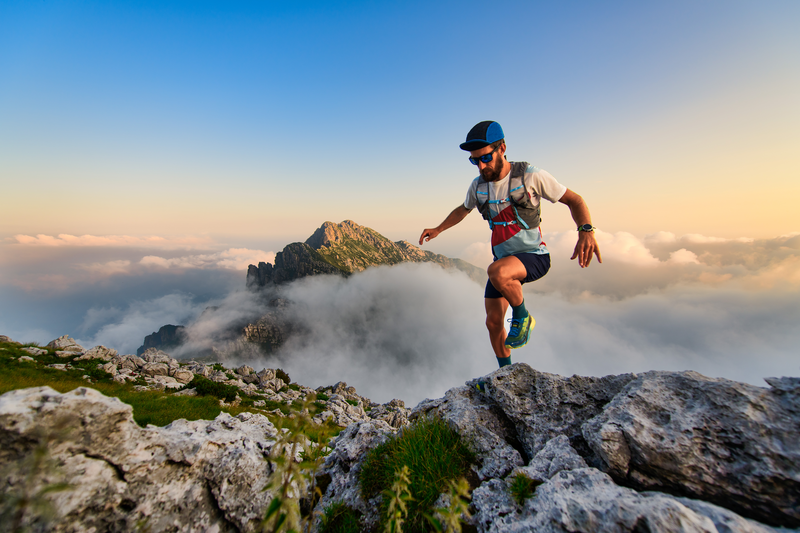
94% of researchers rate our articles as excellent or good
Learn more about the work of our research integrity team to safeguard the quality of each article we publish.
Find out more
ORIGINAL RESEARCH article
Front. Environ. Sci. , 19 October 2021
Sec. Water and Wastewater Management
Volume 9 - 2021 | https://doi.org/10.3389/fenvs.2021.764841
Benzodiazepines are one group of psychoactive drugs widely detected in water environments, and their persistence during conventional wastewater treatment has raised great concerns. Here we investigated the degradation of 17 benzodiazepines in water by UV/H2O2 treatment. The results showed that the UV/H2O2 treatment significantly increased the degradation of 17 benzodiazepines in phosphate buffer solutions at pH 7.0. This can be attributed to the high reactivity of hydroxyl radicals (·OH) towards benzodiazepines with second-order rate constants of 3.48 × 109 M−1 s−1–2.44 × 1010 M−1 s−1. The degradation of alprazolam, a typical benzodiazepine, during the UV/H2O2 treatment was increased with the increasing H2O2 dosage. The solution pH influenced the alprazolam degradation significantly, with the highest degradation at pH 7.0. Water matrix, such as anions (Cl−, HCO3−, NO3−) and humic acid, decreased the degradation of alprazolam by UV/H2O2 treatment. Based on the degradation products identified using quadrupole time-of-flight mass spectrometer, the degradation mechanisms of alprazolam by UV/H2O2 treatment were proposed, and hydroxylation induced by ·OH was the main reaction pathway. The degradation of 17 benzodiazepines by UV/H2O2 treatment in wastewater treatment plant effluent and river water was lower than that in phosphate buffer solutions. The results showed that the benzodiazepine psychoactive drugs in natural water can be effectively removed by the UV/H2O2 treatment.
Pharmaceuticals and personal care products (PPCPs) in water environments are a well-recognized concern due to their widespread occurrence and potential harm to environmental organisms (Yang et al., 2017; Danner et al., 2019; Patel et al., 2019; Lu et al., 2020, 2022). Benzodiazepines, a main class of psychoactive drugs, have pharmacological effects such as sedative-hypnotic, anxiolytic, anticonvulsant, antiepileptic, and muscle relaxant (Cunha et al., 2017). According to the annual report of International Narcotics Control Board (INCB), benzodiazepines have been used in more than 80 countries, and the global manufacture amount of benzodiazepines was over 195 tons in 2019. Most benzodiazepines, which cannot be completely metabolized in the body, are discharged, resulting in their wide occurrences in municipal wastewater, surface waters and seawaters at concentrations of 24.3–95.9 ng L−1 (Subedi and Kannan, 2015; Wu et al., 2015; Fernandez-Rubio et al., 2019). Existing previous studies have found that the conventional water and wastewater treatment processes, such as biological processes, coagulation, filtration and chlorination, are ineffective to remove benzodiazepines, which can lead to their discharge into the receiving waters (Cunha et al., 2017; Fick et al., 2017; Lei et al., 2021). The persistent nature of benzodiazepines may pose a negative impact on aquatic ecosystem. For example, diazepam at low environmental levels can decrease the growth rate of Daphnia magna and increase mortality rates of the young zebrafish (Danio rerio) (Kalichak et al., 2016; Rivetti et al., 2016). The additive effect of benzodiazepines at low concentrations has been confirmed. Benzodiazepines can alter the social behavior and feeding rates of freshwater fish-European perch (Perca fluviatilis) (Brodin et al., 2013; Cerveny et al., 2020). Consequently, it is essential to eliminate the residual benzodiazepines from water.
Advanced oxidation processes (AOPs) have been reported to be highly efficient processes for the degradation of refractory PPCPs (Miklos et al., 2018; Huang et al., 2020; Lee et al., 2020; Wang and Zhuan, 2020; Wu et al., 2020). Some AOPs, such as photo-Fenton, heterogeneous photocatalysis, UV/TiO2 and UV/H2O2 have been explored to remove benzodiazepines in water (Bosio et al., 2019; Cunha et al., 2019; Mitsika et al., 2021). The photocatalytic degradation of the selected benzodiazepines (i.e., alprazolam and diazepam) was proved to be effective with a photo-Fenton reaction system (Mitsika et al., 2021). The heterogeneous photocatalysis using synthesized composites based on TiO2 and activated carbon (TiO2/AC) as catalysts under sunlight-simulated irradiation can remove over 97.5% of bromazepam, clonazepam, and diazepam from water (Cunha et al., 2019). The UV/TiO2 and UV/H2O2 performed better under acidic conditions and removed 60–80% of alprazolam, clonazepam, diazepam, and lorazepam (Bosio et al., 2019). Limited previous studies examined the degradation of two benzodiazepines (i.e., diazepam and oxazepam) by UV/H2O2 treatments (Huber et al., 2003; Kosjek et al., 2012). Our previous studies also showed that the removal of diazepam was significantly enhanced by UV/chlorine and simulated sunlight/chlorine treatments due to the substantial contribution of ·OH (Yang et al., 2018; Yang et al., 2020). However, the kinetics and transformation products for ·OH reaction with other benzodiazepines are still missing.
This work aimed to investigate the degradation mechanism of 17 benzodiazepines by UV/H2O2 treatment in water. The reaction kinetics for 17 benzodiazepines in UV and UV/H2O2 processes were determined, respectively. The experimental parameters such as dosage of H2O2, solution pH and coexisting constituents (i.e., Cl−, HCO3−, NO3−, humic acid) were carefully evaluated. The degradation products and reaction pathways for a typical benzodiazepine (alprazolam) were tentatively revealed based on quadrupole time-of-flight mass spectrometer. Finally, the removal of 17 benzodiazepines by UV/H2O2 treatment was also conducted in wastewater treatment plant (WWTP) effluent and river water for practical application.
Standards of 17 benzodiazepines (alprazolam, bromazepam, chlordiazepoxide, clobazam, clonazepam, clozapine, diazepam, estazolam, flunitrazepam, flurazepam, lorazepam, midazolam, nordiazepam, nitrazepam, oxazepam, prazepam, temazepam) were obtained from Cerilliant Corporation (Texas, United States). The basic parameters of these 17 benzodiazepines were provided in Supplementary Information (Supplementary Table S1). Atrazine and p-chlorobenzoic acid (pCBA) were obtained from Sigma-Aldrich (Shanghai, China). Tert-butyl alcohol (TBA) was obtained from Aladdin Biochemical Technology Corporation (Shanghai, China). Hydrogen peroxide solution (H2O2, 30%, v:v) was obtained from Guangzhou chemical reagent factory (Guangzhou, China). Buffers and all other reagents used in the experiment were of analytical grade. HPLC grade methanol and acetonitrile were obtained from Merck Corporation (Shanghai, China).
The reaction solutions were prepared with Milli-Q water (≥18.2 MΩ cm). River water (RW) and municipal WWTP effluent (WW) used in the irradiation experiments were sampled in Guangzhou, China. The detailed characteristics of RW and WW are presented in Supplementary Table S2.
The UV/H2O2 experiments were performed in a DS-GHX-V photochemical reactor (Doosi Instrument Corporation, Shanghai, China) with a magnetic stirrer, as shown in Supplementary Figure S1. A 20 W low-pressure mercury lamp with an output wavelength of mainly 254 nm was equipped as UV irradiation source. The UV lamp was located in the center of the photochemical reactor surrounded by a quartz cooling jacket, which maintained the solution temperature at 25 ± 1.0°C. Experimental solutions were added in a series of 50 ml quartz tubes with a Teflon-coated stir bar, which were placed on the top of the magnetic stirrer. The rotation speed was 100 rpm/min. Based on Canonica et al. (2008), atrazine was used as a chemical actinometer to determine the photon fluence rate under UV irradiation, and the value of photon fluence rate was determined to be 28.74 μE m−2 s−1 (Supplementary Text S1). The kinetic experiments of 17 benzodiazepines by UV/H2O2 treatment were conducted in the 10 mM phosphate buffer at pH 7.0. The initial concentration of each benzodiazepine, pCBA and H2O2 was set at 100 μg L−1 and 100 μM, respectively. The pCBA was simultaneously added in the reaction solutions to quantify hydroxyl radical (·OH) concentration during the UV/H2O2 treatment. At predetermined time intervals, 1 ml of the reaction solutions were withdrawn and quenched with TBA (10 mM) to measure the residual concentrations of 17 benzodiazepines and pCBA by ultrahigh-performance liquid chromatograph-triple quadrupole mass spectrometry (UPLC-MS/MS) method. All experiments were performed in triplicate with pH variation of below 0.1 unit.
Experiments were also performed to evaluate the effects of experimental parameters, including H2O2 dosage (0–200 μM), solution pH (3.0–11.0), inorganic ions (Cl−, HCO3−, NO3−) and dissolved organic matter (humic acid, HA). A higher initial concentration of alprazolam (3,088 μg L−1) and H2O2 dosage (1 mM) was conducted for UV/H2O2 treatment to facilitate the detection and identification of degradation products by ultrahigh-performance liquid chromatograph-quadrupole time-of-flight mass spectrometer (UPLC-QTOF-MS) method. Besides, the above kinetic experiments were also performed in river water and municipal WWTP effluent.
The water quality parameters were characterized as follows. The pH, conductivity, dissolved oxygen (DO) and oxidation reduction potential (ORP) were determined using a YSI Proplus multiparameter meter (YSI, United States). The dissolved organic carbon (DOC) was measured using a Shimadzu TOC-VCSH/CSN and TNM-1 analyzer (SHIMADZU, Japan). Total alkalinity was determined through acidic-titration using a pH 4.2 titration end-point (Yang et al., 2018). The H2O2 solution was determined by Jinghua UV1800 UV-Vis spectrophotometry (Shanghai, China) based on ɛ = 40.0 M−1 cm−1 at λ = 240 nm.
The 17 benzodiazepines and pCBA were analyzed on a Waters Xevo TQ-S triple quadrupole mass spectrometry equipped with a Waters Acquity ultrahigh-performance liquid chromatograph. A BEH C18 column (2.1 × 50 mm, 1.7 μm) was used for the separation. The (A) buffer solution (5 mM CH3COONH3 + 0.05% HCOOH) and (B) acetonitrile were used for the mobile phase with the gradient elution program. The gradient was programmed as follows: kept in 20% B at initial, increased to 95% at 5 min, decreased to 20% B to 5.5 min and post time was 1 min. The 17 benzodiazepines and pCBA were analyzed by multiple reaction monitoring (MRM) in positive and negative electrospray ionization mode, respectively. Details MRM transitions and collision were shown in Supplementary Table S1. The method quantitation limits (MQLs), method detection limits (MDLs), quality assurance (QA) and quality control (QC) have been reported in our previous study (Lei et al., 2021).
The degradation products of alprazolam during the UV/H2O2 treatment were analyzed by Agilent 6545 quadrupole time-of-flight mass spectrometer equipped with an Agilent 1290 Ⅱ ultrahigh-performance liquid chromatograph. An Agilent Zorbax Eclipse Plus C18 column (3.0 × 150 mm, 1.7 μm) was used for the separation. For positive electrospray ionization (ESI+) analysis, the mobile phase consisted of (A) Milli-Q water with 0.1% formic acid and (B) acetonitrile with 0.1% formic acid at a flow rate of 0.3 ml min−1. For negative electrospray ionization (ESI-) analysis, the mobile phase consisted of (A) buffer solution (1 mM CH3COONH3 + 0.1% CH3COOH) and (B) acetonitrile with 0.1% acetic acid at a flow rate of 0.3 ml min−1. The gradient was programmed as follows: kept in 10% B at first 2 min, increased to 98% at 21 min, kept in 98% B to 24 min, decreased to 10% B to 24.5 min and post time was 5.5 min. The column temperature was set at 30°C. The Agilent 6545 quadrupole time-of-flight mass spectrometer was performed in ESI + or ESI- mode with Dual AJS source. Details spectrometry conditions were shown in Supplementary Table S3. The acquired MS and MS/MS data were processed by Agilent MassHunter Workstation Software, containing Qualitative Analysis, Personal Compound database Libraries Manager and Molecular Structure Correlator.
Figure 1 depicts the removal of 17 benzodiazepines by UV irradiation and UV/H2O2 treatment in phosphate buffer solution at pH 7.0. Under direct UV photolysis, 97.8% of chlordiazepoxide was rapidly removed within 8 min. Clobazam and temazepam were degraded 42.6 and 47.5% within 20 min, respectively. For the other 14 benzodiazepines, the removal rates only ranged from 6.8 to 28.6% after 20 min of UV irradiation. Thus, the UV photolysis has a low potential to remove these benzodiazepines in water. Kosjek et al. (2012) also reported that oxazepam cannot be degraded in 90 min UV irradiation and only 8% of diazepam was degraded. The 17 benzodiazepines cannot be degraded by 100 μM H2O2 treatment within 20 min (data not shown). However, the removal of 17 benzodiazepines was dramatically enhanced by UV/H2O2 treatment. The removal rates of 17 benzodiazepines achieved 73.8–100% in 20 min UV/H2O2 treatment. This should be attributed to the high reactivity of hydroxyl radicals (·OH) towards benzodiazepines.
FIGURE 1. The removal of 17 benzodiazepines and p-chlorobenzoic acid (pCBA) by UV irradiation and UV/H2O2 treatment. Experimental conditions [Benzodiazepines]0 = 100 μg L−1, [pCBA]0 = 100 μg L−1, [H2O2]0 = 100 μM, pH = 7.0, E0p = 28.74 μE m−2 s−1.
The degradation of 17 benzodiazepines followed pseudo-first-order kinetics. The observed rate constants were calculated and are presented in Table 1 and Supplementary Figure S2.
Table 1 shows the
In order to compare the relative contributions of UV photolysis and ·OH reaction during UV/H2O2 treatment, the 17 benzodiazepines were grouped according to the specific value for kUVBZDs and k·OHBZDs. Supplementary Figure S2 shows the pseudo-first-order rate constants (kobs) for the reaction of 17 benzodiazepines by UV/H2O2 treatment. The observed fractions of kUVBZDs and k·OHBZDs were significantly different for 17 benzodiazepines. For chlordiazepoxide in the group I (k·OHBZDs/kUVBZDs <1), the direct UV photolysis played a dominant role in UV/H2O2 treatment. For temazepam, clobazam, clonazepam, prazepam, flunitrazepam, nitrazepam, bromazepam, diazepam, and oxazepam in the group II (1 < k·OHBZDs/kUVBZDs <10), both UV photolysis and ·OH reaction contributed to the degradation. For lorazepam, midazolam, nordiazepam, flurazepam, alprazolam, clozapine, and estazolam in the group III (k·OHBZDs/kUVBZDs >10), the ·OH reaction played a dominant role. The findings suggest that the treatment approach can be selected according to the occurrence of benzodiazepines in real water.
Experiments were performed to evaluate the effects of operational parameters and water quality on the degradation of a typical benzodiazepine, alprazolam, during UV/H2O2 treatment.
The degradation of alprazolam by UV irradiation, H2O2 oxidation and UV/H2O2 treatment in phosphate buffer at pH 7.0 is shown in Figure 2A. Alprazolam was not degraded by 100 μM H2O2 treatment. In the presence of direct UV irradiation, only 18.3% of alprazolam was removed within 20 min. The removal efficiency of alprazolam by UV/H2O2 treatment at a H2O2 concentration of 25 μM was up to 70.2% within 20 min. The apparent rate constant (kobs) of alprazolam increased from 1.0 × 10−3 to 5.5 × 10−3 s−1 when the H2O2 concentration increased from 25 to 200 μΜ. A linear relationship between kobs and H2O2 concentration (R2 = 0.99) was observed, which was due to the enhanced formation of ·OH at higher H2O2 concentrations. Similar results have been reported in degradation of oxytetracycline and tetracycline by UV/H2O2 treatment (Lopez-Penalver et al., 2010; Liu et al., 2016).
FIGURE 2. Effect of H2O2 dosage (A), solution pH (B) and inorganic ions (Cl−, HCO3−, NO3−) and humic acid (HA) (C) on the removal of alprazolam by UV/H2O2 treatment. Experimental conditions: [alprazolam]0 = 308.8 μg L−1, [H2O2]0 = 0–200 μM, pH = 3.0–7.0, [Cl−, HCO3−, NO3−]0 = 0–5 mM and [HA]0 = 0–5 mg/L, E0p = 28.74 μE m−2 s−1.
Figure 2B depicts the effect of solution pH on the alprazolam degradation by UV/H2O2 treatment in phosphate buffer at pH 7.0. Results showed that the degradation of alprazolam was inhibited at both acidic and alkaline conditions, and the highest kobs (3.27 × 10−3 s−1) was observed at pH 7.0. The inhibition at pH 3.0 and 5.0 might be attributed to the strong scavenging effect of H+, H2PO42−, and H2PO4− at acidic conditions (Kong et al., 2016). At alkaline conditions, the self-decomposition rate of H2O2 increased rapidly (Lee et al., 2020). In addition, hydroperoxide anion (HO2−) could consume ·OH and decreased the steady-state concentrations of ·OH (Tan et al., 2013; Liu et al., 2016).
Effects of anions (Cl−, HCO3−, NO3−) and humic acid (HA) that are ubiquitous in water and wastewater on the alprazolam degradation by UV/H2O2 treatment were evaluated. As shown in Figure 2C, adding Cl− (0–5 mM) has a negative impact on UV/H2O2 treatment. When 5 mM NaCl was added to aqueous solutions, the removal rate of alprazolam decreased by 16.7%. A possible reason was that ·OH could react with Cl− to form reactive chlorine species (Cl2−, Cl−, ClHO−), whose redox potentials are much lower than that of ·OH (Truong et al., 2004; Deng et al., 2013). The addition of HCO3− also affected the removal rate of alprazolam. With the increasing concentration of HCO3− (0–5 mM), the removal rate dropped gradually. HCO3− is a well-known scavenger of ·OH in solutions. HCO3− reacted with ·OH to produce carbonate radicals, which are more selective and less reactive towards organic contaminants than ·OH (Xiang et al., 2016; Yang et al., 2019). As exhibited, NO3− showed a negligible impact on the removal rate of alprazolam. With addition of 5 mM NO3− in aqueous solutions, the removal rate only decreased 8.7%. This result may be due to the low concentration level of NO3− was used in this study. Besides, NO3− may have a weak scavenging effect on ·OH. Previous studies reported that low concentration of NO3− had a slight impact on 1,4-dioxane degradation by UV/H2O2 treatment (Lee et al., 2020). The addition of humic acid (HA) had an obviously inhibitory effect on the removal rate of alprazolam. The removal rate reduced from 98.7 to 73.8% when HA was added from 0 to 5 mg/L in aqueous solutions. Generally, the HA is the main component of dissolved organic matter (DOM). It has a fast-quenching effect on ·OH and acts as a scavenger in UV/H2O2 treatment. HA can significantly impact the oxidative degradation of micropollutants through electrostatic interactions (Kim et al., 2017), photosensitization (Latifoglu and Gurol, 2003) and binding effects (Yang et al., 2021). Thus, the possible reasons for our findings were as follows: Firstly, HA can compete with alprazolam for ·OH reaction; Secondly, the high concentration of HA could impede the UV irradiation and reduce the ·OH generation (Xie et al., 2019; Yang et al., 2021).
Six degradation products of alprazolam during UV/H2O2 treatment were detected by quadrupole time-of-flight mass spectrometer. The experimental mass, proposed formula, MS/MS fragments are listed in Table 2, and their chromatographic and mass spectrogram are provided in Supplementary Figures S3–S6. The TP340-1 (m/z 341.0800) and TP340-2 (m/z 341.0800) were firstly reported as degradation products of alprazolam for UV/H2O2 treatment. Two degradation products with m/z 341.0800 were detected at different retention times 9.375 and 12.006 min, indicating the addition of ·OH group on the benzene ring. But the exact substitution position could not be confirmed by MS/MS fragments. The TP248, TP324-1, TP324-2, and TP324-3 have been reported in the removal of alprazolam by heterogeneous photocatalysis and photo-Fenton oxidation processes (Finčur et al., 2017; Jimenez et al., 2017; Mitsika et al., 2021). According to two characteristic fragment ions m/z 220.0271 and 179.0003 amu, TP248 (m/z 249.0534) might be generated from the removal of phenyl group in alprazolam and the simultaneous addition of a hydroxyl group. The TP324 (m/z 325.0864) was observed in three different retention times 10.215, 11.052, and 13.842 min, respectively. Thus, TP324 (m/z 325.0864) should be ·OH adding in different positions of alprazolam.
Based on the identification of the degradation products, the hydroxylation substitution reaction was the major pathway for alprazolam degradation during UV/H2O2 oxidation process. The ·OH is known as a reactive electrophilic reagent that reacts rapidly and non-selectively with most electron-rich sites of organic pollutants, mainly via H-atom abstraction, addition to olefins, and addition to aromatic compounds. As shown in Figure 3, the degradation of alprazolam could be divided into route A and route B. In route A, hydroxylation occurred at the initial of alprazolam degradation, producing hydroxylated alprazolam, TP324-1, TP324-2, and TP324-3. Further hydroxylation can form multi-hydroxylated byproducts TP340 (m/z 341.0800). In route B, the alprazolam (m/z 309.0919) might cleavage between seven number nitrogen-containing heterocycles and the benzene ring, corresponding to detach the phenyl group. Followed by hydroxylation, hydroxylated byproducts TP-248 (m/z 249.0534) was generated, according to the previous studies (Mitsika et al., 2021).
The degradation of 17 benzodiazepines by UV/H2O2 treatment was evaluated in the phosphate buffer solutions, river water (RW) and municipal WWTP effluent (WW), as shown in Figure 4. The kobs for the 17 benzodiazepines decreased from 26.9% (bromazepam) to 65.0% (prazepam) in RW and from 46.1% (chlordiazepoxide) to 84.0% (clobazam) in WW, compared to those in the phosphate buffer. The obvious inhibitory effect can be explained by the anions and dissolved organic matter in the RW and WW, which had been demonstrated according to Figure 2 and previous studies (Deng et al., 2013; Wang et al., 2017; Ribeiro et al., 2019). The WW had the higher DOC concentration (7.2 mg L−1), Cl− concentration (78.1 mg L−1), NO3− concentration (32.1 mg L−1), and alkalinity (2.03 mg L−1) than that of RW (4.2 mg L−1 DOC, 40.3 mg L−1 Cl−, 22.9 mg L−1 NO3−, and 1.28 mg L−1 alkalinity). Consequently, the benzodiazepine psychoactive drugs can be effectively removed by the UV/H2O2 treatment in real water.
FIGURE 4. The degradation kinetics of 17 benzodiazepines by UV/H2O2 treatment in the phosphate buffer solutions, river water (RW) and municipal WWTP effluent (WW). Experimental conditions [benzodiazepines]0 = 100 μg L−1, [H2O2]0 = 100 μM, pH = 7.0, E0p = 28.74 μE m−2 s−1.
The UV/H2O2 treatment dramatically enhanced the removal of benzodiazepines in aqueous solutions, compared to UV irradiation or H2O2 oxidation. This can be attributed to the high reactivity of ·OH reactivity towards benzodiazepines. The determined k·OHBZDs in phosphate buffer solutions at pH 7.0 ranged from 3.48 × 109 M−1 s−1 to 2.44 × 1010 M−1 s−1. The water quality parameters, such as H2O2 dosage, solution pH and co-existing species, had a significant effect on the removal of alprazolam by UV/H2O2 treatment. Six degradation products of alprazolam were detected. The hydroxylation was proposed to be the main reaction mechanism in UV/H2O2 treatment. This study demonstrated that UV/H2O2 treatment is capable of degrading benzodiazepines in natural waters.
The original contributions presented in the study are included in the article/Supplementary Material, further inquiries can be directed to the corresponding author.
W-DY: Formal analysis, Investigation, Writing–original draft. PY: Investigation. BY: Conceptualization, Writing–review and editing, Funding acquisition, Resources, Supervision. XL: Investigation. JF: Investigation. Z-TM: Investigation. J-LS: Writing–review and editing.
We would like to acknowledge the financial support from the National Natural Science Foundation of China (NSFC 41877358 and 21806043) and Scientific Research and Technology Development Program of Guangxi (2018AB36018). BY acknowledges the Pearl River Talent Plan of Guangdong Province (2017GC010244) and Guangdong Provincial Key Laboratory of Chemical Pollution and Environmental Safety (2019B030301008).
The authors declare that the research was conducted in the absence of any commercial or financial relationships that could be construed as a potential conflict of interest.
All claims expressed in this article are solely those of the authors and do not necessarily represent those of their affiliated organizations, or those of the publisher, the editors and the reviewers. Any product that may be evaluated in this article, or claim that may be made by its manufacturer, is not guaranteed or endorsed by the publisher.
The Supplementary Material for this article can be found online at: https://www.frontiersin.org/articles/10.3389/fenvs.2021.764841/full#supplementary-material
Bosio, M., Satyro, S., Bassin, J. P., Saggioro, E., and Dezotti, M. (2019). Removal of pharmaceutically active compounds from synthetic and real aqueous mixtures and simultaneous disinfection by supported TiO2/UV-A, H2O2/UV-A, and TiO2/H2O2/UV-A processes. Environ. Sci. Pollut. Res. 26 (5), 4288–4299. doi:10.1007/s11356-018-2108-x
Brodin, T., Fick, J., Jonsson, M., and Klaminder, J. (2013). Dilute Concentrations of a Psychiatric Drug Alter Behavior of Fish from Natural Populations. Science 339 (6121), 814–815. doi:10.1126/science.1226850
Canonica, S., Meunier, L., and von Gunten, U. (2008). Phototransformation of selected pharmaceuticals during UV treatment of drinking water. Water Res. 42 (1-2), 121–128. doi:10.1016/j.watres.2007.07.026
Carpinteiro, I., Rodil, R., Quintana, J. B., and Cela, R. (2017). Reaction of diazepam and related benzodiazepines with chlorine. Kinetics, transformation products and in-silico toxicological assessment. Water Res. 120, 280–289. doi:10.1016/j.watres.2017.04.063
Cerveny, D., Brodin, T., Cisar, P., McCallum, E., and Fick, J. (2020). Bioconcentration and behavioral effects of four benzodiazepines and their environmentally relevant mixture in wild fish. Sci. Total Environ. 702, 134780. doi:10.1016/j.scitotenv.2019.134780
Cunha, D. L., de Araujo, F. G., and Marques, M. (2017). Psychoactive drugs: occurrence in aquatic environment, analytical methods, and ecotoxicity-a review. Environ. Sci. Pollut. Res. 24 (31), 24076–24091. doi:10.1007/s11356-017-0170-4
Cunha, D. L., Kuznetsov, A., Araujo, J. R., Neves, R. S., Archanjo, B. S., Canela, M. C., et al. (2019). Optimization of Benzodiazepine Drugs Removal from Water by Heterogeneous Photocatalysis Using TiO2/Activated Carbon Composite. Water Air Soil Pollut. 230 (7), 141. doi:10.1007/s11270-019-4202-1
Danner, M.-C., Robertson, A., Behrends, V., and Reiss, J. (2019). Antibiotic pollution in surface fresh waters: Occurrence and effects. Sci. Total Environ. 664, 793–804. doi:10.1016/j.scitotenv.2019.01.406
Deng, J., Shao, Y., Gao, N., Xia, S., Tan, C., Zhou, S., et al. (2013). Degradation of the antiepileptic drug carbamazepine upon different UV-based advanced oxidation processes in water. Chem. Eng. J. 222, 150–158. doi:10.1016/j.cej.2013.02.045
Fernández-Rubio, J., Rodríguez-Gil, J. L., Postigo, C., Mastroianni, N., López de Alda, M., Barceló, D., et al. (2019). Psychoactive pharmaceuticals and illicit drugs in coastal waters of North-Western Spain: Environmental exposure and risk assessment. Chemosphere 224, 379–389. doi:10.1016/j.chemosphere.2019.02.041
Fick, J., Brodin, T., Heynen, M., Klaminder, J., Jonsson, M., Grabicova, K., et al. (2017). Screening of benzodiazepines in thirty European rivers. Chemosphere 176, 324–332. doi:10.1016/j.chemosphere.2017.02.126
Finčur, N. L., Krstić, J. B., Šibul, F. S., Šojić, D. V., Despotović, V. N., Banić, N. D., et al. (2017). Removal of alprazolam from aqueous solutions by heterogeneous photocatalysis: Influencing factors, intermediates, and products. Chem. Eng. J. 307, 1105–1115. doi:10.1016/j.cej.2016.09.008
Huang, Y., Kong, M., Coffin, S., Cochran, K. H., Westerman, D. C., Schlenk, D., et al. (2020). Degradation of contaminants of emerging concern by UV/H2O2 for water reuse: Kinetics, mechanisms, and cytotoxicity analysis. Water Res. 174, 115587. doi:10.1016/j.watres.2020.115587
Huber, M. M., Canonica, S., Park, G.-Y., and von Gunten, U. (2003). Oxidation of pharmaceuticals during ozonation and advanced oxidation processes. Environ. Sci. Technol. 37 (5), 1016–1024. doi:10.1021/es025896h
Jiménez, J. J., Sánchez, M. I., Muñoz, B. E., and Pardo, R. (2017). Persistence of alprazolam in river water according to forced and non-forced degradation assays: adsorption to sediment and long-term degradation products. Drug Test. Anal. 9 (8), 1204–1213. doi:10.1002/dta.2148
Kalichak, F., Idalencio, R., Rosa, J. G. S., Oliveira, T. A. d., Koakoski, G., Gusso, D., et al. (2016). Waterborne psychoactive drugs impair the initial development of Zebrafish. Environ. Toxicol. Pharmacol. 41, 89–94. doi:10.1016/j.etap.2015.11.014
Kim, M.-K., Won, A.-Y., and Zoh, K.-D. (2017). Effects of molecular size fraction of DOM on photodegradation of aqueous methylmercury. Chemosphere 174, 739–746. doi:10.1016/j.chemosphere.2017.02.033
Kong, X., Jiang, J., Ma, J., Yang, Y., Liu, W., and Liu, Y. (2016). Degradation of atrazine by UV/chlorine: Efficiency, influencing factors, and products. Water Res. 90, 15–23. doi:10.1016/j.watres.2015.11.068
Kosjek, T., Perko, S., Zupanc, M., Zanoški Hren, M., Landeka Dragičević, T., Žigon, D., et al. (2012). Environmental occurrence, fate and transformation of benzodiazepines in water treatment. Water Res. 46 (2), 355–368. doi:10.1016/j.watres.2011.10.056
Lado Ribeiro, A. R., Moreira, N. F. F., Li Puma, G., and Silva, A. M. T. (2019). Impact of water matrix on the removal of micropollutants by advanced oxidation technologies. Chem. Eng. J. 363, 155–173. doi:10.1016/j.cej.2019.01.080
Latifoglu, A., and Gurol, M. D. (2003). The effect of humic acids on nitrobenzene oxidation by ozonation and O3/UV processes. Water Res. 37 (8), 1879–1889. doi:10.1016/S0043-1354(02)00583-3
Lee, C.-S., Venkatesan, A. K., Walker, H. W., and Gobler, C. J. (2020). Impact of groundwater quality and associated byproduct formation during UV/hydrogen peroxide treatment of 1,4-dioxane. Water Res. 173, 115534. doi:10.1016/j.watres.2020.115534
Lei, H.-J., Yang, B., Ye, P., Yang, Y.-Y., Zhao, J.-L., Liu, Y.-S., et al. (2021). Occurrence, fate and mass loading of benzodiazepines and their transformation products in eleven wastewater treatment plants in Guangdong province, China. Sci. Total Environ. 755 (Pt 2), 142648. doi:10.1016/j.scitotenv.2020.142648
Liu, Y., He, X., Fu, Y., and Dionysiou, D. D. (2016). Degradation kinetics and mechanism of oxytetracycline by hydroxyl radical-based advanced oxidation processes. Chem. Eng. J. 284, 1317–1327. doi:10.1016/j.cej.2015.09.034
Lopez, A., Bozzi, A., Mascolo, G., and Kiwi, J. (2003). Kinetic investigation on UV and UV/H2O2 degradations of pharmaceutical intermediates in aqueous solution. J. Photochem. Photobiol. A-chem. 156 (1-3), 121–126. doi:10.1016/S1010-6030(02)00435-5
López-Peñalver, J. J., Sánchez-Polo, M., Gómez-Pacheco, C. V., and Rivera-Utrilla, J. (2010). Photodegradation of tetracyclines in aqueous solution by using UV and UV/H2O2 oxidation processes. J. Chem. Technol. Biotechnol. 85 (10), 1325–1333. doi:10.1002/jctb.2435
Lu, J., Wu, J., and Wang, J. (2022). Metagenomic analysis on resistance genes in water and microplastics from a mariculture system. Front. Environ. Sci. Eng. 16 (1), 13. doi:10.1007/s11783-021-1438-y
Lu, J., Zhang, Y., Wu, J., Wang, J., and Cai, Y. (2020). Fate of antibiotic resistance genes in reclaimed water reuse system with integrated membrane process. J. Hazard. Mater. 382, 121025. doi:10.1016/j.jhazmat.2019.121025
Miklos, D. B., Remy, C., Jekel, M., Linden, K. G., Drewes, J. E., and Hübner, U. (2018). Evaluation of advanced oxidation processes for water and wastewater treatment - A critical review. Water Res. 139, 118–131. doi:10.1016/j.watres.2018.03.042
Minakata, D., Li, K., Westerhoff, P., and Crittenden, J. (2009). Development of a Group Contribution Method to Predict Aqueous Phase Hydroxyl Radical (HO) Reaction Rate Constants. Environ. Sci. Technol. 43 (16), 6220–6227. doi:10.1021/es900956c
Minakata, D., Mezyk, S. P., Jones, J. W., Daws, B. R., and Crittenden, J. C. (2014). Development of linear free energy relationships for aqueous phase radical-involved chemical reactions. Environ. Sci. Technol. 48 (23), 13925–13932. doi:10.1021/es504491z
Mitsika, E. E., Christophoridis, C., Kouinoglou, N., Lazaridis, N., Zacharis, C. K., and Fytianos, K. (2021). Optimized Photo-Fenton degradation of psychoactive pharmaceuticals alprazolam and diazepam using a chemometric approach-Structure and toxicity of transformation products. J. Hazard. Mater. 403, 123819. doi:10.1016/j.jhazmat.2020.123819
Ouédraogo, M., Maesschalck, E. D., Soentjens-Werts, V., and Dubois, J. (2009). In vitrocytotoxicity study of oxaziridines generated after chlordiazepoxide, demoxepam, and desmethylchlordiazepoxide UV irradiation. Drug Chem. Toxicol. 32 (4), 417–423. doi:10.1080/01480540903130666
Patel, M., Kumar, R., Kishor, K., Mlsna, T., Pittman, C. U., and Mohan, D. (2019). Pharmaceuticals of Emerging Concern in Aquatic Systems: Chemistry, Occurrence, Effects, and Removal Methods. Chem. Rev. 119 (6), 3510–3673. doi:10.1021/acs.chemrev.8b00299
Rivetti, C., Campos, B., and Barata, C. (2016). Low environmental levels of neuro-active pharmaceuticals alter phototactic behaviour and reproduction in Daphnia magna. Aquat. Toxicol. 170, 289–296. doi:10.1016/j.aquatox.2015.07.019
Romeiro, A., Freitas, D., Emília Azenha, M., Canle, M., and Burrows, H. D. (2017). Effect of the calcination temperature on the photocatalytic efficiency of acidic sol-gel synthesized TiO2nanoparticles in the degradation of alprazolam. Photochem. Photobiol. Sci. 16 (6), 935–945. doi:10.1039/c6pp00447d
Subedi, B., and Kannan, K. (2015). Occurrence and fate of select psychoactive pharmaceuticals and antihypertensives in two wastewater treatment plants in New York State, USA. Sci. Total Environ. 514, 273–280. doi:10.1016/j.scitotenv.2015.01.098
Tan, C., Gao, N., Deng, Y., Zhang, Y., Sui, M., Deng, J., et al. (2013). Degradation of antipyrine by UV, UV/H2O2 and UV/PS. J. Hazard. Mater. 260, 1008–1016. doi:10.1016/j.jhazmat.2013.06.060
Truong, G. L., Laat, J. D., and Legube, B. (2004). Effects of chloride and sulfate on the rate of oxidation of ferrous ion by H2O2. Water Res. 38 (9), 2384–2394. doi:10.1016/j.watres.2004.01.033
Wang, C., Hou, L., Li, J., Xu, Z., Gao, T., Yang, J., et al. (2017). Occurrence of diazepam and its metabolites in wastewater and surface waters in Beijing. Environ. Sci. Pollut. Res. 24 (18), 15379–15389. doi:10.1007/s11356-017-8922-8
Wang, J., and Zhuan, R. (2020). Degradation of antibiotics by advanced oxidation processes: An overview. Sci. Total Environ. 701, 135023. doi:10.1016/j.scitotenv.2019.135023
Wu, J., Sun, Q., and Lu, J. (2020). Synthesis of magnetic core-shell Fe3O4@SiO2@Mg(OH)2 composite using waste bischofite and its catalytic performance for ozonation of antibiotics. J. Environ. Chem. Eng. 8 (5), 104318. doi:10.1016/j.jece.2020.104318
Wu, M., Xiang, J., Que, C., Chen, F., and Xu, G. (2015). Occurrence and fate of psychiatric pharmaceuticals in the urban water system of Shanghai, China. Chemosphere 138, 486–493. doi:10.1016/j.chemosphere.2015.07.002
Xiang, Y., Fang, J., and Shang, C. (2016). Kinetics and pathways of ibuprofen degradation by the UV/chlorine advanced oxidation process. Water Res. 90, 301–308. doi:10.1016/j.watres.2015.11.069
Xie, X., Guo, H., Yan, M., and Korshin, G. (2019). Interactions between natural organic matter (NOM) and the cationic dye toluidine blue at varying pHs and ionic strengths: Effects of NOM charges and Donnan gel potentials. Chemosphere 236, 124272. doi:10.1016/j.chemosphere.2019.07.003
Yang, B., Kookana, R. S., Williams, M., Du, J., Doan, H., and Kumar, A. (2016). Removal of carbamazepine in aqueous solutions through solar photolysis of free available chlorine. Water Res. 100, 413–420. doi:10.1016/j.watres.2016.05.048
Yang, B., Peng, T., Cai, W.-W., and Ying, G.-G. (2020). Transformation of diazepam in water during UV/chlorine and simulated sunlight/chlorine advanced oxidation processes. Sci. Total Environ. 746, 141332. doi:10.1016/j.scitotenv.2020.141332
Yang, B., Wang, C., Cheng, X., Zhang, Y., Li, W., Wang, J., et al. (2021). Interactions between the antibiotic tetracycline and humic acid: Examination of the binding sites, and effects of complexation on the oxidation of tetracycline. Water Res. 202, 117379. doi:10.1016/j.watres.2021.117379
Yang, B., Xu, C., Kookana, R. S., Williams, M., Du, J., Ying, G., et al. (2018). Aqueous chlorination of benzodiazepines diazepam and oxazepam: Kinetics, transformation products and reaction pathways. Chem. Eng. J. 354, 1100–1109. doi:10.1016/j.cej.2018.08.082
Yang, Y., Cao, Y., Jiang, J., Lu, X., Ma, J., Pang, S., et al. (2019). Comparative study on degradation of propranolol and formation of oxidation products by UV/H2O2 and UV/persulfate (PDS). Water Res. 149, 543–552. doi:10.1016/j.watres.2018.08.074
Keywords: benzodiazepines, UV/H2O2, degradation products, water treatment, reaction kinetics, emerging chemical pollutants
Citation: You W-D, Ye P, Yang B, Luo X, Fang J, Mai Z-T and Sun J-L (2021) Degradation of 17 Benzodiazepines by the UV/H2O2 Treatment. Front. Environ. Sci. 9:764841. doi: 10.3389/fenvs.2021.764841
Received: 26 August 2021; Accepted: 04 October 2021;
Published: 19 October 2021.
Edited by:
Xiuping Zhu, Louisiana State University, United StatesReviewed by:
Jun Chen, Pearl River Hydraulic Research Institute, ChinaCopyright © 2021 You, Ye, Yang, Luo, Fang, Mai and Sun. This is an open-access article distributed under the terms of the Creative Commons Attribution License (CC BY). The use, distribution or reproduction in other forums is permitted, provided the original author(s) and the copyright owner(s) are credited and that the original publication in this journal is cited, in accordance with accepted academic practice. No use, distribution or reproduction is permitted which does not comply with these terms.
*Correspondence: Bin Yang, YmluLnlhbmdAbS5zY251LmVkdS5jbg==
Disclaimer: All claims expressed in this article are solely those of the authors and do not necessarily represent those of their affiliated organizations, or those of the publisher, the editors and the reviewers. Any product that may be evaluated in this article or claim that may be made by its manufacturer is not guaranteed or endorsed by the publisher.
Research integrity at Frontiers
Learn more about the work of our research integrity team to safeguard the quality of each article we publish.