- 1Department of Soil Science and Agricultural Chemistry, Birsa Agricultural University, Ranchi, India
- 2Department of Agronomy, Birsa Agricultural University, Ranchi, India
- 3Central Tasar Research and Training Institute, Ranchi, India
In recent decades, climate change induced by enhanced global warming is one of the biggest challenges at the global level. Agriculture sectors significantly contribute to total anthropogenic greenhouse gas emission to the atmosphere. Wheat and maize, cultivated globally, and consumed in different forms, are considered as crucial staple cereal for ensuring food security to global population. The management practices involving land preparation, sowing, fertilizer application, irrigation, pest management, etc. significantly influence the emission of carbon dioxide (CO2) and nitrous oxide (N2O) from agricultural soil. In this study, CO2 and N2O emission were assessed from maize and wheat crops at four different levels of N fertilizer using cool farm tool model. Emissions of CO2 per hectare varied from 331.4 to 1,088.3 kgCO2 in maize and ranged from 292.3 to 765.3 kgCO2 in wheat on application of different doses of N. The total GHG emission in maize crops ranged from 859.5 to 3,003.4 kgCO2 eq per hectare with the application of nitrogen at varying levels (0–240 kg N per hectare). The highest N2O efflux (0.368 kg per ton) was observed at 240 kg N per hectare under wheat crop. The total on-farm emissions, through fertilizer production, account for about 33.7%, and emission of N2O contributes only 65.9%, whereas pesticides account merely 0.4% under maize-wheat cropping. This study confirms that the direct emission of N2O was totally dependent on N fertilizers application rate; however, the indirect emission was controlled by the fuels and energy consumption.
Introduction
The production of food grains (maize, rice, wheat, etc.) is being adversely affected by climate change, and agricultural activities leading to emission of methane (CH4), carbon di-oxide (CO2), and nitrous oxide (N2O) (Malyan et al., 2021) serve as a major contributing factor to future climate change. The main anthropogenic source of CH4 (77%) and N2O (60%) emissions contributes in Indian Agriculture (Sharma et al., 2021). Methane emissions are confined to rice and enteric fermentation (Kumar et al., 2020b), while CO2 and N2O are uniformly released from all agricultural crops as consequences of crop raising activities such as soil manipulation and fertilizer applications (Kumar et al., 2016a; Kumar et al., 2016b; Bhattacharyya et al., 2018; Sapkota et al., 2021). The addition of nitrogen to agricultural soil changes GHG fluxes. Since 1960, consumption of nitrogen fertilizer has increased 66 times (Fagodiya et al., 2020a). In India, the use of irrational nutrient applications has resulted in low input use efficiency, lower income, and enhanced pollution (Pampolino et al., 2012). In India, fertilizer recommendation is based on response of a crop over a broad geographical area which could not concede the spatial variation in soil nutrient supplying ability (Majumdar et al., 2013). It is a well known fact that aeration, soil temperature, soil moisture, organic carbon (OC) supplies, fertilization, pH, and other environmental factors like production and transport influence N2O and CO2 emission in soil (Kumar and Sharma, 2017a; Kumar et al., 2020a; Fagodiya et al., 2020a).
In general, nitrogen is the most critical and limiting nutrient in agricultural production. The global consumption of nitrogen fertilizer has increased from 12 Tg (1960) to 113 Tg (2010) (Fagodiya et al., 2017). Moreover, the main components of nitrogen cycle are nitrification, assimilation, ammonification, and denitrification (Kumar et al., 2016b; Kumar and Sharma, 2017a; Kumar and Sharma, 2017b). Several compounds like NH3, NOx, NO, N2O, NO3 etc. could be released into the atmosphere during the nitrogen cycle, influencing the climate system. The reactive nitrogen (Nr) plays direct and indirect roles on N2O emissions in soil. N2O emissions are of major concern because of their extensive atmospheric lifespan (approx. 116 years), maximum potential of global warming (310 times that of CO2), and high global climate change potential (290 on a100−year basis) (Fagodiya et al., 2020a). N2O emissions can occur both directly and indirectly from fertilizer N input to the soil. Additional fertilizer N application to the same soil is referred as direct emissions (Holka and Bienkowski, 2020). Indirect N2O emissions are those that occur from the different sources except soil that should not be limited, like N2O generated through waters and leaching of NO3 to the soil (IPCC, 2006). Farms and horticultural enterprises account for just about 8% CO2 emission. N2O and CH4 are important GHG which contribute 57% and 35%, respectively, to global warming (FAS, 2014). N2O is a potent GHG that contributes directly to global warming. For a period of 100 years, N2O emissions were more sensitive than other GHG because of its long atmospheric habitation (114 years), global climate change potential (290 times higher than CO2), and global warming potential (265 times higher than CO2). All these gases contribute as the third most abundant GHG after CO2 and CH4. Approx. 18.09% of total GHG and 73.29% of total N2O were emitted from the agricultural sector in India during 2014. The use of nitrogen fertilizer fulfills the demand of food production considering the growing population, resulting in higher N2O emissions from the country’s agriculture.
Maize is an aerobic crop and unlike rice, puddling or submergence is not required for its cultivation. As a result, less energy is needed for tillage operation as well as less water is required for maize, resulting in fewer CH4 emissions in comparison to rice fields. Hence, maize is a better option for minimizing ground water depletion, soil degradation, and CH4 emissions from the Eastern plateau region of India. Maize, the third most important cereal crop, contributes 78.2 million tons to world total food grain production with an area of about 150 million hectares (McCann, 2007; Parihar et al., 2011). India is producing 30.41 million tons from 9.1 million hectares (mha) area with a productivity of 2,771 kgha−1 (USDA, 2019). Maize-wheat cropping system ranked third after rice-wheat and rice-rice cropping systems (Jat et al., 2014). Consequently, wheat can be grown in a variety of climates around the world, covering more than 200 mha. The estimated production of the world crossed 750 million metric tons in 2016–17. India contributed 87 million metric tons from 30.22 mha area in 2016–17, core production of wheat (98.5 million ton) was registered from 30.72 mha in 2017–18, and a forecast of reduction in production in 2018–19 was estimated to 94 million metric ton (USDA, 2018) suggesting a degree of uncertainty in production level. Being less CH4 gas emitter, maize-wheat is appealing as an alternative cropping system compared to rice-wheat system. Most of the studies conducted on mitigation of GHG emission in eastern plateau region of India are mainly based on rice-wheat cropping system. The N2O emissions from MWR have rarely been reported. The GHG emissions associated with the manufacture of synthetic N fertilizers were estimated to be 41.44 and 59.71 Mt CO2-eq year−1 for wheat and maize in China, respectively. And the direct N2O emissions derived from synthetic N fertilization were estimated to be 35.82 and 69.44 Gg N2O year−1 for wheat and maize, respectively (Chai et al., 2019). The carbon sequestration under maize-wheat cropping system provides promising prospects for reducing GHG emission. GHG emission can be measured with the help of Cool Farm Tool (Hillier et al., 2011) which has been defined as an empirical GHG quantification model into a single tool. Cool farm tool (CFT) enables the user to make choices appropriate to existing practices. The CFT is open-source software integrating several globally determined empirical models into a GHG emission calculator. At farm level, the tool identifies context-specific factors like pedo-climatic characteristics, output inputs, and other management activities that affect GHG emission. The Cool Farm Tool (CFT) calculates the total emission of GHG in terms of “per unit area” as well as “per unit product.” The key objective of this study is to estimate the CO2 and N2O emission under maize-wheat cropping system at four different levels of N fertilizer application and three modes of application, by using the CFT model as the direct measurement to GHG emission.
Materials and Methods
Description of Research Site
The research programme was carried out during 2015–16 and 2016–17 at the research farm of Ranchi Agriculture College under Birsa Agricultural University, Ranchi, under Maize-Wheat cropping system (Figure 1). The research field was located at 23°19’ N and 83°17’ E, at altitude of 625 m above mean sea level (MASL) in the Chhotanagpur Plateau, which comes under the eastern section of the Deccan plateau and situated under Agro-climatic Zone V.
The climate of state is falling under tropical and sub-tropical. During the summer season temperatures varied from 18 to 40°C, while during the winter, temperatures ranged from 0 to 22°C. The lowest temperature was recorded in the months of December and January; in some areas of Kanke sometimes the temperature dipping down to the freezing point. The annual total rainfall received by the experimental areas is about 1,430 mm (56.34 inches) and 78–91% rainfall is received during the peak period of monsoon through South West Monsoon (from June to September). The rest is received in different seasonal spells like North East Monsoon (6.5% with amount 92.4 mm) from October to December. In the winter season, it received 3.74% with amount 52.4 mm (January to February) and summer 7.5% with the amount 104.7 mm with thunderstorm showers. During the maize growing season, total rainfall was about 528 mm, with average temperatures ranging from 20.3 to 30.80°C; however, rainfall was around 47 mm, with temperatures ranging from 3.10 to 39.50°C during the wheat cropping season. From the second week of February to the first week of March 2016, the highest average morning relative humidity was 87%, and the lowest average evening relative humidity was 31% in the third week of March.
Treatments and Cropping Systems
This experiment consisted four levels of nitrogen application (0, 80, 160, 240 kg Nha−1 for maize and 0, 50, 100, 150 kg Nha−1 for wheat) with three different modes of application. Methods of application of nitrogen were two splits (50-0–50 kg ha−1) at basal and V10 stages, three splits (33-33-33 kgha−1) at basal, V4, and V10 stage and (33-33-33 on the basis of LCC), in case of wheat apply as basal, and at CRI stage (50-0-50), basal, CRI, and PI stage (33-33-33). The phosphatic (single super phosphate) and potassic fertilizer (Murate of Potash) at the rate of 100 kgP2O5 and 100 kg K2O−1ha were applied as basal irrespective of treatments as shown in Table 1. The date of sowing of maize was 26/June 25, 2015/16 and date of harvesting was 05/October 6, 2015/16. However, the phosphatic and potassic fertilizer at 90 kgP2O5 and 80 kgK2Oha−1 were applied as basal to all the treatment, and date of sowing were 10/December 11, 2015/16 and date of harvesting 12/April 13, 2016/17 respectively.
Methods Used for Calculating CO2 Emission From Farm Operation
The emission of CO2 from on farm and off farm through various activities like irrigation, tillage, fertilizer, and pesticide production are calculated from various published emission factors.
Irrigation
The emission of CO2 after irrigation was calculated at 30% electric pump efficiency (Nelson et al., 2009; Gupta et al., 2015) and 19% electric power transmission and distribution losses in India (World Bank, 2014) by the following formula:
where 2.724 is energy (kWh) needed to lift 1,000 m3 of water from 1 m depth without any loss in pump efficiency and 0.4062 is carbon density (kgC per kWh) of coal-based electricity generation (Nelson et al., 2009).
Tillage and Sowing
Two tillage operations were conducted and CO2 emission was calculated as per Gupta et al. (2015).
where 0.728 is CO2-C emissions (kg) from consumption of 1 L diesel (EPA 2005).
Pesticide Production and Transportation
The CO2 emission from pesticide production and transportation was calculated from the following equation
where 6.3, 5.1, and 3.9 is amount of CO2-C emitted from production and transportation of 1 kg of herbicide, insecticide, and fungicide respectively (Lal, 2004).
Fertilizer Production and Transportation
The CO2 emission from fertilizer production and transportation was calculated from the following equation
where 2.02, 1.84, 0.06, and 0.25 is CO2-C emission (kg) from the production and transport of 1 kg of N (urea), N (DAP), P2O5(SSP), and K2O (MOP) adopted from Kool et al., 2012.
Results and Discussion
Carbon Dioxide Emission
CO2 emitted by maize grown during the wet season emission ranged from 331.4 to 1,088.1 kg ha−1, while in wheat (winter season) it varied from 292.3 to 765.3 kg ha−1 depending on the application rates of nitrogen fertilizer (Table 2). The mean value of emitted CO2 was 1,088.1 kgha−1, when maize received 240 kg N ha−1, while 836.0 kg CO2 ha−1 was released at 160 kg N ha−1. However, the emission of CO2 was lowest at a level of 0 kg N (331.4 kg CO2 ha−1). During the winter season, the highest CO2 emission (765.3 kg CO2 ha−1) was recorded at 150 kg N ha−1, followed by 100 kg N ha−1 (613.7 kg CO2ha−1). At 50 kg level of N fertilizer application, the emission was 456.0 kg CO2ha−1, that was 35.9% higher than that of the emission noted at 0 kg level (292.3 kg CO2ha−1). Soil manipulation like tillage triggers CO2 emission through biological decomposition of soil organic matter (SOM) acts as the primary source of CO2 from agriculture field. Disintegration of soil aggregates through ploughing increases oxygen availability and facilitates organic matter (OM) decomposition of exposed organic material (Bhattacharyya et al., 2018). Other sources of CO2 emissions include the fuel used for various agricultural activities and the burning of crop residues (Gupta et al., 2015). Carbon dioxide production at the time of manufacturing of fertilizers and pesticides is an off-site source (Pathak et al., 2010; Pathak et al., 2016).

TABLE 2. Response of various doses of nitrogen treatments on GHG emission (maize–wheat cropping system).
Nitrous Oxide Emission
Emission of N2O from the soil occurs as a result of additional nitrogenous fertilizer application to the soil (within a defined boundary of producer’s field) referred as direct emissions (Sharma, 2020). N2O gas emission emitted off-site (beyond the boundary) are referred as indirect emissions, which do not include N2O gas produced through receiving runoff water and NO3 leaching in soil (Fagodiya et al., 2019). Nutrient management strategies had a significant impact on the amount of N2O gas released per hectare and per ton of crop yield. Different rates of nitrogen application showed a major impact during the estimation of N2O emission per hectare in both crops (maize and wheat). In maize, application of 160 kg N ha−1 estimated higher N2O emission (4.3 kg N2O ha−1) compared to 80 kg N ha−1 (2.8 kg N2O ha−1). However, calculated N2O emission was highest with N at 240 kg N ha−1 (6.5 kg N2O ha−1) and lowest value of 1.8 kg N2O ha−1 at 0 kg N ha−1 (Table 2). The same pattern of emission was not followed in case of emissions per ton of maize yield than per hectare. That might be directly associated to the crop yield, while per hectare value is preferably reliant on yield, fertilizer doses, and other factors influencing crop production. The calculated value of nitrous oxide emission per ton of maize yield was lower (0.27 kg tonne−1) at application of 160 kg N than that of 240 kg N ha−1 applied plot (0.37 kgton−1). The highest value was observed with the produce recorded at N omitted plot (0.94 kg ton−1). The calculated N2O emission per tone was 0.28 kg ton−1 at 80 kg N ha−1 that was marginally higher than the 160 kg N ha−1 applied plot (0.27 kg tonne−1) (Table 2). This has been found and reported that N inputs gradient in row-crop agriculture directly dependent to the emissions of N2O with different rate of nitrogenous fertilizer (Halvorson et al., 2008; Hoben et al., 2010; Millar et al., 2010). Application of increased nitrogen dose to the soil enhanced N2O emissions in both of crops. The latest IPCC (2006) greenhouse gas inventory calculations are based on many factors, like heat, structure, water holding capacity, and organic matter content of the soil, and these are directly responsible for the rise in N2O emissions. In general, management of crop residues and fertilizer application are major responsible factors for theN2O pollution in agro-ecosystems (Rochette et al., 2008). The estimated value of N2O emission per hectare in the case of wheat grown with 150 kg N ha−1 during winter season was maximum 4.1 kg ha−1, followed by 100 kg N ha−1 (3.5 kg N2O ha−1). The lowest value (1.8 kg N2O ha−1) was recorded with the omission of nitrogenous fertilizer. On the other hand, in terms of N2O emission per ton in wheat was highest (0.63 kg tonne−1) under the N-omitted plot (0 kg N ha−1), followed by the application of 150 kg N ha−1 (0.31 kg ton−1), and the lowest value (0.28 kg tonne−1) was recorded with the application of 100 kg N ha−1. It may be concluded that the variation in NO2 emission per ton is due to the variation in yield and urea application. Jain et al. (2016) recorded that factor of N2O emission varied from 0.48 to 0.58% and 0.40–0.46% in wheat and maize crops, respectively whereas, Bhatia et al. (2005) noted emission factor of 0.58–0.62% with the application of N fertilizers. Moreover, as per IPCC (2014) the default coefficient factor of nitrous oxide emission for N fertilizers was 1%.
Methane Emission
With removal of crop residues from the field at the time of tillage operation before sowing of maize and wheat, no methane gas emission was observed by using the CFT model. Soil CH4 production is reliant on a limited supply of oxygen (anaerobic condition), which is regulated by moisture content of soil. Sowing of wheat in dry soil during winter (devoid of submerged condition) may be one of the key factors which suppressed the methane gas emission (Aryal et al., 2015).
Total Greenhouse Gas Emission
Maize
Global warming potential (GWP) per hectare for total GHG emission and CO2-equivalent (CO2-eq) per ton of crop yield positively varied at various level of nitrogen application (Table 2). The extent of total GHG emissions in maize contributed from 859.5 to 3,004 kg CO2 eq ha−1, depending on the doses of N fertilizers at 0 kg N ha−1–240 kg N ha−1. Emissions of gases increased with the escalating amount of nitrogen fertilizer. The application of 240 kg N ha−1 resulted in the highest GHG emission, followed by 160 kg N ha−1 (2,109.1 kg CO2eq ha−1) and at 0 kg N ha−1 resulted the lowest emission (859.5 kg CO2eq ha−1). While the emission of gases at the rate of 80 kg N ha−1 was 1,417.1 kg CO2 eq ha−1, it was marginally inferior to the use of 160 kg Nha−1. The availability of mineral affected the enormity of N2O emissions throughout the crop growth. Under the control scenario, the overall predictable GHG emission in terms of CO2eqton−1 of product was considerably higher compared to the supplementary treatments. Application of different doses of nitrogen (0, 80, 160, and 240 kg N ha−1) in maize emits 450.0, 143.9, 132.5, and 170.3 kg CO2 eqton−1, respectively.
Wheat
The amount of produced total GHG followed the same trend per ha and per tons maize, with a lower magnitude. The application of nitrogen at150 kg ha−1 had the highest GHG emission per ha (1974.1 kg CO2 eq), subsequently with the addition of 100 kg N ha-1 released 1,650.2 kg CO2 eq per hectare and omission of N fertilizer (0 kg N) showed 820.2 kg CO2 eq per hectare (Table 2). Because of variation in wheat yield, the total anticipated GHG emission with respect to CO2eq ton-1 of produce was lowest at the rate of 100 kg N ha-1 (133.7 kg CO2 eq ton-1) and the highest value 287.5 kg CO2 eq ton-1 was at 0 kg N ha−1. Total GHG emission varied marginally between the application of nitrogen at the rate of 100 kg ha-1 (133.5 kg CO2 eq ton-1) and at 50 kg N per hectare (135.6 kg CO2 eq ton-1), while the higher value 1,545 kg CO2 eq ton-1 was found at the rate of 150 kg N ha−1. The yield, biological produce, and rate of N application all affected the value of total emission of GHGs by using CFT. The findings showed that when a greater amount of input was used, the total emission was higher than that at lesser amount of input. Among various emission sources, manufacture and use of synthetic fertilizers was found to be the most important source of pollution at the farm level. Pesticides account for just 0.50% of total on-farm emissions in maize, while synthesis of fertilizer and emissions of nitrous oxide account for 32 and 67% as whole farm emissions, respectively. Whereas, in case of wheat, fertilizer production and nitrous oxide emissions showed 35 and 64% of emissions, respectively, and pesticides account for only 0.6 percent. GHG emission by the use of nitrogenous fertilizer was split into two categories as intended towards N2O emissions and GHG emission from fertilizer synthesizer unit. Nitrous oxide emissions could occur from microbial activities in soils too (Table 3). In agriculture, the mineralization process of nitrogen (ammonium to nitrates and the reduction of nitrate to gaseous form of nitrogen) plays an important role for N2O production (Granli and Bockman 1994). N2O emission is accounting for approximately 57% of total annual global GHG emission (IPCC, 2006). During synthesis of chemical fertilizers such as ammonia, phosphoric acid, and nitric acid, all emit greenhouse gases (Kongshaug, 1998). In maize and wheat, the regression analysis for yield and fertilizer doses indicated substantial variation in yield with respect to fertilizer doses (R2 value of 0.91 and 0.92, respectively).

TABLE 3. Response of various level of nitrogen application on GHG emission (kg ha−1) from various sources of farm (maize-wheat cropping system).
Correlation Matrix
Maize
Correlation coefficient(r) among yield, total GHG emission, and various soil properties were presented in Table 4. Yield was positively correlated with GHG emission (r = 0.832**) and negatively correlated with available P and K (−0.779** and −0.940**, respectively). Available P content in soil had negative relationship with GHG emission (r = −0.610*) while positively correlated with yield (r = 0.739**). Available K was significantly and negatively correlated with GHG emissions (−0.749**) and yield (−0.940**).
Wheat
Coefficient (r) values in wheat were illustrated in Table 5, with respect to produce and different variables. It was observed that yield had significant and positive association with GHG emission (r = 0.889**). Available P and available K showed significant and negatively correlation with yield (r = −0.739** and −0.649*) and GHG (−0.730** and −0.770**). The correlation coefficient among soil pH, yield (r = −0.860**), and GHG emission (r = −0.868**) was calculated and observed to be a significant and negative correlation, thus it could be stated that increased amounts of N application increases the amount of yield and GHG emission. Similarly, soil’s available P registered a significant and negative correlation with GHG emission (r = −0.730**), which had a positive “r” value with pH (r = 0.710*). Soil available K was found to have a negative relation with GHG emission (r = −0.770**), and positively correlated with available P (r = 0.780**) and pH (r = 0.799**).
The fertilizer recommendation and GHG emission mitigation for the entire cropping system may be given by using the prediction equation with soil test value estimated after harvesting of crops and GHG emission. In derived equations, the relationships are among GHG emission, soil properties after harvest, applied fertilizer quantity, and yield of maize and wheat (Table 6). These equations yielded R2 values significant at 5% of important parameters. Such can be used for prediction of GHG emission, yield of crop, and status of available P and K after crop harvest can be predicted using regression equations and accordingly, optimum fertilizer recommendation can be made with the perspective of environment (Verma and Singh, 1991; Bera et al., 2006). Table 7 stated that split application of N at 160 kg ha−1 to maize (basal, knee high, and tasseling stage) along with 100 kg P2O5 + 100 kg K2O ha−1 as basal and split application of N at 100 kg ha−1 to wheat (basal, crown root initiation, and panicle initiation stage) along with 90 kg P2O5 + 80 kg K2O ha−1 as basal could be the most effective in terms of yield (71.76 and 41.97 qha−1, respectively, Table 7), GHG emission (2,109.1 and 1,650.2 kgCO2 eq ha−1, respectively), economic benefit, and available nutrient status of soil.
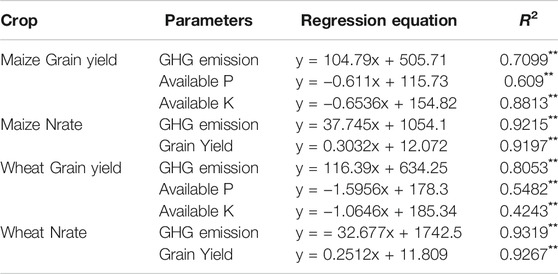
TABLE 6. Regression equation among different parameters (yield of maize and wheat with GHG emission, available P and K in soil, and various doses of nitrogen to GHG emission).
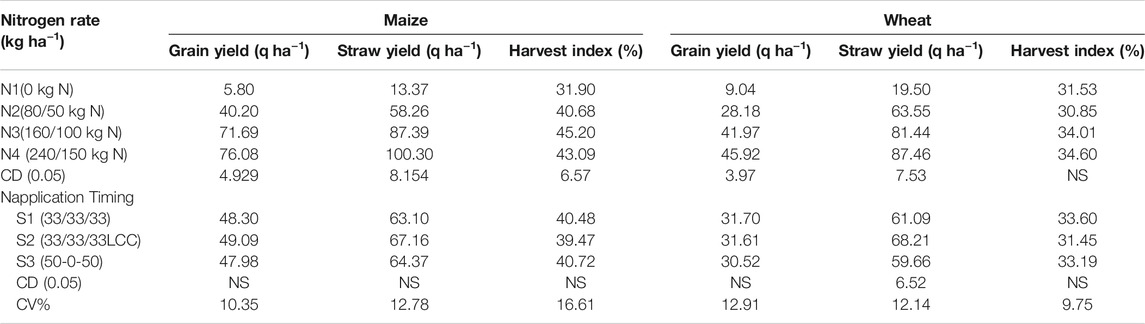
TABLE 7. Response of various doses and time of nitrogen application on yield (q ha−1) and harvest index.
Conclusion
Direct measurement of the GHG is comparatively costlier. The GHG measurement through modeling provides not only the economic options, but variation of the key controlling factors can also be used to trap the minute changes in the GHG effluxes. Therefore, the present investigation is an attempt to utilize the Cool Farm Tool to optimize the N fertilizers and its subsequent effect on the GHG effluxes. In this study, greenhouse data (2015-16 and 2016-17) under maize-wheat cropping system was used to estimate CO2 and N2O emission from maize and wheat crops at four different levels of N fertilizer using cool farm tool model. Results stated that emissions of CO2 per hectare varied from 331.4 to 1,088.3 kg in maize and 292.3–765.3 kg in wheat on application of different doses of N. The total GHG emission in maize crops ranged from 859.5 to 3,003.4 kg CO2 eq per hectare with the application of nitrogen at varying levels (0–240 kg N per hectare). The highest N2O efflux (0.368 kg per ton) was observed at 240 kg N per hectare under wheat crop. The total on-farm emissions, through fertilizer production, account for about 33.7%, and emission of N2O contributes only 65.9%, whereas pesticides account for merely 0.4% under maize-wheat cropping. This study confirms that the direct emission of N2O was totally dependent on N fertilizers application rate; however, the indirect emission was controlled by the fuels and energy consumption. This study establishes the efficacy of nutrient expert (N-management) rather make a prediction in implementing of site-specific nutrient management to smallholder production systems to enhance the crop yields and improve the increment of farmers’ income considering to minimize GHG emission.
Data Availability Statement
The original contributions presented in the study are included in the article/supplementary material, further inquiries can be directed to the corresponding author.
Author Contributions
RK carried out the study and organized the field experiment wrote the first draft of the manuscript. SK performed statistical analysis in collaboration with AM and JS. RK compiled the raw data. AbK provided consultation on statistical analysis of N2O emission data with consultation ArK. All authors are taken keen interest during the preparation of the manuscript.
Conflict of Interest
The authors declare that the research was conducted in the absence of any commercial or financial relationships that could be construed as a potential conflict of interest.
Publisher’s Note
All claims expressed in this article are solely those of the authors and do not necessarily represent those of their affiliated organizations, or those of the publisher, the editors and the reviewers. Any product that may be evaluated in this article, or claim that may be made by its manufacturer, is not guaranteed or endorsed by the publisher.
Acknowledgments
Authors are very much thankful to Director and Dy-Director IPNI (International Plant Nutrient Institute), South East Asia (India Programme), for providing financial assistance to BAU Ranchi, Jharkhand, India.
References
Aryal, J. P., Sapkota, T. B., Jat, M. L., and Bishnoi, D. (2015). On-farmeconomicandenvironmental Impact of Zero Tillage Wheat: a Case of North-West India. Exp. Agric. 51, 1–16. doi:10.1017/S001447971400012X
Bera, R., Seal, A., Bhattacharyya, P., Das, T. H., Sarkar, D., and Kangjoo, K. (2006). Targeted Yield Concept and a Framework of Fertilizer Recommendation in Irrigated rice of Subtropical India. J. Zhejiang Univ. 7, 963–968. doi:10.1631/jzus.2006.B0963
Bhatia, A., Pathak, H., Jain, N., Singh, P. K., and Singh, A. K. (2005). Global Warming Potential of Manure Amended Soils under rice-wheat System in the Indo Gangetic plains. Atmos. Environ. 39, 76–84. doi:10.1016/j.atmosenv.2005.07.052
Bhattacharyya, R., Bhatia, A., Das, T. K., Lata, S., Kumar, A., Tomer, R., et al. (2018). Aggregate-associated N and Global Warming Potential of Conservation Agriculture-Based Cropping of maize-wheat System in the north-western Indo-Gangetic Plains. Soil Tillage Res. 182, 66–77. doi:10.1016/j.still.2018.05.002
Chai, R., Ye, Xinxin., Ma, Chao., Wang, Q., Tu, Renfeng., Zhang, L., et al. (2019). Greenhouse Gas Emissions from Synthetic Nitrogen Manufacture and Fertilization for Main upland Crops in China. Carbon Balance Manage. 14, 20. doi:10.1186/s13021-019-0133-9
EPA (2005). Average Carbon Dioxide Emissions Resulting from Gasoline and Diesel Fuel. EPA420-F-05-001February 2005.
Fagodiya, R. K., Pathak, H., Kumar, A., Bhatia, A., and Jain, N. (2017). Global Temperature Change Potential of Nitrogen Uses in Agriculture: a 50-year Assessment. Sci. Rep. 7, 1–8. doi:10.1038/srep44928
Fagodiya, R. K., Pathak, H., Bhatia, A., Jain, N., Gupta, D. K., Kumar, A., et al. (2019). Nitrous Oxide Emission and Mitigation from maize–wheat Rotation in the Upper Indo-Gangetic Plains. Carbon Manag. 10, 489–499. doi:10.1080/17583004.2019.1650579
Fagodiya, R. K., Pathak, H., Kumar, A., Bhatia, A., Jain, N., and Malyan, S. K. (2020a). Global Warming Impacts of Nitrogen Use in Agriculture: an Assessment for India since 1960. Carbon Manag. 11 (3), 291–301. doi:10.1080/17583004.2020.1752061
Granli, T., and Bockman, O. C. (1994). Nitrous Oxide from Agriculture. Norwegian J. Agric. Sci. Suppl. 12, 128.
Gupta, D. K., Bhatia, A., Kumar, A., Chakrabarti, B., Jain, N., and Pathak, H. (2015). Global Warming Potential of rice (Oryza Sativa)-wheat (Triticum aestivum) Cropping System of the Indo-Gangetic Plains. Indian J. Agric. Sci. 85, 807–816.
Halvorson, A. D., DelGrosso, S. J., and Reule, C. A. (2008). Nitrogen, Tillage, and Crop Rotation Effects on Nitrous Oxide Emissions from Irrigated Cropping Systems. J. Environ. Qual. 37 (4), 1337–1344. doi:10.2134/jeq2007.0268
Hillier, J., Walter, C., Malin, D., Garcia-Suarez, T., Mila-i-Canals, L., and Smith, P. (2011). Afarm-focused Calculator for Emissions from Crop and Livestock Production. Environ. Model Softw. 26, 1070–1078. doi:10.1016/j.envsoft.2011.03.014
Hoben, J. P., Gehl, R. J., Robertson, G. P., Millar, N., and Grace, P. R. (2010). On-farm Nitrous Oxide Response to Nitrogen Fertilizer in Corn Cropping Systems. In review.
Holka, M., and Bienkowski, J. (2020). Carbon Footprint and Life-Cycle Costs of Maize Production in Conventional and Non-inversion Tillage Systems. Agronomy 10, 1877. doi:10.3390/agronomy10121877
IPCC (2006). 2006 IPCC Guidelines for National Greenhouse Gas Inventories, Prepared by the National Greenhouse Gas Inventories Programme. Editors. . Eggleston, L. Buendia, K. Miwa, T. Ngara, and K. Tanabe. Published: (Japan: IGES).
IPCC (2014). Intergorvernmental Panel on Climate Change, Fifth Assessment Report Onclimatechange. Cambridge: Cambridge University Press.
Jain, N., Arora, P., Tomer, R., Mishra, S. V., Bhatia, A., Pathak, H., et al. (2016). Greenhouse Gases Emission from Soils under Major Crops in North West India. Sci. Total Environ. 542, 551561. doi:10.1016/j.scitotenv.2015.10.073
Jat, M. L., Singh, B., and Gerard, B. (2014). Nutrient Management and Use Efficiency in Wheat Systems of South Asia. Adv. Agron. 129, 171–259. doi:10.1016/b978-0-12-800137-0.00005-4
Kongshaug, G. (1998). “Energy Consumption and Greenhouse Gas Emissions in Fertilizer Production,” in IFATechnical Conference Marrakech Morocco.
Kool, A., Marinussen, M., and Blonk, H. (2012). “LCI Data for the Calculation Tool Feed Print for Greenhouse Gas Emissions of Feed Production and Utilization,” in GHG Emissions of N, P and K Fertilizer Production. Editor B Consultants.
Kumar, A., and Kumar, M. (2020b). Estimation of Biomass and Soil Carbon Stock in the Hydroelectric Catchment of India and its Implementation to Climate Change. J. Sustainable For. 39 (6). doi:10.1080/10549811.2020.1794907
Kumar, A., and Sharma, M. P. (2017a). Estimation of Greenhouse Gas Emissions from Koteshwar Hydropower Reservoir, India. Environ. Monit. Assess. 189 (5), 240. doi:10.1007/s10661-017-5958-7
Kumar, A., and Sharma, M. P. (2017b). Estimation of Greenhouse Gas Emissions from Koteshwar Hydropower Reservoir, India. Environ. Monit. Assess. 189 (5), 240–261. doi:10.1007/s10661-017-5958-7
Kumar, A., Tomer, R., Bhatia, A., Jain, N., and Pathak, H. (2016a). “Greenhouse Gas Mitigation in Indian Agriculture,” in Climate Change and Agriculture Technologies for Enhancing Resilience. Editors H. Pathak, and B. Chakrabarti (New Delhi: ICAR-IARI), pp137–149.
Kumar, A., Sharma, M. P., and Kumar, A. (2016b). Greenhouse Gas Emissions from Hydropower Reservoirs: Policy and Challenges. Int. J. Renew. Energ. Res. 6 (2), 472–476.
Kumar, A., Sharma, M. P., and Yang, T. (2018). Estimation of Carbon Stock for Greenhouse Gas Emissions from Hydropower Reservoirs. Stochastic Environ. Res. Risk Assess. 32 (10), 3183–3319. doi:10.1007/s00477-018-1608-z
Kumar, A., Medhi, K., Fagodiya, R. K., Subrahmanyam, G., Mondal, R., Raja, P., et al. (2020a). Molecular and Ecological Perspectives of Nitrous Oxide Producing Microbial Communities in Agro-Ecosystems. Rev. Environ. Sci. Biotechnol. 19, 717–750. doi:10.1007/s11157-020-09554-w
Lal, R. (2004). Carbon Emission from Farm Operations. Environ. Int. 30, 981–990. doi:10.1016/j.envint.2004.03.005
Majumdar, K., Jat, M. L., Pampolino, M., Dutta, S., and Kumar, A. (2013). Nutrient Management in Wheat: Current Scenario, Improved Strategies and Future Research Needs in India. J. Wheat Res. 4, 1–10.
Malyan, S. K., Smita, S. K., Fagodiya, R. K., Ghosh, P., Kumar, A., Singh, R., et al. (2021). Biochar for Environmental Sustainability in the Energy-Water-Agroecosystem Nexus. Renew. Sustainable Energ. Rev. 149, 111379. doi:10.1016/j.rser.2021.111379
McCann, J. C. (2007). Maize and Grace: Africa’s Encounterwith a New World Crop. Cambridge: Harvard University Press, 1500–2000.
Millar, N., Robertson, G. P., Grace, P. R., Gehl, R. J., and Rowlings, D. (2010). The Response of N2O Emissions to Incremental Nitrogen Fertilizer Addition in winter Wheat. In review.
Nelson, G. C., Robertson, R., Msangi, S., Zhu, T., Liao, X., and Jawajar, P. (2009). Greenhouse Gas Mitigation Issues for Indian Agriculture. IFPRID iscussion Paper00900, 1–52.
Pampolino, M. F., Witt, C., Pasuquin, J. M., Johnston, A., and Fisher, M. J. (2012). Developmental Approach Ande Valuation of the Nutrient Experts Software for Nutrien T Management in Cereal Crops. Comput. Electron. Agric. 88, 103–110. doi:10.1016/j.compag.2012.07.007
Parihar, C. M., Jat, S. L., Singh, A. K., Hooda, K. S., Chikkappa, G. K., Singh, D. K., et al. (2011). Maizeproductiontechnologiestechnicalbulletin2011/3. NewDelhi: DirectorateofMaizeResearch, 36.
Pathak, H., Bhatia, A., Jain, N., and Aggarwal, P. K. (2010). “Greenhouse Gas Emission and Mitigation in Indian Agriculture– A Review,” in ING Bulletins on Regional Assessment of Reactive Nitrogen. Bulletin No.19.
Pathak, H., Jain, N., Bhatia, A., Kumar, A., and Chatterjee, D. (2016). Improved Nitrogen Management: a Key to Climate Change Adaptation and Mitigation. Indian J. Fertil. 12 (11), 151–162.
Rochette, P., Worth, D. E., Lemke, R. L., McConkey, B. G., Pennock, D. J., Wagner-Riddle, C., et al. (2008). Estimation of N2O Emissions from Agricultural Soils in Canada. I. Development of a Country-specific Methodology. Can. J. Soil Sci. 88, 641–654. doi:10.4141/cjss07025
Sapkota, T. B., Jat, M. L., Rana, D. S., Khatri-Chhetri, A., Jat, H. S., Bijarniya, D., et al. (2021). Crop Nutrient Management Using Nutrient Expert Improves Yield, Increases Farmers’ Income and Reduces Greenhouse Gas Emissions. Sci. Rep. 11, 1564. doi:10.1038/s41598-020-79883-x
Sharma, A., Kumar, S., Khan, S. A., Kumar, A., Mir, J. I., Sharma, O. C., et al. (2021). Plummeting Anthropogenic Environmental Degradation by Amending Nutrient- N Input Method in Saffron Growing Soils of north-west Himalayas. Sci. Rep. 11, 2488. doi:10.1038/s41598-021-81739-x
Sharma, U. C. (2020). Methane and Nitrous Oxide Emissions from Livestock in India: Impact of Land Use Change. J. Agric. Aquacult. 2 (1).
USDA (2019). Crop Explorer. Available from: https://ipad.fas.usda.gov/cropexplorer/util/new_get_psd_data.aspx?regionid=sasia.
Verma, D., and Singh, K. D. (1991). Changes in Nutrient Status of Soil Caused by Cropping and Fertilization inTypicUstrochrept. Fertilizer Res. 29, 267–274. doi:10.1007/bf01052395
World Bank (2014). Electric Power Transmission and Distribution Losses (% of Output). Available from: https://data.worldbank.org/indicator/EG.ELC.LOSS.ZS.
Keywords: maize, wheat, carbon dioxide, greenhouse gases, nitrous oxide, nitrogen fertilizer
Citation: Kumar R, Karmakar S, Minz A, Singh J, Kumar A and Kumar A (2021) Assessment of Greenhouse Gases Emission in Maize-Wheat Cropping System Under Varied N Fertilizer Application Using Cool Farm Tool. Front. Environ. Sci. 9:710108. doi: 10.3389/fenvs.2021.710108
Received: 15 May 2021; Accepted: 10 August 2021;
Published: 16 September 2021.
Edited by:
Jahangeer A. Bhat, Fiji National University, FijiReviewed by:
Sandeep K. Malyan, National Institute of Hydrology, IndiaGopal Shukla, Uttar Banga Krishi Viswavidyalaya, India
Copyright © 2021 Kumar, Karmakar, Minz, Singh, Kumar and Kumar. This is an open-access article distributed under the terms of the Creative Commons Attribution License (CC BY). The use, distribution or reproduction in other forums is permitted, provided the original author(s) and the copyright owner(s) are credited and that the original publication in this journal is cited, in accordance with accepted academic practice. No use, distribution or reproduction is permitted which does not comply with these terms.
*Correspondence: Rakesh Kumar, rkssacbau@rediffmail.com