisms can grow better and produce desired products (Okonkwo et al., 2018). Too high or too low C/N ratio can be detrimental to biogas production as it can probably cause acidification or ammonia inhibition (Molinuevo-Salces et al., 2012; Wang et al., 2012). Three C/N ratios 10, 15, and 20 were studied. Figure 6 and Table 5 illustrate C/N ratio has a significant effect on biogas production. Fermenters with a C/N ratio of 10 had the best single-day biogas production of 435 mL. This peak occurred on Day 2 of fermentation. At the same time, the digester with a C/N ratio of 10 had a superior performance than the other two digesters in terms of biogas production for the entire fermentation process. Digesters with a C/N ratio of 15 were very close to C/N 10. The peak value of biogas for the C/N ratio of 20 was 265 mL, and appeared on Day 1.
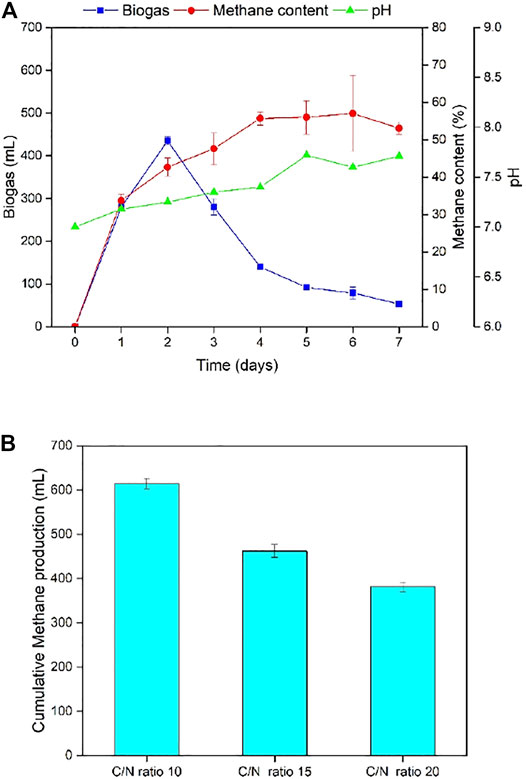
FIGURE 6. (A) Daily biogas production, methane content, and pH for the C/N ratio of 10. (B) Cumulative methane production for TS-AD operated at different C/N ratios.
Methane content trends of the three C/N ratios exhibited rapid rise initially and subsequently got stable between 50% and 60%, accompanied by some fluctuations at the end of fermentation. The digester with a C/N ratio of 10 reached a concentration of 33.7% on Day 1 and continued to rise to 57%, which was the highest among all the three groups. pH change in the methanogenesis stage at different C/N ratio was very likely. Usually, in anaerobic fermentation, more carbon sources mean that more VFA may be produced, thereby lowering pH. On the contrast, more nitrogen sources mean that the content of ammonia-nitrogen substances increases, which may increase pH. Experimental results showed pH for different C/N ratios increased with a similar trend, and the final pH value was in the range of 7.6–7.8, suggesting nominal differences. Study of the cumulative methane production shed more light on the effects of C/N ratio (Figure 6B). Total methane output of TS-AD with a C/N ratio of 10 was 614.42 mL and consistent with earlier experiment for temperature optimization.
Performance Comparison of the Three Different Fermentations
Although cumulative methane yield for monodigestion of SMS is the highest, methane production rate was the lowest among all three fermentation conditions. Cumulative methane yield for TS-AD operated at a C/N ratio of 10 was 84.3 mL/g VS which was higher than SS-AD by 7.5 mL/g VS (Table 6). Methane production rate was also higher for TS-AD than SS-AD confirming that TS-AD had the best performance for methane production. Based on these findings, the C/N ratio of 10 was the optimum condition for higher biogas and methane production as the microbial consortia had the most favorable nutrient content and cultural conditions at a C/N ratio of 10.
Conclusion
Rapid abundance of SMS can be managed by the utilization of SMS as feed stocks for the generation of biogas and methane. This can be beneficial for circular economy as well as reduce the toxicity and pollution to the environment. Co-digestion of SMS and CM is a feasible approach as the process generated higher quantity of methane and biogas; the process was more stable as compared to monodigestion of SMS. TS-AD can be a highly efficient method for biogas and methane production. Heat treatment of sludge is capable of inhibiting methanogenic activity in the initial stage of fermentation and therefore helps in higher VFA production. Temperature plays a vital role in both stages of TS-AD with the optimum temperature at 35°C for the first stage and 50°C for the second stage. C/N ratio influences the outcome of the anaerobic digestion process and hence requires optimization.
Data Availability Statement
The raw data supporting the conclusions of this article will be made available by the authors, without undue reservation.
Author Contributions
Conceptualization and methodology: C-HS and RJ; formal analysis and investigation: RJ, M-dK, and B-HY; writing original draft: RJ; writing, review, editing, and supervision: C-HS.
Funding
The authors would like to gratefully acknowledge the financial support of the Ministry of Science and Technology of the Republic of China (MOST 110-2622-E-008-019).
Conflict of Interest
The authors declare that the research was conducted in the absence of any commercial or financial relationships that could be construed as a potential conflict of interest.
Publisher’s Note
All claims expressed in this article are solely those of the authors and do not necessarily represent those of their affiliated organizations, or those of the publisher, the editors, and the reviewers. Any product that may be evaluated in this article, or claim that may be made by its manufacturer, is not guaranteed or endorsed by the publisher.
Supplementary Material
The Supplementary Material for this article can be found online at: https://www.frontiersin.org/articles/10.3389/fenvs.2021.810678/full#supplementary-material
Abbreviations
AD, Anaerobic digestion; CM, Chicken manure; C/N ratio, Carbon/nitrogen ratio; SMS, Spent mushroom substrate; SS-AD, Single stage anaerobic digestion; TS, Total solids; TS-AD, Two stage anaerobic digestion; VFA, Volatile fatty acids; VS, Volatile solids.
References
APHA (2005). Standard Methods for the Examination of Water and Wastewater. 21th ed. Washington, DC: American Health Public association.
Bellucci, M., Borruso, L., Brusetti, L., Russo, P., and Beneduce, L. (2019). Microbial Community Dynamics and Process Performance of a Full Scale Two‐stage Anaerobic Digester under the Replacement from Energy Crop to Poultry Manure. J. Chem. Technol. Biotechnol. 95, 1064–1072. doi:10.1002/jctb.6286
Carballa, M., Smits, M., Etchebehere, C., Boon, N., and Verstraete, W. (2011). Correlations between Molecular and Operational Parameters in Continuous Lab-Scale Anaerobic Reactors. Appl. Microbiol. Biotechnol. 89, 303–314. doi:10.1007/s00253-010-2858-y
Dai, X., Li, X., Zhang, D., Chen, Y., and Dai, L. (2016). Simultaneous Enhancement of Methane Production and Methane Content in Biogas from Waste Activated Sludge and Perennial Ryegrass Anaerobic Co-digestion: The Effects of pH and C/N Ratio. Bioresour. Tech. 216, 323–330. doi:10.1016/j.biortech.2016.05.100
De Vrieze, J., Saunders, A. M., He, Y., Fang, J., Nielsen, P. H., Verstraete, W., et al. (2015). Ammonia and Temperature Determine Potential Clustering in the Anaerobic Digestion Microbiome. Water Res. 75, 312–323. doi:10.1016/j.watres.2015.02.025
Doan, H. V., Hoseinifar, S. H., Tapingkae, W., Chitmanat, C., and Mekchay, S. (2017). Effects of Cordyceps Militaris Spent Mushroom Substrate on Mucosal and Serum Immune Parameters, Disease Resistance and Growth Performance of Nile tilapia, ( Oreochromis niloticus ). Fish Shellfish Immunol. 67, 78–85. doi:10.1016/j.fsi.2017.05.062
Ghanimeh, S., Al-Sanioura, D., Saikaly, P., and El-Fadel, M. (2019). Comparison of Single-Stage and Two-Stage Thermophilic Anaerobic Digestion of SS-OFMSW during the Start-Up Phase. Waste Biomass Valor. 11, 6709–6716. doi:10.1007/s12649-019-00891-8
Grimm, D., and Wösten, H. A. B. (2018). Mushroom Cultivation in the Circular Economy. Appl. Microbiol. Biotechnol. 102, 7795–7803. doi:10.1007/s00253-018-9226-8
Grujić, M., Dojnov, B., Potočnik, I., Duduk, B., and Vujčić, Z. (2015). Spent Mushroom Compost as Substrate for the Production of Industrially Important Hydrolytic Enzymes by Fungi Trichoderma Spp. And Aspergillus niger in Solid State Fermentation. Int. Biodeterior. Biodegrad. 104, 290–298. doi:10.1016/j.ibiod.2015.04.029
Hu, Y., Kobayashi, T., Qi, W., Oshibe, H., and Xu, K.-Q. (2018). Effect of Temperature and Organic Loading Rate on Siphon-Driven Self-Agitated Anaerobic Digestion Performance for Food Waste Treatment. Waste Manage. 74, 150–157. doi:10.1016/j.wasman.2017.12.016
Labatut, R. A., Angenent, L. T., and Scott, N. R. (2014). Conventional Mesophilic vs. Thermophilic Anaerobic Digestion: a Trade-Off between Performance and Stability? Water Res. 53, 249–258. doi:10.1016/j.watres.2014.01.035
Lam, S. S., Lee, X. Y., Nam, W. L., Phang, X. Y., Liew, R. K., Yek, P. N., et al. (2019). Microwave Vacuum Pyrolysis Conversion of Waste Mushroom Substrate into Biochar for Use as Growth Medium in Mushroom Cultivation. J. Chem. Technol. Biotechnol. 94, 1406–1415. doi:10.1002/jctb.5897
Leong, Y. K., Ma, T.-W., Chang, J.-S., and Yang, F.-C. (2022). Recent Advances and Future Directions on the Valorization of Spent Mushroom Substrate (SMS): A Review. Bioresour. Tech. 344, 126157. doi:10.1016/j.biortech.2021.126157
Li, J., Wei, L., Duan, Q., Hu, G., and Zhang, G. (2014). Semi-continuous Anaerobic Co-digestion of Dairy Manure with Three Crop Residues for Biogas Production. Bioresour. Tech. 156, 307–313. doi:10.1016/j.biortech.2014.01.064
Lin, H.-N., Wang, Y.-T., and Zhu, M.-J. (2017). Evaluation of Spent Mushroom Compost as a Lignocellulosic Substrate for Hydrogen Production by Clostridium Thermocellum. Int. J. Hydrogen Energ. 42, 26687–26694. doi:10.1016/j.ijhydene.2017.09.040
Luo, X., Yuan, X., Wang, S., Sun, F., Hou, Z., Hu, Q., et al. (2017). Methane Production and Characteristics of the Microbial Community in the Co-digestion of Spent Mushroom Substrate with Dairy Manure. Bioresour. Technol. 250, 611–620. doi:10.1016/j.biortech.2017.11.088
Mahari, W. A. W., Peng, W., Nam, W. L., Yang, H., Lee, X. Y., Lee, Y. K., et al. (2020). A Review on Valorization of Oyster Mushroom and Waste Generated in the Mushroom Cultivation Industry. J. Hazard. Mater. 400, 123156. doi:10.1016/j.jhazmat.2020.123156
Mata-Alvarez, J., Dosta, J., Romero-Güiza, M. S., Fonoll, X., Peces, M., and Astals, S. (2014). A Critical Review on Anaerobic Co-digestion Achievements between 2010 and 2013. Renew. Sustain. Energ. Rev. 36, 412–427. doi:10.1016/j.rser.2014.04.039
Mohd Hanafi, F. H., Rezania, S., Mat Taib, S., Md Din, M. F., Yamauchi, M., Sakamoto, M., et al. (2018). Environmentally Sustainable Applications of Agro-Based Spent Mushroom Substrate (SMS): an Overview. J. Mater. Cycles Waste Manag. 20, 1383–1396. doi:10.1007/s10163-018-0739-0
Molinuevo-Salces, B., González-Fernández, C., Gómez, X., García-González, M. C., and Morán, A. (2012). Vegetable Processing Wastes Addition to Improve Swine Manure Anaerobic Digestion: Evaluation in Terms of Methane Yield and SEM Characterization. Appl. Energ. 91, 36–42. doi:10.1016/j.apenergy.2011.09.010
Moset, V., Poulsen, M., Wahid, R., Højberg, O., and Møller, H. B. (2015). Mesophilic versus Thermophilic Anaerobic Digestion of Cattle Manure: Methane Productivity and Microbial Ecology. Microb. Biotechnol. 8, 787–800. doi:10.1111/1751-7915.12271
Okonkwo, U. C., Onokpite, E., and Onokwai, A. O. (2018). Comparative Study of the Optimal Ratio of Biogas Production from Various Organic Wastes and Weeds for Digester/restarted Digester. J. King Saud Univ. - Eng. Sci. 30, 123–129. doi:10.1016/j.jksues.2016.02.002
Pap, B., Györkei, Á., Boboescu, I. Z., Nagy, I. K., Bíró, T., Kondorosi, É., et al. (2015). Temperature-dependent Transformation of Biogas-Producing Microbial Communities Points to the Increased Importance of Hydrogenotrophic Methanogenesis under Thermophilic Operation. Bioresour. Tech. 177, 375–380. doi:10.1016/j.biortech.2014.11.021
Pečar, D., and Goršek, A. (2020). Kinetics of Methane Production during Anaerobic Digestion of Chicken Manure with Sawdust and Miscanthus. Biomass Bioenergy 143, 105820. doi:10.1016/j.biombioe.2020.105820
Wachemo, A. C., Tong, H., Yuan, H., Zuo, X., Korai, R. M., and Li, X. (2019). Continuous Dynamics in Anaerobic Reactor during Bioconversion of rice Straw: Rate of Substance Utilization, Biomethane Production and Changes in Microbial Community Structure. Sci. Total Environ. 687, 1274–1284. doi:10.1016/j.scitotenv.2019.05.411
Wainaina, S., LukitawesaKumar Awasthi, M., Kumar Awasthi, M., and Taherzadeh, M. J. (2019). Bioengineering of Anaerobic Digestion for Volatile Fatty Acids, Hydrogen or Methane Production: A Critical Review. Bioengineered 10, 437–458. doi:10.1080/21655979.2019.1673937
Wang, F., Hidaka, T., and Tsumori, J. (2014). Enhancement of Anaerobic Digestion of Shredded Grass by Co-digestion with Sewage Sludge and Hyperthermophilic Pretreatment. Bioresour. Tech. 169, 299–306. doi:10.1016/j.biortech.2014.06.053
Wang, X., Yang, G., Feng, Y., Ren, G., and Han, X. (2012). Optimizing Feeding Composition and Carbon-Nitrogen Ratios for Improved Methane Yield during Anaerobic Co-digestion of Dairy, Chicken Manure and Wheat Straw. Bioresour. Tech. 120, 78–83. doi:10.1016/j.biortech.2012.06.058
Westerholm, M., Isaksson, S., Karlsson Lindsjö, O., and Schnürer, A. (2018). Microbial Community Adaptability to Altered Temperature Conditions Determines the Potential for Process Optimisation in Biogas Production. Appl. Energ. 226, 838–848. doi:10.1016/j.apenergy.2018.06.045
Xi, Y., Liu, Y., Ye, X., Du, J., Kong, X., Guo, D., et al. (2021). Enhanced Anaerobic Biogas Production from Wheat Straw by Herbal-Extraction Process Residues Supplementation. Front. Bioeng. Biotechnol. 9, 623594. doi:10.3389/fbioe.2021.623594
Yu, D., Kurola, J. M., Lähde, K., Kymäläinen, M., Sinkkonen, A., and Romantschuk, M. (2014). Biogas Production and Methanogenic Archaeal Community in Mesophilic and Thermophilic Anaerobic Co-digestion Processes. J. Environ. Manage. 143, 54–60. doi:10.1016/j.jenvman.2014.04.025
Zábranská, J., Stepová, R., Wachtl, R., Jenícek, P., and Dohányos, M. (2000). The Activity of Anaerobic Biomass in Thermophilic and Mesophilic Digesters at Different Loading Rates. Water Sci. Technol. 42, 49–56. doi:10.2166/wst.2000.0168
Zhang, R.-H., Duan, Z.-Q., and Li, Z.-G. (2012). Use of Spent Mushroom Substrate as Growing media for Tomato and Cucumber Seedlings. Pedosphere 22, 333–342. doi:10.1016/s1002-0160(12)60020-4
Keywords: spent mushroom substrate, chicken manure, anaerobic co-digestion, biomethane, two-stage anaerobic digestion
Citation: Shu C-, Jaiswal R, Kuo M- and Yu B- (2022) Enhancing Methane Production in a Two-Stage Anaerobic Digestion of Spent Mushroom Substrate and Chicken Manure via Activation of Sludge, Optimization of Temperature, and C/N Ratio. Front. Environ. Sci. 9:810678. doi: 10.3389/fenvs.2021.810678
Received: 07 November 2021; Accepted: 28 December 2021;
Published: 10 February 2022.
Edited by:
Bor-Yann Chen, National Ilan University, TaiwanReviewed by:
Surendra Sarsaiya, Zunyi Medical University, ChinaTao Sheng, Heilongjiang University of Science and Technology, China
Copyright © 2022 Shu, Jaiswal, Kuo and Yu. This is an open-access article distributed under the terms of the Creative Commons Attribution License (CC BY). The use, distribution or reproduction in other forums is permitted, provided the original author(s) and the copyright owner(s) are credited and that the original publication in this journal is cited, in accordance with accepted academic practice. No use, distribution or reproduction is permitted which does not comply with these terms.
*Correspondence: Chin-Hang Shu, Y2hpbnNodUBuY3UuZWR1LnR3