- 1Institute of Marine Sciences, Shantou University, Shantou, China
- 2Southern Marine Science and Engineering Guangdong Laboratory, Guangzhou, China
Per- and polyfluoroalkyl substances (PFASs) have captured worldwide attention due to their persistent and bioaccumulative characteristics. The bullfrog is a highly nutritious and edible aquatic product, but the PFAS accumulation characteristics of bullfrogs had not been paid attention. The present study analyzed the PFAS concentrations in different tissues of cultured bullfrogs and relevant water and sediments collected from the aquaculture region in the coastal area of South China. Results showed that total PFAS concentrations were 50.26–364.25 ng/L in water, with a mean of 135.58 ng/L; concentrations in effluent water were significantly higher than those in influent water, which means bullfrog farming could increase contaminants into the surroundings. The total PFAS concentrations varied from 2.89 to 162.26 ng/g·dw in sediment. The distribution profile of PFASs in sediment was similar to that of water, with short chain playing a significant role. Total PFASs in bullfrog tissues were 3.36–84.07 ng/g·dw, with the highest values in the digestive system (intestines and stomach). As for one novel PFAS alternative, chlorinated polyfluorinated ether sulfonate (F-53B) was found at high detectable levels in all habitat samples and had a higher value of logarithmic bioaccumulation factors. The estimated dietary intake (EDI) for residents in the study area was generally higher than that in other regions; however, the hazard ratios (HRs) for most detected PFASs were far below the safety threshold value “1.” These results suggest that frequent consumption of these bullfrogs would basically not cause severe health effects on local residents in terms of PFASs.
1 Introduction
Perfluoroalkyl and polyfluoroalkyl substances (PFASs) are recognized as emerging contaminants capturing worldwide attention (Pan et al., 2018; Long et al., 2021; Troger et al., 2021). They are primarily transported in the environment and spread around the globe due to their persistent and bioaccumulative characteristics (Conder et al., 2008; Simon et al., 2019). PFASs have been found in the air, water, and soil around the world and have been detected even in polar regions (MacInnis et al., 2019; Lin et al., 2020; Xie et al., 2020). Perfluorooctane sulfonate (PFOS), perfluorooctanoate carboxylate (PFOA), and perfluorohexanesulfonic acid (PFHxS), as well as their salts, are listed as persistent organic pollutants (POPs) under the Stockholm Convention (UNEP, 2009; Stockholm Convention, 2017). However, they are still used in the manufacture of liquid crystal displays, semi-conductors, pulp, paper, fabric, and clothing, and have been widely utilized in electrochemical fluorination and telomerization in China (Buck et al., 2011).
It means that these traditional PFASs can only be used for specific exemptions and acceptable purposes, and this trend has led to a shift to the production and usage of fluorinated replacements of short-chain PFASs and other PFAS alternatives, including perfluorobutanesulfonic acid (PFBS) and chlorinated polyfluorinated ether sulfonic acids (Cl-PFESAs). PFASs and their precursors, as anthropogenic organofluorine chemicals, have been manufactured for more than 50 years (Wang et al., 2013; Shi et al., 2017; Ankley et al., 2021). Sustained PFASs will increasingly contribute to global emissions of legacy and novel perfluorinated compounds in the environment. It was reported that 20–6,420 tons of emissions would be emitted from 2016 to 2030 (Wang et al., 2014). The alternative perfluorochemicals have been detected ubiquitously in the environment matrix recently, and even higher than traditional PFASs, especially in areas with high-intensive anthropogenic activities (Yamazaki et al., 2019; Brase et al., 2021; Sun et al., 2021).
Many studies focused on potential toxic effects and bioaccumulation of PFASs on humans (Liu et al., 2011a; Zhao Xiang et al., 2011; Hong et al., 2015; Skogheim et al., 2020). Dietary intake is considered as one of the major routes for human exposure, including drinking water directly and consumption indirectly in aquaculture food (Dassuncao et al., 2018; Lu et al., 2018; Zhou et al., 2021; Zhou et al., 2019). Previous studies have shown that consumption of fish is a significant contributor to human intake of PFASs due to their presence in the aquatic environment and their accumulation (Zhang et al., 2019; Fauconier et al., 2020). However, very few studies had investigated PFAS contamination in bullfrog (Rana catesbiana) and relevant risk towards human health. Bullfrogs play a crucial part ecologically, utilizing both aquatic and terrestrial environments, providing an important prey base in each, and are also commonly used as indicator species for ecosystem health (Smalling et al., 2015). Bullfrog activities can stir and affect the transportation of contamination from sediment to water and then further affects ecological health. Meanwhile, bullfrog also bioaccumulates PFAS contamination from biota and bait. Ingestion is a vital pathway for PFASs to transmit to humans especially through aquatic products such as bullfrog. It is worth noting that the bullfrog is a popular, delicious, and nutritious aquatic product not only in China but also worldwide. Therefore, understanding the occurrence, bioaccumulation of PFAS contamination in bullfrog farming, and potential risk towards human health is critical, especially in rapidly developing South China.
To bridge this knowledge gap, we investigated the concentrations of legacy and emerging PFAS in water, sediments, baits, and bullfrogs during different growth periods from typical bullfrog bases in South China to determine critical contaminants and relevant ecological risk and health effects. The specific objectives of the present study were (1) to measure and compare legacy and emerging PFASs in multi-matrixes including water, sediments, baits, and bullfrogs from the bullfrog aquaculture region in the coastal area of South China; (2) to investigate bioaccumulation of target compounds in various organs during different growth periods of bullfrog; and (3) to assess the potential risks of legacy and emerging PFASs to local residents via aquatic product consumption. Conclusions were proposed that the current study can help better understand food safety, provide fundamental information for risk assessments, and assist local governments to control strategies of legacy and emerging poly- and perfluoroalkyl substances.
2 Materials and Methods
2.1 Sample Collection
All samples including water, sediments, baits, and bullfrogs in different growth periods were collected from typical bullfrog bases around the coast of the South China in June 2021(Figure 1). Water samples were stored in 1-L polypropylene (PP) containers with a narrow mouth and a screw cap, which were collected from downstream and upstream of the water supply river, effluent and influent of the culture ponds in bullfrog base. Corresponding sediment samples were collected with a bottom grab and stored in PP containers from the corresponding locations of water. To reduce individual differences, all mixed biological samples were obtained for tadpoles, juvenile bullfrogs, young bullfrogs, and adult bullfrogs, which were stored in sealed bags and frozen at −20°C in a refrigerator until treatment. All containers and tools were cleaned before use by rinsing sequentially with methanol and Milli-Q water from the sampling site.
2.2 Materials and Reagents
All mass-labeled internal standards and native standards were purchased from Wellington Laboratories (Guelph, ON, Canada), including PFBS, PFHxS, PFOS, perfluorodecane sulfonate (PFDS), PFBA, perfluoropentanoic acid (PFPeA), PFHxA, perfluoroheptanoic acid (PFHpA), PFOA, perfluorononanoic acid (PFNA), perfluorodecanoic acid (PFDA), perfluoroundecanoic acid (PFUnDA), perfluorododecanoic acid (PFDoDA), perfluorotridecanoic acid (PFTrDA), perfluorotetradecanoic acid (PFTeDA), perfluorohexadecanoic acid (PFHxDA), and perfluorooctadecanoic acid (PFODA). Four perfluorinated sulfonic acids (PFSAs), 13 perfluorinated carboxylic acids (PFCAs), and chlorinated polyfluorinated ether sulfonate (9Cl-PFONS; F-53B) were measured for all of the samples. 13C4PFBA, 13C4 PFHxA, 13C4 PFOA, 13C4 PFNA, 13C4 PFDA, 13C4 PFUdA, 13C2 PFDoA, 18O2 PFHxS, and 13C4 PFOS were used as internal standards with purity >98%. Methanol (HPLC grade) and acetonitrile were purchased from J.T. Baker (Phillipsburg, NJ, United States). Detailed information is given in Supplementary Table S1.
2.3 Sample Treatment
2.3.1 Water Samples
PFASs were analyzed in this study following previous methods (Wang et al., 2016a; Zhou et al., 2018; Meng et al., 2019a; Du et al., 2021). Water samples were filtered using glass fiber filters (GFF, Whatman, O.D. 47 mm, 0.7 μm); then, 800 ml of water with 5 ng of mass-labeled standards was extracted by solid-phase extraction (SPE) processes with OASIS WAX cartridges. Firstly, the Oasis WAX cartridges were conditioned with 4 ml of 0.1% NH4OH in methanol, 4 ml of methanol, and 4 ml of Milli-Q water. After loading the sample at a rate of one drop/s, the cartridges were washed with 4 ml of 25 mM ammonium acetate and eluted with 4 ml of methanol and 4 ml of 0.1% NH4OH in methanol. Eluents were collected and then concentrated to 0.5 ml under a gentle stream of high-purity nitrogen. Finally, they were filtered through a nylon filter into a 1.5-ml PP snap top auto-sampler via polyethylene (PE) septa. Details of the extraction processes are given in the Supplementary Information.
2.3.2 Sediment Samples
Sediment samples were freeze-dried, homogenized with mortar and pestle, and extracted by alkaline digestion and ultrasonic extraction methods (Hong et al., 2015; Meng et al., 2018; Gao et al., 2020; Sun et al., 2021). Samples were sonicated in 10 ml of methanol and shaking (250 rpm) and centrifugation, and then the supernatant was concentrated to 1 ml under nitrogen. The supernatant was transported into a 50-ml PP tube and evaporated with a nitrogen stream and subjected to clean up with the SPE method. Eluents were concentrated up to 0.5 ml under a constant and stable high-purity nitrogen airflow and then passed through a nylon filter before being transferred into a 1.5-ml PP snap-top brown glass vial via a sterile syringe. Details of the extraction processes are given in the Supplementary Information.
2.3.3 Biological Samples
All bullfrogs were dissected and divided into heart, skin, liver, muscle, stomach, and intestines. All samples were frozen at −80°C, air-dried, and homogenized in order. One milliliter of 0.5 M tetrabutylammonium hydrogensulfate (TBAHS) solution and 2 ml of 0.25 M sodium carbonate buffer (pH 10) were added into a 50-ml PP centrifuge tube for extraction (Meng et al., 2019b; Zhang et al., 2019). After mixing, add 5 ml of methyl-tert-butyl ether (MTBE) and shake the mixture at 250 rpm for 15 min. Organic and aqueous layers were separated by centrifugation at 3,000 rpm for 10 min. Then 5 ml of MTBE was taken and transferred to a new 50-ml PP tube, and the extraction was repeated twice as above. SPE procedures were the same as those for the sediment samples (Loi et al., 2011; Zhou et al., 2014). Details of the extraction processes are given in the Supplementary Information.
2.4 Instrumental Analysis
PFASs analysis was performed using a Thermo Ultimate 3000 Infinity HPLC System equipped with a Thermo TSQ ENDURA LC/MS System, which was operated with electrospray ionization (ESI) in negative ion mode and with multiple reaction monitoring (MRM) of the target analytes. The column used was Agilent ZORBAX Eclipse Plus C18, 2.1 × 100 mm, 3.5 μm (Agilent) connected with a guard column (Agilent). The gradient elution of the mobile phase was 2 mM ammonium acetate (A) and 100% acetonitrile (B). The flow rate was 0.3 ml/min and a 5-μl aliquot of the extracts was injected into the column. Chemical formula, parent ion, quantitative ion, and qualitative ion of PFASs used in study are listed in Supplementary Table S1. Detailed descriptions of instrument conditions are shown in Supplementary Table S2.
2.5 Quality Assurance and Control
To strictly control background pollution, polytetrafluoroethylene or other fluoropolymer materials were prevented from preliminary treatment and analytical processes, and all containers in the laboratory were rinsed with methanol and Milli-Q water before use. There were analyzed in solvent blanks, process blanks, and duplicate samples to make the data reliable. Matrix spikes were based on an internal standard method, which was applied to quantify PFASs shown in Supplementary Table S3. The calibration curve consisted of a series of concentration gradients (0.1, 0.2, 0.5, 1, 2, 5, 10, 20, 50, 100, 150, and 200 μg/L), ensuring that the deviation between the determined value of each point and its theoretical value did not exceed 20%. The limit of detection (LOD) was determined using a signal-to-noise ratio of 3:1 and the limit of quantification (LOQ) was identified as the analyte peak that yielded a signal-to-noise ratio of 10:1. In the statistical analysis, all values lower than LOD were specified as LOD/√2, and in the case of concentrations lower than LOQ, the value of LOQ/√2 was used. The results of LOD and LOQ are shown in Supplementary Table S3.
2.6 Daily Intake Calculation and Data Analysis
The distribution coefficient (Kd) is used to analyze the ability of chemical substances adsorbed by the solid phase (sediment) from the liquid phase (water) (Zhou et al., 2013). The field-based Kd was calculated as follows:
where Csediment is the concentration of PFASs in sediment (ng/g dw), Cwater is the concentration of PFASs in water (ng/L), and Kd is in L/g.
Bioaccumulation factors (BAFs) were used to investigate the accumulation of PFASs in bullfrogs, which represents chemical uptake from water. BAF is defined as the ratio of the concentrations of PFASs in biota and concentrations in water (Chevreuil, 2010; Wilkinson et al., 2018; Burkhard, 2021). The field-based BAF was calculated as follows:
The intake of PFASs from bullfrog was identified as important exposure for human. Therefore, the estimated dietary intake (EDI) of PFASs for local residents was calculated in order to evaluate the human exposure.
where C is the concentration of PFASs in bullfrog (ng/g dw), CR is the consumption rate of different pathways (g/day), and BW is the body weight (kg).
The health risks of PFASs for local residents were calculated by the hazard ratios (HR) using the following equation:
where RfD is the reference dose (RfD, ng/kg/day), defined as an acceptable daily exposure without apparent toxicity. RfDs for PFHxA, PFOS, PFOA, PFBS, and PFBA were 320, 2, 3, 430, and 2,900 ng/kg/day, respectively (ANSES, 2017; MDH, 2017). HR higher than 1.0 means a potential risk towards human; however, value under 1.0 means low risk.
3 Results and Discussion
3.1 Per- and Polyfluoroalkyl Substances in Bullfrog Habitat From Aquaculture Base
3.1.1 Concentration and Composition of Per- and Polyfluoroalkyl Substances in Water
The compositions and concentrations of target PFASs were detected in effluent and influent water of the culture ponds, upstream and downstream of the water supply river around the bullfrog aquaculture base (Figure 2). The concentrations of PFASs in water samples ranged from 50.26 to 364.25 ng/L with a mean of 135.58 ng/L. PFASs have four classifications in terms of carbon chain length and functional group of the molecular structure, i.e., short-chain PFCAs (C4–C7), long-chain PFCAs (>C8), short-chain PFSAs (C4), and long-chain PFSAs (C8). The concentration of PFASs decreased in the following order: short-chain PFCAs > long-chain PFCAs > short-chain PFSAs> long-chain PFSAs. Short-chain polyfluoroalkyl substances were found in high concentration among most water samples, reaching 97%, while long-chain polyfluoroalkyl substances only accounted for 3%. The short-chain PFASs played a crucial part in water samples, in contrast to the different conclusions from previous reports several years ago (Kelly, 2009; Ahrens et al., 2010; Naile et al., 2010; Schultes et al., 2020). It was reported that short-chain PFASs had a high detection level in recent years, which was consistent with these studies (Xu et al., 2014; Wang et al., 2016a; Li et al., 2020a). As noted above, those could mean that perfluorinated-related industries in China began to take action for the gradual novel substitution effect.
In addition, the concentration of PFCAs was obviously higher than that of PFSAs, which can be explained by the fact that PFCAs could easily transport to a water environment because they are relatively highly water soluble. PFBA had the highest concentrations (37.41–201.53 ng/L) among the tested PFCAs, followed by PFOA (ND to 4.27 ng/L). For PFSAs, PFBS had maximum concentrations ranging from ND to 4.70 ng/L with a mean value of 1.98 ng/L. Among those PFSAs with traditional long carbon chains, mean PFOS concentrations were detected at 0.48 ng/L. Interestingly, F-53B, as novel polyfluoroalkyl substances replaced PFOS, was detected in all water samples, whose concentration ranged from 0.01 to 0.05 ng/L.
Compared to different sampling sites, it can be seen that total concentrations of PFASs in effluent water of the culture ponds were significantly higher in influent water of the culture ponds from bullfrog base (Table 1). Concentrations of PFBA ranged from 93.44 to 201.53 ng/L with a mean of 141.90 ng/L in effluent water at Deff, Feff, and Heff sites; corresponding values were between 51.42 and 79.27 ng/L with a mean of 61.31 ng/L in influent water at Cinf, Einf, and Ginf sites. There were similar trends that PFPeA had a high concentration level in effluent water, with PFPeA concentrations of 232.52, 167.16, and 118.60 ng/L, respectively, but these were not found in influent water. We should pay more attention to pollutants from the bullfrog aquaculture base. The mean concentration of PFASs was slightly higher in upstream (63.58 ng/L) than in downstream (60.79 ng/L), potentially caused by river dilution. A number of studies have noted that the total concentration of PFASs in river are higher than those in sea because estuaries could reduce the transfer of PFASs to the sea via the action that PFASs sink into sediments and thus preventing direct entry into marine environments (Cundy and Croudace, 2017; Celis-Hernandez et al., 2021). The concentration of PFASs in study river was at a lower level than that in the Daling River Basin and Maozhou River Basin (Wang et al., 2016a; Li et al., 2020a).
3.1.2 Concentration and Composition of Per- and Polyfluoroalkyl Substances in Sediment
The sediment samples were tested at the same sites as the water (Figure 2). The total concentration of the PFASs varied from 2.89 to 162.26 ng/g·dw. The distribution profile of the PFASs in sediment was similar to that of water in that short-chain PFASs played a significant role in samples. Among the tested compounds, the detection rate of the PFASs with shorter chains, i.e., PFBA, PFPeA, and PFBS, reached 100%, 82%, and 64%. Thereinto, PFBA was dominant in sediment samples, whose concentration ranged from 1.17 to 124.77 ng/g·dw with a mean concentration of 35.28 ng/g·dw. Note that the detection rate of PFOS (a traditional contaminant of international concern) was only 82%; however, F-53B was detected in all samples (100%).
The total concentration of PFASs was 16.47–71.94 ng/g·dw in sediment in the influent site and 4.42–30.81 ng/g·dw in the effluent site. Contrary to the trend in water samples shown in Table 1, total concentrations of PFASs in sediment samples of effluent sites were significantly lower in influent water due to the fact that bullfrogs stir contaminants from sediment to water and accumulate those into the body. There are some granular sands in sediment instead of slushes that absorb less contaminants in sediment and migrate PFASs to water phase. The concentration of PFASs was 50.03–162.26 ng/g·dw with a mean of 131.44 ng/g·dw in downstream of the water supply river. Comparison with literature showed that the observed PFAS concentration in downstream was much higher than the ΣPFASs of the Songhua River (0.143–1.41 ng/g·dw), the Baiyangdian Lake (0.48–30 ng/g·dw), the southern Beaulieu River (0.2–5.3 ng/g·dw), the Danjiangkou Reservoir (0.07–1.62 ng/g·dw), and the Taihu Lake (1.11–8.21 ng/g·dw) (Guo et al., 2015; Guo et al., 2020; Li et al., 2020b; Celis-Hernandez et al., 2021). Besides, the sediment concentration of PFBA was at a high level compared with the situations of other parts of the world. The pollutant could increase through bullfrog farming, which should be taken seriously and source control measures should be taken to reduce further contamination.
The distribution coefficient was studied for the ability of chemical substances adsorbed by sediment from water, which impacts their environmental behavior and fate (Supplementary Table S4). The results in Supplementary Figure S1 show that Kd values of long-chain compounds were higher than those of short-chain compounds, in agreement with previous findings (Ahrens et al., 2010; Li et al., 2011; Wang et al., 2019); the difference in Kd values shows the change in detection concentration and frequencies between water and sediment for short-chain and long-chain PFASs. In this study, the long-chain PFASs were not the dominant structure in water and sediment, which is not in accordance with other references (Kwadijk et al., 2010; Labadie Chevreuil, 2011; Wang et al., 2017). As stated earlier, perfluorinated industries in China began to take action for the gradual short-chain substitution effect and industry-discharged short-chain contaminants into the environment such that concentrations were increasing sharply in the surroundings.
3.2 Concentration and Composition of Per- and Polyfluoroalkyl Substances in Bullfrogs
Distribution and concentration of PFASs in bullfrog samples during different growth periods were detected from the typical bullfrog bases around the coasts in South China (Figure 3). Total PFASs in all bullfrog tissues were 3.36–84.07 ng/g·dw; PFBA, PFOS, PFOA, PFUdA, PFTrDA, and PFDoA are the predominant PFASs detected in the biota samples. F-53B, which was found at detectable levels in all habitat samples from the aquaculture base, also had a high detection level, reaching 95% with an average concentration of 0.36 ng/g·dw in bullfrog tissues. PFBA, as a short-chain PFAS, was found in all tissues with a concentration of 2.47–48.98 ng/g·dw. Meanwhile, PFBA among all PFASs had the highest concentration in three different particles of baits, which explains why detection level and concentration were high in effluent water at Deff, Feff, and Heff sites. Baits were manufactured by a compressor with a lot of lubricants, possibly containing numerous PFASs, and then biota could ingest contaminated baits or bioaccumulate PFASs via polluted habitats from putting into contaminated baits and industrial pollution (Supplementary Table S5).
PFAS level differences were observed in frogs during different growth periods: adult bullfrog, juvenile bullfrog, young bullfrog, and tadpole. It was evident that sum concentrations of PFASs in adult bullfrog were markedly higher than those in juvenile bullfrog, which could be due to the fact that PFASs had a long-half-life and persistent and bioaccumulative characteristics. Concentrations of PFASs were 184.45 ng/g·dw in the sum of all tested tadpole tissues and 142.65 ng/g·dw in juvenile bullfrog tissues. This result implied the higher total PFAS concentrations observed in tadpoles, which may be responsible for the varying bait between tadpole and juvenile bullfrog. Tadpoles ingested the smaller grain contaminated baits (PFASs: 95.74 ng/g·dw), and juvenile bullfrog ingested the baits of medium grain size (PFASs: 24.77 ng/g·dw). Interestingly, it can be seen that intestines had higher PFAS levels in tadpole than that in juvenile bullfrog on account of undigested contaminated bait in intestines. The concentration of PFOA in tadpole and juvenile bullfrog tissues was 0.01–26.59 ng/g·dw and ND-4.11 ng/g·dw, respectively. As short-chain PFASs, PFHpA (mean concentration: 25.73 ng/g·dw) and PFBA (mean concentration: 6.91 ng/g·dw) had a high detection ratio (100%) and concentration in all tadpole tissues. The current research is not in accordance with previous studies in that PFHxA was the predominant contaminant (80.50 ± 58.31 ng/g and 19.17 ± 12.57 ng/g ww), followed by PFOS (55.02 ± 34.82 and 14.79 ± 6.24 ng/g ww) in both fish from Lake Chaohu, China (Wu et al., 2019).
The distribution in different tissues after uptake largely determines the fate of a chemical and is of great importance for understanding the underlying mechanisms of bioaccumulation. Concentrations of PFASs in samples varied greatly among the different tissues, with the greatest concentrations in the digestive system (intestines and stomach) no matter what stage of growth the bullfrog is in. The concentrations of PFASs in the digestive system (intestines and stomach) were 52%, 45%, 65%, and 52% in adult bullfrog, young bullfrog, juvenile bullfrog, and tadpole, respectively, closely associated with ingesting contaminated bait. It was consistent with other studies in that the highest level in the digestive system among the different tissues was observed in carp and green eel goby, suggesting that PFASs can accumulate by diet from water, sediment, and contaminated bait intake (Hong et al., 2015; Wu et al., 2019). Moreover, liver, as a vital organ for fat metabolism and protein synthesis, also had a higher concentration level of PFASs than muscle. The possible reason for this is that liver could affect the binding of lipid and protein, and the protein content was generally at a higher level in liver while it was at a lower level in muscle (Chevreuil, 2010; Wen et al., 2019; Wu et al., 2019).
Despite the difference in levels, PFASs were found to have the same trend among nearly all muscles of bullfrogs from different growth periods, with PFBA being the most predominant compound in terms of concentration and frequency of detection. The proportion of F-53B in biota samples changed among the different tissues, with the greatest concentrations in liver (0.19–0.74 ng/g·dw). It is worth noting that F-53B was also analyzed from different areas in China, supporting previous findings that this contaminant is widely distributed in the bullfrog tissues from Changshu, Huantai, Zhoushan, and Quzhou (Cui et al., 2018), and consumption of bullfrog may expose humans to PFASs. The scanned diversification among the tissues should be put down to the diverse binding affinity of PFASs for tissue-specific proteins.
3.3 Adverse Effects of Per- and Polyfluoroalkyl Substances Towards Bullfrog Induced by Water
Field-based bioaccumulation factors of PFASs in various bullfrog organizations were calculated based on concentrations in water and biota tissues shown in Figure 4. What is noteworthy is that log BAFs of PFTeDA, PFDoA, and PFUdA were obviously higher than those of shorter-chain PFASs including PFBA and PFPeA. The results of this study were generally in agreement with previous results in that the short-chain PFASs are not considered bioaccumulative, but long-chain PFASs with >10 fluorinated carbons were bioaccumulative, potentially due to their large molecular size (Liu et al., 2011b; Hong et al., 2015). This study indicated that the log BAF value was at a higher level in PFOS but at a lower level in PFOA among adult, juvenile, and young bullfrog samples. Analogously, the log BAF value of PFBS was distinctly greater than that of PFBA in the present study, to some extent, which explained that PFSAs could more easily accumulate than PFCAs for similar substances of the same fluorinated carbon chain length.
F-53B, which was supported by international organizations for the production and substitution of PFOS, had a higher mean value of log BAF (with a mean of 0.44) than PFOS (with a mean of 0.31). Interestingly, the half-life of F-53B in humans is longer than that of PFOS in other studies (Loi et al., 2013). Hence, the government and relevant departments should consider F-53B instead of PFOS. Comparing the different ages of bullfrogs, the values of log BAF for long-chain PFCAs (C > 9) were higher in adult bullfrogs than in young bullfrogs; meanwhile, PFSAs, i.e., PFOS, PFBS and F-53B, had a similar trend in that the values of log BAF were higher in adult bullfrogs than in young bullfrogs. The results in this research suggested that long-chain PFCAs and PFSAs had great bioaccumulation propensity and explained why PFSAs and long-chain PFASs had higher concentration levels in adult bullfrogs than in young bullfrogs. The correlation of BAFs between long-chain and short-chain PFASs showed a helpful observation for comprehending the relative significance of diverse bioaccumulation mechanisms.
The values of log BAF in PFASs were 0.38 and 0.13 in juvenile bullfrogs and tadpoles, showing that the log BAFs of individual PFASs varied during different periods of bullfrogs on account of their complex life history following metamorphosis. The mean log BAF of PFOS in liver was 1.18 in adult bullfrogs, which is higher signally than that in muscle, and this was consistent with the trend observed in other studies. In PFOS, the mean log BAFliver in nine fish species was in the range of 3.5–4.6 from rivers in the Pearl River Delta region, around one log unit higher than in muscle (Pan et al., 2014). The log BAFliver of PFOS in this research is at a lower level than that found in European chub with log BAFliver of 4.3 and in rainbow trout with a log BAFliver of 3.7 (Martin et al., 2003; Labadie and Chevreuil, 2011; Wang et al., 2016b; Liu et al., 2018). This could be explained by the PFAS concentration variability in water, discharge capacity of the surrounding industry, different nutritional habits, and physical and chemical properties of different compounds.
3.4 Assessment of Human Health Risks via Bullfrog Consumption
Food dietary intake is the crucial pathway of human exposure to PFASs (Perez et al., 2014). The human exposure risk assessment was performed by calculating the EDI based on the daily consumption of bullfrog and the concentrations of PFASs shown in Figure 5. As body weights and consumption rates vary with age, the EDI of PFASs for five age groups (2–6, 7–12, 13–17, 18–45, and 46–69 years old) was estimated from China (CN), Guangdong province (GD), and Study area (SA), in which the daily consumptions of bullfrog were calculated to be 0.21, 1.16 and 1.89 g/day, respectively (Yan et al., 2015; Huang, et al., 2019; Su et al., 2019).
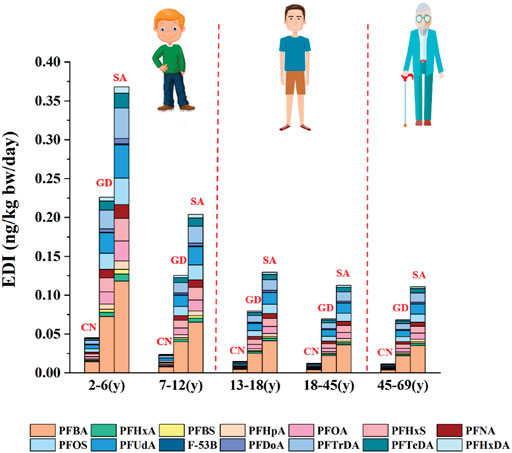
FIGURE 5. Estimated daily intake of PFASs (ng/kg bw/day) via bullfrog for residents of different ages.
The EDIs of PFASs via consumption of bullfrog for local residents of CN, GD, and SA are illustrated in Supplementary Tables S6–S8. In general, the total EDI of PFASs ranged from 1.16 × 10−2 to 4.53 × 10−2 ng/kg bw/day for residents in China, 6.84 × 10−2 to 2.26 × 10−1 ng/kg bw/day for residents in Guangdong province, and 1.11 × 10−1 to 3.68 ×10−1 ng/kg bw/day for residents in the Study area. The EDI decreased with age [(2–6) > (7–12) > (13–18) > (18–45) > (45–69)], among which the EDI of residents aged 2–6 years was 3.3 times than that of residents aged 45–69 years, indicating that ingestion of PFASs via bullfrog induced more severe hazards on residents aged 2–6 years than on residents aged 45–69 years. The result was consistent with estimations in most studies (Meng et al., 2019a). It may be relevant that residents aged 45–69 years weighed significantly more than residents aged 2–7 years. The bullfrog breeding scale in Guangdong province is the largest in CN, which accounts for about 40%. The research area is a high-density bullfrog culture area in GD, and the local residents like to consume bullfrog, which leads to the EDI and the intake of bullfrog being the highest among the data.
PFBA, PFUdA, PFTrDA, PFOS, PFOA, PFBS, and F53-B accounted for about 72% of total EDI of the 14 PFASs in CN, GD, and SA. PFBA contributed most to the EDI because of its highest concentration level, followed by PFUdA, PFTrDA, PFOS, PFOA, PFBS, and F53-B. The EDICN, EDIGD, and EDISA of PFBA were 3.70 × 10−3–1.45 × 10−2, 2.19 × 10−2–7.24 × 10−2, and 3.55 × 10−2–1.18 × 10−1 ng/kg bw/day, respectively. The EDI2-6(y) of PFBA in SA was 1.18 × 10–1 ng/kg bw/day, which was the highest in all data. EDIs of PFOS and PFOA were also dominant in bullfrog. The exposure of PFOS was 1.07 × 10−3–4.20 × 10−3 ng/kg bw/day in CN, 6.32 × 10−3–2.09 × 10−2 ng/kg bw/day in GD, and 1.03 × 10−2–3.40 × 10–2 ng/kg bw/day in SA, respectively. The EDICN, EDIGD, and EDISA of F-53B were 3.93 × 10−5–1.54 × 10−4, 2.32 × 10−4–7.69 × 10−4, and 3.77 × 10−4–1.25 × 10−3 ng/kg bw/day, respectively. In general, humans were exposed to F53-B in low levels via bullfrog consumption in each age group from all study areas.
The measured EDIs in this study were at lower levels than the values extracted from other studies in China. The mean daily intake via farmed fish in Beijing were 2.40 × 10−1 ng/kg bw/day for PFOS and 4.50 × 10−1 ng/kg bw/day for PFASs (Shi et al., 2012). The EDIs of PFASs through seafood consumption were found in Lianyungang City (59.80 ng/kg/day), in Yancheng City (54.70 ng/kg/day), and in Rizhao City (36.00 ng/kg/day) (Zhang et al., 2019). The Scientific Panel on Contaminants in the Food Chain within the European Food Safety Authority (EFSA) has established tolerable daily intake (TDI) for PFOS and PFOA, i.e., 150 ng/kg body weight/day and 1,500 ng/kg body weight/day, respectively (EFSA, 2008). The mean estimated intakes in the present study were about 1,800 and 2,500 times lower than the TDI for PFOA and PFOS, respectively. Therefore, we can conservatively conclude that there is a low potential exposure risk of PFASs via bullfrog consumption for local residents. However, PFASs were detected in bullfrog samples of different ages, and PFASs are difficult to metabolize and easy to accumulate in the body, which suggested that bullfrog consumption could still bioaccumulate PFASs.
HRs were calculated for PFBA, PFOA, PFBS, PFHxA, and PFOS, and the HRs of PFASs were far below the safety threshold value “1” shown in Supplementary Table S9. The results suggest that frequent consumption of these bullfrogs would not cause severe health effects on residents. Similar results were reported in other studies (Wu et al., 2019; Sun et al., 2021). However, besides the bullfrog, other food species also pose risks for human exposure, which may increase the potential risks for local residents. Nevertheless, the possible health risk related to fluorinate organic compounds should not be overlooked in the future. Hence, added information are needed on the occurrence and exposure of PFASs via aquatic product consumption to assess its long-term risk to human health.
4 Conclusion
In this study, we detected the concentrations of individual PFASs in habitat and tissues of bullfrogs during different periods from aquaculture bases in the coastal area of South China, and assessed their bioaccumulation and potential health risks to residents. Generally, short-chain PFASs were found at higher concentrations than long-chain PFASs. Meanwhile, F-53 was found at higher detectable levels and log BAFs values than PFOS in all samples from the aquaculture bases. Bullfrog farming activities could increase PFASs from the water of culture ponds to river so that we should pay more attention to pollutants from the bullfrog aquaculture base. EDIs for local residents in the study area were generally higher than those for residents in other regions. We can conservatively conclude that there is a low potential exposure risk of PFASs via bullfrog consumption for local residents. However, more systemic investigations and additional information are still urgently needed on related occurrence and exposure of PFASs via aquatic product consumptions to assess their long-term risks to human health. Meanwhile, specific countermeasures, standards, and regulations should be paid more attention by local and national governments.
Data Availability Statement
The original contributions presented in the study are included in the article/Supplementary Material. Further inquiries can be directed to the corresponding author.
Ethics Statement
The animal study was reviewed and approved by the Ethics Committee of Shantou University.
Author Contributions
TW designed the project, and wrote and conceptualized the manuscript. QS, YX, ZC, JD, YF and XZ collected and analyzed the samples. QS, YX, RB, and TW designed methodology. CS and ZZ carried out the data statistics. All authors contributed critically to the article and gave final approval for publication.
Funding
This study was supported by the National Natural Science Foundation of China (Grant Nos. 42077375 and 41877509), the Scientific Program for International Cooperation of Guangdong Province (Grant No. 2021A0505030071), and the STU scientific research foundation for talents (NTF20001).
Conflict of Interest
The authors declare that the research was conducted in the absence of any commercial or financial relationships that could be construed as a potential conflict of interest.
Publisher’s Note
All claims expressed in this article are solely those of the authors and do not necessarily represent those of their affiliated organizations, or those of the publisher, the editors, and the reviewers. Any product that may be evaluated in this article, or claim that may be made by its manufacturer, is not guaranteed or endorsed by the publisher.
Supplementary Material
The Supplementary Material for this article can be found online at: https://www.frontiersin.org/articles/10.3389/fenvs.2021.786297/full#supplementary-material
References
Ahrens, L., Taniyasu, S., Yeung, L. W. Y., Yamashita, N., Lam, P. K. S., and Ebinghaus, R. (2010). Distribution of Polyfluoroalkyl Compounds in Water, Suspended Particulate Matter and Sediment from Tokyo Bay, Japan. Chemosphere 79 (3), 266–272. doi:10.1016/j.chemosphere.2010.01.045
Ankley, G. T., Cureton, P., Hoke, R. A., Houde, M., Kumar, A., Kurias, J., et al. (2021). Assessing the Ecological Risks of Per‐ and Polyfluoroalkyl Substances: Current State‐of‐the Science and a Proposed Path Forward. Environ. Toxicol. Chem. 40 (3), 564–605. doi:10.1002/etc.4869
ANSES (2017). “Development of Oral-Administered Treatment for TRV by Perfluorohexanoic Acid (PFHxA),” in French Agency for Food, Environmental and Occupational Health & Safety (ANSES), Maisons-Alfort, France. Available at: https://www.anses.fr/en/system/files/SUBSTANCES2015SA0127EN.pdf.
Brase, R. A., Mullin, E. J., and Spink, D. C. (2021). Legacy and Emerging Per- and Polyfluoroalkyl Substances: Analytical Techniques, Environmental Fate, and Health Effects. Int. J. Mol. Sci. 22, 995–1003. doi:10.3390/ijms22030995
Buck, R. C., Franklin, J., Berger, U., Conder, J. M., Cousins, I. T., de Voogt, P., et al. (2011). Perfluoroalkyl and Polyfluoroalkyl Substances in the Environment: Terminology, Classification, and Originsfluoroalkyl and Polyfluoroalkyl Substances in the Environment: Terminology, Classification, and Origins. Integr. Environ. Assess. Manag. 7, 513–541. doi:10.1002/ieam.258
Burkhard, L. P. (2021). Evaluation of Published Bioconcentration Factor (BCF) and Bioaccumulation Factor (BAF) Data for Per‐ and Polyfluoroalkyl Substances across Aquatic Species. Environ. Toxicol. Chem. 40 (6), 1530–1543. doi:10.1002/etc.5010
Celis-Hernandez, O., Cundy, A. B., Croudace, I. W., Ward, R. D., Busquets, R., and Wilkinson, J. L. (2021). Assessing the Role of the “Estuarine Filter” for Emerging Contaminants: Pharmaceuticals, Perfluoroalkyl Compounds and Plasticisers in Sediment Cores from Two Contrasting Systems in the Southern U.K. Water Res. 189, 116610. doi:10.1016/j.watres.2020.116610
Chevreuil, P. L. M. (2010). Partitioning Behaviour of Perfluorinated Alkyl Contaminants Between Water, Sediment and Fish in the Orge River (Nearby Paris, France). Environ. Pollut. 159 (2), 391–397.
Conder, J. M., Hoke, R. A., Wolf, W. D., Russell, M. H., and Buck, R. C. (2008). Are PFCAs Bioaccumulative? A Critical Review and Comparison with Regulatory Criteria and Persistent Lipophilic Compounds. Environ. Sci. Technol. 42 (4), 995–1003. doi:10.1021/es070895g
Cui, Q., Pan, Y., Zhang, H., Sheng, N., Wang, J., Guo, Y., et al. (2018). Occurrence and Tissue Distribution of Novel Perfluoroether Carboxylic and Sulfonic Acids and Legacy Per/Polyfluoroalkyl Substances in Black-Spotted Frog (Pelophylax Nigromaculatus). Environ. Sci. Technol. 52 (3), 982–990. doi:10.1021/acs.est.7b03662
Cundy, A. B., and Croudace, I. W. (2017). The Fate of Contaminants and Stable Pb Isotopes in a Changing Estuarine Environment: 20 Years On. Environ. Sci. Technol. 51, 9488. doi:10.1021/acs.est.7b00973
Dassuncao, C., Hu, X. C., Nielsen, F., Weihe, P., Grandjean, P., and Sunderland, E. M. (2018). Shifting Global Exposures to Poly- and Perfluoroalkyl Substances (PFASs) Evident in Longitudinal Birth Cohorts from a Seafood-Consuming Population. Environ. Sci. Technol. 52 (6), 3738–3747. doi:10.1021/acs.est.7b06044
Du, D., Lu, Y., Zhou, Y., Li, Q., Zhang, M., Han, G., et al. (2021). Bioaccumulation, Trophic Transfer and Biomagnification of Perfluoroalkyl Acids (PFAAs) in the marine Food Web of the South China Sea. J. Hazard. Mater. 405, 124681. doi:10.1016/j.jhazmat.2020.124681
EFSA (2008). Perfluorooctane Sulfonate (PFOS), Perfluorooctanoic Acid (PFOA) and Their Salts. Parma, Italy: EFSA, 653.
Fauconier, G., Groffen, T., Wepener, V., and Bervoets, L. (2020). Perfluorinated Compounds in the Aquatic Food Chains of Two Subtropical Estuaries. Sci. Total Environ. 719, 135047. doi:10.1016/j.scitotenv.2019.135047
Gao, K., Miao, X., Fu, J., Chen, Y., Li, H., Pan, W., et al. (2020). Occurrence and Trophic Transfer of Per- and Polyfluoroalkyl Substances in an Antarctic Ecosystem. Environ. Pollut. 257, 113383. doi:10.1016/j.envpol.2019.113383
Guo, C., Zhang, Y., Zhao, X., Du, P., Liu, S., Lv, J., et al. (2015). Distribution, Source Characterization and Inventory of Perfluoroalkyl Substances in Taihu Lake, China. Chemosphere 127, 201–207. doi:10.1016/j.chemosphere.2015.01.053
Guo, R., Liu, X., Liu, J., Liu, Y., Qiao, X., Ma, M., et al. (2020). Occurrence, Partition and Environmental Risk Assessment of Per- and Polyfluoroalkyl Substances in Water and Sediment from the Baiyangdian Lake, china. Sci. Rep. 10 (1), 4691. doi:10.1038/s41598-020-61651-6
Hong, S., Khim, J. S., Wang, T., Naile, J. E., Park, J., Kwon, B.-O., et al. (2015). Bioaccumulation Characteristics of Perfluoroalkyl Acids (PFAAs) in Coastal Organisms from the West Coast of South Korea. Chemosphere 129, 157–163. doi:10.1016/j.chemosphere.2014.06.023
Huang, R., Chen, Z., Wang, P., Chen, S., and Huo, W. (2019). Analysis of Aquatic Food Intake of Adult Residents in Guangdong Province in 2015. South China Prev. Med. 45 (03), 283–286.
Kelly, B. C., Ikonomou, M. G., Blair, J. D., Surridge, B., Hoover, D., Grace, R., et al. (2009). Perfluoroalkyl Contaminants in an Arctic Marine Food Web: Trophic Magnification and Wildlife Exposure. Environ. Sci. Technol. 43 (11), 4037–4043. doi:10.1021/es9003894
Kwadijk, C. J. A. F., Korytár, P., and Koelmans, A. A. (2010). Distribution of Perfluorinated Compounds in Aquatic Systems in The Netherlands. Environ. Sci. Technol. 44 (10), 3746–3751. doi:10.1021/es100485e
Labadie, P., and Chevreuil, M. (2011). Partitioning Behaviour of Perfluorinated Alkyl Contaminants between Water, Sediment and Fish in the Orge River (Nearby Paris, France). Environ. Pollut. 159 (2), 391–397. doi:10.1016/j.envpol.2010.10.039
Li, B.-B., Hu, L.-X., Yang, Y.-Y., Wang, T.-T., Liu, C., and Ying, G.-G. (2020a). Contamination Profiles and Health Risks of PFASs in Groundwater of the Maozhou River basin. Environ. Pollut. 260, 113996. doi:10.1016/j.envpol.2020.113996
Li, F., Sun, H., Hao, Z., He, N., Zhao, L., Zhang, T., et al. (2011). Perfluorinated Compounds in Haihe River and Dagu Drainage Canal in Tianjin, China. Chemosphere 84 (2), 265–271. doi:10.1016/j.chemosphere.2011.03.060
Li, J., Ai, Y., Hu, J., Xu, N., Song, R., Zhu, Y., et al. (2020b). Polyfluoroalkyl Substances in Danjiangkou Reservoir, China: Occurrence, Composition, and Source Appointment. Sci. Total Environ. 725, 138352. doi:10.1016/j.scitotenv.2020.138352
Lin, Y., Jiang, J.-J., Rodenburg, L. A., Cai, M., Wu, Z., Ke, H., et al. (2020). Perfluoroalkyl Substances in Sediments from the Bering Sea to the Western Arctic: Source and Pathway Analysis. Environ. Int. 139, 105699. doi:10.1016/j.envint.2020.105699
Liu, C., Gin, K. Y. H., Chang, W. C., Goh, B. P. L., and Reinhard, M. (2011b). Novel Perspectives on the Bioaccumulation of PFCs - the Concentration Dependency. Environ. Sci. Technol. 45 (22), 9758–9764. doi:10.1021/es202078n
Liu, W., He, W., Wu, J., Qin, N., He, Q., and Xu, F. (2018). Residues, Bioaccumulations and Biomagnification of Perfluoroalkyl Acids (PFAAs) in Aquatic Animals from Lake Chaohu, China. Environ. Pollut. 240, 607–614. doi:10.1016/j.envpol.2018.05.001
Liu, X., Jin, Y., Liu, W., Wang, F., and Hao, S. (2011a). Possible Mechanism of Perfluorooctane Sulfonate and Perfluorooctanoate on the Release of Calcium Ion from Calcium Stores in Primary Cultures of Rat Hippocampal Neurons. Toxicol. Vitro 25 (7), 1294–1301. doi:10.1016/j.tiv.2011.04.016
Loi, E. I. H., Yeung, L. W. Y., Mabury, S. A., and Lam, P. K. S. (2013). Detections of Commercial Fluorosurfactants in Hong Kong marine Environment and Human Blood: a Pilot Study. Environ. Sci. Technol. 47 (9), 4677–4685. doi:10.1021/es303805k
Loi, E. I. H., Yeung, L. W. Y., Taniyasu, S., Lam, P. K. S., Kannan, K., and Yamashita, N. (2011). Trophic Magnification of Poly- and Perfluorinated Compounds in a Subtropical Food Web. Environ. Sci. Technol. 45 (13), 5506–5513. doi:10.1021/es200432n
Long, M., Wielsøe, M., and Bonefeld-Jørgensen, E. C. (2021). Time Trend of Persistent Organic Pollutants and Metals in Greenlandic Inuit during 1994-2015. Int. J. Environ. Res. Public Health 18, 2774–2775. doi:10.3390/ijerph18052774
Lu, Z., Lu, R., Zheng, H., Yan, J., Song, L., Wang, J., et al. (2018). Risk Exposure Assessment of Per- and Polyfluoroalkyl Substances (PFASs) in Drinking Water and Atmosphere in central Eastern China. Environ. Sci. Pollut. Res. 25 (10), 9311–9320. doi:10.1007/s11356-017-0950-x
MacInnis, J. J., Lehnherr, I., Muir, D. C. G., Quinlan, R., and De Silva, A. O. (2019). Characterization of Perfluoroalkyl Substances in Sediment Cores from High and Low Arctic Lakes in Canada. Sci. Total Environ. 666, 414–422. doi:10.1016/j.scitotenv.2019.02.210
Martin, J. W., Mabury, S. A., Solomon, K. R., and Muir, D. J. E. T. (2003). Bioconcentration and Tissue Distribution of Perfluorinated Acids in Rainbow trout (Oncorhynchus mykiss). Chemistry 22–1. doi:10.1897/1551-5028(2003)022<0196:batdop>2.0.co;2
Meng, J., Liu, S., Zhou, Y., and Wang, T. (2019b). Are Perfluoroalkyl Substances in Water and Fish from Drinking Water Source the Major Pathways towards Human Health Risk? Ecotoxicology Environ. Saf. 181, 194–201. doi:10.1016/j.ecoenv.2019.06.010
Meng, J., Wang, T., Song, S., Wang, P., Li, Q., Zhou, Y., et al. (2018). Tracing Perfluoroalkyl Substances (PFASs) in Soils along the Urbanizing Coastal Area of Bohai and Yellow Seas, China. Environ. Pollut. 238, 404–412. doi:10.1016/j.envpol.2018.03.056
Meng, J., Zhou, Y., Liu, S., Chen, S., and Wang, T. (2019a). Increasing Perfluoroalkyl Substances and Ecological Process from the Yongding Watershed to the Guanting Reservoir in the Olympic Host Cities, China. Environ. Int. 133, 105224. doi:10.1016/j.envint.2019.105224
Naile, J. E., Khim, J. S., Wang, T., Chen, C., Luo, W., Kwon, B.-O., et al. (2010). Perfluorinated Compounds in Water, Sediment, Soil and Biota from Estuarine and Coastal Areas of Korea. Environ. Pollut. 158 (5), 1237–1244. doi:10.1016/j.envpol.2010.01.023
Pan, C.-G., Zhao, J.-L., Liu, Y.-S., Zhang, Q.-Q., Chen, Z.-F., Lai, H.-J., et al. (2014). Bioaccumulation and Risk Assessment of Per- and Polyfluoroalkyl Substances in Wild Freshwater Fish from Rivers in the Pearl River Delta Region, South China. Ecotoxicology Environ. Saf. 107, 192–199. doi:10.1016/j.ecoenv.2014.05.031
Pan, Y., Zhang, H., Cui, Q., Sheng, N., Yeung, L. W. Y., Sun, Y., et al. (2018). Worldwide Distribution of Novel Perfluoroether Carboxylic and Sulfonic Acids in Surface Water. Environ. Sci. Technol. 52 (14), 7621–7629. doi:10.1021/acs.est.8b00829
Pérez, F., Llorca, M., Köck-Schulmeyer, M., Škrbić, B., Oliveira, L. S., da Boit Martinello, K., et al. (2014). Assessment of Perfluoroalkyl Substances in Food Items at Global Scale. Environ. Res. 135, 181–189. doi:10.1016/j.envres.2014.08.004
Schultes, L., Sandblom, O., Broeg, K., Bignert, A., and Benskin, J. P. (2020). Temporal Trends (1981-2013) of Per‐ and Polyfluoroalkyl Substances and Total Fluorine in Baltic Cod (Gadus morhua). Environ. Toxicol. Chem. 39 (2), 300–309. doi:10.1002/etc.4615
Shi, G., Cui, Q., Pan, Y., Sheng, N., Sun, S., Guo, Y., et al. (2017). 6:2 Chlorinated Polyfluorinated Ether Sulfonate, a PFOS Alternative, Induces Embryotoxicity and Disrupts Cardiac Development in Zebrafish Embryos. Aquat. Toxicol. 185, 67–75. doi:10.1016/j.aquatox.2017.02.002
Shi, Y., Wang, J., Pan, Y., and Cai, Y. (2012). Tissue Distribution of Perfluorinated Compounds in Farmed Freshwater Fish and Human Exposure by Consumption. Environ. Toxicol. Chem. 31 (4), 717–723. doi:10.1002/etc.1758
Simon, J. A., Abrams, S., Bradburne, T., Bryant, D., Burns, M., Cassidy, D., et al. (2019). PFAS Experts Symposium: Statements on Regulatory Policy, Chemistry and Analytics, Toxicology, Transport/fate, and Remediation for Per‐ and Polyfluoroalkyl Substances (PFAS) Contamination Issues. Remediation 29 (4), 31–48. doi:10.1002/rem.21624
Skogheim, T. S., Villanger, G. D., Weyde, K. V. F., Engel, S. M., Surén, P., Øie, M. G., et al. (2020). Prenatal Exposure to Perfluoroalkyl Substances and Associations with Symptoms of Attention-Deficit/hyperactivity Disorder and Cognitive Functions in Preschool Children. Int. J. Hyg. Environ. Health 223 (1), 80–92. doi:10.1016/j.ijheh.2019.10.003
Smalling, K. L., Reeves, R., Muths, E., Vandever, M., Battaglin, W. A., Hladik, M. L., et al. (2015). Pesticide Concentrations in Frog Tissue and Wetland Habitats in a Landscape Dominated by Agriculture. Sci. Total Environ. 502, 80–90. doi:10.1016/j.scitotenv.2014.08.114
Stockholm Convention (2017). Request for Information on Pentadeca Fluorooctanoic Acid (CAS No. 335-67-1, PFOA, Perfluorooctanoic Acid), its Salts and PFOA-Related Compounds.
Su, C., Jiang, H., Jia, x., Wang, H., and Zhang, B. (2019). Food and Nutrient Intake in Chinese Adults under Different Levels of Aquatic Product Consumption. Food Nutr. China 25 (11), 80–85.
Sun, Q., Bi, R., Wang, T., Su, C., Chen, Z., Diao, J., et al. (2021). Are There Risks Induced by Novel and Legacy Poly- and Perfluoroalkyl Substances in Coastal Aquaculture Base in South China? Sci. Total Environ. 779, 146539. doi:10.1016/j.scitotenv.2021.146539
Tröger, R., Ren, H., Yin, D., Postigo, C., Nguyen, P. D., Baduel, C., et al. (2021). What's in the Water? - Target and Suspect Screening of Contaminants of Emerging Concern in Raw Water and Drinking Water from Europe and Asia. Water Res. 198, 117099. doi:10.1016/j.watres.2021.117099
UNEP (2009). The New POPs under the Stockholm Convention. Available at: http://chm.pops.int/TheConvention/ThePOPs/TheNewPOPs/tabid/2511/Default.aspx.
Wang, P., Lu, Y., Wang, T., Zhu, Z., Li, Q., Meng, J., et al. (2016a). Coupled Production and Emission of Short Chain Perfluoroalkyl Acids from a Fast Developing Fluorochemical Industry: Evidence from Yearly and Seasonal Monitoring in Daling River Basin, China. Environ. Pollut. 218, 1234–1244. doi:10.1016/j.envpol.2016.08.079
Wang, Q.-W., Yang, G.-P., Zhang, Z.-M., and Jian, S. (2017). Perfluoroalkyl Acids in Surface Sediments of the East China Sea. Environ. Pollut. 231, 59–67. doi:10.1016/j.envpol.2017.07.078
Wang, Q., Tsui, M. M. P., Ruan, Y., Lin, H., Zhao, Z., Ku, J. P. H., et al. (2019). Occurrence and Distribution of Per- and Polyfluoroalkyl Substances (PFASs) in the Seawater and Sediment of the South China Sea Coastal Region. Chemosphere 231, 468–477. doi:10.1016/j.chemosphere.2019.05.162
Wang, S., Huang, J., Yang, Y., Hui, Y., Ge, Y., Larssen, T., et al. (2013). First Report of a Chinese PFOS Alternative Overlooked for 30 years: its Toxicity, Persistence, and Presence in the Environment. Environ. Sci. Technol. 47 (18), 10163–10170. doi:10.1021/es401525n
Wang, Y., Vestergren, R., Shi, Y., Cao, D., Xu, L., Cai, Y., et al. (2016b). Identification, Tissue Distribution, and Bioaccumulation Potential of Cyclic Perfluorinated Sulfonic Acids Isomers in an Airport Impacted Ecosystem. Environ. Sci. Technol. 50 (20), 10923–10932. doi:10.1021/acs.est.6b01980
Wang, Z., Cousins, I. T., Scheringer, M., Buck, R. C., and Hungerbühler, K. (2014). Global Emission Inventories for C4-C14 Perfluoroalkyl Carboxylic Acid (PFCA) Homologues from 1951 to 2030, Part I: Production and Emissions from Quantifiable Sources. Environ. Int. 70, 62–75. doi:10.1016/j.envint.2014.04.013
Wen, W., Xia, X., Zhou, D., Wang, H., Zhai, Y., Lin, H., et al. (2019). Bioconcentration and Tissue Distribution of Shorter and Longer Chain Perfluoroalkyl Acids (PFAAs) in Zebrafish (Danio rerio): Effects of Perfluorinated Carbon Chain Length and Zebrafish Protein Content. Environ. Pollut. 249, 277–285. doi:10.1016/j.envpol.2019.03.003
Wilkinson, J. L., Hooda, P. S., Swinden, J., Barker, J., and Barton, S. (2018). Spatial (Bio)accumulation of Pharmaceuticals, Illicit Drugs, Plasticisers, Perfluorinated Compounds and Metabolites in River Sediment, Aquatic Plants and Benthic Organisms. Environ. Pollut. 234, 864. doi:10.1016/j.envpol.2017.11.090
Wu, J.-Y., Liu, W.-X., He, W., and Xu, F.-L. (2019). Comparisons of Tissue Distributions and Health Risks of Perfluoroalkyl Acids (PFAAs) in Two Fish Species with Different Trophic Levels from Lake Chaohu, China. Ecotoxicology Environ. Saf. 185, 109666. doi:10.1016/j.ecoenv.2019.109666
Xie, Z., Wang, Z., Magand, O., Thollot, A., Ebinghaus, R., Mi, W., et al. (2020). Occurrence of Legacy and Emerging Organic Contaminants in Snow at Dome C in the Antarctic. Sci. Total Environ. 741, 140200. doi:10.1016/j.scitotenv.2020.140200
Xu, J., Guo, C.-S., Zhang, Y., and Meng, W. (2014). Bioaccumulation and Trophic Transfer of Perfluorinated Compounds in a Eutrophic Freshwater Food Web. Environ. Pollut. 184, 254–261. doi:10.1016/j.envpol.2013.09.011
Yamazaki, E., Taniyasu, S., Ruan, Y., Wang, Q., Petrick, G., Tanhua, T., et al. (2019). Vertical Distribution of Perfluoroalkyl Substances in Water Columns Around the Japan Sea and the Mediterranean Sea. Chemosphere 231, 487–494. doi:10.1016/j.chemosphere.2019.05.132
Yan, S., Cai, Q., Fang, H., Zhang, C., Wu, L., Ma, X., et al. (2015). Investigation and Evaluation of Dietary Structure of Aquatic Products in Xiamen. China Prim. Health Care 29 (3), 72–75.
Zhang, A., Wang, P., Lu, Y., Zhang, M., Zhou, Y., Wang, Y., et al. (2019). Occurrence and Health Risk of Perfluoroalkyl Acids (PFAAs) in Seafood from Yellow Sea, China. Sci. Total Environ. 665, 1026–1034. doi:10.1016/j.scitotenv.2019.02.165
Zhao Xiang, H., Meng, Z., and Chun Xia, L. (2011). Toxicokinetic Behaviors and Modes of Perfluorooctane Sulfonate (PFOS) and Perfluorooctane Acid (PFOA) on tilapia (Oreochromis niloticus). Afr. J. Biotechnol. 10 (60), 12943–12950. doi:10.5897/ajb11.1767
Zhou, Y., Meng, J., Zhang, M., Chen, S., He, B., Zhao, H., et al. (2019). Which Type of Pollutants Need to Be Controlled with Priority in Wastewater Treatment Plants: Traditional or Emerging Pollutants? Environ. Int. 131, 104982. doi:10.1016/j.envint.2019.104982
Zhou, Y., Wang, T., Li, Q., Wang, P., Li, L., Chen, S., et al. (2018). Spatial and Vertical Variations of Perfluoroalkyl Acids (PFAAs) in the Bohai and Yellow Seas: Bridging the gap between Riverine Sources and marine Sinks. Environ. Pollut. 238, 111–120. doi:10.1016/j.envpol.2018.03.027
Zhou, Y., Zhou, Z., Lian, Y., Sun, X., Wu, Y., Qiao, L., et al. (2021). Source, Transportation, Bioaccumulation, Distribution and Food Risk Assessment of Perfluorinated Alkyl Substances in Vegetables: A Review. Food Chem. 349 (8), 129137. doi:10.1016/j.foodchem.2021.129137
Zhou, Z., Liang, Y., Shi, Y., Xu, L., and Cai, Y. (2013). Occurrence and Transport of Perfluoroalkyl Acids (PFAAs), Including Short-Chain PFAAs in Tangxun Lake, China. Environ. Sci. Technol. 47 (16), 9249–9257. doi:10.1021/es402120y
Keywords: PFASs, bullfrog, tissue distribution, bioaccumulation, health risk
Citation: Sun Q, Xiong Y, Bi R, Zhan X, Fan Y, Su C, Chen Z, Zheng Z, Diao J and Wang T (2021) Occurrence, Profile, and Potential Risks of Novel and Legacy Polyfluoroalkyl Substances in Bullfrogs: Pilot Study in an Intensive Aquaculture Region, China. Front. Environ. Sci. 9:786297. doi: 10.3389/fenvs.2021.786297
Received: 30 September 2021; Accepted: 25 October 2021;
Published: 25 November 2021.
Edited by:
Weilan Zhang, University at Albany, United StatesReviewed by:
Yinghao Wen, Texas A&M University, United StatesHongxia Zhang, Ludong University, China
Copyright © 2021 Sun, Xiong, Bi, Zhan, Fan, Su, Chen, Zheng, Diao and Wang. This is an open-access article distributed under the terms of the Creative Commons Attribution License (CC BY). The use, distribution or reproduction in other forums is permitted, provided the original author(s) and the copyright owner(s) are credited and that the original publication in this journal is cited, in accordance with accepted academic practice. No use, distribution or reproduction is permitted which does not comply with these terms.
*Correspondence: Tieyu Wang, d2FuZ3RpZXl1QDE2My5jb20=