- 1Accounting School, Nanfang College, Guangzhou, Guangzhou, China
- 2Higher Education Impact Assessment Center, Nanfang College, Guangzhou, Guangzhou, China
- 3School of Public Administration, Nanfang College, Guangzhou, Guangzhou, China
- 4School of Literature and Communication, Nanfang College, Guangzhou, Guangzhou, China
Carbon neutrality, or “net zero”, has become the impact assessment project of human impact on Earth, increasingly structured to examine the implications, for the environment and people, of proposed actions and consequences of inaction. International and local collaboration efforts have been made with the aim of achieving carbon neutrality or “net-zero” emissions; thus, policies and technological innovations have been developed. Such impact-oriented risk assessment and control efforts amount to carbon-neutral pathways. Although such pathways may diverge in terms of energy, resources, and cost, it is critical to summarize essential and promising preparatory work on related policies and technologies to inform both policy-makers and social scientists to take actions. Through a scientometric analysis and systematic review of the latest social science literature, the study identified the size, scope and exemplar work for each social science discipline on carbon neutrality, based on 907 articles collected in early 2021 from the Web of Science database. This study reveals a set of disciplines focusing on certain common and distinct aspects of carbon neutrality. By outlining the possibilities and application areas for future research and policy development for socio-technical transition towards a net-zero or post-carbon future, this study has contributed to the understanding of the global efforts to achieve a clearer and viable carbon-neutral pathway. In conclusion, as many aspects of the planet and humans have become datafied, digitized, and networked, carbon neutrality, as the ecological indicator that guide human production and consumption patterns, must take on a central role in guiding our conscious green digital transformation of many political, economic, social and psychological aspects of our societies according to the existing and emerging social science knowledge.
Introduction
To prevent global warming, an urgent threat to life on Earth, achieving carbon neutrality has become an important goal that is pursued by various organizations across the world, with the ultimate aim of measuring and controlling direct and indirect emissions of carbon dioxide (CO2) and other greenhouse gasses (GHG), such as methane (CH4) and nitrous oxide (N2O) (Smith and Fletcher, 2020). When governments adopted the Paris Agreement in 2015 at the 21st Conference of the Parties (COP21) in the United Nations Framework Convention on Climate Change (UNFCCC), the Intergovernmental Panel on Climate Change (IPCC) was requested to publish a report. The report was later released in 2018 and was titled, “Global Warming of 1.5°C, an IPCC special report on the impacts of global warming of 1.5°C above pre-industrial levels and related global greenhouse gas emission pathways, in the context of strengthening the global response to the threat of climate change, sustainable development, and efforts to eradicate poverty” (IPCC, 2018). In this report, scientific findings regarding the potential impacts and associated risks of global warming were summarized, with language phrases such as “to neutralize emissions,” “carbon neutrality,” etc. In short, neutralizing the emissions of greenhouse gases caused by human activities [“the root cause of global warming” (IPCC, 2018)] requires both robust scientific understanding of the impacts of global warming on both natural and human systems and collective action on strengthening and implementing the global response. In 2020, China and the European Union (EU), two of the world’s top emitters, pledged to become carbon neutral by 2060 and 2050, respectively (Harvey, 2020), by invoking policies to move the economy towards sustainability (Mallapaty, 2020). Japan also has made a similar pledge for the date of 2050 (METI, 2020). Major companies such as Apple, Amazon, IBM, Microsoft, BP, Ford, General Motors, and FedEx have also pledged to become carbon neutral. Companies’ disclosures of their Greenhouse Gas (GHG) emissions also highlight the vital role of companies in mitigating the effects of global warming, which requires cost-effective and verifiable accounting for emissions (Downie and Stubbs, 2013; Diniz et al., 2021). These carbon neutrality pledges made by major political and economic entities demonstrate the widespread commitment to galvanize action on the climate crisis. Since the pathways used to achieve carbon neutrality require a viable socio-technical transition from industrial processes based on petroleum, coal, and gasoline towards a more environmentally friendly sustainable future (Wang et al., 2020), it is thus essential to focus on the political, economic, social, and psychological aspects of carbon neutrality.
Indeed, the multidisciplinary nature of carbon neutrality goes beyond science and technology and involves issues such as policies, investment, communication, behavior change and adaption, and international relations (Hawken, 2017; Farnworth, 2018). For instance, combining social science knowledge and artificial intelligence techniques can advance innovations, as exemplified by a promising research agenda on transnational industry cooperation (TIC) and transnational university cooperation (TUC) innovations, especially in the context of EU–China science, technology, and innovation cooperation (Cai et al., 2019). In another example, efforts to contribute to a sustainable transition have encountered reactions from entities with vested interests, such as the petroleum and coal industry, often in political lobby activities and misinformation campaigns or “green washing” efforts (Smith and Fletcher, 2020). Ten social, economic and legal insights have also been summarized in climate science research since mid-2019, highlighting the need for advancing knowledge about the interactions between the human world and planetary systems (Pihl et al., 2021). New methods and data are needed to support monitoring and decision-making systems, such as using remote sensing big data for more precise and efficient forest resource management and policy-making (Wang et al., 2021). Thus, to achieve carbon neutrality, social science knowledge must be developed to understand the multiple aspects of preparing human societies for a sustainable transition for the mitigation of climate change. By informing decision-makers and collective actions, such knowledge will help researchers and policy-makers design and implement policies and technologies that work in initiating and implementing individual, organizational, and social changes. This explains the rationales behind global initiatives such as bringing transparency and rigor to the voluntary carbon market (Twidale, 2021) and research that connects mortality with carbon emissions under the notion of “the mortality cost of carbon” (Bressler, 2021; Schwartz, 2021). Social science knowledge matters.
To the best knowledge of the authors, based on our research and analysis of related literature, there has so far been no systematic review of social science knowledge on carbon neutrality using scientometric or bibliometric methods, whereas such bibliometric analysis on the wider and more developed topic such as circular economy have be conducted (e.g., Cui and Zhang, 2018; Meseguer-Sánchez et al., 2021). An updated understanding of carbon neutrality social science literature focusing on the relationship between policies and technologies is expected to be useful in informing the debates and decisions surrounding the goal of achieving carbon neutrality, along with diverse stakeholders across different industrial sectors and knowledge domains. Such an understanding will not only help to guide the urgent policy, research, and technology innovations with an initial road map, but also identify the challenges and opportunities in the design and application of relevant policies and technologies. To gain both general and specific social knowledge, it is important to provide a systematic review of carbon neutrality literature to summarize how social science knowledge can contribute to the worldwide collective efforts to achieve carbon neutrality.
The purpose of this paper is to provide a review of social science literature on the topic of carbon neutrality, asking the following research questions:
1) When has the intellectual structure of the knowledge base developed, and by whom?
2) How and when has the conceptual structure of the knowledge base developed?
3) Where and how has the social structure of the knowledge base developed?
4) Where and what has been contributed from the social science disciplines?
To answer the above questions regarding the overall contribution of social sciences to the topic of carbon neutrality or decarbonization, a scientometric and systematic review was conducted based on Clarivate Analytics’ Web of Science (WoS) database using science mapping tools, as detailed in the next section. By answering these descriptive, analytical, and reflective questions, this paper aims to contribute to the existing human knowledge on the topic of carbon neutrality by focusing on the socio-technical pathways that social science knowledge has already contributed and ought to contribute.
Materials and Methods
Science mapping (Cobo et al., 2011) and scoping review mapping (Fridell et al., 2019) aim to use the visualization (Small, 1999) of “research fronts” (Garfield, 1994), knowledge structure, or intellectual bases (Persson, 1994) to gain insight. For such visualization outcomes to be meaningful, especially for the research questions raised above, a careful research design is needed to analyze social science knowledge in various “research fronts” that are accessible for both policy makers and researchers.
Science Mapping Approach
Our science mapping research, which aims to facilitate theoretical and empirical development across the main and emerging social sciences disciplines, has conducted a scientometric analysis on the topic of carbon neutrality. The ultimate objective of this paper is to provide a holistic and systematic overview of the social science research of carbon neutrality worldwide over the years to shed light on carbon neutrality policies and technologies. Instead of examining a specific policy or technology, the scientometric approach, along with its bibliometric methods and data, can provide a valuable overall picture that allows researchers across different disciplines to understand the composition and structure of the intellectual knowledge base of carbon neutrality. To this end, this paper used quantitative science mapping techniques to categorize and visualize the disciplinary venues and topical focus of carbon neutrality research and then complemented it with brief traditional research reviews of selected articles that are relevant to the overall discussions of carbon neutrality policies and technologies.
Raw Materials
Clarivate Analytics’ Web of Science (WoS) database was used, along with the WoS Core Collection of SCI-Expanded, SSCI, A&HCI, and ESCI indices, to collect the bibliometric data for analysis in this review. It should be noted that SCI-Expanded and ESCI journals were included. Some journals are indexed in more than one index, for instance, one of the top journals, Energy Policy, is indexed both by SCI-Expanded and SSCI.
Web of Science offers a subject categorization scheme called “research areas” that covers five broad disciplinary categories (Clarivate Analytics, 2020):
• Arts and Humanities,
• Life Sciences and Biomedicine,
• Physical Sciences,
• Social Sciences,
• Technology.
As one important dimension of the research fronts is “a posteriori constructs that provide both highly specific and broad access to the subject matter” (Garfield, 1994), the WoS research area’s social sciences category contains 25 disciplines ranging from archaeology to women’s studies. Such categorization has been assigned by the WoS experts at the levels of journals and books. Thus, the WoS research areas are features at the level of journals. Given the multi-disciplinary nature of carbon neutrality, it is important to examine the disciplinary features of the literature by not limiting our scope of search to the social-science-only index such as SSCI.
In terms of the document type, this review examined articles, book chapters, and reviews. Although the WoS does provide conference proceedings such as CPCI, this review does not include them because the quality and quantity of such proceedings may differ widely across various social science disciplines. By focusing on journal articles and books, this review should provide a more succinct picture of the overall knowledge base of the carbon neutrality literature.
In terms of topics, a series of synonyms of carbon neutrality, along with its related topics of carbon accounting and decarbonization, were chosen. The assumption is that the use of these terms in the title, abstract, or keywords of work indicates the authors’ intention to discuss, at the very least, topics such as carbon footprints or decarbonization, which have implications on reaching carbon neutrality.
The overall research design consists of a query design, a data process, and a taxonomy design to explore the policies and technologies that contribute to the goal of carbon neutrality, with a focus on the work across social science disciplines.
Query Design
The query design consists mainly of two parts: topics and disciplines. As shown in the query below, the first part aims to cover the relevant literature, which is expressed by the field tag of “Topic” (i.e., TS) and a series of synonyms and underlying topics of carbon neutrality that are joined by “OR” Boolean operators.
• TS = (“carbon neutrality” OR “carbon neutral*” OR “carbon-neutral*” OR “carbon positive*” OR “carbon-positive*” OR “carbon negative*” OR “carbon-negative*” OR “carbon accounting” OR “net-zero” OR “decarboni?ation”) AND SU = (“Social Sciences” OR “Archaeology” OR “Area Studies” OR “Biomedical Social Sciences” OR “Business and Economics” OR “Communication” OR “Criminology and Penology” OR “Cultural Studies” OR “Demography” OR “Development Studies” OR “Education and Educational Research” OR “Ethnic Studies” OR “Family Studies” OR “Geography” OR “Government and Law” OR “International Relations” OR “Linguistics” OR “Mathematical Methods In Social Sciences” OR “Psychology” OR “Public Administration” OR “Social Issues” OR “Social Sciences Other Topics” OR “Social Work” OR “Sociology” OR “Urban Studies” OR “Women’s Studies”)
Similarly, the second part consists of the field tag of “Research Area” (i.e., SU) and a set of disciplines under the WoS research area’s social sciences category. The two parts of the “Topic” query and “Research Area” query were joined by an “AND” Boolean operator, which should give us initial database search results containing social science literature on carbon neutrality.
It should be noted that the series of synonyms have been gathered through an iterative snowballing process that begins with the baseline terms of decarbonization and carbon neutrality. Based on the top keywords of the initial datasets, closely-related terms such as “net-zero” and “carbon accounting” terminologies (e.g., those containing negative and positive sides) have been included in the search query. Other more detailed methods or terms were not included because the purpose of research aims to focus first on the comprehensive overview of the carbon neutrality literature, and thus adding terms from specific subfields or subdomains may render such comprehensive coverage unbalanced. This component of research design will be further discussed later in the Conclusion section as the trade-off between comprehensiveness and depth.
Data Processes
In accordance with the PRISMA (Preferred Reporting Items for Systematic Reviews and Meta-Analyses) guidelines (Moher et al., 2009), the screened resources for the study consist of searched articles indexed by Clarivate Analytics’ SCI-EXPANDED, SSCI, A&HCI, and ESCI indices for articles published in reliable academic sources. Our initial database searching resulted in 918 articles, which was collected on February 1, 2021. Bibliographic data (the “Full Record and Citation References” option was selected) of the documents were downloaded for further analysis, along with “meta data” such as the article title, author name(s), organization affiliations, keywords, abstracts, publication source, reference citations, and various data.
Second, during the eligibility phase, we first decided to examine the literature written in the English language, leaving out 11 articles. The 907 articles found constitute the basis of this scientometric analysis. We then decided to examine the full-text articles of the most relevant ones, for further qualitative synthesis, in terms of 1) the number of citations received and 2) the most recently published. The selection criteria reflect the research inquiries on 1) knowledge bases and 2) emerging research. For each discipline, we identified the most cited and most recent documents, then the documents were examined based on the relevance of their contribution to the carbon neutrality social science literature. More classic literature review work was conducted on the selected work across different social science disciplines.
Third, during the final “included phase” of the PRISMA, data preparation work was conducted to provide more meaningful insights. Via iterative processes of three experts’ work, two thesaurus files were compiled for both keywords and publication sources to disambiguate concepts and entities. For example, author keywords such as “climate change policy” and “climate policy frameworks” were replaced by the more frequently used term “climate policy”. Different volumes of book sources (e.g., Annual Review of Resource Economics) were replaced by the common name without volume numbers. Such data preparation work facilitated more accurate science mapping (van Eck and Waltman, 2014). These thesaurus files are essential ground work for scientometric methods such as keyword co-occurrence analysis because the preprocessing work can remove duplicate terms and expressions in a more standardized consistent fashion.
To implement scientometric analysis, the data sets were processed by VOSviewer (Yu et al., 2020) Bibliometrix (Aria and Cuccurullo, 2017) and Python data science packages to gain insight. In particular, the use of the Python Data Analysis Library (a.k.a. pandas) allowed the “split-apply combine strategy” (Wickham, 2011) to be implemented to break up the searched outcomes into manageable pieces (e.g., the WoS disciplines of “research areas”, topics, sources, etc.) and then explore how these pieces constitute the whole. Based on the “split-apply-combine strategy”, the largely quantitative data analysis based on a series of combined descriptive statistics, scientometric analyses, or social network analyses of scientometric relationships aimed to provide the empirical structure of the knowledge base.
The statistical network analysis was conducted using the Network Analysis in Python (a.k.a. NetworkX) library, resulting in network centrality metrics (i.e., closeness centrality, eigenvector centrality, and betweenness centrality) and clustering coefficient (Onnela et al., 2005). Although these advanced metrics are not often reported in full by bibliometric analysis (e.g. Cui and Zhang, 2018; Meseguer-Sánchez et al., 2021), the study will report them as complementary verification of the visualization outcomes. Based on the network analysis conventions (Freeman, 1978; Hansen et al., 2020), the centrality measures consists of closeness centrality (how close a node is to the rest of the nodes), eigenvector centrality (how central a node is based on its neighbors), and betweenness centrality (how central a node is in bridging different segments of the network). In addition, different from these centrality measures, the clustering coefficient metric measures the density of the 1.5-degree egocentric network for each node, indicating how connected the connected nodes are. These metrics can then complement and verify the observations of network visualization. Following the bibliometric analysis conventions, the number of clusters are determined by human trial-and-error for meaningful and succinct algorithm-based grouping outcomes for the authors. Such clustering outcomes are reproducible with a set of parameters, but other outcomes can be produced for fewer or more groups.
CiteSpace (Chen, 2016) was also used—mainly for creating the citation burst tables—with the aim to see which topics had gained significantly more attention in recent years or over certain periods of time. This can complement the time-related findings as part of a more holistic understanding over time (Chen, 2004).
Scientometric Methods to Show the Structure of Knowledge Base
This study implements several scientometric methods to determine the overall intellectual, conceptual, and social structure of the knowledge base (Donthu et al., 2021), complemented by systematic review of the top-cited work from the social science disciplinary perspective.
To answer the first question regarding the intellectual structure, the scientometric methods of historiograph and author-co-citation analysis have been implemented to reveal the clusters of organizational and individual authors have been contributed to the main knowledge base. Since published work can accumulate citations over time, the citation approach (Small, 1999) can provide an accurate mapping of clusters of items (e.g., documents, sources, etc.). Based on the most prominent direct citations, the historiograph maps out the intellectual links in a chronological order, revealing the basic works in the dataset. The author co-citation analysis visualizes the relationship network among cited work as the fundamental sources.
To answer the second question on the conceptual structure, the scientometric methods of keyword co-occurrence, keywords with strongest citation bursts, and a series of thematic maps have been conducted to reveal the clusters of ideas and their dynamic evolutions over different time periods. Keyword co-occurrence maps show the existing relationship among topics, and the advanced methods of thematic maps and keywords with citation bursts provide additional temporal insights to the evolution of conceptual structure.
To answer the third question on the social structure, the scientometric methods of bibliometric coupling analysis (Jarneving, 2007; Boyack and Klavans, 2010) have been applied to the journal, discipline, organization, and country information of the bibliometric data. Previous work has also shown that insights into clusters of disciplines can be derived based on WoS disciplinary information (Chen and Liao, 2020).
To answer the last question on the social science contribution, the study uses the bibliometric data provided by the WoS’ subject categorization scheme’s social sciences category. Thus, the disciplinary landscape will be shown according to, and based upon, such a categorization assignment.
Altogether, the scientometric analysis of carbon neutrality social science literature is expected to show the knowledge base as documented and cited in the empirical evidence of academic publications.
Results
The findings of the study are organized into four sections, moving from the main structural work to the emerging peripheral work, with the purpose to cover both the common and distinct features of the social science knowledge on the topic. The first three sections demonstrate the intellectual, conceptual, and social structure of the knowledge base, and the last section samples the main work across various social science sub-disciplines.
Table 1 lists basic information about the dataset, with its size, descriptive statistics, contents, author and author collaboration numbers. It has 907 documents over the timespan of 1983–2021.
For the purpose of examining policy implications, the years of 2015 and 2020 were chosen based on the Paris Agreement in December 2015. The first time period (1983–2015, N = 310) marks the early period before the Paris Agreement which includes the Kyoto Protocol adopted in December 2012. The second time period (2016–2019, N = 382) covers the most of the 5-years circle of the global climate action. The main reason for the third time period (2020–2021, N = 215) is to explore the emerging knowledge by comparisons. Note that the number of publications is relatively even for each period, ranging from 215 to 382.
Intellectual Structure
To explore the intellectual structure of the knowledge base, the study begins with a historiography and an author co-citation network, thereby describing the most cited work and its authors (including the organization authors). The findings should provide some descriptions that may amount to the summary of the main schools of thought, and their shared and divergent concepts/issues. Indeed, for instance, the main individual and organization authors have evolved mostly from European and international organization actors, as evidenced by findings below.
Historiograph
Figure 1 shows the bibliometric historiograph by using science mapping tool bibliometrix (Aria and Cuccurullo, 2017). Such mapping first creates the historical direct citation network from the most-cited work and then visualizes the network in a chronological order (Garfield, 2004). The following subsections will describe these networks, from bottom to the top.
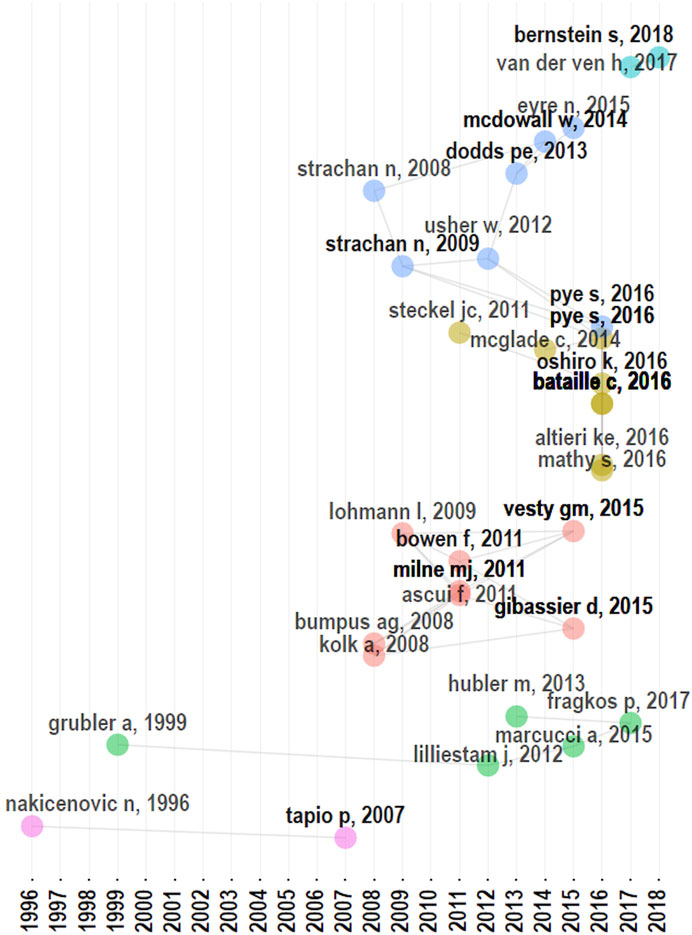
FIGURE 1. Bibliometric historiography graph showing the historical evolution of the most-cited work: with parameter node value 30.
Systems and Technologies Cluster
The two networks at the bottom of Figure 1 can be described as the “systems and technologies” cluster of networks for its focus on technology and systems thinking. The concerns on technological forecasting and socio-economic change have shown in the relatively more early work by Nakićenović (1996) on decarbonization and later by Tapio et al. (2007) on European energy and transport sectorial development on both decarbonization and dematerialization. Both work highlights the strong link between decarbonization and dematerialization, arguing that carbon emission represents one of the largest mass flows in the human activities on Earth. Most cited work is the article by Grübler et al. (1999) on energy technologies and global change, of which Nakićenović is the second author. The main research question is framed as technological choices that have long-term deterministic impacts on industrial societies and the natural environment, with an analytical focus on energy technologies that have been creating problems in the atmospheric environment. Such a research agenda has assumed the historical trend of decarbonization must be accelerated by the conscious technological choice and social change. As shown in the second line of work from the bottom of Figure 1, the article by Grübler et al. (1999) is cited by Lilliestam et al. (2012) on the costs and risks analysis of carbon capture and storage (CCS) versus concentrating solar power (CSP) comparison. In turn, the work is cited by Marcucci and Fragkos (2015) on the exploratory multi-model decomposition analysis of the decarbonization pathways in four major economies of China, India, Europe and U.S., suggesting that while energy efficiency remains the main strategy, the decarbonization strategy after 2030, and the negative carbon emissions after 2050 are critical. The work informs the computable general equilibrium (CGE) analysis of the EU Decarbonisation Roadmap 2050 by Hübler and Löschel (2013). It proposes a framework that integrates a forest sector model, a carbon accounting model, an ecosystem model, and climate change scenarios. The computable general equilibrium (CGE) is also used earlier by Fragkos et al. (2017) to draw policy implications on European Intended Nationally Determined Contribution (INDC). Altogether, such a line of work, as presented in the two networks at the bottom of Figure 1, can thus be called “systems and technologies” for their focus on the technologies and systems perspective.
Accounting and Accountability Cluster
At the middle of Figure 1, the network begins by Bumpus and Liverman (2008) and Kolk et al. (2008) can be described as the “accounting and accountability” cluster. Although Bumpus and Liverman (2008) focus more on international governance of carbon offsets (i.e., Kyoto Protocol’s Clean Development Mechanism), and Bumpus and Liverman (2008) focus more on corporate responses in the form of carbon emission reporting and disclosure mechanisms, both have informed the ensuing carbon accounting research (Lohmann, 2009; Ascui and Lovell, 2011; Bowen and Wittneben, 2011; Milne and Grubnic, 2011; Gibassier and Schaltegger, 2015; Vesty et al., 2015) with the main publication venues such as Accounting, Auditing and Accountability Journal. The premise of such work can be seen as based upon the calculative (or calculative agency) and persuasive features of the social need to perform (i.e., the performativity in the sociology of quantification). As such accounting performativity is applied in the international governance and corporate reporting and disclosure efforts, the notion of accountability via calculative agency becomes central to the cluster.
Transition and Scenario Cluster
At the upper-side of Figure 1, the network begins by Strachan and Kannan. (2008), Strachan et al. (2009) and Steckel et al. (2011) can be described as the “transition and scenario” cluster. Although the work by Strachan and Kannan. (2008); Strachan et al. (2009) appears to be focusing on systems modelling work like the work in the “systems and technologies” cluster, the focus on specific policy-making insights for decarbonization scenarios (United Kingdom), highlights the interface between modeling and policy. Similarly, the work by Steckel et al. (2011) also provides scenarios-modeling work, on the case of China as indispensable role in the global goals of decarbonization, which in turn informed the research on “un-burnable oil” (McGlade and Ekins, 2014).
The cluster features a line of research focusing on pathways, especially on the global and national energy systems’ transition towards decarbonization. It features a global collaborative initiative to share pathway-modeling findings and capacities, under the term “deep decarbonization” (Bataille et al., 2016a; Bataille et al., 2016b). Based on scenario-modelling findings, the decarbonization pathway findings have been published for Japan (Oshiro et al., 2016), South Africa (Altieri et al., 2016), and France and Germany (Mathy et al., 2016), right after the Paris Agreement in December 2015. An exploratory analysis has been conducted on how such decarbonization pathways may impact on global energy trade flows (Pye et al., 2016). An approach for “dynamic adaptive policy pathway” has been proposed (Mathy et al., 2016). In particular, how different modelling approaches can be applied in different national or regional contexts for deep decarbonization pathways have be discussed for both developed and developing countries (Pye and Bataille, 2016).
Although it is beyond the scope of the study to produce a complete stand-alone bibliometric historiography analysis, the three clusters, as identified and discussed above, demonstrate the common fundamental cross-cluster concerns on decarbonization (especially energy-related issues as related to carbon emissions) and different focuses thus their main approaches (i.e., the system-modelling for the systems and technologies cluster, calculative accountability for the accounting and accountability cluster, the pathway research for the transition and scenario cluster).
Author Co-citation Analysis
Providing another perspective into the intellectual structure, the author-co-citation analysis is a bibliometric method commonly used to show the authors’ influence and impact through co-citation networks (Boyack and Klavans, 2010; Aria and Cuccurullo, 2017). At the level of authors commonly cited in the dataset, Figure 2 shows the visualization of the co-citation network.
At the center to the right, the presence of organization authors such as International Energy Agency (IEA), European Commission, United Nations Framework Convention on Climate Change (UNFCCC), Intergovernmental Panel on Climate Change (IPCC), World Bank, the Organisation for Economic Co-operation and Development (OECD), United Nations, the US Energy Information Administration (EIA), and International Renewable Energy Agency (IRENA), clearly demonstrates the significance of these organizations as important sources on the carbon emissions, climate change and energy. The work by individual lead authors consists of Beng Wah Ang’s work on decomposition analysis for energy policymaking (e.g., Ang, 2004); Rogelj et al.’s perspective published in Nature (Rogelj et al., 2016) on the need for additional enhancement of national, sub-national and non-state actions beyond the Intended Nationally Determined Contributions (INDCs) required by the Paris Agreement, and Böhringer et al.’s major work on carbon tariffs (Böhringer et al., 2015). The work also includes an opinion piece on fairness measures (Peters et al., 2015), a major 700-page report for the United Kingdom government (Stern, 2007), and a body of work initiated by Gössling et al. on international tourism (e.g., Gössling et al., 2006).
At the lower left of Figure 2, the blue cluster consists of non-organizational authors’ work, mainly on socio-technical transition. It features the main work by Frank W. Geels and Benjamin K. Sovacool, that culminates to the article called “Sociotechnical transitions for deep decarbonization” (Geels et al., 2017), based on their past and ongoing work such as socio-technical transitions (e.g., Geels, 2005; Geels, 2010; Geels, 2018; Geels, 2019) and transition pathways (e.g., Sovacool, 2017; Köhler et al., 2019). It also presents work by Unruh (2000) on “carbon lock-in” and by Bulkeley and Betsill (2003) on the role of global cities in climate change. The cluster also features work by the aforementioned work by Nakićenović (1996) and the seminal work on energy technology and global environmental changes by Grübler et al. (1999), which will be further discussed in section 3.4.1.
Finally at the upper left of Figure 2, the green cluster consist of major United Kingdom bodies such as Department of Energy and Climate Change (DECC), Office of Gas and Electricity Markets (Ofgem), Department for Business, Energy and Industrial Strategy (BEIS), and Climate Change Committee (CCC), an independent, statutory body established under the United Kingdom Climate Change Act 2008. It also features the work on the analysis of government failure and rent-seeking in climate change policy (Helm, 2010) and the main energy modeling documentation (Loulou et al., 2004).
In short, the historiography (section 3.1.1) and author co-citation network (section 3.1.2) provide several insights on the intellectual structure of the knowledge base, revealing the fundamental concerns, authoritative organizational and individual sources, and divergent focuses.
Conceptual Structure and Its Evolution
To explore the conceptual structure, the study begins with a keyword co-occurrence analysis, followed by a selected list of keywords with the strongest citation bursts, and a set of thematic evolution maps. Together they will provide the relationship and the temporal dynamics among the keywords as the empirical bases to understand the structure of concepts and its evolution. Indeed, for instance, politics and just transition emerge as the most recent component contributed by mostly European actors, as evidenced by multiple findings as follows.
Keyword Co-occurrence Analysis
To detect the main topics and evolving research frontiers, the study has conducted keyword co-occurrence analysis based on the author keywords. Because author keywords represent the succinct concepts and topics of the articles, the keyword co-occurrence analysis can reveal the conceptual structure of knowledge base, as these keywords can be further clustered into categories of concepts based on the network relationship.
Figure 3 and Table 2 show the keyword co-occurrence analysis outcomes of the top 30 keywords in four clusters. The first digit of the ID represents the membership of four clusters: two large-size clusters (A, and B), a mid-size cluster (C) and a smaller cluster (D). The clustering coefficient values are higher for the peripheral and often low-ranking nodes with low-level of betweenness centrality. The measurements confirm the core-periphery structure. Although the top nodes for each cluster (A-2, B-1, C-13, and D-16) can be close near the core of the network, each cluster contains other peripheral nodes with consistently high clustering coefficient values, confirming the clustering outcomes.
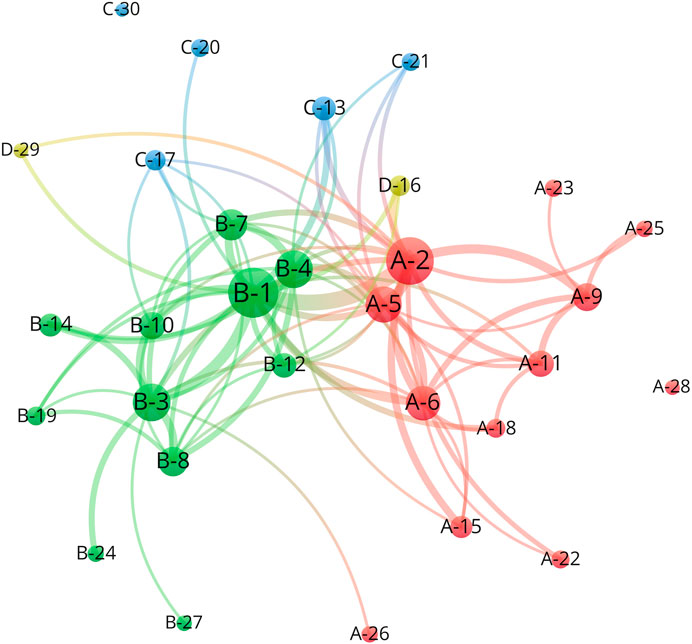
FIGURE 3. Keyword co-occurrence network visualization, with each node representing a keyword (Table 2).
Note that the second part of the ID corresponds to the ranking number based on the metrics of “total links strength” first and then “occurrences,” as shown to the right of the column ID in Table 2. The metric “total link strength” is the derived indicator provided by VOSviewer to attribute the total strength of the links of a node with other nodes in the network, and such an attribution suggests how nodes are closely related in terms of distance (MacDonald and Dressler, 2018; Yu et al., 2020; Elisha and Viljoen, 2021).
The top keyword nodes in terms of the number of occurrences (as visualized in Figure 3 as the size of the nodes and listed in Table 7) are “decarbonization” (B-1), with 148 occurrences, “climate change” (A-2), with 128 occurrences. The study describes each cluster with the top keyword accordingly. Cluster B (decarbonization, in green) and cluster A (climate change, in red) occupy the central positions of the network diagram, taking up at least two-thirds of the space. The two clusters also connect to clusters C (low-carbon transition, in blue) and D (politics, in yellow).
Overall, the conceptual structure is dominated by two major themes of climate change and decarbonization, represented by clusters A and B respectively, while the themes of transition and politics appear to emerge, represented by clusters C and D respectively. The key themes such as carbon accounting, carbon neutrality and carbon trading in cluster A are closely related to the sectors of transport and forestry in the same cluster. The key themes such as decarbonization, climate policy, governance, and net zero in cluster B are closely related to the sector of energy and the entity of European Union in the same cluster. The main conceptual components of knowledge base can thus be summarized as two main set of themes: one is carbon accounting efforts for climate change mitigation (especially in relation to the sectors of transport and forestry), another is decarbonization governance for energy transition (especially in relation to the energy sector and European Union).
The smaller clusters indicate perhaps emerging or declining set of themes. Clusters C and D represents two sets of themes: one is low-carbon and sustainability transition (especially in relation to the Paris Agreement), and another is about politics and just transition.
Keywords With Strongest Citation Bursts
To further clarify the evolution of research themes (i.e., emerging or declining), the findings detailed in Table 3 further reveal the temporal dimensions of the top keywords with strongest citation bursts.
Indicating the intensity of attention over certain time periods, topics with the strongest citation burst provide additional insights, showing the topical transitions of the knowledge base over time (Chen, 2004). For instance, the keyword “global warming” receives relatively long-lasting, intensive attention from 1999 to 2011, indicated by the relative strength (3.05), beginning and end in Table 3.
Table 3 further enriches our understanding of the knowledge base so far as follows. First the concepts of carbon sequestration, afforestation, and the “Reducing Emissions from Deforestation and Degradation” (REDD) mechanism occur intensively around 2006 and 2010, and they are closely related to the forestry sector and its critical role in carbon neutrality discussion. Then, the concepts of bioenergy and carbon accounting occur intensively around 2013, indicating the calculative agency in analyzing technological choices at that time. After the Paris Agreement signed in 2015, the themes of Germany, electricity market and transport emerge. The last 3 years of 2019–2020 show the intensive occurrences of European Union, just transition, energy storage, city, net-zero and energy transition, indicating these themes are indeed emerging, not declining ones.
Thematic Evolution and Maps
To advance further understanding of the conceptual structure, the study has conducted a thematic evolution analysis, following the thematic analysis conventions (Cobo et al., 2011). Using the aforementioned time periods, Figure 4 shows the thematic evolution graph generated from Bibliometrix. It reveals the continued focus on renewable energy and climate change, and the recent rise of carbon accounting, decarbonization and sustainability transitions, especially after the Paris Agreement in 2015.
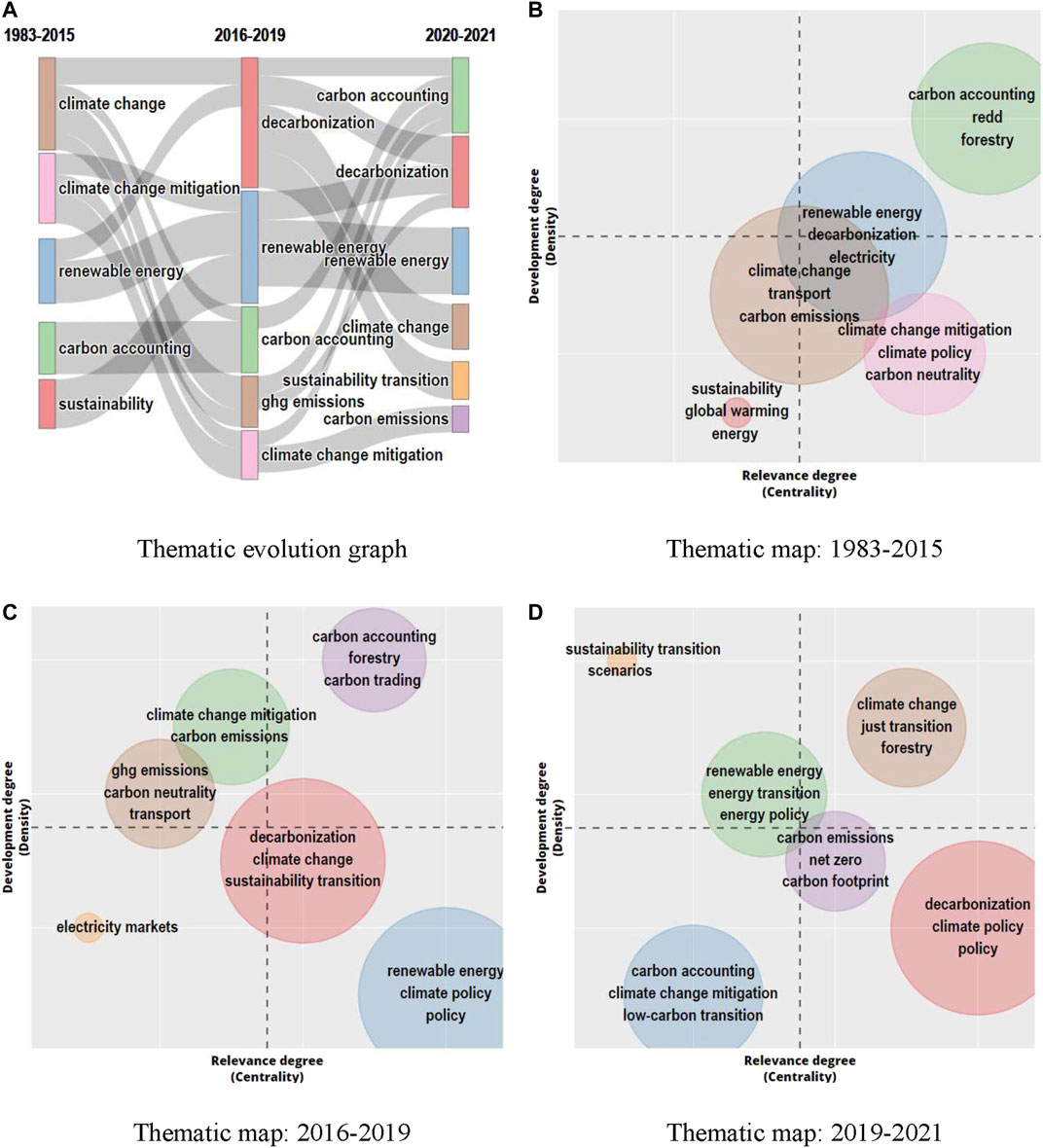
FIGURE 4. Thematic evolutions: the continued focus on renewable energy and the rise of carbon accounting, decarbonization and sustainability transition. (A) Thematic evolution graph, (B) Thematic map: 1983-2015, (C) Thematic map: 2016-2019, (D) Thematic map: 2019-2021.
Using network centrality measures, thematic maps distinguish the centrality (x-axis) and density (y-axis) of themes (topics or keywords here), as shown in Figures 4B–D for each periods. Applying the common interpretations of the four quadrants of themes, the maps show the flowing four themes with distinct features based on the centrality measures:
• Quadrant I: motor-theme, at the upper-right, indicating the well-established structure;
• Quadrant II: niche-theme, at the upper-left, representing well-established but less important structure;
• Quadrant III: emerging-declining-theme, at the lower-left, neither important nor developed;
• Quadrant IV: basic-theme, at the lower-right, important but under-developed;
It is revealed by Figure 4B, for the first period, themes such as carbon accounting, REDD, and forestry constitute the more well-established conceptual structure, whereas the themes such as climate change mitigation, climate policy and carbon neutrality remain in the basic-theme quadrant. For the second period, as shown in Figure 4C, themes such as carbon accounting and forestry remains well-established, joined by the theme of carbon trading. Themes such as carbon neutrality, GHG emissions, transport, climate change mitigation appear in the niche-theme quadrant, whereas renewable energy, climate policy and policy appear in the basic-theme quadrant. Confirming the previous findings based on citation bursts, the theme of electricity markets appears in the emerging-declining-theme quadrant. For the last and most recent period of 2019–2021, Figure 4D shows the new concept of just transition enters the well-established motor-theme quadrant, confirming the findings in section 3.2.2. The policy-related themes remain in the basic-theme quadrant, along with themes such as net zero and carbon footprint. The themes of sustainability transition, scenarios, renewable energy, energy transition and policy appear in the niche-theme quadrant.
Altogether, the conceptual structure of the knowledge base reveals the major and emerging themes such as decarbonization, climate change, carbon accounting, net-zero, and just transition, the most discussed sectors such as energy, forestry and transport, and entities such as the European Union. After first answering the “when” questions, the study now turns to the “where” questions. (Where have such social sciences activities on carbon neutrality occurred?)
Social Structure
To explore the social structure of knowledge production, the study continues to examine the main journal sources and top-performing countries or regions. Together they will provide the contextual understanding of the knowledge productions in terms of their geographic and disciplinary contexts. Indeed, the body of knowledge has spanned across multiple geographic and disciplinary contexts, as evidenced by multiple findings as follows.
Since the distribution of publications across journal sources can provide initial answers to the “where” question, it is then helpful to examine the features of top journal sources.
Top Journal Sources and Their Multi- and Cross-Disciplinary Features
As mentioned earlier, the study employed the “split-apply-combine strategy” (Wickham, 2011) of data analysis. Thus, Table 4 shows the cross-tabulation results by breaking up the bibliographic records according to the publication sources (see the first column) and then exploring their respective disciplines and top topics (see the second and third columns).
The top journal sources are Energy Policy, Climate Policy, Energy Economics, Ecological Economics, Global Environmental Change-Human and Policy Dimensions, and Technological Forecasting and Social Change, as shown in the first column of Table 4. The ranking numbers are shown in parentheses before the journal title names, and the numbers of the publications are shown after the colon following the titles. The titles of these journals reveal the clear presence of policies, economics, and environmental and social changes, indicating dynamic policy and economics concerns regarding such changes.
The dominant social science disciplines are Business and Economics, Public Administration, and Geography, as shown in the second column of Table 4. The four top journal sources also belong to Environmental Sciences and Ecology, within the broader category of “Life Sciences and Biomedicine”, suggesting the significant cross-disciplinary feature across the disciplines of Environmental Sciences and Ecology and the social science disciplines of Business and Economics, Public Administration, and Geography. Additionally, the top journal Energy Policy belongs to the discipline of Energy and Fuels, which belongs to the broader category of “Technology”. The topical features are mostly dominated by the topics of “decarbonization”, “climate change mitigation”, “renewable energy”, “carbon accounting”, and “sustainability transition” for the top journal sources.
Based on the initial answers above on the features of the top five journal sources, this section advances the analysis by examining the bibliographic coupling of all journal sources that have five or more articles. Bibliographic coupling analysis can allow us to map and cluster the journals based on the similarities of the bibliographic references (Jarneving, 2007; Boyack and Klavans, 2010).
In total, 26 journal sources out of the 887 articles of the second time period met the criteria. The bibliographic coupling analysis outcomes resulted in five clusters, as visualized in Figure 5. At the center is the core cluster of journal sources with label initial A in color red, such as Energy Policy (A-1), Climate Policy (A-2), Energy Economics (A-3), Global Environmental Change-Human and Policy Dimensions (A-4), and Technological Forecasting and Social Change (A-6). Since Figure 5 and Table 5 together show the bibliographic coupling relationship among journal sources, the “total link strength” indicates the total strength of such a bibliographic coupling relationship of a given journal source with other sources, thereby substantiating the empirical claims of research fronts as networks of nodes based on links of bibliometric metrics. At the upper right of Figure 5 is the peripheral cluster of journal sources with label initial B in color green, including Accounting Auditing and Accountability Journal (B-8) and Environment and Planning C-Politics and Space (B-12). At the lower right of Figure 5 is the peripheral cluster of sources labeled with the initial C in blue, including Ecological Economics (C-5), and Journal of Forest Economics (C-10). At the lower left of Figure 5 is the peripheral cluster of sources labeled with the initial D in the color yellow, including Transportation Research Part A-Policy and Practice (D-7) and Transport Policy (D-9). Revealing the main research fronts while confirming the validity of bibliometric methods, Figure 5 and Table 5 show the topical and disciplinary closeness of journal sources. Core cluster A not only consists of the top journals, but also indicates the interdisciplinary nature of policy, economics, and social change, especially for energy and climate. The cluster B largely contains cross-disciplinary research fronts across the topics of accounting management, geography, and politics. Cluster C concerns the research fronts of ecological, forest, and resource economics. Cluster D relates to transportation. Cluster E, with one node E-20, concerns sustainable tourism. Additionally, Table 5 shows the citation attributes (i.e., the absolute and normalized numbers) and the network centrality metrics (i.e., closeness centrality, eigenvector centrality, and betweenness centrality) and clustering coefficients.
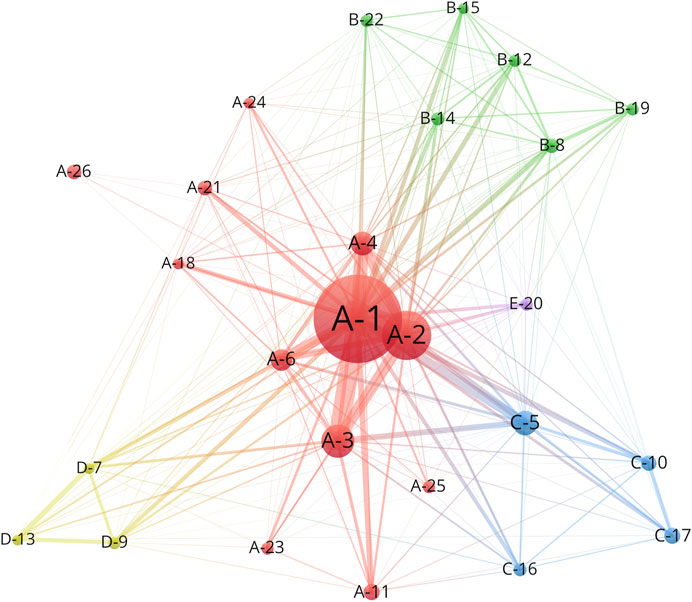
FIGURE 5. Main journal sources: a bibliographic coupling network visualization, with each node representing a journal source (Table 5).
Overall, clusters A, B, C, D, and E show that the core clusters A and B are multi-disciplinary, with C, D, and E focusing on the cross-disciplinary topics of forestry, transportation, and sustainable tourism, respectively.
From the top 5 to the top 26 journals, the multi- and cross-disciplinary features were analyzed and visualized, showing how knowledge publication is distributed across sources with their associated disciplines and topics. Business and Economics, Public Administration, and Geography were shown to be the most dominant disciplines.
Top-Performing Countries or Regions
This sub-section advances the analysis of the features of the countries, with the aim to explore national and regional contributions as well as implicit collaboration (in the form of citing the same knowledge sources) across national and regional boundaries.
At the level of countries, Figure 6 and Table 6 show visualizations of the bibliographic coupling network and key scientometric indicators. The first digit of the ID represents the membership of five clusters: four mid-size clusters (A, B, C, and E) and a smaller cluster (D). The most important node in terms of the number of documents (as visualized in Figure 6 as the size of the nodes and listed in the column titled “Documents” in Table 7) is England (E-1), with 220 documents, as part of cluster E with other United Kingdom regions such as Scotland (E-15) and Wales (E-25), as well as Denmark (E-16). The second-ranking node is the United States (B-2), along with Australia (B-4), Canada (B-5), South Africa (B-27), and Russia (B-30). Shown in the lower part of Figure 6 is cluster C, which contains four European countries: Germany, Italy, the Netherlands, and Austria. Clusters B and C occupy the central positions of the network diagram, connecting to the main clusters A (red) on the left and clusters D (yellow) and E (purple) on the right. With the highest number of nodes, cluster A on the left consists of many more Asian and South American countries, such as China (A-10), Japan (A-21), Brazil (A-23), India (A-2), and Singapore (A-26). Finally, the smaller cluster D (in yellow) is shown to be at the periphery on the lower right of Figure 6, consisting of three Nordic countries—Sweden (D-7), Norway (D-11), and Finland (D-18)—along with New Zealand (D-19). Based on the network visualization (Figure 6) and its centrality indicators (Table 6), the most central nodes are England (E-1), the United States (B-2), and Germany (C-3).
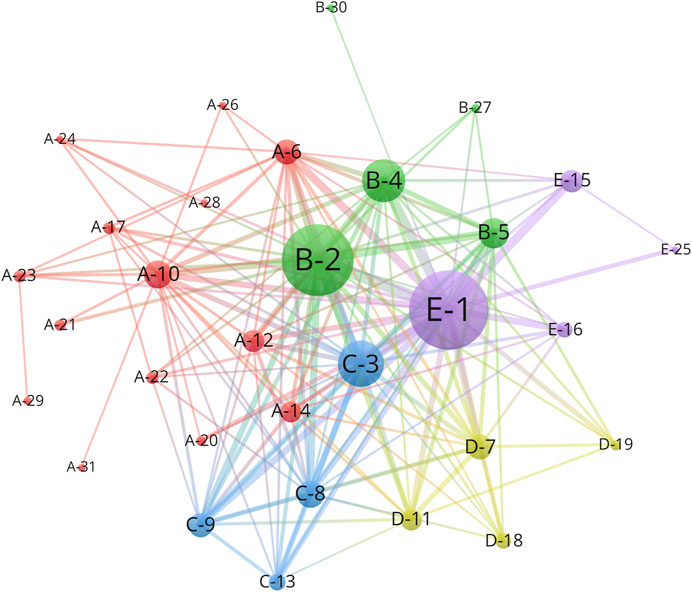
FIGURE 6. Main countries: a bibliographic coupling network visualization, with each node representing a country (Table 6).
Main Research: Most-Cited Work Across Social Science Disciplines
The previous section indicates that the body of related social science knowledge has three core clusters and other scattered peripheral work. To explore the specific details across disciplinary (and often thus geographic) contexts, a total 55 articles were selected for further review based on the top two most-cited articles in each social science discipline. Together they will provide a mosaic of disciplinary research with specific disciplinary and geographic details.
To determine the measurement of “most-cited” work, this research has used the data of the “Total Times Cited Count” (with the field tag Z9), including citation data from the following database: Web of Science Core Collection, BIOSIS Citation Index, Chinese Science Citation Database, Data Citation Index, Russian Science Citation Index, and SciELO Citation Index.
Table 7 lists the most-cited work across different social science disciplines, and it is sorted based on the number of publications (see the column headed “Counts”), which are unevenly distributed. The following discussions were systematically organized based on the clusters found in Table 8, with the aim to highlight the disciplinary features.
Business and Economics Cross-Disciplinary Core Cluster
Based on the business and economics cross-disciplinary core cluster shown in Table 8, this subsection will summarize the major works in the only social science discipline in the business and economics cluster.
First, business and economics research examples include the energy technology and global environmental changes (Grübler et al., 1999), as well as the sustainability of sugarcane ethanol (Goldemberg et al., 2008). Grübler et al. (1999) proposed a useful technical analysis typology for analyzing the impact of energy technology on the global environment, especially global warming (Grübler et al., 1999). Goldemberg et al. (2008) found that the rapid expansion of sugarcane ethanol production in Brazil has caused sustainability issues, including the positive impact of air quality improvement and negative impacts on food security and field working conditions (Goldemberg et al., 2008). Both studies point to the central policy concerns of energy policies and their environmental impact (Grübler et al., 1999; Goldemberg et al., 2008).
Both articles were published in Energy Policy, an international journal for the political, economic, planning, environmental, and social aspects of energy. This exemplar research provides a deep analysis of the intricate relationship between energy and the environment, as well as the impacts of specific technologies and policies. The human energy technology shift from coal to oil to natural gas requires a better understanding of the impact on global environmental changes over time, including measurements such as unit energy of carbon pollution (Grübler et al., 1999). The systematic approach is also required to gain a holistic view of both the positive and negative impacts, as illustrated in the analysis of Brazil’s sugarcane ethanol (Goldemberg et al., 2008). Although carbon dioxide emissions have also been reduced, this has led to worsening the conditions of high-biodiversity areas—for example, through deforestation, water contamination, soil degradation, and soil damage. Indeed, the sustainability aspects of the energy–climate (or even energy–environment) relationship require unpacking and clarity.
Public Policy Cross-Disciplinary Core Cluster
As Table 8 suggests that several disciplines are in close relationship with public administration (i.e., the public policy cross-disciplinary core cluster), this subsection will summarize the major work in these disciplines, as listed in Table 7.
Public administration work examples include climate policy (Hohne et al., 2017) and smart-sustainable cities (Yigitcanlar and Lee, 2014). In specific, the importance and implications of the 2015 Paris Agreement were discussed and examined by Hohne et al. (2017), with the analytical focus on the “individual intended nationally determined contributions” (INDCs); they found that the progress of decarbonization has been made faster than expected in sectors such as solar photovoltaics, onshore wind, and electric cars, and they argued that policies must be implemented in order to meet the INDC pledges made by national governments to resolve the inconsistency between INDCs and global goals such as the 2015 Paris Agreement (Hohne et al., 2017). At the city level, eco-city initiatives were studied by Yigitcanlar and Lee (2014), focusing on the Korean case of ubiquitous-eco-city (u-eco-city), with the goal being to distinguish whether this contributes to an ideal model of sustainable and smart urban forms beyond being a mere branding event (Yigitcanlar and Lee, 2014). Both studies hint at the political, financial, technological, and other forms of support in implementing such policy initiatives (Yigitcanlar and Lee, 2014; Hohne et al., 2017).
Published in the journal Habitat International (Zuo et al., 2012; Kibwami and Tutesigensi, 2016), these exemplar works in urban studies recognize the importance of sustainability agenda in the building sector as one of the biggest contributors of carbon emissions and energy consumption. With the aim of examining the factors that hinder or help carbon neutrality goals regarding reducing emissions in commercial developments, Zuo et al. (2012) conducted semi-structured interviews of practitioners and found that a clear definition of carbon-neutral building is needed, along with other facilitating factors such as material choice, managers’ knowledge, market demand, government support, and leadership (Zuo et al., 2012). Kibwami and Tutesigensi (2016) investigated whether and how the integration of embodied carbon in the development approval process by regulators can facilitate sustainability in construction, based on a set of structured interviews regarding policy proposals in Uganda (Kibwami and Tutesigensi, 2016). In the development approval process, the authors argue, both environmental and social sustainability should be considered, even in developing countries. Similarly, an exemplar work in education discussed sustainability initiatives at a Canadian university and proposed a multi-bottom line approach based on a set of tools using a driving-force–pressure–state–exposure–effect–action framework (Waheed et al., 2011). Stadel et al. (2011) developed engineering education that integrates carbon accounting and building information modeling as the basis for intelligent sustainable design (Stadel et al., 2011).
Social Science Disciplinary Core Cluster
Based on the social science disciplinary core cluster shown in Table 8, this subsection will summarize the most-cited works in these disciplines, as listed in Table 7.
Government and law discipline works include the seminal work by Vandenbergh and Steinemann (2007) on the legal and regulatory basis on the notion of the carbon-neutral individual (Vandenbergh and Steinemann, 2007) and the analysis of carbon management standards by Green (2013). Drawing upon empirical studies and norms theory, the notion of the carbon-neutral individual suggests the possibility of advancing legal reforms that combine the existing norm of personal responsibility with the emerging norm of carbon neutrality (Vandenbergh and Steinemann, 2007). Focusing on the role of private authority, the network analysis of public and private standards for carbon management reveals an emerging climate change governance order that is complex, suggesting the importance of private authority in institutional complexity (Green, 2013). Both articles have contributed to the legal and regulatory imagination to address the governance and management issues surrounding carbon neutrality and carbon management.
Works on major international relations includes the article by Green (2013), which was not only published in Global Environmental Politics but also touches upon the institutional complexity under the Kyoto Protocol (Green, 2013). Additionally, the article by van der Ven et al. (2017) that was published in the same journal examined the effect of the valuable contributions of non-state and subnational actors on climate governance. It proposes an approach that is more comprehensive in identifying how such contributions can become far-reaching and durable (Van der ven et al., 2017). Such an approach is applied to an analysis of the Carbon Trust’s initiative on the creation of product carbon footprints, indicating some initial failures and suggestions for policymakers to measure the contribution to global decarbonization more accurately.
A key “social sciences-other topics” work consists of an article on Norwegian attitudes towards the impact of air travel on climate change. The article concludes that Norway is sensitive to climate issues and thus a vanguard European tourism market (Higham and Cohen, 2011). Additionally, on the topic of tourism Gössling (2009) provides a critical review of carbon neutrality within the context of the UNWTO’s Davos Declaration, which ascribed the responsibility to reduce greenhouse gas emissions to the main tourism industrial actors (Gössling, 2009).
One sociology paper by Scott et al. (2019) also contributes to the understanding of the consequences facing the tourism sector through the systematic construction of the Climate Change Vulnerability Index for Tourism (CVIT) (Scott et al., 2019). This research found that Africa, the Middle East, South Asia, and Small Island Developing States are highly vulnerable and that climate change will hinder the contributions of tourism to the Sustainable Development Goals. From the perspective of everyday life and devices of accounting, another sociology work carried out by Marres (2011) examined the role of technology in public participation in carbon accounting, suggesting the capacity to “co-articulate” participation (Marres, 2011).
From the discipline of social issues, the exemplar work by Pidgeon and Demski (2012) on the public’s attitudes towards energy transformation highlights the importance of public consent and acceptability in the adoption of emerging renewable energy projects (Pidgeon and Demski, 2012). The work by Boehmer-Christiansen (2003) on the need for global treaty obligations to advance the transition to green fuels and technologies asks several questions regarding equity and political instability during the decarbonization of global energy supplies (Boehmer-Christiansen, 2003).
From area studies, the work by Benjaminsen (2017) examined why the global policy framework known as Reducing Emissions from Deforestation and Forest Degradation (REDD+) in Zanzibar is at risk of early enthusiasm and then ensuing abandonment due to its lack of legitimacy in terms of durable community forest management (Benjaminsen, 2017). Another area studies work by Gilley (2017) compared the local success in reducing greenhouse gas emissions in China (Guangzhou) and India (Gujarat), revealing similar patterns of integrated governance solutions but different institutional linkages: mainly intra-governmental linkages for China and mainly state-society linkages for India. The research also showed the insignificant to marginal effects of international negotiations and national frameworks, with implications for central–local relations regarding environmental issues and for international aid and assistance (Gilley, 2017).
Other Peripheral Disciplinary Work
Other social science disciplines consist of Development Studies, Geography, Psychology, Communication, Linguistics, Social Work, Archaeology, and Cultural Studies, each with a few publications (some four and often two). Nonetheless, it is helpful to examine how each social science discipline has contributed to this topic so far.
One major development studies work by Zuo et al. (2012) was previously discussed in the urban studies discipline, not only because it was published in the cross-disciplinary journal Habitat International but also because it discusses the need for cultural change in the construction industry towards sustainability (Green, 2013). Another research article by Andrews (2008) investigated how land use and greenhouse gas emissions are related using exploratory case studies to illustrate the varying per capita carbon dioxide emissions. This study found that buildings typically contribute more emissions than personal transportation and argued that holistic solutions to this problem must incorporate technical fixes (especially green buildings) and land-use pattern changes (considering population densities and transportation).
One important work in the field of geography is an article published in Economic Geography by Bumpus and Liverman (2008) on the governance of carbon offsets. Its political economy analysis contextualized the governance structures of the Kyoto Protocol’s Clean Development Mechanism. The analysis illustrates how carbon offsets represent the strategies of capital accumulation. Another geography research article tested six decarbonization pathways for the United Kingdom, with specific discussions of the role of water in electricity generation and cooling water use (Byers et al., 2014).
Following the traditions of pro-environmental behavior research, one essential psychology work by Quimby and Angelique (2011) analyzed the perceived barriers and catalysts for encouraging pro-environmental behavior within the environmental movement. Based on the perceived barriers they found (such as hopelessness, low efficacy, and time and money resources) and the catalysts identified (such as changing social norms through institutional and educational transformation), the article argues that knowledge of community psychology can contribute to the fight for a carbon-neutral future (Quimby and Angelique, 2011). Based on the fieldwork examining cobalt mining in the Democratic Republic of the Congo (DRC), another important psychology work by Payne (2020) highlighted the injustice of the “decarbonization divide”, connecting decarbonization with issues of environmental destruction, child labor, energy dispossession, and gender inequality, with certain ethnic groups being rendered more psychologically vulnerable (Payne, 2020).
Coming from the discipline of communication research, Hopke and Hestres (2018) visualized the media and climate stakeholder relationship based on Twitter activity relating to the Paris Climate Talks (Hopke and Hestres, 2018). The findings showed that the Twitter accounts of fossil fuel and trade association, as major outliers, had put more emphasis on former US President Barack Obama’s climate policy instead of the more common climate change visual framing used by activists, movement organizations, multinational representatives, and scientific experts. These accounts’ messages aimed to create a visual narrative of the lack of domestic support for his climate policies in the global arena. Additionally, focusing on the discourse surrounding carbon, the media discourse analysis by Koteyko (2012) examined the “market-driven sustainability” in the British media, specifically United Kingdom national newspapers, between 1990 and 2009 (Koteyko, 2012). The findings reveal that finance-related carbon compound phrases (1990–2005) occur before other compound phrases, such as “low-carbon”, “zero-carbon”, and “carbon-neutral” (popular after 2005). This study argues that the climate change debate has been narrowed because carbon compound phrases suggest that carbon emissions management starts from calculation and monetization.
Linguistics works include the communications research work carried out by Koteyko (2012), as discussed earlier, as it was published in a cross-disciplinary journal called Language and Communication. Additionally related to communication research is an article by Dury (2008) from the linguistics journal Terminology, based on a bilingual (French and English) comparable corpus, which shows that the English term “carbon neutral” has followed a particular development pattern: it becomes more widespread in the media over time (i.e., determinologized) and then moves into the ecology lexicon (i.e., terminologized) (Dury, 2008).
From the discipline of social work, the previously discussed work by Quimby and Angelique (2011) is included, since it was published in the cross-disciplinary journal American Journal of Community Psychology (Quimby and Angelique, 2011). The social work article by Chapman and Boston (2007) examines the social implications of decarbonization in the New Zealand economy, outlining the likely economic and distributional impacts of related policies on public attitudes towards and behaviors concerning carbon emission reduction (Chapman and Boston, 2007). The archaeology work by Pigliautile et al. (2019) examines historical buildings to explore an innovative approach to microclimate enhancement using thin-envelope materials (Pigliautile et al., 2019). The cultural studies work by Pallesen (2016) examines the cultural and framing politics of pricing by examining the adoption of tariffs and wind power pricing in France during the decarbonization of the electricity sector, raising the issues of the social and governance dynamics of value attribution and negotiation (Pallesen, 2016).
Discussion
Overall, our findings confirm our assumption that the issues of carbon neutrality and decarbonization are multi- and cross-disciplinary; these detailed and systematic findings provide specific empirical evidence to quantify and qualify the existing knowledge in this area. As carbon-neutral efforts are shown to be made in response to various issues of climate change, collective action requires not only technical innovations for transforming various modern industrial sectors, but also social science understanding of the ways in which carbon information can be instrumental in reshaping our social, economic, and political interactions. Researchers and policy-makers have so far contributed to the discussions on the role of technologies, policies, and markets in achieving carbon neutrality. Facing the climate change mitigation opportunities and challenges, issues such as measuring and accounting decarbonization efforts, sustainability transition, and just transition have been discussed in specific sectors such as energy, transport, forestry, agriculture, forestry, and tourism.
Our descriptive summary of 907 articles revealed the fast-growing publications patterns, especially after the Paris Agreement in 2015, with temporal evolution in intellectual and conceptual structures of the knowledge.
In terms of intellectual structure, the established state-of-the-art knowledge has been built based on the intellectual origin of 1) systems and technology cluster, 2) accounting and accountability cluster, and 3) transition and scenario clusters. Both historiography and author co-citation analysis have revealed the intellectual origins and authoritative sources that have founded and shaped the body of knowledge. The largely macro-, regional, sectorial and country-level of analysis aim to address the fundamental concerns of human technologies’ impact on the environment, with the initial system modelling work growing into technology-, policy- and organization-level analysis towards more sustainable scenarios and pathways.
In terms of conceptual structure, the last few years (2019–2022) have seen the rise of keywords such as decarbonization, energy transition, the Paris Agreement, just transition, European Union, and net-zero. Such a trend indicates the impacts of political actions and policies on carbon neutrality research for just and equal transitions towards sustainability. Undoubtedly, what decarbonization opportunities and challenges may occur for the industry and environment has become the basis for the most relevant and cutting-edge research topics across various social science disciplines.
In terms of social structure of knowledge publication, the bibliographic coupling analysis of journals shows that the two main core journal clusters are multidisciplinary while the other three smaller clusters focus on cross-disciplinary topics such as forestry, transportation, and sustainable tourism. Throughout the years, main journals such as Energy Policy, Climate Policy, Energy Economics, Ecological Economics, Global Environmental Change-Human and Policy Dimensions, and Technological Forecasting and Social Change have accumulated a substantial number of studies, connecting social science disciplines such as Business and Economics, Public Administration, and Geography with other disciplines such as Energy and Fuels and Environmental Sciences and Ecology. Publishing works on carbon neutrality that connect social science and non-social science categories; the journals have been identified in their contribution to have bridged the related disciplines. The empirical evidence on top-performing countries shows the relatively low presence of Asia and Africa, indicating a gap in the knowledge production and collaboration needed to tackle the issues of decarbonization as part of the global goals for sustainable and just transitions.
In terms of social discipline perspective, the scientometric approach of science mapping has revealed the otherwise partial and incomplete impressions of the social science literature in a systematic whole. The overall picture is much more diverse than the aforementioned intellectual structural base. Specific connections have been explored in discussing the specific main research works in section 3.4, revealing a vibrant picture of policy, professional, educational, and academic work that contributes to human knowledge on carbon neutrality and decarbonization. While the backbone of cross-disciplinary carbon neutrality research remains at the intersection between the “Business and Economics” and “Life Sciences and Biomedicine” disciplines, an emerging critical body of work has begun to question the limitations of “market-driven sustainability” and the social and emotional costs and implications of decarbonization, which coincides with the emerging notion of just transitions.
Conclusion
Significant progress has been made, especially since the Paris Agreement in 2015, on the issues of decarbonization and carbon neutrality, as part of the arguably the largest human efforts in transitioning energy, transport, industries, and cities towards low-carbon and net-zero sustainability. It is significant because human-caused climate change needs human efforts and knowledge to change the socio-technical and socio-ecological systems we have constructed. The emphasis on the “socio” requires a comprehensive overview of the state of art in social science knowledge on the topic.
This scientometric and systematic review provides a comprehensive and disciplinary examination of the body of social science knowledge produced so far on the topic of carbon neutrality. However, it is acknowledged that the scope of this review does not include conference proceedings and other works that are not indexed by the Web of Science database. It has limitations in depth because more specific carbon-neutrality keywords such as forest carbon sinks, carbon capture, utilization and storage (CCUS), and so on were not included in the search query design. It is also limited to the trade-off between comprehensiveness and depth that any scientometric and systematic review must decide and justify. More in-depth analysis in the future should include more specific keywords by snowballing specific set of keywords, and the conceptual structure findings here can be useful references. Future work must determine such trade-off in using scientometric and systematic reviews to understand the multi-disciplinary topic of decarbonization.
Nonetheless, the findings presented here amount to the first scientometric analysis of the social science knowledge of carbon neutrality. This study provides an updated understanding and highlights the established and emerging relationship between policies and technologies, especially those relating to energy, transport, construction, education, and other sectors. The main body of work have been policy-oriented to examine the industrial and economic activities, especially those surrounding energy, transport, forestry, and cities-related technologies, with the practical purpose to outline transitioning pathways and scenarios. The emerging conceptual structure findings reveal the substantial impact of just transition, European Union, net-zero, cites, and energy transition on the most recent literature. Such a phenomena can be explained by the social structure of the current knowledge production, where European and North American regions remain dominant.
The main controversy appears to be about justice in the decarbonization processes, as reflected in the recent literature and European Union policy on “just transition.” Research gaps in the case studies beyond European and North American regions, cities, and people must be filled, especially the impact of climate change is expected to be more severe in developing regions such as Asia, Africa, and South America.
In terms of the potential development, we believe that, based on the findings presented here, the existing backbone of cross-disciplinary carbon neutrality research, which remains at the intersection between the “Business and Economics” and “Life Sciences and Biomedicine” disciplines, must engage the emerging critical literature to reflect on the limitations of the mainstream market-driven, business-driven, and data-driven approaches of decarbonization efforts aiming at carbon neutrality. Given the increasing knowledge demand for the development of policies and technologies relating to carbon neutrality across various industrial sectors and different academic disciplines, such a systematic science-mapping work provides significant results that will help to foster new conversations and enrich old ones. The descriptions on the most-cited work in each social science discipline in section 3.4 should begin to provide a more balanced perspective in terms of disciplines. Such knowledge, for instance, can be more personal and emotional at the micro-levels, beyond the initial knowledge base on country- and organization-level scenarios and pathways. Future work is needed, for instance, to deliver in-depth systematic reviews for each social science discipline.
These science-mapping outcomes lead to the final research question we will raise upfront: How can researchers and policy-makers understand this overview of social science literature relating to the topic of carbon neutrality, with a conceptual or taxonomic framework, to address the issues in achieving decarbonization and carbon neutrality, especially in terms of the design and application of relevant policies and technologies? While the findings described here may not be sufficient to generate a definitive conceptual framework, a set of common taxonomic features across disciplines and sectors can be built that includes decarbonization, carbon management, carbon accounting, sustainability, sustainable development, and just transition. Future work can advance more in-depth analysis within a specific social science discipline or a specific cross-disciplinary mix. As carbon-neutral efforts are shown to be carried out in response to various issues of climate change, a collective action that requires not only technical innovation for transforming various modern industrial sectors but also a social science understanding of how carbon information can be instrumental in reshaping our social, economic, and political interactions, especially for coming up with new strategy for green and digital transitions, is needed (European Commission, 2020).
Several directions for future research relating to social science knowledge about carbon neutrality are discussed here. First, since carbon information, including GHG emission information, is key for constructing a sustainable future, it is important to understand the overall and specific datafication processes of selecting, recording, monitoring, and acting upon any specific piece and set of carbon information concerning human activities and the environment, as a necessary and evidence-based grounding. The datafication of carbon information requires better knowledge in data science and social data science that can identify relevant and critical carbon information that contributes to the socio-technical transition to a sustainable future, especially the unjust cost of decarbonization processes. Second, as carbon information-based products, systems, and stories have increasingly involved several important industrial bodies and various human daily activities, it is vital to understand the conflicts, struggles, and negotiations that institutions, organizations, and individuals are likely to encounter. Decisions, interactions, and participation processes relating to carbon information, ranging from carbon trading to sustainable consumption, demands adequate empirical and theoretical knowledge that can help in integrating relevant carbon information into the socio-technical systems of everyday life. Third, collaboration surrounding carbon information, carbon accounting, and carbon neutrality will and should have become the focus of research and development for stakeholders across geographic boundaries, industrial sectors, and academic disciplines. Digital collaborations, especially those leveraging digital technologies such as AI, Blockchains, cloud computing, and data analytics, should track the social and environmental impacts of the human footprint on Earth, and act upon such impacts. Acting upon the understanding of human impacts, it is then possible to create a future that is not only carbon neutral but also sustainable for the planet and its inhabitants. In conclusion, as many aspects of the planet and humans have become datafied, digitized, and networked, carbon neutrality must take on a central role in guiding our conscious green digital transformation of many political, economic, social and psychological aspects of our societies according to the existing and emerging social science knowledge.
Data Availability Statement
The original contributions presented in the study are included in the article/Supplementary Material, further inquiries can be directed to the corresponding author.
Author Contributions
Conceptualization, YZ, H-TL, and C-LP; methodology, H-TL and C-LP; software, H-TL and C-LP; data curation, YZ, H-TL, and C-LP; writing—original draft preparation, H-TL, C-LP, and YZ; writing—review and editing, YZ, H-TL, and C-LP; visualization, H-TL, YZ, and C-LP; funding acquisition, YZ. All authors have read and agreed to the published version of the manuscript.
Funding
This research was funded mainly by the Department of Education of Guangdong Province for the project “Enterprise energy privilege trading scheme in Guangdong-Hong Kong-Macao Greater Bay Area” (Grant Number 2020WTSCX138), partly by the Department of Education of Guangdong Province in the project called “Smart App Design Innovation Research in the Age of New Business, Arts and Engineering Disciplines” (Grant Number 2019GXJK186), and partly by Guangzhou Nanfang College for the curriculum project of “Information Visualization Design” (Grant Number NFU 02-40250).
Conflict of Interest
The authors declare that the research was conducted in the absence of any commercial or financial relationships that could be construed as a potential conflict of interest.
Publisher’s Note
All claims expressed in this article are solely those of the authors and do not necessarily represent those of their affiliated organizations, or those of the publisher, the editors and the reviewers. Any product that may be evaluated in this article, or claim that may be made by its manufacturer, is not guaranteed or endorsed by the publisher.
Acknowledgments
The authors wish to express their gratitude to all colleagues who directly or indirectly contributed to the making of the VOSviewer, Bibliometrix, and Citespace software.
References
Altieri, K. E., Trollip, H., Caetano, T., Hughes, A., Merven, B., and Winkler, H. (2016). Achieving Development and Mitigation Objectives through a Decarbonization Development Pathway in South Africa. Clim. Pol. 16, S78–S91. doi:10.1080/14693062.2016.1150250
Andrews, C. J. (2008). Greenhouse Gas Emissions along the Rural-Urban Gradient. J. Environ. Plann. Manage. 51, 847–870. doi:10.1080/09640560802423780
Ang, B. W. (2004). Decomposition Analysis for Policymaking in Energy:. Energy Policy 32, 1131–1139. doi:10.1016/S0301-4215(03)00076-4
Aria, M., and Cuccurullo, C. (2017). Bibliometrix : An R-Tool for Comprehensive Science Mapping Analysis. J. Informetrics 11, 959–975. doi:10.1016/j.joi.2017.08.007
Ascui, F., and Lovell, H. (2011). As Frames Collide: Making Sense of Carbon Accounting. Acc. Auditing Acc. J 24, 978–999. doi:10.1108/09513571111184724
Bataille, C., Waisman, H., Colombier, M., Segafredo, L., and Williams, J. (2016a). The Deep Decarbonization Pathways Project (DDPP): Insights and Emerging Issues. Clim. Pol. 16, S1–S6. doi:10.1080/14693062.2016.1179620
Bataille, C., Waisman, H., Colombier, M., Segafredo, L., Williams, J., and Jotzo, F. (2016b). The Need for National Deep Decarbonization Pathways for Effective Climate Policy. Clim. Pol. 16, S7–S26. doi:10.1080/14693062.2016.1173005
Benjaminsen, G. (2017). The Bricolage of REDD+ in Zanzibar: from Global Environmental Policy Framework to Community forest Management. J. East. Afr. Stud. 11, 506–525. doi:10.1080/17531055.2017.1357103
Böhringer, C., Müller, A., and Schneider, J. (2015). Carbon Tariffs Revisited. J. Assoc. Environ. Res. Econom. 2, 629–672. doi:10.1086/683607
Boehmer-Christiansen, S. (2003). Science, Equity, and the War against Carbon. Sci. Technol. Hum. Values 28, 69–92. doi:10.1177/0162243902238496
Bowen, F., and Wittneben, B. (2011). Carbon Accounting. Acc. Auditing Acc. J 24, 1022–1036. doi:10.1108/09513571111184742
Boyack, K. W., and Klavans, R. (2010). Co-Citation Analysis, Bibliographic Coupling, and Direct Citation: Which Citation Approach Represents the Research Front Most Accurately? J. Am. Soc. Inf. Sci. 61, 2389–2404. doi:10.1002/asi.21419
Bressler, R. D. (2021). The Mortality Cost of Carbon. Nat. Commun. 12, 4467. doi:10.1038/s41467-021-24487-w
Bulkeley, H., and Betsill, M. M. (2003). Cities and Climate Change: Urban Sustainability and Global Environmental Governance. London, New York: Routledge.
Bumpus, A. G., and Liverman, D. M. (2008). Accumulation by Decarbonization and the Governance of Carbon Offsets. Econ. Geogr. 84, 127–155. doi:10.1111/j.1944-8287.2008.tb00401.x
Byers, E. A., Hall, J. W., and Amezaga, J. M. (2014). Electricity Generation and Cooling Water Use: UK Pathways to 2050. Glob. Environ. Change 25, 16–30. doi:10.1016/j.gloenvcha.2014.01.005
Cai, Y., Ramis Ferrer, B., and Luis Martinez Lastra, J. (2019). Building University-Industry Co-innovation Networks in Transnational Innovation Ecosystems: Towards a Transdisciplinary Approach of Integrating Social Sciences and Artificial Intelligence. Sustainability 11, 4633. doi:10.3390/su11174633
Chapman, R., and Boston, J. (2007). The Social Implications of Decarbonising the New Zealand Economy. Soc. Pol. J. N. Z., 104–136.
Chen, K.-S., and Liao, H.-T. (2020). “Mapping the Field of Educational Assessment and Professional Accreditation in Higher Education: Bibliographic Coupling and Co-Citation Analyses for Green and Digital Transformation,” in 2020 Management Science Informatization and Economic Innovation Development Conference (MSIEID, 18-20 Dec. 2020, Guangzhou, China), New York, NY, USA (IEEE), 281–284. doi:10.1109/MSIEID52046.2020.00058
Chen, C. (2004). Searching for Intellectual Turning Points: Progressive Knowledge Domain Visualization. Proc. Natl. Acad. Sci. 101, 5303–5310. doi:10.1073/pnas.0307513100
Chen, C. (2016). CiteSpace: A Practical Guide for Mapping Scientific Literature. New York: Nova Science Publishers, Inc.
Clarivate Analytics (2020). Research Areas (Categories/Classification). Web of Science Core Collection Help. Available at: http://images.webofknowledge.com//WOKRS535R111/help/WOS/hp_research_areas_easca.html (Accessed February 1, 2021).
Cobo, M. J., López-Herrera, A. G., Herrera-Viedma, E., and Herrera, F. (2011). Science Mapping Software Tools: Review, Analysis, and Cooperative Study Among Tools. J. Am. Soc. Inf. Sci. 62, 1382–1402. doi:10.1002/asi.21525
Cui, T., and Zhang, J. (2018). Bibliometric and Review of the Research on Circular Economy through the Evolution of Chinese Public Policy. Scientometrics 116, 1013–1037. doi:10.1007/s11192-018-2782-y
Diniz, E. H., Yamaguchi, J. A., Rachael dos Santos, T., Pereira de Carvalho, A., Alégo, A. S., and Carvalho, M. (2021). Greening Inventories: Blockchain to Improve the GHG Protocol Program in Scope 2. J. Clean. Prod. 291, 125900. doi:10.1016/j.jclepro.2021.125900
Donthu, N., Kumar, S., Mukherjee, D., Pandey, N., and Lim, W. M. (2021). How to Conduct a Bibliometric Analysis: An Overview and Guidelines. J. Bus. Res. 133, 285–296. doi:10.1016/j.jbusres.2021.04.070
Downie, J., and Stubbs, W. (2013). Evaluation of Australian Companies' Scope 3 Greenhouse Gas Emissions Assessments. J. Clean. Prod. 56, 156–163. doi:10.1016/j.jclepro.2011.09.010
Dury, P. (2008). The Rise of Carbon Neutral and Compensation Carbone. Term 14, 230–248. doi:10.1075/term.14.2.06dur
Elisha, I. L., and Viljoen, A. (2021). Trends in Rooibos Tea (Aspalathus Linearis) Research (1994-2018): A Scientometric Assessment. South Afr. J. Bot. 137, 159–170. doi:10.1016/j.sajb.2020.10.004
European Commission (2020). A New Industrial Strategy for a Green and Digital Europe. Available at: https://ec.europa.eu/commission/presscorner/detail/en/ip_20_416 (Accessed June 28, 2021).
Farnworth, E. (2018). We CAN Build a Carbon-Neutral World by 2050. Here’s How. World Economic Forum. Available at: https://www.weforum.org/agenda/2018/06/the-world-will-be-carbon-neutral-by-2050-but-at-what-cost/.
Fragkos, P., Tasios, N., Paroussos, L., Capros, P., and Tsani, S. (2017). Energy System Impacts and Policy Implications of the European Intended Nationally Determined Contribution and Low-Carbon Pathway to 2050. Energy Policy 100, 216–226. doi:10.1016/j.enpol.2016.10.023
Freeman, L. C. (1978). Centrality in Social Networks Conceptual Clarification. Soc. Networks 1, 215–239. doi:10.1016/0378-8733(78)90021-7
Fridell, M., Edwin, S., von Schreeb, J., and Saulnier, D. D. (2019). Health System Resilience: What Are We Talking about? A Scoping Review Mapping Characteristics and Keywords. Int. J. Health Pol. Manag. 9, 6–16. doi:10.15171/ijhpm.2019.71
Garfield, E. (1994). Research Fronts. Current Comments. Available at: https://clarivate.com/webofsciencegroup/essays/research-fronts/ (Accessed January 27, 2020)..
Garfield, E. (2004). Historiographic Mapping of Knowledge Domains Literature. J. Inf. Sci. 30, 119–145. doi:10.1177/0165551504042802
Geels, F. W., Sovacool, B. K., Schwanen, T., and Sorrell, S. (2017). Sociotechnical Transitions for Deep Decarbonization. Science 357, 1242–1244. doi:10.1126/science.aao3760
Geels, F. W. (2005). The Dynamics of Transitions in Socio-Technical Systems: A Multi-Level Analysis of the Transition Pathway from Horse-Drawn Carriages to Automobiles (1860-1930). Technol. Anal. Strateg. Manage. 17, 445–476. doi:10.1080/09537320500357319
Geels, F. W. (2010). Ontologies, Socio-Technical Transitions (To Sustainability), and the Multi-Level Perspective. Res. Pol. 39, 495–510. doi:10.1016/j.respol.2010.01.022
Geels, F. W. (2018). Socio-Technical Transitions to Sustainability. Oxford Res. Encycl. Environ. Sci. doi:10.1093/acrefore/9780199389414.013.587
Geels, F. W. (2019). Socio-technical Transitions to Sustainability: a Review of Criticisms and Elaborations of the Multi-Level Perspective. Curr. Opin. Environ. Sustainability 39, 187–201. doi:10.1016/j.cosust.2019.06.009
Gibassier, D., and Schaltegger, S. (2015). Carbon Management Accounting and Reporting in Practice. Sustainability 6, 340–365. doi:10.1108/SAMPJ-02-2015-0014
Gilley, B. (2017). Local Governance Pathways to Decarbonization in China and India. China Q. 231, 728–748. doi:10.1017/S0305741017000893
Gössling, S., Bredberg, M., Randow, A., Sandström, E., and Svensson, P. (2006). Tourist Perceptions of Climate Change: A Study of International Tourists in Zanzibar. Curr. Issues Tourism 9, 419–435. doi:10.2167/cit265.0
Gössling, S. (2009). Carbon Neutral Destinations: a Conceptual Analysis. J. Sustain. Tourism 17, 17–37. doi:10.1080/09669580802276018
Goldemberg, J., Coelho, S. T., and Guardabassi, P. (2008). The Sustainability of Ethanol Production from Sugarcane. Energy Policy 36, 2086–2097. doi:10.1016/j.enpol.2008.02.028
Green, J. F. (2013). Order Out of Chaos: Public and Private Rules for Managing Carbon. Glob. Environ. Polit. 13, 1, 25. doi:10.1162/GLEP_a_00164
Grübler, A., Nakićenović, N., and Victor, D. G. (1999). Dynamics of Energy Technologies and Global Change. Energy Policy 27, 247–280. doi:10.1016/S0301-4215(98)00067-6
Hansen, D. L., Schneiderman, B., and Smith, M. A. (2020). Analyzing Social Media Networks with NodeXL: Insights from a Connected World. Second edition. Amsterdam: Morgan Kaufmann.
Harvey, F. (2020). China Pledges to Become Carbon Neutral before 2060. The Guardian. Available at: https://www.theguardian.com/environment/2020/sep/22/china-pledges-to-reach-carbon-neutrality-before-2060.
Hawken, P. (2017). Drawdown: The Most Comprehensive Plan Ever Proposed to Reverse Global Warming. New York, New York: Penguin Books.
Helm, D. (2010). Government Failure, Rent-Seeking, and Capture: the Design of Climate Change Policy. Oxford Rev. Econ. Pol. 26, 182–196. doi:10.1093/oxrep/grq006
Higham, J. E. S., and Cohen, S. A. (2011). Canary in the Coalmine: Norwegian Attitudes towards Climate Change and Extreme Long-Haul Air Travel to Aotearoa/New Zealand. Tourism Manage. 32, 98–105. doi:10.1016/j.tourman.2010.04.005
Höhne, N., Kuramochi, T., Warnecke, C., Röser, F., Fekete, H., Hagemann, M., et al. (2017). The Paris Agreement: Resolving the Inconsistency between Global Goals and National Contributions. Clim. Pol. 17, 16–32. doi:10.1080/14693062.2016.1218320
Hopke, J. E., and Hestres, L. E. (2018). Visualizing the Paris Climate Talks on Twitter: Media and Climate Stakeholder Visual Social Media during COP21. Soc. Media + Soc. 4, 205630511878268. doi:10.1177/2056305118782687
Hübler, M., and Löschel, A. (2013). The EU Decarbonisation Roadmap 2050-What Way to Walk? Energy Policy 55, 190–207. doi:10.1016/j.enpol.2012.11.054
IPCC (2018). “Global Warming of 1.5 °C, an IPCC Special Report on the Impacts of Global Warming of 1.5 °C above Pre-industrial Levels and Related Global Greenhouse Gas Emission Pathways,” in The Context of Strengthening the Global Response to the Threat of Climate Change, Sustainable Development, and Efforts to Eradicate Poverty. Editors V. Masson-Delmotte, P. Zhai, H.-O. Pörtner, D. Roberts, J. Skea, P. R. Shuklaet al. (Switzerland: IPCC). Available at: http://ipcc.ch/report/sr15/.
Jarneving, B. (2007). Bibliographic Coupling and its Application to Research-Front and Other Core Documents. J. Informetrics 1, 287–307. doi:10.1016/j.joi.2007.07.004
Kibwami, N., and Tutesigensi, A. (2016). Enhancing Sustainable Construction in the Building Sector in Uganda. Habitat Int. 57, 64–73. doi:10.1016/j.habitatint.2016.06.011
Köhler, J., Geels, F. W., Kern, F., Markard, J., Onsongo, E., Wieczorek, A., et al. (2019). An Agenda for Sustainability Transitions Research: State of the Art and Future Directions. Environ. Innovation Soc. Trans. 31, 1–32. doi:10.1016/j.eist.2019.01.004
Kolk, A., Levy, D., and Pinkse, J. (2008). Corporate Responses in an Emerging Climate Regime: The Institutionalization and Commensuration of Carbon Disclosure. Eur. Account. Rev. 17, 719–745. doi:10.1080/09638180802489121
Koteyko, N. (2012). Managing Carbon Emissions: A Discursive Presentation of 'market-Driven Sustainability' in the British media. Lang. Commun. 32, 24–35. doi:10.1016/j.langcom.2011.11.001
Lilliestam, J., Bielicki, J. M., and Patt, A. G. (2012). Comparing Carbon Capture and Storage (CCS) with Concentrating Solar Power (CSP): Potentials, Costs, Risks, and Barriers. Energy Policy 47, 447–455. doi:10.1016/j.enpol.2012.05.020
Lohmann, L. (2009). Toward a Different Debate in Environmental Accounting: The Cases of Carbon and Cost-Benefit. Account. Organ. Soc. 34, 499–534. doi:10.1016/j.aos.2008.03.002
Loulou, R., Goldstein, G., and Noble, K. (2004). Documentation for the MARKAL Family of Models. Energy Technol. Syst. Anal. Program., 65–73.
MacDonald, K. I., and Dressler, V. (2018). Using Citation Analysis to Identify Research Fronts: A Case Study with the Internet of Things. Sci. Technol. Libraries 37, 171–186. doi:10.1080/0194262X.2017.1415183
Mallapaty, S. (2020). How China Could Be Carbon Neutral by Mid-Century. Nature 586, 482–483. doi:10.1038/d41586-020-02927-9
Marcucci, A., and Fragkos, P. (2015). Drivers of Regional Decarbonization through 2100: A Multi-Model Decomposition Analysis. Energ. Econ. 51, 111–124. doi:10.1016/j.eneco.2015.06.009
Marres, N. (2011). The Costs of Public Involvement: Everyday Devices of Carbon Accounting and the Materialization of Participation. Econ. Soc. 40, 510–533. doi:10.1080/03085147.2011.602294
Mathy, S., Criqui, P., Knoop, K., Fischedick, M., and Samadi, S. (2016). Uncertainty Management and the Dynamic Adjustment of Deep Decarbonization Pathways. Clim. Pol. 16, S47–S62. doi:10.1080/14693062.2016.1179618
McGlade, C., and Ekins, P. (2014). Un-burnable Oil: An Examination of Oil Resource Utilisation in a Decarbonised Energy System. Energy Policy 64, 102–112. doi:10.1016/j.enpol.2013.09.042
Meseguer-Sánchez, V., Gálvez-Sánchez, F. J., Molina-Moreno, V., and Wandosell-Fernández-de-Bobadilla, G. (2021). The Main Research Characteristics of the Development of the Concept of the Circular Economy Concept: A Global Analysis and the Future Agenda. Front. Environ. Sci. 9, 304. doi:10.3389/fenvs.2021.704387
METI (2020). “Green Growth Strategy through Achieving Carbon Neutrality in 2050” Formulated. Japan: Ministry of Economy, Trade and Industry (METI). Available at: https://www.meti.go.jp/english/press/2020/1225_001.html.
Milne, M. J., and Grubnic, S. (2011). Climate Change Accounting Research: Keeping it Interesting and Different. Acc. Auditing Account. J 24, 948–977. doi:10.1108/09513571111184715
Moher, D., Liberati, A., Tetzlaff, J., and Altman, D. G.The PRISMA Group (2009). Preferred Reporting Items for Systematic Reviews and Meta-Analyses: the PRISMA Statement. Plos Med. 6, e1000097. doi:10.1371/journal.pmed.1000097
N. Stern (Editor) (2007). The Economics of Climate Change: The Stern Review (Cambridge, UK New York: Cambridge University Press).
Nakićenović, N. (1996). Decarbonization: Doing More with Less. Technol. Forecast. Soc. Change 51, 1–17. doi:10.1016/0040-1625(95)00167-010.1016/s0040-1625(96)90029-3
Onnela, J.-P., Saramäki, J., Kertész, J., and Kaski, K. (2005). Intensity and Coherence of Motifs in Weighted Complex Networks. Phys. Rev. E 71, 065103. doi:10.1103/PhysRevE.71.065103
Oshiro, K., Kainuma, M., and Masui, T. (2016). Assessing Decarbonization Pathways and Their Implications for Energy Security Policies in Japan. Clim. Pol. 16, S63–S77. doi:10.1080/14693062.2016.1155042
Pallesen, T. (2016). Valuation Struggles over Pricing - Determining the worth of Wind Power. J. Cult. Econ. 9, 527–540. doi:10.1080/17530350.2016.1212084
Payne, C. (2020). The Decarbonisation Divide. Nat. Hum. Behav. 4, 236. doi:10.1038/s41562-020-0843-8
Persson, O. (1994). The Intellectual Base and Research Fronts ofJASIS 1986-1990. J. Am. Soc. Inf. Sci. 45, 31–38. doi:10.1002/(sici)1097-4571(199401)45:1<31:aid-asi4>3.0.co;2-g
Peters, G. P., Andrew, R. M., Solomon, S., and Friedlingstein, P. (2015). Measuring a Fair and Ambitious Climate Agreement Using Cumulative Emissions. Environ. Res. Lett. 10, 105004. doi:10.1088/1748-9326/10/10/105004
Pidgeon, N., and Demski, C. C. (2012). From Nuclear to Renewable: Energy System Transformation and Public Attitudes. Bull. At. Sci. 68, 41–51. doi:10.1177/0096340212451592
Pigliautile, I., Castaldo, V. L., Makaremi, N., Pisello, A. L., Cabeza, L. F., and Cotana, F. (2019). On an Innovative Approach for Microclimate Enhancement and Retrofit of Historic Buildings and Artworks Preservation by Means of Innovative Thin Envelope Materials. J. Cult. Heritage 36, 222–231. doi:10.1016/j.culher.2018.04.017
Pihl, E., Alfredsson, E., Bengtsson, M., Bowen, K. J., Cástan Broto, V., Chou, K. T., et al. (2021). Ten New Insights in Climate Science 2020 - a Horizon Scan. Glob. Sustain. 4, e5. doi:10.1017/sus.2021.2
Pye, S., and Bataille, C. (2016). Improving Deep Decarbonization Modelling Capacity for Developed and Developing Country Contexts. Clim. Pol. 16, S27–S46. doi:10.1080/14693062.2016.1173004
Pye, S., McGlade, C., Bataille, C., Anandarajah, G., Denis-Ryan, A., and Potashnikov, V. (2016). Exploring National Decarbonization Pathways and Global Energy Trade Flows: a Multi-Scale Analysis. Clim. Pol. 16, S92–S109. doi:10.1080/14693062.2016.1179619
Quimby, C. C., and Angelique, H. (2011). Identifying Barriers and Catalysts to Fostering Pro-environmental Behavior: Opportunities and Challenges for Community Psychology. Am. J. Commun. Psychol. 47, 388–396. doi:10.1007/s10464-010-9389-7
Rogelj, J., den Elzen, M., Höhne, N., Fransen, T., Fekete, H., Winkler, H., et al. (2016). Paris Agreement Climate Proposals Need a Boost to Keep Warming Well below 2 °C. Nature 534, 631–639. doi:10.1038/nature18307
Schwartz, J. (2021). A Carbon Calculation: How Many Deaths Do Emissions Cause? the New York Times. Available at: https://www.nytimes.com/2021/07/29/climate/carbon-emissions-death.html (Accessed August 1, 2021).
Scott, D., Hall, C. M., and Gössling, S. (2019). Global Tourism Vulnerability to Climate Change. Ann. Tourism Res. 77, 49–61. doi:10.1016/j.annals.2019.05.007
Small, H. (1999). Visualizing Science by Citation Mapping. J. Am. Soc. Inf. Sci. 50, 799–813. doi:10.1002/(sici)1097-4571(1999)50:9<799:aid-asi9>3.0.co;2-g
Smith, C. B., and Fletcher, W. D. (2020). Reaching Net Zero: What it Takes to Solve the Global Climate Crisis. 1st ed. Cambridge: Elsevier.
Sovacool, B. K. (2017). Contestation, Contingency, and justice in the Nordic Low-Carbon Energy Transition. Energy Policy 102, 569–582. doi:10.1016/j.enpol.2016.12.045
Stadel, A., Eboli, J., Ryberg, A., Mitchell, J., and Spatari, S. (2011). Intelligent Sustainable Design: Integration of Carbon Accounting and Building Information Modeling. J. Prof. Issues Eng. Educ. Pract. 137, 51–54. doi:10.1061/(ASCE)EI.1943-5541.0000053
Steckel, J. C., Jakob, M., Marschinski, R., and Luderer, G. (2011). From Carbonization to Decarbonization?-Past Trends and Future Scenarios for China's CO2 Emissions. Energy Policy 39, 3443–3455. doi:10.1016/j.enpol.2011.03.042
Strachan, N., and Kannan, R. (2008). Hybrid Modelling of Long-Term Carbon Reduction Scenarios for the UK. Energ. Econ. 30, 2947–2963. doi:10.1016/j.eneco.2008.04.009
Strachan, N., Pye, S., and Kannan, R. (2009). The Iterative Contribution and Relevance of Modelling to UK Energy Policy. Energy Policy 37, 850–860. doi:10.1016/j.enpol.2008.09.096
Tapio, P., Banister, D., Luukkanen, J., Vehmas, J., and Willamo, R. (2007). Energy and Transport in Comparison: Immaterialisation, Dematerialisation and Decarbonisation in the EU15 between 1970 and 2000. Energy Policy 35, 433–451. doi:10.1016/j.enpol.2005.11.031
Twidale, S. (2021). Global Initiative Aims to Bring Transparency to Voluntary Carbon Markets. Reuters. Available at: https://www.reuters.com/business/environment/global-initiative-aims-bring-transparency-voluntary-carbon-markets-2021-07-29/(Accessed August 1, 2021).
Unruh, G. C. (2000). Understanding Carbon Lock-In. Energy Policy 28, 817–830. doi:10.1016/S0301-4215(00)00070-7
van der Ven, H., Bernstein, S., and Hoffmann, M. (2017). Valuing the Contributions of Nonstate and Subnational Actors to Climate Governance. Glob. Environ. Polit. 17, 1–20. doi:10.1162/GLEP_a_00387
van Eck, N. J., and Waltman, L. (2014). “Visualizing Bibliometric Networks,” in In Measuring Scholarly Impact. Editors Y. Ding, R. Rousseau, and D. Wolfram (Cham: Springer International Publishing), 285–320. doi:10.1007/978-3-319-10377-8_13
Vandenbergh, M., and Steinemann, A. (2007). The Carbon-Neutral Individual. N. Y. Univ. L. Rev. 82, 1673–1745.
Vesty, G. M., Telgenkamp, A., and Roscoe, P. J. (2015). Creating Numbers: Carbon and Capital Investment. Account. Auditing Account. J. 28, 302–324. doi:10.1108/AAAJ-10-2013-1507
Waheed, B., Khan, F., Veitch, B., and Hawboldt, K. (2011). An Integrated Decision-Making Framework for Sustainability Assessment: A Case Study of Memorial University. High Educ. Pol. 24, 481–498. doi:10.1057/hep.2011.17
Wang, Z., Liao, H.-T., Zhou, Y., and Pang, S. (2020). “Toward the Design of Socio-Technical Systems for Sustainability: A Scientometric Analysis,” in Proceedings of the 6th International Conference on Humanities and Social Science Research (ICHSSR2020), Hangzhou, China, 2020.04.11, Dordrecht (Atlantis Press). doi:10.2991/assehr.k.200428.095
Wang, R.-Y., Lin, P.-a., Chu, J.-Y., Tao, Y.-H., and Ling, H.-C. (2021). A Decision Support System for Taiwan's forest Resource Management Using Remote Sensing Big Data. Enterprise Inf. Syst., 1–22. doi:10.1080/17517575.2021.1883123
Wickham, H. (2011). The Split-Apply-Combine Strategy for Data Analysis. J. Stat. Soft. 40, 1–29. doi:10.18637/jss.v040.i01
Yigitcanlar, T., and Lee, S. H. (2014). Korean Ubiquitous-Eco-City: A Smart-Sustainable Urban Form or a Branding Hoax? Technol. Forecast. Soc. Change 89, 100–114. doi:10.1016/j.techfore.2013.08.034
Yu, Y., Li, Y., Zhang, Z., Gu, Z., Zhong, H., Zha, Q., et al. (2020). A Bibliometric Analysis Using VOSviewer of Publications on COVID-19. Ann. Transl Med. 8, 816. doi:10.21037/atm-20-4235
Keywords: decarbonization, carbon information, sustainable consumption and production, carbon accounting, social science, just transition, socio-technical transition, carbon management
Citation: Zhang Y, Pan C-L and Liao H-T (2021) Carbon Neutrality Policies and Technologies: A Scientometric Analysis of Social Science Disciplines. Front. Environ. Sci. 9:761736. doi: 10.3389/fenvs.2021.761736
Received: 20 August 2021; Accepted: 20 September 2021;
Published: 18 October 2021.
Edited by:
Tsun Se Cheong, Hang Seng University of Hong Kong, Hong Kong, SAR ChinaReviewed by:
Benson Lam, Hang Seng University of Hong Kong, Hong Kong, SAR ChinaYongping Sun, Hubei University of Economics, China
Copyright © 2021 Zhang, Pan and Liao. This is an open-access article distributed under the terms of the Creative Commons Attribution License (CC BY). The use, distribution or reproduction in other forums is permitted, provided the original author(s) and the copyright owner(s) are credited and that the original publication in this journal is cited, in accordance with accepted academic practice. No use, distribution or reproduction is permitted which does not comply with these terms.
*Correspondence: Han-Teng Liao, h.liao@oxon.org