- 1College of Resources and Environment, Northeast Agricultural University, Harbin, China
- 2Key Laboratory of Soybean Molecular Design Breeding, Northeast Institute of Geography and Agroecology, Chinese Academy of Sciences, Harbin, China
Tillage and straw incorporation are important agricultural practices that can break the plow layer and improve Mollisol fertility. The effect of these practices on the limitation of resources for soil microorganisms, however, is unclear. We established a field experiment in 2018 and collection of soil samples in 2020 to study the acquisition of resources by microbes in a Mollisol region in northeastern China. Four treatments were studied: conventional tillage (CT), straw incorporation with conventional tillage (SCT), subsoil tillage (ST) and straw incorporation with subsoil tillage (SST). The limitation of resources for soil microorganisms was assessed using models of extracellular enzymatic stoichiometry. The soil microbes were generally colimited by C and P but not N. The degree of limitation, however, varied among the treatments. SCT and SST alleviated microbial P limitation in the 0–15 and 15–35 cm layers, respectively, but ST did not significantly affect P limitation relative to CT. Interestingly, N-resource contents were strongly correlated with indicators of C and P limitation. A random forest analysis found that the contents of available N and total dissolved N were the most important factors for microbial C and P limitation, respectively. Straw incorporation alleviated microbial P limitation but did not eliminate P limitation and deep tillage aggravate microbial C limitation. We suggest that N fertilization may be reduced due to the N-rich characteristics of the Mollisols in northeastern China.
Introduction
Microbes play a key role in the decomposition of organic matter and the release of nutrients (Daniela et al., 2011; Leininger et al., 2006). Most microbially controlled processes strongly depend on the biomass and activity of microorganisms, which are mostly limited by resources, including carbon (C), nitrogen (N) and phosphorus (P) (Ekblad and Nordgren., 2002; Hill et al., 2014). The amounts of soil C, N and P, however, have been altered in a Mollisol region in China due to straw incorporation, because straw incorporation can promote the sequestration of C in the soil (Ji et al., 2012; Wang et al., 2015), enhance soil respiration and the activity of soil enzymes (Li et al., 2019; Zhao et al., 2016), increase soil microbial biomass and activity and affect the supply of soil nutrients (Zhao et al., 2015) and can help to reduce the inputs of chemical fertilizers (Linquist et al., 2006). Understanding the impacts of straw incorporation on microbial resource limitation will shed some light to improve the sustainability of agricultural development.
Tillage management can influence the depth of straw incorporation (Chen et al., 2017). Tillage affects soil porosity, moisture content, temperature and the activity of microorganisms in various soil layers (Hojatollah et al., 2018). Straw incorporation has been widely used to increase soil fertility, with good results (Mahdi et al., 2005), but most studies have focused on the effect of straw mulching or mixing straw with the topsoil (Kahlon et al., 2013; Tao et al., 2015). These strategies of tillage management can increase the content of soil organic C (SOC) of the surface soil and reduce soil disturbance (Choudhury et al., 2014) but are not conducive to improving the structure of deep soil, enhancing the use efficiency of residues or accumulating SOC (Liu et al., 2021). Some previous studies have found that deep tillage combined with straw incorporation substantially increased soil C sequestration (Tian et al., 2016; Zhao et al., 2015), soil physical properties and the activities of soil enzymes (Zhao et al., 2016). Both tillage and straw management affect the physical and chemical properties of soil and nutrient recycling to promote crop growth, which intensifies the competition for nutrients between plants and microbes. Microbes also have a competitive advantage over plants in the acquisition of nutrients from soil (Hodge et al., 2000). Some microorganisms acquire nutrients by producing extracellular enzymes to degrade complex organic compounds (Jones et al., 2009). Straw incorporation with deep tillage into the field provide a favorable environment for accelerating the secretion of extracellular enzymes and lead to changes in the contents of soil nutrients (Heinze et al., 2010; Kabiri et al., 2016).
Many methodologies have been used to assess the alteration of microbial resource limitation. Investigating the changes of substrate-induced respiration or microbial biomass has traditionally been used to assess microbial resources. This methodology, however, is relatively time-consuming, and added “unnatural” nutrients may become bound to the soil matrix and have side effects (Sullivan et al., 2014). Microbial resource limitation is also partly due to an unbalanced nutrient stoichiometry. The stoichiometry of extracellular enzymes, which represents the ability of microorganisms to absorb nutrients, can be used as an important indicator to assess the flow of energy in an ecosystem (Sullivan et al., 2014). The stoichiometry of extracellular enzymes is based on the assumption that enzymatic activity can indicate the acquisition of organic C, N and P.
We assayed the activities of four extracellular enzymes, β-D-glucosidase (BG), L-leucine aminopeptidase (LAP), β-N-acetylglucosaminidase (NAG) and acid/alkaline phosphatase (AP), which play important roles as indicators of the decomposition of organic matter (Sinsabaugh et al., 2012). BG is a cellulase responsible for hydrolyzing organic C to glucose. NAG and LAP are two important N-cycle enzymes responsible for depolymerizing chitin and protein, respectively. AP is a key enzyme associated with the hydrolyzation of organic P. This approach has been widely used because it is more effective than the traditional method. For example, analyzing the stoichiometry of extracellular enzymes has been used to determine C, N and P limitations in a karst region (Chen et al., 2019.; Li et al., 2019) and in agro-ecosystems in semiarid areas (Ma et al., 2021) in China. Microbial growth is strongly related to microbial metabolic characteristics, which is controlled by microbial resource status, so that adopting effective soil managements to relief microbial resource limitation and thus increase microbial growth is important. Few studies, however, have used ecological enzymatic stoichiometry to identify the metabolic characteristics of microbes in agro-ecosystems in Mollisols in northeastern China.
To elucidate the metabolic characteristics of microbes, Moorhead et al. (2016) proposed to calculate the ratio of enzyme C:N vs. C:P acquisition the “lengths” and “angles” of the vectors in the activity diagram to quantify the to quantify the relative investment of C vs. nutrient acquisition (vector lengths) or P vs. N acquisition (vector angles). These ratios are converted into vector lengths and angles for determining the simultaneous and relative nutrient demands of the community, independent of changes in total enzymatic activities, thereby providing a clear metric corresponding to relative C limitation and P vs. N limitation (Cui et al., 2018; Moorhead et al., 2013, 2016). The threshold element ratio (TER) can also help to quantify the shift in microbial metabolism between energy limitation (C) and nutrient limitation (N and P) (Sinsabaugh et al., 2009). Microbial resource limitation can therefore be assessed using enzymatic stoichiometry, including enzymatic ratios, vector variables and TER (Zheng et al., 2020). Enzymatic stoichiometry provides strong evidence of microbial C, N or P limitation. The effects of tillage and straw management on soil physical properties and the availability of nutrients have been widely studied, but the functional and metabolic responses of microorganisms to these practices remain unknown.
We set up a field experiment in Mollisol soil in northeastern China to analyze the effects of different strategies of tillage and straw management on soil nutrient contents, microbial biomass C, N and P contents and the activities of soil enzymes to determine the status of soil microbial resource limitation. The main objectives were to 1) determine the effects of subsoil tillage and straw management on microbial resource limitation, and 2) identify the relationships between microbial resource limitation and changes in the contents of soil nutrients.
Materials and Methods
Site Description
This field experiment was established in 2018 at the Hailun National Field Station of Agroecosystems of the Chinese Academy of Sciences in Hailun County, Heilongjiang Province, China (47.27°N, 126.41°E; 240 m a.s.l.) (Figure 1). The soil type in this area is a Mollisol. The experimental region has a typical temperate continental seasonal climate, with hot summers and cold winters. The mean annual temperature and precipitation are 1.5°C and 550 mm, respectively. The highest mean monthly temperature of 21°C in July and the lowest of −23°C in January. The precipitation is mainly concentrated from July to September. The frost-free period is 125 days. The study site has no gradient slope and no erosion processes, and corn and soybeans are mainly planted. Corn was the crop at the time of sampling.
Experimental Design and Treatments
The experiment tested four treatments: conventional tillage (CT, tillage depth to 15 cm with no straw incorporation), straw incorporation with conventional tillage (SCT, tillage depth to 15 cm), subsoil tillage (ST, tillage depth to 35 cm) and straw incorporation with subsoil tillage (SST, tillage depth to 35 cm). The experiment had a complete randomized block design with three replicates. All plots were manually plowed. The rate of straw incorporation in SCT and SST was 10,000 kg ha−1, with the straw clipped to sizes >5 cm and evenly mixed into the soil. The rate of straw incorporation was determined by the total straw production for returning the soil. The treatments were continuous croppings of maize in 12 m2 (4 × 3 m) plots. Each plot was fertilized with 180.0 kg N ha−1, 70.0 kg P2O5 ha−1 and 60.0 kg K2O ha−1. The practices of field management were the same as local practices.
Collection of Soil Samples
Soil samples were collected in July 2020 from the 0–15 and 15–35 cm layers for each treatment at three randomly selected points and then mixed into composite samples. These composite samples were packed in sterile bags and transported to the laboratory. Each sample was then divided into two subsamples for analysis. One subsample was air-dried and used for determining the chemical properties. The other subsample was passed through a 2-mm sieve and stored at 4°C for analyzing the activities of soil extracellular enzymes.
Measurements of Soil Properties and Activities of Extracellular Enzymes
The soil particle composition was determined with a laser Particle Size Analyzer (Mastersizer 2000; Malvern Company, United Kingdom) after removing organic matter by digestion in heated hydrogen peroxide solution with sodium hexametaphosphate as dispersant. Based on the international soil particle size fraction system, the percentages of clay (<0.002 mm), silt (0.002–0.02 mm) and sand (0.02–2 mm) in 0–15 cm layer soil were 40.00, 25.84 and 34.16%, respectively, and the percentages in 15–35 cm layer soil were 35.05, 22.87 and 43.09%, respectively. Soil-moisture content was measured by weighing the soil before and after drying at 105°C for 48 h. Soil pH (1:2.5 soil:water) was determined using an FE28-Standard composite electrode (Mettler Toledo). Soil total N (TN) content was determined using the Kjeldahl digestion-distillation method. The contents of SOC, available N (AN), available P (AP), available K (AK), dissolved organic C (DOC) and total dissolved N (TDN) were measured as described by Taylor and Francis (2012) (Carter and Gregorich, 2012). Microbial biomass C (MBC), microbial biomass P (MBP) and microbial biomass N (MBN) were measured using chloroform fumigation-extraction (Vance et al., 1987). Enzymatic activity was measured within 2 weeks after soil sampling. The activities of four enzymes were determined using fluorometric and oxidative enzymatic assays (Ai et al., 2012): one C-acquiring enzyme (β-D-glucosidase, BG), two N-acquiring enzymes (β-N-acetylglucosaminidase, NAG, and L-leucine aminopeptidase, LAP) and one P-acquiring enzyme (acid/alkaline phosphatase, AP). The enzymatic activities are expressed as nmol g−1 dry soil h−1.
Assays of Enzymatic Stoichiometry
We used four methods to analyze microbial resource limitation (Zheng et al., 2020). The first method was based on a scatter plot, which used (NAG + LAP)/AP activities as the X-axis and BG/(NAG + LAP) activities as the Y-axis (Hill et al., 2012). The four quadrants of the scatter plot represented N limitation, P limitation, C and P colimitation and N and P colimitation.
The second method calculated three ratios, BG/(NAG + LAP) activities, BG/AP activities and (NAG + LAP)/AP activities, where higher BG/(NAG + LAP) and BG/AP ratios represent lower N limitation and P limitation, respectively (Waring et al., 2014).
The third method was based on vector analysis (Moorhead et al., 2016). Vector length (L, unitless) and vector angle (A, °) were calculated as:
Increases in vector length indicate that the limitation of microorganism C increases, and vector angles >45° and <45° indicate P and N limitation, respectively. A larger vector angle indicates higher P limitation (Sinsabaugh et al., 2009).
The fourth method used differences between TER for C:N (TERC:N) and the DOC:TDN ratio (RC:N) and between TER for C:P (TERC:P) and the DOC:AP ratio (RC:P). TERC:N and TERC:P were calculated as (Moorhead et al., 2016):
where BC:N and BC:P indicate the ratios of microbial biomass C:N and C:P, respectively, n0 and p0 are intercepts for the type II standard major-axis regressions of ln(BG) vs. ln(NAG + LAP) and ln(BG) vs. ln(AP), respectively. When RC:N or RC:P is larger than TERC:N or TERC:P, N or P are limited for soil microbes, respectively. A higher RC:N-TERC:N or RC:P-TERC:P indicates greater N or P limitation, respectively (Zheng et al., 2020).
Statistical Analysis
Correlations between the soil properties and microbial resource limitations were determined using generalized linear models. Structural equation modeling (SEM) was performed using Amos v.24 (IBM, Armonk, United States) to identify the possible ways in which variables could influence microbial resource restriction. The importance of the influencing factors for microbial metabolic limitation were identified using a random forest (RF) analysis for classification by Rv. 4.0.4 (R Development Core Team, 2021) with the random forest package (Trivedi et al., 2016). Percentage increases in the mean squared error (MSE) of variables were used to estimate the importance of these indices: a high MSE% implied the importance of the variables (Breiman, 2001). Other statistical analyses were performed using IBM SPSS Statistical software v. 22 (SPSS Inc., Chicago, United States).
Results
Soil Properties
Most soil properties were affected by tillage depth and straw incorporation (Table 1). SOC, AN, AP, AK, MBN and MBP contents were significantly higher in the 0–15 than the 15–35 cm layer in CT and SCT but did not differ significantly in ST and SST. SOC content was significantly higher by 11.8% in SCT than CT in the 0–15 cm layer. AN and AK contents were significantly higher by 15.7 and 11.0% in SST than CT, respectively, in the 15–35 layer but did not differ significantly in the other treatments in the 0–15 cm layer. Soil pH and AP content did not differ significantly among CT, SCT, ST and SST in either layer. DOC (215.3 mg kg−1) and TDN (20.12 mg kg−1) contents were highest in SST in the 0–15 cm layer. MBC content was significantly higher by 81.5% in SST than ST and by 33.9% in SCT than CT in the 0–15 cm layer. MBN and MBP contents were significantly higher in SST than the other treatments in the 0–15 cm layer.
Activity of Soil Enzymes
The activities and stoichiometries of the extracellular enzymes varied among the treatments (Figure 2). The activities of the C- and P-acquiring enzymes were significantly higher in the 0–15 than the 15–35 cm layer in CT and SCT. BG activity was significantly higher by 46.2, 38.1 and 87.6% in SCT, ST and SST, respectively, than CT in the 0–15 cm layer and was significantly higher in ST and SST than CT in the 15–35 cm layer. NAG and LAP activities were significantly higher in SST and SCT than CT in the 0–15 cm layer and significantly higher in SST than CT, SCT and ST in the 15–35 cm layer. AP activity was significantly higher in SCT, ST and SST than CT in the 0–15 cm layer. AP activity was highest in SST (299.8 nmol g−1 h−1) in the 0–15 cm layer.
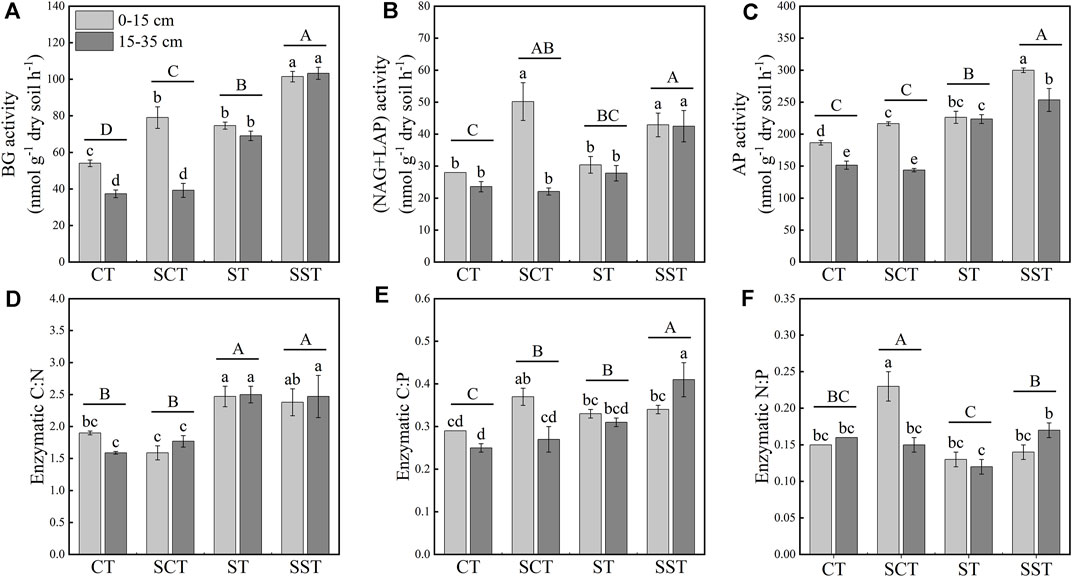
FIGURE 2. Responses of the activities of soil extracellular enzymes to tillage and straw management in the 0–15 and 15–35 cm layers (A–C). Responses of enzymatic activity ratios to tillage and straw management in the 0–15 and 15–35 cm layers (D–F). Different capital letters represent significant differences among four different treatments. Different lowercase letters represent significant differences among four different treatments in different soil layers. See Materials and methods for abbreviation definitions.
The enzymatic C:N activity ratio was significantly higher in SST and ST than SCT and CT. The enzymatic C:P activity ratio was significantly higher in SCT than CT in the 0–15 cm layer and significantly higher in SST than CT in the 15–35 cm layer. The enzymatic N:P activity ratio was significantly highest in SCT in the 0–15 cm layer.
Enzymatic Stoichiometry
The scatter plot of the stoichiometry of the extracellular enzymes indicated that all data and all plots were C- and P-limited (Figure 3). The microbial C and P limitations were quantified by calculating the vector lengths and angles. The vectors were significantly longer for SCT and SST than CT in the 15–35 cm layer and were significantly longer for SST than SCT in the 0–15 cm layer. The vector angles were >45° in all treatments and were lowest in SST in the 15–35 cm layer. Tillage depth and straw incorporation had no significant interactive effect on RC:N-TERC:N for any of the treatments. RC:P-TERC:P was significantly larger than zero in all treatments and was significantly highest in SST in the 0–15 cm layer.
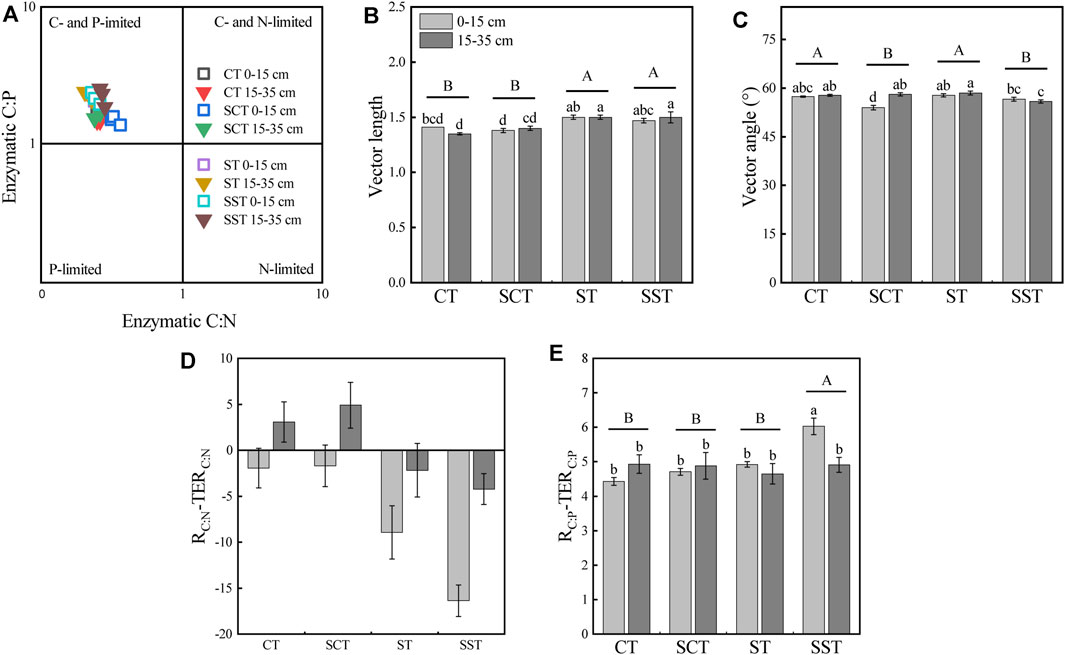
FIGURE 3. Scatter plot of soil enzymatic stoichiometry showing the general pattern of microbial resource limitation (A). Variation of vector length (B), vector angle (C), RC:N-TERC:N (D) and RC:P-TERC:P (E) among the treatments. Different capital letters represent significant differences among four different treatments. Different lowercase letters represent significant differences among four different treatments in different soil layers. See Materials and methods for abbreviation definitions.
Relationships Between Soil Properties and Vector Characteristics of the Enzymatic Activities
Soil properties were strongly correlated with enzymatic activities (Table 2). BG activity was significantly correlated with TN, AK, DOC, TDN, MBC, MBN and MBP contents. NAG + LAP activity was significantly correlated with all soil properties except pH. AP activity was significantly correlated with TN, AK, DOC, TDN, MBC, MBN and MBP contents. Linear regression analysis indicated that microbial C limitation increased with MBN content (p < 0.05; Figure 4) and that microbial P limitation decreased as SOC, TN, AN, AK and MBP contents increased (p < 0.001; Figure 5).
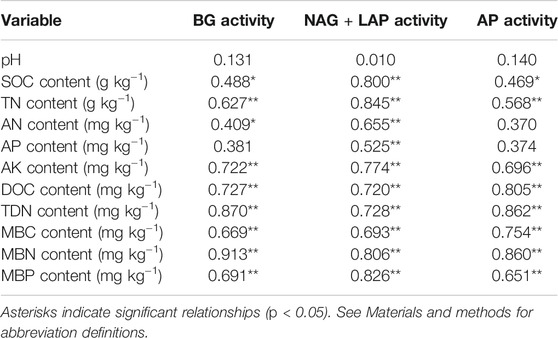
TABLE 2. Correlation coefficients for the relationships between the activities of soil enzymes (nmol g−1 soil h−1) and soil properties.
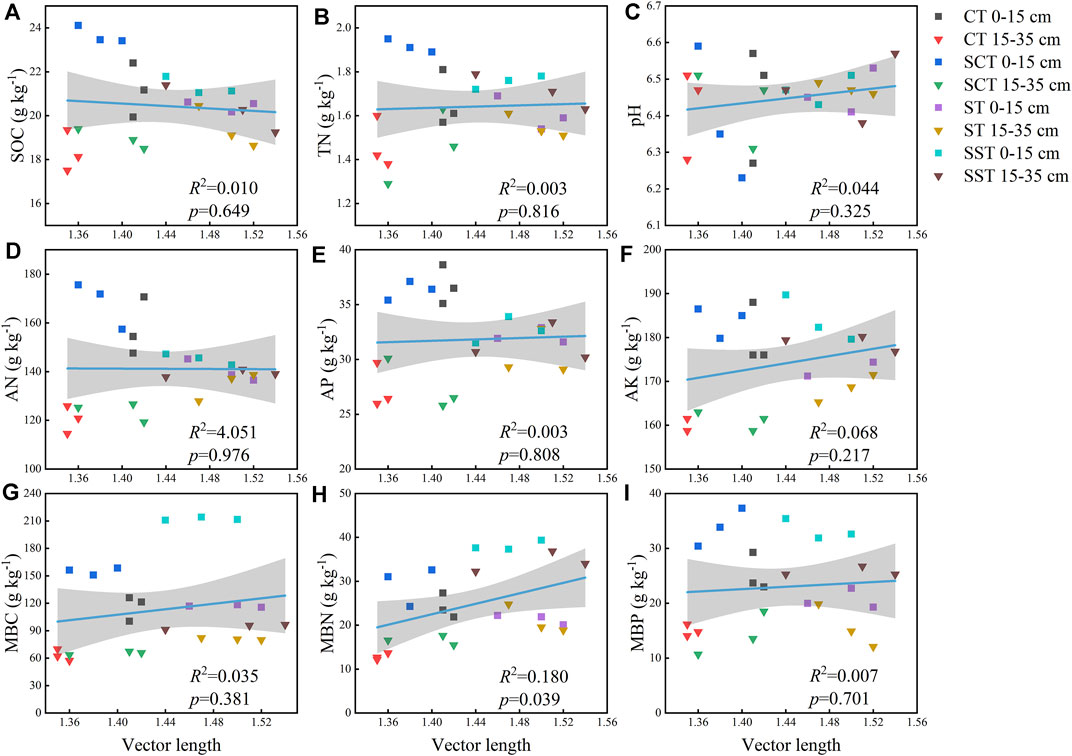
FIGURE 4. Vector lengths for the soil properties (A–I). Solid lines indicate that the model fit the vector lengths and the properties well, and the gray areas are the 95% confidence intervals of the models. See Materials and methods for abbreviation definitions.
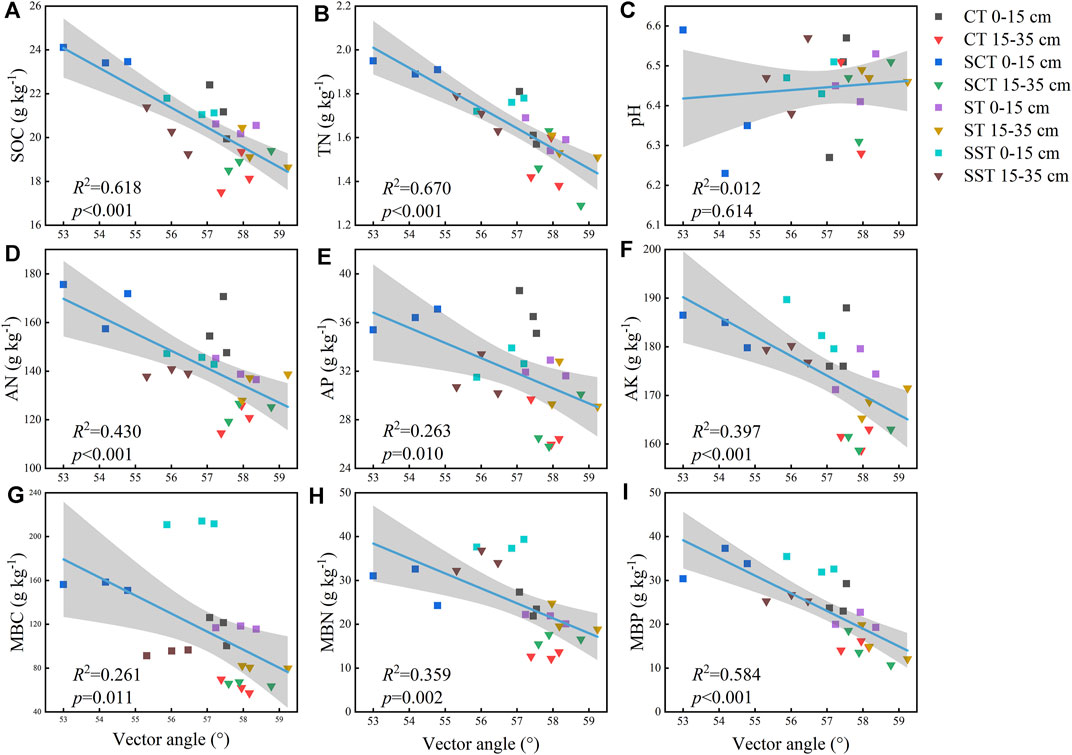
FIGURE 5. Vector angles for the soil properties (A–I). Solid lines indicate that the model fit the vector angles and properties well, and the gray areas are the 95% confidence intervals of the models. See Materials and methods for abbreviation definitions.
SEM was used to analyze the relationships between the chemical properties and the microbial nutrient limitations of the soil (length, χ2 = 2.93, p = 0.82; angle, Chi-square = 1.20, p = 0.75) (Figure 6). Vector lengths were correlated positively with AN content and the enzymatic C:N and C:P activity ratios and negatively with DOC content. The enzymatic C:N and N:P activity ratios were primarily correlated with AN content. The enzymatic C:N activity ratio was correlated positively with the enzymatic C:P activity ratio and negatively with the enzymatic N:P activity ratio. Vector angles were primarily negatively correlated with TDN content and the enzymatic C:P and N:P activity ratios. The enzymatic C:P activity ratio was primarily correlated with TDN content, and the enzymatic N:P activity ratio was primarily correlated with TDN and SOC contents. The enzymatic N:P activity ratio was correlated positively with the enzymatic C:P activity ratio and negatively with the enzymatic C:N activity ratio. The enzymatic C:P activity ratio was positively correlated with the enzymatic C:N activity ratio. The random forest analysis demonstrated that the importance of each factor was determined by vector length and angle. We estimated the importance of the indices using percent increases in the mean squared error of the variables, where higher values denoted more important variables. The most important factors for vector length were AN content followed by AP content, and the most important factor for the vector angle was TDN content.
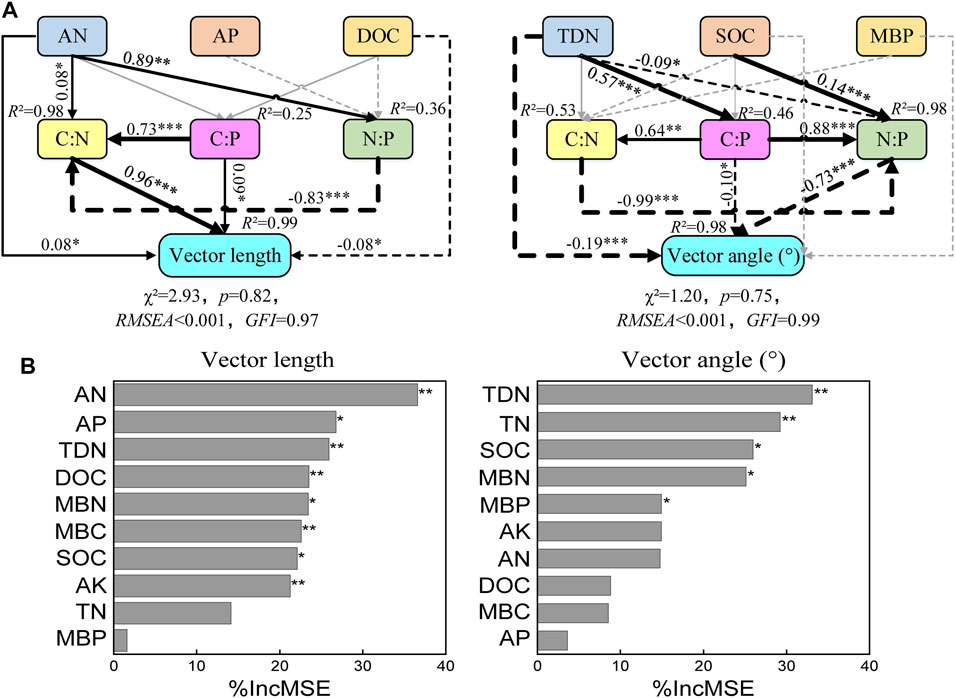
FIGURE 6. Relationships of microbial resource limitation with soil properties. (A) Structural equation modeling was used to identify the possible pathways by which attributes controlled microbial C limitation (represented by vector length) and microbial P limitation (represented by vector angle). Solid and dashed arrows indicate positive and negative relationships (p < 0.05), respectively. The numbers on the arrows indicate significant standardized path coefficients. R2 indicates the variance of the dependent variable explained by the model. (B) Importance analysis of factors affecting microbial metabolic limitation in soil (random forest analysis). **, p < 0.01; *, p < 0.05. See Materials and methods for abbreviation definitions.
Discussion
Effects of Tillage and Straw Management on the Activities of the Soil Enzymes
Tillage and straw management strongly influenced the activities of the C-, N- and P-acquiring enzymes (Figure 2). The activity of the C-acquiring enzyme was significantly higher in SCT than CT in the 0–15 cm layer, indicating that the decomposition of the straw incorporated into the surface soil could supply sufficient C for the growth of soil microorganisms (Li et al., 2021). The activity of the C-acquiring enzyme was also higher in ST and SST than CT, perhaps due to the input of root C, because deep tillage breaks plow pan layers and provides favorable soil ventilation that promotes root development (Mu et al., 2016). Deep tillage proved to be a measure to enhance soil C and N, by translocating large amounts of not easily decomposable soil C and N the greater depth soil. Deep tillage also transfers the carbon resources from the surface soil to the deeper layers, which leads to the homogenization of the carbon resources throughout the entire tilled layer (Urioste et al., 2006). In support of this, we did find a significantly positive negative relationship between DOC with BG activity in the current study (Table 2) and the DOC contents were significantly higher not only in the 0–15 cm soil layer but also in the 15–35 cm soil layer of the SST compared with ST treatment, it can be assumed that downward transport DOC is relatively high.
The activities of both N-acquiring enzymes were higher in SCT than CT, perhaps due to the input of organic C with the application of straw, which improved microbial demand for available N to maintain a stoichiometric balance. The activities of N-acquiring enzymes were higher in SST than CT in the 15–35 cm layer, perhaps due to the deep tillage involves incorporation of straw into the subsoil, and can significantly improve nutrient supply and soil physical properties (Xu et al., 2019). Meanwhile, the activity of the P-acquiring enzyme was also higher in SCT than CT, because straw incorporation increased the availability of C and N (Table 1), which improved microbial demand in activity P to maintain a stoichiometric balance.
Straw incorporation facilitates the mineralization of soil organic P, so microorganisms release more P-acquiring enzymes to compete with plants for the available P (Waqas et al., 2020). Some previous studies have also reported that the activities of these enzymes were statistically correlated with pH (Sinsabaugh et al., 2008; Zheng et al., 2020), but pH and the activity of the P-acquiring enzyme in our study were not significantly correlated. Our short-term study of tillage and straw management may not have been long enough to identify a large impact on soil pH, which did not differ between CT and the other treatments (Table 1). A change in the activity of only one enzyme, however, cannot fully represent microbial resource limitation, because resource limitation is not determined by a single nutrient but by the relative availabilities of multiple nutrients (Zhou et al., 2013). Studying microbial resource limitation thus also needs an analysis of enzymatic stoichiometry.
Effects of Tillage and Straw Management on Microbial C, N and P Limitation
Our results suggest that the growth of soil microorganisms tended to be co-limited by C and P but not N. This finding is supported by three lines of evidence. Firstly, the scatter plot of the stoichiometry of the extracellular enzymes provided intuitive evidence that all soil microbes were limited by C and P (Figure 3). Secondly, the vector angles were >45° and RC:P-TERC:P was larger than zero for all treatments and RC:N-TERC:N was larger than zero in some treatments. These results all suggest that the soil microbes were limited by P rather than N for all treatments. Thirdly, the enzymatic C:P and N:P activity ratios were 0.29 and 0.15 in CT in the 0–15 cm layer, much lower than the means for terrestrial ecosystems (0.62 and 0.44, respectively) (Sinsabaugh et al., 2009), and the enzymatic C:N activity ratio (1.91) was higher than the mean ratio for terrestrial ecosystems (1.41). These results also indicated that the soil microbes tended to be limited by P.
The variation of the enzymatic C:P activity ratio among the treatments, however, indicated that the range of microbial P limitation varied (Figure 2). The ratio was significantly higher in SCT than CT in the 0–15 cm layer, indicating that straw incorporation could substantially reduce the limitation of microbial P. The alleviation of P limitation was likely due to the effect of straw incorporation on the availability of soil P. Vector angles indicate whether soil microbes are limited by the availability of P with a greater value, implying more P limitation (Moorhead et al., 2013). Based on the vector angle and the enzymatic C:P activity ratio in our study, the incorporation of straw may have increased the amount of available C, thereby increasing the enzymatic C:P activity ratio and reducing microbial P limitation (Figures 2, 3). Based on this, the incorporation of straw provides additional substrate and a favorable environment for the production of soil enzymes, which leads to dynamic changes in soil nutrients and is conducive to the sustainable production of crops. Based on the vector angle, RC:P-TERC:P and the enzymatic C:P activity ratio, however, deep tillage did not significantly affect microbial P limitation; the vector angle, RC:P-TERC:P and the enzymatic C:P activity ratio did not differ significantly between ST and CT in the 0–15 cm layer, perhaps because ST lacked C inputs relative to SCT (Tian et al., 2020). In support, ST did not significantly affect SOC relative to CT in the 0–15 cm layer (Table 1).
Vector length is a useful index of whether soil microbes are limited by the availability of C, with a longer vector implying more C limitation (Moorhead et al., 2013). The indicators of microbial C limitation (vector length and the enzymatic C:N activity ratio) were higher in ST and SST but did not differ significantly between SCT and CT (Figures 2, 3), indicating that deep tillage could aggravate microbial C limitation. Deep tillage transfers surface soil with high SOC content to deeper layers, leading to the homogenization of SOC throughout the entire tilled layer, thus contributing to the increase in SOC content in the subsoil layer (Urioste et al., 2006).
The variations in these P-limitation indicators were associated with changes in TDN and SOC contents and the enzymatic C:P and N:P activity ratios (Figures 5, 6), consistent with the results of the random forest analysis (TDN content was the most important factor of microbial P limitation). Microbial C limitation was also mainly affected by AN, AP and MBN contents (Figures 4, 6). We inferred that the soil in this region was N-saturated, and N saturation leads to microbial C and P limitation. This conclusion was supported by the following evidence. The straw-incorporation treatments had higher levels of organic matter, and the addition of a large amount of organic matter would theoretically lead to the lack of N in crops, which would lead to competition between microorganisms and crops for N (Bowles et al., 2014). The difference in our study was that microbial resource limitation was not converted to N limitation, although straw incorporation provided a large source of C. This difference also implied that the microorganisms in the Mollisol in our study area were N-saturated. A previous study reported that N saturation causes microbial C limitation and that N addition could aggravate microbial C limitation (Jiří et al., 2013). N addition has aggravated microbial C limitation in some N-addition experiments (Treseder et al., 2008; Chen et al., 2019; Li et al., 2019).
Tillage and straw management did not weaken the status of N saturation and even exacerbated it; RC:N-TERC:N was less than zero and RC:N-TERC:N was higher in SST than CT in the 0–15 cm layer. The enzymatic C:N activity ratio also suggested that deep tillage increased microbial N saturation because the enzymatic C:N activity ratio was higher in ST than CT. These results indicated that the region was saturated with N resources. When the application of fertilizers caused new N inputs exceeding the holding capacity of living and non-living organisms, has serious implications for the structure and function of terrestrial ecosystems. It is suggested to reduce the application of conventional N fertilizer and improve the utilization efficiency of N fertilizer.
Conclusion
We investigated the changes in microbial resource limitation by tillage and straw management using enzymatic stoichiometry in a Mollisol in northeastern China. The results indicated that the growth of microorganisms was mainly colimited by soil C and P and not N. The incorporation of straw alleviated the P limitation of the microorganisms, likely due to the increased level of available C. Deep tillage tended to aggravate microbial C limitation. The soil in this region is saturated with N resources, so the status of microbial N saturation was not affected, regardless of management strategy, which also influenced microbial C and P limitation. The application of N fertilizer can thus be appropriately reduced in the management of conventional farmland in Mollisols in this region in China. This study provides critical information on the effects of tillage and straw management on microbial nutrient acquisition and elsewhere in the world with similar climates and soil types.
Data Availability Statement
The original contributions presented in the study are included in the article/Supplementary Materials, further inquiries can be directed to the corresponding authors.
Author Contributions
XH and WZ conceived and designed the experiments. NZ and XC performed the experiments and analyzed the data and XL, JY, and LY revised the manuscript.
Funding
The study was funded by the Strategic Priority Research Program of the Chinese Academy of Sciences (Grant No. XDA28070100), National Natural Science Foundation of China (41807085), Applied Technology Research and Development Program of Heilongjiang (GA19B101, GA19B105) and Chinese Agriculture Research System (CARS04).
Conflict of Interest
The authors declare that the research was conducted in the absence of any commercial or financial relationships that could be construed as a potential conflict of interest.
Publisher’s Note
All claims expressed in this article are solely those of the authors and do not necessarily represent those of their affiliated organizations, or those of the publisher, the editors and the reviewers. Any product that may be evaluated in this article, or claim that may be made by its manufacturer, is not guaranteed or endorsed by the publisher.
Abbreviations
AN, Available N; AP, Available P; AK, Available K; AP, Acid/alkaline phosphatase; BG, β-D-glucosidase; CT, Conventional tillage; DOC, Dissolved organic C; LAP, L-leucine aminopeptidase; MBC, Microbial biomass C; MBP, microbial biomass P; MBN, microbial biomass N; NAG, β-N-acetylglucosaminidase; SCT, Straw incorporation with conventional tillage; SOC, Soil organic C; ST, Subsoil tillage; SST, Straw incorporation with subsoil tillage; TER, Threshold element ratio; TN, Soil total N; TDN, Total dissolved N.
References
Ai, C., Liang, G., Sun, J., Wang, X., and Zhou, W. (2012). Responses of Extracellular Enzyme Activities and Microbial Community in Both the Rhizosphere and Bulk Soil to Long-Term Fertilization Practices in a Fluvo-Aquic Soil. Geoderma 173-174, 330–338. doi:10.1016/j.geoderma.2011.07.020
Al-Kaisi, M. M., Yin, X., and Yin, X. (2005). Tillage and Crop Residue Effects on Soil Carbon and Carbon Dioxide Emission in Corn-Soybean Rotations. J. Environ. Qual. 34, 437–445. doi:10.2134/jeq2005.0437
Bowles, T. M., Acosta-Martínez, V., Calderón, F., and Jackson, L. E. (2014). Soil Enzyme Activities, Microbial Communities, and Carbon and Nitrogen Availability in Organic Agroecosystems across an Intensively-Managed Agricultural Landscape. Soil Biol. Biochem. 68, 252–262. doi:10.1016/j.soilbio.2013.10.004
Carter, M. R., and Gregorich, E. G. (2012). Soil Sampling and Methods of Analysis. 2nd edition. Oxfordshire UK: Taylor & Francis.
Chen, H., Li, D., Mao, Q., Xiao, K., and Wang, K. (2019). Resource Limitation of Soil Microbes in Karst Ecosystems. Sci. Total Environ. 650, 241–248. doi:10.1016/j.scitotenv.2018.09.036
Chen, J., Zheng, M.-j., Pang, D.-w., Yin, Y.-p., Han, M.-m., Li, Y.-x., et al. (2017). Straw Return and Appropriate Tillage Method Improve Grain Yield and Nitrogen Efficiency of winter Wheat. J. Integr. Agric. 16, 1708–1719. doi:10.1016/s2095-3119(16)61589-7
Choudhury, S. G., Srivastava, S., Singh, R., Chaudhari, S. K., Sharma, D. K., Singh, S. K., et al. (2014). Tillage and Residue Management Effects on Soil Aggregation, Organic Carbon Dynamics and Yield Attribute in rice–wheat Cropping System under Reclaimed Sodic Soil. Soil Till. Res. 136, 76-83. doi:10.1016/j.still.2013.10.001
Cui, Y., Fang, L., Deng, L., Guo, X., Han, F., Ju, W., et al. (2018). Patterns of Soil Microbial Nutrient Limitations and Their Roles in the Variation of Soil Organic Carbon across a Precipitation Gradient in an Arid and Semi-arid Region. Sci. Total Environ. 658, 1440–1451. doi:10.1016/j.scitotenv.2018.12.289
Daniela, F. C., Whendee, L. S., Margaret, S. T., Sarah, D. B., and Mary, K. F. (2011). Changes in Microbial Community Characteristics and Soil Organic Matter with Nitrogen Additions in Two Tropical Forests. Ecology 92, 621–632. doi:10.1007/s13595-014-0444-7
Ekblad, A., and Nordgren, A. (2002). Is Growth of Soil Microorganisms in Boreal Forests Limited by Carbon or Nitrogen Availability? Plant Soil 242, 115–122. doi:10.1023/A:1019698108838
Heinze, S., Rauber, R., and Joergensen, R. G. (2010). Influence of Mouldboard Plough and Rotary Harrow Tillage on Microbial Biomass and Nutrient Stocks in Two Long-Term Experiments on Loess Derived Luvisols. Appl. Soil Ecol. 46, 405-412. doi:10.1016/j.apsoil.2010.09.011
Hill, B. H., Elonen, C. M., Jicha, T. M., Kolka, R. K., Lehto, L. L. P., Sebestyen, S. D., et al. (2014). Ecoenzymatic Stoichiometry and Microbial Processing of Organic Matter in Northern Bogs and Fens Reveals a Common P-Limitation between Peatland Types. Biogeochemistry 120, 203–224. doi:10.1007/s10533-014-9991-0
Hill, B. H., Elonen, C. M., Seifert, L. R., May, A. A., and Tarquinio, E. (2012). Microbial Enzyme Stoichiometry and Nutrient Limitation in Us Streams and Rivers. Ecol. Indicators 18, 540–551. doi:10.1016/j.ecolind.2012.01.007
Hodge, A., Robinson, D., and Fitter, A. (2000). Are Microorganisms More Effective Than Plants at Competing for Nitrogen? Trends Plant Sci. 5, 304–308. doi:10.1016/S1360-1385(00)01656-3
Hojatollah, L., Deng, A., Mohsin, N. M., Liang, L., Chen, Z., Zheng, Y., et al. (2018). Integrative Impacts of Rotational Tillage on Wheat Yield and Dry Matter Accumulation under Corn-Wheat Cropping System. Soil Till. Res. 184, 100–108. doi:10.1016/j.still.2018.07.008
Ji, X. H., Wu, J. M., Peng, H., Shi, L. H., Zhang, Z. H., Liu, Z. B., et al. (2012). The Effect of rice Straw Incorporation into Paddy Soil on Carbon Sequestration and Emissions in the Double Cropping rice System. J. Sci. Food Agr. 92, 1038–1045. doi:10.1002/jsfa.5550
Jones, D. L., Kielland, K., Sinclair, F. L., Dahlgren, R. A., Newsham, K. K., Farrar, J. F., et al. (2009). Soil Organic Nitrogen Mineralization across a Global Latitudinal Gradient, 23. New Jersey, US: John Wiley & Sons, a–n. doi:10.1029/2008GB003250
Kabiri, V., Raiesi, F., and Ghazavi, M. A. (2016). Tillage Effects on Soil Microbial Biomass, Som Mineralization and Enzyme Activity in a Semi-arid Calcixerepts. Agric. Ecosyst. Environ. 232, 73–84. doi:10.1016/j.agee.2016.07.022
Kahlon, M. S., Lal, R., and Ann-Varughese, M. (2013). Twenty Two Years of Tillage and Mulching Impacts on Soil Physical Characteristics and Carbon Sequestration in central ohio. Soil Tillage Res. 126, 151–158. doi:10.1016/j.still.2012.08.001
Kopáček, J., Cosby, B. J., Evans, C. D., Hruška, J., Moldan, F., Oulehle, F., et al. (2013). Nitrogen, Organic Carbon and sulphur Cycling in Terrestrial Ecosystems: Linking Nitrogen Saturation to Carbon Limitation of Soil Microbial Processes. Biogeochemistry 115, 33–51. doi:10.1007/s10533-013-9892-7
Leininger, S., Urich, T., Schloter, M., Schwark, L., Qi, J., Nicol, G. W., et al. (2006). Archaea Predominate Among Ammonia-Oxidizing Prokaryotes in Soils. Nature 442, 806–809. doi:10.1038/nature04983
Li, H., Zhang, Y., Yang, S., Wang, Z., Feng, X., Liu, H., et al. (2019). Variations in Soil Bacterial Taxonomic Profiles and Putative Functions in Response to Straw Incorporation Combined with N Fertilization during the maize Growing Season. Agric. Ecosyst. Environ. 283, 106578. doi:10.1016/j.agee.2019.106578
Li, Y.-m., Duan, Y., Wang, G.-l., Wang, A.-q., Shao, G.-z., Meng, X.-h., et al. (2021). Straw Alters the Soil Organic Carbon Composition and Microbial Community under Different Tillage Practices in a Meadow Soil in Northeast china. Soil Tillage Res. 208, 104879. doi:10.1016/j.still.2020.104879
Liu, N., Li, Y., Cong, P., Wang, J., Guo, W., Pang, H., et al. (2021). Depth of Straw Incorporation Significantly Alters Crop Yield, Soil Organic Carbon and Total Nitrogen in the North China Plain. Soil Tillage Res. 205, 104772. doi:10.1016/j.still.2020.104772
Ma, Z., Zhang, X., Zheng, B., Yue, S., Zhang, X., Zhai, B., et al. (2021). Effects of Plastic and Straw Mulching on Soil Microbial P Limitations in maize fields: Dependency on Soil Organic Carbon Demonstrated by Ecoenzymatic Stoichiometry. Geoderma 388, 114928. doi:10.1016/j.geoderma.2021.114928
Moorhead, D. L., Rinkes, Z. L., Sinsabaugh, R. L., and Weintraub, M. N. (2013). Dynamic Relationships between Microbial Biomass, Respiration, Inorganic Nutrients and Enzyme Activities: Informing Enzyme-Based Decomposition Models. Front. Microbiol. 4, 223. doi:10.3389/fmicb.2013.00223
Moorhead, D. L., Sinsabaugh, R. L., Hill, B. H., and Weintraub, M. N. (2016). Vector Analysis of Ecoenzyme Activities Reveal Constraints on Coupled C, N and P Dynamics. Soil Biol. Biochem. 93, 1–7. doi:10.1016/j.soilbio.2015.10.019
Mu, X., Zhao, Y., Liu, K., Ji, B., Guo, H., Xue, Z., et al. (2016). Responses of Soil Properties, Root Growth and Crop Yield to Tillage and Crop Residue Management in a Wheat-maize Cropping System on the North China Plain. Eur. J. Agron. 78, 32–43. doi:10.1016/j.eja.2016.04.010
Sinsabaugh, R. L., Follstad Shah, J. J., Hill, B. H., and Elonen, C. M. (2012). Ecoenzymatic Stoichiometry of Stream Sediments with Comparison to Terrestrial Soils. Biogeochemistry 111, 455–467. doi:10.1007/s10533-011-9676-x
Sinsabaugh, R. L., Hill, B. H., and Follstad Shah, J. J. (2009). Ecoenzymatic Stoichiometry of Microbial Organic Nutrient Acquisition in Soil and Sediment. Nature 462, 795–798. doi:10.1038/nature08632
Sinsabaugh, R. L., Lauber, C. L., Weintraub, M. N., Ahmed, B., Allison, S. D., Crenshaw, C., et al. (2008). Stoichiometry of Soil Enzyme Activity at Global Scale. Ecol. Lett. 11, 1252–1264. doi:10.1111/j.1461-0248.2008.01245.x
Sullivan, B. W., Alvarez-Clare, S., Castle, S. C., Porder, S., Reed, S. C., Schreeg, L., et al. (2014). Assessing Nutrient Limitation in Complex Forested Ecosystems: Alternatives to Large-Scale Fertilization Experiments. Ecology 95, 668–681. doi:10.1890/13-0825.1
Tao, Z., Li, C., Li, J., Ding, Z., Xu, J., Sun, X., et al. (2015). Tillage and Straw Mulching Impacts on Grain Yield and Water Use Efficiency of spring maize in Northern Huang-Huai-Hai Valley. Crop J. 3, 445–450. doi:10.1016/j.cj.2015.08.001
Tian, P., Lian, H., Wang, Z., Jiang, Y., Li, C., Sui, P., et al. (2020). Effects of Deep and Shallow Tillage with Straw Incorporation on Soil Organic Carbon, Total Nitrogen and Enzyme Activities in Northeast china. Sustainability 12, 8679. doi:10.3390/su12208679
Tian, S. Z., Ning, T. Y., Wang, Y., Liu, Z., Li, G., Li, G. J., et al. (2016). Crop Yield and Soil Carbon Responses to Tillage Method Changes in North China. Soil Till. Res. 163, 207–213. doi:10.1016/j.still.2016.06.005
Treseder, K. K., Ning, T. Y., Wang, Y., Liu, Z., Li, G., Li, Z. J., et al. (2008). Nitrogen Additions and Microbial Biomass: a Meta-Analysis of Ecosystem Studies. Ecol. Lett. 11, 1111–1120. doi:10.1111/j.1461-0248.2008.01230.x
Trivedi, P., Delgado-Baquerizo, M., Trivedi, C., Hu, H., Anderson, I. C., Jeffries, T. C., et al. (2016). Microbial Regulation of the Soil Carbon Cycle: Evidence from Gene-Enzyme Relationships. ISME J. 10, 2593–2604. doi:10.1038/ismej.2016.65
Urioste, A. M., Hevia, G. G., Hepper, E. N., Anton, L. E., Bono, A. A., and Buschiazzo, D. E. (2006). Cultivation Effects on the Distribution of Organic Carbon, Total Nitrogen and Phosphorus in Soils of the Semiarid Region of Argentinian Pampas. Geoderma 136, 621–630. doi:10.1016/j.geoderma.2006.02.004
Vance, E. D., Brookes, P. C., and Jenkinson, D. S. (1987). An Extraction Method for Measuring Soil Microbial Biomass C. Soil Biol. Biochem. 19, 703–707. doi:10.1016/0038-0717(87)90052-6
Wang, W., Lai, D. Y. F., Wang, C., Pan, T., and Zeng, C. (2015). Effects of rice Straw Incorporation on Active Soil Organic Carbon Pools in a Subtropical Paddy Field. Soil Tillage Res. 152, 8–16. doi:10.1016/j.still.2015.03.011
Waqas, A., Muhammad, Q., Huang, J., Dong, W., Sun, G., Liu, K., et al. (2020). Tillage Practices Improve rice Yield and Soil Phosphorus Fractions in Two Typical Paddy Soils. J. Soil Sediment. 20, 850–861. doi:10.1007/s11368-019-02468-3
Waring, B. G., Weintraub, S. R., and Sinsabaugh, R. L. (2014). Ecoenzymatic Stoichiometry of Microbial Nutrient Acquisition in Tropical Soils. Biogeochemistry 117, 101–113. doi:10.1007/s10533-013-9849-x
Xu, J., Han, H., Ning, T., Li, Z., and Lal, R. (2019). Long-term Effects of Tillage and Straw Management on Soil Organic Carbon, Crop Yield, and Yield Stability in a Wheat-maize System. Field Crops Res. 233, 33–40. doi:10.1016/j.fcr.2018.12.016
Zhao, H., Sun, B. F., Jiang, L., Lu, F., Wang, X. K., and Ouyang, Z. Y. (2015). Erratum to: How Can Straw Incorporation Management Impact on Soil Carbon Storage? A Meta-Analysis. Mitig. Adapt. Strat. Gl. 20, 1545–1568. doi:10.1007/s11027-014-9586-z
Zhao, S., Li, K., Zhou, W., Qiu, S., Huang, S., and He, P. (2016). Changes in Soil Microbial Community, Enzyme Activities and Organic Matter Fractions under Long-Term Straw Return in north-central china. Agric. Ecosyst. Environ. 216, 82–88. doi:10.1016/j.agee.2015.09.028
Zheng, L., Chen, H., Wang, Y., Mao, Q., Zheng, M., Su, Y., et al. (2020). Responses of Soil Microbial Resource Limitation to Multiple Fertilization Strategies. Soil Tillage Res. 196, 104474. doi:10.1016/j.still.2019.104474
Keywords: ecoenzymatic stoichiometry, tillage management, straw incorporation, nutrient acquisition, Chinese Mollisol
Citation: Zhang N, Chen X, Han X, Lu X, Yan J, Zou W and Yan L (2021) Responses of Microbial Nutrient Acquisition to Depth of Tillage and Incorporation of Straw in a Chinese Mollisol. Front. Environ. Sci. 9:737075. doi: 10.3389/fenvs.2021.737075
Received: 25 August 2021; Accepted: 09 November 2021;
Published: 30 November 2021.
Edited by:
Ilan Stavi, Dead Sea and Arava Science Center, IsraelReviewed by:
Joan Estrany, University of the Balearic Islands, SpainDima Chen, China Three Gorges University, China
Copyright © 2021 Zhang, Chen, Han, Lu, Yan, Zou and Yan. This is an open-access article distributed under the terms of the Creative Commons Attribution License (CC BY). The use, distribution or reproduction in other forums is permitted, provided the original author(s) and the copyright owner(s) are credited and that the original publication in this journal is cited, in accordance with accepted academic practice. No use, distribution or reproduction is permitted which does not comply with these terms.
*Correspondence: Wenxiu Zou, em91d2VueGl1QGlhZy5hYy5jbg==; Lei Yan, eWFubGVpXzc0QDE2My5jb20=
†These authors have contributed equally to this work