- 1Facultad de Ingeniería, Universidad Autónoma de Querétaro, Santiago de Querétaro, México
- 2Red de Investigación OAC optimización, Automatización y Control, Querétaro, México
- 3Centro de Investigación en Óptica (CIO), León, México
- 4Ingeniería Mecatrónica, Universidad Politecnica de Chiapas, Tuxtla Gutiérrez, Mexico
Post-consumer polymers require viable actions to transfer value to the final consumer to reduce environmental impact. Worldwide, initiatives are being developed to promote the culture of recycling and thus reduce waste generation. These initiatives seek to make the work of thousands of people visible, encourage job creation, and promote businesses through incentive schemes for effective separation, collection, classification, reuse, and recycling, in the hands of consumers, collectors, and scavenges. It is necessary to emphasize that Mexico needs to develop techniques to increase the productivity of collection centers for recyclable materials and to face the challenges that recycling implies. For these reasons, the Mexican government has spoken of waste management as a national priority due to the COVID-19 pandemic, which increased urban solid waste between 3.3 % and 16.5 % in addition to what was generated under normal conditions. Also, the recycling chain provides economic income to more than 35 thousand Mexicans. Polyethylene terephthalate (PET) is an example of the most notable circular economy in Mexico because 60 % of PET bottles are recycled. Therefore, this article addresses the methods and systems in the management of urban solid waste. It focuses on post-consumer recycled plastic bottles to provide an overview of cost-effective strategies for designing and developing an affordable sorting system in Mexico from the academic field. In addition, to simplify the solution that we propose, it is recommended to combine optical techniques such as infrared spectroscopy and Raman spectroscopy with others methods that work together, such as computer vision, to develop affordable systems that address the limitations of mechanical systems.
1 Introduction
The viable disposal of post-consumer polymers can be defined for this paper as the political, economic, social, and technological actions necessary to control and supply the demands of the different industries that require the use of recyclable polymers. Recycling is a continuous process and helps develop economies around the world. Generating new contributions to make the operations of transfer, classification, storage more efficient produces economic development and care for the environment (Wen et al., 2021). As the world population increases, also the viable disposal of post-consumer polymers should increase. According to data of the United Nations, the world population reached 7.7 billion in mid-2019 and estimates that the world population will be at least 8.5 billion by 2030 and 9.7 billion by 2050 (United Nations and Affairs, 2019). Thus, world population growth contributes to the exploitation of natural resources and environmental pollution. Notwithstanding, waste plastics recycling is used to make different plastic products considered low-value, like bags and toys (Qu et al., 2019). The plastic recycling rate is around 9% worldwide (Wen et al., 2021), but it varies by region. However, many benefits have been derived from the industrialization of recyclable materials, such as the generation of jobs and the reduction of energy to process them. They have also brought unwanted consequences such as creating new plastic products that are increasingly difficult to classify. In recent years, the use of plastics for domestic purposes has increased to the extent that China banned the entry of plastics for recycling (Qu et al., 2019). In addition, recyclable polymers are excellent sources of pollution in cities and seas (Shen et al., 2020). Secondly, as stated in (Jung et al., 2021), it is critical to recycle household waste and to establish circular economy policies to avoid the substantial impact on pollution. A review of urban solid waste management in China concluded that food waste dominates urban solid waste and shows that the highest proportion of waste is in food with 61.2%, polymers 9.8%, and paper 9.6% (Gu et al., 2017). Furthermore, around the world, 60 million metric tons of PET are produced per year. Twenty million metric tons are to produce bottles, which is equivalent to one million sold every minute. It is estimated that annual consumption will reach half a trillion by 2021 (Lundell and Thomas, 2020). In the Mexican industry, recycling plastic bottles directly employs around 98 thousand people and generates more than one million indirect jobs (de Coca-Cola, IMCC, 2019). Thirty-five thousand Mexicans are benefited from the collection and stockpiling. Also, atypical phenomena just as the COVID-19 worldwide pandemic increased the use of single-use plastics and reduced the recycling of plastics due to closures of non-essential activities (Ardusso et al., 2021). Various organizations and countries have set a goal for the next 10 years to reduce single-use plastics and increase the number of tons recycled (Kumagai et al., 2020). The correct classification of recovered resins can maintain properties very similar to virgin resins, so there are various applications in which developers can manipulate them for quality products. Therefore, it is worth examining available technologies and trends for producing post-consumer resins, as well as plastics identification tools and plastics separation processes. The following sections will address the existing technologies to process and classify polymers present in various fields of the industry and end with a conclusion referring to which systems could be implemented in Mexico to develop technology with affordable designs that allow managing better plastic waste.
2 The Importance of Polymers in the Industry
Every year, 300 million metric tons of plastic are produced worldwide, and depending on the country, it is estimated that between 9 and 10% are recycled, 10% are burned, and 80% are discarded, or not given a second use (Lundell and Thomas, 2020). The growth of plastic production worldwide reached 280 million tons in 2011, 322 million tons in 2015, and it is estimated that by 2021 it will be around 400 to 414 million tons. This estimate does not consider the impact of COVID-19 (Sahin and Kirim, 2018), (Grigore, 2017). More than 80% of plastics in Europe are composed of PET, polystyrene (PS), polyethylene (PE), polyurethane, polyvinyl chloride (PVC), polypropylene (PP), among others. Plastics are used in various fields of industry for the different properties that allow them to be used in combination with other materials and are present in packaging materials, agriculture, automotive, and building construction (Thakur et al., 2018). Thermoplastic polymers such as polyetheretherketone (PEEK), poly (methyl methacrylate) (PMMA), and polyimide (PI) are widely used in the fields of aerospace, optical engineering, and biological engineering industry (Yan et al., 2021). In the electronics industry, the presence of polymers is mainly based on styrenes such as PS, acrylonitrile butadiene styrene (ABS), and high-impact polystyrene (HIPS). Cars have up to 27 different types of plastics (Buekens and Zhou, 2014), but ABS and PP are the two most widely used plastics in the automotive industry (Camacho and Karlsson, 2001). In comparison, more than 150 resins and 230 types of additives are reportedly manufactured (Kumagai et al., 2020). It should be noted that plastic recycling developments from electronic components are increasing. Such is the case in (Grigorescu et al., 2020) that generates thermoplastic compounds based on recycled polypropylene and waste printed circuit boards. In (Jaidev et al., 2021), they use computer keyboards to formulate a mixture and promote recycling in electronic waste applying a methodology to improve the properties of polymers and return to the supply chain. In recycling solid plastic waste, there are methods for electronic materials when they end their life cycle with the possibility that they can be recycled. In addition, most electronic waste is mixed with metals that have a high value for the recycling industry (Qu et al., 2006).
2.1 The Gap Between Countries Without Infrastructure to Process Their Solid Waste
Most industrial countries with advanced waste management systems are far from adequately collecting and recycling disposable polymers. That is why in the last decade, one of their actions has been to send their waste to Asian countries to classify, clean, and process it. One of the essential consequences was that China banned importing 24 types of solid waste, including plastic waste. Some Asian countries and regions experienced significant increases in imports of plastic waste from 2016 to 2018. Asian countries are beginning to limit imports of plastic waste from other countries (Liang et al., 2021; Wen et al., 2021). The prohibitions by China can be understood because of the Jiangcungou landfill in the Shaanxi province that received 10,000 tons of waste per day. For that reason, the mega-landfill was filled 25 years earlier than estimated (Lundell and Thomas, 2020). There is a large gap between countries regarding treatment systems and technical advances in plastic waste management. Waste disposal in developing countries has a higher environmental cost this implies that the countries that exported their waste must take the necessary measures to collect and properly recycle disposable polymers. Mexico, being a developing country, requires joining efforts to face this problem of plastic waste (Qu et al., 2019). In general, current recovery technologies do not include the previous treatment of the waste. Each technology varies and depends on the material being treated because these may need different previous treatments to separate and purify the waste. The level of scrubbing that the polymers can achieve through pretreatment technologies directly affects the potential for waste recovery (van Ewijk et al., 2018). There is research aimed at finding better ways to live with polymers and propose alternatives to replace them and thus reduce their use or use their physical and mechanical properties (Ren et al., 2015). The construction field has shown interest in finding applications in thermal insulation using post-consumer PET physical and mechanical properties (Foti and Lerna, 2020). In (Foti, 2016) they experimented with plastic waste, using them as structural reinforcement elements to be applied in buildings and structures. Current trends seek to begin to value the use of new and sustainable raw materials such as biomass (Langsdorf et al., 2021) and degradation and biodegradation techniques (Grigore, 2017; Wang et al., 2019a). In such a way that in the following decades, the classification and treatment of polymers will continue to be topics of interest to develop technological systems that help reduce the contamination of polymers.
2.2 Importance of Polymers Classification
In Mexico, separating ferrous, non-ferrous materials and paper, cardboard from polymers, among other materials from mixed waste, requires a large amount of labor. Therefore, the proper classification of polymers in developing countries represents an economic activity for individuals and corporations that contribute to the collection, storage, transfer, and processing of different recyclable materials (de Coca-Cola, IMCC, 2019; Qu et al., 2019; cuentame.inegi.org.mx, 2020). More than 44 million tons of waste are generated annually, and the figure is expected to increase to 65 million by 2030. In Mexico, only 5% is recycled or recovered, equivalent to about 2.2 million tons yearly (Josefa González Blanco Ortíz Mena, 2019). China’s ban on plastics imports causes a shortage of recycled materials, leading to higher product prices throughout the supply chain. The dependence on recycled materials from foreign waste in various countries globally, including Mexico, could lead these countries to switch to raw materials or household waste (Qu et al., 2019). In Mexico, as of January 1st, 2021, through the Ministry of Environment and Natural Resources, the import and export of plastic waste are restricted by the Plastics Amendment of the Basel Convention. The preceding is by the trade policies implemented in recent years by several countries to curtail the introduction of plastic waste (de Medio Ambiente y Recursos Naturales, 2021). Therefore, Latin American developing countries require systems that provide solutions to move, classify and process solid waste after its useful life (Villegas Pinuer et al., 2021).
3 Methods for Handling Solid Plastic Waste
According to a World Bank report published in 2012 (Hoornweg and Bhada-Tata, 2012; Fazeli et al., 2016), the generation of urban solid waste worldwide reached 1.3 billion tonnes per year. In 2025 and 2050, it is expected to increase to 2.2 billion tonnes per year and 4.2 billion tonnes per year (Fazeli et al., 2016). Each country must develop public policies in the area of handling plastic waste. Methods and developments must adapt and evolve by region, such as the case of landfills in Mexico, which can be official or clandestine, in which polymers are dumped along with the organic waste. There are methods to manipulate plastic waste, such as incineration, but they directly affect the environment because they form harmful ash and carbon dioxide (Sharma et al., 2020). Another example is the handling of plastic waste in incineration, and landfills woven from PET fiber enter the biosphere carbon dioxide (Chu et al., 2021). Another method is chemical recycling, in which plastic waste is converted into chemical raw materials and even fuels, as well as mechanical recycling methods used to form the product with the same characteristics of a virgin resin (Thakur et al., 2018) and the method by disassembling electronic waste housings (Qu et al., 2006). Recycling rates decrease with a low population density in rural communities. They occur due to the distance from recycling centers, which contributes to recycling not adding to a circular economy (Kreiger et al., 2013).
3.1 Landfills in Mexico
Worldwide annually, around 150 million tons of plastics end up in landfills (Ragaert et al., 2017). Open landfills are 23 times more dangerous to the environment than carbon dioxide (Chowdhury, 2021). PET bottles in landfills release and disperse the hydrosphere, pedosphere, and atmosphere more than 1,108 tons of antimony (Chu et al., 2021). Landfill gas is estimated to account for more than half of greenhouse gas emissions, yet it remains a popular form of solid waste management worldwide (Majdinasab and Yuan, 2017). A PET bottle takes around 500 years to decompose in landfills, whereas a bottle in the ocean will decompose into micro-plastics that will last 500 years (Lundell and Thomas, 2020). The municipalities in Mexico are in charge of the functions of integral management of urban solid waste, see Table 1, which consists of the collection, transfer, treatment, and final disposal. Still, they lack projects that guarantee integral management of urban solid waste (de Medio Ambiente y Recursos Naturales, 2020). Therefore, in Mexico, there are efforts to identify potential areas for the location of landfills (Saldaña Durán and Nájera González, 2019), despite the environmental impact they entail. On the other hand, there are also efforts to design semi-automatic systems for the reuse of recyclable materials such as glass, a material that is not as valued (Guerrero et al., 2021). Mexico generated 102,895 tons of waste daily in 2017. 83.93% of tons of waste was collected, and 78.54% was transferred to final disposal sites, unlike countries such as Switzerland, the Netherlands, Germany, Belgium, Sweden, Austria, and Denmark; where the final disposal of waste is less than 5% in sanitary landfills. It is estimated that in Mexico, the 16.07% of tons of waste that is not collected plus the 5.39% that has a separation process by the government contributes to the recycling of only 9.63% of the waste generated (de Medio Ambiente y Recursos Naturales, 2017b). Europe has shown an increase in recycling and energy recovery, which has resulted in less waste being thrown into landfills (Ragaert et al., 2017). The environment requires initiatives from society to generate a positive impact, and it is imperative to improve the classification of waste that reaches landfills. For example, in (Turner and Filella, 2021), it is proposed that garbage be classified as hazards depending on the concentrations of lead they have, mainly in plastics that have already been recycled to avoid that these contaminate other materials and natural resources such as air, soil, and water.
3.2 Incineration
Modern incineration plants, economically speaking, are very profitable because they can run continuously all year round with only one or two scheduled shutdowns (Buekens and Zhou, 2014). The incineration of mixed plastic waste has significant consequences on the environment because it releases toxic substances into the air, such as dioxins, a severe and persistent environmental pollutant (Grigore, 2017; Jeswani et al., 2021). Incineration is classified into two main categories, the method of combustion and gasification (Al-Salem et al., 2009). The incineration industry in which plastic waste is burned is used to generate electrical energy. It is accepted as a low-cost energy source and an adequate waste disposal method since no initial sorting is necessary of the material (Sadat-Shojai and Bakhshandeh, 2011; Sahin and Kirim, 2018). Polymers have a higher calorific value than carbon. A ton of plastics represents 1.3 tons of carbon (Kang and Schoenung, 2005). In 2013, of the 78 million tons produced for plastic packaging, 14% was used for incineration worldwide. Japan reported in 2018 more than 5 million tons of household plastic waste to generate energy (Kumagai et al., 2020). In the United States, only 12.5% of municipal solid waste is incinerated. It is estimated that the industry will grow at an annual rate of 5%, generated by the increases in solid waste. Still, since the use of incineration varies widely throughout the world, the estimate may vary. In Europe, there are around 231 incinerators in operation, and as of 2016, 41.6% of plastic waste is burned. China has 300 operating plants, and another 103 are being built or planned (David Azoulay, CIEL et al., 2019). In Morris (2005), a study was carried out comparing residential recycling with the collection and disposal of those same materials in a waste-to-energy combustion facility used to generate electricity for sale in the regional energy network as well as with the gas generated by landfills, and they showed that recycling recyclable materials saves more energy than landfills and incineration. Recycling plastic bottles has more advantages despite the use of fuel and energy involved in transporting, sorting, storing, and cleaning them (Grigore, 2017; Hull et al., 2020). Table 2 summarizes the incineration and landfill method and the environmental consequences if it continues to be implemented in Mexico.
3.3 Mechanical Recycling
Conventional mechanical recycling is the physical-mechanical process of recovering solid plastic waste that can be mechanically manufactured. The more complex and polluted the waste is, often contaminated with food particles, the more difficult it is to recycle it mechanically (Ragaert et al., 2017). The separation, washing, and waste preparation are essential to produce high-quality end products using mechanical recycling (Al-Salem et al., 2009). Such is the case of the disassembly of plastic components in electronics that with a suitable classification, the purity of the resins can be improved, and the value of the recycled resins increases (Qu et al., 2006). Mechanical recycling uses plastic which is collected, separated, cleaned, crushed, pelletized, and enters conversion and injection processing cycles, then re-processed and mixed to produce a new component, but without modifying the chemical composition of the material (Sadat-Shojai and Bakhshandeh, 2011; Hamad et al., 2013; Grigore, 2017; Jeswani et al., 2021). Recyclable polymers change their properties due to the treatments and substances to which they are exposed, such as wetting agents that have been shown to alter the buoyancy of polymeric materials (Wang et al., 2014). A complication in industrial processing involves a series of mechanical treatments that in recyclable car parts, aging affects hydrophobicity, and if flotation systems are used, it can be further affected (Fraunholcz, 2004). Although thermoplastic waste can lose its initial properties approximately between 70 and 80%, with the proper additive treatment, it can regain its initial properties (Al-Salem et al., 2009). Due to the low cost of mechanical recycling, most PET fibers waste is recycled in this way. It is important to emphasize that both mechanical recycling and incineration, when processing PET, release around 784,284 tons of antimony into the atmosphere (Chu et al., 2021). In the same way, depositing waste to the landfill or processing it through mechanical recycling also negatively impacts the environment.
3.4 Chemical Recycling
Chemical recycling uses advanced technology processes that convert plastic materials. Chemical recycling is exclusive to plastics, although various investigations use it to apply it to biomass. Chemical recycling consists of the depolymerization of polymers into monomers, decomposing the molecules of plastics into raw materials (Hamad et al., 2013) that can be used generally in liquids or gases, which are suitable for use as raw material for the production of new petrochemicals and plastics (Al-Salem et al., 2009). Twelve chemical reactions are used to decompose polymers. The already commercialized techniques are glycolysis and methanolysis, but for degradation research questions, pyrolysis and gasification stand out (Buekens and Zhou, 2014; Wang et al., 2014; Grigore, 2017). Likewise, pyrolysis is the most attractive technology (Sahin and Kirim, 2018; Kumagai et al., 2020). In pyrolysis, plastics are crushed and subsequently melted to be refined and converted into diesel, and other petrochemical products (Horvat and Ng, 1999). A study in Jeswani et al. (2021) suggests that pyrolysis has up to 50% less impact on climate change compared to energy recovery, mechanical recycling, and the production of virgin fossil resources. The creation of these new plastics used less oxygen than gasification. In chemolysis, the PET waste can be converted to monomers and polymerized again, but it requires a prior selection of materials and high quality. It applies to specific condensation polymers such as polyesters, polyurethanes, polyamides, and polyacetals. This method would imply a higher cost (Lin et al., 2011; Ragaert et al., 2017). Another way of converting waste into energy is by the gasification method that melts plastics with oxygen and steam at temperatures between 1,200 and 1,500°C. It does not generate toxins such as dioxins or furans (Ragaert et al., 2017). Despite these benefits, gasification plants are not competitive when compared to the low prices of natural gas. Chemical recycling is an alternative to recycling that eliminates some of the limitations of mechanical recycling and incineration, but with the restriction that chemical recycling has higher costs and requirements for its implementation (Hamad et al., 2013; Grigore, 2017). If the environmental impact of implementing this method in PET recycling is taken into account, it releases less than 25 tons of antimony into the atmosphere. By way of conclusion, the amount of PET tissue recycled through mechanical recycling is 7,264,360 tons, the incineration of PET is 2,636,459 tons, and the amount of PET that was chemically recycled was the lowest with 228,106 tons (Chu et al., 2021).
3.5 Recycling of Plastics by Disassembling Electronics
A press release issued by the Secretaría de Medio Ambiente y Recursos Naturales (SEMARNAT) reported that around 1.1 million tons of electronic and electrical waste is produced annually in Mexico, and a growth of 17% is estimated for 2026. In 2019, global e-waste production reached 53.6 million tons. Electronic waste, for its different components, is a source of toxic metals and various pollutants. Electronic products contain polychlorinated biphenyls, lead, cadmium, and mercury, as well as gold, palladium, copper, bronze, aluminum, and plastics. In addition, there is the possibility that various outdated electronic devices can be reused for research or academic development (Kang and Schoenung, 2005). Electronic waste grows at an annual rate of approximately 2 million tons. Only 17.4% of the world’s electronic waste was recycled, and 82.6% was left untreated or processed informally (Nithya et al., 2021). Every year, millions of electronic products reach the end of their useful life and represent thousands of tons of thermoplastics such as ABS, HIPS, and others (Qu et al., 2006). Electrical and electronic equipment can fall into two categories: reusable equipment that conserves useful parts and recyclable equipment (Kang and Schoenung, 2005). The waste management, construction industries, electrical and electronic equipment are pioneers in implementing the circular economy (Mhatre et al., 2021). The two main types of plastic resins used in electronics are thermosets and thermoplastics. Due to their insulating properties and malleability, polymers have a wide variety of applications in electronic devices. Proper handling and processing of polymers in electronics can be sold for dollars per pound, compared to pennies per pound for PET bottles (Kang and Schoenung, 2005). There are more than ten types of plastics found in electronics. The two plastics most used in the electronics industry are HIPS (56% by weight) and ABS (20% by weight) (Arola et al., 1999; Kang and Schoenung, 2005; Qu et al., 2006; Grigorescu et al., 2019). Polyolefin plastics are recyclable and represent half of all plastics such as HDPE, LDPE, and PP (Kumagai et al., 2020).
4 Technologies for the Classification of Polymers
Various technologies are available to process and classify polymers, including disassembling plastics into electronic products (Williams, 2006). The recycling process has several stages, and one of these stages is called pre-classification and is carried out mainly in collection centers in Mexico. Most of the recycling centers for electronic products and various materials choose the manual classification and manual disassembly. In Mexico, these recycling centers even lack power tools to carry out this activity. The collection centers find it challenging to identify what type of plastic it is, so they apply various tests to determine how to burn the plastic. In Grigorescu et al. (2019), show results and techniques of how to identify plastics by considering the color of the flame, the intensity of the flame, smell, color of the smoke, as well as the type of ash. It is widely known that jobs that are carried out manually translate into high costs. This means that for developing countries like Mexico, it represents low profitability, and as a consequence, the circular economy grows slowly, and an impact is not reflected in the region.
4.1 Plastics Identification Technologies
For the identification of plastics, there are technologies and methods. Manual identification methods are carried out by detecting their codes. Others analyze their physical and chemical properties such as their density, solubility, rigidity, flexibility, thermal analysis, incineration, optical identification with tracers, eddy current separation, spectroscopy, surface free energy, surface roughness, and its chemical structure. To identify the physical properties of polymers, non-destructive techniques can be implemented to analyze surfaces by studying spectra. Separation can be automated using infrared spectroscopy, X-ray fluorescence spectroscopy, and Raman spectroscopy (Allen et al., 1999; Ahmad, 2004; Williams, 2006; Buekens and Zhou, 2014; Ragaert et al., 2017; Shameem et al., 2017; Wang et al., 2019a; Grigorescu et al., 2019; Belyamani et al., 2021; Turner and Filella, 2021). An outstanding feature that should be considered before choosing which spectroscopy to work with is identifying the application and needs because each technique has advantages and limitations. For example, X-ray fluorescence spectroscopy is widely used for the benefits of separating PET from PVC with high precision but not recommended with other plastics (Carroll, 1994; Qu et al., 2006; Sadat-Shojai and Bakhshandeh, 2011). On the other hand, infrared spectroscopy has positioned itself to identify the leading recyclable plastics. Its implementation lies in the identification that occurs in polymers when there is a loss in radiation intensity when passing through plastics and the plastics’ properties that reflect light. Two infrared ranges are mainly used to identify recyclable plastics, the near-infrared NIR and the mid-infrared MIR (Florestan et al., 1994; Arola et al., 1999; Grigorescu et al., 2019; Rani et al., 2019). NIR spectroscopy reliably identifies five types of post-consumer plastics compared to MIR spectroscopy which is sensitive to the surface conditions of the plastic, which implies a limitation in the identification in post-consumer bottles. The vast majority of bottles may have different surface conditions that would prevent MIR spectroscopy from being an excellent option to implement (Florestan et al., 1994). NIR spectroscopy can quickly identify PET, PVC, PE, PS, and PP (Camacho and Karlsson, 2001), but placing dark-colored plastics such as those found in the automotive industry is not recommended (Qu et al., 2006; Šuštar et al., 2014). In contrast, Raman spectroscopy is a technique that reliably identifies plastics based on their vibration signatures. Under the right conditions and using a low-power laser, Raman spectroscopy can identify dark-colored plastics. It can also reliably identify rough-surfaced plastics, plastic powders, and even mineral fillers, as well as naturally colored plastics in less than a second between neighboring plastics (Allen et al., 1999; Florestan et al., 1994; Kumar et al., 2000). Although Raman spectroscopy has multiple advantages, it is necessary to control different factors to be automated in an industrial environment. Various investigations focused on previous technologies that can be developed to identify various forms of plastic parts that require disassembly in household appliances.
4.2 Mechanical Plastic Separation Technologies
For automation in the separation of materials, mechanical techniques can be based on density, solubility, visual, optical classification, air classification, hydro cyclone, flotation method, float-sump separation, foam-flotation separation, flame treatment and flotation, and electrostatic separation as a cost-effective alternative using a Tribo electrostatic process (Pascoe and O’Connell, 2003; Kang and Schoenung, 2005; Williams, 2006; Park et al., 2007; Sadat-Shojai and Bakhshandeh, 2011; Boukhoulda et al., 2013; Wang et al., 2019a; Benabderrahmane et al., 2020). There are processes of free-fall electrostatic separators under other particle charge distributions, which can be coupled to the needs and material with which one works (Wei and Realff, 2003; Benabderrahmane et al., 2020). It is advisable to combine mechanical sorting methods to increase efficiencies, such as air sorting and hydro cyclone, separating the lighter particles from the heavier ones using air and water. The efficiency of the separation depends on the difference in density and the uniformity of the size of the particles, and the same effect occurs with flotation systems in general (Super et al., 1993; Shent et al., 1999; Fraunholcz, 2004; Grigorescu et al., 2019).
5 Infrastructure and Technological Options for Mexico
The development and implementation of technologies focusing on the classification and production of pure post-consumer resins from plastic waste, whether it comes from homes, industry, landfills, and electronics, are of great importance to maintain the circular economy and return materials to the system. The recyclable material available for processing depends on factors that vary by different factors such as the season of the year and the quality, the cost of sorting, and regeneration (Sadat-Shojai and Bakhshandeh, 2011). Manual plastics sorting and electronic housing disassembly tools to separate metals and materials that contaminate plastics are the most widely used techniques due to their low cost and a large amount of labor. The diversity of electronic and plastic products is constantly changing, and infrastructure is required in each country to meet this challenge. Recycling centers and separation centers face this problem every day. Therefore, precise identification technologies are needed to determine the type of plastic in different plastic products.
5.1 Infrastructure in Waste Management
In Mexico, a guide was established that presents the iconography for eight different wastes grouped into two classifications: primary (organic and inorganic) and secondary (paper, plastic, metal, glass, wood, and fabric) (de Medio Ambiente y Recursos Naturales, 2017a). Since 2008, through the SEMARNAT, Mexico developed a program to establish an environmental policy for waste, promoting the prevention and comprehensive management of waste through prevention, separation, reuse, and recycling. Despite these efforts, it is unclear whether the program produced any results for implementing viable waste disposal. In the 32 states of Mexico in 2010, around 1,440 collection centers were reported in which automotive oil, vegetable oil, tires, electrical appliances, multi-layer containers (tetrapak), aluminum wrappers, books, wood, metals, demolition material, paper, cardboard, paraffin, wax, batteries, batteries, accumulators, plastics, electronic waste, toner, particular waste, organic waste, sanitary waste, glass among others. Table 3 shows the number of recycling centers for plastic and electronic waste in the various states of Mexico (de Medio Ambiente y Recursos Naturales, 2010). In Mexico, there is little infrastructure for adequate recycling processes. There are various alternatives that Mexico can follow to minimize the impact of the lack of infrastructure by copying models from other countries and with policies that benefit the population. For example, in (Nagurney and Toyasaki, 2005), an algorithm was proposed to analyze and calculate solutions taking electronic waste factors as processors, recyclers, and consumers to reverse the problems of the supply chain network for the management and recycling of electronic waste that can serve as a reference to be implemented in Mexico (Nagurney and Toyasaki, 2005). There is also research in developing methodologies that facilitate engineering students’ learning to develop skills in a control loop design. Such efforts are necessary to train human capital that provides automation ideas from new methods even focused on plastic waste solutions (Torres-Salinas et al., 2020). Table 4 describes some methods that can be generated at an academic level to contribute to the technological development of Mexico.
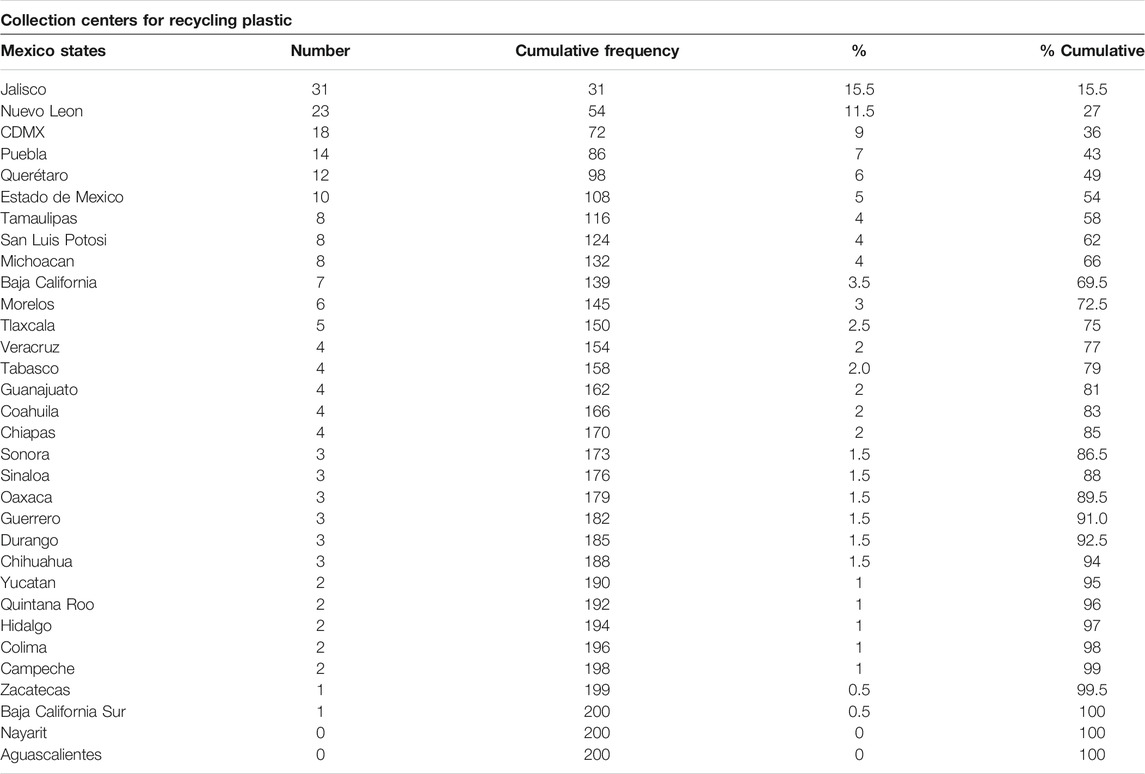
TABLE 3. The infrastructure of the main states of Mexico where People can recycle plastic and electronics.
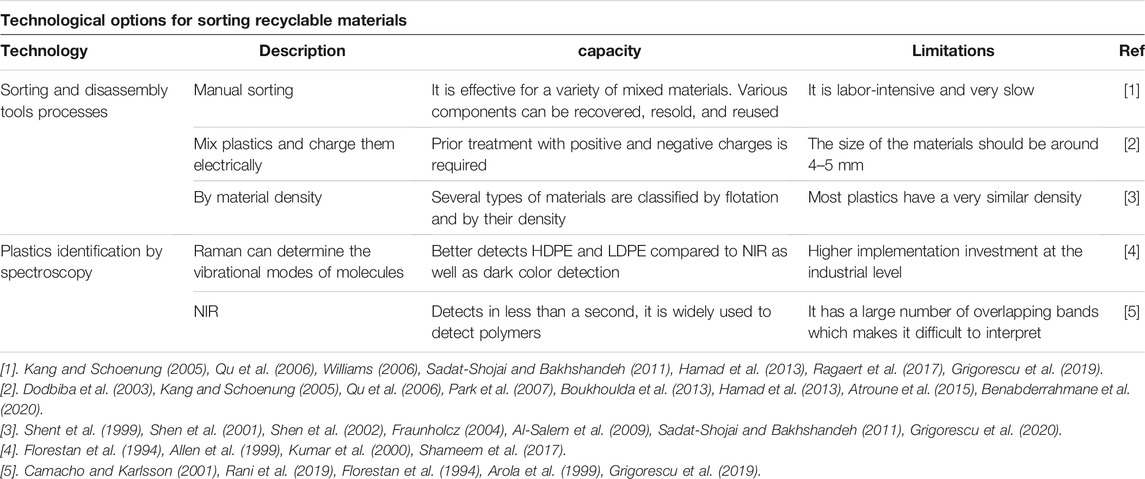
TABLE 4. Summary of the existing methods and technological options in the management of post-consumer plastic waste.
5.2 Technological Options to Develop Post-Consumer PET Bottle Classification Systems in Mexico
On May 17th, 2021, through a press release by the Mexican Coca-Cola Industry, it was reported that they generate more than 2,900 direct jobs and more than 35 thousand indirect ones within the recycling chain. A large number of plastic bottles generated worldwide requires systems that detect the properties of the containers and discard other materials at high speed. There are automated technologies for classifying plastic bottles. These technologies do not apply to most plastics due to the variety of sizes, shapes, coatings, paint of plastics, thickness, and opacity of plastics, making it challenging to use techniques for classification (Kang and Schoenung, 2005). For the classification of plastic bottles, certain technologies crush the bottles to sort two types of material. The system, employing friction, generates that the materials have negative and positive charges later to separate them by an electric field (Hamad et al., 2013). They can also be separated by their density from the plastic, although it has several non-viable implications because the polymers have very similar densities (Al-Salem et al., 2009). Commercially some companies manufacture and distribute classification systems, see Table 5, such as the BottleSort system that detects any material that does not meet a specific size. The method removes contaminants in its first stage, and then a pneumatic system is activated to separate the bottles and place them on a high-speed conveyor belt. The conveyor belt carries the bottles to a 16 infrared detection unit and processes more than 5,000 readings per second. The classification of the resins is done in less than 5 milliseconds. After identification, the bottle is transported to the location where it is placed in a container, and the bottle is expelled by air. This system can identify transparent PET from green PET (Milgrom, 1994; Bruno, 2000). The Poly-Sort system has similar characteristics to the BottleSort system, which refers to the separation of plastic containers that need to be ordered individually. The bottles go through a vibration system that eliminates contaminants with near-infrared sensors. The identification speed is 15 times per second in less than 19 milliseconds and complementing the system with a camera that processes colors so that a light Strobe allows the identification of the color of each bottle. If a material is not identified, it is taken to a container at the end of the belt (Bruno, 2000; Bob Graham of Entec Consulting Ltd., 2006). Another automatic system for the separation of plastic resins is Near-infrared reflectance spectroscopy. This identification method consists of two filters and is capable of distinguishing between PET, HDPE, PVC, PP, and PS (Scott, 1995). Filters make it possible to distinguish between labels and caps, but it is not suitable for identifying dark colors. The biggest problem with the two-filter method is limiting the wavelength region of the light and the acquisition of the data obtained employing two Indium-Gallium-Arsenide photodiodes (Masoumi and Safavi, 2012). The reflection and transmission of X-rays are similar to the infrared spectroscopic method, and depending on the application, it turns out to be considered another classification option. The separation consists of the entrance of plastic and, through waves, the object’s response is studied when working with the use of the X-ray spectrum that consists of transmission and reflection. This method is highly recommended to detect PVC. Similarly, the VS-2 system has a capacity of 200 analyzes per second and classifies two to three bottles per second (Bruno, 2000; Foundation, 2015). Most of the prevailing techniques for classifying plastics are based on the near-infrared spectroscopy method because each plastic has an absorbed wavelength in its spectra (Safavi et al., 2010; House et al., 2011). Classification systems show a trend in methods that use the principle of image processing that uses combinations of various techniques such as principal component analysis, spectroscopy (Rani et al., 2019), and Hough transform (Duda and Hart, 1972; Prasad and Vinu, 2012). Another technique used to classify plastic bottles is by extracting their characteristics in the intensity of the image and support vector machine (SVM), one of the leading classification techniques used in various classification applications (Nawrocky et al., 2010; Sanchez-Reyes et al., 2021). It can also find an approximation of the containers with morphological operations to describe the structure or shape of the image (Shahbudin et al., 2010). As well as implementing a set of classification algorithms based on the statistical learning theory better known as Machine learning, this modality can be understood as algorithms that learn from the data entered by a person (Yi and Guo-Ji, 2005) and are implemented in various applications. Systems developed in intelligent machines that classify bottles in real-time are used for their benefit. For example, a plan was implemented using an FPGA in (House et al., 2011). Other systems combine infrared spectroscopy with neural networks to identify PET, HDPE, LDPE, PVC, PP, and PS (Huth-Fehre et al., 1995; Allen et al., 1999; Shameem et al., 2017). Each of the described methods uses different classification tools themselves that provide advantages and disadvantages; see Table 6. Therefore, they give us a reference to the trend in the implementation of a high-speed classification system. Each of the commercially available classification methods and current methods has different characteristics, designs, and implementation costs. On the other hand, these automated systems have a very high investment grade. The implementation of these automated systems is between 186,000 and 230,000 dollars (Bob Graham of Entec Consulting Ltd., 2006; Wang et al., 2019b). Mexico is a country of culture and with different ways of facing challenges. It differs according to state resources, geographical position, state development in technology, and the availability of each municipality to face plastic waste management. The implementation of technology with an affordable approach in the classification of plastics that can be implemented in collection centers in Mexico should consider combining mechanical recycling and other types of recycling, seeking a balance in the available options.
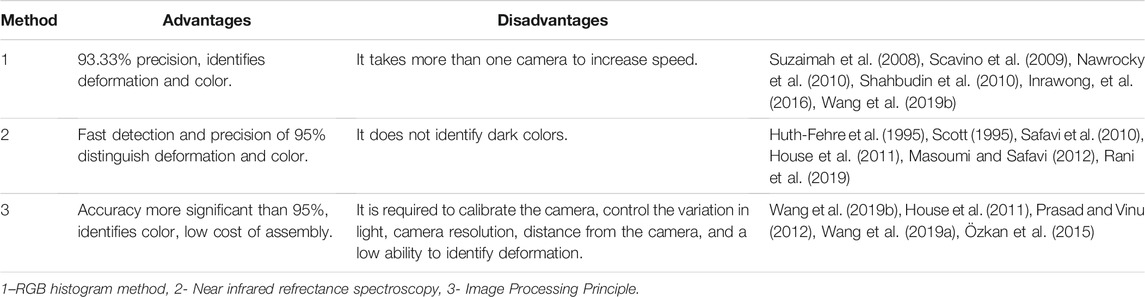
TABLE 6. Comparison of current methods for identifying plastic bottles using various machine learning techniques.
6 Trends to Improve Post-consumer PET Bottle Detection
Recent research proposes new methods such as the principle of image processing, using combinations of various techniques such as principal component analysis, NIR spectroscopy, Rama (Serranti et al., 2011; Shameem et al., 2017; Rani et al., 2019) and Hough transform (Duda and Hart, 1972). One of the limitations of this method is the conditions at the time of taking a photograph, the variation in the light, resolution, background, and the camera’s distance to obtain an accuracy greater than 95% (Prasad and Vinu, 2012). Spectroscopy and PCA are also used with the principle of optical sorting, and they can distinguish plastics through color (Serranti et al., 2011; Wang et al., 2019a). Some of these systems are based on the multiple correlation method for the classification of PET and non-PET bottles, photos are taken, and the correlation of each image of the PET bottle is calculated from histograms of the colors red, green, and blue from each bottle. The test uses 200 images of plastic bottles, 100 images of PET bottles, and 100 images other than PET, with 93.33% identification results (Inrawong et al., 2016). In Suzaimah et al. (2008), it is proposed to convert the images to grayscale. The main characteristics are extracted from the image ending with the linear discriminant analysis method, obtaining a pressure of 97.5%. These methods allow discriminating the bottles that have a different color (Scavino et al., 2009; Wang et al., 2019b). It is necessary to consider environmental factors in (Salazar-Colores et al., 2019) to implement such systems. A restoration algorithm is proposed to reduce the effects of environmental pollution in image processing. Another technique used to classify plastic bottles is by extracting their characteristics in the intensity of the image, and they are detected with a support vector machine. The method is robust to the factors of bottle size, shape, label types, and deformation. Its focus is on rotation, translation, and invariable scaling because it uses features derived from image histograms. The system achieves an accuracy of over 96% using grayscale images from the camera (Nawrocky et al., 2010). An approximation of packaging can also be found with morphological operations to describe the structure or shape of the image, using the two-dimensional description of the silhouette of plastic bottles. The edge of the outlines of objects is detected by the erosion process and the vector feature extraction process using SVM, which is used to classify the image of two groups. As a result, a performance of more than 90% precision is obtained (Shahbudin et al., 2010). A system that classifies bottles in real-time using FPGA was proposed in (House et al., 2011). The method proposes to identify two types of materials. At high speed in real-time, PET and tetra pack bottles automatically locate the regions with the highest probability of containing these materials within the image and removes the background from the image. A histogram is created for the extraction of features from the image and is used to classify the bottles using SVM. They report that the method has 93% recognition and is capable of working with speed. Other systems are based on the use of infrared spectroscopy neural with networks in conjunction for the efficient identification of polymers (Huth-Fehre et al., 1995; Shameem et al., 2017). Each of the described methods uses machine learning in the same way. They provide advantages and disadvantages. See Table 6.
7 Analysis and Conclusion
Mexico faces great challenges in solid waste management and automated classification complicated by the variety of pollutants that can cause misidentification. Different plastics are spliced or bonded. Another limitation is the coatings and designs with geometries that differ significantly from the conventional ones. Mexico needs to develop systems that allow collection and separation centers to be more profitable to increase the recycling rate. We could say that currently there are two ways to classify the different types of plastics: individualized feeding systems that require the objects to be fed to a method of sensors that pass one by one, and mass feeding systems that require the flow of solids to be spread out on a conveyor belt. Both of these systems require that the bottles pass through the measuring system more than once. Each of the investigated classification methods has different capacities and costs that can be modified for collection centers in Mexico. Systems that detect bottle size and have the highest speeds use a more significant number of infrared sensors but do not detect the label, cap, or waste inside the bottle. Systems that consider the type of color of the bottle order them individually, making the system slower and using infrared sensors. Others rely on the frequency of a strobe light to improve plastics detection. When working with X-rays, higher speeds are obtained, but it is only recommended for materials such as PVC. The trend is inclined in the systems that use the Near-Infrared spectroscopy and Raman method to detect various plastics. They can limit the working region of the wavelength. Mexico needs to develop a classification system with an affordable design for collection centers. It is advisable to combine spectroscopy with other methods that work together, such as image processing, to resolve limitations.
Author Contributions
All authors listed have made a substantial, direct, and intellectual contribution to the work and approved it for publication.
Conflict of Interest
The authors declare that the research was conducted in the absence of any commercial or financial relationships that could be construed as a potential conflict of interest.
Publisher’s Note
All claims expressed in this article are solely those of the authors and do not necessarily represent those of their affiliated organizations, or those of the publisher, the editors and the reviewers. Any product that may be evaluated in this article, or claim that may be made by its manufacturer, is not guaranteed or endorsed by the publisher.
Acknowledgments
We thank Consejo Nacional de Ciencia y Tecnología CONACYT and the Universidad Autónoma de Querétaro for the support provided for the development of this paper.
References
Ahmad, S. R. (2004). A New Technology for Automatic Identification and Sorting of Plastics for Recycling. Environ. Technol. 25, 1143–1149. doi:10.1080/09593332508618380
Al-Salem, S. M., Lettieri, P., and Baeyens, J. (2009). Recycling and Recovery Routes of Plastic Solid Waste (Psw): A Review. Waste Management 29, 2625–2643. doi:10.1016/j.wasman.2009.06.004
Allen, V., Kalivas, J. H., and Rodriguez, R. G. (1999). Post-consumer Plastic Identification Using Raman Spectroscopy. Appl. Spectrosc. 53, 672–681. doi:10.1366/0003702991947324
Ardusso, M., Forero-López, A. D., Buzzi, N. S., Spetter, C. V., and Fernández-Severini, M. D. (2021). Covid-19 Pandemic Repercussions on Plastic and Antiviral Polymeric Textile Causing Pollution on Beaches and Coasts of South america. Sci. Total Environ. 763, 144365. doi:10.1016/j.scitotenv.2020.144365
Arola, D. F., Allen, L. E., and Biddle, M. B. (1999). Evaluation of Mechanical Recycling Options for Electronic Equipment. IEEE International Symposium on Electronics and the Environment, May 11-13, 1999, Guangzhou, China, 187–191.
Atroune, S., Tilmatine, A., Alkama, R., Samuila, A., and Dascalescu, L. (2015). Comparative Experimental Study of Triboelectric Charging of Two Size Classes of Granular Plastics. Particulate Sci. Technology 33, 652–658. doi:10.1080/02726351.2015.1019020
Belyamani, I., Maris, J., Bourdon, S., Brossard, J. ., Cauret, L., Fontaine, L., et al. (2021). Toward Recycling ”unsortable” post-consumer Weee Stream: Characterization and Impact of Electron Beam Irradiation on Mechanical Properties. J. Clean. Prod. 294. doi:10.1016/j.jclepro.2021.126300
Benabderrahmane, A., Richard, G., Medles, K., Zeghloul, T., Tilmatine, A., and Dascalescu, L. (2020). Dielectric Barrier Discharge Treatment of Granular Plastic Mixtures in View of Their Triboelectrostatic Separation. IEEE Trans. Ind. Applicat. 56, 693–703. doi:10.1109/tia.2019.2947660
Bob Graham of Entec Consulting Ltd., (2006). A Review of Optical Technology to Sort Plastics Other Containers. Canadian Plastics Industry Association, 1–11.
Boukhoulda, M. F., Miloudi, M., Medles, K., Rezoug, M., Tilmatine, A., and Dascalescu, L. (2013). Experimental Modeling of a New Triboelectrostatic Separation Process for Mixed Granular Polymers. IEEE Trans. Ind. Applicat. 49, 2375–2381. doi:10.1109/tia.2013.2263113
Buekens, A., and Zhou, X. (2014). Recycling Plastics from Automotive Shredder Residues: A Review. J. Mater. Cycles Waste Manag. 16, 398–414. doi:10.1007/s10163-014-0244-z
Camacho, W., and Karlsson, S. (2001). Nir, Dsc, and Ftir as Quantitative Methods for Compositional Analysis of Blends of Polymers Obtained from Recycled Mixed Plastic Waste. Polym. Eng. Sci. 41, 1626–1635. doi:10.1002/pen.10860
Carroll, W. F. (1994). Recycling Postconsumer Pvc Bottles: Technology and Market Development. J. Vinyl Addit. Technol. 16, 169–176. doi:10.1002/vnl.730160314
Chowdhury, T. H. (2021). Technical-economical Analysis of Anaerobic Digestion Process to Produce Clean Energy. Energ. Rep. 7, 247–253. doi:10.1016/j.egyr.2020.12.024
Chu, J., Hu, X., Kong, L., Wang, N., Zhang, S., He, M., et al. (2021). Dynamic Flow and Pollution of Antimony from Polyethylene Terephthalate (Pet) Fibers in china. Sci. Total Environ. 771, 144643. doi:10.1016/j.scitotenv.2020.144643
cuentame.inegi.org.mx, (2020). Medio ambiente - basura; fecha de la consulta 06/05/2020 (INEGI). Available at: http://cuentame.inegi.org.mx/territorio/ambiente/basura.aspx?tema=T
David Azoulay, Y., Villa, P., and Miller, K. (2019). Plastic Health the Hidden Costs of a Plastic Planet. Available at: https://www.ciel.org/wp-content/uploads/2019/02/Plastic-and-Health-The-Hidden-Costs-of-a-Plastic-Planet-February-2019.pdf
de Coca-Cola (Imcc), I. M. (2019). Entérate qué es la industria mexicana de coca-cola y quiénes formamos parte de ella 27/05/2019 (IMCC). México.
de Medio Ambiente, y., and Recursos Naturales, S. (2010). Directorio de centros de acopio de materiales provenientes de residuos en méxico 2010 (SEMARNAT), 1–98.
de Medio Ambiente, y., and Recursos Naturales, S. (2017a). Guía de diseño para la identificación gráfica del manejo integral de los residuos sólidos urbanos. Ciudad de México, México: Gobierno de México, 1–24.
de Medio Ambiente, y., and Recursos Naturales, S. (2021). Aplicación de la enmienda bc1412 del convenio de basilea sobre movimientos transfronterizos de desechos y su eliminación enmiendas de los anexos ii, viii y ix. Ciudad de México, México: Gobierno de México, 1–8.
de Medio Ambiente, y., and Recursos Naturales, S. (2020). Diagnóstico básico para la gestión integral de los residuos 2020.
de Medio Ambiente, y., and Recursos Naturales, S. (2017b). Residuos sólidos urbanos (rsu) información sobre residuos sólidos urbanos. Ciudad de México, México.
Dodbiba, G., Shibayama, A., Miyazaki, T., and Fujita, T. (2003). Triboelectrostatic Separation of Abs, Ps and Pp Plastic Mixture. Mater. Trans. 44, 161–166. doi:10.2320/matertrans.44.161
Duda, R. O., and Hart, P. E. (1972). Use of the Hough Transformation to Detect Lines and Curves in Pictures. Commun. ACM 15, 11–15. doi:10.1145/361237.361242
Fazeli, A., Bakhtvar, F., Jahanshaloo, L., Che Sidik, N. A., and Bayat, A. E. (2016). Malaysia׳s Stand on Municipal Solid Waste Conversion to Energy: A Review. Renew. Sustainable Energ. Rev. 58, 1007–1016. doi:10.1016/j.rser.2015.12.270
Florestan, J., Lachambre, A., Mermilliod, N., Boulou, J. C., and Marfisi, C. (1994). Recycling of Plastics: Automatic Identification of Polymers by Spectroscopic Methods. Resour. Conservation Recycling 10, 67–74. doi:10.1016/0921-3449(94)90039-6
Foti, D. (2016). Innovative Techniques for concrete Reinforcement with Polymers. Construction Building Mater. 112, 202–209. doi:10.1016/j.conbuildmat.2016.02.111
Foti, D., and Lerna, M. (2020). New Mortar Mixes with Chemically Depolymerized Waste Pet Aggregates. Adv. Mater. Sci. Eng. 2020. doi:10.1155/2020/8424936
Fraunholcz, N. (2004). Separation of Waste Plastics by Froth Flotation-Aa Review, Part I. Minerals Eng. 17, 261–268. doi:10.1016/j.mineng.2003.10.028
Grigore, M. E. (2017). Methods of Recycling, Properties and Applications of Recycled Thermoplastic Polymers. Recycling 2. doi:10.3390/recycling2040024
Grigorescu, R. M., Ghioca, P., Iancu, L., David, M. E., Andrei, E. R., Filipescu, M. I., et al. (2020). Development of Thermoplastic Composites Based on Recycled Polypropylene and Waste Printed Circuit Boards. Waste Management 118, 391–401. doi:10.1016/j.wasman.2020.08.050
Grigorescu, R. M., Grigore, M. E., Iancu, L., Ghioca, P., and Ion, R. (2019). Waste Electrical and Electronic Equipment: A Review on the Identification Methods for Polymeric Materials. Recycling 4. doi:10.3390/recycling4030032
Gu, B., Jiang, S., Wang, H., Wang, Z., Jia, R., Yang, J., et al. (2017). Characterization, Quantification and Management of China's Municipal Solid Waste in Spatiotemporal Distributions: A Review. Waste Management 61, 67–77. doi:10.1016/j.wasman.2016.11.039
Guerrero, J. G., Reséndiz, J. R., Reséndiz, H. R., Álvarez Alvarado, J. M., and Abreo, O. R. (2021). Sustainable Glass Recycling Culture-Based on Semi-automatic Glass Bottle Cutter Prototype. Sustainability (Switzerland) 13. doi:10.3390/su13116405
Hamad, K., Kaseem, M., and Deri, F. (2013). Recycling of Waste from Polymer Materials: An Overview of the Recent Works. Polym. Degrad. Stab. 98, 2801–2812. doi:10.1016/j.polymdegradstab.2013.09.025
Hoornweg, D., and Bhada-Tata, P. (2012). What a Waste: A Global Review of Solid Waste Management. Washington, DC.
Horvat, N., and Ng, F. T. (1999). Tertiary Polymer Recycling: Study of Polyethylene Thermolysis as a First Step to Synthetic Diesel Fuel. Fuel 78, 459–470. doi:10.1016/S0016-2361(98)00158-6
House, B. W., Capson, D. W., and Schuurman, D. C. (2011). Towards Real-Time Sorting of Recyclable Goods Using Support Vector Machines. Proceedings of the 2011 IEEE International Symposium on Sustainable Systems and Technology, ISSST 2011. Chicago, IL, USA. 16-18 May 2011. IEEE. doi:10.1109/ISSST.2011.5936845
Hull, M. M., Spitzer, P., and Hopf, M. (2020). Facts about Plastics and the Environment that Every Physics Teacher Should Know. Phys. Teach. 58, 86–88. doi:10.1119/1.5144784
Huth-Fehre, T., Kantimm, T., and Feldhoff, R. (1995). Nir - Remote Sensing and Artificial Neural Networks for Rapid Identification of post Consumer Plastics. J. Mol. Struct. 348, 142–146. doi:10.1016/0022-2860(95)08609-Y
Inrawong, P. S. W., and Kupimai, M. (2016). Plastic Classification Base on Correlation of Rgb Color, 2016 13th International Conference on Electrical Engineering/Electronics, Computer, Telecommunications and Information Technology, August 18-21, 2005, Guangzhou, China. Nakhon Ratchasima, Thailand: ECTI-CON 2016, 1–5.
Jaidev, K., Biswal, M., Mohanty, S., and Nayak, S. K. (2021). Compatibility Effect of R-Abs/r-Hips/r-Ps Blend Recovered from Waste Keyboard Plastics: Evaluation of Mechanical, thermal and Morphological Performance. J. Polym. Res. 28.
Jeswani, H., Krüger, C., Russ, M., Horlacher, M., Antony, F., Hann, S., et al. (2021). Life Cycle Environmental Impacts of Chemical Recycling via Pyrolysis of Mixed Plastic Waste in Comparison with Mechanical Recycling and Energy Recovery. Sci. Total Environ. 769. doi:10.1016/j.scitotenv.2020.144483
Josefa González Blanco Ortíz Mena, S. S. M. (2019). Visión nacional hacia una gestión sustentable (cero residuos). Ciudad de México, México: Gobierno de México, 1–27.
Jung, S., Lee, M., Lee, S., and Ahn, J. W. (2021). A Study on the Trend of Domestic Waste Generation and the Recognition of Recycling Priorities in Korea. Sustainability (Switzerland) 13, 1–12. doi:10.3390/su13041732
Kang, H.-Y., and Schoenung, J. M. (2005). Electronic Waste Recycling: A Review of u.S. Infrastructure and Technology Options. Resour. Conservation Recycling 45, 368–400. doi:10.1016/j.resconrec.2005.06.001
Kreiger, M., Anzalone, G. C., Mulder, M. L., Glover, A., and Pearce, J. M. (2013). Distributed Recycling of post-consumer Plastic Waste in Rural Areas. MRS Proc. 1492, 91–96. doi:10.1557/opl.2013.258
Kumagai, S., Nakatani, J., Saito, Y., Fukushima, Y., and Yoshioka, T. (2020). Latest Trends and Challenges in Feedstock Recycling of Polyolefinic Plastics. J. Jpn. Petrol. Inst. 63, 345–364. doi:10.1627/jpi.63.345
Kumar, S., Grant, E. R., and Duranceau, C. M. (2000). Use of Raman Spectroscopy to Identify Automotive Polymers in Recycling Operations. SAE Tech. Pap. doi:10.4271/2000-01-0739
Langsdorf, A., Volkmar, M., Holtmann, D., and Ulber, R. (2021). Material Utilization of green Waste: a Review on Potential Valorization Methods. Bioresources and Bioprocessing 8. doi:10.1186/s40643-021-00367-5
Liang, Y., Tan, Q., Song, Q., and Li, J. (2021). An Analysis of the Plastic Waste Trade and Management in Asia. Waste Management 119, 242–253. doi:10.1016/j.wasman.2020.09.049
Lin, Y.-H., Tseng, C.-C., Wei, T.-T., and Hsu, C.-T. (2011). Recycling of Dual Hazardous Wastes in a Catalytic Fluidizing Process. Catal. Today 174, 37–45. doi:10.1016/j.cattod.2011.04.029
Lundell, C., and Thomas, J. (2020). “PET: Polyethylene Terephthalate – the Ubiquitous 500 Ml Water Bottle,” in AISC of Advances in Intelligent Systems and Computing, 1202.
Majdinasab, A., and Yuan, Q. (2017). Performance of the Biotic Systems for Reducing Methane Emissions from Landfill Sites: A Review. Ecol. Eng. 104, 116–130. doi:10.1016/j.ecoleng.2017.04.015
Masoumi, H., Safavi, M., and Khani, Z. (2012). Identification and Classification of Plastic Resins Using Near Infrared Reflectance. Int. J. Mech. Ind. Eng. 6, 213–220. doi:10.5281/zenodo.1076916
Mhatre, P., Panchal, R., Singh, A., and Bibyan, S. (2021). A Systematic Literature Review on the Circular Economy Initiatives in the european union. Sustainable Prod. Consumption 26, 187–202. doi:10.1016/j.spc.2020.09.008
Milgrom, J. (1994). Trends in Plastics Recycling. Lancaster, Pennsylvania): Technomic Publishing Company.
Morris, J. (2005). Comparative LCAs for Curbside Recycling versus Either Landfilling or Incineration with Energy Recovery (12 Pp). Int. J. Life Cycle Assess. 10, 273–284. doi:10.1065/lca2004.09.180.10
Nagurney, A., and Toyasaki, F. (2005). Reverse Supply Chain Management and Electronic Waste Recycling: A Multitiered Network Equilibrium Framework for E-Cycling. Transportation Res. E: Logistics Transportation Rev. 41, 1–28. doi:10.1016/j.tre.2003.12.001
Nawrocky, M., Schuurman, D. C., and Fortuna, J. (2010). Visual Sorting of Recyclable Goods Using a Support Vector Machine, Canadian Conference on Electrical and Computer Engineering, Calgary, AB, Canada, 2-5 May 2010, doi:10.1109/CCECE.2010.5575231IEEE
Nithya, R., Sivasankari, C., and Thirunavukkarasu, A. (2021). Electronic Waste Generation, Regulation and Metal Recovery: a Review. Environ. Chem. Lett. 19, 1347–1368. doi:10.1007/s10311-020-01111-9
Özkan, K., Ergin, S., Işik, S., and Işikli, I. (2015). A New Classification Scheme of Plastic Wastes Based upon Recycling Labels. Waste Management 35, 29–35. doi:10.1016/j.wasman.2014.09.030
Park, C., Jeon, H., and Park, J. (2007). Pvc Removal from Mixed Plastics by Triboelectrostatic Separation. J. Hazard. Mater. 144, 470–476. doi:10.1016/j.jhazmat.2006.10.060
Pascoe, R. D., and O’Connell, B. (2003). Development of a Method for Separation of Pvc and Pet Using Flame Treatment and Flotation. Minerals Eng. 16, 1205–1212. doi:10.1016/s0892-6875(03)00171-7
Prasad, K., and Vinu, D. (2012). Image Processing Techniques to Recognize and Classify Bottle Articles. Natl. Conf. Adv. Computer Sci. Appl. Int. J. Computer Appl. 75, 1–5.
Qu, S., Guo, Y., Ma, Z., Chen, W. ., Liu, J., Liu, G., et al. (2019). Implications of china’s Foreign Waste Ban on the Global Circular Economy. Resour. Conservation Recycling 144, 252–255. doi:10.1016/j.resconrec.2019.01.004
Qu, X., Williams, J. S., and Grant, E. R. (2006). Viable Plastics Recycling from End-Of-Life Electronics. IEEE Trans. Electron. packaging manufacturing 29, 25–31. doi:10.1109/tepm.2005.862629
Ragaert, K., Delva, L., and Van Geem, K. (2017). Mechanical and Chemical Recycling of Solid Plastic Waste. Waste Management 69, 24–58. doi:10.1016/j.wasman.2017.07.044
Rani, M., Marchesi, C., Federici, S., Rovelli, G., Alessandri, I., Vassalini, I., et al. (2019). Miniaturized Near-Infrared (Micronir) Spectrometer in Plastic Waste Sorting. Materials 12. doi:10.3390/ma12172740
Ren, H., Qiao, F., Shi, Y., Knutzen, M. W., Wang, Z., Du, H., et al. (2015). PlantbottleTM Packaging Program Is Continuing its Journey to Pursue Bio-Mono-Ethylene Glycol Using Agricultural Waste. J. Renew. Sustainable Energ. 7. doi:10.1063/1.4929336
Sadat-Shojai, M., and Bakhshandeh, G. (2011). Recycling of Pvc Wastes. Polym. Degrad. Stab. 96, 404–415. doi:10.1016/j.polymdegradstab.2010.12.001
Safavi, S., Masoumi, H., Mirian, S., and Tabrizchi, M. (2010). Sorting of Polypropylene Resins by Color in Msw Using Visible Reflectance Spectroscopy. Waste Management 30, 2216–2222. doi:10.1016/j.wasman.2010.06.023
Sahin, O., and Kirim, Y. (2018). Material Recycling of Comprehensive Energy Systems, Vols. 2–5, 1018–1042. doi:10.1016/b978-0-12-809597-3.00260-1
Salazar-Colores, S., Cabal-Yepez, E., Ramos-Arreguin, J. M., Botella, G., Ledesma-Carrillo, L. M., and Ledesma, S. (2019). A Fast Image Dehazing Algorithm Using Morphological Reconstruction. IEEE Trans. Image Process. 28, 2357–2366. doi:10.1109/tip.2018.2885490
Saldaña Durán, C. E., and Nájera González, O. (2019). Identification of Potential Sites for Urban Solid Waste Disposal in the Municipality of Tepic, Nayarit, mexico. Revista Internacional de Contaminacion Ambiental 35, 69–77. doi:10.20937/rica.2019.35.esp02.07
Sanchez-Reyes, L. ., Rodriguez-Resendiz, J., Avecilla-Ramirez, G. N., Garcia-Gomar, M. ., and Robles-Ocampo, J. (2021). Impact of Eeg Parameters Detecting Dementia Diseases: A Systematic Review. IEEE Access 9, 78060–78074. doi:10.1109/access.2021.3083519
Scavino, E., Wahab, D., Hussain, A., Basri, H., and Mustafa, M. (2009). Application of Automated Image Analysis to the Identification and Extraction of Recyclable Plastic Bottles. J. Zhejiang Univ. Sci. A 10, 794–799. doi:10.1631/jzus.A0820788
Scott, D. (1995). A Two-Colour Near-Infrared Sensor for Sorting Recycled Plastic Waste. Meas. Sci. Technology 6, 156–159. doi:10.1088/0957-0233/6/2/004
Serranti, S., Gargiulo, A., and Bonifazi, G. (2011). Characterization of post-consumer Polyolefin Wastes by Hyperspectral Imaging for Quality Control in Recycling Processes. Waste Management 31, 2217–2227. doi:10.1016/j.wasman.2011.06.007
Shahbudin, S., Hussain, A., Wahab, D. A., and Mustafa, M.(2010). Support Vector Machines for Automated Classification of Plastic Bottles. 2010 6th International Colloquium on Signal Processing and Its Applications, May 21-23, 2010, Melaka, 344–348. doi:10.1109/cspa.2010.5545265
Shameem, K. M. M., Choudhari, K. S., Bankapur, A., Kulkarni, S. D., Unnikrishnan, V. K., George, S. D., et al. (2017). A Hybrid Libs–Raman System Combined with Chemometrics: an Efficient Tool for Plastic Identification and Sorting. Anal. Bioanal. Chem. 409, 3299–3308. doi:10.1007/s00216-017-0268-z
Sharma, P., Gaur, V. K., Kim, S., and Pandey, A. (2020). Microbial Strategies for Bio-Transforming Food Waste into Resources. Bioresour. Technol. 299. doi:10.1016/j.biortech.2019.122580
Shen, H., Forssberg, E., and Pugh, R. J. (2002). Selective Flotation Separation of Plastics by Chemical Conditioning with Methyl Cellulose. Resour. Conservation Recycling 35, 229–241. doi:10.1016/s0921-3449(02)00003-4
Shen, H., Forssberg, E., and Pugh, R. J. (2001). Selective Flotation Separation of Plastics by Particle Control. Resour. Conservation Recycling 33, 37–50. doi:10.1016/s0921-3449(01)00056-8
Shen, M., Huang, W., Chen, M., Song, B., Zeng, G., and Zhang, Y. (2020). (micro)plastic Crisis: Un-ignorable Contribution to Global Greenhouse Gas Emissions and Climate Change. J. Clean. Prod. 254. doi:10.1016/j.jclepro.2020.120138
Shent, H., Pugh, R. J., and Forssberg, E. (1999). A Review of Plastics Waste Recycling and the Flotation of Plastics. Resour. Conservation Recycling 25, 85–109. doi:10.1016/s0921-3449(98)00017-2
Super, M. S., Enick, R. M., and Beckman, E. J. (1993). Density-based Separation of Thermoplastics Found in the post-consumer Waste Stream. Resour. Conservation Recycling 9, 75–88. doi:10.1016/0921-3449(93)90034-d
Šuštar, V., Kolar, J., Lusa, L., Learner, T., Schilling, M., Rivenc, R., et al. (2014). Identification of Historical Polymers Using Near-Infrared Spectroscopy. Polym. Degrad. Stab. 107, 341–347.
Suzaimah, R., Marzuki, M. M., Hussan, A., and Abdul Wahab, D. (2008). Histogram of Intensity Feature Extraction for Automatic Plastic Bottle Recycling System Using Machine Vision. Am. J. Environ. Sci., 583–588.
Thakur, S., Verma, A., Sharma, B., Chaudhary, J., Tamulevicius, S., and Thakur, V. K. (2018). Recent Developments in Recycling of Polystyrene Based Plastics. Curr. Opin. Green Sustainable Chem. 13, 32–38. doi:10.1016/j.cogsc.2018.03.011
Torres-Salinas, H., Rodríguez-Reséndiz, J., Estévez-Bén, A. A., Cruz Pérez, M. A., Sevilla-Camacho, P. Y., and Perez-Soto, G. I. (2020). A Hands-On Laboratory for Intelligent Control Courses. Appl. Sci. (Switzerland) 10, 1–21. doi:10.3390/app10249070
Turner, A., and Filella, M. (2021). Lead in Plastics – Recycling of Legacy Material and Appropriateness of Current Regulations. J. Hazard. Mater. 404. doi:10.1016/j.jhazmat.2020.124131
United Nations, D. o. E., and Affairs, S. (2019). World Population Prospects 2019: Highlights. New York.
van Ewijk, S., Park, J. Y., and Chertow, M. R. (2018). Quantifying the System-wide Recovery Potential of Waste in the Global Paper Life Cycle. Resour. Conservation Recycling 134, 48–60. doi:10.1016/j.resconrec.2018.02.026
Villegas Pinuer, F. J., Llonch Andreu, J., Belbeze, P. L., and Valenzuela-Fernández, L. (2021). Waste Management. The Disconnection between Normative and Smes Reality. Sustainability (Switzerland) 13, 1–20. doi:10.3390/su13041787
Wang, H., Wang, C., Fu, J., and Gu, G. (2014). Flotability and Flotation Separation of Polymer Materials Modulated by Wetting Agents. Waste Management 34, 309–315. doi:10.1016/j.wasman.2013.11.007
Wang, H., Zhang, Y., and Wang, C. (2019a). Surface Modification and Selective Flotation of Waste Plastics for Effective Recycling——a review. Separation Purif. Technology 226, 75–94. doi:10.1016/j.seppur.2019.05.052
Wang, Z., Peng, B., Huang, Y., and Sun, G. (2019b). Classification for plastic bottles recycling based on image recognition. Waste Management 88, 170–181. doi:10.1016/j.wasman.2019.03.032
Wei, J., and Realff, M. J. (2003). Design and optimization of free-fall electrostatic separators for plastics recycling. AIChE J. 49, 3138–3149. doi:10.1002/aic.690491214
Wen, Z., Xie, Y., Chen, M., and Dinga, C. D. (2021). China’s plastic import ban increases prospects of environmental impact mitigation of plastic waste trade flow worldwide. Nat. Commun. 12. doi:10.1038/s41467-020-20741-9
Williams, J. A. S. (2006). A review of electronics demanufacturing processes. Resour. Conservation Recycling 47, 195–208. doi:10.1016/j.resconrec.2005.11.003
Yan, Y., Mao, Y., Li, B., and Zhou, P. (2021). Machinability of the thermoplastic polymers: Peek, pi, and pmma. Polymers 13, 1–14.
Keywords: recycled plastic bottles, polyethylene terephthalate, infrastructure in Mexico, culture of recycling, solid waste
Citation: Ángeles-Hurtado L, Rodríguez-Reséndiz J, Salazar-Colores S, Torres-Salinas H and Sevilla-Camacho PY (2021) Viable Disposal of Post-Consumer Polymers in Mexico: A Review. Front. Environ. Sci. 9:749775. doi: 10.3389/fenvs.2021.749775
Received: 30 July 2021; Accepted: 13 September 2021;
Published: 28 September 2021.
Edited by:
Andrew Hursthouse, University of the West of Scotland, United KingdomReviewed by:
Dora Foti, Politecnico di Bari, ItalyRajendra Kumar Foolmaun, Ministry of Environment, Solid Waste Management and Climate Change, Mauritius
Copyright © 2021 Ángeles-Hurtado, Rodríguez-Reséndiz, Salazar-Colores, Torres-Salinas and Sevilla-Camacho. This is an open-access article distributed under the terms of the Creative Commons Attribution License (CC BY). The use, distribution or reproduction in other forums is permitted, provided the original author(s) and the copyright owner(s) are credited and that the original publication in this journal is cited, in accordance with accepted academic practice. No use, distribution or reproduction is permitted which does not comply with these terms.
*Correspondence: Juvenal Rodríguez-Reséndiz, anV2ZW5hbEB1YXEuZWR1Lm14
†These authors have contributed equally to this work