- 1Institute of Environmental Microbiology, College of Resources and Environment, Fujian Agriculture and Forestry University, Fuzhou, China
- 2Agricultural College, Guangxi University, Nanning, China
Soil pollution by multiple metal(loid)s is a common problem, and it is not easy to synchronously reduce their uptake in crops. Compounds containing iron (Fe) are often used to efficiently remediate soil metal(loid) pollution; however, its associated risks did not receive much attention especially under unsuitable soil water conditions. Pot experiments were set up using an antimony (Sb) and cadmium (Cd) co-contaminated soil treated with a continued submergence condition plus 5, 10, or 20 mg kg−1 FeCl3 (Experiment I), or treated with different water management including submergence, intermittent irrigation, and dry farming (Experiment II). Our results showed that the continued submergence resulted in excessive accumulation of arsenic (As) in different tissues of rice plants even if the soil As background concentration is low. High soil moisture content increased the available concentrations of Sb and As, but reduced that of Cd in rhizosphere soils, which was in line with their concentrations in different tissues of rice plants (Experiment II). Under a continued submergence condition, FeCl3 significantly stimulated As concentration in the shoots, roots (excluded Fe20 treatment), and husks, but reduced it in the grains. FeCl3 reduced Sb concentration only in the roots and grains, and reduced Cd concentration only in the husks, suggesting a limited efficiency of FeCl3 to reduce Cd uptake under a submergence condition. In this study, the dynamic changes of As, Sb, and Cd concentrations in soil solution, their available concentrations in rhizosphere soils, their accumulation in root iron/manganese plaques, and the relationships among the above parameters were also discussed. We suggested that if FeCl3 would be used to remediate the contaminated soils by Sb and Cd, dry farming for a short time is needed to avoid As accumulation, and intermittent irrigation is a potential choice to avoid the excessive accumulation of As, Sb, and Cd in the edible parts of rice plants.
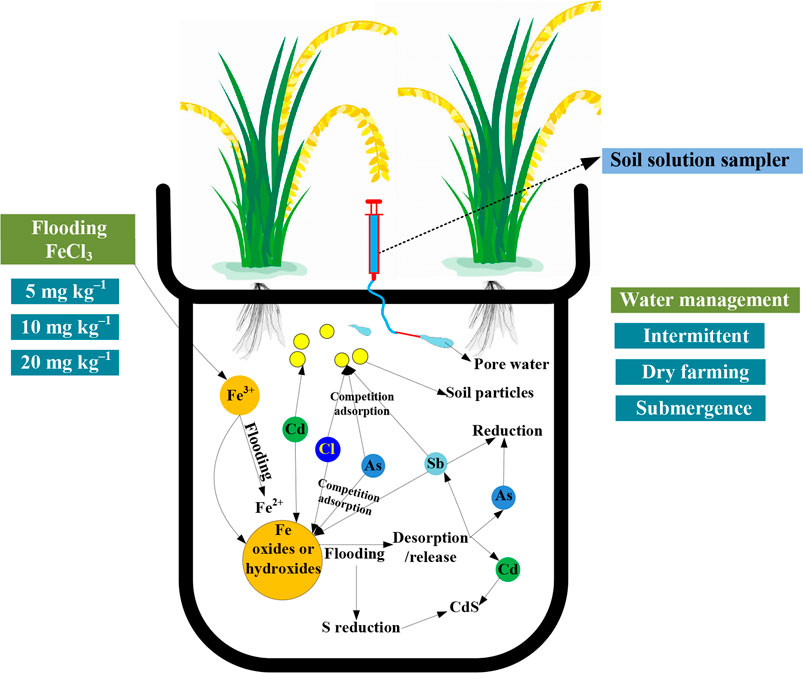
GRAPHICAL ABSTRACT. Schematic diagram of experimental design and processes of adsorption/desorption of elements and reduction of As, Sb and ferric iron in flooded soils.
Introduction
Anthropogenic activities such as mining, smelting, and waste disposal usually release multiple metal(loid)s into the environment (Macgregor et al., 2015; Tabelin et al., 2018; Tabelin et al., 2021). Ever-increasing levels of arsenic (As), antimony (Sb), and cadmium (Cd) have been found in the environment in Canada (Fawcett et al., 2015) and China (Fu et al., 2010; Fu and Wei, 2013; Fu et al., 2016). Therefore, it is important to simultaneously immobilize toxic metal(loid)s in soils to reduce their accumulation in crops.
Few technologies are explored to repair the contaminated soils by multiple metal(loid)s, especially for Sb contamination. Materials containing Fe are often used to remediate the contaminated soils by As (Chou et al., 2016), Sb (Van Caneghem et al., 2016) and Cd (Huang et al., 2018) via some mechanisms, such as promoting Sb adsorption by iron (hydr)oxides (Van Caneghem et al., 2016) or forming a stable tripuhyite under strong acidic conditions (Leverett et al., 2012). In addition, Fe will stimulate the formation of Fe/manganese (Mn) plaques on the surface of rice roots (Huang et al., 2012). However, the inhibition and stimulation of Sb uptake in plants resulting from the actions of root Fe/Mn plaques were simultaneously observed in many studies (Ren et al., 2014; Cui et al., 2015; Huang et al., 2012). The above contradictory results might suggest that there will be some factors controlling the outcomes of compounds containing Fe to restrict Sb uptake.
The normal cultivation pattern for rice plants needs a long-time submerged condition and a short-time dry farming for 5 to 10 days at the end of tillering stage or the beginning of jointing stage (Yao et al., 2012). This water cycle will increase the release risk of metal(loid) pollution in soils (Huyen et al., 2019). In China, there is plenty of rain within most of rice cultivation areas (mainly locate in the south provinces of China), where the situation of soil metal(loid) contamination is also heavy (Mao et al., 2019). Thus, it is possible that the time of dry farming for these contaminated paddy soils will not be enough. The submerged conditions will stimulate the release of metalloids into soil solution and result in their excessive accumulation in plants, such as As and Sb (Takahashi et al., 2004; Xu et al., 2008; Wan et al., 2013; Liao et al., 2016; Matsumoto et al., 2016). In contrast, high soil moisture will be beneficial to restrict Cd uptake in rice plants (Simmons et al., 2008; Liao et al., 2016) via combination of Cd with sulfur (S) to form an insoluble compound (CdS) under an anaerobic condition (Bingham et al., 1976). Some researchers have attempted to use the water management technology to control the availability of targeted metal(loid)s in soils and thus reduce their accumulation in crops (Hu et al., 2015). However, the optimal water management manner is not clear when using this technology to remediate the contaminated paddy soils by multiple metal(loid)s.
In addition, when using the compounds containing Fe or the technology of water management to reduce uptake of metal(loid)s in crops, few concerns were paid on their effects on quality of crop products. The fill-up of the above knowledge gaps will make people notice the risks when using FeCl3 or water management to remediate Sb and Cd co-contaminated soils under an impeded drainage condition. Therefore, this study was conducted using FeCl3 (under a continued submergence condition) and different water management patterns in potted experiments to investigate: (1) the efficiency and risks of FeCl3 and water management to reduce uptake of Cd and Sb in a rice plant (Yangdao No.6); (2) the geochemical evolution of pore water with elongation of exposure time; (3) the formation of root Fe/Mn plaques and their roles in controlling uptake of elements; and (4) the uptake of essential elements and the yield of rice plants.
Materials and Methods
Soil Preparation and Experimental Design
The tested soil was collected from a farmland in the vicinity of a mining area (Xikuangshan) in Lengshuijiang city, Hunan province, China. The pH value of the soil is 5.30, and the concentration of potassium (K), Fe, calcium (Ca), magnesium (Mg), Mn, and zinc (Zn) is 2.56 g kg−1, 6.87 g kg−1, 28.66 g kg−1, 0.26 g kg−1, 29.79 mg kg−1, and 49.41 mg kg−1, respectively. The concentration of soil organic matter, available phosphorus (P), available K, total nitrogen, and total P is 22.30 g kg−1, 12.88 mg kg−1, 0.09 g kg−1, 2.81 g kg−1, and 23.86 g kg−1, respectively. The concentration of Sb, As, and Cd is 84.79, 10.89, and 0.43 mg kg−1, respectively. The available concentration of Sb, Cd, and As is 0.37 g kg−1, 0.47 mg kg−1, and 0.12 mg kg−1, respectively. According to the Environmental Quality Standards for soils in China (GB 15618–2008), the soil concentration of Cd and Sb is 1.72 and 8.48 times higher than their individual Quality Standard, respectively [pH ≤ 5.5 for a paddy field, 0.25 mg kg−1 for Cd, and 10 mg kg−1 for Sb (a suggested value)]. Although the soil As concentration was lower than its Quality Standard (pH ≤ 5.5 for a paddy field, 35 mg kg−1), the unsuitable water management would lead to As accumulation in edible parts of crops (Liao et al., 2016). Therefore, in this study, we also monitored the As concentration. The collected soil was air dried, sieved through a 2-mm nylon screen, mixed thoroughly, and finally placed in darkness until use.
There were two experiments in this study. The first one was conducted to study the effects of FeCl3 on the uptake of Sb, Cd, and As by rice plants. There were four treatments: control (CK), Fe5 (5 mg kg−1), Fe10 (10 mg kg−1), and Fe20 (20 mg kg−1). The soil was submerged with a water depth of 3–4 cm above topsoil throughout the rice growing period (soil cultivation continued for 95 days).
The second experiment was designed to investigate the efficiency of different water management patterns to control the uptake of the above metal(loid)s by rice plants. There were three treatments as follows: (1) Flooding: The water management was the same as in Experiment I. (2) Intermittent irrigation: The soil was flooded first, and next naturally dried to make the soil moisture content be about 50% of field capacity (weight method), and then re-submerged. The above processes were repeated until harvest. (3) Dry farming: The soil moisture content was always maintained at 60%–80% of field capacity. Each treatment of the above two experiments had triple replications. The two experiments were performed synchronously.
Basal fertilizers were added to each pot as follows: 0.20 g CO(NH)2, 0.12 g NaH2PO4, and 0.26 g KCl for both experiments. The soil was thoroughly mixed with the basal fertilizers (Experiment I and II) and the compound containing iron (Experiment I) as the designs, and then potted. Each pot was filled with 5 kg soil. To investigate the changes of Sb, Cd and As concentrations in soil pore water, a soil solution sampler (19.21.26F RHIZON FLEX, Agro Business Park Wageningen Netherlands) was buried into the soil of each pot (considered as a replication) to collect soil pore water. After that, the soil was flooded with de-ionized water to rebalance in a glass greenhouse for 2 weeks. Two weeks later, the rice seedlings were transplanted into the pots (two seedlings per pot).
Plant Culture and Management
Rice seeds (Oryza sativa L., Yangdao No.6) were disinfected by 30% H2O2 for 20 min, and then rinsed thoroughly with de-ionized water. These seeds were sown in a moist culture medium composed of vermiculite and perlite (1 : 1, v: v), germinated, and grew in a greenhouse for 3 weeks.
Three weeks later, the rice seedlings with a uniform size were transplanted into a half-strength Hoagland–Aron solution (Hoagland and Arnon., 1950) for their further development for 2 weeks. The nutrient solution was replaced every 3 days and its pH was adjusted to 5.5 using 0.1 mol L−1 NaOH and HNO3. The 50% HN solution was composed of 2.5 mM KNO3, 0.5 mM NH4NO3, 0.5 mM NH4H2PO4, 2 mM Ca(NO3)2•4H2O, 1 mM MgSO4•7 H2O, 4.5 μM MnCl2•4H2O, 23 μM H3BO3, 0.4 μM ZnSO4•7H2O, 0.15 μM CuSO4•5H2O, 0.05 μM H2MoO4, and 4.5 μM EDTA-Fe. The growth conditions of the greenhouse were 25/20°C (day/night) temperatures, a 60–70% relative humidity, and a 16-h photoperiod with a light intensity of 100 μmol m−2·s−1.
Two weeks later, the rice seedlings were transplanted into plastic pots. During the soil cultivation process, the rice plants were placed in a glass greenhouse under a natural light at temperatures of 15–38°C.
Sampling and Analysis
Sampling of Soil Pore Water
The soil solution was collected by a soil solution sampler at the first, third, seventh, 10th, 20th, and 60th days after the transplanting of seedlings into soils. After being filtered through a 0.22-μM filter, diluted nitric acid solution (1%) was added into the soil solution to prevent the precipitation of ions, and then stored at 4°C until the determination of elemental concentrations using Inductively Coupled Plasma Mass Spectrometry (ICP-MS, iCAP Qc ICP-MS, Thermo Fisher, United States).
Plant Harvest
The plant height and rice spike numbers were recorded before the seedlings were harvested (upon no visible water in the pots at the ninety-fifth day). The attached soil on the root surface was brushed off, collected, and assigned as the rhizosphere soils. After that, the seedlings were separated into the roots, shoots, and grains (with husks). The grains (with husks) were air dried, and the yield of each pot was recorded. Next, the grains (with husks) were de-hulled, and then the shelled grains and husks were separately ground using a micro-mill for the determination of elemental concentrations. The roots and shoots were rinsed with de-ionized water thoroughly, and the water adhering to the surface of samples was removed using filter papers. After that, the fresh weights of roots and shoots were recorded.
A part of fresh root samples was gathered to extract root Fe/Mn plaques using a modified dithionite–citrate–bicarbonate (DCB) method (Li et al., 2016). Briefly, approximately 1.00 g of fresh rice roots was weighed and incubated in a 30-ml DCB extract (0.03 mol L−1 sodium citrate, 0.125 mol L−1 NaHCO3, and 0.5 g sodium hydrosulfite) for 1 h at 25°C. After that, the above rice root samples, the remaining root samples (excluding the root samples used for the extract of root plaques), and the shoot samples were oven-dried at 70°C for 48 h to a constant. The dry weights of the above oven-dried samples were recorded, and then pulverized for the determination of elemental concentrations.
Soil Sampling and Treatment Procedure
The air-dried rhizosphere soil was sieved through a 0.15-mm nylon screen and used to measure the pH value and the available concentrations of Sb, As, and Cd. The available concentrations of Sb, As, and Cd were measured according to the method of Liu et al. (2016). Briefly, approximately 5 g rhizosphere soil sample was weighted and transferred into a 50-ml centrifuge tube, and then 25 ml of 0.1 M HCl was added. The centrifuge tube was shaken at 180 r min−1 for 2 h at 25°C, and then the mixture was filtered into a volumetric flask, topped up to 100 ml, and stored at 4°C in a refrigerator until use.
Digestion Methods
An ED54 DigiBlock digestion system (Lab Tech, Inc., Hopkinton, MA, United States) was used to digest the plant and soil samples. The digestion method was described in the studies of Liao et al. (2016) and Wu et al. (2017). For the digestion of soil samples, 10 ml of HNO3, 4 ml of HF, and 0.25 g of soil samples were mixed in a digestion tube and incubated overnight. Samples were heated first at 120°C for 1 h, and then at 150°C for 2 h. Next, the liquid in the tube was evaporated at 180°C until the liquid volume is approximately 1 ml. After that, the liquid volume was topped up to 25 ml using de-ionized water, filtered through a 0.22-μm filter, and then used to determine the elemental concentrations by ICP–MS.
The digestion method for plant samples was as follows: 15 ml of HNO3 and 0.2 g of plant samples were mixed in a digestion tube and incubated overnight. The samples were first heated to 80°C for 1.5 h, and then at 120°C for 1.5 h, and at 150°C for 3 h. Finally, the liquid in the tube was evaporated to be approximately 1 ml under a temperature of 180°C. After that, the volume of the above liquid was topped up to 50 ml in a volumetric flask, and filtered through a 0.22-μm filter for the determination of elemental concentrations using ICP-MS.
The concentrations of Fe and Mn in the extraction solution were determined using a Flame atomic absorption spectrometer (Zeenit700P, Analytik jena, Germany) and the concentrations of other elements in the extraction solution were determined using ICP-MS.
Standard reference materials including green tea leaves (GBW10052), grains (GBW10045), and soils (GBW07452) were purchased from the Institute of Geophysical and Geochemical Exploration, China. When using ICP-MS to determine the elemental concentrations, the relative standard deviation (RSD) of each sample is less than 10% and the recovery rate is between 90% and 120%.
Data Analysis
The bioconcentration factors (BCFs) and translocation factors (1 and 2) of Sb, Cd, and As were calculated using Eqs 1–3, respectively (Takarina and Pin., 2017; Otones et al., 2011).
where CSb/As/Cd(roots) denotes the concentration of Sb, As, and Cd in roots of rice plants, respectively; CSb/As/Cd(soil) denotes the concentration of Sb, As, and Cd in soils, respectively; and CSb/As/Cd(grains) indicates the concentration of Sb, As, or Cd in grains, respectively.
One-way ANOVA analysis combined with multiple comparisons (Tukey’s test) was employed to compare significant differences between different treatments (p ≤ 0.05). Results were expressed as means with standard errors (n = 3). Data analysis was performed using the SPSS18.0 (SPSS Inc., Chicago, IL, United States) software and the figures were drawn using a SigmaPlot software14.0.
Results and Discussion
Plant Growth and Yield
This study was conducted to investigate the efficiency and risks of using FeCl3 or water management to control the uptake of Sb and Cd in a rice plant. The results showed that 20 mg kg−1 Fe significantly increased the fresh weights of shoots and roots but decreased the height of this plant (Table 1). However, this fresh weight stimulation was not accompanied with enhanced spike numbers and hundred-grain weight (Table 1). These results might indicate that the addition of Fe facilitated the vegetative growth but did not show beneficial effects on the reproductive growth of this rice plant under a continued submergence condition. The above results were not in line with the results of Huang et al. (2018), who reported that FeSO4•7H2O as a base fertilizer could significantly increase rice grain yield. This inconsistence might be partially due to the continued submergence condition. Because alternate wetting and drying farming regimes could increase grain yield and plant height in mature stages of rice plants (Maneepitak et al., 2019). In this study, similar stimulation on spike numbers and plant height were also demonstrated when compared to the flooding treatment (Table 1).
Dynamic Changes of Elements in Soil Solution and Their Available Concentrations in Rhizosphere Soils
Soil solution plays an important role in regulating dynamic balance of nutrient elements in soil (Li and Hou, 1998), which will affect their uptake in plants. On the first day, the addition of Fe resulted in obviously lower Sb concentration relative to the CK treatment (Figure 1A and Supplementary Figure S1), possibly indicating a co-precipitation of Fe and Sb since previous studies suggested that the Fe may precipitate with Sb in soils (Tandy et al., 2017). However, with the elongation of exposure time, the soil Eh under a submerged condition will decrease (Zheng et al., 2019) and result in the reduction of Fe3+ to Fe2+ (Frohne et al., 2011), As(V) to As(III) (Makino et al., 2016), and Sb(V) to Sb(III) (Wan et al., 2013). As(III) had a lower affinity to the soil solid phase than As(V) (Suriyagoda et al., 2018); however, Sb(III) showed a higher affinity with hydrous ferric oxide and goethite than Sb(V) (Zhu et al., 2020). Therefore, the reducing dissolution of added Fe led to the steady release of As and Sb with time from dissolved Fe oxides into the soil solution (Figures 1A,B), and the enhanced Sb available concentration in the rhizosphere soil (Table 1).
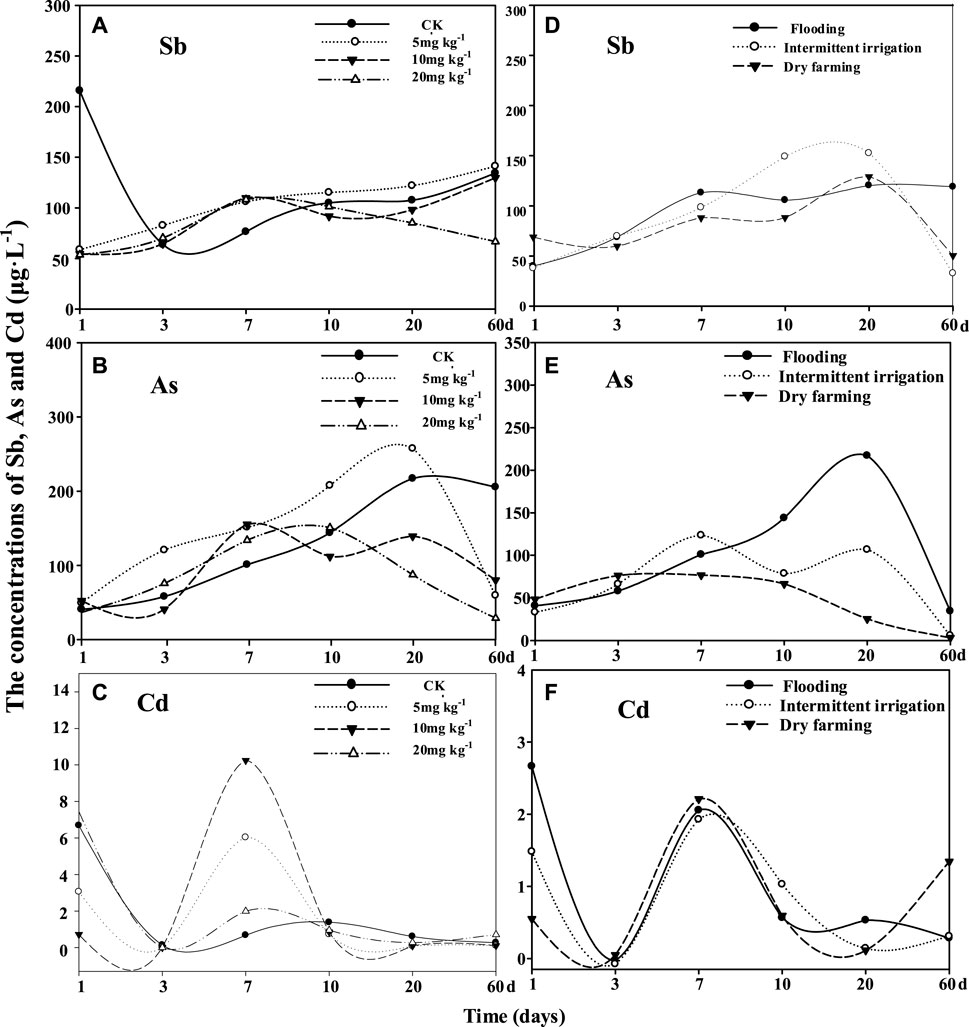
FIGURE 1. Dynamic changes of Sb, As, and Cd concentrations in the soil solution with the elongation of time. Panels (A–C) indicates the changes of Sb, As, and Cd concentrations in the soil solution under different FeCl3 treatments, respectively. Panels (D–F) indicate the changes of Sb, As and Cd concentrations in the soil solution under the flooding, intermittent irrigation, and dry farming treatments, respectively.
Reports have shown that the materials containing Fe can effectively immobilize As and Sb in soils (Ko et al., 2015; Yuan et al., 2017; Mitsunobu et al., 2006). However, there were no regular differences in the concentrations of As (third day to 20th day) and Sb (third day to 20th day) under Fe treatments relative to the CK treatment (Figure 1 and Supplementary Figure S1). This might be due to the dynamic balance of plant uptake and the processes of biological as well as physicochemical occurring in soils. On the 60th day, the solution Sb concentration at 20 mg kg−1 Fe and the solution As concentration in all Fe treatments were obviously lower than that in the CK treatment (Figure 1 and Supplementary Figure S1). The related reasons for these changes were discussed below.
1) Cl¯ had a weak effect on the desorption of [Sb(OH)6]− (Biver et al., 2011) and H2AsO4¯ (Ryden et al., 1987) for the same binding sites. Therefore, the supplement of Cl¯ was not the reason for the decreased Sb and As concentrations in the soil solution on the 60th day.
2) The adsorption of As and Sb by soil Fe oxides was weakened, because of the reducing dissolution of Fe and thus the more release of adsorbed As (DeLemos et al., 2006) and Sb under a submerged condition (He et al., 2015). After the addition of FeCl3, the unchanged As bioavailable concentration in the rhizosphere soils (Table 1) was not well in line with the unregular changes in As concentration in the soil solution (Figure 1B). The above results might suggest that FeCl3 did not show a significant effect on the available concentration of As at harvest.
3) The decreased soil solution Sb and As concentrations on the 60th day seemed not to be due to the competitive adsorption between Sb(V) and As(III) or between As(III) and Sb(III). Because (1) although the flooding condition is supposed to facilitate the more transformation of Sb(V) to Sb(III) (Wan et al., 2013) and As(V) to As(III) (Makino et al., 2016), Sb(V) will be often detected in water under anaerobic conditions, which mainly exists in the water as [Sb(OH)6]− (Filella et al., 2002). (2) As(III) is predominant under anaerobic conditions, as well as As(III) and Sb(III) mainly exist in the environment as neutral molecules (McFarlane et al., 2003).
4) It was speculated that the decreased soil solution Sb and As concentration is probably ascribed to the combined effects of (1) their adsorption/desorption in soil, (2) reducing dissolution of Fe accompanying with more release of As and Sb (Cui et al., 2015; Matsumoto et al., 2016; Karimian et al., 2019), (3) effects of soil microorganisms, and (4) the huge uptake of Sb and As at the stage of high nutritional demand in this rice plant.
Nevertheless, as compared to the CK treatment, the unchanged soil Cd available concentration in the rhizosphere soil (Table 1) matched well with the relatively constant concentrations of Cd in the soil solution on the 20th day to 60th day (Figure 1 and Supplementary Figure S1). The above results might be related to the continued submergence condition, which resulted in the co-precipitation of Cd and S (Cornu et al., 2008).
The results of Experiment II further confirmed the important roles of soil moisture in regulating the concentrations of As, Sb, and Cd in soil and soil solution. We found that soil solution concentration (Figure 1E, on the 10th to 60th day) and available concentration (Table 1) of As were both higher under a high soil moisture than that under a low soil moisture. On the 60th day, the Sb concentration in soil solution under a high soil moisture was significantly higher than that under a lower soil moisture content (Figure 1D and Supplementary Figure S1). In contrast, the available concentration of Cd was higher under a low soil moisture than that under high soil moisture (Table 1). All of the above results suggested that high soil moisture will facilitate the release of As and Sb but reduce Cd availability. Similar results were also reported in the studies of Li and Xu (2017).
Roles of Fe/Mn plaques in Constraining Sb, As, and Cd Might Be Ion-level-dependent in Soils
In this study, exogenous Fe stimulated the formation of Fe/Mn plaques (Figure 2A), which was well in line with the results of a previous study by Huang et al. (2012). Intermittent irrigation and dry farming treatments promoted the Mn plaque when compared to the flooding treatment (Figure 2B), suggesting that a low soil moisture will be beneficial for the formation of root Mn plaques. Reports suggest that the formation of Fe/Mn plaques needs an oxidation process of Fe/Mn (Pittman, 2005), and the stimulation of a low soil moisture on Mn plaque formation is thus expected.
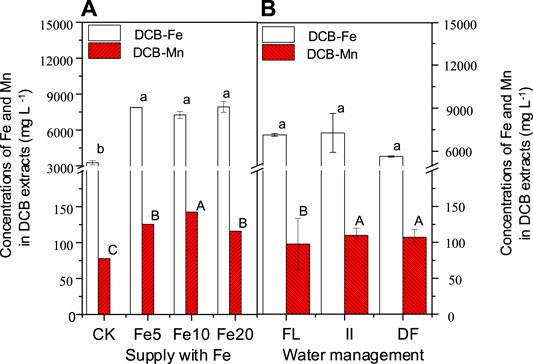
FIGURE 2. Effects of FeCl3 and water management on the formation of iron/manganese plaques. Bars are means and standard deviations for three replications. Different lowercase letters, capital letters, denote significant differences (at p ≤ 0.05) for Fe and Mn concentrations in the DCB extracts among different treatments, respectively.
Exogenous Fe significantly enhanced As concentration but did not significantly affect Sb and Cd concentrations in DCB extracts when compared to the control (Figure 3A). The enhanced As accumulation in root Fe plaque of rice plants was also observed in the study of Li et al. (2015). However, the enhanced accumulation of As in root Fe/Mn plaques did not result in less accumulation of As in most tissues of rice plants (Figure 4). The above results further questioned the roles of Fe/Mn plaques acting as a barrier, and it was speculated that their roles acting as a barrier should be condition-dependent, such as dose-dependent (Ren et al., 2014; Cui et al., 2015) and speciation-dependent (Huang et al., 2012). Here, the Fe/Mn plaques will play a storage role for most elements, because (1) the unchanged As available concentration (Table 1) and unregular changes of As concentration in the soil solution (Figure 1) cannot explain the significantly enhanced As accumulation in the most tissues of this rice plant (Figure 4); (2) exogenous Fe generally enhanced concentrations of Mg, K, and Zn in root plaques, and K, Ca, Mn, and Zn in roots; and (3) the essential metal elements in the roots of this rice plant were much lower than that in the roots of normal growth rice plant in the study of Liu et al. (2019), especially for Mg, K, Ca, Mn, and Zn. Therefore, we speculated that when the concentrations of essential metal elements in soils are low, Fe/Mn plaques will accumulate these elements to support normal growth of plants (Supplementary Table S1). However, this process will result in synchronously the accumulation of toxic metal(loid)s because there are not enough cations to combine anions and thus keep anion–cation balance.
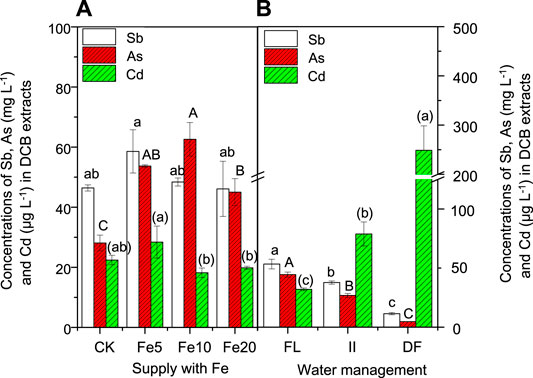
FIGURE 3. Effects of FeCl3 and water management on the concentrations of Sb, As, and Cd in the iron/manganese plaques. Bars are means and standard deviations for three replications. Different lowercase letters, capital letters, and parenthesized letters denote significant differences (at p ≤ 0.05) for Sb, As, and Cd concentrations in the DCB extracts among different treatments, respectively.
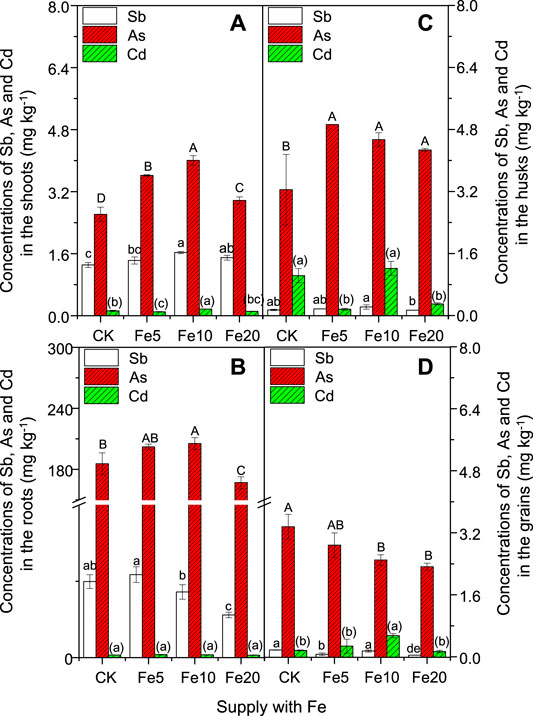
FIGURE 4. Effects of FeCl3 on the concentrations of Sb, As, and Cd in the shoots, roots, husks, and grains. Bars are means and standard deviations for three replications. Different lowercase letters, capital letters and parenthesized letters denote significant differences (at p ≤ 0.05) for Sb, As, and Cd concentrationsin the shoots, roots, husks and grains among different treatments, respectively.
Unlike As, the addition of Fe had no significant effect on the DCB-Sb concentration (Figure 3A), but significantly enhanced the available concentration of Sb in the rhizosphere soils (Table 1). The unregular changes in Sb concentration in soil solution did not match well with the unaffected concentrations of Sb in Fe/Mn plaques and enhanced available concentration in the rhizosphere soil. Unexpectedly, the addition of Fe did not significantly affect the Cd concentration in DCB extracts (Figure 3A) and the available Cd concentration in the rhizosphere soils (Table 1). Liu et al. (2007) found that the addition of 50 mg L−1 Fe-EDTA significantly increased the concentration of Cd in root Fe plaque. However, root Mn plaque of cattail (Typha latifolia L.) was also found to loss its roles in inhibiting Cd uptake in a nutrient solution culture (Ye et al., 2003). In this study, the unaffected Sb and Cd concentrations in the root Fe/Mn plaques might be due to the scarce capacity of Fe/Mn plaques under a continued submergence condition to adsorb so many kinds of metal(loid)s. Because the addition of Fe also increased the concentrations of Mg, K, and Zn in the DCB extracts (Supplementary Table S1).
In Experiment II, we found that low soil moisture content facilitated the formation of Mn plaque (Figure 2B), which showed a negative effect on the accumulation of As and Sb, and a positive effect on the Cd accumulation in the root DCB extracts. The above results might suggest that under dry farming conditions, root Mn plaque might exert its functions on restraining Cd. A previous study showed that a large amount of As could be accumulated on Mn plaque of rice roots (Liu et al., 2005).
Exogenous Fe Stimulated as Accumulation in Most Tissues of This Rice Plant Under a Continued Flooding Condition and Thus Possessed Risks
Unexpectedly, the flooding condition results in excess accumulation of As in the shoots, roots, husks, and brown rice (Figure 4), even when the soil As concentration was as low as 10.89 mg kg−1. In addition, exogenous Fe only significantly reduced the brown rice As concentration and in most cases increased As accumulation in the shoots, roots, and husks (Figures 4A–C). The unregular changes in soil solution As concentration and the unchanged available concentration of As in the rhizosphere soil (Table 1) did not match well with the enhanced As concentrations in the shoots, roots, and husks. Only the enhanced As concentration in DCB extracts (Figure 3A) was in line with the As accumulation in the above tissues, which might suggest the role of Fe/Mn plaques acting as a storage for As uptake at this moment. Although the FeCl3 stimulated the As uptake in some tissues, it reduced the translocation of As from roots to shoots (decreased BCF value), and roots to grains (decreased TF2 value) (Supplementary Table S2).
Unlike As, the addition of FeCl3 only significantly enhanced the shoot Sb concentration but reduced grain and root Sb concentration (Figures 4B,D). Similar to As, the addition of Fe reduced the translocation of Sb from roots to shoots (decreased BCF value), and roots to grains (decreased TF2 value) (Supplementary Table S2). The significant increases in soil available Sb concentration agreed with the enhanced shoot Sb concentration (5 and 10 mg kg−1 Fe, Figure 4A). The unchanged Sb concentration in the DCB extracts (Figure 3A) indicated that root Fe/Mn plaques played a limited role in controlling Sb translocation at this moment. Similar results were obtained in other studies, where Fe plaques had no obvious effect on Sb uptake by wheat (Triticum aestivum L. Sella) (Ji et al., 2018).
It was also strange that the addition of FeCl3 showed a dose-dependent effect on the Cd concentration in the shoots, husks, and grains. For example, 10 mg kg−1 FeCl3 significantly increased the grain and shoot Cd concentrations (Figures 4A,D), and the other treatments containing FeCl3 either non-significantly affected or significantly reduced the Cd concentration in different tissues of this rice plants (Figures 4A–D). The above results suggested complicated physicochemical reactions plus biological effects of plant roots and microorganisms in soils. In this study, the addition of FeCl3 did not have negative effects on the concentrations of Mg, K, Ca, Mn, and Zn in the grains (Supplementary Table S3).
A Moderate Soil Moisture is Needed for Rice Plants Growing in Sb and Cd Co-contaminated Soils
In the Experiment II, a low soil moisture resulted in low DCB-As and DCB-Sb concentrations (Figure 3B), available As and Sb concentrations (Table 1), and total concentrations of As and Sb in all tissues of this rice plant (Figure 5). However, a low soil moisture at the same time increased Cd concentration in all tissues of rice plants (Figure 5) and decreased the concentrations of Mg, K, Ca, Fe, Mn, and Zn in the grains (Supplementary Table S4). The above results indicated that when growing rice plants in Sb and Cd co-contaminated soils, a moderate field drying strategy is necessary to control the accumulation of Cd and Sb in tissues of rice plants, because (1) a high soil moisture will enhance the risks of As accumulation in plants despite a very low As concentration in soils, which was in line with the results reported by Carrijo et al. (2019); (2) a low soil moisture will increase the risks of Cd accumulation in plant tissues (Figure 5); and (3) a low soil moisture is also not beneficial for the accumulation of essential elements in the grains, just proven in this study (Supplementary Table S4) and the study of Li et al. (2009).
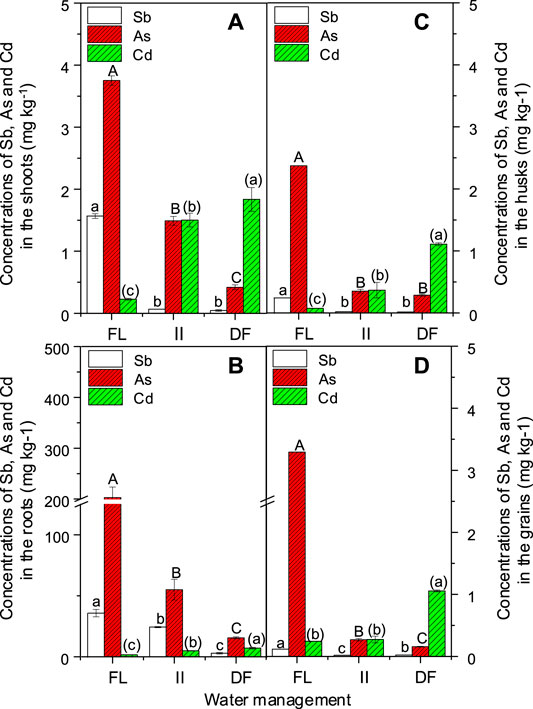
FIGURE 5. Effect of different water management on the concentrations of Sb, As and Cdshoots, roots, husks and grains. Bars are means and standard deviations for three replications. Different lowercase letters, capital letters, and parenthesized letters denote significant differences (at p ≤ 0.05) for Sb, As, and Cd concentrations in the shoots, roots, husks and grains among different treatments, respectively.
When using FeCl3 to remediate contaminated soils by Sb and Cd in natural fields, some aspects should be taken into consideration. In actual field conditions, the growth conditions for crops are hard to be controlled, especially for excess rain, fertilization, and cultivation patterns. (1) Excess rain will result in more release of Sb and As (just mentioned above) and elevate the difficulty of using passivators to immobilize As and Sb in soils. (2) The fertilizers containing phosphorus (P) will stimulate the release of As and Sb via competition adsorption (Cao and Ma., 2004), but will reduce Cd availability via a co-precipitation reaction (Raicevic et al., 2005). Therefore, it is important to supply phosphorus fertilizers during the process of using passivators to remediate As- and/or Sb-contaminated soils. (3) In practice, a crop rotation measure is often used to improve soil physicochemical properties and soil microorganism communities (N’Dayegamiye et al., 2015). A rotation measure (like a rotation of paddy rice and wheat) will help soil re-organize its soil texture, and affect adsorption/desorption of elements and speciation transformation of As and Sb in soils (Datta and Sarkar., 2004; He et al., 2018). When cultivating a contaminated farmland using a rotation pattern, FeCl3 can be used to reduce As, Sb, and Cd accumulation in grains of rice plants on the condition that the soil water will be well-controlled. Otherwise, an uncontrolled accumulation of As and Cd in grains of rice plants will very likely happen.
Conclusion
In this study, the dynamic changes in the concentrations of As, Sb, and Cd in the soil solution did not match well with their final available concentrations in the rhizosphere soils. After the addition of FeCl3, the unchanged available concentration of As in the rhizosphere soils was not in line with the significantly enhanced As concentration in most tissues of the rice plants. However, the significantly enhanced available Sb concentration and unchanged available Cd concentration in the rhizosphere soils generally agreed with the enhanced shoot Sb concentration and unchanged root Cd concentration, respectively. The long-term submergence resulted in the excessive accumulation of As in all tissues of this rice plant. The addition of FeCl3 could reduce the grain As, grain Sb, and husk Cd concentration in many cases. Different FeCl3 treatments stimulated the formation of Fe/Mn plaques, and the Fe/Mn plaques accumulated a large amount of As. However, the above accumulation did not produce a less accumulation of As in many plant tissues. In addition, the accumulation of Sb and Cd in the Fe/Mn plaques did not differ within different Fe treatments. Low soil moisture content was found to be beneficial to the formation of Mn plaque, which accumulated more Cd but less As and Sb with the decreased soil moisture content. In line with the changes of these three element concentrations in the Fe/Mn plaques, the low soil moisture led to a higher accumulation of Cd but a lower accumulation of As and Sb in all tissues of rice plants. In summary, when using strategies to reduce the uptake of Cd and Sb in rice plants, adding FeCl3 to the growth medium may pose risks when the plants are subjected to a continued submergence condition, including (1) the unexpected stimulation for As accumulation in rice plant tissues even when the As background concentration is low, and (2) decreased accumulation of many essential elements in the grains. In addition, the intermittent irrigation is the best way to simultaneously reduce the accumulation of As, Sb, and Cd in the grains of rice plants grown in the contaminated soils by Sb and Cd.
Data Availability Statement
The original contributions presented in the study are included in the article/Supplementary Material, Further inquiries can be directed to the corresponding author.
Author Contributions
RF and ZF postulated and supervised the study. QW planned the experiment, obtained the data, and carried out the data analysis and interpretation. JY prepared the first draft, and RF thoroughly revised the manuscript and provided financial support. RF, ZF, JY, and QW read and approved the final manuscript.
Funding
This study was financially supported by the National Science Foundation of China (41473114).
Conflict of Interest
The authors declare that the research was conducted in the absence of any commercial or financial relationships that could be construed as a potential conflict of interest.
Publisher’s Note
All claims expressed in this article are solely those of the authors and do not necessarily represent those of their affiliated organizations, or those of the publisher, the editors, and the reviewers. Any product that may be evaluated in this article, or claim that may be made by its manufacturer, is not guaranteed or endorsed by the publisher.
Supplementary Material
The Supplementary Material for this article can be found online at: https://www.frontiersin.org/articles/10.3389/fenvs.2021.780961/full#supplementary-material
References
Bingham, F. T., Page, A. L., Mahler, R. J., and Ganje, T. J. (1976). Cadmium Availability to Rice in Sludge-Amended Soil under "Flood" and "Nonflood" Culture. Soil Sci. Soc. America J. 40, 715–719. doi:10.2136/sssaj1976.03615995004000050030x
Biver, M., Krachler, M., and Shotyk, W. (2011). The Desorption of Antimony(V) from Sediments, Hydrous Oxides, and clay Minerals by Carbonate, Phosphate, Sulfate, Nitrate, and Chloride. J. Environ. Qual. 40, 1143–1152. doi:10.2134/jeq2010.0503
Cao, X., and Ma, L. Q. (2004). Effects of Compost and Phosphate on Plant Arsenic Accumulation from Soils Near Pressure-Treated wood. Environ. Pollut. 132 (3), 435–442. doi:10.1016/j.envpol.2004.05.019
Carrijo, D. R., Li, C., Parikh, S. J., and Linquist, B. A. (2019). Irrigation Management for Arsenic Mitigation in rice Grain: Timing and Severity of a Single Soil Drying. Sci. Total Environ. 649, 300–307. doi:10.1016/j.scitotenv.2018.08.216
Chou, M.-L., Jean, J.-S., Yang, C.-M., Hseu, Z.-Y., Chen, Y.-H., and Wang, H.-L. (2016). Inhibition of Ethylenediaminetetraacetic Acid Ferric Sodium Salt (EDTA–Fe) and Calciumperoxide (CaO2) on Arsenic Uptake by Vegetables in Arsenic–Rich Agricultural Soil. J. Geochem. Explor. 163,19–27. doi:10.1016/j.gexplo.2016.01.004
Cornu, J. Y., Denaix, L., Schneider, A., and Pellerin, S. (2008). Temporal Evolution of Redox Processes and Free Cd Dynamics in a Metal-Contaminated Soil after Rewetting. Chemosphere 70, 306–314. doi:10.1016/j.chemosphere.2007.06.003
Cui, X. D., Wang, Y. J., Hockrnann, K., and Zhou, D. M. (2015). Effect of Iron Plaque on Antimony Uptake by rice (Oryza Sativa L.). Environ. Pollut. 204, 133–140. doi:10.1016/j.envpol.2015.04.019
Datta, R., and Sarkar, D. (2004). Arsenic Geochemistry in Three Soils Contaminated with Sodium Arsenite Pesticide: An Incubation Study. Environ. Geosciences 11 (2), 87–97. doi:10.1306/eg.08220303013
DeLemos, J. L., Bostick, B. C., Renshaw, C. E., Sturup, S., and Feng, X. H. (2006). Landfill–stimulated Iron Reduction and Arsenic Release at the Coakley Superfund Site (NH). Environ. Sci. Technol. 40, 67–73. doi:10.1021/es051054h
Fawcett, S. E., Jamieson, H. E., Nordstrom, D. K., and McCleskey, R. B. (2015). Arsenic and Antimony Geochemistry of Mine Wastes, Associated Waters and Sediments at the Giant Mine, Yellowknife, Northwest Territories. Appl. Geochem. 62, 3–17. doi:10.1016/j.apgeochem.2014.12.012
Filella, M., Belzile, N., and Chen, Y. W. (2002). Antimony in the Environ Ment:a Review Focused on Natural Waters I .Occurrence. Earth –Sci.Rev . 57, 125–176. doi:10.1016/s0012-8252(01)00070-8
Frohne, T., Rinklebe, J., Diaz–Bone, R. A., and Laing, G. D. (2011). Controlled Variation of Redox Conditions in a Floodplain Soil: Impact on Metal Mobilization and Biomethylation of Arsenic and Antimony. Geoderma 160, 414–424. doi:10.1016/j.geoderma.2010.10.012
Fu, S., and Wei, C. Y. (2013). Multivariate and Spatial Analysis of Heavy Metal Sources and Variations in a Large Old Antimony Mine. J. Soils Sediments. 13, 106–116. doi:10.1007/s11368-012-0587-9
Fu, Z. Y., Wu, F. C., Amarasiriwardena, D., Mo, C. L., Liu, B. J., Zhu, J., et al. (2010). Antimony, Arsenic and Mercury in the Aquatic Environment and Fish in a Large Antimony Mining Area in Hunan. Sci. Total. Environ. 408, 3403–3410. doi:10.1016/j.scitotenv.2010.04.031
Fu, Z. Y., Wu, F. C., Mo, C. L., Deng, Q. J., Meng, W., and Giesy, J. P. (2016). Comparison of Arsenic and Antimony Biogeochemical Behavior in Water, Soil and Tailings from Xikuangshan. Sci. Total. Environ. 539, 97–104. doi:10.1016/j.scitotenv.2015.08.146
He, M., Wang, N., Long, X., Zhang, C., Ma, C., Zhong, Q., et al. (2018). Antimony Speciation in the Environment: Recent Advances in Understanding the Biogeochemical Processes and Ecological Effects. J. Environ. Sci. 75, 14–39. doi:10.1016/j.jes.2018.05.023
He, Z., Liu, R., Liu, H. J., and Qu, J. H. (2015). Adsorption of Sb(III) and Sb(V) on Freshly Prepared Ferric Hydroxide (FeOxHy). Environ. Eng. Sci. 32 (2), 95–102. doi:10.1089/ees.2014.0155
Hoagland, D. R., and Arnon, D. I. (1950). The Water–Culture Method for Growing Plants without Soil. Cal. Agric. Exp. Sta. Cir. 347, 1–32. doi:10.1016/S0140–6736(00)73482–9
Hu, P. J., Ouyang, Y. N., Wu, L. H., Shen, L. B., Luo, Y. M., and Christie, P. (2015). Effects of Water Management on Arsenic and Cadmium Speciation and Accumulation in an upland rice Cultivar. J. Environ. Sci. (China). 27, 225–231. doi:10.1016/j.jes.2014.05.048
Huang, G. X., Ding, C. F., Hu, Z. Y., Cui, C. H., Zhang, T. L., and Wang, X. X. (2018). Topdressing Iron Fertilizer Coupled with Pre–immobilization in Acidic Paddy fields Reduced Cadmium Uptake by rice (Oryza Sativa L.). Sci. Total Environ. 636, 1040–1047. doi:10.1016/j.scitotenv.2018.04.369
Huang, Y., Chen, Z., and Liu, W. (2012). Influence of Iron Plaque and Cultivars on Antimony Uptake by and Translocation in rice (Oryza Sativa L.) Seedlings Exposed to Sb(III) or Sb(V). Plant Soil 352, 41–49. doi:10.1007/s11104-011-0973-x
Huyen, D. T., Tabelin, C. B., Thuan, H. M., Dang, D. H., Truong, P. T., Vongphuthone, B., et al. (2019). The Solid-phase Partitioning of Arsenic in Unconsolidated Sediments of the Mekong Delta, Vietnam and its Modes of Release under Various Conditions. Chemosphere 233, 512–523. doi:10.1016/j.chemosphere.2019.05.235
Ji, Y., Vollenweider, P., Lenz, M., Schulin, R., and Tandy, S. (2018). Can Iron Plaque Affect Sb(III) and Sb(V) Uptake by Plants under Hydroponic Conditions. Environ. Exp. Bot. 148, 168–175. doi:10.1016/j.envexpbot.2018.01.014
Karimian, N., Burton, E. D., and Johnston, E. S. (2019). Antimony Speciation and Mobility during Fe(II)–induced Transformation of Humic acid–Antimony(V)–Iron(III) Coprecipitates. Environ. Pollut. 253, 113112. doi:10.1016/j.envpol.2019.113112
Ko, M. S., Kim, J. Y., Park, H. S., and Kim, K. W. (2015). Field Assessment of Arsenic Immobilization in Soil Amended with Iron Rich Acid Mine Drainage Sludge. J. Clean. Prod. 108, 1073–1080. doi:10.1016/j.jclepro.2015.06.076
Leverett, P., Reynolds, J. K., Roper, A. J., and Williams, P. A. (2012). Tripuhyite and Schafarzikite: Two of the Ultimate Sinks for Antimony in the Natural Environment. Mineral. Mag. 76, 891–902. doi:10.1180/minmag.2012.076.4.06
Li, J. R., and Xu, Y. M. (2017). Immobilization Remediation of Cd–Polluted Soil with Different Water Condition. J. Environ. Manage. 193, 607–612. doi:10.1016/j.jenvman.2017.02.064
Li, R. M., and Hou, C. T. (1998). Research of Soil Solution in Agroecological Geology. Acta Geosicientia Sinica 4, 434–437.
Li, R., Zhou, Z., Zhang, Y., Xie, X., Li, Y., and Shen, X. (2015). Uptake and Accumulation Characteristics of Arsenic and Iron Plaque in Rice at Different Growth Stages. Commun. Soil Sci. Plan. 46, 2509–2522. doi:10.1080/00103624.2015.1089259
Li, S. X., Wang, Z. H., Malhi, S. S., Li, S. Q., Gao, Y. J., and Tian, X. H. (2009). Nutrient and Water Management Effects on Crop Production, and Nutrient and Water Use Efficiency in Dryland Areas of china. Adv. Agron. 102, 223–265. doi:10.1016/S0065-2113(09)01007-4
Li, Y. Y., Zhao, J. T., Zhang, B. W., Liu, Y. J., Xu, X. H., Li, Y. F., et al. (2016). The Influence of Iron Plaque on the Absorption, Translocation and Transformation of Mercury in rice (Oryza Sativa L.) Seedlings Exposed to Different Mercury Species. Plant Soil 398, 87–97. doi:10.1007/s11104-015-2627-x
Liao, G. J., Wu, Q. H., Feng, R. W., Guo, J. K., Wang, R. G., Xu, Y. M., et al. (2016). Efficiency Evaluation for Remediating Paddy Soil Contaminated with Cadmium and Arsenic Using Water Management, Variety Screening and Foliage Dressing Technologies. J. Environ. Manage. 170, 116–122. doi:10.1016/j.jenvman.2016.01.008
Liu, H. J., Zhang, J. L., Christie, P., and Zhang, F. S. (2007). Influence of External Zinc and Phosphorus Supply on Cd Uptake by rice (Oryza Sativa L.) Seedlings with Root Surface Iron Plaque. Plant Soil 300, 105–115. doi:10.1007/s11104-007-9393-3
Liu, W. J., Zhu, Y. G., and Smith, F. A. (2005). Effects of Iron and Manganese Plaques on Arsenic Uptake by rice Seedlings (Oryza Sativa L.) Grown in Solution Culture Supplied with Arsenate and Arsenite. Plant Soil 277, 127–138. doi:10.1007/s11104-005-6453-4
Liu, W. X., Wang, B. B., Wang, Q. L., Hou, J. Y., Wu, L. H., Wood, J. L., et al. (2016). Characteristics of Metal–Tolerant Plant Growth–Promoting Yeast (Cryptococcus Sp NSE1) and its Influence on Cd Hyperaccumulator Sedum Plumbizincicola. Environ. Sci. Pollut. R. 23, 18621–18629. doi:10.1007/s11356-016-7041-2
Liu, Y., Lv, H. Q., Yang, N., Li, Y. P., Liu, B. X., Feng, R. W., et al. (2019). Roles of Root Cell wall Components and Root Plaques in Regulating Elemental Uptake in rice Subjected to Selenite and Different Speciation of Antimony. Environ. Exp. Bot. 163, 36–44. doi:10.1016/j.envexpbot.2019.04.005
Macgregor, K., MacKinnon, G., Farmer, J. G., and Graham, M. C. (2015). Mobility of Antimony, Arsenic and lead at a Former Antimony Mine. Glendinning. Sci. Total Environ. 529, 213–222. doi:10.1016/j.scitotenv.2015.04.039
Makino, T., Nakamura, K., Katou, H., Ishikawa, S., Ito, M., Honma, T., et al. (2016). Simultaneous Decrease of Arsenic and Cadmium in rice (Oryza Sativa L.) Plants Cultivated under Submerged Field Conditions by the Application of Iron–Bearing Materials. Soil Sci. Plant Nutr. 62, 340–348. doi:10.1080/00380768.2016.1203731
Maneepitak, S., Ullah, H., Paothong, K., Kachenchart, B., Datta, A., and Shrestha, R. P. (2019). Effect of Water and rice Straw Management Practices on Yield and Water Productivity of Irrigated lowland rice in the Central Plain of Thailand. Agr. Water Manage. 211, 89–97. doi:10.1016/j.agwat.2018.09.041
Mao, C. P., Song, Y. X., Chen, L. X., Ji, J. F., Li, J. Z., Yuan, X. Y., et al. (2019). Human Health Risks of Heavy Metals in Paddy rice Based on Transfer Characteristics of Heavy Metals from Soil to rice. Catena 175, 339–348. doi:10.1016/j.catena.2018.12.029
Matsumoto, S., Kasuga, J., Makino, T., and Arao, T. (2016). Evaluation of the Effects of Application of Iron Materials on the Accumulation and Speciation of Arsenic in rice Grain Grown on Uncontaminated Soil with Relatively High Levels of Arsenic. Environ. Exp. Bot. 125, 42–51. doi:10.1016/j.envexpbot.2016.02.002
McFarlane, N. M., Ciavarella, T. A., and Smith, K. F. (2003). The Effects of Waterlogging on Growth: Photosynthesis and Biomass Allocation in Perennial Ryegrass (Lolium Perenne L.) Genotypes with Contrasting Root Development. Agric. Sci. 141, 241–248. doi:10.1017/S0021859603003502
Mitsunobu, S., Harada, T., and Takahashi, Y. (2006). Comparison of Antimony Behavior with that of Arsenic under Various Soil Redox Conditions. Environ.Sci. Tech. 40, 7270–7276. doi:10.1021/es060694x
N’Dayegamiye, A., Whalen, J. K., Tremblay, G., Nyiraneza, J., Grenier, M., Drapeau, A., et al. (2015). The Benefits of Legume Crops on Corn and Wheat Yield, Nitrogen Nutrition, and Soil Properties Improvement. Agron. J. 107 (5), 1653. doi:10.2134/agronj14.0416
Otones, V., Álvarez-Ayuso, E., García-Sánchez, A., Santa Regina, I., and Murciego, A. (2011). Arsenic Distribution in Soils and Plants of an Arsenic Impacted Former Mining Area. Environ. Pollut. 159 (10), 2637–2647. doi:10.1016/j.envpol.2011.05.027
Pittman, J. K. (2005). Managing the Manganese: Molecular Mechanisms of Manganese Transport and Homeostasis. New Phytol. 167 (3), 733–742. doi:10.1111/j.1469-8137.2005.01453.x
Raicevic, S., Kaludjerovic-Radoicic, T., and Zouboulis, A. I. (2005). In Situ stabilization of Toxic Metals in Polluted Soils Using Phosphates: Theoretical Prediction and Experimental Verification. J. Hazard. Mater. 117 (1), 41–53. doi:10.1016/j.jhazmat.2004.07.024
Ren, J. H., Ma, L. Q., Sun, H. J., Cai, F., and Luo, J. (2014). Antimony Uptake, Translocation and Speciation in rice Plants Exposed to Antimonite and Antimonate. Sci. Total Environ. 475, 83–89. doi:10.1016/j.scitotenv.2013.12.103
Ryden, J. C., Syers, J. K., and Tillman, R. W. (1987). Inorganic Anion Sorption and Interactions with Phosphate Sorption by Hydrous Ferric Oxide Gel. Eur. J. Soil Sci. 38, 211–217. doi:10.1111/j.1365-2389.1987.tb02138.x
Simmons, R. W., Noble, A. D., Pongsakul, P., Sukreeyapongse, O., and Chinabut, N. (2008). Analysis of Field–Moist Cd Contaminated Paddy Soils during rice Grain Fill Allows Reliable Prediction of Grain Cd Levels. Plant Soil 302, 125–137. doi:10.1007/s11104–007–9460–910.1007/s11104-007-9460-9
Suriyagoda, L., Dittert, K., and Lambers, H. (2018). Arsenic in rice Soils and Potential Agronomic Mitigation Strategies to Reduce Arsenic Bioavailability: a Review. Pedosphere 28, 3–22. doi:10.1016/s1002-0160(18)60026-8
Tabelin, C. B., Igarashi, T., Villacorte-Tabelin, M., Park, I., Opiso, E. M., Ito, M., et al. (2018). Arsenic, Selenium, boron, lead, Cadmium, Copper, and Zinc in Naturally Contaminated Rocks: A Review of Their Sources, Modes of Enrichment, Mechanisms of Release, and Mitigation Strategies. Sci. Total Environ. 645, 1522–1553. doi:10.1016/j.scitotenv.2018.07.103
Tabelin, C. B., Park, I., Phengsaart, T., Jeon, S., Villacorte-Tabelin, M., Alonzo, D., et al. (2021). Copper and Critical Metals Production from Porphyry Ores and E-Wastes: A Review of Resource Availability, Processing/recycling Challenges, Socio-Environmental Aspects, and Sustainability Issues. Resour. Conserv. Recycl. 170, 105610. doi:10.1016/j.resconrec.2021.105610
Takahashi, Y., Minamikawa, R., Hattori, K. H., Kurishima, K., Kihou, N., and Yuita, K. (2004). Arsenic Behavior in Paddy Fields during the Cycle of Flooded and Non–flooded Periods. Environ. Sci. Technol. 38, 1038–1044. doi:10.1021/es034383n
Takarina, N. D., and Pin, T. G. (2017). Bioconcentration Factor (BCF) and Translocation Factor (TF) of Heavy Metals in Mangrove Trees of Blanakan Fish Farm. Makara J. Sci. 21 (2). doi:10.7454/mss.v21i2.7308
Tandy, S., Meier, N., and Schulin, R. (2017). Use of Soil Amendments to Immobilize Antimony and lead in Moderately Contaminated Shooting Range Soils. J. Hazard. Mater. 324, 617–625. doi:10.1016/j.jhazmat.2016.11.034
Van Caneghem, J., Verbinnen, B., Cornelis, G., de Wijs, J., Mulder, R., Billen, P., et al. (2016). Immobilization of Antimony in Waste–To–Energy Bottom Ash by Addition of Calcium and Iron Containing Additives. Waste Manage 54, 162–168. doi:10.1016/j.wasman.2016.05.007
Wan, X. M., Tandy, S., Hockmann, K., and Schulin, R. (2013). Changes in Sb Speciation with Waterlogging of Shooting Range Soils and Impacts on Plant Uptake. Environ. Pollut. 172, 53–60. doi:10.1016/j.envpol.2012.08.010
Wu, Q. H., Feng, R. W., Guo, J. K., Wang, R. G., Xu, Y. M., Fan, Z. L., et al. (2017). Interactions between Selenite and Different Forms of Antimony and Their Effects on Root Morphology of Paddy rice. Plant Soil 413, 231–242. doi:10.1007/s11104-016-3096-6
Xu, X. Y., McGrath, S. P., Mehang, A. A., and Zhao, F. J. (2008). Growing rice Aerobically Markedly Decreases Arsenic Accumulation. Environ. Sci. Technol. 42, 5574–5579. doi:10.1021/es800324u
Yao, F. X., Huang, J. L., Cui, K. H., Nie, L. X., Xiang, J., Liu, X. J., et al. (2012). Agronomic Performance of High–Yielding rice Variety Grown under Alternate Wetting and Drying Irrigation. Field Crops Res. 126, 16–22. doi:10.1016/j.fcr.2011.09.018
Ye, Z. H., Cheung, K. C., and Wong, M. H. (2003). Cadmium and Nickel Adsorption and Uptake in Cattail as Affected by Iron and Manganese Plaque on the Root Surface. Commun. Soil Sci. Plan. 34, 2763–2778. doi:10.1081/css-120025202
Yuan, Y., Chai, L., Yang, Z., and Yang, W. (2017). Simultaneous Immobilization of lead, Cadmium, and Arsenic in Combined Contaminated Soil with Iron Hydroxyl Phosphate. J. Soils Sediments. 17, 432–439. doi:10.1007/s11368-016-1540-0
Zheng, H., Wang, M., Chen, S. B., Li, S. S., and Lei, X. Q. (2019). Sulfur Application Modifies Cadmium Availability and Transfer in the Soil–rice System under Unstable pe+pH Conditions. Ecotoxicol. Environ. Saf. 184, 109641. doi:10.1016/j.ecoenv.2019.109641
Zhu, Y. M., Yang, J. G., Wang, L. Z., Lin, Z. T., Dai, J. X., Wang, R. J., et al. (2020). Factors Influencing the Uptake and Speciation Transformation of Antimony in the Soil–Plant System, and the Redistribution and Toxicity of Antimony in Plants. Sci. Total Environ. 738, 140–232. doi:10.1016/j.scitotenv.2020.140232
Keywords: dynamic change, arsenic, multiple metal(loid) contamination, soil solution, FeCl3, water management
Citation: Yang J, Wu Q, Fan Z and Feng R (2021) Risks for Using FeCl3 Under a Submerged Condition, and Different Water Management to Reduce Uptake of Antimony and Cadmium in a Rice Plant. Front. Environ. Sci. 9:780961. doi: 10.3389/fenvs.2021.780961
Received: 22 September 2021; Accepted: 22 November 2021;
Published: 23 December 2021.
Edited by:
Rosa Francaviglia, Council for Agricultural and Economics Research (CREA), ItalyReviewed by:
Carlito Baltazar Tabelin, University of New South Wales, AustraliaAgnieszka Klimkowicz-Pawlas, Institute of Soil Science and Plant Cultivation, Poland
Copyright © 2021 Yang, Wu, Fan and Feng. This is an open-access article distributed under the terms of the Creative Commons Attribution License (CC BY). The use, distribution or reproduction in other forums is permitted, provided the original author(s) and the copyright owner(s) are credited and that the original publication in this journal is cited, in accordance with accepted academic practice. No use, distribution or reproduction is permitted which does not comply with these terms.
*Correspondence: RenWei Feng, frwzym@aliyun.com
†These authors have contributed equally to this work