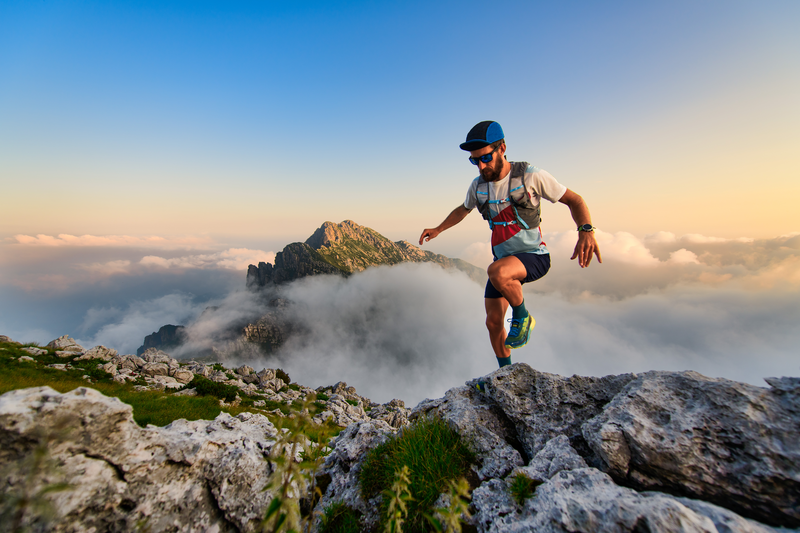
94% of researchers rate our articles as excellent or good
Learn more about the work of our research integrity team to safeguard the quality of each article we publish.
Find out more
BRIEF RESEARCH REPORT article
Front. Environ. Sci. , 08 December 2021
Sec. Freshwater Science
Volume 9 - 2021 | https://doi.org/10.3389/fenvs.2021.806697
This article is part of the Research Topic The Urban Fluvial and Hydro-Environment System View all 21 articles
Green roof systems could help reduce peak discharge and retain rainwater in urban areas. The objective of this study was to investigate the hydrological behavior of a green roof system by using the SEEP/W model. The rainfall-runoff relationship within the green roof system was simulated and the results were compared with actual data from a test bed for green roof systems to verify the applicability of SEEP/W. Then, the verified SEEP/W model was used to simulate the green roof system by varying four factors (soil type, rainfall intensity, substrate depth, and green roof slope) to explore the hydrological performance through the peak discharge to rainfall intensity (PD/RI) ratio and the rain water retention rate. The results show that the model presents slightly faster and greater peak time and peak discharge values, respectively, as compared to the observational data. This is attributed to the vegetation conditions in the real green roof system. However, it is also shown that the SEEP/W model can be used to design green roof systems and evaluate their hydrological behavior because of its modeling efficiency. Thus, the SEEP/W model can be used to reliably design and manage green roof systems by further considering the vegetation conditions and water flow dynamics. Furthermore, it would be desirable to consider additional factors, such as vegetation and an insulating pebble layer, in the design and management of green roofs in future work.
The acceleration of urbanization has induced the stronger impermeability of the subsurface, as farmland, green land, and forest have been replaced by buildings, roads, and pavement (Mentens et al., 2006; Li et al., 2018; Yan et al., 2019). The main environmental problem caused by these impermeable sub-surfaces is that urban hydrological systems should manage a highly fluctuating runoff, which is significantly high during stormwater season (White, 2002). Conventional stormwater systems have only been used for flood reduction and management, while the environmental problems related to urbanization have not been considered (Carter and Fowler, 2008). However, green roof systems can be an effective and eco-friendly rainfall management tool for problems related to uncertain water flows. In addition, green roof systems can also provide environmental benefits such as rainwater retention, peak discharge reduction, and runoff delay (Getter and Rowe, 2006; Feitosa and Wilkinson, 2016; Soulis et al., 2017; Shafique et al., 2018). They can also contribute to reducing the risk of overflow in runoff drainage systems for urban areas (Mentens et al., 2006; Stovin et al., 2017).
Previously, several studies have been conducted to explore how green roofs can absorb rainwater in their planting media, substrate, and drain layer (Scholz, 2004; Teemusk and Mander, 2007; Hilten et al., 2008; Fioretti et al., 2010; Ouldboukhitine et al., 2012; Vijayaraghavan, 2016; Baryła et al., 2018). For instance, Vijayaraghavan (2016) focused on green roof components, such as vegetation, growth substrate, filter layer, and drainage layer, for water absorption and Baryła et al. (2018) investigated the role of substrate in limiting rainwater runoff by assessing its absorption capacity. In addition, Ouldboukhitine et al. (2012) reported that vegetated roof systems, which are a type of green roof system, can reduce the total volume of runoff and peak discharge and can delay the runoff peak time. This helps to mitigate the potential risk of flash flooding, which can be an impact of heavy rain in urban areas (Villarreal et al., 2004). Green roofs have been shown to reduce the total volume of runoff by 60–100%, depending on the type of green roof used (Liesecke, 1998; Moran et al., 2003; Rowe et al., 2003; VanWoert et al., 2005; DeNardo et al., 2005; Hathaway et al., 2008; Speak et al., 2013). Furthermore, the runoff peak time can be delayed by periods ranging from 95 min to 4 h, compared with bare roofs (Liu, 2003; Moran et al., 2003; Simmons et al., 2008; Stovin et al., 2012).
Most of the previous studies on this topic have rarely considered water flow behavior within green roof systems. Several studies have focused on the numerical modeling of green roof systems while considering water flow behavior. She and Pang (2009) established a model that calculates the water content of medium from rainfall using the Storm Water Management Model (SWMM). However, the SWMM is limited in that it does not simulate the detailed physical processes of green roofs. In addition, Kroes et al. (2000) applied the Soil-Water-Atmosphere-Plant (SWAP) model to simulate the influencing factors of green roof systems, but this application was limited to monolithic green roofs, without drainage or storage. Radcliffe and Simunek (2010) developed the HYDRUS model for simulating physical soil changes; however, it was not able to account for green roof water storage structures well. It should be noted that the design of modern green roof systems can permit the storage of rainwater during dry time periods and can allow for rapid drainage during heavy rainfall events.
Thus, the objective of this study was to investigate the hydrological behavior of a green roof system by using the SEEP/W model for design and management purposes. The SEEP/W model was used to simulate water flow behavior within green roofs, considering the physical processes of water flow in a medium and simulating a transient steady state, which presents the difference between inflow and outflow. The simulation results were compared with observational data from a test bed for green roofs established at Nanyang Technological University (NTU) in Singapore. The methodology is presented in the next section, followed by a case study, analyses and discussions of results, and conclusions.
The SEEP/W model was designed to calculate water flow in both saturated and unsaturated porous materials. It uses the finite element method (FEM) for numerical analysis (Thieu et al., 2001; Krahn, 2004; Chu-Agor et al., 2008). In this numerical analysis, the modeling geometry is primarily created to perform grid generation by defining the element size and shape (e.g., rectangular). Then, the material properties are determined by adopting the soil type (e.g., saturated/unsaturated silt or clay). This step defines the particle size and distribution, which together affect the volumetric water content. Notably, the particle size distribution, which can be represented via pore size distribution, determines hydraulic properties such as the soil-water characteristic curve and permeability (Zhai et al., 2019). However, pore size distribution has been rarely used for hydraulic conductivity estimation (Zhai et al., 2018). In this study, the geometric grid generation step is used to represent the pore size distribution, even though it cannot entirely represent the actual pore size distribution. In addition, volumetric water content, which is a function of the material’s moisture content, is reflected. Then, the hydraulic conductivity function defines the permeability with respect to the material moisture content of each element, including the residual water content. For the volumetric water content and hydraulic conductivity, the functions are set to estimate water flow. The volumetric water content, which is significantly affected by particle size and distribution, describes the portion of pores that are filled with water. When setting the volumetric water content, is important to specify the hydraulic conductivity of the porous materials that have unsaturated pores. In addition, there are options in the SEEP/W model for material properties and boundary conditions. The model considers two sets of partial differential equilibrium equations and the first governing equation for soil mass is described as follows:
where σij represents the components of the total stress tensor, ρ is the total mass density, and gj is jth component of the gravitational acceleration vector. The second governing equation for water flow is expressed as follows:
where qx, qy, and qz are the flow velocities of the solid matrix in the x, y, and z directions, respectively. θw is the volumetric water content and t is time. Using Darcy’s law, flow velocities, qi, can be estimated from the following relationship:
where ki is the unsaturated permeability in the i direction, uw is the pore-water pressure, ρw is the water density, g is the gravitational acceleration constant, and z is the vertical coordinate. Then, the initial condition that specifies the pore water pressure distribution is determined. For the green roof test bed simulation, the ground water table is set at the bottom of the drainage layer, and an unsaturated condition, which generates a negative pore pressure, is considered for the substrate. However, as the maximum pore pressure is only 0.25 kPa because of the shallow substrate depth (25 cm), the substrate is set as fully saturated in this study. Finally, the boundary conditions are determined to set the head or total flow rate, which provide a driving force of water flow with the water pressure head at the outlet of the green roof system. More detailed information for the physics in terms of soil water content, hydraulic conductivity, total head, and pore pressure can be found in GEOSLOPE International Ltd. (2017).
Numerical simulations using the SEEP/W model are useful for scheme selection, as one factor can be adjusted while the other factors remain constant. This approach can be used to estimate the most influential factor by simulating the water flow behavior within green roof systems. Through the simulation results, the degree of influence of each factor on the hydrological performance of green roofs can be estimated. Different values for each factor can be applied to evaluate the impact of changing each factor on the runoff characteristics in the SEEP/W model.
This study considers four factors: soil type, rainfall intensity, substrate depth, and green roof slope. These factors have been found to be sensitive to the hydrological performance of green roof systems, and can be adjusted by changing their geometry, material properties, volumetric water content, hydraulic conductivity, and boundary conditions. The properties and parameters used in the simulations are summarized in Table 1. First, the silt and clay soil types were selected for the evaluation of the material properties effects. Note that the green roof soil type that has been generally used is silty clay because of its significant influence on infiltration, water retention, and plant survival in dry conditions (Eksi et al., 2020; Liu et al., 2020). The hydraulic conductivity of silt and clay was set to 5e-05 and 1e-06 mm/s, respectively. It is worth noting that the hydraulic conductivity of silt is typically larger than 1e-05 mm/s and that of clay is typically less than 1e-05 mm/s (Reynolds and Zebchuk, 1996; Chandel and Kumar, 2016). Secondly, the rainfall intensities were set to 2.5 and 15 mm/min to consider changes in the volumetric water content. Substrate depths of 100 and 300 mm were adopted to determine the impact of water storage on flow rates. Finally, the green roof slopes were set to be 0 and 10 degrees, relevant to the effect of the head and flow rates, which determine the hydraulic conductivity.
For conventional roofs, heavy rain induces a large amount of runoff, as the roof instantaneously releases the rainwater during a rainfall event. Green roof systems, however, gradually release rainwater over a period of time, as they can store the rainwater through infiltration into the substrate and drainage layers. In particular, they alleviate potential damage from heavy rainfall by delaying the peak time of runoff, temporarily retaining rainwater through infiltration, reducing peak discharge of runoff, and storing water in the substrate and drainage layer, owing to the absorption capacity of soils.
Green roof systems are basically composed of the substrate and drainage layers, which can mitigate problems from dramatic runoff after heavy rain in urbanized areas. The substrate layer retains water, and the drainage layer discharges the infiltrated water flow. Green roofs can be divided into either extensive or intensive systems with respect to the depth of the substrate layer. Extensive systems have substrate layers with depths of less than 100 mm and can be incorporated on sloped surfaces with angles up to 45degrees (VanWoert et al., 2005; Kosareo and Ries, 2007). In addition, extensive systems can be used on the roofs of buildings that are limited by weight restrictions. Conversely, the intensive system used in this study had a substrate depth of more than 100 mm, and it can only be used on slopes with angles of less than 10 degrees (Kosareo and Ries, 2007).
Green roof systems have been promoted as a method to manage rainwater in urbanized countries such as Germany, Japan, Belgium, and Singapore (Osmundson, 1999; Wong et al., 2003; Dunnett and Kingsbury, 2004). In this study, a test bed of a green roof was considered to evaluate the numerical results of the SEEP/W model from observational data located at Nanyang Technological University (NTU) in Singapore. Figure 1 shows an overall layout of the green roofs at NTU and shows a design concept of its main frame. The dimensions of the green roof system were 2°m × 2°m, comprising four plant boxes, each 1°m × 1°m. It had an angle of 5 degrees. It is worth noting that previous studies demonstrated that a green roof covering over 70% of the rooftop allows stormwater quantity management (Carter and Fowler, 2008). The dimensions of the green roof in this study are limited owing to the lack of rooftop coverage; however, the test bed is installed for the prototype chamber test.
FIGURE 1. Green roof test bed at NTU in Singapore: (A) overall layout; (B) design concept of a main frame.
The test bed of the green roofs was elevated 400 mm from the ground, in order to have a sufficient height to measure the flow rate. Figure 2 shows that each plant box was filled with soil and plants (cuphea with grass) to a depth of 250 mm, and those plants were placed above the substrate layer. The bottom filtration tray, which had a depth of 50 mm filled with pebbles, was located beneath the substrate layer to allow rainwater drainage. In addition, two tanks were prepared to collect the infiltrated water discharge from the surface runoff and the base flow, including the leachate.
FIGURE 2. Structural components of the green roofs at NTU (Qin et al., 2012).
The SEEP/W model numerically simulated the hydrological behavior of the green roof systems, as shown in Figure 3, which presents typical models of green roof systems with 300-mm-thick substrate layers with slopes of 0 and 10 degrees. The upper and right-side boundaries were set to be permeable, whereas the bottom and left-side boundaries were set to be impervious, to simulate the interface conditions of the green roof systems. In addition, the colors of the substrate zones represent the water head within each specific section. The water flows from the left side to the right side because of the head difference during rainfall, and the black arrows indicate the direction of water flow during runoff processes. Here, the size of each black arrow represents the amount of runoff discharge.
FIGURE 3. Interface of the SEEP/W model for the simulation of green roof systems: (A) without a slope; (B) with a slope of 10 degrees.
The SEEP/W model simulated the actual green roof system at the test bed at NTU, Singapore. The set values of factors for the SEEP/W model followed the actual data from the green roofs at NTU, which have a slope of 5 degrees. The substrate layer, shown in yellow in Figure 3, was filled with soil, and the additional layer in green represents the infiltration tray beneath the soil substrate. Furthermore, the arrows in blue and black represent the rainfall on the green roof and the runoff through the outlet at the right-hand side bottom corner, respectively. Figure 4 summarizes the comparison between the simulated and experimental rainfall-runoff relationship results.
FIGURE 4. Rainfall-runoff relationship of the simulated results from the SEEP/W model and the observed data of the green roof system at NTU.
Due to the rainfall event shown in Figure 4, the peak time and peak discharge from the SEEP/W simulation were calculated as 92 min and 99.79 cm3/s, respectively, while those from the observation data were 102 min and 89.5 cm3/s, respectively. Specifically, the increase of runoff discharge starts at 85 min in the simulation, while it starts at 86 min in the observational data. The increasing trend continues in both until approximately 91 min. Thereafter, the difference in peak discharge between the simulation and the observational data is 10.29 cm3/s. Finally, the direct runoff from the test bed ended at ∼ 150 min in the simulation case, which was approximately 50 min earlier than in the observational data.
The peak time in the SEEP/W simulation result was faster than the equivalent value in the observational data, while the simulated peak discharge was greater than the observational value. This difference may result from the modeling interface, which simulates only water flow through the substrate layer and does not consider the vegetation in the green roof system. In the actual green roof system, the vegetation can absorb rainwater and hinders the flow of infiltrated water from the surface to the substrate layer. The vegetation may delay the peak time and lower the peak discharge from the observational data, compared with that from the numerical results of the SEEP/W model. However, the simulated and observational runoff discharge trends over time are similar.
The simulation results of the green roofs at NTU show that the SEEP/W model can be used for the design and management of green roofs despite the vegetation consideration limitation. It can therefore contribute to improving the current understanding of flow dynamics within green roof systems. As the SEEP/W model can also describe the hydrological behavior of green roofs under different conditions, and can quantify flow responses at the outlet of the system, the effectiveness of green roofs can be evaluated from the results of its numerical modeling, with respect to storm water mitigation, as a measure for rainwater management in urban areas.
This study explores the impacts of different factors in the SEEP/W model, regarding the hydrological performance of a green roof system according to four factors, soil type, rainfall intensity, substrate depth, and green roof slope. In the SEEP/W model simulation, the initial conditions depend on material properties and the interface setting. Two soil types, clay and silty clay, were considered in the sensitivity analysis. The hydraulic conductivity of clay and silty clay were 5e-05 and 1e-06 mm/s, respectively. Additionally, rainfall duration in the simulation was set to 10 min. Two indicators were considered to evaluate the effect of each factor in the SEEP/W model, these indicators were the peak discharge to rainfall intensity (PD/RI) ratio and the rainwater retention rate. The PD/RI ratio is calculated as the peak discharge divided by rainfall intensity, and the rainwater retention rate is the ratio of rainfall that does not run off the green roof to potential runoff of total rainfall (Getter et al., 2007). Figure 5 provides a comparison between the results of various groups, depending on the values of the factors considered.
FIGURE 5. Results of the sensitivity analysis of different factors to assess the hydrological performance of green roof systems: (A) PD/RI ratio; (B) Rainwater retention rate. Note: red-clay and blue-silty clay; circle-2.5 mm/min and square-15.0 mm/min; open-300 mm and closed-100 mm; small-0 degrees and large-10 degrees.
First, the mean PD/RI ratio value of clay (groups 1–8) was approximately 24.6% of that of silty clay (groups 9–16), and the mean rainwater retention rate value of clay was approximately 261.5% of that of silty clay. The PD/RI and rainwater retention values indicate that the hydrological performance of clay was better than that of silty clay. The hydraulic conductivity determined the drainage characteristics, which is a function of soil properties, such as particle size, particle distribution, and chemical composition. The hydraulic conductivity of clay is typically less than 1e-05 mm/s, and it tends to be impermeable. Silt and silty clay, however, typically have a hydraulic conductivity between 1e-05 and 1e-03 mm/s (Chandel and Kumar, 2016). In addition, the negative ionic characteristics of the clay particle’s surfaces may generate hydrogen bonding with water molecules (Barshad, 1952). For this reason, the chemical composition of clay particles mean that they absorb water and form double-layer water, which is one of the reasons for the observed water retention. Thus, the lower hydraulic conductivity and chemical composition of clay may have resulted in the observed lower PD/RI ratio and greater rainwater retention in clay compared with silty clay.
Second, the mean PD/RI value under a rainfall intensity of 2.5 mm/min (groups 1–4 and 9–12) was approximately 88.7% of that under a rainfall intensity of 15 mm/min (groups 5–8 and 13–16). Conversely, the mean rainwater retention value of the groups with relatively lower rainfall intensities were approximately 116.2% of that of the other groups. This is consistent with the results of Teemusk and Mander (2007), who identified the volume and intensity of rainwater as important factors determining the amount of water that is retained in a green roof system. Even though the rainfall intensity, which can be a source of water retention, is high, the water retention rate becomes lower if the rainfall intensity is extremely high, as in the case of storm events (Carter and Rasmussen, 2005; Moran et al., 2005). Thus, the green roof system design should provide practical alternatives, such as selection of clay materials for the substrate, for delaying and attenuating runoff discharge at source.
Third, the mean PD/RI value in substrate layers with depths of 300 mm (groups 1–2, 5–6, 9–10, and 13–14) was approximately 95.0% of that when the depth was 100 mm (groups 3–4, 7–8, 11–12, and 15–16). The mean rainwater retention rate value of the first group was approximately 107.3% of that of the other groups. During a rainfall event, one of the key hydrological mechanisms in a green roof system is storage and infiltration in the substrate layer (Stovin, 2010). This process is directly related to the retention capacity of the soil in a green roof system, and the shape of the hydrograph at the outlet is determined from the relationship between the rainfall characteristics during a storm event and the potential absorption rates in the soil column. Even though there was no significant difference in hydrological performance between the two different substrate layers considered in this study, previous studies have shown that rainfall-runoff relationships in green roof systems can be significantly affected by the substrate layer depth over a long time period (Mentens et al., 2006).
Finally, the mean PD/RI value of green roofs with a slope of 0 degree (groups 1, 3, 5, 7, 9, 11, 13, and 15) was approximately 98.7% of that of green roofs with slopes of 10 degrees (groups 2, 4, 6, 8, 10, 12, 14, and 16). The mean rainwater retention rate value of the first group was approximately 102.7% of that of the other groups. The decrease in slope of a green roof is slightly beneficial in terms of decreasing the PD/RI ratio and increasing the rainwater retention rate. However, the slope of a green roof was found to have less of an impact on its hydrological performance than the other factors (Liesecke, 1998; Mentens et al., 2006).
The objective of this study was to investigate the hydrological behavior of a green roof system by using the SEEP/W model, for design and management purposes. The interface of the SEEP/W model was set to simulate a green roof, and its geometry, material property, volumetric water content, hydraulic conductivity, and boundary conditions were explored. A green roof test bed was installed at NTU to measure actual hydrometeorological data, including rainfall and runoff data. The actual data were compared with the simulated results from the SEEP/W model to evaluate the applicability of the model to green roof design and management. For the simulations, different values for four factors (soil type, rainfall intensity, substrate depth, and green roof slope) were considered in order to determine their impact on the hydrological performances of green roofs. The hydrological performance was estimated via the PD/RI ratio and the rainwater retention rate. The main findings of this study are as follows:
1) Comparing the simulation results and observational data revealed that the simulated peak time and peak discharge values were slightly faster and greater than those of the observational data, which is attributed to the vegetation conditions in the real green roof system. However, the results show that the SEEP/W model can be used in green roof design because of its effectiveness in terms of modeling the hydrological behavior of a green roof system, while considering water flow dynamics. In future work, additional factors, such as vegetation and an insulating pebble layer, should be considered for the design and management of green roofs. In addition, it should be noted that this study can be further extended to the design, construction, rehabilitation, operation, and maintenance of low-impact development or green infra systems, which are being widely used for critical infrastructure elements in water sensitive urban design.
2) The decrease in the PD/RI ratio and increase in the rainwater retention rate denote a better hydrological performance of the green roofs. The simulation results revealed that the hydrological performance was better for clay than for silty clay because of the lower hydraulic conductivity of the former, and the effects of chemical composition. In addition, a lower rainfall intensity and a thicker substrate layer were shown to result in a better hydrological performance, as they mainly determine the rainfall-runoff relationship. The slope of the green roof, however, was shown to have no significant effect on the hydrological performance, resulting in a negligible difference in the PD/RI ratio and rainwater retention rate. In conclusion, the water flow characteristics are primarily dependent on soil type, which in turn determine the hydrological performance of a green roof system.
The original contributions presented in the study are included in the article/Supplementary Material, further inquiries can be directed to the corresponding author.
Conceptualization, CJ and SK; methodology, CJ, HS, and YK; software, SK and WN; validation, SK and WN; formal analysis, CJ; investigation, CJ, SK, and WN; resources, CJ and SK; data curation, CJ; writing—original draft preparation, CJ and SK; writing—review and editing, CJ, SK, WN, HS, and YK; visualization, SK and WN.
This research was supported by the National Research Foundation of Korea (NRF) grant funded by the Korea Government (MSIT) (2020R1G1A101362412) and in part by the Chung-Ang University Research Grants in 2021.
The authors declare that the research was conducted in the absence of any commercial or financial relationships that could be construed as a potential conflict of interest.
All claims expressed in this article are solely those of the authors and do not necessarily represent those of their affiliated organizations, or those of the publisher, the editors and the reviewers. Any product that may be evaluated in this article, orclaim that may be made by its manufacturer, is not guaranteed or endorsed by the publisher.
The corresponding author was formerly affiliated with the School of Civil and Environmental Engineering, Nanyang Technological University. We deeply appreciate the data support from Professor Xiaosheng Qin at Nanyang Technological University.
Barshad, I. (1952). Adsorptive and Swelling Properties of Clay-Water System. Clays and Clay Minerals 1 (1), 70–77. doi:10.1346/CCMN.1952.0010108
Baryła, A., Karczmarczyk, A., and Bus, A. (2018). Role of Substrates Used for green Roofs in Limiting Rainwater Runoff. J. Ecol. Eng. 19 (5), 86–92. doi:10.12911/22998993/91268
Carter, T., and Fowler, L. (2008). Establishing green Roof Infrastructure through Environmental Policy Instruments. Environ. Manage. 42 (1), 151–164. doi:10.1007/s00267-008-9095-5
Carter, T., and Rasmussen, T. (2005). Use of green Roofs for Ultra-urban Stream Restoration in the Georgia piedmont (USA),” in Proceedings of the 3rd North American Green Roof Conference: Greening Rooftops for Sustainable Communities, Washington, DC, US. May 4-6, 2005.
Castiglia Feitosa, R., and Wilkinson, S. (2016). Modelling green Roof Stormwater Response for Different Soil Depths. Landscape Urban Plann. 153, 170–179. doi:10.1016/j.landurbplan.2016.05.007
Chandel, A., and Kumar, U. (2016). “Permeability Characteristics of Clayey Soil Added with Fly Ash. Proceedings of International Conference on Emerging Trends in Civil Engineering (ICETCE-2016),” in Sultanpur, India, October 2016. 207–209.
Chu-Agor, M. L., Wilson, G. V., and Fox, G. A. (2008). Numerical Modeling of Bank Instability by Seepage Erosion Undercutting of Layered Streambanks. J. Hydrol. Eng. 13 (12), 1133–1145. doi:10.1061/(asce)1084-0699(2008)13:12(1133)
DeNardo, J. C., Jarrett, A. R., Manbeck, H. B., Beattie, D. J., and Berghage, R. D. (2005). Stormwater Mitigation and Surface Temperature Reduction by green Roofs. Trans. ASAE. 48 (4), 1491–1496. doi:10.13031/2013.19181
Dunnett, N., and Kingsbury, N. (2004). Planting Green Roofs and Living Walls. Portland, OR: Timber Press.
Eksi, M., Sevgi, O., Akburak, S., Yurtseven, H., and Esin, İ. (2020). Assessment of Recycled or Locally Available Materials as green Roof Substrates. Ecol. Eng. 156, 105966. doi:10.1016/j.ecoleng.2020.105966
Fioretti, R., Palla, A., Lanza, L. G., and Principi, P. (2010). Green Roof Energy and Water Related Performance in the Mediterranean Climate. Building Environ. 45 (8), 1890–1904. doi:10.1016/j.buildenv.2010.03.001
GEOSLOPE International Ltd (2017). Heat and Mass Transfer Modeling with GeoStudio 2018. 2nd Edition. Calgary, Alberta, Canada: GEOSLOPE International Ltd.
Getter, K. L., Rowe, D. B., and Andresen, J. A. (2007). Quantifying the Effect of Slope on Extensive green Roof Stormwater Retention. Ecol. Eng. 31 (4), 225–231. doi:10.1016/j.ecoleng.2007.06.004
Getter, K. L., and Rowe, D. B. (2006). The Role of Extensive green Roofs in Sustainable Development. HortSci 41 (5), 1276–1285. doi:10.21273/HORTSCI.41.5.1276
Hathaway, A. M., Hunt, W. F., and Jennings, G. D. (2008). A Field Study of green Roof Hydrologic and Water Quality Performance. Trans. ASABE 51 (1), 37–44. doi:10.13031/2013.24225
Hilten, R. N., Lawrence, T. M., and Tollner, E. W. (2008). Modeling Stormwater Runoff from green Roofs with HYDRUS-1D. J. Hydrol. 358 (3-4), 288–293. doi:10.1016/j.jhydrol.2008.06.010
Kosareo, L., and Ries, R. (2007). Comparative Environmental Life Cycle Assessment of green Roofs. Building Environ. 42 (7), 2606–2613. doi:10.1016/j.buildenv.2006.06.019
Krahn, J. (2004). Seepage Modeling with SEEP/W: An Engineering Methodology. Calgary, Alberta, Canada: GEOSLOPE International Ltd.
Kroes, J. G., Wesseling, J. G., and Van Dam, J. C. (2000). Integrated Modelling of the Soil–Water–Atmosphere–Plant System Using the Model SWAP 2.0 an Overview of Theory and an Application. Hydrol. Process. 14 (11-12), 1993–2002. doi:10.1002/1099-1085(20000815/30)14:11/12<1993:aid-hyp50>3.0.co;2-#
Li, W., Wu, C., and Choi, W. (2018). Predicting Future Urban Impervious Surface Distribution Using Cellular Automata and Regression Analysis. Earth Sci. Inform. 11 (1), 19–29. doi:10.1007/s12145-017-0312-8
Liesecke, H. J. (1998). Das retentionsvermogen von dachbegrunungen (Water retention capacity of vegetated roofs). Stadt und Grun 47, 46–53.
Liu, K. (2003). Engineering Performance of Rooftop Gardens through Field Evaluation. “Proceedings of the 18th International Convention of the Roof Consultants Institute,” in Tampa, Florida, March 13-18, 2003. 93–103.
Liu, L., Sun, L., Niu, J., and Riley, W. J. (2020). Modeling green Roof Potential to Mitigate Urban Flooding in a Chinese City. Water 12 (8), 2082. doi:10.3390/w12082082
Mentens, J., Raes, D., and Hermy, M. (2006). Green Roofs as a Tool for Solving the Rainwater Runoff Problem in the Urbanized 21st century? Landscape Urban Plann. 77 (3), 217–226. doi:10.1016/j.landurbplan.2005.02.010
Moran, A., Hunt, B., and Jennings, G. (2003). “A North Carolina Field Study to Evaluate Greenroof Runoff Quantity, Runoff Quality, and Plant Growth,” in Proceedings of the World Water & Environmental Resources Congress 2003, Philadelphia, Pennsylvania, US, June 23-26, 2003, 1–10. doi:10.1061/40685(2003)335
Moran, A., Hunt, B., and Smith, J. (2005). “Hydrologic and Water Quality Performance from green Roofs in Goldsboro and Raleigh, North Carolina,” in Proceedings of the 3rd North American Green Roof Conference: Greening Rooftops for Sustainable Communities, Washington, DC, US, May 4-6, 2005.
Ouldboukhitine, S.-E., Belarbi, R., and Djedjig, R. (2012). Characterization of green Roof Components: Measurements of thermal and Hydrological Properties. Building Environ. 56, 78–85. doi:10.1016/j.buildenv.2012.02.024
Qin, X., Wu, X., Chiew, Y. M., and Li, Y. (2012). A green Roof Test Bed for Stormwater Management and Reduction of Urban Heat Island Effect in Singapore. Bjecc 2 (4), 410–420. doi:10.9734/BJECC/2012/2704
Radcliffe, D. E., and Simunek, J. (2010). Soil Physics with HYDRUS—Modelling and Applications. Boca Raton, FL: CRC Press.
Reynolds, W. D., and Zebchuk, W. D. (1996). Hydraulic Conductivity in a clay Soil: Two Measurement Techniques and Spatial Characterization. Soil Sci. Soc. America J. 60 (6), 1679–1685. doi:10.2136/sssaj1996.03615995006000060011x
Rowe, D. B., Rugh, C. L., VanWoert, N., Monterusso, M. A., and Russell, D. K. (2003). Green Roof Slope, Substrate Depth, and Vegetation Influence Runoff,” in Proceedings of the 1st North American Green Roof Conference: Greening Rooftops for Sustainable Communities, Chicago, IL, USA, May 29-30, 2003. 354–362.
Scholz, M. (2004). Case Study: Design, Operation, Maintenance and Water Quality Management of Sustainable Storm Water Ponds for Roof Runoff. Bioresour. Tech. 95 (3), 269–279. doi:10.1016/j.biortech.2003.07.015
Shafique, M., Kim, R., and Rafiq, M. (2018). Green Roof Benefits, Opportunities and Challenges - A Review. Renew. Sust. Energ. Rev. 90, 757–773. doi:10.1016/j.rser.2018.04.006
She, N., and Pang, J. (2009). Physically Based green Roof Model. J. Hydrol. Eng. 15 (6), 458–464. doi:10.1061/(ASCE)HE.1943-5584.0000138
Simmons, M. T., Gardiner, B., Windhager, S., and Tinsley, J. (2008). Green Roofs Are Not Created Equal: the Hydrologic and thermal Performance of Six Different Extensive green Roofs and Reflective and Non-reflective Roofs in a Sub-tropical Climate. Urban Ecosyst. 11 (4), 339–348. doi:10.1007/s11252-008-0069-4
Soulis, K. X., Ntoulas, N., Nektarios, P. A., and Kargas, G. (2017). Runoff Reduction from Extensive green Roofs Having Different Substrate Depth and Plant Cover. Ecol. Eng. 102, 80–89. doi:10.1016/j.ecoleng.2017.01.031
Speak, A. F., Rothwell, J. J., Lindley, S. J., and Smith, C. L. (2013). Rainwater Runoff Retention on an Aged Intensive green Roof. Sci. Total Environ. 461-462 (1), 28–38. doi:10.1016/j.scitotenv.2013.04.085
Stovin, V. (2010). The Potential of green Roofs to Manage Urban Stormwater. Water Environ. J. 24 (3), 192–199. doi:10.1111/j.1747-6593.2009.00174.x
Stovin, V., Vesuviano, G., and De-Ville, S. (2017). Defining green Roof Detention Performance. Urban Water J. 14 (6), 574–588. doi:10.1080/1573062X.2015.1049279
Stovin, V., Vesuviano, G., and Kasmin, H. (2012). The Hydrological Performance of a green Roof Test Bed under UK Climatic Conditions. J. Hydrol. 414-415 (11), 148–161. doi:10.1016/j.jhydrol.2011.10.022
Teemusk, A., and Mander, Ü. (2007). Rainwater Runoff Quantity and Quality Performance from a Greenroof: the Effects of Short-Term Events. Ecol. Eng. 30 (3), 271–277. doi:10.1016/j.ecoleng.2007.01.009
Thieu, N. T. M., Fredlund, M. D., Fredlund, D. G., and Vu, H. Q. (2001). “Seepage Modeling in a Saturated/unsaturated Soil System,” in Proceedings of the International Conference on Management of the Land and Water Resources, Hanoi, Vietnam, October 2001. 20–22.
VanWoert, N. D., Rowe, D. B., Andresen, J. A., Rugh, C. L., Fernandez, R. T., and Xiao, L. (2005). Green Roof Stormwater Retention. J. Environ. Qual. 34 (3), 1036–1044. doi:10.2134/jeq2004.0364
Vijayaraghavan, K. (2016). Green Roofs: A Critical Review on the Role of Components, Benefits, Limitations and Trends. Renew. Sust. Energ. Rev. 57, 740–752. doi:10.1016/j.rser.2015.12.119
Villarreal, E. L., Semadeni-Davies, A., and Bengtsson, L. (2004). Inner City Stormwater Control Using a Combination of Best Management Practices. Ecol. Eng. 22 (4-5), 279–298. doi:10.1016/j.ecoleng.2004.06.007
Wong, N. H., Tay, S. F., Wong, R., Ong, C. L., and Sia, A. (2003). Life Cycle Cost Analysis of Rooftop Gardens in Singapore. Building Environ. 38 (3), 499–509. doi:10.1016/S0360-1323(02)00131-2
Yan, Z., Teng, M., He, W., Liu, A., Li, Y., and Wang, P. (2019). Impervious Surface Area Is a Key Predictor for Urban Plant Diversity in a City Undergone Rapid Urbanization. Sci. Total Environ. 650, 335–342. doi:10.1016/j.scitotenv.2018.09.025
Zhai, Q., Rahardjo, H., and Satyanaga, A. (2018). A Pore-Size Distribution Function Based Method for Estimation of Hydraulic Properties of Sandy Soils. Eng. Geol. 246, 288–292. doi:10.1016/j.enggeo.2018.09.031
Keywords: green roof systems, hydrological performance, rainfall-runoff, SEEP/W model, urban area
Citation: Kim SY, Na W, Jun C, Seo H and Kim Y (2021) Hydrological Performance of Green Roof Systems: A Numerical Investigation. Front. Environ. Sci. 9:806697. doi: 10.3389/fenvs.2021.806697
Received: 01 November 2021; Accepted: 25 November 2021;
Published: 08 December 2021.
Edited by:
Alfrendo Satyanaga, Nazarbayev University, KazakhstanReviewed by:
Robby Yussac Tallar, Universitas Kristen Maranatha, IndonesiaCopyright © 2021 Kim, Na, Jun, Seo and Kim. This is an open-access article distributed under the terms of the Creative Commons Attribution License (CC BY). The use, distribution or reproduction in other forums is permitted, provided the original author(s) and the copyright owner(s) are credited and that the original publication in this journal is cited, in accordance with accepted academic practice. No use, distribution or reproduction is permitted which does not comply with these terms.
*Correspondence: Changhyun Jun, Y2p1bkBjYXUuYWMua3I=
Disclaimer: All claims expressed in this article are solely those of the authors and do not necessarily represent those of their affiliated organizations, or those of the publisher, the editors and the reviewers. Any product that may be evaluated in this article or claim that may be made by its manufacturer is not guaranteed or endorsed by the publisher.
Research integrity at Frontiers
Learn more about the work of our research integrity team to safeguard the quality of each article we publish.