- 1Institute of Environmental Science and Technology, Tra Vinh, Vietnam
- 2Centre of Excellence for Innovation and Technology for Water Treatment, Naresuan University, Phitsanulok, Thailand
- 3Department of Academic Affairs and Testing, Dong Nai Technology University, Dong Nai, Vietnam
- 4Department of Hydraulic Engineering, College of Technology, Can Tho University, Can Tho, Vietnam
- 5Department of Chemistry, Faculty of Science, Naresuan University, Phitsanulok, Thailand
- 6Department of Industrial Engineering, Faculty of Engineering, Naresuan University, Phitsanulok, Thailand
- 7Department of Civil Engineering, Faculty of Engineering, Naresuan University, Phitsanulok, Thailand
Agitation was a significant factor in achieving the high performance of the electrocoagulation (EC) system. Three EC systems with four parellal monopolar Al electrodes were established to clarify the influence of agitation methods on pollutants removal efficiency; magnetic stirring, continuous aeration, and combination of magnetic stirring and aeration. The aim of this work was to maximize industrial wastewater treatment in a short detention time and to understand the mechanisms that occurred in different EC systems. The coolant wastewater from the aluminum product industry was represented as industrial wastewater. The hybrid stirring-aeration EC system obtained a lower COD removal compared to the stirring EC system and the aeration EC system. Although aeration can cause an increase in COD removal due to complete circulation and effective coagulant formation of Fe (OH), however, the combination of aeration and stirring negatively affected the performance of CE. The possible reason was that the excessive agitation led to a rapid mixing of the solution, and then the coagulants and pollutants obtained insufficient time to form flocs to precipitate. The best EC performance was observed in the aeration EC system, followed by the stirring EC system, control system (without agitations), and the stirring aeration EC system, respectively, in the short detention time of 15 min. Furthermore, all EC systems could achieve an excellent COD removal of 91% when the detention time was sufficient (eg, 45 min for the stirring aeration EC system). Furthermore, the decreasing number of electrodes affected the COD removal efficiency, whereas the NaCl additive was insignificantly affected.
Introduction
Coolant is a form of industrial waste and wastewater, and it is widely used in various metal-working industries for cooling systems and lubricating the interfaces of machines and workpieces. Its significant characteristic is oily wastewater containing high nonbiodegradable organic content (as represented in a low biodegradable index), high turbidity, and some levels of metals (Hilal et al., 2004; Yu et al., 2017). Coolant waste and wastewater are considered hazardous and require special treatment and disposal. Due to waste management in Thailand, coolant wastewater is separated from other industrial wastewaters which can be treated by industrial treatment plants. Coolant wastewater is stored and sent to a private waste disposal service for further treatment and disposal. Meanwhile, illegal disposal of coolant waste and wastewater into the environment has been observed (Marfe and Di Stefano, 2016).
The electrocoagulation (EC) process is known as one of the outstanding techniques with many advantages, such as high efficiency in a short treatment time, simplicity of design and operation, mild condition for treatment, and low cost of material and operation (Coimbra et al., 2021; Lucakova et al., 2021). The CE process was widely utilized to treat various types of industrial wastewater, such as tannery wastewater (Azarian et al., 2018a; Eryuruk et al., 2018), poultry slaughterhouse and egg processing wastewater (Godini, 2012; Azarian et al., 2018a; Azarian et al., 2018b; Rahmani et al., 2018; Ehsani et al., 2020), and crude vegetable oil refinery wastewater (Preethi et al., 2020), Due to the conceptual EC process, iron (Fe) or aluminum (Al) was introduced as electrodes to generate iron hydroxide or aluminum hydroxide flocs [that is, Fe (OH) 2, Fe (OH) 3, Al (OH)3] to destabilize pollutants and allow them to coagulate. However, a better performance of the EC process, including a techno-economic trade-off, was observed in the system combining Fe and Al as electrodes rather than the Fe/Fe and Al/Al systems (Chezeau et al., 2020; Kobya et al., 2014).
In addition, several other factors were mentioned as key factors influencing the EC performance and treatment efficiency, such as electrode number and supporting electrolyte type and concentration. The increase in the number of electrodes from two to six improved the performance of EC, while it also increased the energy consumption during the EC process (Gusa et al., 2020). Among the most favorable supporting electrolyte of NaCl, Na2SO4, NaNO3, and HClO4 (Yıldız et al., 2008; Rahmani et al., 2018), the NaCl significantly achieved the highest pollutants removal rate. Recently, external air addition has been reported to enhance CE performance for organic content (as presented in COD) and arsenite removals (Kumar et al., 2018; Syam Babu et al., 2021; Akansha et al., 2020), due to the presence of adequate dissolved oxygen necessary for the conversion of pollutants and chemical reactions. However, the concept of the hybrid electrochemical reaction and aeration system to improve wastewater treatment efficiency was unclearly explained.
The objective of this present work was to clarify the pollutants removal that occurred in the hybrid stirring-aeration EC system using Fe as anode and Al as cathode. The performance of the hybrid system to treat the wastewater from the coolant was compared with that of the traditional EC system using magnetic stirring and the alternative system using aeration. Moreover, the other operating factors, including electrode number and salt additive, were simply discussed.
Methodology
Wastewater
The actual coolant wastewater was collected from an aluminum product industry, located in Amata City Chonburi (Thailand). The appearance of the wastewater was a light white solution and slightly suspended, and its characteristics were listed as the following; pH of 6, 1,333 mg/L of total suspended solids, 56,400 mg/L of chemical oxygen demand (COD), 16,800 mg/L of biochemical oxygen demand (BOD), and 19,600 mg/L of oil and grease. The coolant wastewater without any pretreatments and pH adjustments was used in this work.
Electrocoagulation System
In the present work, the EC reactor was made of acrylic material with a dimension of 8 cm (length) x 7.5 cm (width) x 15 cm (height). The Fe and Al plates were used as anode and cathode, respectively, with a size of 7.5 cm (width) x 10 cm (height) x 0.1 cm (thickness). The electrodes were dipped in the solution to maintain an effective area of 45 cm2 per electrode and the interelectrode distance between electrodes was controlled at 1 cm. In the stirring EC system, the EC reactor was placed on a magnetic stirrer and stirred at ∼150 rpm which was the optimal stirring rate as suggested by Bayer et al. (2011). In the EC aeration system, two aquatic aerators were placed on the reactor base and aerated at ∼0.5 L/min. In the stirring-aeration EC system, both stirring and aeration were supplied to the reactor at ∼150 rpm and ∼0.5 L/min respectively. Another reactor was set up as a control system with no stirring and no aeration. For all EC systems, the electric current from the DC power supply was supplied to the electrodes to provide the current density of approximately 20 mA/cm2 which was suggested by Ehsani et al. (2020) and Pantorlawn et al. (2017).
Experimental Procedure
First, the coolant was mixed with 5 g/L of NaCl to increase the conductivity (Pantorlawn et al., 2017) before treating in different types of EC systems. Subsequently, the number of electrodes was reduced from four (2 Fe and 2 Al) to two (1 Fe and 1 Al) with the same distance between electrodes. Finally, the NaCl additive was varied from 1 to 10 g/L to optimize the conductivity of the wastewater and the efficiency of coolant treatment.
The performance of the EC system was identified by reduction of organic content, which was represented in the COD value. The COD measurement method corresponded to DIN ISO 15705 and was analogous to EPA 410.4, APHA 5220 D, and ASTM D1252-06 B. The COD solutions A + B were purchased from Sigma-Aldrich Canada Co. Ltd. The efficiency of COD removal was calculated as the following equation:
where COD0 was the initial concentration of COD in the coolant wastewater and CODt was the concentration of COD in the treated water at the detention time of t.
Results and Discussions
A significant factor for achieving wastewater treatment by the EC process was agitation to maintain a uniform condition and avoid the concentration gradient in the system. The EC system was normally agitated by a magnetic stirrer. From Figure 1, the traditional stirring EC system obtained a COD removal efficiency of 72.9%; the COD value was decreased from the initial 56,400 mg/L to 16,800 mg/L in 15 min of detention time. The COD removal efficiency was increased to a maximum of 91.0% in 45 min. The main reactions were that the iron cations were dissolved into ferrous ion (Fe2+) and/or ferric ion (Fe3+) from the sacrificial Fe electrode (anode), and hydroxide ion (OH-) occurred on the Al electrode (cathode) from water hydrolysis, and eventually coagulants of Fe(OH)2 and/or Fe(OH)3 were formed (as explained in equations Eqs. 2–6) (Ghanbari et al., 2014; Hakizimana et al., 2017). The organic content in the coolant wastewater was removed through destabilization, coagulation, and sorption processes by Fe (OH) 2 or Fe(OH)3. The dispersion of pollutants in the stirring EC system is illustrated in Figure 2A. As a result of the agitation, the pollutants were mainly exiting in the electric field, which was a reactive area for the electrocoagulation process.
The aeration method was another widely used agitation method in a biological treatment system and was applied in the EC aeration system. The COD removal efficiency of the EC aeration system reached the maximum efficiency of 90% in 15 min of detention time. The explanation was that aeration provided excellent homogeneous conditions and also served as an oxygen source, which caused an improving oxidation reaction in the system. Aeration initiated sufficient dissolved oxygen for converting generated Fe2+ into Fe3+ (Kumar et al., 2018). Since Fe(OH)3 was a more effective coagulant than Fe(OH)2 (Naje et al., 2017), therefore the performance of the EC aeration system was greater than that of the EC stirring system. In addition, continuous aeration can improve the EC performance through vertical movement and complete circulation between the electric field and the external electric field. Figure 2B shows that air was diffused along the system and pollutants were easily separated to the surface due to air bubbles.
In addition, the control system without stirring and without aeration achieved a lower COD removal efficiency of 63.2% in 15 min of detention time. Only pollutants existing in the electric field was coagulated and removed (Figure 2D). During treatment, the pollutants were slowly transported to the electric field by H2 gas generated at the cathode. Consequently, complete circulation occurred in the control EC system, as indicated by the high COD removal of 90.6% in 45 min of detention time. It should be noted that the homogenous mixture can occur under no agitation in the small-scale system.
The stirring-aeration EC system was established with the aim of enhancing the performance of the EC system by double stirring and aeration; the stirring speed and aeration rate were the same values as the stirring EC system and the aeration EC system, respectively. However, a relatively low COD removal was observed in 15 min; its efficiency was worse than the control EC system. Double agitations led to a rapid mixing of the solution; then coagulants and pollutants obtained insufficient time in the electric filing to form flocs, as illustrated in Figure 2C. However, the performance of the stirring-aeration EC system can reach a similar maximal COD removal efficiency of 90.5% in the longer detention time of 45 min. Although the floc formation of coagulants and pollutants was reduced, the EC performance can be recovered eventually by increasing the collision of coagulants and pollutants due to the extended detention time. Meanwhile, the rapid mixing solution affected the consumption of electricity from ions transfer (Ilhan et al., 2008). It should be noted that the stirring-aeration EC system was operated under a specific speed of stirring and a specific air flow rate. Therefore, the performance of the stirring-aeration EC system is possibly increased when the stirring speed and air flow rate were decreased to an optimal combination; coagulants and pollutants were perfectly dispersed and collided in the solution (Bayar et al., 2011). The optimum of the aeration and stirring values should be further studied.
The stirring EC system was continuously operated at a decreasing electrode number of two in order to reduce the operating cost and electric consumption. From Figure 3, the stirring EC system with two electrodes obtained the lower COD removal efficiency in 15–30 min rather than that with four electrodes; ranging 27–50% for the EC system of two electrodes and 73–84% for the EC system of four electrodes. Since the distance between the electrodes of both systems was unchanged, there was no effect of electrostatic force to degrade the flocs (very short distance between the electrodes and strong electrostatic force) or develop the lower flocs (long distance between the electrodes and weak electrostatic force) (Naje et al., 2017). The lower COD removal efficiency was due to the decrease in the electric field which referred to the decrease in Fe (OH) 2 and flocs formation. When sufficient flocs were produced, the organic compounds containing in the coolant wastewater were effectively removed, as illustrated by 90% of the COD removal in 45 min. The number of electrodes was negatively correlated with the operating time of the EC system. The supplied electrical current could be lowered by decreasing the number of electrodes (under constant current density condition); however, the operating cost was increased by extending the detention times to achieve the excellent EC performance and wastewater treatment efficiency.
The maximum efficiency of COD removal in this study was found in the longer detention time of 45 min rather than in the literature of 20–30 min (Azarian et al., 2018a; Ehsani et al., 2020). The main reason was related to a different source of wastewater. The coolant wastewater that contains high oil and grease and nonbiodegradable organic carbon. Therefore, it might take longer to degrade the large complex molecule to smaller compounds, which are easier to coagulate. An increasing biodegradability index (BOD5/COD) and a stable COD concentration were found during the CE process in the previous study (Pantorlawn et al., 2017). Other possible reasons were reactor design, electrode material, number of electrodes, and submerged area of electrode. When these parameters mentioned are kept in the best condition, the excellent treatment efficiency can be obtained at a short detention time, leading to an acceptable treatment cost and a practical system at the site.
In the stirring EC system, the pollutants removal efficiency and energy consumption were related to the solution conductivity due to the movement of generated ions and the flow of electric current. NaCl was one of the common salt additives to improve the solution conductivity. The increase in NaCl concentration was a direct function of conductivity, as presented in Figure 4. The stirring EC system with four electrodes was operated at various NaCl concentrations of 1–10 mg/L and 45 min of detention time. Coolant wastewater containing 1.4 mS/cm of conductivity obtained a COD removal efficiency of 52%. The COD removal efficiency was immediately to 91% after adding 1 g/L NaCl and achieving a conductivity of 3.1 mS/cm. At higher NaCl concentrations and conductivities, the COD removal efficiency was approximately 91%, which was the highest efficiency for coolant wastewater in this work.
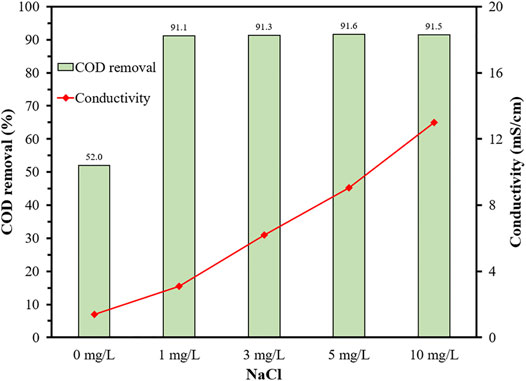
FIGURE 4. COD removal efficiency of the stirring EC system with different NaCl concentrations and conductivity values at 60 min of detention time.
In principle, the cell voltage decreases with an increase in conductivity at a constant current density; the total resistance in the solution decreases, and thus the electrical energy consumption decreases. However, excessive NaCl possibly caused an overconsumption of electrodes due to corrosion pitting and irregular electrode dissolution (Calvo et al., 2003). The optimized NaCl additive (e.g., <1 g/L) and the minimal detention time (e.g., <45 min) should be maintained for a cost-efficient coolant treatment using the CE process. Furthermore, metal ions were normally found in coolant waste and wastewater (Yeon and Moon, 2003; Teodosiu et al., 2014), the metal types and concentrations significantly influenced the solution conductivity as well as optimized NaCl additive. Therefore, it should be another concerning factor for different industrial wastewater.
Conclusion
The actual coolant wastewater was treated by EC systems with different agitations, stirring, aeration, and hybrid stirring and aeration. The aeration EC system achieved the maximal COD removal efficiency of 90% in a short detention time of 15 min, while the stirring EC system required 45 min of detention time. The significant reasons were that the complete circulation in the aeration EC system led to a homogeneous dispersion of coagulants and flocs, and dissolved oxygen also played a role in converting Fe2+ to Fe3+ and generated effective coagulant of Fe(OH)3. However, the combination of stirring and aeration could not provide the best performance in this work. In addition, the optimal stirring speed and aeration rate should be considered to further improve the EC process.
Data Availability Statement
The raw data supporting the conclusion of this article will be made available by the authors, without undue reservation.
Author Contributions
DT has performed experiment and data analysis. TT has did data analysis and revised manuscript. QB has did data analysis and revised manuscript. DC did experimental design, data analysis and wrote manuscript. AN design, data analysis, and wrote main manuscript. WK initiated the project, designed the experiment, did data analysis and wrote manuscript.
Conflict of Interest
The authors declare that the research was conducted in the absence of any commercial or financial relationships that could be construed as a potential conflict of interest.
Publisher’s Note
All claims expressed in this article are solely those of the authors and do not necessarily represent those of their affiliated organizations, or those of the publisher, the editors and the reviewers. Any product that may be evaluated in this article, or claim that may be made by its manufacturer, is not guaranteed or endorsed by the publisher.
Acknowledgments
The authors would like to thank Kairop and Milint Nakaruk for the donation of their living allowance; without their sacrifices, this work could not be done.
References
Akansha, J., Nidheesh, P. V., Gopinath, A., Anupama, K. V., and Suresh Kumar, M. (2020). Treatment of Dairy Industry Wastewater by Combined Aerated Electrocoagulation and Phytoremediation Process. Chemosphere. 253, 126652. doi:10.1016/j.chemosphere.2020.126652
Azarian, G., Miri, M., and Nematollahi, D. (2018a). Combined Electrocoagulation/Electrooxidation Process for the COD Removal and Recovery of Tannery Industry Wastewater. Environ. Prog. Sustainable Energ. 37 (2), 637–644. doi:10.1002/ep.12711
Azarian, G., Rahmani, A. R., Masoudi khoram, M., Atashzaban, Z., and Nematollahi, D. (2018b). New Batch Electro-Coagulation Process for Treatment and Recovery of High Organic Load and Low Volume Egg Processing Industry Wastewater. Process Saf. Environ. Prot. 119, 96–103. doi:10.1016/j.psep.2018.07.025
Bayar, S., Yıldız, Y. Ş., Yılmaz, A. E., and İrdemez, Ş. (2011). The Effect of Stirring Speed and Current Density on Removal Efficiency of Poultry Slaughterhouse Wastewater by Electrocoagulation Method. Desalination. 280 (1), 103–107. doi:10.1016/j.desal.2011.06.061
Calvo, L. S., Leclerc, J.-P., Tanguy, G., Cames, M. C., Paternotte, G., Valentin, G., et al. (2003). An Electrocoagulation Unit for the Purification of Soluble Oil Wastes of High COD. Environ. Prog. 22 (1), 57–65. doi:10.1002/ep.670220117
Chezeau, B., Boudriche, L., Vial, C., and Boudjemaa, A. (2020). Treatment of Dairy Wastewater by Electrocoagulation Process: Advantages of Combined Iron/Aluminum Electrodes. Separation Sci. Technology. 55 (14), 2510–2527. doi:10.1080/01496395.2019.1638935
Coimbra, E. C. L., Mounteer, A. H., do Carmo, A. L. V., Michielsen, M. J. F., Tótola, L. A., Guerino, J. P. F., et al. (2021). Electrocoagulation of Kraft Pulp Bleaching Filtrates to Improve Biotreatability. Process Saf. Environ. Prot. 147, 346–355. doi:10.1016/j.psep.2020.09.039
Ehsani, H., Mehrdadi, N., Asadollahfardi, G., Bidhendi, G. N., and Azarian, G. (2020). A New Combined Electrocoagulation-Electroflotation Process for Pretreatment of Synthetic and Real Moquette-Manufacturing Industry Wastewater: Optimization of Operating Conditions. J. Environ. Chem. Eng. 8 (5), 104263. doi:10.1016/j.jece.2020.104263
Eryuruk, K., Tezcan, Un., and Bakır Ogutveren, U. (2018). Electrochemical Treatment of Wastewaters from Poultry Slaughtering and Processing by Using Iron Electrodes. J. Clean. Prod. 172, 1089–1095. doi:10.1016/j.jclepro.2017.10.254
Ghanbari, F., Moradi, M., Eslami, A., and Emamjomeh, M. M. (2014). Electrocoagulation/Flotation of Textile Wastewater With Simultaneous Application of Aluminum and Iron as Anode. Environ. Process. 1 (4), 447–457. doi:10.1007/s40710-014-0029-3
Godini, K, A. G., Nematollahi, D., Rahmani, A. R., and Zolghadrnasab, H. (2012). Electrochemical Treatment of Poultry Slaughterhouse Wastewater Using Iron and Aluminium Electrodes. Res. J. Chem. Environ. 16 (3), 98–103.
Gusa, R. F., Sari, D. N., Afriani, F., Sunanda, W., and Tiandho, Y. (2020). Effect of Electrode Numbers in Electrocoagulation of Batik Cual Wastewater: Analysis on Water Quality and Energy Used. IOP Conf. Ser. Earth Environ. Sci. 599, 012061. doi:10.1088/1755-1315/599/1/012061
Hakizimana, J. N., Gourich, B., Chafi, M., Stiriba, Y., Vial, C., Drogui, P., et al. (2017). Electrocoagulation Process in Water Treatment: A Review of Electrocoagulation Modeling Approaches. Desalination. 404, 1–21. doi:10.1016/j.desal.2016.10.011
Hilal, N., Busca, G., Talens-Alesson, F., and Atkin, B. P. (2004). Treatment of Waste Coolants by Coagulation and Membrane Filtration. Chem. Eng. Process. Process Intensification. 43 (7), 811–821. doi:10.1016/s0255-2701(03)00099-0
Ilhan, F., Kurt, U., Apaydin, O., and Gonullu, M. T. (2008). Treatment of Leachate by Electrocoagulation Using Aluminum and Iron Electrodes. J. Hazard. Mater. 154 (1), 381–389. doi:10.1016/j.jhazmat.2007.10.035
Kobya, M., Akyol, A., Demirbas, E., and Oncel, M. S. (2014). Removal of Arsenic From Drinking Water by Batch and Continuous Electrocoagulation Processes Using Hybrid Al-Fe Plate Electrodes. Environ. Prog. Sustainable Energ. 33 (1), 131–140. doi:10.1002/ep.11765
Kumar, A., Nidheesh, P. V., and Suresh Kumar, M. (2018). Composite Wastewater Treatment by Aerated Electrocoagulation and Modified Peroxi-Coagulation Processes. Chemosphere. 205, 587–593. doi:10.1016/j.chemosphere.2018.04.141
Lucakova, S., Branyikova, I., Kovacikova, S., Pivokonsky, M., Filipenska, M., Branyik, T., et al. (2021). Electrocoagulation Reduces Harvesting Costs for Microalgae. Bioresour. Technology. 323, 124606. doi:10.1016/j.biortech.2020.124606
Marfe, G., and Di Stefano, C. (2016). The Evidence of Toxic Wastes Dumping in Campania, Italy. Crit. Rev. Oncology/Hematology. 105, 84–91. doi:10.1016/j.critrevonc.2016.05.007
Naje, A. S., Chelliapan, S., Zakaria, Z., Ajeel, M. A., and Alaba, P. A. (2017). A Review of Electrocoagulation Technology for the Treatment of Textile Wastewater. Rev. Chem. Eng. 33 (3), 263–292. doi:10.1515/revce-2016-0019
Pantorlawn, W., Threrujirapapong, T., Khanitchaidecha, W., Channei, D., and Nakaruk, A. (2017). Electrocoagulation for Spent Coolant From Machinery Industry. J. Water Reuse Desalination. 8 (4), 497–506. doi:10.2166/wrd.2017.057
Preethi, V., Ramesh, S. T., Gandhimathi, R., and Nidheesh, P. V. (2020). Optimization of Batch Electrocoagulation Process Using Box-Behnken Experimental Design for the Treatment of Crude Vegetable Oil Refinery Wastewater. J. Dispersion Sci. Technology. 41 (4), 592–599. doi:10.1080/01932691.2019.1595640
Rahmani, A. R., Nematollahi, D., Samarghandi, M. R., Samadi, M. T., and Azarian, G. (2018). A Central Composite Design to Optimize In-Situ Electrochemically Produced Ozone for Removal of Reactive Red 198. J. Electrochem. Soc. 165 (3), E121–E128. doi:10.1149/2.0201803jes
Syam Babu, D., Nidheesh, P. V., and Suresh Kumar, M. (2021). Arsenite Removal From Aqueous Solution by Aerated Iron Electrocoagulation Process. Separation Sci. Technology. 56 (1), 184–193. doi:10.1080/01496395.2019.1708932
Teodosiu, C., Wenkert, R., Tofan, L., and Paduraru, C. (2014). Advances in Preconcentration/Removal of Environmentally Relevant Heavy Metal Ions From Water and Wastewater by Sorbents Based on Polyurethane Foam. Rev. Chem. Eng. 30 (4), 403–420. doi:10.1515/revce-2013-0036
Yeon, K.-H., and Moon, S.-H. (2003). A Study on Removal of Cobalt from a Primary Coolant by Continuous Electrodeionization With Various Conducting Spacers. Separation Sci. Technology. 38 (10), 2347–2371. doi:10.1081/ss-120021628
Yu, L., Han, M., and He, F. (2017). A Review of Treating Oily Wastewater. Arabian J. Chem. 10, S1913–S1922. doi:10.1016/j.arabjc.2013.07.020
Keywords: electrocoagulation, coolant wastewater, Fe-Al electrodes, stirring, aeration
Citation: Trinh DTT, Binh QA, Ty TV, Channei D, Nakaruk A and Khanitchaidecha W (2021) Evaluation of Magnetic Stirring and Aeration on Electrocoagulation Performance in Actual Industrial Treatment. Front. Environ. Sci. 9:719248. doi: 10.3389/fenvs.2021.719248
Received: 02 June 2021; Accepted: 08 July 2021;
Published: 16 August 2021.
Edited by:
Paula Oulego, University of Oviedo, SpainReviewed by:
Ghasem Azarian, Hamadan University of Medical Sciences, IranAitbara Adel, University of El-Tarf, Algeria
Copyright © 2021 Trinh, Binh, Ty, Channei, Nakaruk and Khanitchaidecha. This is an open-access article distributed under the terms of the Creative Commons Attribution License (CC BY). The use, distribution or reproduction in other forums is permitted, provided the original author(s) and the copyright owner(s) are credited and that the original publication in this journal is cited, in accordance with accepted academic practice. No use, distribution or reproduction is permitted which does not comply with these terms.
*Correspondence: Wilawan Khanitchaidecha, wilawank1@gmail.com