- DSM Nutritional Products Ltd., R&D Personal Care, Kaiseraugst, Switzerland
Ultraviolet (UV) filter compounds are the key constituents in sunscreens that protect human skin from detrimental solar radiation. Some of these products have attracted public attention due to claims of their potential negative impact on the environment, in particular marine fauna and flora. At present, consumers who want to make an eco-conscious decision cannot find suitable product details and need to rely on fragmented information or complex scientific literature. The same is true for sunscreen developers, typically formulation chemists, who at present can only work with black- and whitelists which recommend or omit compounds. The ecological impact evaluation system proposed in this publication makes it easier to choose UV filters. Selection is based intrinsically on specialized knowledge which is built on environmental safety data. By embedding this in an existing in-silico sunscreen design system, new products can be created during the project’s design phase without time and cost intensive investigations. In contrast to currently available methods, the proposed tool includes comparison with a virtual market benchmark so users can determine whether a newly developed product will be an improvement on the state of the art in terms of environmental compatibility. The level of improvement can be displayed as a logo which has been designed to be immediately understandable to consumers. The system can be re-adjusted in regular time frames, depending on market development, to motivate and drive the sunscreen industry towards more eco-compatible products.
Introduction
Humans love to expose themselves to sunlight, whether this means simply being outdoors, taking part in sports or enjoying holidays in sunny locations. This desire to be in the sun is probably strongly motivated by the mood enhancement that sun induces via different channels (Young et al., 2017). However, there are also more beneficial effects such Vitamin D synthesis through ultra-violet B (UVB) light (Reichrath, 2007), and a reduction in blood pressure (Neville et al., 2021). Unfortunately, there is a downside to sun exposure. This is due to the presence of a small, but significant amount of ultra-violet B (UVB) and A (UVA) radiation which causes long term tissue damage, leading to signs of aging and raising skin cancer incidence (Leiter et al., 2020). Sunscreens provide one way to minimize exposure to UV irradiation while still enjoying benefits such as Vitamin. D synthesis, which seem to occur even at lower UV dosage (Neale et al., 2019; Neville et al., 2021), or benefits triggered by visible light. They also have the potential to reduce skin cancer and signs of skin aging (Pérez and Bashline, 2019). Sunscreens are therefore a popular group of consumer products and play a role in almost everyone’s life. Nonetheless, there is also some resistance to using sunscreens–correctly, in sufficient amounts, or indeed at all, (Portilho and Leonardi, 2020; Vollhardt and Marchini, 2021), likely unconsciously triggered by sub-optimal application features or fear on possible skin reactions (Nash and Tanner, 2014). Sunscreen use can be jeopardized further if bad press occurs linking potential hazards to their main ingredients, namely the UV filter compounds. Studies have been published postulating human (Schlumpf et al., 2010; Abbasi, 2020) and environmental hazards (Downs et al., 2014; Apel et al., 2018). A couple of studies detecting man-made materials, including pharmaceutical and agricultural actives (Molins-Delgado et al., 2018a; Molins-Delgado et al., 2018b; Munz et al., 2018; Díaz-Cruz et al., 2019), already exist. UV absorbing substances have also been found in the environment (Barón et al., 2013; Tashiro and Kameda, 2013; Ramos et al., 2016; Apel et al., 2018; Schneider and Lim, 2019; Mitchelmore et al., 2021; Watkins and Sallach, 2021). The occurrence of a material in the environment represents a potential risk due to possible exposure. However, knowledge of hazardous properties (adverse effect concentrations), or details of mode of action (MOA) (Markert et al., 2020) are needed to perform a detailed risk analysis and call for action (Mitchelmore et al., 2021; Watkins and Sallach, 2021). Even so, sometimes, political activity is initiated without further risk evaluation (e.g., Hawaii ban of sunscreen filters (Hawaii, Senate of State, 2018).
Environmental hazard data on UV Filters published in scientific literature eventually reach consumers and, rightly, make them concerned (Downs et al., 2021). As they are unable to perform an expert risk analysis themselves, a typical response is to apply the avoidance principle and not use sunscreens at all to protect the environment. This may seem like a successful approach to environmental risk management, however, in the case of sunscreens, this leads to health risks for the individual they had not considered e.g., a higher chance of getting skin cancer. Therefore, an easy-to-understand environmental impact score could help with making educated choices.
UV filters require regulatory approval for use in personal care products or sunscreens, but different regions have different approval processes (Pistollato et al., 2021; Sabzevari et al., 2021). It is common for considerable efforts to be needed to get a new compound approved as a UV filter for use in sunscreens, particularly in the United States (US), where sunscreens are regulated as over-the-counter drugs by the Food and Drug Administration (FDA). Therefore, the number of available registered compounds is rather low, and even lower on a global basis due to regional differences in approval status.
Currently, a detailed environmental impact analysis is not a direct part of the UV filter approval process for granting permission for human use, nor it is part of the mandatory safety report required in product information files (PIF) for marketed products regulated by the European Cosmetics Directive. (European Council, 2009). However, in the European Union (EU), most materials used must be registered under the regulation on Registration, Evaluation, Authorization and Restriction of Chemicals (REACH, Council European Parliament, 2006), a system which specifically considers this topic and requires a significant set of data. Some authorities (e.g., National Industrial Chemicals Notification and Assessment Scheme, NICNAS/Australia, Danish Ministry of Environment and Food) already collect and review current eco-toxicological criteria for their own risk assessments (Mikkelsen et al., 2015). Independently, but linked to cosmetic ingredient regulation (Pistollato et al., 2021), a chemically produced compound for use in the EU needs to be registered in accordance with the REACH directive managed by the European Chemical Agency (ECHA). The purpose of this process is to identify and declare potential risks and hazards that may require corresponding declarations (Council European Parliament, 2008). In this context, environmental data are requested for the evaluation of environmental impact. Furthermore, registered substances may also undergo reassessment if the authorities, a member state, or an applicant request this. The Community Rolling Action Plan (CoRAP, based on Article 44 Materials and Methods REACH directive (ECHA, 2021) was initiated for this purpose. It is noteworthy that substances on this list are primarily due for re-evaluation for a range of reasons. e.g., wide-spread use. After evaluation, depending on the results, adjustments to permitted use may be made. Currently 31% of approved UV filters in the EU are on the CoRAP list (Status: 9 out of 29, October 2021) underlining the visibility and importance of this substance class.
These regulatory requirements have generated an extensive set of environmental data for UV filters which can be used to assign an environmental score. Knowledge and data on the environmental impact of materials will likely continue to grow even further in the coming years of research, leading to an increased level of detail and, as an outcome, more complexity around this matter. This calls for a robust evaluation scheme based on widely accepted endpoints, and that connects and condenses data in a transparent way and can be extended to harbor further endpoints.
There have been previous attempts to create product scores based on environmental data. Long et al. (2006) published a system that first utilized aqua toxicity and biodegradability together with a couple of other parameters to form an evaluative parameter. Human data also played a role in this system, although in the case of UV filters this is generally covered by the registration process already. Later, Predale et al. (2017) introduced a system based on the three parameters of aquatic toxicity, biodegradability and persistence, similar to the Persistence, Bioaccumulation and Toxicity (PBT) concept utilized by the ECHA (Council European Parliament, 2006). A similar approach, dedicated to UV filters, has also been taken by Pawlowski et al. (2020). All these systems create a score that has some absolute character and a scale that does not start at zero impact. It is possible now to compare different products to each other. Nevertheless, even the score for the best sunscreen possible would still communicate an environmental impact to the consumer, which could perhaps suggest to them that the optimal point of zero impact is using no sunscreen. Regarding potential health protection, this outcome is undesirable in a group of products that already meets with resistance and compliance from consumers.
Therefore, it would be beneficial for the creators of sunscreens, the formulators, to have a characterizing parameter that scales with a single parameter for a sunscreen formula’s eco-compatibility, thus establishing a minimum regarding environmental impact. This parameter should be created with open access and be automatically based on the individual contributions of different UV filters, removing the need for a detailed, manual environmental risk analysis each time. Additionally, it is necessary to communicate this complex matter to consumers adequately and in an easy-to-understand way, so that they are motivated to use sun protection.
Materials and Methods
Translation of Substance Related Eco-Endpoints Into Absolute Eco-Scores for UV Filters and Sunscreens
1. Determination of the Environmental Friendliness of UV Absorber and Sun Protection Products
Rating systems, also proprietary versions, have been created previously (Long et al., 2006; Predale et al., 2017; Pawlowski et al., 2020). However, these systems do not have a benchmarking concept, meaning that their scores have absolute characters, and product designers and end consumers need to be taught the scoring system and scale with the above-mentioned disadvantages.
In our proposed novel approach, first, each UV filter is analyzed individually based on standardized eco-toxicological relevant data (Figure 1), delivering an Eco impact score for each substance (blue box, Figure 1). In the second step, to rate the overall impact for a sunscreen composed of several UV filters, each individual UV filter score is weighted according to its concentration (User data, Orange box in Figure 1) and then summarized to yield a sunscreen eco-impact score (Green box, Figure 1). In the final and essential third step, this finding is further correlated to a virtual average sunscreen eco-impact score per sun protection factor (SPF) category, created from hundreds of currently available commercial products. The virtual average represents the current state of sunscreens on the market. By comparing the individual sunscreen eco-impact score with the corresponding market average, an easy-to-understand categorization in seven classes can be established.
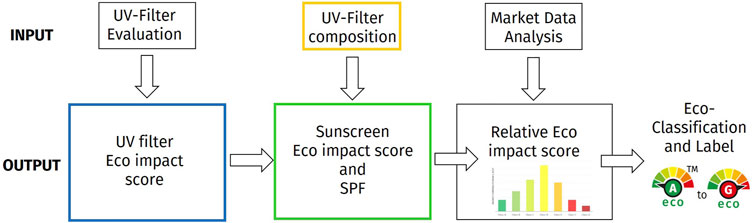
FIGURE 1. Flow chart for the in-silico formulation evaluation, UV Filter evaluation, Filter composition and supporting market data relevance estimation-leading in combination to Eco classification and corresponding Ecolabel.
The REACH registration dossiers at ECHA served as our source of eco-data. Most of these data are publicly available. The ECHA is an EU authority that regulates the technical, scientific and administrative aspects involved in the registration, evaluation and approval of chemicals in accordance with Regulation No. EG 1907/2006 of December 18, 2006 (Council European Parliament, 2006).
The environmental impact of chemicals is also part of the assessment (ECHA, 2017). Substances are evaluated according to their impact in terms of persistence (inverse: biodegradability), bioaccumulation and toxic effects in certain selected species. The aim is to identify persistent (P), bio accumulative (B), toxic (T) and very persistent (vP) or very bio accumulative (vB) properties of chemicals. This regulatory classification can lead to the designation “PBT” or “vPvB” (very persistent, very bioaccumulative) for the respective substance, if all three categories are assessed as being applicable. Due to this quantity-dependent, mandatory notification and the resulting classification, extensive data sets exist both for registered UV filters and other materials. These data have been created through generally recognized, standardized and validated test systems such as the Organisation for Economic Co-operation and Development (OECD) guidelines collected (e.g., OECD 309 OECD, 2004) in the corresponding dossiers at the ECHA. Additionally, reliability information is given in line with the system proposed by Klimisch et al. (1997).
Based on these ECHA data sets, we analyzed the UV absorbers individually within the three parameters of Persistence, Bioaccumulation, and species relevant Toxicity–without the intention to generate a regulatory PBT/vPvB classification for the chemical.
P-Parameter: Biodegradation/Persistence
B-Parameter: Bioaccumulation
Relevant T-Parameter: Toxic effects
• On fish with short term exposure (Acute Tox Fish)
• On fish with long term exposure (Chronic Tox Fish)
• On invertebrates with short term exposure (Acute Tox Invertebrates)
• On invertebrates with long term exposure (Chronic Tox invertebrates)
• On algae, water plants (e.g., Lemna gibba)
• On microorganisms (e.g., measured by their oxygen consumption) during exposure
Although the data set currently available is sufficiently complete, new findings can easily be incorporated into the system for fine tuning. When accepted and validated methodologies have been developed, data - e.g. on gammarids or corals - can be included. We suggest including parameters such as confirmed endocrine disruption activity, specific environmental hazard classifications (e.g. Category Acute/Chronic 1) or substance hydrolysis and/or solubility information as modifiers for the classification. Research data generated using methods that have not been harmonized and properly validated are currently excluded. By way of example, as no European Centre for the Validation of Alternative Methods (ECVAM) validated/approved method for the effect of chemicals on corals currently exists, these publications are not yet included. OECD guidelines or respective precursors are the chosen documents for all evaluations as they allow direct comparison of results from different sources or applicants. Consequently, and due to evaluation complexity, toxicological publications using different, modified determination setups and methods are disregarded (e.g., Fastelli and Renzi, 2019; He et al., 2019). In such cases the comparability or transfer of measured endpoints is very complex or even not possible. When validated methods are established and new datasets become available, this can be addressed, and they can be integrated into the system through a system update.
Each parameter has been awarded 100 points and the maximum number of points achievable is set to 100. We use a weighting between the PBT parameters of 20:20:60. The highest weighting is given to the toxicity endpoints, as these are most important and most relevant. Nevertheless, focusing only on toxicity may disregard materials that are known to be unstable in the environment and would not lead to issues, due to their fast disappearance. Therefore, Biodegradation in combination with the test item’s solubility is addressed in case chronic exposure evaluations are missing (See Table 2 and explanations).
Scoring in Detail
P-Parameter
The maximum number of points for persistence can be 20, if the ingredient is considered “rapidly biodegradable (readily biodegradable).” 10 points are awarded if the substance is considered “inherently or ultimately biodegradable.” A score of 0 applies for “non-biodegradable” chemicals (Table 1); no further interim values are intended in the P-Parameter. The assessment of biodegradability properties of a substance is strongly dependent on the OECD method used. A substance that has already been evaluated using OECD 301/302 (OECD, 1992) studies may not show any degradation, but can still be considered biodegradable defined by OECD method 307–309. In such situation the latter study is used for the rating (OECD, 2004). In one special case, additional bonus points are granted, even if the substance is not readily biodegradable on one side (based on OECD criteria) but is rapidly hydrolyzed (half-life of less than 10 days). In this case, e.g., Homosalate, the ingredient is awarded 10 points (half of the maximal score P-Parameter).
Should there be no reliable data, the score is set to zero. Half the maximal score may then be allocated, but only if an acceptable alternative method–such as a non-OECD/ECVAM validated test, a reliable Quantitative Structure-Activity Relationship (QSAR) assessment, or sound data bridging–has been applied. Examples include missing studies on Homosalate with bridging to Ethylhexyl salicylate (EHS), or QSAR modelling for replacing chronic invertebrate testing in the case of Dibutyl triazine (DBT).
B-Parameter
The maximum number of points for bioaccumulation - 20- is awarded for a substance that is regarded as “not bioaccumulative” (Bioconcentration factor, BCF < 2000), and 10 points are assigned if the “considered” not bioaccumulative” claim is based on in-silico evaluations or physical/chemical property argumentation, without experimental measurements. Zero points are given if the ingredient is considered bioaccumulative or no data exists. Similarly to above, if data is available from an acceptable alternative method (e.g., non-OECD/ECVAM validated test), half the maximal score can be allocated.
T-Parameter
For the Toxicity criteria, six toxicological endpoints representing key indicator species for the aquatic environment are considered (see above) and a maximum of 10 points per parameter is awarded for each endpoint.
Substances classified as “not toxic” in the test system will receive the highest score of 10 points. Substances that are considered “potentially not toxic” in the test setup based on weight-of-evidence approaches, in-silico evaluations, related hazard assesments, or data bridging with a homologous substance, will be awarded 5 points. Substances that are “toxic” score zero points. Penalties apply based on additional information, such as confirmed endocrine disruptive effects based on experiments listed in the OECD Guidance (OECD, 2018) or regulatory classifications (e.g., acutely/chronically toxic for aquatic organisms class 1 according to CLP EG 1272/2008 (Council European Parliament, 2008). In such cases, the deduction for each is 5 points.
Preferably, all environmental and toxicological endpoints used in the assessment are based on and determined by the related OECD Guidelines. If this is not the case and/or the data collection is deemed “not reliable” [evaluation according to Klimisch et al. (1997)], or reference is made to a related molecule (structural bridging), or the evaluation is done exclusively by means of computer modelling (in-silico, QSAR), a deduction of 50% is applied to reflect this situation.
Ideally, full OECD data sets are available for all six toxicological endpoints. The solubility characteristics of a substance can interact with the T-Parameter assessment. Special cases are represented by the lack of chronic datasets under certain circumstances. Chronic ecotoxicity data for water soluble substances may not be available, as–according to the EU REACH Regulation (Council European Parliament, 2006)– testing for chronic ecotoxicity endpoints can be waived if the chemical safety assessment indicates “no risk” based on existing acute data. Acute ecotoxicity data has often been generated in the past using concentrations above the water solubility of the substance, whereas chronic evaluations may have been performed with concentrations near the water-solubility values. The rules shown in Table 2 apply in those cases where a UV filter is classified as “readily biodegradable” or regarded as “water-soluble”. If a substance is water soluble, bio-accumulation is less likely to appear, and the acute data receives a slightly higher weighting. A biodegradable substance is not expected to remain in the aquatic environment for a long period of time, also resulting in a adapted weighting of the acute data. An expample for this is the readily biodegradable UVB filter Ethylhexyl salicylate (EHS). Some specific combinations have been defined in Table 2.

TABLE 2. Overview of specific data set situations to take solubility and incomplete OECD datasets into account.
For example, if a water-soluble substance shows no effects for acute toxicity (fish and invertebrates) and no data on chronic exposure are available, the score is upgraded by +5. The reasoning is that within a water-based test setup, the information weight of an acute study of a water-soluble substance is higher than for a similar study of an oil-soluble substance. The reliability, having exposed the organisms to the test item in the correct way (aspired test concentration), is higher compared to a poorly water-soluble ingredient. In addition, bioaccumulation of the test substance also has a lower probability. Similarly, a lower bonus is allocated if chronic exposure data for oil-soluble UV filters are not available, but the substance is considered readily biodegradable. In this case, provided that the available toxicological data do not show negative effects, an additional 2.5 points are counted for acute toxicity (fish and invertebrates). In a case where an oil-soluble substance is found to be not biodegradable and no chronic data is present, this is treated as a simple “missing dataset,” resulting in 0 points for chronic toxicity without any additional scores. Again, ideally–independent of the test substance’s solubility–acute and chronic OECD tests will have been performed. If both show no effects, each biological endpoint will receive the maximum 10 points. Generally applicable for all included parameters–in case interpretation is based on weight of evidence (e.g., two or more datasets from various sources with different reliability scores are assessed together) or only tendencies towards endpoints–scoring can be gradational, in steps of 2.5 (e.g., at the T-parameters 0, 2.5, 5.0, 7.5, and 10). For example, bridging a test substance to a structural homologue with no effect in the T-parameter, can only deliver a maximum score of 5. If the bridging in this hypothetical case is classified as “not reliable,” a maximal 50% is assigned-so the score would be 2.5.
2. Sunscreen Eco-Scores Containing a Mix of UV Filters
The environmental impact of the UV filter is assessed by adding up the number of points awarded within each evaluation criteria (Sum of points, SP). To transfer this to the individual environmental impact (EIi), each sum of points needs to be subtracted from the maximum possible value of 100. The choice of filters and their usage concentration create the efficacy (e.g., SPF) of the sun protection product. In Toxicology the dose response relationship is a key element (Grandjean, 2016). Present amounts are also a key parameter required in most toxicological evaluations, e.g., generating values for the Threshold of Toxicological Concern (TTC) (Committee et al., 2019), or calculating the margin of safety (MOS) as an essential part of the safety evaluation of finished consumer products described by the Scientific Committee for Consumer Safety (SCCS) (Bernauer et al., 2021). In the MOS evaluation of a cosmetic ingredient, the used concentration and the expected skin permeation behavior are correlated and set in relation to existing toxicological endpoint animal data. Similarly, for environmental evaluations, environmental monitoring data and effect-concentrations are crucial (Backhaus and Faust, 2012; Markert et al., 2020). A strong dilution effect can be expected in the environment that results in isolation of the individual substances from initial mixtures. Therefore the used approximation of a linear additive effect might be realistic for the UV filter substances (Heys et al., 2016). With selections of different filters, individual environmental impact values are therefore multiplied with their corresponding usage concentration (ci) in the selection to emphasize the significance of usage concentration for environmental effects. This delivers the UV filter’s total combined environmental impact (EIc) as shown in Eq. 1:
EIc: Environmental impact of UV absorber composition.SPi: Sum of points of UV absorber (i) in the formulation.ci: Individual concentration of UV absorber (i) in the combination.i: Number of UV absorbers in the sun protection combination.
The EIc value as defined above is independent of the sunscreen product’s performance. Naturally low SPF products need less UV filter content and will therefore have a better EIc score–but they do not protect as effectively as products with a higher SPF. Moreover, there will be no sunscreen that has an EIc score of zero. This may suggest to consumers that it would be better to adopt an avoidance principle and not use sunscreen at all; ignoring the detrimental health effects that such behavior may cause. Furthermore, it requires training to comprehend the meaning of EIc. We therefore introduced a relative parameter that also links to the sunscreen’s performance. To do this we connected the EIc with the SPF by using the SPF classes as defined in the EU (Comission European, 2006), e.g., SPF 30 or 50 or 50+. This requires either a formula’s claimed SPF or an SPF calculated with an in-silico tool (such as the DSM SUNSCREEN OPTIMIZER™). Together, this yields the “SPF class” of the formula and its EIc., which then needs to be compared to the average EIc for that SPF class to obtain a relative parameter.
Ideally, to calculate a SPF-specific, market-average, eco-impact score, compositional information on SPF claims needs to be known for many products on a defined market, e.g., Europe. Unfortunately, such information is not available in the public domain. To overcome this difficulty, we used ingredient information for >1,000 products launched in the EU between January 2016 and October 2019. We created a read out algorithm to generate such data automatically and used the results to feed the DSM SUNSCREEN OPTIMIZER™ with sunscreen active ingredients individually. An automated in-silico optimization algorithm then estimated the most likely composition to receive a product’s reported SPF claim and its eco-impact score. By inputting several hundreds of products analyzed by this method, we arrived at an EIc distribution per SPF class and set the median to define the market “average” (see Table 3 and Figure 2).
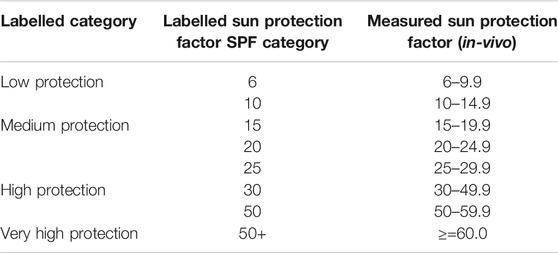
TABLE 3. Categories of sun protection products, their respective labels, and measured SPFs according to European legislation.
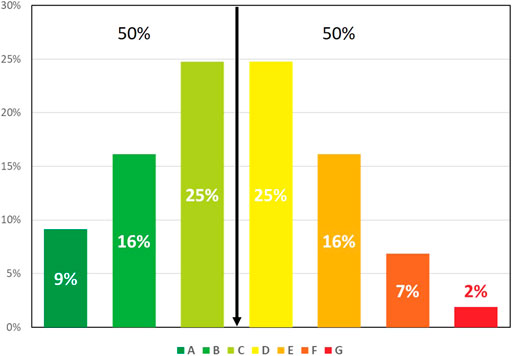
FIGURE 2. Suggested ECO Class classification ranges; Eco Classes generated on market analysis clustered in contribution of respective SPF reference group and related EIcs classification ranges.
The intrinsic values of individual market products-standardized per SPF category for environmental compatibility of the combination of UV filters (EIc)–were calculated using Eq. 2.
EIcs: Standardized environmental impact of UV absorber composition.SPi: Sum of points of UV absorber (i) in the formulation.ci: Individual concentration of UV absorber (i) in the combination.i: Number of UV absorbers in the sun protection combination.SPF Label category: EU SPF category as defined in Table 3.
For each SPF class, this yields both the median eco-impact and the distribution eco-impact for all sunscreen products included-which in our case follows a log-normal distribution. From this distribution, seven impact classes can be defined as shown in Table 4. Figure 2 represents the log-normal distribution with a global median in the middle of class C and D, and the Histogram in Figure 3 shows the corresponding frequency of samples used. This median defines the benchmark for comparing all products analyzed and classified.
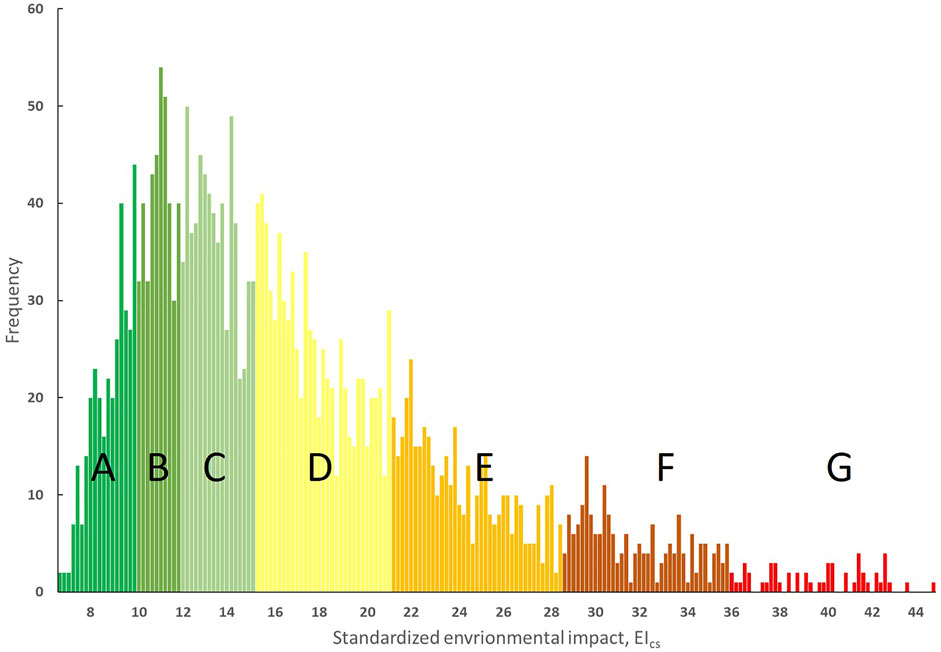
FIGURE 3. Histogram showing frequency of samples with different standardized Eco Impacts (EIcs) from the market analysis.
3. Classification Visualization
A relative classification will enable our industry to strive for the most eco-compatible sunscreens and sunscreen market in general. However, this will only be successful if consumers understand and accept the concept. Therefore, it is important to communicate to consumers in a quick and easy-to-comprehend way that does not require in-depth background knowledge. Other industries have already used graphical symbols with a red to green, truncated rainbow color system. To the best of our knowledge, no approach based on existing commercial references has yet been established for cosmetic, medical, or pharmaceutical products. For consumers to benefit it is essential that they recognize and understand the main information. The system we propose is therefore based on efficiency categories which are allocated a letter from A to G and a corresponding color on our gradient from green (A) to red (G). This is shown in Figure 4.

FIGURE 4. Label “Environmental compatibility of a sunscreen,” the visuals as used in the DSM SUNSCREEN OPTIMIZER™
Exemplary filter combinations (Combination 1–6) with SPF 25, 30 and SPF 50 + are shown in Figure 5 with related calculation steps embedded. The colored parts represent the actions (ci, concentration input by the user, orange) and values (e.g., SPi with corresponding individual impact EIi, blue and filter combination impact EIc, green) within the methodological flow (Figure 1). Combinations 1 and 2 have an overlap in filter composition, but differences in used concentrations. These differences result in distinct SPF categories (SPF 50+ and SPF 30). Filter combination 3 demonstrates that it is possible to generate the same protection efficacy in the form of sun protection factor with a different UV Filter combination. However, the calculated eco-impact is higher compared to Eq. 2. Formula 3 also needs 6.1% more UV filter content to achieve a similar SPF and is therefore less favorable. Combination 4 and 5 demonstrate similar situations for the SPF 25 category. The 6th combination of UV filters is an example where in-vivo SPF data (SPF = 60) already exists and has been manually entered in the system. In such a case, the in-vivo results overrule the calculated SPF of 59.1 resulting in a change in SPF category (SPF 50 to SPF 50+) and consequently to the use of a different market benchmark.
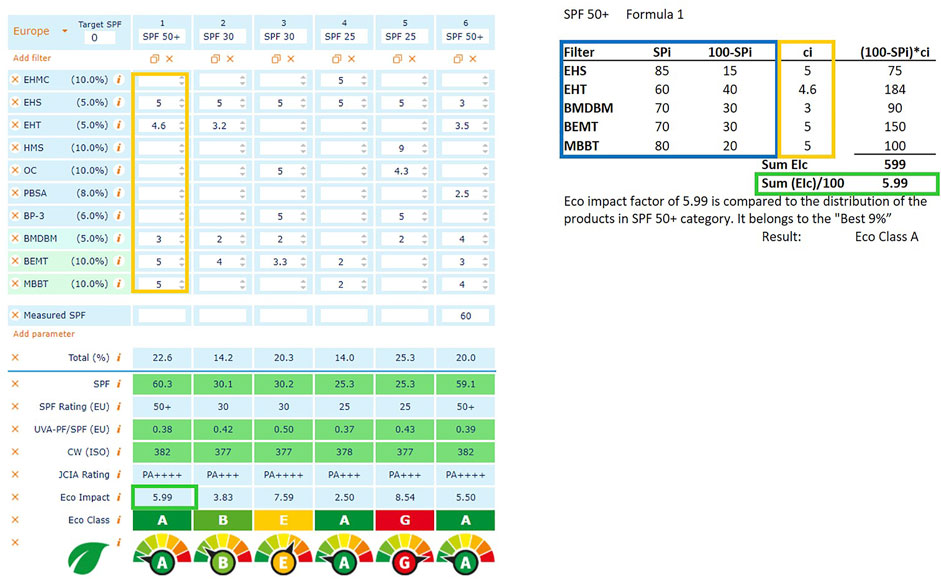
FIGURE 5. Example of UV filter combinations and calculation values leading to the creation of an Eco impact value and corresponding classification. (Abbreviations used: see below), Inserted Table: Blue box show values related to Eq. 1, Orange Frame: User input / concentration of individual UV Filters, Green box: EIc-Result used for classification according to Eq. 2.
The sum of points (SPi) generates the correlated impact (100-SPi) which is multiplied with the ingredient concentration. The sum of all filters generates the environmental impact of the composition (EIc). For simplicity, the EIc is divided by a factor of 100 resulting in the compositional eco-impact value returned by the tool. This value is instantly compared with the dataset of the corresponding SPF class. In the case of Eq. 1, the EIc of 5.99 corresponds to the best 9% (see Figure 2) and is therefore rated as Eco-Class A.
By way of example, two distinct combinations for a product in the SPF 25 category are shown as formulations 4 and 5. Once again, this example shows that products with the same protection efficacy for UVB irradiation (SPF Rating 25) could display extreme differences when it comes to environmental impact. This is due to the different individual UV absorber points in the first part of the evaluation. In the second part, the eco-impact of Combination 4 is much lower compared to the chosen filters in Combination 5–2.50 and 8.54 respectively. Our eco-impact scores are simple numeric values that make it possible to compare the two formulations. For consumer communication, the half-clock system at the bottom of each column illustrates the marked difference. Not only is the specific formulation in Combination 5 less favorable from an eco-score perspective, but additionally, more UV Filters were needed to achieve the same performance (25.3% instead of 14.0%). Our example illustrates how a formulator can use this system to experiment with different formulation designs and evaluate them against each other before progressing to actual laboratory work. Furthermore, using this system, which has been newly implemented into the DSM SUNSCREEN OPTIMIZER™, UV absorbers can be easily combined and selected, not only according to different parameters for sun protection performance, oil load and cost level, but for ecological impact as well.
Discussion
Sunscreens have a widespread use. Although their main constituents, the UV filters, need to comply with various regulations which involve data-intensive evaluations, concerns and mistrust about the safety and environmental compatibility of these products have built up on the consumer side. The safety of UV filters is already amply evaluated by independent bodies, however, such data-intensive analyses are not explicitly addressed at consumers. For example the SCCS proposed methodology for the safety evaluation of personal care products (Bernauer et al., 2021) takes exposure and potential effects on humans into account. The process assumes a non-synergistic or antagonistic interaction of ingredients, a limitation that is likely to cover most cases. Nevertheless, it is an approximation awaiting more granularity in the future. This approach is also intrinsically embedded into the official approval process for UV filters, however, as such, it is not communicated to consumers who remain unaware that the ingredients and the final product has undergone mandatory evaluations before it arrives at the point of sale. Moreover, this approach does not assess potential environmental impact. To address the issue of communication on environmental compatibility, we created the tool described above. This then led to our creating information graphics to support consumers in choosing the most appropriate products. Sunscreen developers can use the tool for preselection at laboratory scale, including direct correlation with market benchmarks.
At present, our tool only covers UV filters in a sunscreen, which from a mass perspective account for more than 50% of non-volatile (water, ethanol) additional ingredients. It also includes those ingredients currently receiving most attention and concern, particularly since the Hawaiian ban (effective January 2021) on sun products containing Benzophenone-3 (Oxybenzone) and Ethylhexyl methoxycinnamate (Octinoxate) (Hawaii, Senate of State, 2018) was announced. Nevertheless, it can be opened to include more ingredient categories in the future, working towards a whole formula evaluation. The interaction between persistence, bioaccumulation and toxicity (P, B and T) is still the cornerstone of assessing environmental compatibility and represents an ideal starting point for creating a score, as others in the past have used those criteria (Long et al., 2006; Predale et al., 2017; Pawlowski et al., 2020). The aquatic system is an important pathway for environmental entry of UV filters (Posthuma et al., 2020; Mitchelmore et al., 2021) and therefore different exposure scenarios and toxicological parameters (e.g., soil related toxicity testing) are not included in the current version of the tool. In principle, however, these could be added at a later stage.
The tool assumes that individual components have a linear, additive, and concentration-dependent impact on the environment, an approximation likely to fit most cases, however, it does not include any synergistic or antagonistic interactions that could also occur (Markert et al., 2020). At present, this is the best approach we have to carrying out analysis, but to further improve the accuracy of predictions, such factors could be incorporated into the tool should they become assessable.
Environmental compatibility continues to be an important and hot topic for sunscreens. Consumers want to make the right choice, but the information they need to make an educated decision is often fragmented and complex. Furthermore, they lack the in-depth, expert knowledge required to understand the results of vast scientific studies in any meaningful way. Here, we propose a system that condenses all available data–based on OECD guideline conformance studies–into a score and compares this score to a market benchmark for each SPF class. For an eco-symbol, we suggested a graphical clock type figure: there is a very high density of information behind this symbol, but it is instantly understandable. With an online application, the DSM Sunscreen Optimizer, eco-classifications can be calculated either from the composition of existing sunscreens or during the development and design phase for new sunscreens. The advantage of this eco-classification is its intrinsic power to push our sunscreen industry towards even more eco-friendly formulas and new innovations in sun care, supported by the buying decisions of the consumer. This system can demonstrate a strong commitment to the environment.
Data Availability Statement
The raw data supporting the conclusions of this article will be made available by the authors, without undue reservation.
Author Contributions
Conceptualization, GK, JV, and AS; methodology, GK and AS; investigation, GK; AS, and EJ; data curation, GK; AS, and EJ; writing—original draft, GK and JV; supervision, NH and KB.
Conflict of Interest
Authors GK, AS, NH, EJ, KB, and JV were employed by the company DSM Nutritional Products Ltd.
Publisher’s Note
All claims expressed in this article are solely those of the authors and do not necessarily represent those of their affiliated organizations, or those of the publisher, the editors and the reviewers. Any product that may be evaluated in this article, or claim that may be made by its manufacturer, is not guaranteed or endorsed by the publisher.
Acknowledgments
The authors also thank Marc Heidl for essential input at early stage of the project as well as all further colleagues at DSM Nutritional Products for their support during this project.
References
Abbasi, J. (2020). FDA Trials Find Sunscreen Ingredients in Blood, but Risk Is Uncertain. Jama 323, 1431–1432. doi:10.1001/jama.2020.0792
Apel, C., Joerss, H., and Ebinghaus, R. (2018). Environmental Occurrence and hazard of Organic UV Stabilizers and UV Filters in the Sediment of European North and Baltic Seas. Chemosphere 212, 254–261. doi:10.1016/j.chemosphere.2018.08.105
Backhaus, T., and Faust, M. (2012). Predictive Environmental Risk Assessment of Chemical Mixtures: A Conceptual Framework. Environ. Sci. Technol. 46, 2564–2573. doi:10.1021/es2034125
Barón, E., Gago-Ferrero, P., Gorga, M., Rudolph, I., Mendoza, G., Zapata, A. M., et al. (2013). Occurrence of Hydrophobic Organic Pollutants (BFRs and UV-Filters) in Sediments from South America. Chemosphere 92, 309–316. doi:10.1016/j.chemosphere.2013.03.032
Bernauer, U., Bodin, L., Chaudhry, Q., Coenraads, P., Dusinska, M., Ezendam, J., et al. (2021). The SCCS Notes of Guidance for the Testing of Cosmetic Ingredients and Their Safety Evaluation – 11th Revision - SCCS/1628/21. Regul. Toxicol. Pharmacol. 127, 105052. Scientific Committee for Consumer Safety (SCCS, EC. doi:10.1016/j.yrtph.2021.105052
Comission European (2006). Commission Recommendation of 22 September 2006 on the Efficacy of Sunscreen Products and the Claims Made Relating Thereto (2006/647/EC). Available at: http://data.europa.eu/eli/reco/2006/647/oj.
Committee, E. S., More, S. J., Bampidis, V., Benford, D., Bragard, C., Halldorsson, T. I., et al. (2019). Guidance on the Use of the Threshold of Toxicological Concern Approach in Food Safety Assessment. EFSA J. 17, e05708. doi:10.2903/j.efsa.2019.5708
Council European Parliament (2008). Regulation (EC) No. 1272/2008 of the European Parliament and of the Council of 16 December 2008 on Classification, Labelling and Packaging of Substances and Mixtures, Amending and Repealing Directives 67/548/EEC and 1999/45/EC, and Amending Regulation (EC) No 1907/2006 (Text with EEA Relevance). Available at: http://data.europa.eu/eli/reg/2008/1272/oj.
Council European Parliament (2006). REGULATION (EC) No 1907/2006 of the EUROPEAN PARLIAMENT and of the COUNCIL of 18 December 2006 Concerning the Registration, Evaluation, Authorisation and Restriction of Chemicals (REACH), Establishing a European Chemicals Agency, Amending Directive 1999/45/EC and Repealing Council Regulation (EEC) No. 793/93 and Commission Regulation (EC) No. 1488/94 as Well as Council Directive 76/769/EEC and Commission Directives 91/155/EEC, 93/67/EEC, 93/105/EC and 2000/21/EC. Available at: http://data.europa.eu/eli/reg/2006/1907/2014-04-10.
Díaz-Cruz, M. S., Molins-Delgado, D., Serra-Roig, M. P., Kalogianni, E., Skoulikidis, N. T., and Barceló, D. (2019). Personal Care Products Reconnaissance in EVROTAS River (Greece): Water-Sediment Partition and Bioaccumulation in Fish. Sci. Total Environ. 651, 3079–3089. doi:10.1016/j.scitotenv.2018.10.008
Downs, C. A., DiNardo, J. C., Stien, D., Rodrigues, A. M. S., and Lebaron, P. (2021). Benzophenone Accumulates over Time from the Degradation of Octocrylene in Commercial Sunscreen Products. Chem. Res. Toxicol. 34, 1046–1054. doi:10.1021/acs.chemrestox.0c00461
Downs, C. A., Kramarsky-Winter, E., Fauth, J. E., Segal, R., Bronstein, O., Jeger, R., et al. (2014). Toxicological Effects of the Sunscreen UV Filter, Benzophenone-2, on Planulae and In Vitro Cells of the Coral, Stylophora Pistillata. Ecotoxicology 23, 175–191. doi:10.1007/s10646-013-1161-y
ECHA (2017). Guidance on Information Requirments and Chemical Safety Assessment - Chapter R.11: PBT/vPvB Assessment. Helsinki: ECHA.
European Council (2009). Regulation (EC) No 1223/2009 of the European Parliament and the Council of 30 November 2009 on Cosmetic Products. Available at: http://data.europa.eu/eli/reg/2009/1223/oj.
Fastelli, P., and Renzi, M. (2019). Exposure of Key marine Species to Sunscreens: Changing Ecotoxicity as a Possible Indirect Effect of Global Warming. Mar. Pollut. Bull. 149, 110517. doi:10.1016/j.marpolbul.2019.110517
Grandjean, P. (2016). Paracelsus Revisited: The Dose Concept in a Complex World. Basic Clin. Pharmacol. Toxicol. 119, 126–132. doi:10.1111/bcpt.12622
Hawaii, Senate of State (2018). SB 2571 Act 104 Relating to Water Pollution. Hawaii: Hawaii State Legislature.
He, T., Tsui, M. M. P., Tan, C. J., Ma, C. Y., Yiu, S. K. F., Wang, L. H., et al. (2019). Toxicological Effects of Two Organic Ultraviolet Filters and a Related Commercial Sunscreen Product in Adult Corals. Environ. Pollut. 245, 462–471. doi:10.1016/j.envpol.2018.11.029
Heys, K. A., Shore, R. F., Pereira, M. G., Jones, K. C., and Martin, F. L. (2016). Risk Assessment of Environmental Mixture Effects. RSC Adv. 6, 47844–47857. doi:10.1039/C6RA05406D
Klimisch, H.-J., Andreae, M., and Tillmann, U. (1997). A Systematic Approach for Evaluating the Quality of Experimental Toxicological and Ecotoxicological Data. Regul. Toxicol. Pharmacol. 25, 1–5. doi:10.1006/rtph.1996.1076
Leiter, U., Keim, U., and Garbe, C. (2020). Epidemiology of Skin Cancer: Update 2019. Adv. Exp. Med. Biol. 1268, 123–139. doi:10.1007/978-3-030-46227-7_6
Long, D. C., Martin, F. H., and Weeks, J. A. (2006). “Methods for Evaluating Chemical Components Based on Their Proposed Function in the Product They Are to Be Used in,”. US7096084B2 (United States: SC Johnson and Son Inc).
Markert, N., Rhiem, S., Trimborn, M., and Guhl, B. (2020). Mixture Toxicity in the Erft River: Assessment of Ecological Risks and Toxicity Drivers. Environ. Sci. Eur. 32, 51. doi:10.1186/s12302-020-00326-5
Mikkelsen, S., Lassen, C., Warming, M., Hansen, E., Brinch, A., Brooke, D., et al. (2015). “Survey and Health Assessment of UV Filters,” Survey of chemical substances in consumer products No. 142 (Denmark: The Danish Environmental Protection Agency).
Mitchelmore, C. L., Burns, E. E., Conway, A., Heyes, A., and Davies, I. A. (2021). A Critical Review of Organic Ultraviolet Filter Exposure, Hazard, and Risk to Corals. Environ. Toxicol. Chem. 40, 967–988. doi:10.1002/etc.4948
Molins-Delgado, D., Muñoz, R., Nogueira, S., Alonso, M. B., Torres, J. P., Malm, O., et al. (2018). Occurrence of Organic UV Filters and Metabolites in Lebranche Mullet (Mugil liza) from Brazil. Sci. Total Environ. 618, 451–459. doi:10.1016/j.scitotenv.2017.11.033
Molins-Delgado, D., Olmo-Campos, M. d. M., Valeta-Juan, G., Pleguezuelos-Hernández, V., Barceló, D., and Díaz-Cruz, M. S. (2018). Determination of UV Filters in Human Breast Milk Using Turbulent Flow Chromatography and Babies' Daily Intake Estimation. Environ. Res. 161, 532–539. doi:10.1016/j.envres.2017.11.033
Munz, N. A., Fu, Q., Stamm, C., and Hollender, J. (2018). Internal Concentrations in Gammarids Reveal Increased Risk of Organic Micropollutants in Wastewater-Impacted Streams. Environ. Sci. Technol. 52, 10347–10358. doi:10.1021/acs.est.8b03632
Nash, J. F., and Tanner, P. R. (2014). Relevance of UV Filter/sunscreen Product Photostability to Human Safety. Photodermatol. Photoimmunol. Photomed. 30, 88–95. doi:10.1111/phpp.12113
Neale, R. E., Khan, S. R., Lucas, R. M., Waterhouse, M., Whiteman, D. C., and Olsen, C. M. (2019). The Effect of Sunscreen on Vitamin D: a Review. Br. J. Dermatol. 181, 907–915. doi:10.1111/bjd.17980
Neville, J. J., Palmieri, T., and Young, A. R. (2021). Physical Determinants of Vitamin D Photosynthesis: A Review. JBMR Plus 5, e10460. doi:10.1002/jbm4.10460
OECD (2004). OECD. Test No. 309: Aerobic Mineralisation in Surface Water–Simulation Biodegradation Test. Rossdorf, Germany: OECD Guidelines for the Testing of Chemicals, Section 3, OECD Publishing. doi:10.1787/9789264070547-en
OECD (2018). Revised Guidance Document 150 on Standardised Test Guidelines for Evaluating Chemicals for Endocrine Disruption. Paris: OECD Publishing.
Pawlowski, S., Herzog, B., Sohn, M., Petersen-Thiery, M., and Acker, S. (2020). EcoSun Pass: A Tool to Evaluate the Ecofriendliness of UV Filters Used in Sunscreen Products. Int. J. Cosmet. Sci. 43, 201–210. doi:10.1111/ics.12681
Pistollato, F., Madia, F., Corvi, R., Munn, S., Grignard, E., Paini, A., et al. (2021). Current EU Regulatory Requirements for the Assessment of Chemicals and Cosmetic Products: Challenges and Opportunities for Introducing New Approach Methodologies. Arch. Toxicol. 95, 1867–1897. doi:10.1007/s00204-021-03034-y
Portilho, L., and Leonardi, G. R. (2020). The Real protection of Facial Sunscreens. Br. J. Dermatol. 182, 1050–1052. doi:10.1111/bjd.18628
Posthuma, L., Zijp, M. C., De Zwart, D., Van de Meent, D., Globevnik, L., Koprivsek, M., et al. (2020). Chemical Pollution Imposes Limitations to the Ecological Status of European Surface Waters. Sci. Rep. 10, 14825. doi:10.1038/s41598-020-71537-2
Predale, R. A., Sharples, R., and Saxe, J. (2017). “Method for Improving the Environmental Impact of Formulated Products,”. US9595012B2 (United States: Johnson and Johnson Consumer Companies LLC).
Ramos, S., Homem, V., Alves, A., and Santos, L. (2016). A Review of Organic UV-Filters in Wastewater Treatment Plants. Environ. Int. 86, 24–44. doi:10.1016/j.envint.2015.10.004
Reichrath, J. (2007). Sunlight, Skin Cancer and Vitamin D: What Are the Conclusions of Recent Findings that protection against Solar Ultraviolet (UV) Radiation Causes 25-hydroxyvitamin D Deficiency in Solid Organ-Transplant Recipients, Xeroderma Pigmentosum, and Other Risk Groups? J. Steroid Biochem. Mol. Biol. 103, 664–667. doi:10.1016/j.jsbmb.2006.12.029
Sabzevari, N., Qiblawi, S., Norton, S. A., and Fivenson, D. (2021). Sunscreens: UV Filters to Protect Us: Part 1: Changing Regulations and Choices for Optimal Sun protection. Int. J. Women's Dermatol. 7, 28–44. doi:10.1016/j.ijwd.2020.05.017
Schlumpf, M., Kypke, K., Wittassek, M., Angerer, J., Mascher, H., Mascher, D., et al. (2010). Exposure Patterns of UV Filters, Fragrances, Parabens, Phthalates, Organochlor Pesticides, PBDEs, and PCBs in Human Milk: Correlation of UV Filters with Use of Cosmetics. Chemosphere 81, 1171–1183. doi:10.1016/j.chemosphere.2010.09.079
Schneider, S. L., and Lim, H. W. (2019). Review of Environmental Effects of Oxybenzone and Other Sunscreen Active Ingredients. J. Am. Acad. Dermatol. 80, 266–271. doi:10.1016/j.jaad.2018.06.033
Tashiro, Y., and Kameda, Y. (2013). Concentration of Organic Sun-Blocking Agents in Seawater of Beaches and Coral Reefs of Okinawa Island, Japan. Mar. Pollut. Bull. 77, 333–340. doi:10.1016/j.marpolbul.2013.09.013
Vollhardt, J., and Marchini, M. (2021). What Are the Options Beyond SPF 50+? A View on Consumer Behavior and the Sensory Features of Sunscreens. Curr. Probl. Dermatol. 55, 112–123. doi:10.1159/000517656
Watkins, Y. S. D., and Sallach, J. B. (2021). Investigating the Exposure and Impacts of Chemical UV-Filters in Coral Reef Ecosystems: Review and Research gap Prioritisation. Integrated Environ. Assess. Manag. 17 (5), 967–981. doi:10.1002/ieam.4411
Keywords: eco-friendly, market benchmark, persistence-bioaccumulation-toxicity approach, environmental impact, UV-filter
Citation: Kunze G, Schlifke A, Jackson E, Hefner N, Berg K and Vollhardt J (2021) A Novel, Benchmark-Centered, Eco-Impact Rating System for Sunscreens and Sunscreen Formulation Design. Front. Environ. Sci. 9:727404. doi: 10.3389/fenvs.2021.727404
Received: 22 June 2021; Accepted: 28 October 2021;
Published: 09 December 2021.
Edited by:
Anabela Cachada, University of Porto, PortugalReviewed by:
Adela Jing Li, South China Agricultural University, ChinaAndré Rolim Baby, University of São Paulo, Brazil
Riccardo Catalano, Aix-Marseille Université, France
Copyright © 2021 Kunze, Schlifke, Jackson, Hefner, Berg and Vollhardt. This is an open-access article distributed under the terms of the Creative Commons Attribution License (CC BY). The use, distribution or reproduction in other forums is permitted, provided the original author(s) and the copyright owner(s) are credited and that the original publication in this journal is cited, in accordance with accepted academic practice. No use, distribution or reproduction is permitted which does not comply with these terms.
*Correspondence: Gernot Kunze, gernot.kunze@dsm.com
†These authors have contributed equally to this work