- 1Key Laboratory for Aerosol-Cloud-Precipition of China Meteorological Administration, Collaborative Innovation Center on Forecast and Evaluation of Meteorological Disaster, Nanjing University of Information Science and Technology, Nanjing, China
- 2State Key Laboratory of Severe Weather, Chinese Academy of Meteorological Sciences, Beijing, China
- 3Pingliang Land Surface Process and Severe Weather Research Station, Gansu Land Surface Process and Severe Weather Observation and Research Station, Chinese Academy of Sciences, Lanzhou, China
In this work, we studied the waveforms of all lightning discharges from about 15 min. Eighty-three percent of all lightning discharges contain particular waveforms called regular pulse bursts (RPBs), which have regular microsecond-scale electric or magnetic field pulses. Maximum proportion of RPBs occur in middle or rear of lightning discharges. Prior to or after RPBs, there is always a chaotic pulse period. The analysis indicated that RPBs are caused by a secondary discharge in the fractured old breakdown channel, likeness to dart-stepped leader occuring in negative cloud-to-ground discharge (-CG). Four types of RPBs, namely, category of normal RPBs, category of back RPBs, category of symmetric RPBs, and category of reversal RPBs, were sorted in the light of the evolution of the pulse amplitude, interval between neighboring pulses and pulse polarity. In addition, the difference between normal RPBs and back RPBs was considered to be caused by the distance between neighboring charge pockets and the magnitude of the charge in every charge pocket. The symmetric RPBs were considered to be caused by a discharge channel with a large central charge area. Reversal RPBs were considered to be caused by a bending channel or superposition of two or more RPBs. We located some RPBs in a typical intra-cloud flash (IC) in three-dimensional. The analysis showed that the developing velocity of RPBs ranged from approximately 1.2 × 106 m/s to 3.0 × 106 m/s, which slower less than both of the dart leader or dart-stepped leader process from previous studies. And we found it is several meters to dozens of meters that the lengths range of discharge step which between two adjacent pulses.
Highlights
• Most lightning discharges have regular pulse bursts.
• The physical mechanisms of different types of RPBs are discussed.
• RPBs are located with high spatial and temporal resolution.
1 Introduction
Researchers have performed excellent work using high-speed cameras or coaxial shunts (Wang, D. H. et al., 2014; Jiang, R. B. et al., 2015; Tran and Rakov, 2017). However, compared with lightning discharges close to the ground (e.g., return strokes and step leaders), the discharge portion of a lightning discharges in the cloud cannot be observed directly by instruments (e.g., high-speed cameras, coaxial shunts, etc.) under certain conditions. Studies rely on radio waveforms detected by ground-based instruments [Rison et al., 1999; Qie et al., 2009; Zhang et al., 2010; Liu et al., 2013; Wang et al., 2021]. Generally, the pulse trains in waveforms generated by lightning discharges can be classified into chaotic pulse trains and regular pulse trains. Chaotic pulse trains were first studied by Wiedman (1982), who pointed out that the subsequent strokes were preceded by ‘chaotic leaders’. Moreover, further studies have been performed on chaotic pulse trains (Bailey et al., 1988; Rakov and Uman, 1990; Willett et al., 1989). Chaotic pulse train events in negative cloud-to-ground (CG) flashes were imaged by three-dimensional very high-frequency (VHF) broadband digital interferometers by Liu et al., 2013, who found that the breakdown process associated with a chaotic pulse train is negative and similar to an attempt leader or dart leader; moreover, they suggested that some chaotic pulse trains may be a part of dart or dart-stepped leaders. Regular pulse trains include stepped leaders or dart-stepped leaders (Beasley et al., 1983; Cooray and Lundquist, 1985; Krider et al., 1977; Weidman and Krider, 1978) in cloud-to-ground and RPBs (Krider et al., 1975; Muller-Hillebrand D., 1962; Rakov et al., 1996; Kolmasová and Santolík, 2013; Ismail et al., 2017) associated with K-changes and M-components. RPBs have high rate of fluctuation of lightning discharges and may play an important role in lightning discharges (Füllekrug Martin, 2011; Kolmasová and Santolík, 2013), and they were earliest studied in detail by Krider et al., 1975, they observed microsecond-scale RPBs in a large part of the waveforms generated by long-distance lightning discharges in Florida and Arizona. These RPBs are basically unipolar pulses. Based on their observations, the RPBs exhibited two principal characteristics, first is a typical duration of 100–400 μs and second is mean time intervals of several microseconds between adjacent pulses. Generally, a pulse lasts for 1–2 μs and the rising edge is about 0.75 μs and followed by a weak overshoot waveform. RPBs usually begin with the largest pulses, and the pulse amplitudes decrease with time. Krider et al., 1975 suggested that the RPBs are related to “an intra-cloud (IC) dart-stepped leader process” and possibly associated with K streamers (Ogawa and Brook, 1964) that developed in the previously formed channels. Muller-Hillebrand (1962) and Krider et al., 1975 suggested that interference or upset can occur in sensitive electronic systems. Rakov et al., 1996 analyzed RPBs in both CG and IC lightning discharges and found that large proportion of RPBs occurred in falling edge of K-changes in both IC and CG. However, Krider et al., 1975 stated that pulse amplitudes often decrease during bursts, and they observed that pulse amplitudes often increase first and then decrease with time and that the interval between adjacent pulses tends to increase toward the end of RPBs (Rakov et al., 1996). According to these observations, Rakov et al., 1996 suggested that an adequate definition of the standard lightning environment needed to develop after considering more experimental data in aircraft-measured lightning currents and in lightning electromagnetic radiation.
Using a multi-point measurement of the time derivative of the electric field with five stations, Davis et al., 1999 estimated the three-demensional locations and develop speeds of the leaders. The average intervals between two pulses of RPBs were 2.8 μs in dart-stepped leaders, 7.6 μs in leaders preceding new ground termination, and 5.1 μs in intra-cloud (IC) discharges respectively. They found that the polarity of 66% pulses was consistent with the current flowing direction in discharge channel. The polarity reversal may be caused by change of channel develop direction and may be caused by development of branches. Kolmasová and Santolík (2013) pointed out that the distances changed between adjacent charge pockets and increase of leader propagation speed could probably also explain the different sort of the trains of the pulses. Ismail et al., 2017 analyzed chaotic pulse bursts and pointed out that RPBs are probably caused by dart-stepped leaders or K-changes in cloud; moreover, they suggested that superposition of electric fields of two and more pulses trains which propagating simultaneously formed chaotic pulse trains. This hypothesis is supported by the observation fact that regular pulse trains tend to ocurred at beginning, middle or later stages of RPBs.
In this work, the RPBs in lightning discharges were analyzed with high time resolution in the inland plateau in China using a lightning mapping system working in VHF and VLF/LF frequency bands (Qie et al., 2009; Zhang et al., 2010; Wang et al., 2010). We analyzed four typical RPBs and mapped some RPBs. A comparative analysis of RPBs parameters in a positive cloud-to-ground discharge (+CG), a negative cloud-to-ground discharge (–CG), and an intra-cloud discharge (IC) are presented. Some location results of RPBs are analyzed and discussed in this work.
2 Observation and Classification of RPBs
In this work, the data was observed by a lightning mapping system (Zhang et al., 2010). The study area was the northeastern edge of the Qinghai-Tibet Plateau (center station: Mingde (MD); 37.01°N, 101.62°E; other station: Yaocao (YC), Liangjiao (LJ), Xiegou (XG), Jile (JL), Miaopu (MP), Xinzhuang (XZ)), and data were collected from 2009 to 2011. The stations were evenly spaced approximately 10 km around the center station, as shown in Figure 1. Each station was arranged with a lightning mapping system detector working at VHF and VLF/LF passband (Qie et al., 2009; Zhang et al., 2010), the bandwidth of VHF detector is about 267–273 MHz and the bandwidth of VLF/LF detector is approximately 200 kHz to 10 MHz. This lightning mapping system can mapped radiation sources which indicate spatial and temporal development of lightning discharges similar to a lightning mapping array (LMA) (Rison et al., 1999). In addition, a high-precision GPS clock (±25 ns) was equipped to synchronize all equipment at each station. Data collection was controlled either by the central station via a broadband wireless network system or operated freely. The arrival time of radiation pulses were caught by all seven stations, and then a set of nonlinear equations was used to get solution of equation each station as follows:
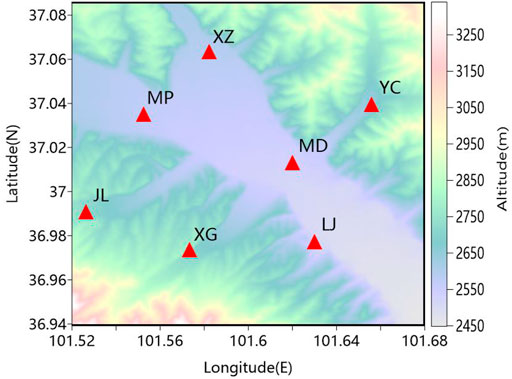
FIGURE 1. Layout of the detectors (unit = meter; longitude and latitude of the central stations = E101.6,200,592 and N37.0133,483, respectively).
We checked all data during a period of 00:00:00–00:15:00 on August 15, 2011 (GMT+8). The results showed that 430 RPBs occurred in 67 cases of all 81 lightning discharges in this period. The rest of the lightning discharges that did not contain RPBs were considered to have lost some detailed sections in their waveforms and occurred far from the observation site in this work. A statistical analysis of all these 430 RPBs shows that the typical width of pulses in RPBs was 1.0 μs, the typical interval between pulses of RPBs was approximately 5.0 μs and the typical duration of RPBs was several hundred μs. Obviously, the ranges of typical intervals and durations of RPBs were greater than those observed by Krider et al., 1975 and Rakov et al., 1996 with the application of broad electric change (EFC) receivers in this paper. A chaotic pulse period always occurred prior to or after an RPB. Maximum proportion of RPBs occur in middle or rear of lightning discharges, which is similar to the observations of Krider et al., 1975 and Rakov et al., 1996. Moreover, most RPBs were associated with VHF radiation. Generally, RPBs were associated with K-changes or M process. RPBs were considered widely distributed events in lightning discharges in this work. We found that the initial part of a normal RPBs is a periodic vibration similar to that observed by Rakov et al., 1996. In addition, some other RPBs did not show regular pulses similarities with those observed by (Rakov et al., 1996; Kolmasová and Santolík, 2013; Ismail et al., 2017). To further understand the RPBs, the waveforms of RPBs are sorted into four categories as follows.
2.1 Normal RPBs
The category of normal RPBs tend to occur in the latter part of a ramp-like [ Rakov et al., 1996] field change (see Figure 2A). The initial part of RPBs waveform has a chaotic wave shape and changes to a periodic vibration. RPBs gradually develop into intensive pulses as the pulse amplitude increases. Then, the pulse amplitude decreases and the interval between two pulses gradually increases with time. It is intense that the VHF radiation synchronized with RPBs. The VHF pulses and EFC pulses are one-to-one correspondence. The amplitude of RPBs pulse in VHF band does not decrease obviously with time for logarithmic amplification. More RPBs belong to normal RPBs, which is similar to the findings of Rakov et al., 1996 and Kolmasová and Santolík (2013). Kolmasová and Santolík (2013) explained that the distances between adjacent charge pockets in thundercloud is nearly constant and they imaged a periodic charge structure which spatial scales is about order of 10 m. The speed of RPBs development decreases with time; therefore, the wave shape shows that the interval between two pulses increases with time. These authors pointed out that the amplitude of the pulses decreases with time, which could be explained reasonably based on the opinion of Uman and McLain (1970), who suggested that the radiated magnetic field is related to the develop speed of leader. However, we can see that the amplitude of pulses increases with time in VLF/LF band of radiation in the initial part of RPBs in Figure 2 in our work. The amplitude of pulses is stable in the VHF band and dissimilar to that in VLF/LF. Almost all normal RPBs have this characteristic in wave shape. In general, the wavelength of radiation in air gap discharge depends on the discharge gap and discharge velocity. We consider that the discharge is weaker in the initial stage than in the later stage; therefore, the discharge step size is shorter than that in the later stage. Because its radiation spectrum focuses on an even higher VHF band, the quantity of radiant energy in the VLF/LF band is less than that in the VHF band in the initial stage. Furthermore, we think the distribution of charge pockets is not regular absolute in detail; thus, small charge pockets that are uniformly distributed in the vicinity of a large charge pocket was named the charge group, although the distance between the neighboring charge groups in the thundercloud on the macro level is nearly constant, which is similar to the finding of Kolmasová and Santolík (2013). The small charge pockets uniformly distributed in the vicinity of large charge pockets may represent cessation sites of the leader step in the initial stage of RPBs for lower discharge energy. Therefore, the discharge gaps in the initial stage of RPBs are shorter than those in the later stage.
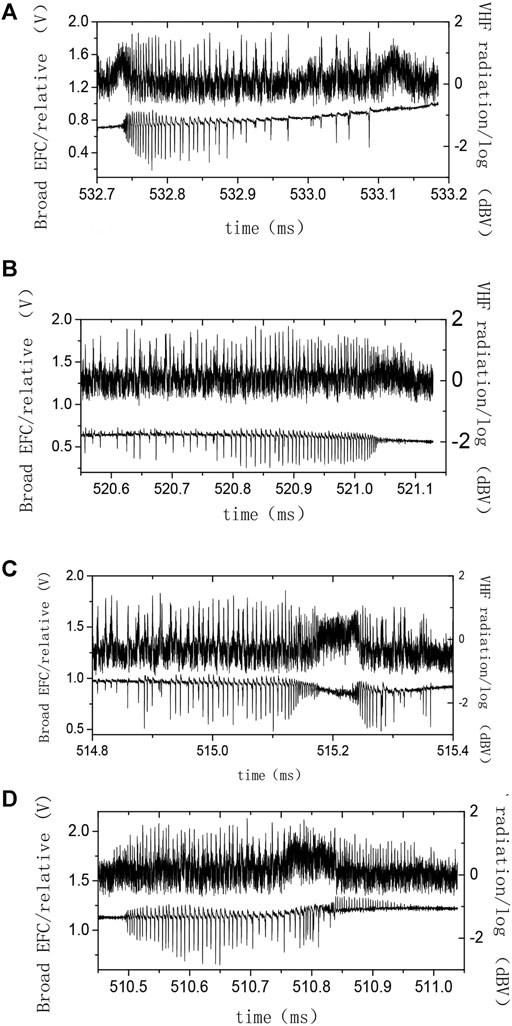
FIGURE 2. Categories of RPBs. Here (and same below), the upper part is the relative change in VHF radiation and the lower part is the relative change in the VLF/LF radiation in picture. (A) Normal RPBs, (B) back RPBs, (C) symmetric RPBs, and (D) reversal RPBs.
2.2 Back RPBs
The category of back RPBs represent a recoil version of the normal type in the time series (see Figure 2B). The pulses in the initial portion of RPBs are sparse, and the amplitude of pulses is lower in the beginning period. Over time, the time interval decreases gradually, the pulse amplitude increases, the pulse amplitude decreases and the interval between two pulses decreases so that the wave shape reaches a chaotic waveshape at all times. The VHF radiation is intense, and the VHF pulses and EFC pulses are one-to-one correspondence similar to normal RPBs. The proportion of this type of RPBs is less than that of normal RPBs in lightning discharges. Based on the hypothetical periodic charge structure of Kolmasová and Santolík (2013), the distances changed between adjacent charge pockets and increase of leader propagation speed could probably also explain the different sort of the trains of the pulses. We suggest that the speed increase of the leader propagation provides a better explanation of the discharge process of the back RPBs.
2.3 Symmetric RPBs
In this study, some RPBs developed symmetrically in the time series (see Figure 2C), and these pairs consisted of one normal RPBs and one back RPBs, with the latter followed by the former. The distances between neighboring pulses are large, and the amplitude of pulses is lower in the initial portion. Over time, the time interval decreases gradually, the pulse amplitude increases, the pulse amplitude decreases and the interval between neighboring pulses decreases and then changes to a chaotic periodic vibration wave shape. After a period of intensive and chaotic wave shape, the wave shape reached periodic vibration again and developed into pulses that burst gradually as the pulse amplitude increased. Then, the pulse amplitude decreased and the interval between two pulses increased with time. The VHF radiation also is intense, and the VHF pulses and EFC pulses are one-to-one correspondence, which is similar to normal RPBs. Ismail et al., 2017 suggested possible mechanisms it is that superposition of electric fields of two and more pulses trains which propagating simultaneously formed chaotic pulse trains, which could explain chaotic pulse trains of symmetric RPBs. However, the detailed evolution of pulse amplitude and time intervals cannot be explained reasonably by the opinion of Ismail et al., 2017. We consider that there is a neighboring large charge area at the discharge channel, with back RPBs occurring before this charge area and normal RPBs occurring after this charge area.
2.4 Reversal RPBs
In reversal RPBs, the pulse polarity of the RPBs inverts one or more times in the develop process (see Figure 2D). The reverse develop process is always associated with more intense VHF radiation. Some RPBs of this type could be explained by the mechanism suggested by Ismail et al., 2017, which is the superposition of electric fields caused by two RPBs with different polar pulses simultaneously. It is conceivable that the proportional wave shapes of RPBs belong to the type that is superposed by two RPBs with different or the same polar pulses. Of course, not all reverse RPBs present this mechanism, as suggested by Ismail et al., 2017, and we have obtained direct evidence proving this supposition (see ‘4. Three-dimensional map and characterization of RPBs’).
3 Statistical Data on the PRBs Under Various Lightning Discharges
A statistical analysis of all 430 RPBs over a period of 15 min indicates that the width of the pulses in RPBs is approximately 0.5–2.0 μs, with a typical width value of 1.0 μs; the interval of neighboring pulses of RPBs is approximately 2.0–10.0 μs, with a typical interval value of approximately 5.0 μs; and the duration of RPBs is approximately 20.0–2000.0 μs, with a typical duration value of several hundred μs. A total of 224 normal RPBs accounted for approximately 52% of all RPBs, 73 back RPBs accounted for approximately 17% of all RPBs, 89 symmetric RPBs accounted for approximately 21% of all RPBs, and 44 reverse RPBs accounted for approximately 10% of all RPBs.
Six typical lightning discharges, including two + CG, two -CG and two IC lightning discharges, are analyzed in detail in this work. Table 1 and Table 2 show comparisons between the statistical parameters presented by Rakov et al., 1996 and those from our observations. All parameters in our work are similar to the RPBs observed by Rakov et al., 1996. Moreover, the average duration and pulse number from our observation are slightly greater than those from Rakov et al., 1996. The number of RPBs was five in the-CG (00:02:28), three in the-CG (00:04:26), 10 in the + CG (00:03:27), six in the +CG (00:05:24), eight in the IC (00:01:57), and seven in the IC (00:02:49).
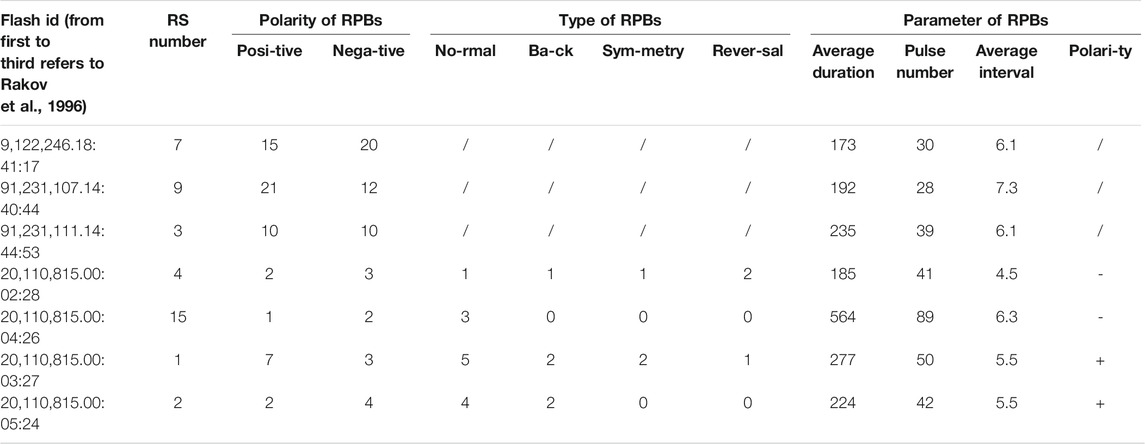
TABLE 1. Statistical comparison of data from CG. Rows one to three are statistical data given by Rokav et al., 1996; rows five to six are statistical data of–CG from Qinghai; and rows seven to eight are statistical data of +CG from Qinghai.

TABLE 2. Statistical comparison of data from IC. Rows one to three are statistical data from Rokav et al., 1996; and rows five to six are statistical data of IC from Qinghai.
In this study, we only considered regular RPBs with atypical characteristics. The sign ‘/’ indicates absence for this data. The average interval between adjacent pulses of RPBs in lightning discharges observed in Qinghai was slightly lower than that of Rakov et al., 1996. The number of normal-type RPBs shown in Table 1 is greater than that of the other RPBs, which indicates that most RPBs originate from the hypothetical periodic charge structure which spatial scales is about order of 10 m, as suggested by Kolmasová and Santolík (2013).
RPBs tend to occur at the end of discharge, where many K-changes often occur. More RPBs discharge always occurs in the latter part of a ramp-like field change characteristic of a K-changes. Many RPBs were found to be related with hook-shaped field change M-components (Rakov et al., 1996). In this work, the RPBs can be synchronously detected by broad EFC and VHF detectors. Krider et al., 1975 and Rakov et al., 1996 considered that maximum proportion of RPBs occur in middle or rear of lightning discharges. However, some weak RPBs occurred in the initial stage of lightning discharge in this work. These RPBs were due to “an intra-cloud dart-stepped leader process”, as suggested by Krider et al., 1975. Therefore, we think that many secondary breakdowns similar to dart leaders occurred for–CG in the initial stage of lightning discharges.
4 Three-Dimensional Map and Characterization of RPBs
The lightning mapping system (LMS) takes advantage of 1.2 s uninterrupted waveforms after the detector was triggered, so we can analyze every fragments of lightning discharge with high time resolution (time resolution is 50 ns). The power waveform was converted from a VLF/LF waveforms using the Hilbert transform (see Figure 3), which is similar to the work of Shao et al., 2005. Accordingly, we developed a algorithm which could automatically match pulses from seven stations based sevral intervals between pulses of RPBs (see Figure 3). This algorithm improved the efficiency of locating RPBs in three dimensions in a high time resolution although limited by different amplitudes of pulses in different stations. We initially located 11 RPBs in three dimensions and one regular pulse train associated with a dart-stepped leader.
Figure 4 shows a VHF map of a typical IC which including four process of RPBs. In addition, Figure 5 shows a three-deimensional picture of a typical RPBs in Figure 4. The analysis shows that the ranges of RPBs developing velocity is about from approximately 1.2 × 106 m/s to 3.0 × 106 m/s, which is slightly less than that of the dart-stepped leader process or dart leader (Biagi et al., 2010), and the lengths range of break step which marked by pulses is about from approximately several meters to dozens of meters. The developmental span of RPBs reaches 5 km in this work. The developed channel of RPBs always along the original channel which formed in earlier discharges, which indicates RPBs are re-breakdown processes in IC and similar to dart-stepped leader processes in CG. Many VHF three-dimensional pictures of RPBs show clusters in position of earlier formed channels, and their developing diameters are less than 1 km. Generally, the developmental scan of RPBs cannot reach the ends of old channels.
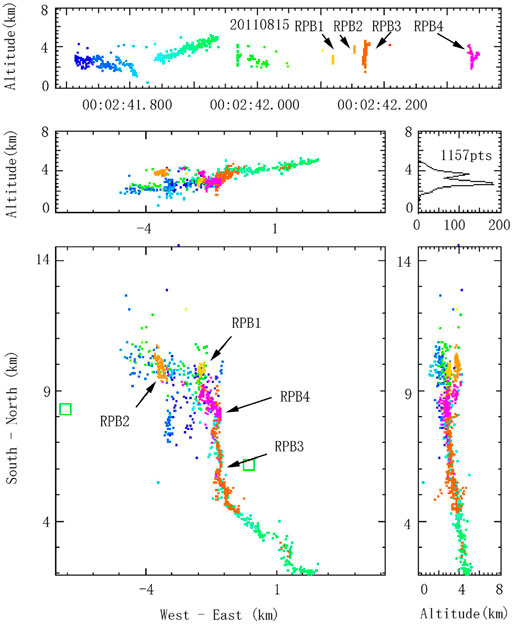
FIGURE 4. Typical intra-cloud lightning discharges includes four RPBs (In this work, the time-variant of radiation sources marked by different colors from blue, green, yellow to red. The green square denotes the LMS station. The height versus time plot (the units of time are hour:minute:second:microsecond) exhibited in top panel; the north-south vertical projection exhibited in mid-left panel; the height distribution of number of radiation events exhibited in mid-right panel shows; the plan view exhibited in bottom-left panel; and the east-west vertical projections exhibited in bottom-right panel).
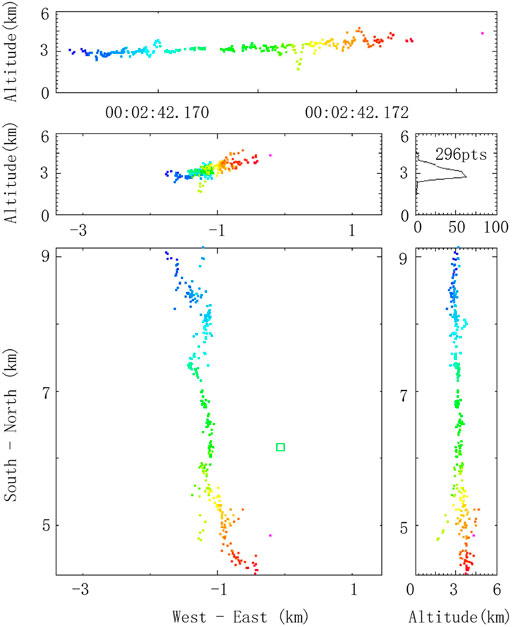
FIGURE 5. Third RPBs in Figure 4.
Multi-polarity inversions of these RPBs are shown in Figure 6. The enlarged wave shape of part of the RPBs indicates that the initial half of wave of pulse one is entirely positive. Then, the polarity starts to reverse in the second pulse, with a slightly negative change in the wave shape in the initial half of the wave. Then, the negative change wave shape increases with each pulse until the ninth pulse, which forms an obvious negative half wave pulse, and in the eleventh pulse, the amplitude of the negative half wave is greater than that of the positive half wave and polarity reversal is completed. This phenomenon could not be explained by Ismail et al., 2017. We think that the formation of reverse RPBs may be caused by bending of the lightning discharges channel. The entire RPBs process has multiple reversals and generally develops with snaking discharge channels from the three-dimensional picture in Figure 5, although the details are indistinguishable in detail. In addition, a dart-stepped leader was located in three-dimensional in this work (Figure 7). The developed velocity of the dart-stepped leader is approximately 1.2 × 106 m/s (Figure 7).
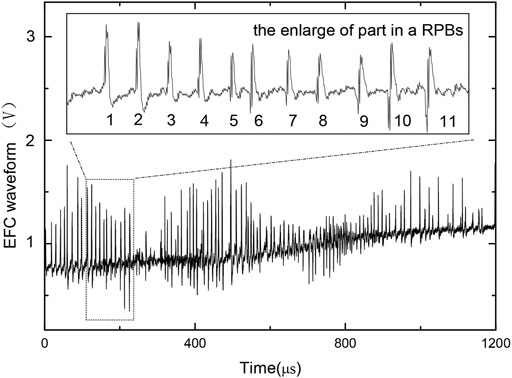
FIGURE 6. Part of the RPBs waveform related to the third RPBs is shown in Figure 4.
5 Summary and Discussion
In this work, we studied the waveforms of lightning discharges from about 15 min.In addition, some typical RPBs were loacted in three dimensions and analyzed in detail. Several conclusions are presented below.
1) The typical width of pulses in RPBs is approximately 1 μs, and the typical interval between pulses of RPBs is approximately 5 μs. According to the interpulse interval and pulse polarity, category of normal RPBs, back RPBs, symmetric RPBs, and reversal RPBs are sorted. Moreover, these four types were related to the different discharge processes and structures of the discharge channels.
2) A total of 83% of all lightning discharges include one or more RPB. RPBs are similar in both broad EFC and VHF radiation waveforms. Generally, RPBs are associated with K-changes, M processes or dart-stepped leaders. Maximum proportion of RPBs occur in middle or rear of lightning discharges. However, some RPBs was found to occur at the preliminary stage of lightning discharges.
3) The lasting time of RPBs is about from 20 to 2000 μs which larger than that in earlier literature (Krider et al., 1975; Rakov et al., 1996).
4) The RPBs developing velocity ranges from approximately 1.2 × 106 m/s to 3.0 × 106 m/s, which is slightly less than the dart-stepped leader process. The developmental span of RPBs could reach 5 km in this work.
Compared with the primary discharge channel of lightning discharges, such as the preliminary breakdown process and step leader, the secondary breakdown activity is more difficult to be caught by the instrument due to its short time duration and speed of development. Obviously, RPBs are a typical secondary discharge. The dart-stepped leader in CG is similar to this phenomenon. The development of high-speed acquisition technology has allowed detailed observations of the secondary discharge, which can even be mapped in three dimensions.
RPBs are typical waveshapes in the process of lightning discharges. Four types, i.e., normal RPBs, back RPBs, symmetric RPBs, and reversal RPBs, were sorted according to the interpulse interval and pulse polarity. More RPBs belong to normal RPBs. The classification in this paper can enable a more thorough analysis of the different RPBs. Kolmasová and Santolík (2013) investigated the evolution of the pulse amplitude and interval in normal RPBs and suggested that the observed time interval between the adjacent pulses increases with time based on a hypothesis that the equal distance between the neighboring charge pockets and speed of the movement of the RPBs decrease with time. The back RPBs represent a recoil version of the normal type in the time series, which could be explained by the suggestions of Kolmasová and Santolík (2013). In the observation data, we also found some interesting examples, such as symmetric RPBs and reversal RPBs. Symmetric RPBs present normal RPBs followed by back RPBs. A chaotic process occurs between normal RPBs and back RPBs. The superposition of electric fields of two and more pulses trains which propagating simultaneously formed chaotic pulse trains suggusted by (Ismail et al., 2017); however, this explanation cannot easily be applied to symmetric RPBs because these RPBs included a whole back RPBs followed by a whole normal RPBs in the waveform. Thus, the evolution of the amplitude of pulses and the interval between neighboring pulses cannot be well explained by Ismail et al., 2017. We consider that there is a neighboring large charge area at the discharge channel, with back RPBs observed before this charge area and normal RPBs observed after this charge area. For the reversal RPBs, the pulse polarity of RPBs inverts one time or more in their life cycle. (see Figure 2 4) and Figure 6). Some RPBs of this type could be explained by the mechanism suggested by Ismail et al., 2017, which is the simultaneous superposition of electric fields caused by two RPBs with different polar pulses. However, some cases, including chaotic waveshapes, could not be explained by Ismail et al., 2017. Inverse processes can be observed in the EFC waveform in Figure 6, and they are associated with a part of the RPBs channel in Figure 5. From the waveform, we can see that the inverse process is frequent and gradually changes in the enlarged waveform in Figure 6. We considered that some inverse pulse polarity in RPBs originates from bending of the discharge channel in RPBs.(Wang et al., 2014).
Data Availability Statement
The original contributions presented in the study are included in the article/Supplementary Material further inquiries can be directed to the corresponding author.
Author Contributions
Data acquisition, WY; methodology, FX and WT; software, MY and LY; validation, WT, MY, and LY; investigation, FX and WY; writing—original draft preparation, WY; writing—review and editing, WY, FX, and WT All authors have read and agreed to the published version of the manuscript.
Funding
This work was supported by the National Key R and D Program of China (2017YFC1501502), Second Tibetan Plateau Scientific Expedition and Research (STEP) program (2019QZKK0104), National Natural Science Foundation of China (41675006, 41875002), Open Research Program of the State Key Laboratory of Severe Weather (2019LASW-B11) and Startup Foundation for Introducing Talent of NUIST (2017r013#).
Conflict of Interest
The authors declare that the research was conducted in the absence of any commercial or financial relationships that could be construed as a potential conflict of interest.
Publisher’s Note
All claims expressed in this article are solely those of the authors and do not necessarily represent those of their affiliated organizations, or those of the publisher, the editors and the reviewers. Any product that may be evaluated in this article, or claim that may be made by its manufacturer, is not guaranteed or endorsed by the publisher.
Acknowledgments
The authors are indebted to all members of the Qinghai lightning observation experiment (especially professor Zhang Guangshu) and Qinghai Province. For further details, please contact WY (wangyanh@nuist.edu.cn).
References
Bailey, J., Willett, J., Krider, E., and Leteinturier, C. (1988), Submicrosecond Structure of the Radiation fields from Multiple Events in Lightning Flashes, Proceedings of the paper presented at International Conference on Atmospheric Electricity, 8 th, Uppsala, Sweden.
Beasley, W. H., Uman, M. A., Jordan, D. M., and Ganesh, C. (1983). Simultaneous Pulses in Light and Electric Field from Stepped Leaders Near Ground Level. J. Geophys. Res. 88 (C13), 8617–8619. doi:10.1029/jc088ic13p08617
Biagi, C. J., Uman, M. A., Hill, J. D., Jordan, D. M., Rakov, V. A., and Dwyer, J. (2010). Observations of Stepping Mechanisms in a Rocket-And-Wire Triggered Lightning Flash. J. Geophys. Res. Atmospheres 115 (D23). doi:10.1029/2010jd014616
Cooray, V., and Lundquist, S. (1985). Characteristics of the Radiation fields from Lightning in Sri Lanka in the Tropics. J. Geophys. Res. 90 (D4), 6099–6109. doi:10.1029/jd090id04p06099
Davis, S. (1999). Properties of Lightning Discharges from Multiple Station Wideband Measurements, Dissertation. Grad. Sch. Univ. Fla. UMI Microform 9945961.
Füllekrug Martin, F.-S. A. C. (2011). The Earth's Electromagnetic Environment. Geophys. Res. Lett. 38 (21). doi:10.1029/2011gl049572
Ismail, M. M., Rahman, M., Cooray, V., Fernando, M., Hettiarachchi, P., and Johari, D. (2017). On the Possible Origin of Chaotic Pulse Trains in Lightning Flashes. Atmosphere 8 (2), 29. doi:10.3390/atmos8020029
Jiang, R., Qie, X., Wang, Z., Zhang, H., Lu, G., Sun, Z., et al. (2015). Characteristics of Lightning Leader Propagation and Ground Attachment. J. Geophys. Res. Atmos. 120, 11988–12002. doi:10.1002/2015jd023519
Kolmasová, I., and Santolík, O. (2013). Properties of Unipolar Magnetic Field Pulse Trains Generated by Lightning Discharges. Geophys. Res. Lett. 40 (8), 1637–1641.
Krider, E. P., Radda, G. J., and Noggle, R. C. (1975). Regular Radiation Field Pulses Produced by Intracloud Lightning Discharges. J. Geophys. Res. 80 (27), 3801–3804. doi:10.1029/jc080i027p03801
Krider, E. P., Weidman, C. D., and Noggle, R. C. (1977). The Electric fields Produced by Lightning Stepped Leaders. J. Geophys. Res. 82 (6), 951–960. doi:10.1029/jc082i006p00951
Liu, H. Y., Dong, W. S., Zhang, Y. J., Zheng, D., Yan, B. W., Shi, Y. H., et al. (2013). Three-Dimensional Observation of Chaotic Pulse Train Event in Negative Cloud-To-Ground Lightning Using VHF Broadband Digital Interferometer. Plateau Meteorology 32 (4), 1186–1194.
Muller-Hillebrand, D. (1962), The Magnetic Field of the Lightning Discharge, in Gas Discharge and the Electricity Supply Industry, edited, pp. 89–111. London, England.
Ogawa, T., and Brook, M. (1964). The Mechanism of the Intracloud Lightning Discharge. J. Geophys. Res. 69 (24), 5141–5150. doi:10.1029/jz069i024p05141
Qie, X., Yang, Z., Zhang, Q., Jing, Y., Feng, G., Kong, X., et al. (2009). Characteristics of Triggered Lightning during Shandong Artificial Triggering Lightning experiment (SHATLE). Atmos. Res. 91 (2), 310–315. doi:10.1016/j.atmosres.2008.08.007
Rakov, V. A., Uman, M. A., Hoffman, G. R., and Brook, M. W. M. (1996). Burst of Pulses in Lightning Electromagnetic Radiation: Observations and Implications for Lightning Test Standards. IEEE Trans. Electromagn. Compat. 38 (2), 156–164. doi:10.1109/15.494618
Rakov, V. A., and Uman, M. A. (1990). Waveforms of First and Subsequent Leaders in Negative Lightning Flashes. J. Geophys. Res. 95 (D10), 16561–16577. doi:10.1029/jd095id10p16561
Rison, W., Thomas, R. J., Krehbiel, P. R., Hamlin, T., and Harlin, J. (1999). A GPS-Based Three-Dimensional Lightning Mapping System: Initial Observations in central New Mexico. Geophys. Res. Lett. 26 (23), 3573–3576. doi:10.1029/1999gl010856
Shao, X. M., Jacobson, A. R., and Fitzgerald, T. J. (2005). Radio Frequency Radiation Beam Pattern of Lightning Return Strokes: Inferred from FORTE Satellite Observations. J. Geophys. Res. Atmospheres 110 (D24). doi:10.1029/2005jd006010
Tran, M. D., and Rakov, V. A. (2017). A Study of the Ground-Attachment Process in Natural Lightning with Emphasis on its Breakthrough Phase. Sci. Rep. 7 (1), 15761. doi:10.1038/s41598-017-14842-7
Uman, M. A., and Mclain, D. K. (1970). Radiation Field and Current of the Lightning Stepped Leader. J. Geophys. Res. 75 (6), 1058–1066. doi:10.1029/jc075i006p01058
Wang, D., Gamerota, W. R., Uman, M. A., Takagi, N., Hill, J. D., Pilkey, J., et al. (2014). Lightning Attachment Processes of an “Anomalous” Triggered Lightning Discharge. J. Geophys. Res. Atmos. 119, 1524–1533. doi:10.1002/2013JD020787
Wang, H., Pei, Y., Yin, Y., Shen, L., Chen, K., Shi, Z., et al. (2021). Observational Evidence of Lightning‐Generated Ultrafine Aerosols. Geophys. Res. Lett. 48, e2021GL093771–14. doi:10.1029/2021gl093771
Wang, Y., Zhang, G., Tong, Z., Li, Y., Zhao, Y., Zhang, T., et al. (2010). The Regular Pulses Bursts in Electromagnetic Radiation from Lightning, Asia-Pacific International Symposium on Electromagnetic Compatibility, Beijing, China, December 4, 2010, IEEE.
Wang, Y., Zhang, G., Li, Y., Tong, Z., and Rong, Z. (2014). Regular Pulses Bursts Observation Using a Time of Arrival Lightning Mapping System with Dual Channels, Intemational Conference of Lighbling Protection, IEEE, 11 Oct. 2014, Shanghai, China
Weidman, C. D., and Krider, E. P. (1978). The Fine Structure of Lightning Return Stroke Wave Forms. J. Geophys. Res. 83 (C12), 6239–6247. doi:10.1029/jc083ic12p06239
Weidman, C. D. (1982). The Submicrosecond Structure of Lightning Radiation Fields. Thesis. Arizona, US: The univesity of Arizona.
Willett, J. C., Bailey, J. C., and Krider, E. P. (1989). A Class of Unusual Lightning Electric Field Waveforms with Very strong High-Frequency Radiation. J. Geophys. Res. 94 (D13), 16255–16267. doi:10.1029/jd094id13p16255
Keywords: regular pulse burst, lightning discharge, lightning location, dart-stepped leader, VHF radiation
Citation: Yanhui W, Xiangpeng F, Tuo W, Yingchang M, Yali L and Guo Z (2021) Characteristics of Regular Pulse Bursts Generated From Lightning Discharges. Front. Environ. Sci. 9:799115. doi: 10.3389/fenvs.2021.799115
Received: 21 October 2021; Accepted: 11 November 2021;
Published: 06 December 2021.
Edited by:
Lijuan Shen, University of Toronto, CanadaReviewed by:
Xiufeng Guo, Wuxi University, ChinaPengguo Zhao, Chengdu University of Information Technology, China
Kun Liu, Chengdu University of Information Technology, China
Caixia Wang, Beijing Information Science and Technology University, China
Copyright © 2021 Yanhui, Xiangpeng, Tuo, Yingchang, Yali and Guo. This is an open-access article distributed under the terms of the Creative Commons Attribution License (CC BY). The use, distribution or reproduction in other forums is permitted, provided the original author(s) and the copyright owner(s) are credited and that the original publication in this journal is cited, in accordance with accepted academic practice. No use, distribution or reproduction is permitted which does not comply with these terms.
*Correspondence: Wang Yanhui, wangyanh@nuist.edu.cn
†Present address: Wang Yanhui,Key Laboratory for Aerosol-Cloud-Precipition of China Meteorological Administration, Collaborative Innovation Center on Forecast and Evaluation of Meteorological Disaster, Nanjing University of Information Science and Technology, Nanjing, China