- 1African Sustainable Agriculture Research Institute (ASARI), Mohammed VI Polytechnic University (UM6P), Laâyoune-Morocco, Morocco
- 2AgroBioSciences Department, Mohammed VI Polytechnic University (UM6P), Ben Guerir, Morocco
- 3Department of Biology, Bahir Dar University, Bahir Dar, Ethiopia
- 4Institut National de la Recherche Agronomique (INRA), Rabat, Morocco
- 5Laboratory of Microbial Biotechnologies Agrosciences and Environment (BioMAgE), Labelled Research Unit CNRST, Faculty of Sciences Semlalia, Cadi Ayyad University (UCA), Marrakech, Morocco
The proliferation of the invasive Water hyacinth (WH) plant leads to ecological, economic, public health, and agricultural problems. Several efforts have been deployed to control its spread, but no concreate results have been obtained. Only few studies dealing with systematic approaches for the WH control have been conducted. To establish a road map for the best control methods to be adopted, this review highlights the control programs that have been tested worldwide and describes, through a deep literature analysis and comparison, the most effective and sustainable control programs for managing the proliferation of this aquatic weed. Through a critical analysis, this review evaluates the advantages and drawbacks of the main proposed control methods including biological, chemical and physical methods. The obtained results suggested that short and medium term physical control promptly manages the plant’s proliferation and thus could complement the effect of the biological control. Moreover, to be economically viable, the harvested WH through physical means must be valorized to generate high value-added products. Furthermore, run-off nutrients control could reduce the end-of-catchment loads and would help the resilience of freshwater bodies and promote plant removal. Descriptive results analysis confirmed that an integrated control approach combining “biological and physical’’ is the most sustainable and cost-effective approach. The adaptation of these methods based on the socio-economic context of each country, could promote ecosystem restoration, self-generation, and conservation for a sustainable development.
Highlights
- Biological and physical mono-controls of WH were the most used methods worldwide.
- Mono-control approach of WH is not effective.
- Integrated Physical-Biological control (IPB) is the most used and cost-effective program worldwide.
- Integrated “Control-Valorization’’ approach is strongly recommended.
Introduction
Water hyacinth (Eichhornia crassipes) (Figure 1) is commonly considered the most harmful aquatic weed in the world. The first appearance of the species dates back to 1816 in Brazil. Subsequently, it was introduced as an ornamental plant to America at the end of the 1800s (Brown et al., 2020). Its appearance in Africa was in the early 1900s, and in Europe in the 1930s (Yan et al., 2016). In Africa, WH has substantially invaded Lake Tana (Ethiopia), the largest freshwater body in the country with 3,074 km2 surface area, thereby, negatively affecting the aquatic ecosystem. A study conducted by Dersseh et al. (2019), predicted the maximum invasion area of 30,728 ha in 2020, representing about 10% of the total surface of the Lake.
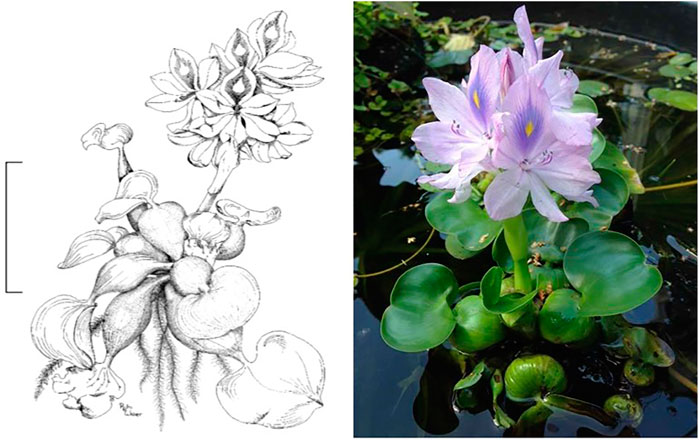
FIGURE 1. Water hyacinth, Eichhornia crassipes (Martius) Solms-Laubach (Drawn by Rita Weber, SANBI). Scale bar = 10 cm.
Water hyacinth is recognized by its fast-spreading rate, it was reported that 2 plants can multiply to 1,200 plants in 120 days, which allows the plant to cover large water surfaces in a short period of time (Ajithram et al., 2020). The spread of WH and its colonization of other areas, can also increase by strong winds or high waves. WH grows rapidly in highly acidic and alkaline water, but its growth is slightly observed in neutral water as well. One of the main causes of its huge proliferation is water enrichment through nutrient runoff from agricultural and human wastes (Villamagna and Murphy, 2010). It consumes high amounts of nitrogen and phosphorus under optimum growing conditions (Krisnawati et al., 2020). Nutrient concentrations and temperature (of air and water) are considered the strongest determinants for WH growth and reproduction (Wilson et al., 2005).
Water hyacinth is considered as an invasive plant species and represents various ecological and economic negative impacts (Figure 2). It causes deleterious damage to biodiversity, freshwater ecosystems, and native species (Tewabe and Asmare, 2020). The plant forms dense mats that prevent the penetration of light into the water, thereby, decreasing its temperature, which and inhibiting plant photosynthesis (Wilson et al., 2005). The fast distribution of WH results in the occurrence of sedimentation and causes a siltation phenomenon in water bodies (Ratnani et al., 2020). If its growth is not controlled properly, WH could have severe environmental problems.
Therefore, these plant nuisances have led to the implementation of several control programs in all fields (physical, chemical, biological). Physical control (PC) consists of directly removing the plant by hand or with appropriate equipment, while the chemical control (CC) involves the use of herbicides, and biological control (BC) means the intentional use of natural enemies of the target invasive species. These methods can be used solely or in combined (George and Goldman, 2007).
Neochetina spp. weevils have been successfully used as biocontrol agents in several countries around the world (Julien, 2001). The most limiting factor for using this type of controls is the need for a long time to achieve high performance (Abtew and Dessu, 2019). Chemical control using herbicides is very efficient to control WH (Villamagna and Murphy, 2010), but, it is unfortunately, harmful for the environment. The development of pesticide-resistant weeds may reduce its effectiveness (Kyser et al., 2021). Physical control, however, is the most ecofriendly method to remove WH from water bodies, but requires high investment (Malik, 2007). To overcome the high cost of the physical control, it is strongly recommended to valorize the recovered biomass (Mathew et al., 2015; Hernández-Shek et al., 2016; Priya et al., 2018).
Despite its high negative impact on the aquatic ecosystem, the WH presents diverse potential financial benefits (Figure 2). The plant is famous for many potential industrial applications. It could be used as a phytoremediation agent because of its ability to grow in wastewater and its capacity to accumulate metals, radionuclides, nanoparticles, and other pollutants (Lazim et al., 2020). Numerous studies on its capacity to purify domestic wastewater have been carried out. A study conducted by Rezania et al. (2016), proved that the plant could treat successfully domestic wastewater, and improve its purification efficiency to 96% of nutrient removal. The plant can also be used to produce valuable products such as biopolymers fibers and composite. The biodegradable fibers of WH have proven to be an excellent way to treat wastewater via adsorption as compared to other expensive industrial technics (Arumugam et al., 2018). In addition, WH has been considered as a source of biofertilizers, animal feed and bioenergy (Jafari, 2010). It also exhibits robust biological activities including antioxidant, antimicrobial, antitumor, anti-aging, wound healing, anti-inflammatory, anticancer and antidiabetic (Kiristos et al., 2018; Sharma et al., 2020). Furthermore, WH biomass produces other valuables products such as enzymes and capacitors, and is used as a raw material to make furniture and handicrafts (Patel, 2012).
Given the complexity of the ecological system where the WH is proliferating as well as the sensibility of its habitat, the most suitable control methods must be well identified. Few literature studies have been conducted to evaluate the effectiveness of WH controlling programs. All papers published in this field described only experiences that have been adopted by the countries that faced this invasion. No study has analyzed these experiences outputs in order to identify the most cost-effective control programs.
To establish a road map for the best control methods to be adopted, this review highlights the control programs that have been tested worldwide and describes, through a deep literature analysis and comparison, the most effective and sustainable control programs for managing the proliferation of this aquatic weed. Through a critical analysis, this review evaluates the advantages and drawbacks of the main proposed control methods including biological, chemical and physical methods. In addition, this paper reviewed the most recent WH valorization pathways.
Methodology
An extensive search was conducted based on Scopus and Web of Science. The scope of the control methods research focused on published papers from 1980 to 2022, while the valorization has focused only on recent articles from 2016 to 2022. The following keywords were used: (water and hyacinth) and (control and methods); (water and hyacinth) and (biological and control); (water and hyacinth) and (chemical and control); (water and hyacinth) and (physical and control); (water and hyacinth) and (integrated and control); (water and hyacinth) and (run off and nutrients); (water and hyacinth) and (cost and benefits); (water and hyacinth) and (valorization). As a results, 990 sources of literature were identified from which 345 duplicates were removed and 105 were selected and analyzed. The screening process is described in Figure 3.
Evolution of the Articles and Citations Over the Years
Figure 4 shows the annual scientific production and citations related to WH control methods. The research is considered relatively old and started 50 years ago. In fact, the first paper in this research area was published in 1974. The number of published research papers and citations have fluctuated over the last 2 decades, reaching a peak of 26 paper in 2020. This leads us to infer that this rise in the number of articles and citations over the years represents an increasing interest in this research area.
Geographical Distribution of Articles
Our screening process reveals that accessible published research on the WH control methods in the world, is concentrated in India (9 articles), followed by South Africa (8 articles), and China (5 articles) (Figure 5). These three countries together published significant number of research papers in the past 2 decades.
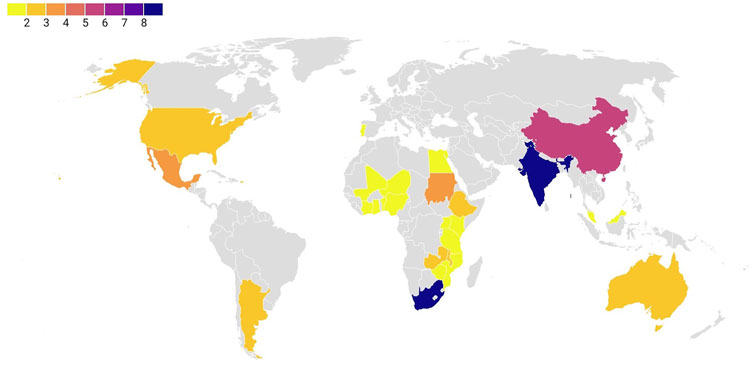
FIGURE 5. Number of published research articles worldwide. Numbers in caption indicate the number of research articles published in the field of WH control. India and South Africa were the most frequently studied countries, followed by China.
Water Hyacinth Control Methods
Nutrients drainage from agricultural and human activities towards water resources contributes to the rapid proliferation of WH. Hence, the first critical step for the management of WH would be the implementation of large-scale nutrient reduction solutions before eradication in order to minimize the amount of biomass to be removed. The main objective of this approach is to reduce the vegetation density and generate biomass to be used for the production of high value-added products.
The WH eradication consists of using chemical, physical (mechanical and manual removal), biological control and/or an integrated approach (Figure 6). However, no single method is suitable for all the infested area (Ajithram et al., 2020), and each method has its one limitations. The particularity of the infested area (size, spatial configuration, weather, water body uses, and the chronology of infestation) is one of the key elements for choosing a control method (Tewabe and Asmare, 2020).
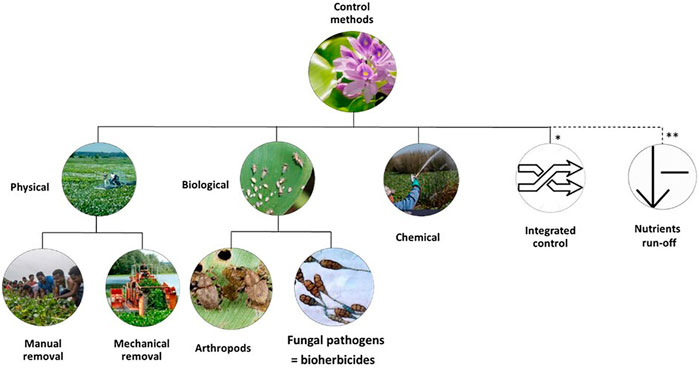
FIGURE 6. Controlling mechanisms of water hyacinth. * Integrated control is the combination of two or more than one control method: Integrated biological and physical (IBP); Integrated biological and chemical (IBC); Integrated physical and chemical (IPC); Integrated physical, biological, and chemical (IPBC). ** Reduction of nutrient run-of, could be used with all kinds of control methods.
Nutrient’s Run-off
Declining freshwater quality, through land-based run-off, is recognized as one of the most significant threats to the long-term health of freshwater areas. Nutrient run-off, along with sediment run-off and pesticides, is particularly crucial in many freshwater bodies. Nutrient run-off is associated with algae and WH blooms, and changes in microorganism species, which pose a risk to aquatic organisms (Emirhüseyinoğlu and Ryan, 2019).
Lake Victoria, the world’s second largest freshwater Lake, suffered from the high growth of WH. Soil erosion, nutrient runoff, as well as urban and industrial pollution have induced a rapid eutrophication of the Lake over the last 50 years. High levels of nutrients in the Lake caused a dramatic invasion of WH, considering that eutrophication is the main cause of the plant growth (Kaur et al., 2018). Reducing the loss of nutrients, sediments and pesticides surrounding freshwater bodies by managing the use of agricultural amendments, and by avoiding deforestation could reduce the end-of-catchment loads and enhance the resilience of freshwater bodies (Sharpley et al., 2006).
Drainage through agricultural soils can lead to the leaching of soluble nutrients and pesticides, which they could find their way to reach downstream waters as well as groundwater. Although nitrogen and phosphorus occur naturally, increased nitrogen and phosphorus from fertilizer leaching is harmful to the freshwater bodies and promote the proliferation of WH (George and Goldman, 2007).
The best way to manage the WH long-term effects is to better match nitrogen and phosphorus inputs to crop needs (Swallow et al., 2001). Furthermore, control of organic pollution can be also done by substituting synthetic chemicals fertilizers with organic fertilizers (Krisnawati et al., 2020). By optimizing nutrient application there is less chance of surplus nutrients being lost to waterways, where they can harm the environment, and increase the proliferation of WH.
Physical Control of WH
Physical methods consist of two kinds of control: manual and mechanical removal. Manual removal is done by human, to remove the WH plants from the water bodies (Ajithram et al., 2020), while the mechanical removal method is carried out by machines. Mechanical removal of WH consists of using weed cutters, harvesters, chaining, shredder boats, and dredging process. Mowing, netting, and barriers are also used as ways of mechanical removal. Barriers in the form of booms or cables have been effectively used in some countries such as Ivory Coast, Zambia, and South Africa to protect hydropower water intake pumps and water extraction pumps (Hill and Coetzee, 2008).
Physical control remains the only method through which WH can be transformed into value-added products. It allows the collection of the raw material and its potential valorization to compost or green energy and other uses. Physical control immediately opens physical space (habitat) for fish, boat traffic, fishing and recreation (Yigermal and Assefa, 2019). A study on mechanical harvesting of WH from an Australian lagoon has allowed an increase in dissolved oxygen, which was related to a potential increase of the water inflow (Perna and Burrows, 2005). Thus, physical control is the most suitable method to remove this plant, for economic and commercial benefits. Hand removal is most effective for small infestations while mechanical harvesting can be an effective tool for removing larger infestations. However, mechanical shredding and in-situ cutting processes, where plants are left to die and decompose in the water, can decrease dissolved oxygen in the water, and then catalyze the releases of phosphorus from the sediment. This process accelerates eutrophication phenomena, and can lead to a subsequent increase in WH or algae blooms (Mitan and Merry, 2019). A previous study carried out in laboratory using a water column, designed to mimic nutrient conditions of Lake Chivero (Uganda) reported that total nitrogen and phosphorus levels increased following mechanical (shredding) control of WH. Increased nutrient concentrations may be attributed to a release of these nutrients from decomposing plants (Villamagna and Murphy, 2010). Therefore, to overcome this eutrophication problem, the mechanical control must be associated with an integrated approach of valorization to produce biofertilizers and/or bioenergy from the plant (Tewabe and Asmare, 2020).
Chemical Control
Chemical control is an immediate and short time solution to remove WH using chemical herbicides. Chemical control has been used in several countries including Ghana, Nigeria, South Africa, Zambia, and Zimbabwe. The most effective and cheap herbicides used to reduce WH spread are glyphosate (Isopropilamine salt of N-phosphomethyl glycine), diquat (6,7-dihydrodipyridol pyrazinediumon) and 2,4-D (2,4-dichlorophenoxy) (Güereña et al., 2015).
Herbicides are very efficient to control WH (Villamagna and Murphy, 2010). However, they can affect and damage the aquatic system. It is considered harmful for the environment compared to the mechanical and the BC approaches (Ajithram et al., 2020). Furthermore, this method may lead to significant socioeconomic impacts if the quality of the freshwater is affected (Villamagna and Murphy 2010). For this reason, in many countries there are restrictions that prohibit the use of chemicals in water, intended for drinking purposes.
Using herbicides can be considered only under certain conditions such as in case of small-scale infestations and in emergency situations. Tewabe and Asmare (2020) reported that herbicides such as 2,4-D, diquat, paraquat, and glyphosate have resulted in successful control in small, single-purpose water systems such as irrigation canals and dams of around 1 ha in size. However, Souza et al. (2020) reported that the use of glyphosate herbicide at its highest recommended dose of 7.0 L ha−1 or 3,360 g of acid equivalent per ha, showed a low potential of environmental impact in the control of WH in reservoirs, but it should be used properly in specific infested sites and never in overall application to the whole infested area. In addition, an integrated approach with other control methods is recommended and seems to be unavoidable in certain circumstances. For example, interactions between chemical and BCs have been demonstrated. Previous studies reported that herbicides can complement BC (Cilliers et al., 2002; Perna and Burrows, 2005; Villamagna and Murphy, 2010). Paraquat, glyphosate and 2,4-D have been used to enhance the effects of Neochetina spp. species. Herbicides reduce WH populations to low levels prior to the introduction of biocontrol agents, thereby giving the agents time to better establish itself and suppress the regrowth of the weed. Moreover, creating barriers where WH can accumulate as whole block makes herbicidal control more effective. Combining mechanical and chemical control methods is a good option that saves time and energy following small or individual plants targeted for spraying (Cilliers et al., 2002; Perna and Burrows, 2005; Villamagna and Murphy, 2010).
A recent experimental study carried out on the effect of different concentrations of acetic acid on WH, aquatic life, and the physicochemical properties of water under pond conditions on the shores of Lake Tana in Ethiopia showed that increasing acetic acid concentrations gradually by 5, 10, 15 and 20% removed WH. However, it did not affect the survival of Nile tilapia but reduced water quality. Spraying a 20% concentration of acetic acid caused complete damage to the WH, while 15 and 10% concentrations allowed the development of new daughter plants and requires follow-up spraying, whereas 5% was less effective (Birhanie et al., 2020). Table 1 summarizes the world’s most used herbicides against WH, their principles, effectiveness, duration, doses, pretreatment methods, cost, advantages, and drawbacks.
Biological Control
Biological control is also one of the processes employed to remove or stop the growth of WH plants. In this method, some organisms including insects, pathogenic bacteria, fungi and parasites, among others were used (Yigermal and Assefa, 2019). The aim of any BC is not to eradicate the weed, but to reduce its abundance to a level where it is no longer problematic.
Biological Agents Used to Control of WH
Five insects (two weevils, two moths and a sucking bug), and a mite are among the most used arthropods worldwide. Microorganisms such as fungus have been also applied as control bioagents (Table 2). The most enemy species used are Neochetina eichhorniae and Neochetina bruchi weevils (Figure 7). Neochetina weevils can have devastating effects on WH populations leading to reduction of the plant by more than 95% (Jayanth, 1988). Adult weevils feed on the external plant surfaces and petiole reducing photosynthetic capabilities and productivity of the plant (Julien, 2001).
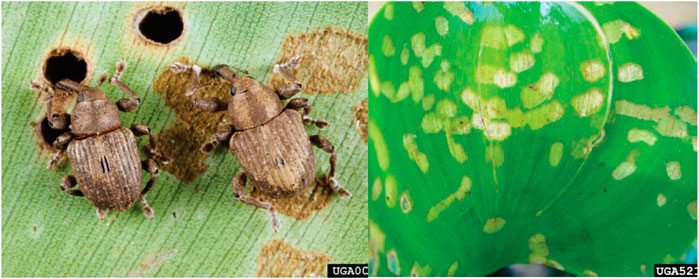
FIGURE 7. Adult Neochetina spp. feeding scars on water hyacinth (Photo credit Willey Durde and Katherine Parys, USDA-ARS, Bugwood.org).
Biological control was considered the only viable method for reducing the impact of WH on Lake Victoria (Kenya-Rwanda-Uganda), where two weevils Neochetina eichhorniae Warner and Neochetina bruchi Hustache (Coleoptera, Curculionidae) were used. Several million weevils individuals were released in the Lake via weevil-rearing stations resulting in a drastic collapse of the plant’s extensive mats (Cilliers et al., 2002).
Agjee et al. (2016) reported that Neochetina spp. is effective to overcome the proliferation of WH in South Africa. Weevils induced both morphological and physiological damage to WH. Adult weevils feed by forming rectangular scars on the surface of the leaf, thus exposing the leaf to desiccation (Agjee et al., 2016). Over time, extensive feeding damage causes a reduction in plant growth and reproduction (Agjee et al., 2016). According to Tewabe, (2015) Neochetina bruchi populations grow better under eutrophic conditions. However, Neochetina eichhorniae is less dependent on good quality plants for growth than N. bruchi. Consequently, the relative abundance varies between sites: more N. eichhorniae at sites, lower quality of WH is observed, and vice versa. In polluted waterways, N. bruchi may complement the damage caused by N. eichhorniae.
Neochetina spp. has been successfully used as biocontrol agents in several countries around the world. Neochetina bruchi has been released alone or with Neochetina eichhorniae in 30 countries, and Neochetina eichhorniae was released in another 32 countries (Julien, 2001). Table 3 shows examples of biocontrol at large and laboratory scales with details on the bioagents and the individual numbers used, the seasons, the control periods and effectiveness of the control.
Because of the WH invasion problem in the biggest Lake in Ethiopia (Lake Tana), where the proliferation is increasing rapidly, a previous study was conducted to predict the possibility of applying BC with the two Neochetina weevils using a simulation model (Firehun et al., 2015). The authors evaluated the climatic similarities between Rift Valley (Ethiopia) and some other locations in West Africa, where biocontrol of WH using both weevils has been successfully used. The study results showed that Neochetina bruchi could be considered as a promising candidate for controlling the WH under the Ethiopian climate conditions. The country is currently, trying at the experimental level (greenhouse) to control the weed using Neochetina bruchi and fungi (Rhizoctonia solani, Aspergillus flavus, Tricothcium roseum and Aspergillus niger). The biocontrol experiments have shown a high efficiency in controlling the weed (Yigermal and Assefa, 2019; Admas et al., 2020).
The most limiting factor of using the BC of WH is the long time required to achieve high performances (the process usually requires 2–4 years). Biocontrol methods can have inadequate results when there are factors affecting weevil growth such as weather, disease, predators, plant quality (host quality), and interferences after herbicide application. Consequently, it is mandatory to assess the infestation rate of weevil over time in order to ensure the process efficacy (Hauptfleisch, 2015).
Suitable Conditions and Limitations of Biocontrol
The biological control should be conducted in areas with subtropical or tropical climate because the low temperature reduces or stop weevil population increase and allow the weed to recover. It is effective on floating plants not on those retained in the mud beneath the water by the roots, and it is more successful in larger water bodies where wind and wave action increases the mortality of agent-stressed plants. In addition, the stable mats for long periods contribute to the multiplication of bioagents. Among biocontrol limitations, the cold winters, which increase the time for controlling the weed, and periodic or annual floods and drought which reduce the host plant leading to the high reductions of the weevil populations and their local extinction. Moreover, the high pollution, contamination by nutrients and concentrations of heavy metal pollutants reduce the host plant quality for bioagents establishment, because the uptake of heavy metals by WH may reduce the fecundity of the weevils that feed on those plants. Finally, biological control should not be combined with other control method in the same place and time, its interference from other controls notably inappropriate herbicide applications will directly or indirectly kill the bioagents by reducing the number of plants necessary for weevil’s growth and survival.
Integrated Control of WH
Integrated control is the use of two or three control methods together (Table 4). Its includes aspects of BC, herbicide applications, physical removal as well as the management of nutrients feeding the aquatic ecosystem (Van Wyk and Van Wilgen, 2002). It was reported that the key element of this approach is first the appointment of an organization to drive the control program, the involvement of all interested and affected parties, the division of the study area into management units, and finally, the application of the appropriate control methods. The control methods combination promotes weed stands reduction and growth of natural enemies for effective BC. Moreover, the use of combined control methods, in many cases, shows that it can be cost-effective than the application of only one control method in the entire study area (Hill and Coetzee, 2008). Table 4 summarizes the advantages and disadvantages of WH control combined methods.
Valorization of WH
After WH removal, this plant constitutes a valuable biomass for biofertilizers and bioenergy production (biogas, biohydrogen, bioethanol, liquid, and solid biochar), water treatment, charcoal briquettes, paper making, fish feed, animal feed, furniture making, fiberboard making, yarn and rope manufacture. All these potential ways have been deeply reviewed (Lu et al., 2008; Ratnani et al., 2020; Harun et al., 2021).
The water hyacinth is a potential candidate for bioenergy production because it is a lignocellulosic biomass. which could be used for sugar production, and fermentation to bioethanol and biohydrogen (Rezania et al., 2017). Another research has evaluated the bioconversion of fermentable sugars derived from WH into microbial lipids and single cell proteins by an oleaginous yeast. This study indicated the feasibility of sustainable valorization of WH-derived liquid hydrolysate towards a biorefinery framework Alankar et al. (2021). By the way, Arutselvy et al. (2020), have conducted a sequential valorization strategy for dairy wastewater (DW) and WH to produce fuel and fertilizer. Anaerobic co-digestion of WH and DW improved the process efficiency and increased biomethane production. Biomethane yield from the codigestion was higher compared to the monodigestion. The residual digestate was used for pyrolysis to obtain bio-oil and biochar. Another study indicated that the biochar obtained from WH pyrolysis could serve for heavy metal adsorption, and could be used for bioremediation application (Hasan et al., 2021). In addition, an integration strategy of hydrothermal carbonization (HTC) and anaerobic digestion (AD) using WH, showed that hydrochars and process waters from HTC process, could be valorized for a solid combustion fuel and biomethane production (Brown et al., 2020).
Besides bioenergy, WH valorization showed its importance in the agricultural sector as well. A novel approach of formulating an enriched N-P-K (nitrogen-phosphorous-potassium) fertilizer from WH and other residues (wet blue leather and poultry bone) has been performed. The developed organic N-P-K fertilizer resulted in significantly higher plants compared to commercial fertilizers (Majee et al., 2019). Moreover, WH could be transformed to a high-quality forage for animal feed. A previous study showed that the cultivation of edible mushrooms on WH biomass degrades almost all the lignin-cellulose of the substrate through enzymes, and enriches it with high protein content, making the spent substrate an excellent forage (Araiza et al., 2016).
Water hyacinth has been a subject for the development of cement composites and bioplastics. A study conducted by Salas-Ruiz et al. (2019), showed that WH root ash could be used as an alternative to pozzolans in cement matrices to fix these pollutants. This developed composite is cheap, green, and promotes waste and pollutants recycling and elimination. WH with other agricultural residues (bagasse and rice straw) could be transformed to produce bioplastic with a high biodegradability rate. This ecofriendly product could be used as an alternative to synthetic plastics (Ungprasoot et al., 2021).
Results obtained from this bibliographic research showed how it is beneficent to adopt these kind of strategies for efficient WH management. An economic feasibility study performed by Wang et al. (2019), showed that integrated valorization approaches could be beneficial, especially in the case of costly valorization technics such as bioethanol production. The study confirmed that coupled use of WH as a phytoremediation plant and bioethanol feedstock is a potential response to green development strategies.
Results and Discussion
Effectiveness of WH Control Programs
The water hyacinth in the absence of its natural enemies, causes serious problems worldwide and especially in Africa, where it has rapidly invaded rivers and freshwater bodies, especially in tropical and temperate-warm regions. It has grown enormously in the last decades, becoming an agricultural, environmental, and public health problem.
Control of this weed is difficult, and all appropriate options available should be considered. If necessary, a variety of control measures can be used, depending on the nature of the problem and the local conditions of the affected area. Therefore, available environmentally friendly, and sustainable methods should be adopted (Labrada and Fornasari, 2002).
Different control programs of this weed have been applied worldwide on large-scale. Some countries have adopted the mono-control approach, by using one control method for the whole invaded area, while others have applied integrated control approach, which consists of combining the available control methods, at the same time but separated in space, according to the site’s specificity and conditions.
According to our extensive bibliographic research carried out on the different control methods and their use worldwide, BC appeared to be effective and sustainable for controlling WH. Ndunguru et al. (2001) reported that the Neochetina weevils had an effective and long-term impact, leading to a gradual reduction in the WH vigor and thus increasing its susceptibility to competition from other aquatic vegetation. Moreover, a study conducted by Firehun et al. (2015) has shown that Neochetina weevils are a promising candidate for BC of WH under tropical conditions.
The applications of herbicides over wide areas results in large quantities of WH biomass sinking into the water (Ajithram et al., 2020). The anaerobic decomposition of this biomass decreases dissolved oxygen in the water and results in massive biodiversity damage and greenhouse gas emissions. In addition, herbicides kill non targeted plants that are native, beneficial, and necessary for healthy functioning of the Lakes’s ecosystem (Rosic et al., 2020). In Mexico a combined chemical-mechanical program, using the herbicide 2,4-dichlorophenoxyacetic acid (2,4-D) and a mechanical harvester, was implemented to control WH on the Trigomil Dam. Reasonably successful results were obtained (Gutiérrez et al., 1996). In China, integrating Roundup at different concentrations (41% salt of glyphosate) and Caoganlin (10% salt of glyphosate)) with weevils (N. eichhorniae and N. bruchi) greatly suppressed the plants. The tests indicated that herbicides had to be used at a lower concentration than normal, to not kill the plants too rapidly and not deprive the insects of food and habitat. The combined biological-chemical approach was effective (Mallya et al., 2001). Nevertheless, herbicide application on WH is forbidden in many other areas of China. For this reason, an asexual reproduction inhibitor named KWH02 was invented for controlling WH. The results indicated that KWH02 is a suitable control agent in areas, where water is used for drinking and in Lakes, pools, and creeks, where the water flow rate is slow (Chu et al., 2006).
On the other hand, it was reported that only physical removal, either manual or mechanical, of WH can eliminate the plant’s biomass from the water. It is considered as a selective approach and short-term solution (George and Goldman, 2007). However, disposal of the WH biomass after the physical removal remains problematic and creates terrestrial disposal issues. Plant disposal leads to a new bloom, causing environmental risks (Gunnarsson and Petersen, 2007). If WH is properly disposed of and collected, it could be used as a primary source to generate high value-added products and energy (Ayanda et al., 2020). It can offer the economic incentives to facilitate a sustained and effective WH management program. Although, recent studies have shown that it has diverse uses (Mathew et al., 2015; Hernández-Shek et al., 2016; Sindhu et al., 2017; Priya et al., 2018). These studies further recommended that the potential of this macrophyte should be fully harnessed, which could generate significant incomes. In wide infested areas the exploitation of this plant should no longer pose problems of profitability since productivity will be ensured by harvesting a surplus of the plants. Frequent and controlled harvesting of excess WH is essential to better control the rate of water plant coverage of the water surface. Additionally, using BC combined with the physical one improved the management process. Ndunguru et al. (2001) reported that WH infestation in Lake Victoria was reduced by 78% using integrated management strategies such as BC using the Neochetina weevils and manual removal. Since the physical control leads to an immediate elimination of WH, the BC ensures the establishment of bioagents in the study area and maintain the program sustainability in case of future proliferation of the plant. To visualize the approaches feasibility, and to evaluate their effectiveness in terms of application and performance, a descriptive analysis on WH control approaches and programs used worldwide was conducted.
The objective of this analysis was to identify successful control programs around the world and to evaluate the most used and effective ones. Results obtained from previous large-scale WH control experiences were considered. The analysis was carried out in 26 countries, in America, Asia, Australia, Europe and Africa. Table 5 summarizes the bibliographic research results.
As shown in Table 5, in the 26 large-scale trials, 70 control methods have been applied. Each country adopted one or more control methods throughout the invasion period of the plant. In the experiments performed, the control methods have been used either separately or in combination.
Control methods classification in terms of application for the studied countries is presented in Figure 8. Out of the 70 control methods, the biological control method has been used 25 times with a percentage of 36%, followed by the physical treatment which has been applied 23 times with 33%, and finally the chemical and the integrated control have been applied 11 times (16%) for each one in all experiments. The most adopted control methods consisted of using the biological and the physical controls separately, followed by the integrated approach, and then the separated chemical control.
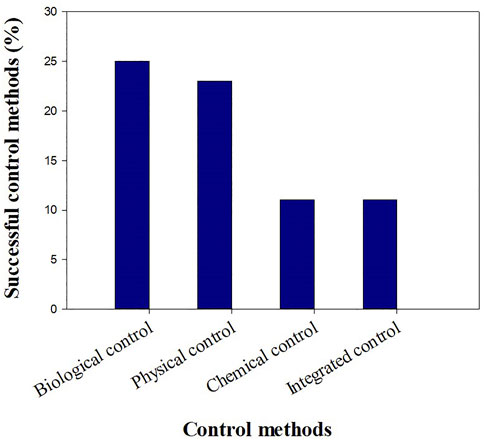
FIGURE 8. Classification of separate and integrated control methods, in terms of application based on 26 large-scale trials.
Most countries started with physical control alone followed by the biological one, and few countries have adopted the chemical and integrated control. The mono-physical control has failed in most of the case studies, while the mono-biological has been successful in the major cases. The chemical control has been successful as well; but has not been used many times. The integrated control has been used in few countries but it was successful in all of them.
The classification of the control methods in terms of effectiveness for the studied countries is presented in Figure 9. From 25 biological control programs, 21 were effective. The biological control has shown its effectiveness in some cases where it has been used alone, and has been 100% effective in all integrated program cases.
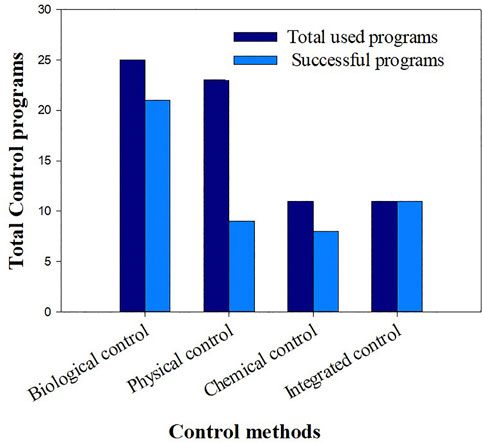
FIGURE 9. Classification of separate and integrated control methods, in terms of effectiveness based on 26 large-scale trials.
For the separate physical control, despite being used widely in several countries, only few cases (9 countries out of 23) were successful while, the chemical control has not been too much adopted, because it’s costly, harmful for the environment, and forbidden in some countries (Cabrera Walsh et al. (2017)). As shown in Figure 9, despite its negative impact, and being adopted only in few countries, the chemical control was effective.
The integrated control approach is the combination of one or more control method. It involves the test of at least two control methods simultaneously but separately in space, according to the site’s specificity and conditions. The combinations consist of integrated biological and physical control methods (IBP), integrated biological and chemical control methods (IBC), integrated physical and chemical methods (IPC) and integrated biological, physical and chemical methods (IBPC). In all case studies, 11 integrated control programs have been used and all of them were successful. The integrated methods IPB, ICB, IPCB, and IPC have been used 4, 3, 3 and 1 times respectively. As shown in Figure 10, the IPB was the most used program (37%) compared to the other integrated approaches. Although all integrated programs were successful, the combined physical and biological approach was the most effective. Therefore, it is recommended to adopt this integrated approach or to switch to an integrated “biological-physical and chemical’’ approach in case of emergency. In this latter case, the chemical control should be used properly in very specific and limited infested sites. Control methods must consider such scale dependent characteristics of WH invasions and ecosystem structure (Lu et al., 2007). Moreover, an integrated “control-valorization’’ approach is highly recommended. Awareness about WH benefits by developing new product locally based on this plant should be raised. This approach has two objectives: it will allow both WH removal and income generation. In addition, nutrient run-off reduction should be considered in management programs of WH. Because nutrient run-off is the major factor leading to the proliferation of WH in freshwater bodies, its control is necessary to decrease the vigorous growth of this plant (Chen et al., 2021). It was reported that an integrated control strategy is considered as the most effective method in South Africa, including biological control, manual removal, herbicide applications, and nutrient reduction (Paterson et al., 2019). Mitigation measures of nutrients’ input from agriculture activities and the monitoring of the streams feeding the freshwater systems should be implemented. All stakeholders and policy makers should be aware of the need to reduce the impact of pollution on freshwater bodies to succeed in managing the WH invasion.
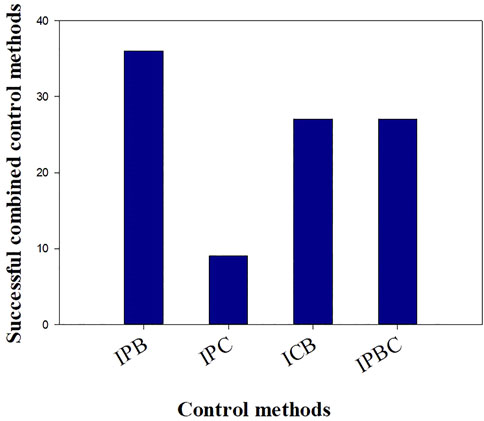
FIGURE 10. Classification of integrated control approaches, in terms of application based on 26 large-scale trials.
Economical Evaluation of Water Hyacinth Controls Program
Table 6 shows the cost/benefit of the 3 separate control methods and that of the integrated approach. It is worth mentioning here that the monetary investment per hectare (US$/ha) cleared for each separate control method was calculated based on research data (surface area infested and control cost in different countries) except for South Africa and Sudan, for which the cost of the manual and mechanical removal has been taken from previous studies (Van Wyk and Van Wilgen 2002; Jones, 2009; Tayeb and Nagat Mubarak, 2012; Ilo et al., 2020).
As shown in Table 6, the use of the physical or the chemical control separately requires high investment. However, in Sudan, Benin, South Africa and United States, the cost of the biological control was about (16.6), (20.9), (18) and (12.8 US$/ha) respectively (Van Wyk and Van Wilgen et al., 2020; De Groote et al., 2003; Tayeb and Nagata Mubarak, 2012; Wainger et al., 2018). The cost of the separate biological control seems to be the lowest compared to the other control methods. Furthermore, all successful programs consisted mainly of biological control that is considered the most cost-effective and sustainable control method but long-lasting and a slow-acting one. For example, in Benin, biological control has been conducted from 1991 until 2003 (De Groote et al., 2003). As a result of this large-scale experiment, Neochetina eichhorniae showed a significant reduction of the WH covered area.
However, biological control cannot be suitable for all regions, especially in areas that experience periodic floods and frost that cause high mortality of the bioagents since the plant is able to regrow after winter. In these regions, it is necessary to switch from a purely biological to a more integrated management approach, which includes also herbicide applications, mechanical and manual removal in addition to the biological treatment. For an effective biological control, the combined control methods promote weed stands reduction and growth of natural enemies (Hill and Coetzee, 2008).
A review on the benefits of WH (Eichhornia crassipes) for Southern Africa conducted by Ilo et al. (2020) clearly showed the economic feasibility of WH using control methods. The paper revealed that the integrated control (biological with chemical) of WH was the most cost effective method (16 US$/ha) followed by the biological (18 US$/ha) and the chemical (86 US$/ha) methods. Similar results were found in China where more than US$ 10 million/year was spent for the physical control even though it was not effective. From 2002 to 2006, the Shanghai government invested US$ 1.2 million for integrated management of WH that was effective (Chu, et al., 2006). From the obtained results, we can deduce that the separate physical and chemical controls require a big investment, while the integrated control approach gives the best return on investment and decreases the period of the program achievement.
Conclusion
Water Hyacinth is reported as an invasive aquatic plant causing many dramatic problems, mainly on the environment and the socioeconomic activities, in lakes, rivers, and many reservoirs around the globe, particularly in Africa.
The current research described and compared the existing solutions for managing and controlling WH worldwide, provided a concise conclusion on the most used, cost-effective, and sustainable programs, and highlighted the most recent and innovative studies on the WH valorization pathways.
This review demonstrated clearly that an integrated control program using different methods, nutrients run-off monitoring and biomass valorization seems to be the most effective and balanced approach for WH management. Water hyacinth management plans should be multi-scaled, integrated, and adaptive. Reduction of nutrient levels leaching into fresh water bodies should be considered as an integral part of any large-scale WH management because polluted water bodies promote WH infestations. Valorization technics of the harvested WH should be scaled up to remove the biomass and generate additional incomes.
In summary, this review has three main objectives: 1) help local stakeholders and decision makers of each infested area to deliver sustainable WH management, 2) encourage researchers to develop new technics to valorize the weed, and 3) motivate industrials to set up new commercials units for the valorization of the harvested WH, or to incorporate it in their present conversion processes.
Author Contributions
FK: Conceptualization, Methodology, Investigation, Analysis, Interpretation, Writing-Original Draft, Review and Editing WB: Methodology, Review and Editing AE: Methodology, Review and Editing MS: Methodology, Review and Editing MK: Methodology, Review and Editing AY: Methodology, Review and Editing Project administration, Supervision. MH: Conceptualization, Methodology, Validation, Review and Editing, Supervision LK: Conceptualization, Methodology, Validation, Review and Editing, Project administration, Supervision.
Conflict of Interest
The authors declare that the research was conducted in the absence of any commercial or financial relationships that could be construed as a potential conflict of interest.
Publisher’s Note
All claims expressed in this article are solely those of the authors and do not necessarily represent those of their affiliated organizations, or those of the publisher, the editors and the reviewers. Any product that may be evaluated in this article, or claim that may be made by its manufacturer, is not guaranteed or endorsed by the publisher.
Acknowledgments
This review is a part of the project: “Integrated and sustainable management of the Water hyacinth in Lake Tana, Ethiopia (AS 55)”. The authors are very grateful to OCP-Ethiopia for funding this technical review.
References
Abtew, W., and Dessu, S. (2019). The Grand Ethiopian Renaissance Dam on the Blue Nile. Springer International Publishing AG, part of Springer, Nature Springer Geography. doi:10.1007/978-3-319-97094-3_10
Admas, A., Sahile, S., Agidie, A., Menale, H., Gedefaw, T., and Teshome, M. (2020). Controlling Water Hyacinth Infestation in Lake Tana Using Fungal Pathogen from Laboratory Level Upto Pilot Scale. Gondar Zuria Wereda, Lemeba Kebela, 2020060357. doi:10.20944/preprints202111.0359.v1
Agjee, N. e. H., Ismail, R., and Mutanga, O. (2016). Identifying Relevant Hyperspectral Bands Using Boruta: a Temporal Analysis of Water Hyacinth Biocontrol. J. Appl. Remote Sens 10, 042002. doi:10.1117/1.JRS.10.042002
Ajithram, A., Winowlin Jappes, J. T., and Brintha, N. C. (2021). Water Hyacinth (Eichhornia crassipes) Natural Composite Extraction Methods and Properties - A Review. Mater. Today Proc. 45, 1626–1632. doi:10.1016/j.matpr.2020.08.472
Alankar, S. S. L., Sajesh, N., Rastogi, S., Sakhuja, S., Rajeswari, G., Kumar, V., et al. (2021). Bioprocessing of Fermentable Sugars Derived from Water Hyacinth into Microbial Lipids and Single Cell Proteins by Oleaginous Yeast Rhodosporidium Toruloides NCIM 3547. Biomass Conv. Bioref. doi:10.1007/s13399-021-02007-6
Arumugam, N., Chelliapan, S., Kamyab, H., Thirugnana, S., Othman, N., and Nasri, N. (2018). Treatment of Wastewater Using Seaweed: A Review. Ijerph 15 (12), 2851. doi:10.3390/ijerph15122851
Arutselvy, B., Rajeswari, G., and Jacob, S. (2020). Sequential Valorization Strategies for Dairy Wastewater and Water Hyacinth to Produce Fuel and Fertilizer. J. Food Process. Eng. 44. doi:10.1111/jfpe.13585
Ayanda, O. I., Ajayi, T., and Asuwaju, F. P. (2020). Eichhornia crassipes (Mart.) Solms: Uses, Challenges, Threats, and Prospects. Scientific World J. 2020, 1–12. doi:10.1155/2020/3452172
Beshir, M. O., and Bennett, F. D. (1985). “Biological Control of Water Hyacinth on the White Nile, Sudan,” in Proceedings of the Sixth International Symposium on the Biological Control of Weeds, 1980, Brisbane, August 19-25, 1984. Editor E. Delfosse (Ottawa, Canada: Canadian Government Publishing Centre), 491–496.
Birhanie, M., Zegeye, W., Melaku, A., Abate, E., Asaye, G., and Mekonnen, M. (20202020). Effect of Different Concentrations of Acetic Acid on Water Hyacinth [Eichhornia crassipes (Mart.) Solms], Aquatic Life and Physicochemical Properties of Water under Pond Conditions. Abyss. J. Sci. Technol. 5 (No. 1), 34–41.
Brown, A. E., Adams, J. M. M., Grasham, O. R., Camargo-Valero, M. A., and Ross, A. B. (2020). An Assessment of Different Integration Strategies of Hydrothermal Carbonisation and Anaerobic Digestion of Water Hyacinth. Energies 13, 5983. doi:10.3390/en13225983
Cabrera Walsh, G., Hernández, M. C., McKay, F., Oleiro, M., Guala, M., and Sosa, A. (2017). Lessons from Three Cases of Biological Control of Native Freshwater Macrophytes Isolated from Their Natural Enemies. Aquat. Ecosystem Health Manag., 00. doi:10.1080/14634988.2017.1403264
Carvalho, L. B. d., and Cerveira Junior, W. R. (2019). Control of Water Hyacinth: A Short Review. Commun. Plant Sci. 9, 129–132 ref.44. doi:10.26814/cps2019021
Chen, J., Chen, S., Fu, R., Wang, C., Li, D., Jiang, H., et al. (20212021). Simulation of Water Hyacinth Growth Area Based on Multi-Source Geographic Information Data: An Integrated Method of WOE and AHP. Ecol. Indicators 125, 107574. doi:10.1016/j.ecolind.2021.107574
Chu, Jj., Ding, Y., and Zhuang, Qj. (2006). Invasion and Control of Water Hyacinth (Eichhornia crassipes) in China. Univ. - Sci. B 7, 623–626. doi:10.1631/jzus.2006.B0623
Cilliers, C. J., Hill, M. P., Ogwang, J. A., and Ajuonu, O. (2003). “Aquatic Weeds in Africa and Their Control,” in Biological Control in IPM Systems in Africa. Editors P. Neuenschwander, C. Borgemeister, and J. Langewald (Wallingford: CABI), 161–178. doi:10.1079/9780851996394.0161
Cock, M. W., Day, R., Herren, H., Hill, M. P., Julien, M. H., Neuenschwander, P., et al. (2000). Harvesters Get that Sinking Feeling. Biocontrol News Inf. 21, N1–N8.
Cofrancesco, Alfred. F. (1998). Overview and Future Direction of Biological Control Technology. J. Aquat. Plant Manage. 36, 49–53.
De Graft, J. K. (1995). “Integrated Control of Aquatic Weeds in Ghana,” in Strategies for Water Hyacinth Control. Editors R. Charudattan, R. Labrada, T. D. Center, and C. Kelly-Begazo (Florida, USA: Food and Agricultural Organization of the United Nations), 28–44.
De Groote, H., Ajuonu, O., Attignon, S., Djessou, R., and Neuenschwander, P. (2003). Economic Impact of Biological Control of Water Hyacinth in Southern Benin. Ecol. Econ. 45, 105–117. doi:10.1016/S0921-8009(03)00006-5
Deloach, C. J., and Cordo, H. A. (1983). Control of Water Hyacinth by Neochetina Bruchi (Coleoptera: Curculionidae: Bagoini) in Argentina. Environ. Entomol. 12 (1), 19–23. doi:10.1093/ee/12.1.19
Dersseh, Kibret., Kibret, Worqlul., Tilahun, Dagnew., Worqlul, Melesse., Moges, fnm., Dagnew, fnm., et al. (2019). Potential of Water Hyacinth Infestation on Lake Tana, Ethiopia: A Prediction Using a GIS-Based Multi-Criteria Technique. Water 11, 1921. doi:10.3390/w11091921
Emirhüseyinoğlu, Gö., and Ryan, S. M. (2019). Land Use Optimization for Nutrient Reduction under Stochastic Precipitation Rates. Environ. Model. Softw. 123, 104527. doi:10.1016/j.envsoft.2019.104527
Enyew, B. G., Assefa, W. W., and Gezie, A. (2020). Socioeconomic Effects of Water Hyacinth (Echhornia Crassipes) in Lake Tana, North Western Ethiopia. Plos One 15, e0237668. doi:10.1371/journal.pone.0237668
Firehun, Y., Struik, P. C., Lantinga, E. A., and Taye, T. (2015). Joint Use of Insects and Fungal Pathogens in the Management of Water Hyacinth (Eichhornia crassipes): Perspectives for Ethiopia. J. Aquat. Plant Manage. 13.
Frieman, G., Garrett, L., Quinn, J., and Wittmann, M. (2004). A Cost and Environmental Analysis of Aquatic Plant Management in California. [Master's Thesis] (California: University of California).
Gebregiorgis, F. Y. (2017). Management of Water Hyacinth (Eichhornia crassipes [Mart.] Solms) Using Bioagents in the Rift Valley of Ethiopia. [PhD Thesis]. Wageningen: Wageningen University. doi:10.18174/401611
George, M. S., and Goldman, L. (2007). Assessing the Assessments: Case Study of an Emergency Action Plan for the Control of Water Hyacinth in Lake Victoria. Int. J. Water Resour. Dev. 23 (3), 443–455. doi:10.1080/07900620701488521
Greenfield, B. K., Siemering, G. S., Andrews, J. C., Rajan, M., Andrews, S. P., and Spencer, D. F. (2007). Mechanical Shredding of Water Hyacinth (Eichhornia crassipes): Effects on Water Quality in the Sacramento-San Joaquin River Delta, California. Estuaries and Coasts 30, 627–640. doi:10.1007/BF02841960
Güereña, D., Neufeldt, H., Berazneva, J., and Duby, S. (2015). Water Hyacinth Control in Lake Victoria: Transforming an Ecological Catastrophe into Economic, Social, and Environmental Benefits. Sustainable Prod. Consumption 3, 59–69. doi:10.1016/j.spc.2015.06.003
Gunnarsson, C. C., and Petersen, C. M. (2007). Water Hyacinths as a Resource in Agriculture and Energy Production: A Literature Review. Waste Manag. 27 (Issue 1), 117–129. doi:10.1016/j.wasman.2005.12.011
Gutiérrez, E., Huerto, R. n., Saldaña, P., and Arreguín, F. (1996). Strategies for Waterhyacinth (Eichhornia crassipes) Control in Mexico. Hydrobiologia 340, 181–185. doi:10.1007/BF00012752
Harun, I., Pushiri, H., Amirul-Aiman, A. J., and Zulkeflee, Z. (2021). Invasive Water Hyacinth: Ecology, Impacts and Prospects for the Rural Economy. Plants 10, 1613. doi:10.3390/plants10081613
Hasan, S., Girindran, R., Zacharia, P. U., Jaya, H., Kooren, R., Sayooj, P., et al. (2021). Climate Resilient Products Development through Valorization of Eichhornia crassipes to Biofuel and Biochar. Int. J. Environ. Sci. Technol. doi:10.1007/s13762-021-03523-8
Hauptfleisch, K. A. (2015). A Model for Water Hyacinth Biological Control. [Master's thesis]. Johannesburg (South Africa): University of the Witwatersrand.
Hermoso-López Araiza, J. P., Quecholac-Piña, X., Beltrán-Villavicencio, M., Espinosa-Valdemar, R. M., Vázquez-Morillas, A., and Vazquez-Morillas, A. (2016). Integral Valorization of the Water Hyacinth from the Canals of Xochimilco: Production of Edible Mushrooms and Forage. Waste Biomass Valor. 7, 1203–1210. doi:10.1007/s12649-016-9526-0
Hernández-Shek, M. A., Cadavid-Rodríguez, L. S., Bolaños, I. V., and Agudelo-Henao, A. C. (2016). Recovering Biomethane and Nutrients from Anaerobic Digestion of Water Hyacinth (Eichhornia crassipes) and its Co-digestion with Fruit and Vegetable Waste. Water Sci. Technol. 73, 355–361.
Hill, M. P., and Coetzee, J. A. (2008). Integrated Control of Water Hyacinth in Africa1. EPPO Bull. 38, 452–457. doi:10.1111/j.1365-2338.2008.01263.x
Hill, M. P., Martin, Julie., Coetzee, J. A., and Ueckermann, C. (2012). Toxic Effect of Herbicides Used for Water Hyacinth Control on Two Insects Released for its Biological Control in South Africa. Biocontrol Sci. Tech. 22 (11), 1321–1333. doi:10.1080/09583157.2012.725825
Ilo, O. P., Simatele, M. D., Nkomo, S. p. L., Mkhize, N. M., and Prabhu, N. G. (2020). The Benefits of Water Hyacinth (Eichhornia crassipes) for Southern Africa: A Review. Sustainability 12, 9222. doi:10.3390/su12219222
Ismail, S. N., Subehi, L., Mansor, A., and Mashhor, M. (2019). Invasive Aquatic Plant Species of Chenderoh Reservoir, Malaysia and Jatiluhur Reservoir, Indonesia. IOP Conf. Ser. Earth Environ. Sci. 380, 012004. doi:10.1088/1755-1315/380/1/012004
Jafari, N. (2010). Ecological and Socio-Economic Utilization of Water Hyacinth (Eichhornia crassipes Mart Solms). J. Appl. Sci. Environ. Manag. 14. doi:10.4314/jasem.v14i2.57834
Jayanth, K. P. (1988). Successful Biological Control of Water Hyacinth(Eichhornia Crassipes)byNeochetina Eichhorniae (Coleoptera:curculionidae) in Bangalore, India. Trop. Pest Manag. 34, 263–266. doi:10.1080/09670878809371254
Jones, R. W. (2009). The Impact on Biodiversity, and Integrated Control, of Water Hyacinth, Eichhornia crassipes (Martius) Solms-Laubach (Pontederiaceae) on the Lake Nsezi – Nseleni River System. [Thesis] (Grahamstown (South Africa): Rhodes University).
Julien, M. H. (2001). “Biological and Integrated Control of Water Hyacinth,” in Eichhornia crassipes proceedings of the second meeting of the Global working group for the biological and integrated control of Water hyacinth, 9-12 Oct. 2000 (Beijing, ChinaCanberra: ACIAR).
Kaur, M., Kumar, M., Sachdeva, S., and Puri, S. K. (2018). Aquatic Weeds as the Next Generation Feedstock for Sustainable Bioenergy Production. Bioresour. Tech. 251, 390–402. doi:10.1016/j.biortech.2017.11.082
Kiristos, T. G., Kebede, A., Chaithanya, K. K., and Zenebe Teka, M. (2018). Evaluation of In Vitro Antibacterial Potential of Eichhornia crassipes Leaf Extracts. Drug Invent. Today 10.
Krisnawati, A., Masykuri, M., and Sunarto, (2020). The Dynamics of Organic Pollutant Pollution of Phosphate, Sulphate, Nitrite, and Nitrate Parameters in Cengklik Reservoir Water. AIP Conf. Proc. 2296 (1), 020005–020007. doi:10.1063/5.0030685
Kyser, B., Madsen, J. D., Miskella, J., and O'brien, J. (2021). New Herbicides and Tank Mixes for Control of Water Hyacinth in the Sacramento‐San Joaquin Delta. J. Aquat. Plant Manage. 59s, 78–81.
Labrada, R., and Fornasari, L. (2002). Management of Problematic Aquatic Weeds in Africa: FAO Efforts and Achievements during the Period 1991-2001. Rome, Italy: FAO.
Langa, S. D., Hill, M. P., and Compton, S. G. (2020). Agents Sans Frontiers: Cross-Border Aquatic weed Biological Control in the Rivers of Southern Mozambique. Afr. J. Aquat. Sci. 45, 329–335. doi:10.2989/16085914.2020.1749551
Lazim, M. Z., SalmiatiSamaluddin, A. R., Razman, M. S., and Zaiha, A. N. (2020). Toxicity of Silver Nanoparticles and Their Removal Applying Phytoremediation System to Water Environment: An Overview. J. Environ. Treat. Tech. 8 (3), 978–9842. 2309.
Lu, J., Fu, Z., and Yin, Z. (2008). Performance of a Water Hyacinth (Eichhornia crassipes) System in the Treatment of Wastewater from a Duck Farm and the Effects of Using Water Hyacinth as Duck Feed. J. Environ. Sci. (China) 20 (5), 513–519. doi:10.1016/S1001-0742(08)62088-4
Lu, J., Wu, J., Fu, Z., and Zhu, L. (2007). Water Hyacinth in China: A Sustainability Science-Based Management Framework. Environ. Manage. 40, 823–830. PMID: 17768654. doi:10.1007/s00267-007-9003-4
Majee, S., Halder, G., and Mandal, T. (2019). Formulating Nitrogen-Phosphorous-Potassium Enriched Organic Manure from Solid Waste: A Novel Approach of Waste Valorization. Process Saf. Environ. Prot. 132, 160–168. doi:10.1016/j.psep.2019.10.013
Malik, A. (2007). Environmental challenge Vis a Vis Opportunity: The Case of Water Hyacinth. Environ. Int. 33 (2007), 122–138. doi:10.1016/j.envint.2006.08.0010.1016/j.envint.2006.08.004
Mallya, G. A., Mjema, P., and Ndunguru, J. (2001). “Water Hyacinth Control through Integrated Pest Management Strategies in Tanzania,” in Proceedings of the second meeting of global working group for the biological and integrated control of Water hyacinth, Beijing, China. Canberra, Australia, 9–12 October 2000. Editors M. H. Julien, M. P. Hill, and D. Jianqing (ACIAR), 102, 120–122.
Mangas-Ramírez, E., and Elías-Gutiérrez, M. (2004). Effect of Mechanical Removal of Water Hyacinth (Eichhornia crassipes) on the Water Quality and Biological Communities in a Mexican Reservoir. Aquat. Ecosystem Health Manag. 7, 161–168. doi:10.1080/14634980490281597
Martínez Jiménez, M., Gómez Balandra, M. A., and Ma, A. (2007). Integrated Control of Eichhornia crassipes by Using Insects and Plant Pathogens in Mexico. Crop Prot. 26, 1234–1238. doi:10.1016/j.cropro.2006.10.028
Mathew, A. K., Bhui, I., Banerjee, S. N., Goswami, R., Chakraborty, A. K., Shome, A., et al. (2015). Biogas Production from Locally Available Aquatic Weeds of Santiniketan through Anaerobic Digestion. Clean. Techn Environ. Pol. 17, 1681–1688. doi:10.1007/s10098-014-0877-6
Mellhorn, M. (2013). Water Hyacinths (Eichornia crassipes) and Their Presence in Shire River, Malawi, Problems Caused by them and Ways of Utilize them Elsewhere. [Thesis]. Uppsala (Sweden): Uppsala University.
Merry, M., Mitan, N., and Merry, M. (2019). Water Hyacinth: Potential and Threat. Mater. Today Proc. 19, 1408–1412. doi:10.1016/j.matpr.2019.11.160
Miller, B. E., Coetzee, J. A., and Hill, M. P. (2020). Mind the gap: The Delayed Recovery of a Population of the Biological Control Agent Megamelus Scutellaris Berg. (Hemiptera: Delphacidae) on Water Hyacinth after winter. Bull. Entomol. Res. 111, 1–9. doi:10.1017/S0007485320000516
Mitan, N., and Merry, M. (2019). Water Hyacinth: Potential and Threat. Mater. Today Proc. 19, 1408–1412. doi:10.1016/j.matpr.2019.11.160
Moyo, P., Chapungu, L., and Mudzengi, B. (2013). A Proposed Integrated Management Approach to the Control of Water Hyacinth: The Case of Shagashe River in Masvingo, Zimbabwe. Greener J. Phys. Sci. 3 (6), 229–240. doi:10.15580/gjoms.2013.1.170913843
Nang’alelwa, M. (2008). The Environmental and Socio-Economic Impacts of Eichhornia crassipes in the Victoria Falls/Mosi-oa-Tunya World Heritage Site, Livingstone, Zambia. EPPO Bull., 38 (3), 470–476. doi:10.1111/j.1365-2338.2008.01266.x
Ndunguru, J., Mjema, P., Rajabu, C. A., and Katagira, F. (2001). Water Hyacinth Infestation in Ponds and Satellite Lakes in the Lake Victoria Basin on Tanzania: Status and Efforts to Manage it. Tanzania: Plant Protection Division.
Nesslage, G. M., Genevieve, Lisa., Wainger, L. A., Nathan, E., Harms, Alfred., Harms, N. E., et al. (2016). Quantifying the Population Response of Invasive Water Hyacinth, Eichhornia crassipes, to Biological Control and winter Weather in Louisiana, USA. Biol. Invasions 18, 2107–2115. doi:10.1007/s10530-016-1155-9,
Ogwang, J. A., and Molo, R. (1999). “Impact Studies on Neochetina Bruchi and Neochetina Eichhorniae in Lake Kyoga, Uganda,” in Proceedings of the first IOBC global working group meeting for the biological and integrated control of Water hyacinth, St Lucia Park Hotel, Harare, Zimbabwe, 16 – 19 November 1998. Editors M. P. Hill, M. H. Julien, and T. Center, 10–13.
Osmond, R., and Petroeschevsky, A. (2013). Control Options for Water Hyacinth (Eichhornia crassipes) in Australia. ISBN 978 1 74256 585 9. The state of new South wales, NSW department of primary industries.
Patel, S. (2012). Threats, Management and Envisaged Utilizations of Aquatic weed Eichhornia crassipes: An Overview. Rev. Environ. Sci. Biotechnol. 11, 249–259. doi:10.1007/s11157-012-9289-4
Paterson, I. D., Iain, Julie., Coetzee, J. A., Philip, Weyl., Griffith, P., Griffith, T. C., et al. (2019). Cryptic Species of a Water Hyacinth Biological Control Agent Revealed in South Africa: Host Specificity, Impact, and thermal Tolerance. Entomol. Exp. Appl., 1–10. doi:10.1111/eea.12812
Perna, C., and Burrows, D. (2005). Improved Dissolved Oxygen Status Following Removal of Exotic weed Mats in Important Fish Habitat Lagoons of the Tropical Burdekin River Floodplain, Australia. Mar. Pollut. Bull. 51, 138–148. doi:10.1016/j.marpolbul.2004.10.050
Perna, C. N., Cappo, M., Pusey, B. J., Burrows, D. W., and Pearson, R. G. (2011). Removal of Aquatic Weeds Greatly Enhances Fish Community Richness and Diversity: An Example from the Burdekin River Floodplain, Tropical Australia. River Res. Applic. 28, 1093–1104. doi:10.1002/rra.1505
Phiri, P. M., Day, R. K., Chimatiro, S., Hill, M. P., Cock, M. J. W., Hill, M. G., et al. (2001). “Progress with Biological Control of Water Hyacinth in Malawi,” in Proceedings of the second meeting of global working group for the biological and integrated control of Water hyacinth, Beijing, China, Canberra, Australia, 9–12 October 2000. Editors M. H. Julien, M. P. Hill, and D. Jianqing (ACIAR), Proceedings 102, 47–52.
Priya, P., Nikhitha, S. O., Anand, C., Dipin Nath, R. S., and Krishnakumar, B. (2018). Biomethanation of Water Hyacinth Biomass. Bioresour. Tech. 255, 288–292. doi:10.1016/j.biortech.2018.01.119
Ratnani, Rita. Dwi., Hadiyanto, , and Widiyanto, (2020). Effect of Temperature and Pyrolysis Time in Liquid Smoke Production from Dried Water Hyacinth. J. Environ. Treat. Tech. 9 (1), 164–171. doi:10.47277/JETT/9(1)171
Reddy, A. M., Pratt, P. D., Hopper, J. V., Cibils-Stewart, X., Walsh, G. C., and Mc Kay, F. (2019). Variation in Cool Temperature Performance between Populations of Neochetina Eichhorniae (Coleoptera: Curculionidae) and Implications for the Biological Control of Water Hyacinth, Eichhornia crassipes, in a Temperate Climate. Biol. Control. 128, 85–93. January 2019. doi:10.1016/j.biocontrol.2018.09.016
Rezania, S., Din, M. F. M., Taib, S. M., Dahalan, F. A., Songip, A. R., Singh, L., et al. (2016). The Efficient Role of Aquatic Plant (Water Hyacinth) in Treating Domestic Wastewater in Continuous System. Int. J. Phytoremediation 18 (7), 679–685. doi:10.1080/15226514.2015.1130018
Rezania, S., Din, M. F. M., Taib, S. M., Sohaili, J., Chelliapan, S., Kamyab, H., et al. (2017). Review on Fermentative Biohydrogen Production from Water Hyacinth, Wheat Straw and rice Straw with Focus on Recent Perspectives. Int. J. Hydrogen Energ. 42 (33), 20955–20969. doi:10.1016/j.ijhydene.2017.07.007
Rogers, D. J., Terblanche, J. S., and Owen, C. A. (2020). Low‐temperature Physiology of Climatically Distinct South African Populations of the Biological Control Agent Neochetina Eichhorniae. Ecol. Entomol. 46, 138–141. doi:10.1111/een.12935
Rosic, N., Bradbury, J., Lee, M., Baltrotsky, K., and Grace, S. (2020). The Impact of Pesticides on Local Waterways: A Scoping Review and Method for Identifying Pesticides in Local Usage. Environ. Sci. Pol. 106, 12–21. doi:10.1016/j.envsci.2019.12.005
Salas-Ruiz, A., María, B., María, R., and Asensio, E. (2019). Water Hyacinth Cement Composites as Pollutant Element Fixers. Waste Biomass Valor. 11 (7), 3833–3851. doi:10.1007/s12649-019-00674-1
Shabana, Y., Fayad, Y., Al-Dobai, S., and Elkahky, M. (2018). Management of Aquatic Weed Water Hyacinth ''Eichhornia crassipes''. FAO. #175 p. ISBN: 978-92-5-130656-7. Training Workshop. Mansoura, Egypt, 25–27. August 2015
Sharma, A. K., Sharma, Varruchi., Sharma, Vandana., Sharma, J. K., and Singh, R. (2020). Multifaceted Potential of Eichhornia crassipes (Water Hyacinth) Ladened with Numerous Value Aided and Therapeutic Properties. Plant Arch. 20, 2059–2065.
Sharpley, A. N., Daniel, T., Gibson, G., Bundy, L., Cabrera, M., Sims, T., et al. (2006). Best Management Practices to Minimize Agricultural Phosphorus Impacts on Water Quality, 22161. Springfield, VA, 553–6847.
Simberloff, D., and Stiling, P. (1996). Risks of Species Introduced for Biological Control. Biol. Conservation 78, 185–192. doi:10.1016/0006-3207(96)00027-4
Simpson, M., Armando, M., Nagendra, P., Deepayan, B., Srikanth, R., Aviraj, D., et al. (2020). Monitoring Water Hyacinth in Kuttanad, india Using sentinel-1 Sar Data. IEEE, 978–981. 7281-3114-6/20/$31.00 2020.
Sindhu, R., Binod, P., Pandey, A., Madhavan, A., Alphonsa, J. A., Vivek, N., et al. (2017). Water Hyacinth a Potential Source for Value Addition: An Overview. Bioresour. Tech. 230, 152–162. doi:10.1016/j.biortech.2017.01.035
Souza, E. L. C., Evandro, D., Filho, J. T., Velini, E. D., Silva, J. R. M., Tonello, K. C., et al. (2020). Water Hyacinth Control by Glyphosate Herbicide and its Impact on Water Quality. Jwarp 1201, 60–73. Article ID:97755,14 pages. doi:10.4236/jwarp.2020.121004
Swallow, B. M., Walsh, M., Mugo, F., Ong, C., Shepherd, K., Place, F., et al. (2001). Improved Land Management in the Lake Victoria Basin: Annual Technical Report. to June 2001. The Centre. Available at: https://ecommons.aku.edu/eastafrica_eai/11.
Tayeb, El., and Nagat Mubarak, Ms. (2012). “Successful Biological Control of Water Hyacinth (Eichhornia Crassipes) by Neochetina Weevils in Sudan. International Trade and Invasive Alien Species,” in Conference Room CRI. Standards and trade development facility, WTO Building, 12-13 July 2012.
Tewabe, D., and Asmare, E. (2020). Assessment of Some Invasive Aquatic Weeds and Water Hyacinth Effect on Fishery and Other Aquatic Biota in Lake Tana. J. Vet. Marine. Sci. 2 (1), 23–29.
Tobias, V. D., Anessa, J., Conrad, L., Conrad, J. L., Mahardja, B., and Khanna, S. (2019). Impacts of Water Hyacinth Treatment on Water Quality in a Tidal Estuarine Environment. Biol. Invasions 21, 3479–3490. doi:10.1007/s10530-019-02061-2(0123456789
Ungprasoot, P., Muanruksa, P., Tanamool, V., Winterburn, J., and Kaewkannetra, P. (2021). Valorization of Aquatic weed and Agricultural Residues for Innovative Biopolymer Production and Their Biodegradation. Polymers 13, 2838. doi:10.3390/polym13172838
Valk, V. A. (2015). Valorization of Water Hyacinth as a Renewable Source of Animal Feed and Biogas: A Business Case for Lake Victoria, Kenya. [Master's Thesis] (Wageningen (Netherlands): Wageningen University). Available at: http://resolver.tudelft.nl/uuid:415bce32-3782-49ba-99ce-1a433d826143.
Van Driesche, R. (2002). Biological Control of Invasive Plants in the Eastern United States, USDA Forest Service Publication FHTET-2002-04. Georgia, USA: University of Georgia, 413.
Van Oijstaeijen, W., Passel, S. V., Cools, J., Bisthoven, L. J., Hugé, J., Berihun, D., et al. (2020). Farmers’ Preferences towards Water Hyacinth Control: A Contingent Valuation Study. Int. Assoc. Great Lakes Res. 46 (5), 1459–1468. doi:10.1016/j.jglr.2020.06.009.0380-1330/
van Wilgen, B. W., Raghu, S., Sheppard, A. W., and Schaffner, U. (2020). Quantifying the Social and Economic Benefits of the Biological Control of Invasive Alien Plants in Natural Ecosystems. Curr. Opin. Insect Sci. 38, 1–5. Parasites/parasitoids/biological control. doi:10.1016/j.cois.2019.12.004
Van Wyk, E., and Van Wilgen, B. W. (2002). The Cost of Water Hyacinth Control in South Africa: A Case Study of Three Options. Afr. J. Aquat. Sci. 27, 141–149. doi:10.2989/16085914.2002.9626585
Villamagna, A. M., and Murphy, B. R. (2010). Ecological and Socio-Economic Impacts of Invasive Water Hyacinth (Eichhornia crassipes): a Review. Freshw. Biol. 55, 282–298. doi:10.1111/j.1365-2427.2009.02294.x
Wainger, L. A., Harms, N. E., Magen, C., Liang, D., Nesslage, G. M., McMurray, A. M., et al. (2018). Evidence-based Economic Analysis Demonstrates that Ecosystem Service Benefits of Water Hyacinth Management Greatly Exceed Research and Control Costs. PeerJ 6, e4824. doi:10.7717/peerj.4824
Waltham, N. J., and Fixler, S. (2017). Aerial Herbicide spray to Control Invasive Water Hyacinth (Eichhornia crassipes): Water Quality Concerns Fronting Fish Occupying a Tropical Floodplain Wetland. Trop. Conservation Sci. 10, 194008291774159–10. doi:10.1177/1940082917741592
Wang, Z., Zheng, F., and Xue, S. (2019). The Economic Feasibility of the Valorization of Water Hyacinth for Bioethanol Production. Sustainability 11, 905. doi:10.3390/su11030905
Wilson, R. J., Gutiérrez, D., Gutiérrez, J., Martínez, D., Agudo, R., and Monserrat, V. J. (2005). Changes to the Elevational Limits and Extent of Species Ranges Associated with Climate Change. Ecol. Lett. 8, 1138–1146. doi:10.1111/j.1461-0248.2005.00824.x
Yan, S.-H., Song, W., and Guo, J.-Y. (2016). Advances in Management and Utilization of Invasive Water Hyacinth (Eichhornia crassipes) in Aquatic Ecosystems - a Review. Crit. Rev. Biotechnol. 37, 218–228. doi:10.3109/07388551.2015.1132406
Yigermal, H., and Assefa, F. (2019). Impact of the Invasive Water Hyacinth (Eichhornia crassipes) on Socio-Economic Attributes: A Review. Environ. Sci. 4, 11.
Keywords: Water hyacinth, Control, Valorization, Costs, Benefits, Sustainibility
Citation: Karouach F, Ben Bakrim W, Ezzariai A, Sobeh M, Kibret M, Yasri A, Hafidi M and Kouisni L (2022) A Comprehensive Evaluation of the Existing Approaches for Controlling and Managing the Proliferation of Water Hyacinth (Eichhornia crassipes): Review. Front. Environ. Sci. 9:767871. doi: 10.3389/fenvs.2021.767871
Received: 01 September 2021; Accepted: 20 December 2021;
Published: 01 February 2022.
Edited by:
Shahabaldin Rezania, Sejong University, South KoreaReviewed by:
Hesam Kamyab, University of Technology Malaysia, MalaysiaEwa Wojciechowska, Gdansk University of Technology, Poland
Amirreza Talaiekhozani, Jami Institute of Technology, Iran
Nicole Nawrot, Gdansk University of Technology, Poland
Copyright © 2022 Karouach, Ben Bakrim, Ezzariai, Sobeh, Kibret, Yasri, Hafidi and Kouisni. This is an open-access article distributed under the terms of the Creative Commons Attribution License (CC BY). The use, distribution or reproduction in other forums is permitted, provided the original author(s) and the copyright owner(s) are credited and that the original publication in this journal is cited, in accordance with accepted academic practice. No use, distribution or reproduction is permitted which does not comply with these terms.
*Correspondence: Mohamed Hafidi, hafidi@uca.ac.ma