- 1Centre for Applied Water Science, Institute for Applied Ecology, University of Canberra, Canberra, ACT, Australia
- 2Australian Rivers Institute, Griffith University, Nathan, QLD, Australia
Environmental water management is increasingly used to restore riverine, wetland and floodplain ecosystems and requires an understanding of what the flow regime or restoration objectives are, why these objectives are being targeted and how outcomes will be evaluated. This perspective paper focuses on non-woody vegetation, an important component of river-floodplain ecosystems and a targeted outcome for many environmental flow management programs, such as the Basin wide environmental watering strategy for the Murray-Darling Basin in Australia. Effective management of non-woody vegetation using environmental water requires identifying a suite of measurable condition outcomes (the “what”), understanding how these relate to broader functions and values (the “why”) and developing clear cause-and-effect relationships between management and outcomes (the “how”). A critical component of this process is to characterise what constitutes management success, which requires reimagining current definitions of condition to better incorporate dynamic functions and diverse values. We identify the need to characterise condition in a structured framework using both ecological data and societal values. This approach will not only help inform the development of benchmarks, watering objectives and monitoring metrics, but will also facilitate engagement by a broader spectrum of the community with the management and outcomes of environmental watering.
Introduction
Evaluating success is a key challenge in restoration ecology (Palmer et al., 2005; Wortley et al., 2013; Prach et al., 2019). The answer is seemingly simple—“success occurs when the ecological restoration reaches its goal” (Prach et al., 2019). However, the reality is a melting pot of divergent expectations, definitions and measures of success (Wortleyet al. 2013; Prach et al., 2019) with ongoing debates over how to define or categorize success or failure (Wortley et al., 2013; Prach et al., 2019; Galbraith et al., 2021; Marchand et al., 2021). Evaluating outcomes from environmental management actions, such as environmental flows, is important as it enables learning by doing to inform future management activities (Pollard et al., 2011; Vietz et al., 2018; McLoughlin et al., 2020). Evaluation also strengthens theoretical understanding (Török and Helm 2017), improving the predictability of restoration outcomes (Bullock et al., 2011; Brudvig et al., 2017).
Central to evaluating outcomes of management actions such as environmental flows is our understanding of what the restoration objectives are, why these objectives are being targeted and how outcomes will be assessed in relation to these. This paper focuses on non-woody vegetation condition as a targeted outcome of environmental water actions. While improved vegetation condition is a common objective of environmental water management, the construct of condition is often poorly defined (Gibbons et al., 2006; Gibbons and Freudenberger 2006). In particular, naturally dynamic responses of vegetation to variable water regimes are not well represented by static descriptions of condition (Campbell et al., 2021). Following the principles of Campbell, James et al. (2021), wetland ecological condition needs to consider i) various scales and levels of ecological organisation, ii) temporal context and complexity, iii) non-hydrological modifying factors, and iv) align with management objectives and ecological, sociocultural and economic functions and values. In this perspective article we consider what we want to achieve by targeting watering for wetland vegetation condition. We explore the idea of success, specifically in terms of reimagining current definitions of condition to better incorporate dynamic functions and diverse values. We conclude by highlighting the potential for a framework of condition, structured around the principles of Campbell, James et al. (2021), to provide the building blocks for a data-driven narrative that synthesises disparate pieces of information.
What Do We Want to Achieve by Watering Non-Woody Wetland-Floodplain Vegetation and why Do We Target These Outcomes?
Determining objectives for environmental management or restoration projects remains a critical first step (Prach et al., 2019) and processes and principles for setting objectives have been dealt with extensively in the literature (Tear et al., 2005; Lindenmayer et al., 2008; Horne A et al., 2017; Campbell et al., 2021). Ultimately why we water rivers, wetlands and floodplains reflects what we value about them (Gonzalez Lopez and Amerigo Cuervo-Arango, 2008; Arsenio et al., 2020). An outcome may be valued because of its intrinsic worth, such as biodiversity or the inherent right of a species to exist (Dudgeon 2014; Jax and Heink 2015). In some situations management is focussed on maintaining or restoring ecological functions (de Groot et al., 2002; Capon et al., 2013), such as the provision of habitat or food (Valinoti et al., 2011; Bice et al., 2014; McGinness et al., 2014). Increasingly management is focused on achieving multiple ecosystem services including: aesthetics (Cottet et al., 2013), cultural connection (Douglas et al., 2019; Moggridge and Thompson 2021), recreation (Gitau et al., 2019), education (Flitcroft et al., 2016), or tourism (Balmford et al., 2009; Harrison et al., 2010). There are also calls for environmental water management to increase the focus on managing for resilience to better link environmental water management to ecological sustainability and social well-being (Arthington et al., 2018; Poff 2018). Research has shown that the level of support for the implementation of environmental management activities is linked to stakeholder engagement, including the ability to relate to environmental outcomes by a shared belief in the value of the outcome (Conallin et al., 2018; Okumah et al., 2020; Liguori et al., 2021).
Non-woody vegetation (NWV) can comprise a significant proportion of biodiversity in many catchments and is fundamental to many ecosystem functions. The Murray-Darling Basin (MDB) in Australia, for example, supports a tremendous diversity of both plants and vegetation communities (Brooks 2020; Capon and James 2020), including more than 700 native plant species (Dyer et al., 2021) and a wide diversity of vegetation communities (Brooks 2021). Many of these plant species can be described as non-woody—which in the context of this paper refers to all vascular plant species expect for trees and large shrubs as well as macro-algae such as charophytes. NWV comprises floating plants, submerged macrophytes, herbs, grasses, sedges, sub-shrubs, and tall reeds. NWV communities tend to be highly dynamic in space and time (Keddy 2010; Capon and Reid 2016; Hunter 2021), reflecting variation in inundation patterns over multiple scales (Thoms et al., 2006; Leblanc et al., 2012; Tulbure and Broich 2019).
Functionally, non-woody plant species and vegetation communities are critical components of river-floodplain ecosystems, providing food and habitat for a large array of biota including fish (Bice et al., 2014), woodland and waterbirds (Kingsford and Thomas 2004; Ma et al., 2010; McGinness et al., 2018), frogs (Wassens et al., 2010; McGinness et al., 2014), and macroinvertebrates (Warfe and Barmuta 2006). NWV also supports a wide range of ecological functions such as carbon and nutrient cycling (Carpenter and Lodge 1986; Baldwin et al., 2013), bank stabilisation (Marden et al., 2005; Docker and Hubble 2008), sediment and flow dynamics (Neary et al., 2012), water quality (Withers and Jarvie 2008) and regulation of microclimates (Reeder 2011; Choi et al., 2014; James et al., 2015). Furthermore, NWV supports many social, cultural and economic values, often playing a key role in shaping aesthetically beautiful places (Cottet et al., 2013; Saha et al., 2020), having cultural importance for food, medicine, or fibre (Conroy et al., 2019; Higgisson et al., 2021), contributing to the enjoyment of recreational pursuits (Harrison et al., 2010; Zhu et al., 2011), or supporting tourism (Siikamaki et al., 2015; Hausmann et al., 2020). Thus, by watering wetlands and floodplains and supporting the vegetation communities within them, we can support a range of ecological, socio-cultural, and economic functions.
Environmental water management can also be targeted to promote ecological resilience, i.e., the ability for ecosystems to resist or respond to change and implies long-term sustainability (Capon and Reid 2016; Chambers et al., 2019). To persist into the future, plant species and vegetation communities, as well as the functions these underpin, need to be resilient to factors such as a changing climate (Capon et al., 2013) that includes changes to rainfall, temperature, fire regimes and the intensity of extreme events (CSIRO and Bureau of Meteorology 2015). Plant species and vegetation communities also need to be resilient to pressures from development, land use, pollution, pest plants and animals, and ongoing competition for water resources (Reis et al., 2017; Dudgeon 2019; Reid et al., 2019). Managing for resilience is a function of understanding the different traits of taxa and communities that contribute to resilience (Combroux et al., 2001; Santamaria 2002; Clarke et al., 2015) to particular environmental disturbances (Chambers et al., 2019), key factors which underpin recovery mechanisms such as dormant seed banks (Brock 2011; Haines-Young et al., 2012; Capon and Reid 2016; Liu et al., 2020) as well as spatial resilience (Chambers et al., 2019). In many modified catchments, environmental water actions are likely to play a critical role in building resilience, helping to restore the health of vegetation from a degraded state (Overton et al., 2014; Bond et al., 2018), which can require a recovery flow regime that is, more frequent than the long-term average (Campbell et al., 2021). Environmental water can also help fill the role of small to medium floods (Bond et al., 2014) which, because of regulation, have been lost from the hydrograph in locations such as the mid to lower River Murray (Maheshwari et al., 1995).
How Do We Know if We’ve Succeeded in Meeting the Objectives?
Evaluating outcomes against goals or objectives remains pivotal to determining the success of environmental water management and ecological restoration projects (Prach et al., 2019), though what constitutes success or failure is still a topic of debate (Wortley et al., 2013; Prach et al., 2019; Galbraith et al., 2021; Marchand et al., 2021). Evaluation is “the process of judging or calculating the quality, importance, amount or value of something” (Cambridge-English-Dictionary 2020), and inevitably involves some degree of subjectivity albeit informed by expert opinion and experience (Prach et al., 2019). Considerable benefit in evaluating outcomes to environmental water management comes from the knowledge gained in relation to managing future environmental water management actions. For example, determining the factors that led to objectives not being met, such as water availability, climate or other factors, can help inform future water management. Results may indicate the need for complementary actions, such as the control of pest animals, or the need to adjust future objectives or expectations. Because environmental water management is often carried out at scales that prevent the establishment of unmanaged “controls” for comparison, evaluation often requires a sophisticated “teasing apart” of the roles of different drivers and potential confounding variables (Konrad et al., 2011; Gawne et al., 2019). For long-term goals and objectives, this may require multiple scenarios of expectations based on a range of potentially interacting variables and management options that may influence water availability, or broader scale factors such as climate.
The definition of evaluation given in this paper highlights the need to know the state of what is being evaluated, i.e., its quality, importance, amount or value—which in the context of assessing vegetation outcomes to management activities such as environmental flows can be broadly defined as vegetation condition. There is no standard definition for “vegetation condition” (Gibbons et al., 2006), rather it is seen as a continuum of “good” to “bad” depending on the context or goal (Gibbons and Freudenberger 2006). Condition is also inherently a comparative concept (Parkes and Lyon 2006) and involves value judgements—good condition for whom or good condition for what (Gibbons and Freudenberger 2006). Viewing condition as representing “the quality, importance, amount, or value of something” links to key elements of what we’re trying to achieve by watering NWV namely the contribution NWV makes to ecological, socio-cultural and economic functions and values, biodiversity, and the need to support resilience to maintain these values into the future. It also aligns with Driver-Pressure-State-Impact-Response (DPSIR) models used internationally (Tscherning et al., 2012; Robele Gari et al., 2015) in environmental assessments, such as Australian State of the Environment (SoE) reporting (Jackson 2017). The SoE approach describes the “State” as incorporating both current condition as well as recent trends in condition (Jackson 2017), highlighting the importance of temporal trajectories.
Definitions of condition, however, are not straightforward. Defining the state of something, such as NWV, is complex. NWV responses occur across different spatial and temporal scales. As highlighted by the SoE approach (Jackson 2017), state needs to consider current condition along with trends. Trend changes in NWV are influenced by a number of factors including flow regimes and climatic cycles across different temporal scales (Ryo et al., 2019). Considering the spatial scale across which responses and trends are assessed is important and influences ecological and spatial resilience (Chambers et al., 2019). Condition, as described in the paragraph above, is framed by the functions and values provided by NWV—“the quality, importance, amount, or value of something.” Functions and values also inform targets which are further used in the evaluation of the quality of condition in relation to targets (Figure 1). NWV provides numerous ecological, socio-cultural and economic functions and values, that can be broadly grouped as providing i) habitat, ii) regulating, iii) production, and iv) information functions and values (de Groot et al., 2002; Capon et al., 2013). NWV also contributes to biodiversity at different levels of ecological organisation (Noss 1990), such as individual plants, populations, species, communities, and vegscapes, which are landscape-scale mosaics of plant communities, and through the expression of a variety of attributes—composition, structure and process (Noss 1990) (Figure 1).
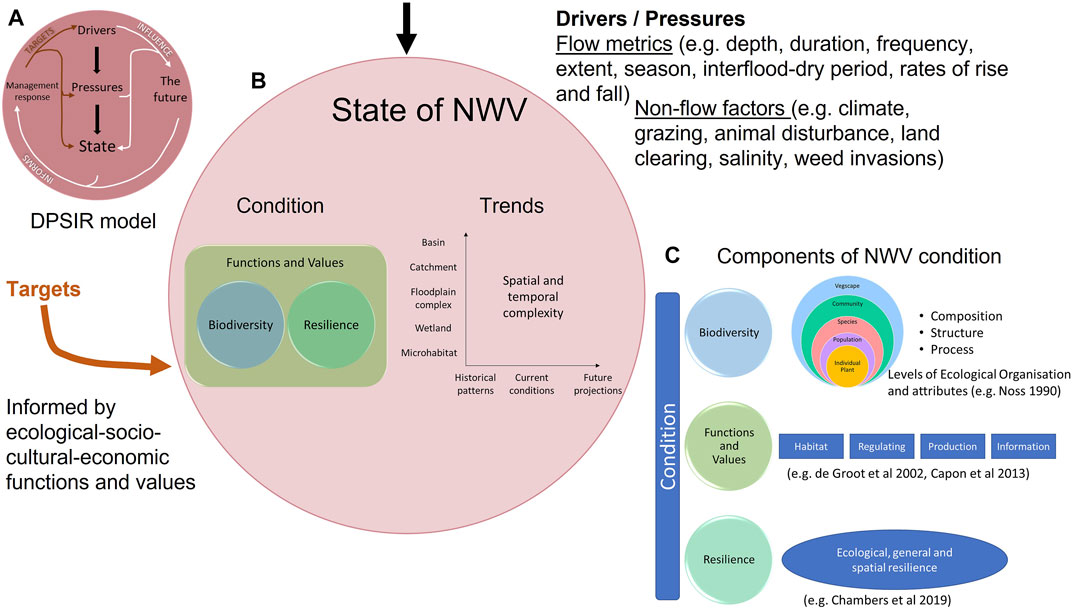
FIGURE 1. Conceptual framework depicting key components in the characterisation of non-woody vegetation (NWV) condition in floodplain-wetland environments: (A) represents the common use of “state” in Driver-Pressure-State-Impact-Response models and the links with targets and drivers and pressures [redrawn from (Jackson 2017)]; (B) depicts the state of NWV as condition and trends, where condition incorporates functions and values, biodiversity and resilience and trends covers both spatial and temporal complexity; (C) represents the components of NWV condition within biodiversity, functions and values and resilience.
As depicted in DPSIR models, the state of NWV, is further influenced by drivers and pressures (Figure 1). For NWV in floodplain-wetland systems key drivers and pressures include aspects of flow regimes across multiple temporal scales, such as individual pulses or inundation events, short-term flow regimes (e.g., annual to 10 years), and longer-term flow patterns over decades to centuries. NWV responses are also influenced by a range of non-flow drivers and stressors such as climate, pest plants and animals and land use development (Reid et al., 2019). The above components are depicted graphically in Figure 1 and further discussed in Campbell et al. (2021). Campbell et al. (2021) summarised characteristics of NWV responses into four principles to guide the development of objectives and evaluation approaches: i) identify indicators that align with management objectives and ecological functions that support ecological, socio-cultural and economic values; ii) identify appropriate spatial scales and levels of ecological organisation; iii) identify relevant temporal dynamics, trajectories and uncertainties; and iv) identify non-hydrological modifying drivers. In the following section we propose a vision for NWV condition and the evaluation of outcomes, particularly across broad spatial scales such as whole-of-Basin, that encapsulates these principles, as well as the construct of condition as representing “the quality, importance, amount or value of something” as presented here. While the MDB has been the backdrop for developing these ideas, the proposed characterisation of condition is applicable to other floodplain-wetland systems where environmental water management is used to achieve non-woody vegetation outcomes.
What Does Good Condition Look Like for Wetland-Floodplain NWV?
We propose that good condition should reflect a combination of ecological-socio-cultural and economic functions and values, biodiversity, and resilience—where resilience incorporates current and projected changes to flow regimes, climate, and other non-flow stressors and drivers, and that these components of condition should be assessed across multiple spatiotemporal scales and across multiple levels of ecological organisation (Figure 1).
This characterisation of NWV condition requires a multifaceted approach that can be informed by reference to literature, ecological theory, analysis of data, and human insights. There is a wealth of knowledge and insight held by practitioners working in the environmental water management space that is, rarely captured in published literature [with notable exceptions such as Jahnig et al. (2011); Horne A. C. et al., 2017; Wineland et al. (2021)]. Relationships between vegetation responses and flow regimes to inform definitions of condition, can be derived from quantitative analysis of data (e.g., Bowen 2019), expert elicitation (e.g., Sinclair et al., 2015; DELWP 2016; Sinclair et al., 2018), professional insights (Jahnig et al., 2011; Cook et al., 2012), traditional knowledge (Jackson et al., 2015; Douglas et al., 2019; Moggridge et al., 2019), structured literature reviews (e.g., Greet et al., 2011; Webb et al., 2012), conceptual models (Capon et al., 2009; Casanova 2015), or approaches which combine multiple aspects (e.g., Webb et al., 2015).
Similarly, the values and functions supported by NWV need to be derived from a broad range of stakeholders using appropriate techniques to facilitate engagement (Moggridge et al., 2019; Sterling et al., 2019; Liguori et al., 2021). Community understanding and support for environmental flows is an important part of social license and the ability to effectively implement environmental water management actions (Dare et al., 2014), with community understanding and support inevitably linked to personal values (Gonzalez Lopez and Amerigo Cuervo-Arango, 2008). To reflect the diversity of values, functions also need to encompass a range of services from ecological, such as habitat and regulation, to economic production and other social and cultural functions such as information, aesthetics, education and wellbeing (de Groot et al., 2002; Capon et al., 2013).
Using the conceptual model in Figure 1 components of condition can be structured in a framework that explicitly incorporates i) ecological, socio-cultural and economic functions and values, ii) biodiversity at multiple levels of ecological organisation and across a range of attributes, and iii) resilience to changes in flow, climate and other non-flow drivers, and to assess the trends in these components across multiple temporal and spatial scales.
Table 1 provide an example of the information that could be collated to help characterise condition for two NWV communities, ephemeral herbfield vegetation, and submerged macrophyte communities. This applies the concepts from Figure 1. While these examples are both at the community level of ecological organization a similar process could be undertaken for species or landscape mosaics. Socio-cultural and economic functions and values are underrepresented in the current examples and require further research. By having a structured framework, that considers both ecological data and societal values, guidance can be given to water managers to help inform the development of benchmarks, watering objectives and monitoring metrics. There are, however, key pieces of information required to apply such a framework.
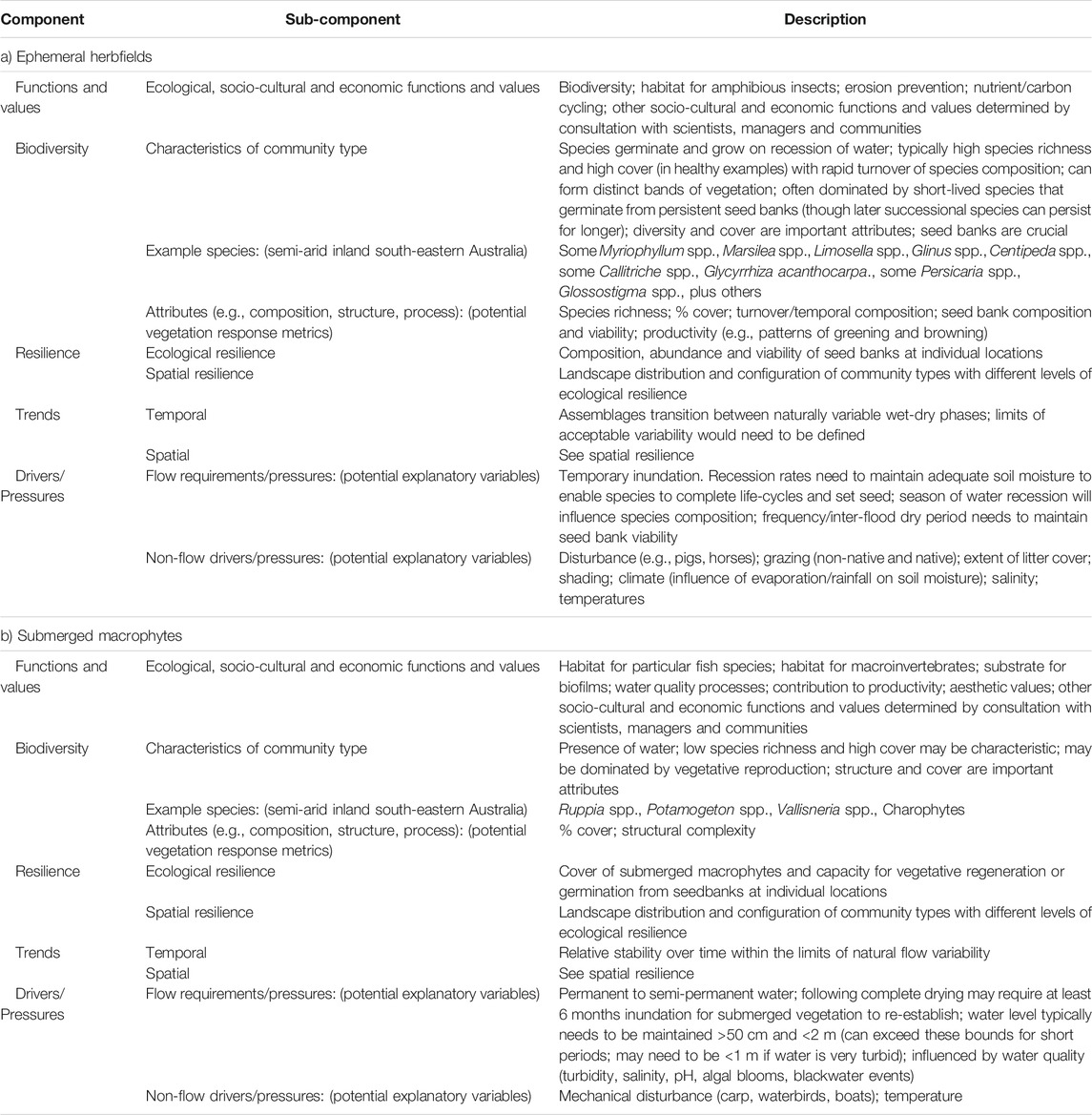
TABLE 1. Example application of the proposed framework for (a) ephemeral herbfield vegetation and (b) submerged macrophyte vegetation. Components used to characterise the condition of non-woody vegetation (see also Figure 1) would be defined by workshopping with stakeholders. Example specific characteristics are provided based on ephemeral herbfields and submerged macrophytes in arid inland south-eastern Australia.
First, condition needs to be described at different levels of ecological organisation and explicitly relate to wet-dry phases. In highly dynamic systems, such as wetlands that cycle through wet-dry phases (Boulton et al., 2014), different points in the wet-dry phase should inform condition. The relative importance or influence of various phases is likely to vary for different wetland types or communities, for example, the inundated phase for submerged vegetation compared with flow recession and the drawdown phase for some ephemeral herbfields. Thapa et al. (2020) examined the productivity response of four vegetation communities to four phases of the wet-dry cycle and acknowledge the need to expand response patterns to wet-dry cycles to other biological attributes such as flowering, seed set and germination. In relation to levels of ecological organisation, a high proportion of studies investigating vegetation responses to flow regimes focus on the level of community (CJC unpublished data). More work is required to define the characteristics of condition at other levels of ecological organisation, such as area, spatial configuration, as well as flow and vegetated connectivity at landscape scales.
Second, expected response trajectories for NWV outcomes need to be defined. These trajectories need to be explicit about the timescale across which responses are expected to occur (Ryo et al., 2019), for example, species may germinate only weeks after inundation, while target cover values or the full complement of species in the seedbank may only be expressed after multiple wet-dry inundation events across multiple years. The state of vegetation prior to management interventions will also impact expected response trajectories (Bond et al., 2018). The flow and non-flow conditions conducive to meeting expected response trajectories also need to be explicitly stated (Bino et al., 2015). For example, a series of drought years with below-average rainfall and above-average temperatures will interact with expected outcomes from environmental flow. Similarly, land use factors such as grazing pressure (Nicol et al., 2007; Souther et al., 2019), animal disturbance (Vilizzi et al., 2014) and nutrient runoff (Smith 2003) are also likely to impact outcomes from environmental flows. Expected responses also need to provide an indication of the shape of the trajectory, be that a return to some “pre-disturbance” condition state, slowing the rate of decline, or facilitating transition to novel but functional systems (Hobbs et al., 2014).
Third, there needs to be a better understanding of the ecological, socio-cultural and economic functions and values provided by different types of vegetation outcomes as well as appropriate metrics to address these (Capon et al., 2013; Campbell et al., 2021). An additional important consideration is the way in which values and appropriate participation are incorporated as highlighted by Moggridge et al. (2019) and Douglas et al. (2019) in relation to the integration of cultural values into water planning.
Characterisation of NWV condition needs to move beyond single-state, ecologically derived definitions to capture ecological, socio-cultural and economic functions and values (de Groot et al., 2002; Capon et al., 2013), incorporate scale (Rolls et al., 2018) and dynamic temporal trajectories (Ryo et al., 2019), consider trade-offs or transitions to novel ecosystem types (Hobbs et al., 2014), while not losing sight of the inherent value of biodiversity (Dudgeon 2014). Adequate characterisation of condition is required to successfully evaluate dynamic NWV outcomes to environmental flows. The ability to evaluate outcomes is critical in terms of learning by doing to inform future management activities (Conallin et al., 2018; Watts et al., 2020). Evaluation also improves the theoretical basis for predicting outcomes (Bullock et al., 2011; Brudvig et al., 2017; Török and Helm 2017), is an important component in achieving the aims of restoration projects (Prach et al., 2019), such as environmental flows, and for effective communication of and engagement with outcomes (Conallin et al., 2018). Improving the health of our rivers, wetlands and floodplains is important not only in terms of supporting ecosystems but in terms of the deep connection people have with these environments that affects their physical and mental wellbeing (Russell et al., 2013; Reeves et al., 2021). How we characterise and evaluate condition may have benefits to both ecosystem health and how people connect with environmental flows and the rivers, wetlands and floodplains to which they’re delivered.
Recommendations
We conclude by proposing recommendations for the future of NWV monitoring and evaluation, particularly at large spatial scales:
• Develop indicators of condition at different levels of ecological organisation that incorporate biodiversity, ecological, socio-cultural and economic functions and values, and resilience
• Undertake monitoring and evaluation across multiple levels of ecological organisation to address biodiversity, functions and values and resilience, for example:
o Population or species level: e.g., monitoring of individual species or populations of interest, such as rare and threatened species, species significantly contributing to the character of Ramsar sites, important food or habitat for fauna, or species identified for other values such as importance to aboriginal people.
o Community level: e.g., monitoring, at both the field level and via remote sensing, of community assemblages and dynamics at multiple spatiotemporal scales in terms of the contribution to biodiversity and functional processes.
o Vegscape level: e.g., monitoring of spatiotemporal configuration of ecosystem patches and evaluating the requirements for long-term resilience, for example, what and how much, where and when?
• Undertake research to establish the links between different levels of ecological organisation and the influence of flow and non-flow drivers, e.g., species or community assemblages associated with different ecosystem types, different flow regimes, and other non-flow drivers
• Establish trajectories of expected responses for defined outcomes that incorporate different flow scenarios and scenarios for key non-flow drivers such as climate
• Invest in better understanding the ecological, socio-cultural and economic functions and values of NWV, e.g., how these change in space and time, appropriate indicators for monitoring, and appropriate ways of integrating values.
• Establish processes to synthesize outcomes and knowledge across multiple levels of ecological organisation
Data Availability Statement
The original contributions presented in the study are included in the article/supplementary material, further inquiries can be directed to the corresponding author.
Author Contributions
Conceptualisation, CC, RT, SC, and FD; visualisation, CC, FD; writing—original draft preparation, CC; writing—review and editing, CC, RT, SC, and FD. All authors listed have made a substantial, direct, and intellectual contribution to the work and approved it for publication.
Funding
CC has funding from an Australian Government Research Training Program (RTP) Scholarship and a top-up scholarship from the Commonwealth Environmental Water Office’s (CEWO) Basin-scale Monitoring Evaluation and Research project (Flow-MER). FD, RT, and SC are supervisors and their role in the paper had no specific funding.
Conflict of Interest
The authors have, over many years, received funding from various Australian government departments, such as the Murray Darling Basin Authority and the Commonwealth Environmental Water Office, to undertake river and wetland research, and to provide advice on technical issues and policy implications.
Publisher’s Note
All claims expressed in this article are solely those of the authors and do not necessarily represent those of their affiliated organizations, or those of the publisher, the editors and the reviewers. Any product that may be evaluated in this article, or claim that may be made by its manufacturer, is not guaranteed or endorsed by the publisher.
Acknowledgments
The authors acknowledge the broader teams as part of the Long Term Intervention Monitoring (LTIM), Environmental Water Knowledge and Research (EWKR) and Flow-MER projects for discussions around condition and evaluation.
References
Arsénio, P., Rodríguez‐González, P. M., Bernez, I., S. Dias, F., Bugalho, M. N., and Dufour, S. (2020). Riparian Vegetation Restoration: Does Social Perception Reflect Ecological Value. River Res. Applic 36 (6), 907–920. doi:10.1002/rra.3514
Arthington, A. H., Kennen, J. G., Stein, E. D., and Webb, J. A. (2018). Recent Advances in Environmental Flows Science and Water Management-Innovation in the Anthropocene. Freshw. Biol. 63 (8), 1022–1034. doi:10.1111/fwb.13108
Baldwin, D. S., Rees, G. N., Wilson, J. S., Colloff, M. J., Whitworth, K. L., Pitman, T. L., et al. (2013). Provisioning of Bioavailable Carbon between the Wet and Dry Phases in a Semi-arid Floodplain. Oecologia 172 (2), 539–550. doi:10.1007/s00442-012-2512-8
Balmford, A., Beresford, J., Green, J., Naidoo, R., Walpole, M., and Manica, A. (2009). A Global Perspective on Trends in Nature-Based Tourism. Plos Biol. 7 (6), e1000144. doi:10.1371/journal.pbio.1000144
Bice, C. M., Gehrig, S. L., Zampatti, B. P., Nicol, J. M., Wilson, P., Leigh, S. L., et al. (2014). Flow-induced Alterations to Fish Assemblages, Habitat and Fish-Habitat Associations in a Regulated lowland River. Hydrobiologia 722 (1), 205–222. doi:10.1007/s10750-013-1701-8
Bino, G., Sisson, S. A., Kingsford, R. T., Thomas, R. F., and Bowen, S. (2015). Developing State and Transition Models of Floodplain Vegetation Dynamics as a Tool for Conservation Decision-Making: a Case Study of the Macquarie Marshes Ramsar Wetland. J. Appl. Ecol. 52 (3), 654–664. doi:10.1111/1365-2664.12410
Bond, N., Costelloe, J., King, A., Warfe, D., Reich, P., and Balcombe, S. (2014). Ecological Risks and Opportunities from Engineered Artificial Flooding as a Means of Achieving Environmental Flow Objectives. Front. Ecol. Environ. 12 (7), 386–394. doi:10.1890/130259
Bond, N. R., Grigg, N., Roberts, J., McGinness, H., Nielsen, D., O'Brien, M., et al. (2018). Assessment of Environmental Flow Scenarios Using State-And-Transition Models. Freshw. Biol. 63, 804–816. doi:10.1111/fwb.13060
Boulton, A. J., Brock, M. A., Robson, B. J., Chambers, J. M., and Davis, J. A. (2014). Australian Freshwater Ecology: Processes and Management. Second Edition. West Sussex, UK: John Wiley & Sons.
Bowen, S. M. (2019). Quantifying the Water Needs of Flood-dependent Plant Communities in the Macquaries Marshes, South-Eastern AustraliaDoctor of Philosophy. Sydney: University of Technology.
Brock, M. A. (2011). Persistence of Seed banks in Australian Temporary Wetlands. Freshw. Biol. 56 (7), 1312–1327. doi:10.1111/j.1365-2427.2010.02570.x
Brooks, S. (2020). 2019-19 Murray-Darling Basin Long Term Intervention Monitoring Project - 2018-19 Basin-scale Evaluation of Commonwealth Environmental Water - Ecosystem Diversity, 248. Final Report prepared for the Department of Agriculture, Water and the Environment, Commonwealth Environmental Water Office by La Trobe University, Centre for Freshwater Ecosystems, CFE Publication, 54. May 2020.
Brooks, S. (2021). Basin-scale Evaluation of 2019-20 Commonwealth Water: Ecosystem Diversity Flow-MER Program. Canberra, Australia: Commonwealth Environmental Water Office (CEWO): Monitoring, Evaluation and Research Program, Department of Agriculture, Water and the Environment, 81pp.
Brudvig, L. A., Barak, R. S., Bauer, J. T., Caughlin, T. T., Laughlin, D. C., Larios, L., et al. (2017). Interpreting Variation to advance Predictive Restoration Science. J. Appl. Ecol. 54 (4), 1018–1027. doi:10.1111/1365-2664.12938
Bullock, J. M., Aronson, J., Newton, A. C., Pywell, R. F., and Rey-Benayas, J. M. (2011). Restoration of Ecosystem Services and Biodiversity: Conflicts and Opportunities. Trends Ecol. Evol. 26 (10), 541–549. doi:10.1016/j.tree.2011.06.011
Cambridge-English-Dictionary. (2020). Meaning of Evaluate in English. (Accessed , December 2020), Available at: https://dictionary.cambridge.org/dictionary/english/evaluate?q=evaluation%29
Campbell, C. J., Freestone, F. L., Duncan, R. P., Higgisson, W., and Healy, S. J. (2021). The More the Merrier: Using Environmental Flows to Improve Floodplain Vegetation Condition. Mar. Freshw. Res. 72 (8), 1185. doi:10.1071/mf20303
Campbell, C. J., James, C. S., Morris, K., Nicol, J. M., Thomas, R. F., Nielsen, D. L., et al. (2021). Blue, green and In-Between: Objectives and Approaches for Evaluating Wetland Flow Regimes Based on Vegetation Outcomes. Mar. Freshw. Res. doi:10.1071/mf20338
Capon, S. J., Chambers, L. E., Mac Nally, R., Naiman, R. J., Davies, P., Marshall, N., et al. (2013). Riparian Ecosystems in the 21st Century: Hotspots for Climate Change Adaptation. Ecosystems 16 (3), 359–381. doi:10.1007/s10021-013-9656-1
Capon, S. J., and James, C. (2020). Murray-darling Basin Long Term Intervention Monitoring Project - Vegetation Diversity Report, 249. Final Report prepared for the Department of the Agriculture, Environment and Water, Commonwealth Environmental Water Office by La Trobe University, Centre for Freshwater Ecosystems, CFE Publication, 129pp. May 2020.
Capon, S. J., James, C. S., Mackay, S. J., and Bunn, S. E. (2009). Environmental Watering for Understorey and Aquatic Vegetation in the Living Murray Icon Sites: A Literature Review and Identification of Research Priorities Relevant to the Environmental Watering Actions of Flow Enhancement and Retaining Floodwater on Floodplains, Report to the Murray-Darling Basin Authority.
Capon, S. J., and Reid, M. A. (2016). Vegetation Resilience to Mega-Drought along a Typical Floodplain Gradient of the Southern Murray-Darling Basin, Australia. J. Veg Sci. 27 (5), 926–937. doi:10.1111/jvs.12426
Carpenter, S. R., and Lodge, D. M. (1986). Efects of Submersed Macrophytes on Ecosystem Processes. Aquat. Bot. 26 (3-4), 341–370. doi:10.1016/0304-3770(86)90031-8
Casanova, M. (2015). Review of Water Requirements for Key Floodplain Vegetation for the Northern Basin: Literature Review and Expert Knowledge Assessment. Lake Bolac, Report to the Murray-Darling Basin Authority. Charophyte Services.
Chambers, J. C., Allen, C. R., and Cushman, S. A. (2019). Operationalizing Ecological Resilience Concepts for Managing Species and Ecosystems at Risk. Front. Ecol. Evol. 7. doi:10.3389/fevo.2019.00241
Choi, J.-Y., Jeong, K.-S., Kim, S.-K., La, G.-H., Chang, K.-H., and Joo, G.-J. (2014). Role of Macrophytes as Microhabitats for Zooplankton Community in Lentic Freshwater Ecosystems of South Korea. Ecol. Inform. 24, 177–185. doi:10.1016/j.ecoinf.2014.09.002
Clarke, P. J., Bell, D. M., and Lawes, M. J. (2015). Testing the Shifting Persistence Niche Concept: Plant Resprouting along Gradients of Disturbance. The Am. Naturalist 185 (6), 747–755. doi:10.1086/681160
Combroux, I., Bornette, G., Willby, N. J., and Amoros, C. (2001). Regenerative Strategies of Aquatic Plants in Disturbed Habitats: the Role of the Propagule Bank. Archiv Fur Hydrobiologie 152 (2), 215–235.
Conallin, J., McLoughlin, C. A., Campbell, J., Knight, R., Bright, T., and Fisher, I. (2018). Stakeholder Participation in Freshwater Monitoring and Evaluation Programs: Applying Thresholds of Potential Concern within Environmental Flows. Environ. Manage. 61 (3), 408–420. doi:10.1007/s00267-017-0940-2
Conroy, C., Knight, A. R., Wassens, S., and Allan, C. (2019). Consequences of Changed Water Management for Aboriginal Australians in the Murrumbidgee Catchment, NSW. Aust. Geographer 50 (2), 169–184. doi:10.1080/00049182.2018.1545275
Cook, C. N., Carter, R. W., Fuller, R. A., and Hockings, M. (2012). Managers Consider Multiple Lines of Evidence Important for Biodiversity Management Decisions. J. Environ. Manage. 113, 341–346. doi:10.1016/j.jenvman.2012.09.002
Cottet, M., Piégay, H., and Bornette, G. (2013). Does Human Perception of Wetland Aesthetics and Healthiness Relate to Ecological Functioning. J. Environ. Manage. 128, 1012–1022. doi:10.1016/j.jenvman.2013.06.056
CSIRO and Bureau of Meteorology (2015). Climate Change in Australia Information for Australia's Natural Resource Management Regions: Technical Report. Australia: CSIRO and Bureau of Meteorology.
Dare, M., Schirmer, J., and Vanclay, F. (2014). Community Engagement and Social Licence to Operate. Impact Assess. Project Appraisal 32 (3), 188–197. doi:10.1080/14615517.2014.927108
de Groot, R. S., Wilson, M. A., and Boumans, R. M. J. (2002). A Typology for the Classification, Description and Valuation of Ecosystem Functions, Goods and Services. Ecol. Econ. 41 (3), 393–408. doi:10.1016/s0921-8009(02)00089-7
DELWP (2016). Quality Assessment Metric for 'Seasonal Herbaceous Wetlands', Melbourne Strategic Assessment, the State of Victoria. Land, Water and Planning: Department of Environment.
Docker, B. B., and Hubble, T. C. T. (2008). Quantifying Root-Reinforcement of River Bank Soils by Four Australian Tree Species. Geomorphology 100 (3-4), 401–418. doi:10.1016/j.geomorph.2008.01.009
Douglas, M. M., Jackson, S., Canham, C. A., Laborde, S., Beesley, L., Kennard, M. J., et al. (2019). Conceptualizing Hydro-Socio-Ecological Relationships to Enable More Integrated and Inclusive Water Allocation Planning. One Earth 1 (3), 361–373. doi:10.1016/j.oneear.2019.10.021
Dudgeon, D. (2014). Accept No Substitute: Biodiversity Matters. Aquat. Conserv: Mar. Freshw. Ecosyst. 24 (4), 435–440. doi:10.1002/aqc.2485
Dudgeon, D. (2019). Multiple Threats Imperil Freshwater Biodiversity in the Anthropocene. Curr. Biol. 29 (19), R960–R967. doi:10.1016/j.cub.2019.08.002
Dyer, F., Campbell, C., Higgisson, W., Tschierschke, A., and Doody, T. M. (2021). Basin-scale Evaluatio of 2019-20 Commonwealth Environmental Water: Vegetation Flow-MER Program. Canberra, AustraliaAustralia: Commonwealth Environmental Water Office (CEWO): Monitoring, Evaluation and Research Program, Department of Agriculture, Water and the Environment, 152pp.
Flitcroft, R. L., Bottom, D. L., Haberman, K. L., Bierly, K. F., Jones, K. K., Simenstad, C. A., et al. (2016). Expect the Unexpected: Place-Based Protections Can lead to Unforeseen Benefits. Aquat. Conserv: Mar. Freshw. Ecosyst. 26, 39–59. doi:10.1002/aqc.2660
Galbraith, M., Towns, D. R., Bollard, B., and MacDonald, E. A. (2021). Ecological Restoration success from Community and agency Perspectives: Exploring the Differences. Restoration Ecol. 29 (5). doi:10.1111/rec.13405
Gari, S. R., Newton, A., and Icely, J. D. (2015). A Review of the Application and Evolution of the DPSIR Framework with an Emphasis on Coastal Social-Ecological Systems. Ocean Coastal Manage. 103, 63–77. doi:10.1016/j.ocecoaman.2014.11.013
Gawne, B., Hale, J., Stewardson, M. J., Webb, J. A., Ryder, D. S., Brooks, S. S., et al. (2019). Monitoring of Environmental Flow Outcomes in a Large River basin: The Commonwealth Environmental Water Holder's Long-Term Intervention in the Murray-Darling Basin. Australia: River Research and Applications.
Gibbons, P., and Freudenberger, D. (2006). An Overview of Methods Used to Assess Vegetation Condition at the Scale of the Site. Ecol. Manage. Restor 7 (s1), S10–S17. doi:10.1111/j.1442-8903.2006.00286.x
Gibbons, P., Zerger, A., Jones, S., and Ryan, P. (2006). Mapping Vegetation Condition in the Context of Biodiversity Conservation. Ecol. Manage. Restor 7 (s1), S1–S2. doi:10.1111/j.1442-8903.2006.00282.x
Gitau, P. N., Ndiritu, G. G., and Gichuki, N. N. (2019). Ecological, Recreational and Educational Potential of a Small Artificial Wetland in an Urban Environment. Afr. J. Aquat. Sci. doi:10.2989/16085914.2019.1663721
González López, A., and Amérigo Cuervo-Arango, M. (2008). Relationship Among Values, Beliefs, Norms and Ecological Behaviour. Psicothema 20 (4), 623–629.
Greet, J., Angus Webb, J., and Cousens, R. D. (2011). The Importance of Seasonal Flow Timing for Riparian Vegetation Dynamics: a Systematic Review Using Causal Criteria Analysis. Freshw. Biol. 56 (7), 1231–1247. doi:10.1111/j.1365-2427.2011.02564.x
Haines-Young, R., Potschin, M., and Kienast, F. (2012). Indicators of Ecosystem Service Potential at European Scales: Mapping Marginal Changes and Trade-Offs. Ecol. Indicators 21, 39–53. doi:10.1016/j.ecolind.2011.09.004
Harrison, P. A., Vandewalle, M., Sykes, M. T., Berry, P. M., Bugter, R., de Bello, F., et al. (2010). Identifying and Prioritising Services in European Terrestrial and Freshwater Ecosystems. Biodivers Conserv 19 (10), 2791–2821. doi:10.1007/s10531-010-9789-x
Hausmann, A., Toivonen, T., Fink, C., Heikinheimo, V., Kulkarni, R., Tenkanen, H., et al. (2020). Understanding Sentiment of national park Visitors from Social media Data. People Nat. 2 (3), 750–760. doi:10.1002/pan3.10130
Higgisson, W., Doody, T. M., Campbell, C., and Dyer, F. J. (2021). The Response to Environmental Flows of a Culturally Significant Flood-dependent Species: Centipeda Cunninghamii (Asteraceae). Mar. Freshw. Res. 72 (7), 1086–1091. doi:10.1071/mf20314
Hobbs, R. J., Higgs, E., Hall, C. M., Bridgewater, P., Chapin, F. S., Ellis, E. C., et al. (2014). Managing the Whole Landscape: Historical, Hybrid, and Novel Ecosystems. Front. Ecol. Environ. 12 (10), 557–564. doi:10.1890/130300
Horne, A. C., Webb, J. A., O'Donnell, E., Arthington, A. H., McClain, M., Bond, N., et al. (2017). Research Priorities to Improve Future Environmental Water Outcomes. Front. Environ. Sci. 5, 89. doi:10.3389/fenvs.2017.00089
Horne, A., Webb, A., Stewardson, M., Richter, B., and Acreman, M. (2017). Water for the Environment : From Policy and Science through to Implementation. Saint Louis, UNITED STATES: Elsevier Science & Technology.
Hunter, J. T. (2021). Vegetation Change in Semi-permanent or Ephemeral Montane Marshes (Lagoons) of the New England Tablelands Bioregion. Aust. J. Bot. doi:10.1071/bt20028
Jackson, S., Pollino, C., Maclean, K., Bark, R., and Moggridge, B. (2015). Meeting Indigenous Peoples' Objectives in Environmental Flow Assessments: Case Studies from an Australian Multi-Jurisdictional Water Sharing Initiative. J. Hydrol. 522, 141–151. doi:10.1016/j.jhydrol.2014.12.047
Jackson, W. J. (2017). Australia State of the Environment 2016: Approach. Canberra, Independent Report to the Australian Government Minister for Environment and Energy. Australian Government Department of the Environment and Energy.
Jähnig, S. C., Lorenz, A. W., Hering, D., Antons, C., Sundermann, A., Jedicke, E., et al. (2011). River Restoration success: a Question of Perception. Ecol. Appl. 21 (6), 2007–2015. doi:10.1890/10-0618.1
James, C. S., Capon, S. J., and Quinn, G. P. (2015). Nurse Plant Effects of a Dominant Shrub (Duma Florulenta ) on Understorey Vegetation in a Large, Semi-arid Wetland in Relation to Flood Frequency and Drying. J. Veg Sci. 26 (5), 985–994. doi:10.1111/jvs.12297
Jax, K., and Heink, U. (2015). Searching for the Place of Biodiversity in the Ecosystem Services Discourse. Biol. Conservation 191, 198–205. doi:10.1016/j.biocon.2015.06.032
Keddy, P. A. (2010). Wetland Ecology: Principles and Conservation. Cambridge, UK: Cambridge University Press.
Kingsford, R. T., and Thomas, R. F. (2004). Destruction of Wetlands and Waterbird Populations by Dams and Irrigation on the Murrumbidgee River in Arid Australia. Environ. Manage. 34 (3), 383–396. doi:10.1007/s00267-004-0250-3
Konrad, C. P., Olden, J. D., Lytle, D. A., Melis, T. S., Schmidt, J. C., Bray, E. N., et al. (2011). Large-scale Flow Experiments for Managing River Systems. Bioscience 61 (12), 948–959. doi:10.1525/bio.2011.61.12.5
Leblanc, M., Tweed, S., Van Dijk, A., and Timbal, B. (2012). A Review of Historic and Future Hydrological Changes in the Murray-Darling Basin. Glob. Planet. Change 80-81, 226–246. doi:10.1016/j.gloplacha.2011.10.012
Liguori, A., McEwen, L., Blake, J., and Wilson, M. (2021). Towards 'Creative Participatory Science': Exploring Future Scenarios through Specialist Drought Science and Community Storytelling. Front. Environ. Sci. 8. doi:10.3389/fenvs.2020.589856
Lindenmayer, D., Hobbs, R. J., Montague-Drake, R., Alexandra, J., Bennett, A., Burgman, M., et al. (2008). A Checklist for Ecological Management of Landscapes for Conservation. Ecol. Lett. 11 (1), 78–91. doi:10.1111/j.1461-0248.2007.01114.x
Liu, H., Zheng, L., Wu, J., and Liao, Y. (2020). Past and Future Ecosystem Service Trade-Offs in Poyang Lake Basin under Different Land Use Policy Scenarios. Arabian J. Geosciences 13 (2). doi:10.1007/s12517-019-5004-x
Ma, Z., Cai, Y., Li, B., and Chen, J. (2010). Managing Wetland Habitats for Waterbirds: An International Perspective. Wetlands 30 (1), 15–27. doi:10.1007/s13157-009-0001-6
Maheshwari, B. L., Walker, K. F., and McMahon, T. A. (1995). Effects of Regulation on the Flow Regime of the River Murray, Australia. Regul. Rivers: Res. Mgmt. 10 (1), 15–38. doi:10.1002/rrr.3450100103
Marchand, L., Castagneyrol, B., Jimenez, J. J., Benayas, J. M. R., Benot, M.-L., Martinez-Ruiz, C., et al. (2021). Conceptual and Methodological Issues in Estimating the success of Ecological Restoration. Ecol. Indicators 123. doi:10.1016/j.ecolind.2021.107362
Marden, M., Rowan, D., and Phillips, C. (2005). Stabilising Characteristics of New Zealand Indigenous Riparian Colonising Plants. Plant and Soil 278 (1-2), 95–105. doi:10.1007/s11104-004-7598-2
McGinness, H. M., Arthur, A. D., and Davies, M. (2018). Flood Regimes Driving Vegetation and Bird Community Transitions in Semiarid Floodplain Woodlands. Ecohydrology 11 (5). doi:10.1002/eco.1954
McGinness, H. M., Arthur, A. D., Ward, K. A., and Ward, P. A. (2014). Floodplain Amphibian Abundance: Responses to Flooding and Habitat Type in Barmah Forest, Murray River, Australia. Wildl. Res. 41 (2), 149–162. doi:10.1071/wr13224
McLoughlin, C. A., Thoms, M. C., and Parsons, M. (2020). Reflexive Learning in Adaptive Management: A Case Study of Environmental Water Management in the Murray Darling Basin, Australia. River Res. Applic 36 (4), 681–694. doi:10.1002/rra.3607
Moggridge, B. J., Betterridge, L., and Thompson, R. M. (2019). Integrating Aboriginal Cultural Values into Water Planning: a Case Study from New South Wales, Australia. Australasian J. Environ. Manage. 26 (3), 273–286. doi:10.1080/14486563.2019.1650837
Moggridge, B. J., and Thompson, R. M. (2021). Cultural Value of Water and Western Water Management: an Australian Indigenous Perspective. Australasian Journal of Water Resources. doi:10.1080/13241583.2021.1897926
Neary, V. S., Constantinescu, S. G., Bennett, S. J., and Diplas, P. (2012). Effects of Vegetation on Turbulence, Sediment Transport, and Stream Morphology. J. Hydraul. Eng. 138 (9), 765–776. doi:10.1061/(asce)hy.1943-7900.0000168
Nicol, J., Muston, S., D'Santos, P., McCarthy, B., and Zukowski, S. (2007). Impact of Sheep Grazing on the Soil Seed Bank of a Managed Ephemeral Wetland: Implications for Management. Aust. J. Bot. 55 (2), 103–109. doi:10.1071/bt04137
Noss, R. F. (1990). Indicators for Monitoring Biodiversity: A Hierarchical Approach. Conservation Biol. 4 (4), 355–364. doi:10.1111/j.1523-1739.1990.tb00309.x
Okumah, M., Yeboah, A. S., and Amponsah, O. (2020). Stakeholders' Willingness and Motivations to Support Sustainable Water Resources Management: Insights from a Ghanaian Study. Conservation Sci. Pract. 2 (3). doi:10.1111/csp2.170
Overton, I., Pollino, C., Roberts, J., Reid, J., Bond, N., McGinness, H., et al. (2014). Development of the MurrayDarling Basin Plan SDL Adjustment Ecological Elements Method. Report prepared by. Canberra, Australia: CSIRO for the Murray–Darling Basin Authority, 190.
Palmer, M. A., Bernhardt, E. S., Allan, J. D., Lake, P. S., Alexander, G., Brooks, S., et al. (2005). Standards for Ecologically Successful River Restoration. J. Appl. Ecol. 42 (2), 208–217. doi:10.1111/j.1365-2664.2005.01004.x
Parkes, D., and Lyon, P. (2006). Towards a National Approach to Vegetation Condition Assessment that Meets Government Investors' Needs: A Policy Perspective. Ecol. Manage. Restor 7 (s1), S3–S5. doi:10.1111/j.1442-8903.2006.00283.x
Poff, N. L. (2018). Beyond the Natural Flow Regime? Broadening the Hydro-Ecological Foundation to Meet Environmental Flows Challenges in a Non-stationary World. Freshw. Biol. 63 (8), 1011–1021. doi:10.1111/fwb.13038
Pollard, S., du Toit, D., and Biggs, H. (2011). River Management under Transformation: The Emergence of Strategic Adaptive Management of River Systems in the Kruger National Park. Koedoe 53 (2). doi:10.4102/koedoe.v53i2.1011
Prach, K., Durigan, G., Fennessy, S., Overbeck, G. E., Torezan, J. M., and Murphy, S. D. (2019). A Primer on Choosing Goals and Indicators to Evaluate Ecological Restoration success. Restor Ecol. 27 (5), 917–923. doi:10.1111/rec.13011
Reeder, B. C. (2011). Assessing Constructed Wetland Functional success Using Diel Changes in Dissolved Oxygen, pH, and Temperature in Submerged, Emergent, and Open-Water Habitats in the Beaver Creek Wetlands Complex, Kentucky (USA). Ecol. Eng. 37 (11), 1772–1778. doi:10.1016/j.ecoleng.2011.06.018
Reeves, J. P., John, C. H. D., Wood, K. A., and Maund, P. R. (2021). A Qualitative Analysis of UK Wetland Visitor Centres as a Health Resource. Int. J. Environ. Res. Public Health 18 (16). doi:10.3390/ijerph18168629
Reid, A. J., Carlson, A. K., Creed, I. F., Eliason, E. J., Gell, P. A., Johnson, P. T. J., et al. (2019). Emerging Threats and Persistent Conservation Challenges for Freshwater Biodiversity. Biol. Rev. 94 (3), 849–873. doi:10.1111/brv.12480
Reis, V., Hermoso, V., Hamilton, S. K., Ward, D., Fluet-Chouinard, E., Lehner, B., et al. (2017). A Global Assessment of Inland Wetland Conservation Status. Bioscience 67 (6), 523–533. doi:10.1093/biosci/bix045
Rolls, R. J., Heino, J., Ryder, D. S., Chessman, B. C., Growns, I. O., Thompson, R. M., et al. (2018). Scaling Biodiversity Responses to Hydrological Regimes. Biol. Rev. 93 (2), 971–995. doi:10.1111/brv.12381
Russell, R., Guerry, A. D., Balvanera, P., Gould, R. K., Basurto, X., Chan, K. M. A., et al. (2013). Humans and Nature: How Knowing and Experiencing Nature Affect Well-Being. Annu. Rev. Environ. Resour. 3838, 473–502. doi:10.1146/annurev-environ-012312-110838
Ryo, M., Aguilar-Trigueros, C. A., Pinek, L., Muller, L. A. H., and Rillig, M. C. (2019). Basic Principles of Temporal Dynamics. Trends Ecol. Evol. 34 (8), 723–733. doi:10.1016/j.tree.2019.03.007
Saha, D., Das, D., Dasgupta, R., and Patel, P. P. (2020). Application of Ecological and Aesthetic Parameters for Riparian Quality Assessment of a Small Tropical River in Eastern India. Ecol. Indicators 117. doi:10.1016/j.ecolind.2020.106627
Santamaría, L. (2002). Why Are Most Aquatic Plants Widely Distributed? Dispersal, Clonal Growth and Small-Scale Heterogeneity in a Stressful Environment. Acta Oecologica 23 (3), 137–154. doi:10.1016/s1146-609x(02)01146-3
Siikamäki, P., Kangas, K., Paasivaara, A., and Schroderus, S. (2015). Biodiversity Attracts Visitors to National parks. Biodivers Conserv 24 (10), 2521–2534. doi:10.1007/s10531-015-0941-5
Sinclair, S. J., Bruce, M. J., Griffioen, P., Dodd, A., and White, M. D. (2018). A Condition Metric for Eucalyptus woodland Derived from Expert Evaluations. Conservation Biol. 32 (1), 195–204. doi:10.1111/cobi.12941
Sinclair, S. J., Griffioen, P., Duncan, D. H., Millett-Riley, J. E., and White, M. D. (2015). Quantifying Ecosystem Quality by Modeling Multi-Attribute Expert Opinion. Ecol. Appl. 25 (6), 1463–1477. doi:10.1890/14-1485.1
Smith, V. H. (2003). Eutrophication of Freshwater and Coastal marine Ecosystems a Global Problem. Environ. Sci. Pollut. Res. 10 (2), 126–139. doi:10.1065/espr2002.12.142
Souther, S., Loeser, M., Crews, T. E., and Sisk, T. (2019). Complex Response of Vegetation to Grazing Suggests Need for Coordinated, Landscape-Level Approaches to Grazing Management. Glob. Ecol. Conservation 20. doi:10.1016/j.gecco.2019.e00770
Sterling, E. J., Zellner, M., Jenni, K. E., Leong, K., Glynn, P. D., BenDor, T. K., et al. (2019). Try, Try Again: Lessons Learned from success and Failure in Participatory Modeling. Elementa-Science of the Anthropocene 7. doi:10.1525/elementa.347
Tear, T. H., Kareiva, P., Angermeier, P. L., Comer, P., Czech, B., Kautz, R., et al. (2005). How Much Is Enough? the Recurrent Problem of Setting Measurable Objectives in Conservation. BioScience 55 (10), 835–849. doi:10.1641/0006-3568(2005)055[0835:hmietr]2.0.co;2
Thapa, R., Thoms, M. C., Reid, M., and Parsons, M. (2020). Do adaptive Cycles of Floodplain Vegetation Response to Inundation Differ Among Vegetation Communities. River Res. Applic 36 (4), 553–566. doi:10.1002/rra.3538
Thoms, M. C., Beyer, P. J., Rogers, K. H., and Kingsford, R. (2006). “Variability, Complexity and Diversity: the Geomorphology of River Ecosystems in Dryland Regions,” in Ecology of Desert Rivers (New York: Cambridge University Press).
Török, P., and Helm, A. (2017). Ecological Theory Provides strong Support for Habitat Restoration. Biol. Conservation 206, 85–91. doi:10.1016/j.biocon.2016.12.024
Tscherning, K., Helming, K., Krippner, B., Sieber, S., and Paloma, S. G. y. (2012). Does Research Applying the DPSIR Framework Support Decision Making. Land Use Policy 29 (1), 102–110. doi:10.1016/j.landusepol.2011.05.009
Tulbure, M. G., and Broich, M. (2019). Spatiotemporal Patterns and Effects of Climate and Land Use on Surface Water Extent Dynamics in a Dryland Region with Three Decades of Landsat Satellite Data. Sci. Total Environ. 658, 1574–1585. doi:10.1016/j.scitotenv.2018.11.390
Valinoti, C. E., Ho, C.-K., and Armitage, A. R. (2011). Native and Exotic Submerged Aquatic Vegetation Provide Different Nutritional and Refuge Values for Macroinvertebrates. J. Exp. Mar. Biol. Ecol. 409 (1-2), 42–47. doi:10.1016/j.jembe.2011.08.001
Vietz, G. J., Lintern, A., Webb, J. A., and Straccione, D. (2018). River Bank Erosion and the Influence of Environmental Flow Management. Environ. Manage. 61 (3), 454–468. doi:10.1007/s00267-017-0857-9
Vilizzi, L., Thwaites, L. A., Smith, B. B., Nicol, J. M., and Madden, C. P. (2014). Ecological Effects of Common Carp (Cyprinus carpio) in a Semi-arid Floodplain Wetland. Mar. Freshw. Res. 65 (9), 802–817. doi:10.1071/mf13163
Warfe, D. M., and Barmuta, L. A. (2006). Habitat Structural Complexity Mediates Food Web Dynamics in a Freshwater Macrophyte Community. Oecologia 150 (1), 141–154. doi:10.1007/s00442-006-0505-1
Wassens, S., Saintilan, N., and Overton, I. (2010). Flooding Regimes for Frogs in lowland Rivers of the Murray-Darling Basin.
Watts, R. J., Dyer, F., Frazier, P., Gawne, B., Marsh, P., Ryder, D. S., et al. (2020). Learning from Concurrent Adaptive Management in Multiple Catchments within a Large Environmental Flows Program in Australia. River Res. Applic 36 (4), 668–680. doi:10.1002/rra.3620
Webb, J. A., Little, S. C., Miller, K. A., Stewardson, M. J., Rutherfurd, I. D., Sharpe, A. K., et al. (2015). A General Approach to Predicting Ecological Responses to Environmental Flows: Making Best Use of the Literature, Expert Knowledge, and Monitoring Data. River Res. Applic. 31 (4), 505–514. doi:10.1002/rra.2832
Webb, J. A., Wallis, E. M., and Stewardson, M. J. (2012). A Systematic Review of Published Evidence Linking Wetland Plants to Water Regime Components. Aquat. Bot. 103, 1–14. doi:10.1016/j.aquabot.2012.06.003
Wineland, S. M., Fovargue, R., York, B., Lynch, A. J., Paukert, C. P., and Neeson, T. M. (2021). Is There Enough Water? How Bearish and Bullish Outlooks Are Linked to Decision Maker Perspectives on Environmental Flows. J. Environ. Manage. 280, 111694. doi:10.1016/j.jenvman.2020.111694
Withers, P. J., and Jarvie, H. P. (2008). Delivery and Cycling of Phosphorus in Rivers: A Review. Sci. Total Environ. 400 (1-3), 379–395. doi:10.1016/j.scitotenv.2008.08.002
Wortley, L., Hero, J.-M., and Howes, M. (2013). Evaluating Ecological Restoration Success: A Review of the Literature. Restor Ecol. 21 (5), 537–543. doi:10.1111/rec.12028
Keywords: vegetation condition assessment, environmental flow, environmental water management, flooding, floodplain, restoration, wetland plants
Citation: Campbell CJ, Thompson RM, Capon SJ and Dyer FJ (2022) Rethinking Condition: Measuring and Evaluating Wetland Vegetation Responses to Water Management. Front. Environ. Sci. 9:801250. doi: 10.3389/fenvs.2021.801250
Received: 25 October 2021; Accepted: 31 December 2021;
Published: 21 January 2022.
Edited by:
Avril C. Horne, the University of Melbourne, AustraliaReviewed by:
Catherine M. Febria, University of Windsor, CanadaAndrea Funk, University of Natural Resources and Life Sciences Vienna, Austria
Copyright © 2022 Campbell, Thompson, Capon and Dyer. This is an open-access article distributed under the terms of the Creative Commons Attribution License (CC BY). The use, distribution or reproduction in other forums is permitted, provided the original author(s) and the copyright owner(s) are credited and that the original publication in this journal is cited, in accordance with accepted academic practice. No use, distribution or reproduction is permitted which does not comply with these terms.
*Correspondence: Cherie J. Campbell, Cherie.Campbell@canberra.edu.au