- Department of Civil and Environmental Engineering, Yonsei University, Seoul, South Korea
The increasing cancer risk (CR) due to sediment- and soil-bound polycyclic aromatic hydrocarbons (PAHs) is one of the major threats to public health. The CR of sediment- and soil-bound carcinogenic PAHs was estimated for the first time in coastal and residential areas near an industrial zone in Korea. Monte Carlo probabilistic simulations and sensitivity tests were conducted to calculate the CR and to identify the most sensitive parameters. The CR was found to be highest in the coastal areas of Korea. Ulsan, which is located on the southeastern coast of Korea, was classified as a high cancer risk zone according to United States Environmental Protection Agency standards, while the western coast and Mohang Harbor were classified as moderate cancer risk zones. Fish consumption was identified as the main contributor (94–99%) to the total risk levels in the coastal areas. The biota-to-sediment accumulation factor (43–76%) and PAH levels (8–44%) in sediment were identified as the parameters that were most sensitive to the CR. In the residential area, the CR was found to be within the range of 10–6–10–4, which categorized it as a low cancer risk zone. Furthermore, the CR for residents in the industrial area was estimated to be 12 and 5 times higher than that for residents in the rural and urban areas, respectively. The exposure duration (55–85%) and skin adherence factor for soil (35–42%) were identified as the most sensitive parameters for the overall CR in the residential area. Korea generally has high fish and seafood consumption, which has been recognized as the most significant exposure route for CR in the studied coastal areas. Thus, the consumption of fish and seafood from coastal areas, especially those near Ulsan, might be responsible for the increasing number of cancer patients in Korea.
Highlights
Fish/seafood consumption may be associated with cancer incidence rates in Korea
The Ulsan industrial area is recognized as a high-risk cancer zone in Korea
The parameters BSAF, ED, and AF were identified as the most sensitive for cancer risk levels.
Introduction
Anthropogenic sources such as fossil fuel combustion, industrial pyrolysis, vehicular exhaust emissions, the excessive use of synthetic fertilizers for agriculture, and the reuse of sewage sludge are primarily responsible for the increasing levels of polycyclic aromatic hydrocarbons (PAHs) in soil environments (Zhang et al., 2015). Similarly, oil tank spills, offshore drilling, runoff from industrial and urban areas, natural seeps, and creosoted piling are the key sources of PAHs in sediment (Singare, 2015). In the environment, the adsorption of PAHs onto organic components in the soil and sediment is rapid due to their stable chemical structure and hydrophobicity (Abdel-Shafy and Mansour, 2016). The direct and indirect exposure to soils and sediments containing PAHs are thus also a route for accumulation in the human body. PAHs are well-known for their carcinogenic and mutagenic properties due to the presence of fused aromatic rings (USEPA, 2003a). Long- and short-term exposure to PAHs has been associated with the development of cancer tumors in the human reproductive, neurological, hematopoietic, and immune systems (Armstrong et al., 2004; IARC, 2010). Moreover, mutations of cells in the urinary tract, lung, liver, and skin can also occur from direct or indirect exposure of occupational source of carcinogenic PAHs (Petry et al., 1996; Boffetta et al., 1997; Bosetti et al., 2007; Roy et al., 2017; Roy et al., 2019c).
Exposure through ingestion, inhalation, and dermal absorption are the three routes by which PAHs in the soil enter the human body. The accumulation of soil-bound PAHs in food, the erosion of soil particles followed by inhalation, and the deposition onto the skin and subsequent absorption are particularly main mechanisms behind the three routes. In addition, toxic PAHs in the sediment can bioaccumulate in the lipids of fish through the food chain. The subsequent ingestion of fish and seafood transfers these PAHs into the human bloodstream. Additionally, human water-based activity such as swimming can lead to the dermal absorption of sediment-bound PAHs.
Monte Carlo simulations are used to analyze probabilistic uncertainty. Monte Carlo probabilistic simulations combined with sensitivity analysis are commonly employed to estimate human health risks (Roy et al., 2019a, b, c, d; Roy et al., 2020; Tarafdar and Sinha, 2017a; Chawda et al., 2020). The parameter values for exposure equations are randomly selected from distributions generated from the probability density functions for individual input parameters. The role of these parameters in determining the total health risk can be established using sensitivity analysis.
The South Korean peninsula is surrounded on three sides by the ocean. Coastal areas thus play an important role in the country’s land-use patterns and economy. Korea has a high fish and seafood consumption, with an average of 55 kg/capita per year (FAO, 2020), and the production of fish and seafood in coastal areas is a major economic activity in Korea. In Korea, the increasing levels of PAHs detected in sediment and soil requires attention. Previous studies have focused on the monitoring and distribution of PAHs in agricultural soil, source identification of toxic PAHs, PAH levels in soils in rural, urban, and industrial areas, organic carbon and PAH levels in sediment in coastal areas, and PAH levels in coastal fish. Recently, the CR for roadside soil in traffic-dense regions was investigated (Kim et al., 2019). However, an exhaustive literature review shows that there has been a lack of detailed studies and sensitivity analyses of the CR for sediment-and soil-bound PAHs in coastal and residential areas on the Korean peninsula. The present study has designed to fill this gap by comparing the CR of sediment-and soil-bound PAHs in coastal regions and rural, urban, and industrial residential areas near an industrial cluster in Korea and identifying the parameters that are most sensitive to this CR and the most common PAH exposure routes.
Methodology
The present study focused on assessing the CR for sediment-and soil-bound PAHs in coastal and residential areas near an industrial cluster in Korea. Previously published data on soil- and sediment-bound PAHs were acquired to calculate the CR. The concentration of 16 potentially carcinogenic PAHs (Naphthalene (NaP), Acenaphthylene (Acy), Acenaphthene (Ace), Fluorene (Flu), Phenanthrene (Phe), Anthracene (Ant), Fluoranthene (Flt), Pyrene (Pyr), benzo [a] anthracene (BaA), Chrysene (Chr), Benzo [b] fluoranthene (BbF), Benzo [k] fluoranthene (BkF), Benzo [a] pyrene (BaP), Indeno [1,2,3-c,d] pyrene (IcdP), Dibenz [a,h] anthrathene (DBahA), and Benzo [g,h,i]perylene (BghiP)) were considered in the present study. A description of the study area and the sampling methods are provided in Table 1.
Description of the Study Area and Data Collection
In the present study, sediment samples were collected from the southeastern and western coasts of the peninsula. On the western coast, samples were collected from Mohang Harbor (six sites: mh1, mh2, mh3, mh4, mh5, and mh6), Lake Sihwa (LS1), Lake Asan (LS2), Lake Sapgyo (LS3), the Geun River estuary (RS1), and the Yeongsan River estuary (RS2). On the southeastern coast, sediment samples were collected from 17 sites (S1–S17) in Ulsan Bay and Taehwa River (Figure 1). Sediment-bound PAH and total organic carbon levels were taken from previous studies (Kim et al., 2014; Sim et al., 2018; Kim et al., 2020; Seo et al., 2020).
The grab sampling method was used to collect bottom sediment from Mohang Harbor, in Ulsan, and on the western coast. The sediment samples were freeze-dried in sealed glass jars with a Teflon-lined cap and stored until analysis. Acetone, sodium hydroxide, anhydrous sodium sulfate, ethanol, and n-hexane at the required specifications were used as reagents to extract the PAHs from the sediment samples. Soxhlet extraction and clean-up using silica gel columns were conducted to prepare PAH solutions for analysis. The extracted PAH solutions were analyzed using gas chromatography–mass spectrometry (GC-MS) after calibrating the instrument using standard solutions for the target compounds. In addition, the total organic carbon in the dried sediment samples was analyzed after removing the carbonates with 1M HCL using an elemental analyzer (Thermo Flash EA 1112 series, United Kingdom).
Soil samples were collected from residential areas near Ulsan and industrial zone. The residential areas were classified as rural, urban, or industrial. Soil-bound PAH data were taken from Kwon and Choi (2014). In total, 10, 10, and five soil samples were collected for the industrial, urban, and rural areas, respectively. Polyethylene bags were used to collect and store the soil samples in a refrigerator at −4 °C. Soxhlet extraction was conducted after mixing the homogenized soil samples with anhydrous sodium sulfate. The extracted samples were dried in a nitrogen evaporator and cleaned up with a silica gel column. A GC–ion trap mass spectrometer (GC/ITMS) with a DB-5MS column was used to analyze PAH levels in the extracts after standardizing the instrument. Further details on the sampling method, sample preparation, analysis, instrumental conditions, and quality assurance/quality control (QA/QC) can be found in the studies summarized in Table 1.
Human Cancer Risk Assessment
The human CR from exposure of sediment-and soil-bound carcinogenic 16 PAHs were assessed. The relative potency equivalency factor (PEF) in relation to BaP was used to convert the concentration of each PAH into the BaP equivalent (BaPeq) (USEPA, 1993). In the present study, six-years-old children and 80-years-old adults were considered the target population at the study sites. Exposure through ingestion and dermal absorption was considered for sediment-bound PAHs, while exposure through ingestion, dermal absorption, and inhalation was considered for soil-bound PAHs in the residential areas. The values for the risk assessment parameters (e.g., HR, ED, BW, IRfish, and Flipid) followed the Korean standards given in Table 2. Other model parameters were assessed using the standard values reported by the USEPA (2011). For the sediment samples, the ingestion of toxic lipid-bound PAHs in fish and seafood was calculated using the biota-to-sediment accumulation factor (BSAF). The CR > 10–6 has been considered as a significant risk level (USEPA, 2003b).
The chronic daily intake (CDI) of PAHs in the soil was calculated using the following equations (USEPA, 1989; USEPA, 2004; USEPA, 2009):
where CDIing, CDIinh, and CDIderm were estimated in mgKg−1d−1. CSsoil (mg kg−1) is the BaPeq for all16 carcinogenic PAHs in the soil, IRds (mg d−1) is the ingestion rate of soil, ED (year) is the exposure duration, EF (d year−1) is the exposure frequency, BW (kg) is the standard body weight, AT (d year−1) is the averaging time, CF is the conversion factor, HR (m3 d−1) is the inhalation rate, PEFsoil is the emission factor for the soil particles, SA (cm2 d−1) is the exposed skin surface area (i.e., hands, head, and forearms), AF (mg cm−2) is the relative skin-to-soil adherence factor, and ABS is the dermal absorption fraction. The values for each parameter are given in Table 2.
The total concentration of all PAHs (ƩPAH) in fish was calculated from the BSAF as described by the USEPA (USEPA, 1989; Tarafdar and Sinha, 2017b). Exposure to sediment-bound PAHs through the ingestion of fish was calculated using the following equations:
where BSAF is the biota-to-sediment accumulation factor, CSfish (mg kg−1) is the BaPeq for ƩPAH in fish, flipid (g lipid/g wet weight) is the fraction of lipids in fish, CSsedi (µg kg−1 dry weight) is the BaPeq for ƩPAH in sediment, OC is the organic carbon fraction in sediment, and IRfish (kg d−1) is the fish intake rate. The values for each parameter are provided in Table 2.
The CR for individual exposure paths was calculated using the following equation (USEPA, 1989):
where x represents ingestion, dermal absorption, or inhalation, and CSF (mg kg−1 d−1) is the cancer slope factor for BaP.
The total CR for exposure to residential soils and coastal sediments was calculated using Eqs 7, 8, respectively (Chiang et al., 2009).
According to the USEPA, a CR of >10–6 is considered a significant CR (Wang et al., 2011; Li et al., 2014). The lifetime CR can be classified as very low (CR ≤ 10–6), low (10–6< CR < 10–4), moderate (10–4 ≤ CR < 10–3), high (10–3 ≤ CR < 10–1), and very high (CR ≥ 10–1) (NYSDOH, 2012). Monte Carlo simulations with 10,000 iterations were conducted using Oracle Crystal Ball (11.1.2.4.600) to calculate the CR. Sensitivity tests were conducted simultaneously to determine the effect of the input parameters on the overall CR.
Uncertainty Analysis
Determining CR is prone to uncertainty due to the variation in the health risk assessment parameters employed in the calculations. Values for the parameters HR, IRfish, ED, BW, and flipid were thus taken from Korea to minimize this uncertainty. Values for the BASF, which has been identified as the most sensitive parameter for risk level assessment in sediment samples, were taken from the USEPA database. Determining the BASF for Korean sediments was beyond the scope of the present study. There was also a possibility that the sample collection and analysis methods could have led to uncertainty in CR assessment; in particular, the loss or gain of PAHs in the cooking process was not considered in the present study. For the risk assessment analysis, Monte Carlo simulations with 10,000 iterations were used to reduce the uncertainty.
Results and Discussion
Concentration of Polycyclic Aromatic Hydrocarbons and Organic Carbon
The concentrations of sediment- and soil-bound PAHs exhibited a wide range in the present study. In the coastal areas, the highest sediment-bound PAH levels were found in Ulsan (0.704 ± 0.21 μg/g), followed by Mohang Harbor (0.194 ± 0.154 μg/g) and the western coast (0.053 ± 0.032 μg/g) (Table 3 and Supplementary Tables 1–3). The standard for sediment-bound PAHs is unpublished. The BaPeq of ƩPAH was also highest in Ulsan, followed by Mohang Harbor and the western coast, with mean levels of 0.055 μg/g, 0.016 μg/g, and 0.004 μg/g, respectively. The ƩPAH and the BaPeq of ƩPAH levels in the Ulsan sediment samples were thus found to be four and 14 times higher than those at Mohang Harbor and on the western coast, respectively.
As per the Environmental Systems Research Institute (ESRI) report, the shipping density and activities (especially oil tankers) around the South Korea are high (Supplementary Figure 1). The accidental or intentional oil spills and its deposition on the coastal sediments (especially on southern part) through hydrodynamic currents could be the possible source for elevated PAHs levels. Apart from the marine derived sources, atmospheric transportation from neighboring countries also could be the reason for the additional PAHs levels in the coastal sediments (Lee and Kim, 2007). The diagnostic ratio analysis results showed that the petrogenic (crude oil) as one of the major components in all costal sediment in South Korea (Supplementary Table 4). The petrogenic sources are the most viable at the Mohang Harbor. At West coast, petrogenic, coal, wood combustions have been identified as common sources. At Ulsan sediments, basically from coal and softwood combustion (industrial sources) have been recognized as a major source of PAHs along with the petrogenic sources. Additionally, the Ulsan coastal sediment was found to be particularly polluted with potentially carcinogenic PAHs when compared with other coastal regions in South Korea. Petrochemical industrial processes, vehicles, non-ferrous metal smelting, the natural spilling of oil, and shipbuilding are possible sources of PAHs in the coastal area in Ulsan. In Asian countries, high levels of ƩPAH have been reported in India (mean: 274.08 μg/g) and China (range: 0.79–10.47 μg/g) (Tarafdar and Sinha, 2017; Zhao et al., 2017). In Ulsan, Flt and Pyr were found to be the most common PAHs, with contribution levels of 19 and 18%, respectively. Of the other PAHs, BaP accounted for 54% of the total BaPeq in this area. Organic carbon is an important parameter for calculating the bioaccumulation of sediment-bound PAHs. The geometric mean sediment-bound organic carbon levels were estimated to be 0.010, 0.008, and 0.004 at Mohang Harbor, in Ulsan, and on the western coast, respectively.
The soil-bound ƩPAH concentration was highest in the industrial area (mean: 1.91 μg/g), followed by the urban (mean: 0.39 μg/g) and rural (mean: 0.22 μg/g) areas (Supplementary Table 4). Nap was the dominant PAH in the industrial, rural, and urban areas, with contributions of 18, 17, and 16%, respectively. BaPeq was also highest in the industrial area, followed by the urban and rural areas, with levels of 0.172 μg/g, 0.037 μg/g, and 0.015 μg/g, respectively. Moreover, BaP was identified as a most significant contributor to ΣPAH as BaPeq in the industrial, urban, and rural residential areas (58–71%). The ƩPAH levels in Ulsan were higher than those reported for other cities in Korea, Tarragona (Spain), Bangkok (Thailand), and Tokushima (Japan) (Jeon and Oh, 2019). The standard for soil-bound PAH levels are not reported for Korea. Previous studies have compared the PAH levels with Polish (0.02–0.05 μg/g), Mexican (0–6 μg/g), and Dutch (0.02–0.05 μg/g) standards. In the present study, PAH levels were classified using Polish standards, by which the rural and urban areas were classified as low-pollution zones, while the industrial area was classified as a highly polluted site.
Emissions from industrial activities have been suspected as the major sources of soil pollution in residential areas (Supplementary Table 5). In particular, diesel, gasoline, and heavy oil combustion have been identified as a major source of soil-bound PAHs (Jeon and Oh, 2019). Ulsan is the largest industrial city in Korea, containing petrochemical and automobile factories, non-ferrous metal smelting plants, refineries, shipbuilding complexes, and their vendors (Jeon and Oh, 2019).
Human Health Risk Levels
For the present study, the age-specific human exposure parameters ED, AF, BW, SA, EF, HR, and IRfish were considered to calculate the CR for sediment- and soil-bound PAHs. Ingestion (CDIing), inhalation (CDIinh), and dermal absorption (CDIderm) were assumed to be the primary exposure routes for soil, while CDIing for fish and CDIderm were measured for the sediment-bound PAHs. The ƩPAH concentration (BaPeq) was used as input for Monte Carlo simulations to calculate the 95% confidence levels for the CR for the child and adult age groups (Figures 2, 3). In this study, the Mohang Harbor, Ulsan, and western coastal areas were considered for the CR assessment. As shown in Figures 4, 5, the total CR for all three coastal areas was significant (>10–6) (USEPA, 2003; Wang et al., 2011; Li et a1., 2014). The Ulsan coastal area (KS2) had the highest CR of the individual sites (5.59E-03 and 1.60E-03 for children and adults, respectively). The total CR for the child group was highest at KS1 and KS3 (6.04E-04 and 2.47E-04, respectively). The total CR for the child group was three times higher than that for the adult group at both KS1 and KS2. Exposure through fish ingestion was identified as the most viable route for total CR (94–99%) in all of the coastal areas. The CRing levels were estimated to be 5.58E-03, 6.07E-04, and 2.47E-04 at KS2, KS1, and KS3, respectively. Exposure through dermal absorption was insignificant (<10–6) with a very low CR (CRderm ≥ 10–6) at KS1 and KS3 (coastal areas in the western region of Korea) following New York State Department of Health (NYS DOH) standards. However, significant CRderm levels were recorded in Ulsan (KS2), with a levels of 10–5 and 10–6 for children and adults, respectively, which classifies these sites as low-risk zones (10–6< CR < 10–4). Significant CR through dermal absorption has been reported for India (Tarafdar and Sinha, 2017). Total CR and CRing were classified as moderate (10–4 ≤ CR < 10–3) at KS1 and KS3. Ulsan, which is located in the southeastern region of Korea, was identified as a high cancer risk area (10–3 ≤ CR < 10–1) for total CR and CRing.
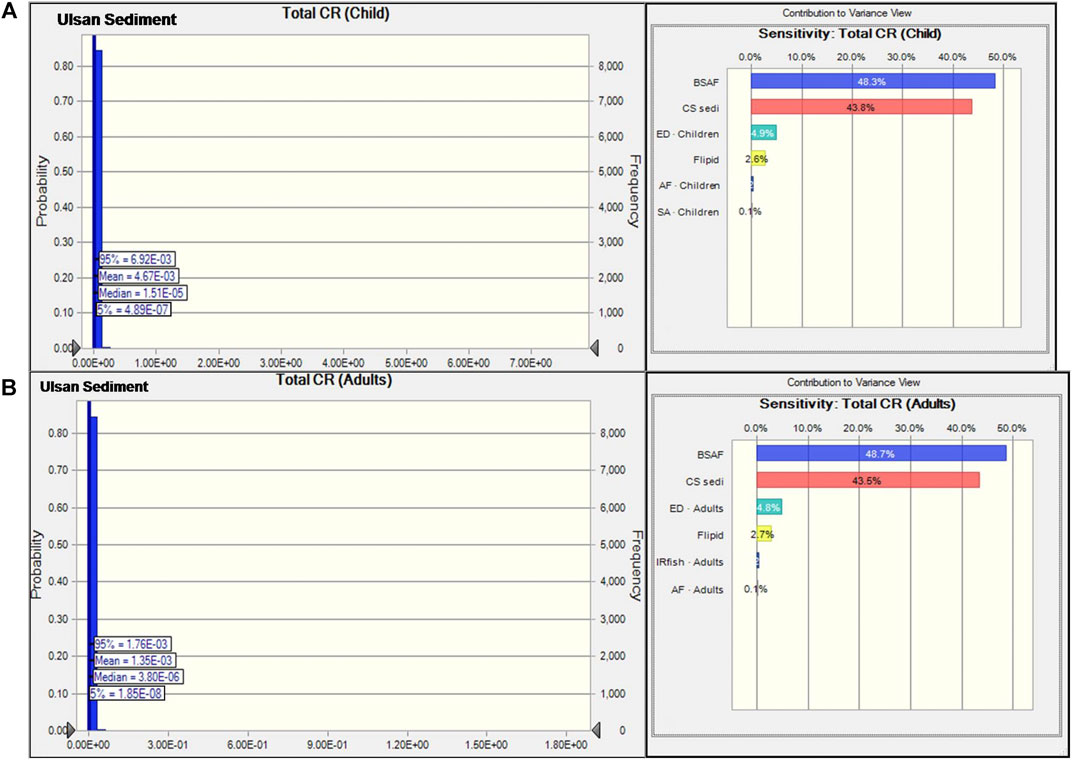
FIGURE 2. Probability density functions and sensitivity chart from Monte Carlo simulations used to predict the cancer risk from sediment-bound PAHs for children (A) and adults (B) in the Ulsan coastal area.
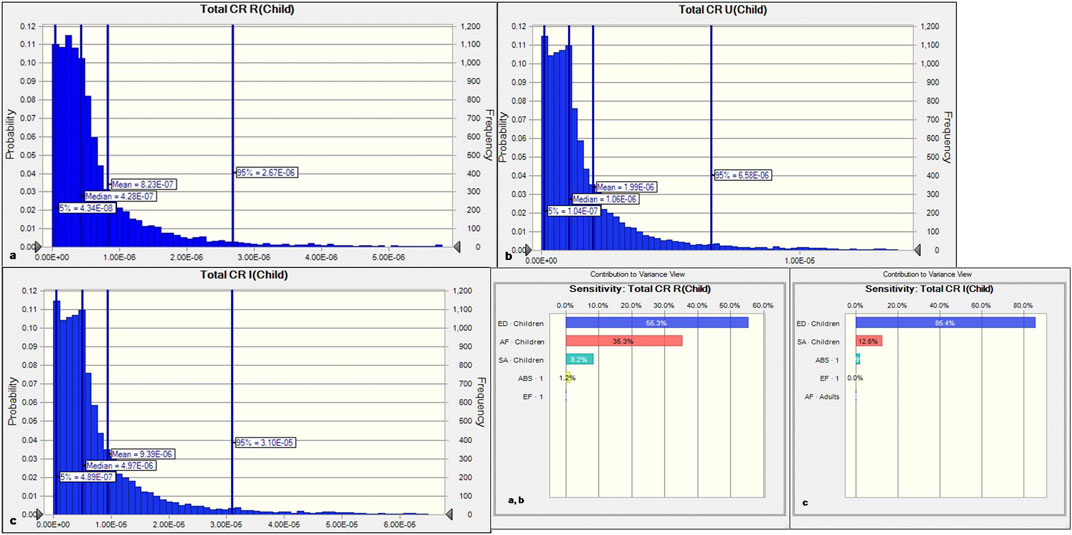
FIGURE 3. Probability density functions and sensitivity chart from Monte Carlo simulations used to predict the cancer risk from soil-bound PAHs for children (A) and adults (B) in Ulsan residential (C) areas.
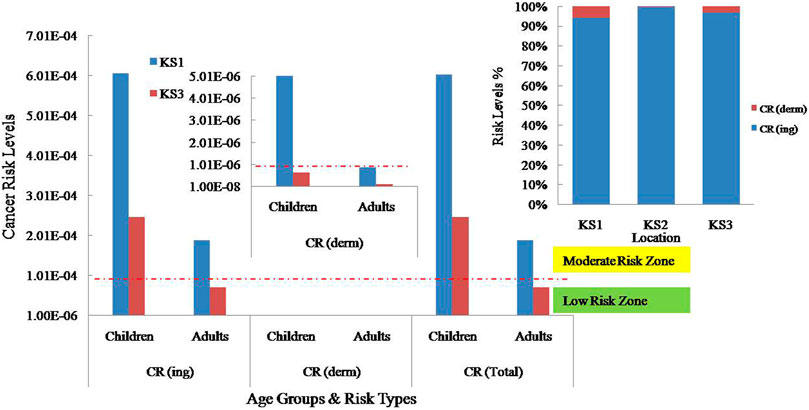
FIGURE 4. Cancer risk from sediment-bound PAHs at Mohang Harbor (KS1) and the western coastal area (KS3).
The CR for sediment-bound PAHs has been reported to be moderate in South Africa at Algoa Bay and the US at Midway Atoll (1 × 10–3 and 1 × 10–4 respectively) (Yang et al., 2014; Adeniji et al., 2019). In India, a very high CR in the range of 3.64E-02 and 7.21E-02 has been reported for children and adults, respectively (Tarafdar and Sinha, 2017). In these studies, food ingestion was identified as a major route for CR. The present study also identified fish ingestion in Ulsan coastal areas as a major source of CR. This location is the largest industrial belt in Korea. The Food and Agriculture Organization of the US has reported that South Korea has one of the highest fish consumption rates in the world at 55 kg/capita per year (FAO, 2020). Ocean fish consumption maybe one of the major reasons for the rising number of cancer cases in Korea. In the previous study, (Moon et al., 2002), collected fish/seafood samples from the local fish markets located at coastal areas in Korea to analyze the residue and 16 PAHs profile. The presence of significant amount of marine environment related PAHs in fish/seafood suggest strong accumulation of sediment-bound PAHs in seafood/fish. Moreover, natural oil spills, runoff from industrial areas, shipyard transportation, and other associated industrial activity maybe major sources of sediment-bound PAHs (Jeon and Oh, 2019).
The CR for soil-bound PAH levels was estimated for the rural, urban, and industrial areas (Figure 6). The highest total CR was found in the industrial area (3.10E-05), followed by the urban (6.58E-6) and rural (2.67E-06) areas. Of the three possible exposure routes, dermal absorption was significant for both age groups and at all locations (<10–6). The highest CRderm, CRing, and CRinh levels were 4.94E-06–2.76E-05, 4.71E-11–2.30E-10, and 4.66E-07–4.39E-06, respectively, in the industrial area. Exposure through ingestion was found to be significant (4.39E-06) for the child group in the industrial area. The CR was 6 times higher for children than for adults. In the rural and urban areas, the CR was five and 12 times lower, respectively, than that in the industrial area. The total CR was significant for children in the rural area but not for adults. All three areas were classified as low CR zones (10–6< CR < 10–4) following NYSDOH standards. Of the three potential exposure routes, dermal absorption was the highest (84–89%), followed by ingestion (10–15%) and inhalation (0.5–1.0%).
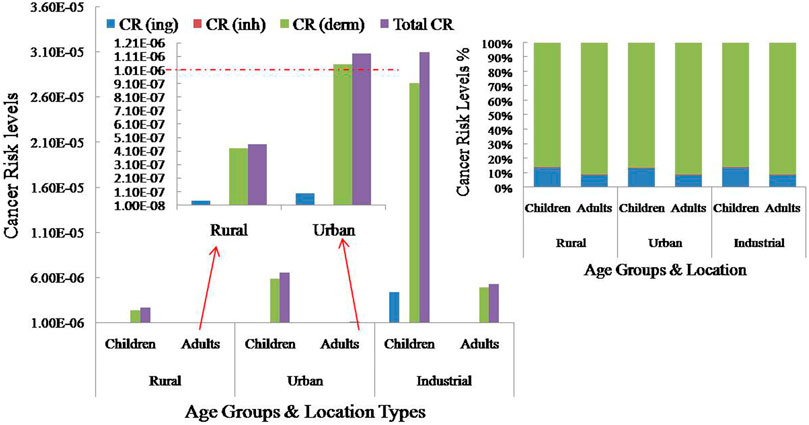
FIGURE 6. Cancer risk from soil-bound PAHs in rural, urban, and industrial residential areas near the Ulsan industrial belt.
Significant CR from soil-bound PAHs has been reported for other Asian countries, including China (10−6–10–4) and India (10−6–10–5) (Shi et al., 2020; Tarafdar and Sinha, 2017). Dermal absorption has also been identified as a major exposure pathway, followed by ingestion and inhalation. In Korea, Kim et al. (2019) reported a significant CR (5.50E-06) for roadside soil-bound PAHs with the common exposure routes (dermal absorption > ingestion > inhalation) for the CR levels. The soil in Ulsan industrial areas is six times more carcinogenic compare to the soils from roadside traffic-dense areas as reported by Kim et al., 2019. Industrial activity could be a major cause of the elevated CR levels in the industrial area. Jeon and Oh (2019) reported that refining, automobile manufacturing, petrochemical processes, shipbuilding, and smelting plants could be responsible for the CR due to soil-bound PAHs in Ulsan industrial areas.
Sensitivity Analysis
The results of the sensitivity test are summarized in Table 4. The PAH concentration levels and BSAF were identified as the most sensitive parameters for the CR at KS1 and KS2, with a range of 44–48% at both locations. At KS3, BSAF and ED were found to be most sensitive with 76 and 10%, respectively. The BSAF was identified as the most sensitive (47–82%) for CRing. Tarafdar and Sinha, 2017 and Yang et al. (2014) have also identified the BSAF as a key factor in the CR for coastal areas. For dermal exposure, CSsoil, AF, and ED were the most sensitive and significant parameters for CR. The sensitivity test revealed that ED (55–85%), AF (35–42%), and SA (13%) were most significant for the total CR across the study area. For CRing, CRinh, and CRderm, ED (99%), ED (72–97%), and AF (55–59%), respectively were found to be the most significant.
In previous study, AF and ED were identified as the most sensitive factors for CR due to soil exposure (Tarafdar and Sinha, 2017; Chen and Liao, 2006; Chiang et al., 2009; Wu et al., 2011). The risk assessment parameters CSsedi, BSAF, BW, ED, AF, SA, and IRfish have been reported as the most commonly identified sensitive parameters for CR. In the present study, BW, ED, IRfish, and CSsedi were calculated according to the Korean standards reported in several studies, while the other parameters were taken from USEPA data. Determining the important risk assessment parameters BSAF, AF, and SA for Korea is thus recommended for future research.
Conclusion
PAH levels in sediment and soil are of great concern due to their carcinogenicity. The sediments and soils in Ulsan coastal and industrial areas have been found to have high levels of carcinogenic PAHs. These areas are recognized as being more highly polluted than other cities and coastal areas in Korea. In the present study, the CR was determined for sediment- and soil-bound carcinogenic PAHs in coastal and residential areas in Korea. In coastal areas, the CR was estimated to be moderate and high at Mohang Harbor and Ulsan, respectively. This study also found that the risk assessment parameters BSAF, ED, IRfish, SA, and AF were most sensitive to the total CR. Fish consumption has been recognized as the most significant route to the human body for sediment-bound PAHs in Korea. A significant CR was identified for soil-bound PAHs in rural, urban, and industrial areas near the Ulsan industrial zone. Additionally, the CR found to be significantly higher in the industrial area than in the urban and rural areas, respectively. Exposure through dermal absorption and food ingestion was identified as the most significant route to the human body for soil-bound PAHs. The soil and sediments in Ulsan industrial and coastal areas thus have high PAH contamination and represent high cancer risk zones. High seafood and fish consumption may thus be responsible for the rise in the number of cancer cases in Korea. Further research into this possibility is required. The collection of fish sample and analysis of sediment related PAHs in fish/seafood could be the possible further research scope of my present research.
Data Availability Statement
The original contributions presented in the study are included in the article/Supplementary Material, further inquiries can be directed to the corresponding author.
Author Contributions
DR and JP conceived of the study design. DR, JK, WJ, and ML collected and interpreted the data. DR drafted the manuscript. DR and JP revised the manuscript.
Funding
This research was supported by the Basic Science Research Program through the National Research Foundation of Korea (NRF) funded by the Ministry of Education (No. 2018R1A6A1A08025348) and Korea Ministry of Environment (The Subsurface Environment Management, Project No. 2020002480003).
Conflict of Interest
The authors declare that the research was conducted in the absence of any commercial or financial relationships that could be construed as a potential conflict of interest.
Supplementary Material
The Supplementary Material for this article can be found online at: https://www.frontiersin.org/articles/10.3389/fenvs.2021.719243/full#supplementary-material
References
Abdel-Shafy, H. I., and Mansour, M. S. M. (2016). A Review on Polycyclic Aromatic Hydrocarbons: Source, Environmental Impact, Effect on Human Health and Remediation. Egypt. J. Pet. 25, 107–123. doi:10.1016/j.ejpe.2015.03.011
Adeniji, A. O., Okoh, O. O., and Okoh, A. I. (2019). Distribution Pattern and Health Risk Assessment of Polycyclic Aromatic Hydrocarbons in the Water and Sediment of Algoa Bay, South Africa. Environ. Geochem. Health 41, 1303–1320. doi:10.1007/s10653-018-0213-x
Armstrong, B., Hutchinson, E., Unwin, J., and Fletcher, T. (2004). Lung Cancer Risk after Exposure to Polycyclic Aromatic Hydrocarbons: a Review and Meta-Analysis. Environ. Health Perspect. 112, 970–978. doi:10.1289/ehp.689510.1289/ehp.6895
Boffetta, P., Jourenkova, N., and Gustavsson, P. (1997). Cancer Risk from Occupational and Environmental Exposure to Polycyclic Aromatic Hydrocarbons. Cancer Causes Control 8, 444–472. doi:10.1023/a:1018465507029
Bosetti, C., Boffetta, P., and La Vecchia, C. (2007). Occupational Exposures to Polycyclic Aromatic Hydrocarbons, and Respiratory and Urinary Tract Cancers: a Quantitative Review to 2005. Ann. Oncol. 18, 431–446. doi:10.1093/annonc/mdl172
Chawda, S., Tarafdar, A., Sinha, A., and Mishra, B. K. (2020). Profiling and Health Risk Assessment of PAHs Content in Tandoori and Tawa Bread from India. Polycyclic aromatic Compd. 40 (1), 21–32. doi:10.1080/10406638.2017.1349679
Chen, S. C., and Liao, C. M. (2006). Health Risk Assessment on Human Exposed to Environmental Polycyclic Aromatic Hydrocarbons Pollution Sources. Sci. Total Environ. 366, 112–123. doi:10.1016/j.scitotenv.2005.08.047
Chen, J. W., Wang, S. L., Hsieh, D. P. H., Yang, H. H., and Lee, H. L. (2012). Carcinogenic Potencies of Polycyclic Aromatic Hydrocarbons for Back-Door Neighbors of Restaurants with Cooking Emissions. Sci. Total Environ. 417-418 (418), 68–75. doi:10.1016/j.scitotenv.2011.12.012
Chiang, K. C., Chio, C. P., Chiang, Y. H., and Liao, C. M. (2009). Assessing Hazardous Risks of Human Exposure to temple Airborne Polycyclic Aromatic Hydrocarbons. J. Hazard. Mater. 166, 676–685. doi:10.1016/j.jhazmat.2008.11.084
FAO (2020). The State of World Fisheries and Aquaculture 2020. Sustainability in Action. Rome: FAO. doi:10.4060/ca9229en
Hussain, M., Rae, J., Gilman, A., and Kauss, P. (1998). Lifetime Health Risk Assessment from Exposure of Recreational Users to Polycyclic Aromatic Hydrocarbons. Arch. Environ. Contam. Toxicol. 35, 527–531. doi:10.1007/s002449900412
IARC (2010). IARC Monographs on the Evaluation of Carcinogenic Risks to Humans: Some Non-heterocyclic Polycyclic Aromatic Hydrocarbons and Some Related Exposures. IARC Monogr. Eval. Carcinog Risks Hum. 92, 1–853.
Jeon, H. D., and Oh, S. Y. (2019). Distribution, Toxicity, and Origins of Polycyclic Aromatic Hydrocarbons in Soils in Ulsan, South Korea. Environ. Monit. Assess. 191, 409. doi:10.1007/s10661-019-7558-1
Kim, C. J., Hong, G. H., Kim, H. E., and Yang, D. B. (2014). Polycyclic Aromatic Hydrocarbons (PAHs) in Starfish Body and Bottom Sediments in Mohang Harbor (Taean), South Korea. Environ. Monit. Assess. 186 (7), 4343–4356. doi:10.1007/s10661-014-3703-z
Kim, S. J., Park, M. K., Lee, S. E., Go, H. J., Cho, B. C., Lee, Y. S., et al. (2019). Impact of Traffic Volumes on Levels, Patterns, and Toxicity of Polycyclic Aromatic Hydrocarbons in Roadside Soils. Environ. Sci. Process. Impacts 21, 174–182. doi:10.1039/c8em00532j
Kim, S., Hong, S., Lee, J., Kim, T., Yoon, S. J., Lee, J., et al. (2020). Long-term Trends of Persistent Toxic Substances and Potential Toxicities in Sediments along the West Coast of South Korea. Mar. Pollut. Bull. 151. 110821. doi:10.1016/j.marpolbul.2019.110821
Kwok, C. K., Liang, Y., Leung, S. Y., Wang, H., Dong, Y. H., Young, L., et al. (2013). Biota-sediment Accumulation Factor (BSAF), Bioaccumulation Factor (BAF), and Contaminant Levels in Prey Fish to Indicate the Extent of PAHs and OCPs Contamination in Eggs of Waterbirds. Environ. Sci. Pollut. Res. 20, 8425–8434. doi:10.1007/s11356-013-1809-4
Kwon, H. O., and Choi, S. D. (2014). Polycyclic Aromatic Hydrocarbons (PAHs) in Soils from a Multi-Industrial City, South Korea. Sci. Total Environ. 470-471, 1494–1501. doi:10.1016/j.scitotenv.2013.08.031
Lee, J. Y., and Kim, Y. P. (2007). Source Apportionment of the Particulate PAHs at Seoul, Korea: Impact of Long Range Transport to a Megacity. Atmos. Chem. Phys. 7, 3587–3596. doi:10.5194/acp-7-3587-2007
Li, H. L., Liu, G. L., and Cao, Y. (2014). Content and Distribution of Trace Elements and Polycyclic Aromatic Hydrocarbons in Fly Ash from a Coal-Fired CHP Plant. Aerosol Air Qual. Res. 14, 1179–1188. doi:10.4209/aaqr.2013.06.0216
Moon, H. B., Choi, H. G., Kim, S. S., and Lee, P. Y. (2002). Accumulation and Characterization of Polycyclic Aromatic Hydrocarbons in Seafood from the Coastal Areas of Korea. Fish. Aquat. Sci. 5 (2), 127–135. doi:10.5657/fas.2002.5.2.127
NYSDOH (2012). Health Consultation: Hopewell Precision Area Groundwater Contamination Site Town of East Fishkill, Dutchess County, New York, NY. Appendix C, DOH Procedure for Evaluating Potential Health Risks for Contaminants of Concern. New York: EPA.
Petry, T., Schmid, P., and Schlatter, C. (1996). The Use of Toxic Equivalency Factors in Assessing Occupational and Environmental Health Risk Associated with Exposure to Airborne Mixtures of Polycyclic Aromatic Hydrocarbons (PAHs). Chemosphere 32, 639–648. doi:10.1016/0045-6535(95)00348-7
Roy, D., Seo, Y. C., Sinha, S., Singh, G., Bhattacharya, A., and Biswas, P. K. (2017). Human Health Risk Exposure with Respect to Particulate-Bound Polycyclic Aromatic Hydrocarbons at Mine Fire-Affected Coal Mining Complex. Environ. Sci. Pollut. Res. 26, 19119–19135. doi:10.1007/s11356-017-9202-3
Roy, D., Seo, Y. C., Kim, S., and Oh, J. (2019a). Human Health Risks Assessment for Airborne PM10-Bound Metals in Seoul, Korea. Environ. Sci. Pollut. Res. 26, 24247–24261. doi:10.1007/s11356-019-05213-y
Roy, D., Seo, Y. C., Namgung, H. G., and Kwon, S. B. (2019b). Inhalation Cancer Risk from PM10 in the Metropolitan Subway Stations in Korea. J. Transport Health 14, 100580. doi:10.1016/j.jth.2019.100580
Roy, D., Singh, G., and Seo, Y. C. (2019c). Coal Mine Fire Effects on Carcinogenicity and Non-carcinogenicity Human Health Risks. Environ. Pollut. 254, 113091. doi:10.1016/j.envpol.2019.113091
Roy, D., Singh, G., and Seo, Y. C. (2019d). Cancer and Non-cancer Risks from PM10-And PM2.5-bound Metal in a Critically Polluted Coal Mining Complex. Atmos. Pollut. Res. 10, 1964–1975. doi:10.1016/j.apr.2019.09.002
Roy, D., Ahn, S. H., Lee, T. K., Seo, Y. C., and Park, J. (2020). Cancer and Non-cancer Risk Associated with PM10-Bound Metals in Subways. Transport Res. D-tr Environ. 89, 102618. doi:10.1016/j.trd.2020.102618
Seo, S. H., Kwon, H. O., Park, M. K., Lee, I. S., and Choi, S. D., 2020. Contamination Characteristics of Polycyclic Aromatic Hydrocarbons in River and Coastal Sediments Collected from the Multi-Industrial City of Ulsan, South Korea. Marpollut Bull. 160, 11166. doi:10.1016/j.marpolbul.2020.111666
Shi, R., Li, X., Yang, Y., Fan, Y., and Zhao, Z. (2021). Contamination and Human Health Risks of Polycyclic Aromatic Hydrocarbons in Surface Soils from Tianjin Coastal New Region, China. Environ. Pollut. 268, 115938. doi:10.1016/j.envpol.2020.115938
Sim, B. R., Kim, H. C., Kim, C. S., Hwang, D. W., Park, J. H., Cho, Y. S., et al. (2018). Spatio-temporal Changes of Sediment Environment in the Taehwa River Estuary, Ulsan of Korea. J. Coastal Res.85 (10085), 41–45. doi:10.2112/SI85-009.1
Singare, P. U. (2015). Studies on Polycyclic Aromatic Hydrocarbons in Surface Sediments of Mithi River Near Mumbai, India: Assessment of Sources, Toxicity Risk and Biological Impact. Mar. Pollut. Bull. 101, 232–242. doi:10.1016/j.marpolbul.2015.09.057
Tarafdar, A., and Sinha, A. (2017a). Cancer Risk Assessment of Polycyclic Aromatic Hydrocarbons in the Soils and Sediments of India: A Meta-Analysis. Environ. Manage. 60, 784–795. doi:10.1007/s00267-017-0920-6
Tarafdar, A., and Sinha, A. (2017b). Estimation of Decrease in Cancer Risk by Biodegradation of PAHs Content from an Urban Traffic Soil. Environ. Sci. Pollut. Res. 24, 10373–10380. doi:10.1007/s11356-017-8676-3
USEPA (1989). Risk Assessment Guidance for Superfund, Vol. I: Human Health Evaluation Manual (Part A). I:291. Washington, DC: USEPA. EPA/540/1-89/002.
USEPA (1993). Provisional Guidance for Quantitative Risk Assessment of Polycyclic Aromatic Hydrocarbons. Washington, DC: USEPA.
USEPA (2002). Supplemental Guidance for Developing Soil Screening Levels for Superfund Sites. Washington, DC: USEPA.
USEPA (2003a). Integrated Risk Information System. Washington, DC: USEPAAvailable at: https://www.epa.gov/iris (Accessed 602. 04 10 2020).
USEPA (2003b). Priority Pollutants. Code Fed Regul (CFR) Title 40, Chapter IPart 423, Append A to Part 423. Washington, DC: USEPA, 423.
USEPA (2004). Risk Assessment Guidance for Superfund (RAGS), Vol. I: Human Health Evaluation Manual (Part E, Supplemental Guidance for Dermal Risk Assessment). Washington, DC: USEPA.
USEPA. 2009. Risk Assessment Guidance for Superfund, Vol. I: Human Health Evaluation Manual (Part F, Supplemental Guidance for Inhalation Risk Assessment). Washington, DC: USEPA.
Wang, W., Huang, M. J., Kang, Y., Wang, H. S., Leung, A. O. W., Cheung, K. C., et al. (2011). Polycyclic Aromatic Hydrocarbons (PAHs) in Urban Surface Dust of Guangzhou, China: Status, Sources and Human Health Risk Assessment. Sci. Total Environ. 409, 4519–4527. doi:10.1016/j.scitotenv.2011.07.030
Wu, B., Zhang, Y., Zhang, X. X., and Cheng, S. P. (2011). Health Risk Assessment of Polycyclic Aromatic Hydrocarbons in the Source Water and Drinking Water of China: Quantitative Analysis Based on Published Monitoring Data. Sci. Total Environ. 410-411, 112–118. doi:10.1016/j.scitotenv.2011.09.046
Yang, W., Lang, Y., and Li, G. (2014). Cancer Risk of Polycyclic Aromatic Hydrocarbons (PAHs) in the Soils from Jiaozhou Bay Wetland. Chemosphere 112, 289–295. doi:10.1016/j.chemosphere.2014.04.074
Yoon, H., Yoo, S. K., Seo, J., Kim, T., Kim, P., Kim, P. J., et al. (2020). Development of General Exposure Factors for Risk Assessment in Korean Children. Ijerph 17, 1988. doi:10.3390/ijerph17061988
Zhang, J., Fan, S., Du, X., Juncheng, Y., Wenyan, W., Hong, H., et al. (2015). Accumulation, Allocation, and Risk Assessment of Polycyclic Aromatic Hydrocarbons (PAHs) in Soil-brassica Chinensis System. PLoS One 10, 1–16. doi:10.1371/journal.pone.0115863
Keywords: cancer risk, soil, sediments, polycyclic aromatic hydrocarbons, coastal area, South Korea
Citation: Roy D, Jung W, Kim J, Lee M and Park J (2021) Cancer Risk Levels for Sediment- and Soil-Bound Polycyclic Aromatic Hydrocarbons in Coastal Areas of South Korea. Front. Environ. Sci. 9:719243. doi: 10.3389/fenvs.2021.719243
Received: 02 June 2021; Accepted: 28 June 2021;
Published: 09 July 2021.
Edited by:
Abhrajyoti Tarafdar, Korea University, South KoreaReviewed by:
Mehrzad Keshavarzifard, Iranian Fisheries Research Organization, IranVeerasingam S., Qatar University, Qatar
Copyright © 2021 Roy, Jung, Kim, Lee and Park. This is an open-access article distributed under the terms of the Creative Commons Attribution License (CC BY). The use, distribution or reproduction in other forums is permitted, provided the original author(s) and the copyright owner(s) are credited and that the original publication in this journal is cited, in accordance with accepted academic practice. No use, distribution or reproduction is permitted which does not comply with these terms.
*Correspondence: Joonhong Park, parkj@yonsei.ac.kr