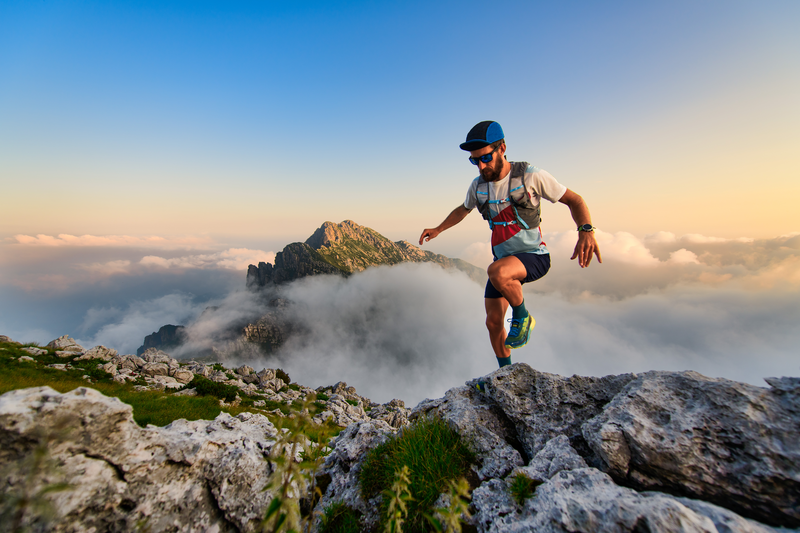
95% of researchers rate our articles as excellent or good
Learn more about the work of our research integrity team to safeguard the quality of each article we publish.
Find out more
ORIGINAL RESEARCH article
Front. Environ. Sci. , 02 December 2021
Sec. Water and Wastewater Management
Volume 9 - 2021 | https://doi.org/10.3389/fenvs.2021.706730
This article is part of the Research Topic Resource Recovery from Wastewater Treatment View all 4 articles
The aim of this research was to assess the simultaneous effect of zeolite and bischofite on estruvite production in an anaerobic digester treating pig slurry as substrate. Three ratios (5:1, 6:1 and 7:1) of Mg-P were used for evaluating the effect of only bischofite on anaerobic digestion. For assessing the simultaneous effect of zeolite and bischofite on anaerobic digestion, three mass ratios of zeolite:bischofite (1, 5 and 10%) were used. As results, bischofite as Mg+2 source served to decrease the total phosphorous (TP) concentration in the anaerobic digestion of pig manure without affecting the maximum methane production rate or methane yield of the system. An average 82.5% TP removal was found in the reactors with bischofite during the first 5 days of digestion. Nevertheless, bischofite increased the lag-phase of the system. The simultaneous presence of zeolite and bischofite (1% ratio with respect to bischofite) caused a TP removal of 65.6% and increased the methane yield by up to 19.9% compared to a system without zeolite or bischofite. Thus, it is feasible to use bischofite and zeolite as enhancers for the simultaneous production of biogas and struvite inside an anaerobic digester treating piggery wastewater.
Piggery slurry is a waste that contains a mixture of feces, urine and water, together with other wastes from animal husbandry such as straw, sawdust and food residues. One of the most common treatments applied to piggery slurry is anaerobic digestion (AD), which has several advantages over other purification processes: it decreases greenhouse gas emissions, mineralizes organic matter, produces an effluent with good fertilizing qualities and, above all, allows recovery of carbon through methane production (Romero-Güiza et al., 2015). Effluents from an anaerobic digester still contain high amounts of nitrogen (as total ammonia nitrogen, TAN) and phosphorous (as phosphate), which make them suitable for use as fertilizers. However, the huge volume generated by the industry cannot be used entirely as fertilizers so other technologies for reducing the nitrogen and phosphorous concentrations are necessary.
One way to recover not only nitrogen, but also phosphorous, is struvite precipitation. Struvite can be considered a by-product of the anaerobic digestion that appears spontaneously in turbulent areas of digesters or accessory pipes and pumps (Li et al., 2019). In turbulent zones a great amount of carbon dioxide is removed, causing an increase in pH to values which favor the reaction between Mg2+, PO43− and NH4+ and produce magnesium ammonium phosphate (Li et al., 2019). Struvite is a crystal in which Mg2+, NH4+, and PO43− are combined in the molar ratio or stoichiometric proportions of 1:1:1. Struvite production from anaerobic digestate is a successful procedure to remove and recycle nutrients to nature because it is a good slow-release fertilizer due to its contents in Mg, P and N (Tao et al., 2016). A better option could be to reduce the N and P contents inside the anaerobic digester in order to decrease the ammonia content, which inhibits the AD process at certain concentrations. However, few attempts at investigating this option have been reported in the literature. Lee et al. (2004) studied the improvement of food waste anaerobic digestion by adding Mg2+ in the system. They achieved a PO4−3-P removal of 65% when applying Mg2+, along with an improvement in biogas production due to the decrease in NH3 in the bulk liquid. Uludag-Demirer et al. (2008) proposed the coupling of AD and controlled struvite precipitation in the same reactor to minimize the nitrogen removal costs and increase the performance of the AD using MgCl2∙6H2O. They found that both organic matter and ammonia were lower in the system with Mg2+ addition. Romero-Romero-Güiza et al. (2015) evaluated the feasibility of combining anaerobic digestion and struvite precipitation in the same reactor through five different magnesium sources. They found that high concentrations of all sources of Mg2+ can inhibit the anaerobic digestion due to an increase in K+ and pH, thus requiring the use of a solid stabilizer (low-grade magnesium oxide mixed with phosphoric acid) in order to maintain the anaerobic digestion activity. Sánchez-Sánchez-Ramírez et al. (2019) studied uncontrolled phosphorous precipitation in anaerobic digesters under thermophilic and mesophilic conditions, and reported that it was possible to obtain struvite in bioreactors at pilot-scale when the influent brings enough Mg2+. Yuan et al. (2019) also studied the addition of MgCl2 to an anaerobic digester in addition to biogas recirculation in order to improve the retention of N and P in the sludge. They found that Mg2+ addition serves to retain 87% orthophosphate and 19% ammonia N in the solid phase of digested sludge. As can be seen, the focus was placed more on the decrease in ammonia N in order to improve methane generation than on phosphorous removal. Furthermore, the Mg2+sources were salts, which are expensive and decrease the economy of the process (Luo et al., 2018). The key for economical nutrient removal from AD is the utilization of an economical source of magnesium such as brine, waste from magnesium oxide production from magnesite or bischofite by-product from lithium production (Li et al., 2019). The last by-product has a magnesium concentration close to 12% in the form of magnesium chloride and has not been used up to now as a source of Mg2+ for struvite precipitation.
On the other hand, it has been demonstrated that struvite crystallization could be improved by the utilization of seeds for nucleation which favor the growth of crystals (Li et al., 2019). According to Li et al. (2019), various seeds such as lignite, egg shell powder, quartz sand and zeolite have been used for this purpose. Among them, zeolite has shown several advantages for use in an anaerobic reactor because of its capacity for adsorption of ammonia (Montalvo et al., 2020). Indeed, several works (Achi et al., 2020; Cardona et al., 2021) have shown its capacity for improving anaerobic digestion, not only by increasing methane production, but also in Chemical Oxygen Demand (COD) removal. Therefore, it is possible that zeolite could help to improve not only anaerobic digestion but also the nucleation of struvite inside the bioreactor.
Finally, another aspect to be considered is the pH value within the anaerobic digester. For struvite precipitation, optimal pH values are around 8.5 (Kataki et al., 2016); however, anaerobic digestion works adequately at pH values of around 7–7.5, a situation that could decrease and hinder the feasibility of struvite precipitation inside the bioreactor. Nevertheless, the addition of a seed such as zeolite could help to maintain the precipitation of struvite in the bioreactor despite the low pH values.
Based on these three points, the aim of the present work was the removal of P through struvite precipitation with the application of bischofite, a novel source of magnesium, and the utilization of zeolite as nucleation site for struvite crystallization, both used inside an anaerobic digester. Despite its interest, and to the best of our knowledge, this matter has not been reported in the literature up to now.
Piggery wastewater was used as substrate. It was obtained from a piggery farm (AASA Chile). Its characteristics are shown in Table 1. The anaerobic inoculum was obtained from an industrial anaerobic digester that uses piggery wastewater as substrate in the same piggery farm (AASA). The biodigester works at an hydraulic retention time of 30 days, with a solid concentration of 45–50 g/L of Volatile Suspended Solids (VSS). This digester is located in Melipilla, Metropolitan Region, Chile. The characteristics of the anaerobic inoculum are also presented in Table 1.
Bischofite was used as the Mg2+-source (CAS Number 7791-18-6). Bischofite is a white solid from Lithium mining. The main components of the bischofite in % w/w were: Mg+2: 10–12.8; Cl−: 29–40; Li+: 0.1–0.6; other components (Ca2+, Na+, K+, Ba2+, SO42−): 1–10. Therefore, it was considered that 100 g of bischofite are equivalent to 11.4 g of Mg on average. The zeolite used in the experiments was a mixture of clinoptilolite and mordenite from the enterprise “Zeolitas Maule” with a particle size of 0.25–0.42 mm. Full details concerning zeolite are reported in Huiliñir et al. (2020).
The biochemical methane potential (BMP) tests were conducted at mesophilic temperature (35 ± 1°C) in 300 ml glass reactors with a working volume of 200 ml. The digester temperature was maintained using automatically controlled aquarium heaters. Each bottle was fed with an appropriate amount of piggery wastewater and inoculum to maintain the ratio g TVS of wastewater/g TVS of inoculum at 1. All vessels were properly sealed with rubber stoppers and silicone to ensure anaerobic conditions and then covered with aluminum foil to avoid the growth of photosynthetic organisms. Afterwards, the reactors were connected to graduated cylinders filled with a solution of NaOH (3% w/w) to dissolve CO2 from the biogas. Finally, the volume of methane was measured by liquid displacement according to Huiliñir et al. (2015).
The effect of bischofite was studied with three different Mg2+:PO43− molar ratios: 5:1, 6:1 and 7:1, taking into account that the amount of Mg2+ in the bischofite was only 11.4%. A control system was used, with only substrate and inoculum. Twelve replicates were used for each ratio (5:1, 6:1 and 7:1), sacrificing 8 of them for the analysis of the liquid phase. The other four reactors were not opened, but used for the analysis of the gaseous phase. In the liquid phase, the following parameters were measured: soluble chemical oxygen demand (sCOD), total ammonia nitrogen (TAN), volatile suspended solids (VSS), total phosphorous as phasphate (TP) and pH.
The simultaneous effect of bischofite and zeolite was studied with three different bischofite-zeolite mass percentage ratios: 1, 5 and 10%. These percentages were considered with respect to the mass of bischofite added to each assay. For instance, if 100 g of bischofite were used, then 1 g of zeolite was used for obtaining a ratio of 1%. Here, a control system was also used, with only substrate and inoculum in order to compare the effect of these two components. Twelve replicates were used for each ratio, sacrificing 8 of them for the analysis of the liquid phase. The other four reactors were not opened, but used for the analysis of the gaseous phase. In the liquid phase, the following parameters were measured: soluble chemical oxygen demand (sCOD), total ammonia nitrogen (TAN), volatile suspended solids (VSS), total phosphorous as phosphate (TP) and pH.
The t-Student statistical analysis with α = 0.05 was used to compare data between the control system and the assays with different ratios of bischofite and zeolite. This analysis was performed using the 2010 Excel software.
In order to evaluate the effect of bischofite and zeolite on the anaerobic digestion process, a kinetic study was performed. The model used was the Modified-Gompertz model, which has been used extensively in this type of study (Baghbanzadeh et al., 2021; Marañón et al., 2021; Qi et al., 2021). This model is given for the following equation:
where A represents the potential methane production or ultimate methane production (mL CH4/g TVSadded), µm is the maximum methane production rate (mL CH4/g TVS d), λ is the lag time phase (d), y is the accumulated methane volume at time t (mL CH4/g TVSadded), t is the measured time (d), and e is the base of the natural logarithm [2.718282].
The model presented was used to obtain the calculated values of specific methane volume (mL CH4/g TVSadded) for every time at which experimental specific methane volume values were measured. The parameters of the kinetic model were then calculated by minimizing the estimated error variance using the ‘‘Solver” tool from Microsoft Excel 2010. The estimated error variance was calculated as:
where
The following parameters were determined in the liquid phase: soluble COD (sCOD), total and suspended solids, volatile suspended solids, pH, total ammonia nitrogen (TAN) and total phosphorous as phosphate (TP). All these parameters were determined according to the Standard Methods of the American Public Health Association Standard Methods (APHA, 2012). Total ammonia nitrogen (TAN) was determined by flow injection analysis based on the Berthelot reaction (QuikChem® Method). The total solids and volatile solids were determined after drying to constant weight at 105°C ( ∼ 24 h) and 550°C ( ∼ 2 h). COD was measured according to the colorimetric standard method 5220D (APHA, 2012). pH was determined using a Crison model 20 Basic pH-meter. Total phosphate was measured through a phosphate kit from HANNA Instruments, which reacts with the HI93717 reactive and is then measured by spectrophotometry for high-range phosphate HI96717.
The effect of bischofite on the anaerobic digestion of piggery wastewater is shown in Figure 1. As can be seen in Figure 1A, it was possible to obtain methane for all the bischofite concentrations studied, with similar volumes to the volume obtained from the control (without bischofite), especially at the end of the digestion process. The methane profiles obtained using bischofite were similar for the different concentrations used: first 10 days of a slow methane production, a fast production from day 10 to 22 and then a slight decrease in methane production until day 28. According to the statistical analysis, the tendency has not significant differences between each bishofite condition. This behavior was different with respect to the control assay. The control produced a higher methane volume during the first 10 days and after that the methane production rate was lower than the system with bischofite. However, according to the t-student analysis, the tendency of the control has not significant differences with respect to each bishofite condition. It is important to note that the maximum methane volumes produced in all the assays, including the control, obtained similar values of between 365 and 421 ml CH4/g VS. Therefore, the effect of bischofite on anaerobic digestion was more pronounced on the kinetics of the process, increasing the lag phase of the anaerobic digestion process. Several authors have also reported that different Mg2+ sources affected anaerobic digestion by increasing the lag phase or decreasing the maximum potential volume with respect to the control (Romero-Güiza et al., 2015). It is known that Mg2+ is an inorganic cation that is present in the methanogenic archaea, acting as cofactor in some enzymatic reactions and reducing Na toxicity in the methanogenesis (Tao et al., 2016; Li et al., 2019).
FIGURE 1. (A): Variation in methane volume with digestion time in the assays at different bischofite: P ratios. (B): Total phosphorous profiles with different bischofite: P ratios. (C): Soluble COD profiles with different bischofite: P ratios. (D): Volatile suspended solid (VSS) profiles with different bischofite: P ratios.
However, the effect of Mg2+ on the anaerobic digestion shows contradictory results in the literature. Negative effects were found by Romero-Güiza et al. (2015) and Uludag-Demirer et al. (2008), who indicated that the use of MgCl2 at concentrations of 3.3 g/L (N:P:Mg ratio = 1) and MgCl2·6H2O at concentrations between 1.75 and 7.00 g/L generated a significant decrease in methane production. On the contrary, Lee et al. (2004) also studied the use of MgCl2, but found that by applying this compound at pH = 7.7, methane production was improved by up to 50% because of the decrease in ammonia inhibition in the anaerobic digestion process. Similar findings were reported by Farrow et al. (2017) using poultry manure as substrate and MgCl2∙6H2O as Mg2+ source, although in this case the struvite precipitation was performed in a different reactor, not inside the biodigester. Recently, Koirala et al. (2021) studying anaerobic co-digestion (ACoD) of food waste (FW) and domestic wastewater (DWW) in an UASB reactor indicated that the Mg2+ addition at a concentration of 150 mg/L improved the performance of the co-digestion of these two substrates, increasing methane production by 10.63%. Finally, Yuan et al. (2019) used sewage sludge as substrate and indicated that MgCl2 as Mg+2 source did not affect anaerobic digestion, even at high concentrations (1.428 g/L). In our case, anaerobic digestion was not stimulated or inhibited, but it affected the kinetics of the process, increasing the lag-phase of the reaction, in spite of the high P:Mg ratio used in the present research.
The modified-Gompertz model was used in an in-depth study on the effect of bischofite on the process kinetics. Table 2 shows the model parameters obtained for the different bischofite concentrations tested. As can be seen, the maximum or ultimate methane yield (A) in the control was higher than those obtained in the other experiments in presence of bischofite; although with a 7:1 ratio the decrease in the A value compared to the control was practically negligent. The decrease was more pronounced with a ratio of 5:1, with a −20.63% variation compared to the control; while with a ratio of 6:1 the variation was around 12%. Regarding the maximum specific methane production rate (μm), it increased in all the assays with bischofite compared to the control. The highest increase was achieved with a ratio of 7:1, with 48.09% improvement compared to the control. With ratios of 5:1 and 6:1, the increase was around 23%, with very similar values between them. Finally, the length of the lag period increased substantially, between 150 and 272%, compared to the value obtained for the control. The highest λ obtained was with a ratio of 7:1; while the lowest increase was obtained with a 5:1 ratio.
TABLE 2. Kinetic parameters obtained from Gompertz-modified model applied for the different bischofite concentrations tested in this anaerobic digestion process.
In general, the kinetic analysis showed that bischofite had a strong effect on the start-up of the anaerobic process, although the effects on ultimate methane production or maximum methane yield were not great. These effects can be attributed to the higher presence of Mg2+ in the digester with bischofite, which could negatively affect the anaerobic digestion process because of a possible higher ionic strength in the digester with bischofite (Zhao et al., 2018). Nevertheless, the biomass was able to recover its activity and the methane yield was not affected because the concentration obtained in this work was around 280 mg/L of Mg2+, while inhibitory effect was observed at concentrations higher than 30 g/L MgCl2 (Zhao et al., 2018). The same behavior was observed by Romero-Güiza et al. (2015), who also showed that concentrations below 400 mg/L Mg2+ did not affect the anaerobic digestion process. It is important to note that bischofite contains other impurities that could also affect the anaerobic digestion process. More studies are required to elucidate these aspects.
As can be seen in Figure 1B the TP decreased in a higher percentage in all the assays in the presence of bischofite in comparison to the system with no bischofite (control). No significant differences were obtained among the different bischofite concentrations used, as was confirmed by the statistical analysis (t-student); however, the control showed significant statistical differences regarding to the different bischofite: P ratios. There is an erratic behavior in the control system, with increases and decreases in concentration; however, the tendency is clearly towards the decreasing. A possible explanation of this behavior could be the release and precipitation of P due to the equilibrium on different solids, such as struvite, with the Mg2+ that comes from the susbtrate. At ratio 5:1, the system obtained the lowest TP concentration at the end of the experiment. On the other hand, the highest drop in the TP concentration in the systems with bischofite was produced during the first 5 days, where TP decreased from 220 mg/L to an average value of 55 mg/L, which entails a 82.5% removal during this time period. This fast decrease showed that the TP removal was related to a fast chemical reaction involving the struvite formation instead of a biological reaction. The system with no bischofite presented a decrease of 35% in the TP concentration which could be related to the formation of struvite with the Mg2+ that comes from the susbtrate, as was mentioned previously. Therefore, although the TP is removed in anaerobic digestion without external sources of Mg2+, the presence of bischofite improved TP removal by 50%.
According to these results on TP concentration, the presence of bischofite with all the concentrations studied promoted a higher decrease in the TP concentratration in the process, probably because of the struvite formation (See Supplementary Figure S1 in supplementary data).
Table 3 shows the initial and final pH values in all the assays carried out with different bischofite concentrations. As was expected, there was an increase in the pH value for all anaerobic reactors, but this increase was very similar in the systems with bischofite and the control, so, in this case, the presence of bischofite did not generate significant changes in the pH system. According to Romero-Güiza et al. (2015), the use of Mg(OH)2 increases the pH value in the bioreactor, improving struvite production. However, this increase can also provoke an inhibition in methanogenesis, with no production of methane at the end of the process. In our case, the pH increase was milder and the inhibition of methanogenesis was not produced, indicating that bischofite can be used as a source of Mg2+ without affecting methane generation. On the other hand, the process had a pH lower than the optimal pH value for struvite formation (superior to 8.5), a situation that did not entail struvite formation. This can be explained by the high ion concentrations present in these systems.
The other two aspects that were analyzed in these experiments were the behavior and variation with time in soluble COD (sCOD) and VSS in the bioreactors. Soluble COD decreased in all the assays, without differences between the systems with bischofite and the control (Figure 1C). According to the profiles obtained, there was a strong consumption of sCOD during the first 4–5 days. After that, there was an increase in sCOD during days 5–10. This increase is related to the solubilization and hydrolysis of solids in the system, as can be observed in Figure 1D, where it is shown that the VSS decreased in a higher percentage during the first 10 days. From day 10 up to day 18, there was a strong consumption of sCOD and finally, a stabilization of sCOD concetration took place to achieve a final value of around 3.0 g/L. The very similar sCOD profiles show that the anaerobic degradation of soluble organic matter does not suffer any negative effects by the presence of the bischofite, as was confirmed by the statistical analysis (t-student). This result disagrees with results provided by other researchers. In this sense, Koirala et al. (2021) reported a slight increase in COD removal (10% average) when a source of Mg2+ was supplemented in the anaerobic co-digestion process of food waste and domestic wastewater.
Regarding the variation in VSS concentration with time, Figure 1D shows that the solid degradation was more pronounced during the first 10 days for all the experiments. As was observed for sCOD, no statistical differences were obtained among the assays. Slightly higher VSS concentrations were obtained for the control system and for the system with a 7:1 ratio, with values at around 6 g/L at the end of the assays. With 7:1 and 6:1 ratios, final VSS concentratios were around 4 g/L, showing that a slightly higher VSS removal was obtained with these ratios.
Therefore, the bischofite did not exert any perturbation on the anaerobic digestion system, and the VSS and sCOD removals were similar for systems with and without bischofite.
The very similar sCOD and VSS removal values obtained in the systems with and without bischofite could be explained by the effect of bischofite on the CH4 concentration in the liquid bulk. There is evidence that methane reacts with halogens such as chlorine (Horn and Schlögl, 2015), which is added by the bischofite. Thus, the methane generated could react with Cl− in the liquid bulk, decreasing the methane concentration in the liquid phase and explaining the lower CH4 generation rate in the presence of bischofite.
Thus, bischofite can be used to improve TP removal inside an anaerobic biodigester, without greatly affecting the methane yield. Nevertheless, bischofite negatively affects the methane generation rate, slightly increasing the lag phase.
Once the effect of bischofite was clear, the simultaneous effect of zeolite and bischofite was studied. Figure 2 shows the main results obtained from this set of experiments. The variation in the specific methane volume with time for the different zeolite concentrations used is shown in Figure 2A. As can be seen, all the reactors with zeolite and bischofite achieved a higher methane volume than that obtained for the control reactor at the end of the assays. Furthermore, the methane production rate also appeared to be greater in bioreactors with bischofite and zeolite in comparison to the control. On the other hand, a higher lag phase still appears in the systems with bischofite and zeolite in comparison to the control, although this increase is smaller than that achieved in the system with only bischofite (Figure 1A). Finally, the statistical analysis (t-student) showed that no significant differences were obtained among the different conditions studied.
FIGURE 2. (A): Variation in methane production with time at a ratio P:Mg2+ = 1:5 and different zeolite concentrations (1, 5 and 10%). (B): Total ammonia nitrogen profiles with a ratio P:Mg2+ = 1:5 and different zeolite concentrations (1%, 5% and 10%). (C): pH value profiles with a ratio of P:Mg+2 = 1:5 and different zeolite concentrations (1%, 5% and 10%). (D): Soluble COD profiles with a ratio of P:Mg+2 = 1:5 and different zeolite concentrations (1%, 5% and 10%). (E): Volatile suspended solids (VSS) profiles with a ratio of P:Mg+2 = 1:5 and different zeolite concentrations (1%, 5% and 10%).
In order to quantify these aspects, the kinetics was again evaluated through the Gompertz modified model and the results shown in Table 4. The maximum methane yields (A) in all the systems with zeolite and bischofite were higher than in the control, with increases between 19.92 and 8.36% compared to the control. The highest increase was observed with a ratio of 1%. This behavior was different from that observed in the system with bischofite alone (Table 2), in which the A value obtained was lower in comparison to the control, showing that the simultaneous presence of zeolite was able to improve methane production. Regarding the maximum methane production rate (μm), for all the systems with zeolite and bischofite this parameter was higher than that obtained for the control, with increase percentages varying between 25.97 and 12.44%. This result agrees with the improvement obtained with bischofite alone (Table 2) and shows that zeolite does not affect the improvement in the kinetics obtained from the adding of bischofite in the system. Finally, in relation to the lag-phase (λ), there was still an increase in this phase with respect to the control system, with increases between 98.98 and 315.31%, similar to the behavior found in the system just with bischofite (Table 2). Therefore, according to these results, zeolite mainly improves the methane yield in the systems with bischofite, also providing a higher μm with respect to the control system.
TABLE 4. Kinetic parameters obtained from the Gompertz-modified model applied to the experiments to assess the simultaneous effect of bischofite and zeolite on anaerobic digestion.
The improvement in anaerobic digestion by the presence of zeolite has been widely demonstrated (Wijesinghe et al., 2018; Pérez-Pérez et al., 2021) and it can be explained by three points: a.- Its capacity for immobilizing microorganisms (Fernández et al., 2007) due to its porosity and superficial area; b.- Its capacity for NH4+ adsorption, which decreases the NH3 levels in the liquid bulk and decreases the possible ammonia inhibition in the anaerobic digestion (Montalvo et al., 2020) c.- Its capacity for adsorption of Ca2+ and Mg2+, which enhance the microbial utilization of these cations during the anaerobic digestion (Wijesinghe et al., 2018). This last point could also be useful for struvite production and its formation on the zeolite surface.
Figure 2B shows the profiles obtained for TAN in all the assays, indicating that TAN increased its concentration throughout the assays, a situation explained by the hydrolytic and acidogenesis processes, where proteins and aminoacids were degraded, releasing TAN. In general, the profiles were similar, although the statistical analysis showed that there were statistically significant differences between the control and the reactors with zeolite. TAN concentration in the systems with zeolite and bischofite was slightly lower than the control system, a situation that could be related to the adsorption of TAN on the zeolite and the consumption of N by struvite formation.
Regarding TP, Table 5 shows the initial concentration, final concentration and TP removal percentages obtained in all the assays. It clearly shows that the presence of zeolite and bischofite improved the TP removal when compared to the system without zeolite and bischofite, being that TP removal was around 80% higher in comparison to the control. Nevertheless, the TP removal percentage (67% average) was slightly lower than the removal obtained with bischofite alone, which was around 80% (see Figure 1B). This could be explained by the pH values obtained in reactors with bischofite and zeolite, which were always lower than the control system (Figure 2C) and lower than the values obtained in the systems with only bischofite (Table 3). pH values over 8 allow the struvite to precipitate (Stratful et al., 2001; Huang et al., 2011), while pH values close to the neutrality decreased the feasibility of struvite precipitation. Indeed, according to Wijesinghe et al., 2018 (Wijesinghe et al., 2018), higher pH values favored P removal in the presence of zeolite, because of the precipitacion of P with Ca+2. Therefore, the lower pH values in systems with zeolite and bischofite prevented the increase in TP removal, even with the presence of Mg2+. On the other hand, zeolite has also been used as seeding, which promotes crystal growth by enabling the crystallization of fine struvite particles on the zeolite itself (Li et al., 2019). In our case, this fact can explain the removal of P even though pH values were closer to neutrality in the systems with zeolite.
TABLE 5. Soluble TPs at the beginning and end of the experiments with addition of bischofite to the TP at a ratio of 5:1 and zeolite concentrations of 1, 5 and 10% according to the amount of bischofite added.
The differences in pH values between the control (pH around 7.8 at the end of the assay) and the systems with zeolite and bischofite (pH around 7.4 at the end of the assay) can be attributed to the zeolite’s adsorption capacity. It is known that zeolite can adsorb cations such as NH4+ onto its surface, a situation that decreases the presence of cations in the liquid bulk and allows pH to maintain values closer to neutrality. This situation was also observed by Wijesinghe et al. (2018).
The statistical analysis of pH values (t-student) did not show significant differences between the assays with different zeolite ratios; however, there were statistically significant differences between the control and the systems with zeolite, especially from day 15 onward. The change in the tendency presented by pH from day 15 could be explained by the higher degradation in VSS from day 15 (Figure 2E), a situation that released more TAN which in turn increased the alkalinity of the system.
The behavior of soluble organic matter (measured as soluble COD) is shown in Figure 2D. As can be seen, the profiles are very similar to the profiles obtained in Figure 1 under conditions with bischofite alone, i.e., an increase during the first 8 days due to the solubilization of solids and then a rapid decrease to stabilize its concentration to around 0.8 g/L after 18 days. No significant statistical differences were observed between the systems with and without zeolite and bischofite. This tendency agrees with the methane volume profile, where the highest production was observed from day 8 to 18 (Figure 2A).
It is important to note that the systems with bischofite and zeolite presented higher sCOD removal after day 20, especially with ratios of 1 and 10%. This fact can also explain the highest methane yield obtained at a ratio of 1% and can be related to the presence of zeolite.
The VSS profile is shown in Figure 2E. As can be seen the VSS showed erratic behavior, without a clear tendency or difference between assays. Nevertheless, it is clear that there was a general decrease in VSS concentration under all the conditions studied, with a removal percentage of around 15% for all the assays.
• The bischofite as Mg2+ source serves to decrease the TP concentration in the anaerobic digestion of pig manure, without affecting the maximum methane production rate or methane yield of the system. Nevertheless, bischofite slightly increased the lag-phase of the system.
• The simultaneous presence of zeolite and bischofite (ratio 1% with respect to bischofite) served to obtain a TP removal higher than 65% and improved the methane yield by up to 19.9% with respect to a system without zeolite or bischofite.
The raw data supporting the conclusion of this article will be made available by the authors, without undue reservation.
NP: Methodology, Investigation, formal analysis. AC: Methodology, Investigation. LG: Supervision, Funding acquisition. RB: Formal analysis, Writing—Review and Editing. CH: Conceptualization, formal analysis, Writing—Review and Editing, Supervision, Funding acquisition.
FONDEF Project ID18I10053, FONDECYT project 1170103 and DICYT-USACH.
The authors declare that the research was conducted in the absence of any commercial or financial relationships that could be construed as a potential conflict of interest.
All claims expressed in this article are solely those of the authors and do not necessarily represent those of their affiliated organizations, or those of the publisher, the editors and the reviewers. Any product that may be evaluated in this article, or claim that may be made by its manufacturer, is not guaranteed or endorsed by the publisher.
The authors gratefully acknowledge the financial support provided by CONICYT, Chile through a FONDEF Project ID18I10053 and FONDECYT Project 1170103. Also to the University of Santiago de Chile through DICYT-USACH support. This work is dedicated to the memory of our esteemed and wonderful colleague Dr. Silvio Montalvo Martinez who led this research until his death in October 2019.
The Supplementary Material for this article can be found online at: https://www.frontiersin.org/articles/10.3389/fenvs.2021.706730/full#supplementary-material
Supplementary Figure S1 | Photos of struvite obtained in the bioreactors with an optical microscopy at 25x.
Achi, C. G., Hassanein, A., and Lansing, S. (2020). Enhanced Biogas Production of Cassava Wastewater Using Zeolite and Biochar Additives and Manure Co-digestion. Energies 13 (2), 491. doi:10.3390/en13020491
Apha, A. (2012). “WPCF: Standard Methods for the Examination of Water and Wastewater,” in American Public Health Association. 22th ed. (Washington, D.C.
Baghbanzadeh, M., Savage, J., Balde, H., Sartaj, M., VanderZaag, A. C., Abdehagh, N., et al. (2021). Enhancing Hydrolysis and Bio-Methane Generation of Extruded Lignocellulosic wood Waste Using Microbial Pre-treatment. Renew. Energ. 170, 438–448. doi:10.1016/j.renene.2021.01.131
Cardona, L., Mazéas, L., and Chapleur, O. (2021). Zeolite Favours Propionate Syntrophic Degradation during Anaerobic Digestion of Food Waste under Low Ammonia Stress. Chemosphere 262, 127932. doi:10.1016/j.chemosphere.2020.127932
Farrow, C., Crolla, A., Kinsley, C., and McBean, E. (2017). Anaerobic Digestion of Poultry Manure: Process Optimization Employing Struvite Precipitation and Novel Digestion Technologies. Environ. Prog. Sustain. Energ. 36 (1), 73–82. doi:10.1002/ep.12442
Fernández, N., Montalvo, S., Fernández-Polanco, F., Guerrero, L., Cortés, I., Borja, R., et al. (2007). Real Evidence about Zeolite as Microorganisms Immobilizer in Anaerobic Fluidized Bed Reactors. Process Biochem. 42 (4), 721–728. doi:10.1016/j.procbio.2006.12.004
Horn, R., and Schlögl, R. (2015). Methane Activation by Heterogeneous Catalysis. Catal. Lett. 145 (1), 23–39. doi:10.1007/s10562-014-1417-z
Huang, H., Xu, C., and Zhang, W. (2011). Removal of Nutrients from Piggery Wastewater Using Struvite Precipitation and Pyrogenation Technology. Bioresour. Technol. 102 (3), 2523–2528. doi:10.1016/j.biortech.2010.11.054
Huiliñir, C., Fuentes, V., Esposito, G., Montalvo, S., and Guerrero, L. (2020). Nitrification in the Presence of Sulfide and Organic Matter in a Sequencing Moving Bed Biofilm Reactor (SMBBR) with Zeolite as Biomass Carrier. J. Chem. Technol. Biotechnol. 95 (5), 1614. doi:10.1002/jctb.6346
Huiliñir, C., Montalvo, S., and Guerrero, L. (2015). Biodegradability and Methane Production from Secondary Paper and Pulp Sludge: Effect of Fly Ash and Modeling. Water Sci. Technol. 72 (2), 230–237. doi:10.2166/wst.2015.210
Kataki, S., West, H., Clarke, M., and Baruah, D. C. (2016). Phosphorus Recovery as Struvite from Farm, Municipal and Industrial Waste: Feedstock Suitability, Methods and Pre-treatments. Waste Manage. 49, 437–454. doi:10.1016/j.wasman.2016.01.003
Koirala, N., Odey, E. A., Lu, Q., Iu, H. I., Lok, K. S., and Shim, H. (2021). Stimulatory Effect of Magnesium Supplement on Anaerobic Co-digestion of Food Waste and Domestic Wastewater. J. Water Process Eng. 40, 101773. doi:10.1016/j.jwpe.2020.101773
Lee, J. J., Choi, C. U., Lee, M. J., Chung, I. H., and Kim, D. S. (2004). A Study of NH3-N and P Refixation by Struvite Formation in Hybrid Anaerobic Reactor. Water Sci. Technol. 49 (5-6), 207–214. doi:10.2166/wst.2004.0755
Li, B., Huang, H. M., Boiarkina, I., Yu, W., Huang, Y. F., Wang, G. Q., et al. (2019). Phosphorus Recovery through Struvite Crystallisation: Recent Developments in the Understanding of Operational Factors. J. Environ. Manage. 248, 109254. doi:10.1016/j.jenvman.2019.07.025
Luo, Y., Li, H., Huang, Y.-R., Zhao, T.-L., Yao, Q.-Z., Fu, S.-Q., et al. (2018). Bacterial Mineralization of Struvite Using MgO as Magnesium Source and its Potential for Nutrient Recovery. Chem. Eng. J. 351, 195–202. doi:10.1016/j.cej.2018.06.106
Marañón, E., Negral, L., Suárez-Peña, B., Fernández-Nava, Y., Ormaechea, P., Díaz-Caneja, P., et al. (2021). Evaluation of the Methane Potential and Kinetics of Supermarket Food Waste. Waste Biomass Valor. 12 (4), 1829–1843. doi:10.1007/s12649-020-01131-0
Montalvo, S., Huiliñir, C., Borja, R., Sánchez, E., and Herrmann, C. (2020). Application of Zeolites for Biological Treatment Processes of Solid Wastes and Wastewaters - A Review. Bioresour. Technol. 301, 122808. doi:10.1016/j.biortech.2020.122808
Pérez-Pérez, T., Pereda-Reyes, I., Correia, G. T., Pozzi, E., Kwong, W. H., Oliva-Merencio, D., et al. (2021). Performance of EGSB Reactor Using Natural Zeolite as Support for Treatment of Synthetic Swine Wastewater. J. Environ. Chem. Eng. 9 (1), 104922. doi:10.1016/j.jece.2020.104922
Qi, N., Zhao, X., Zhang, L., Gao, M., Yu, N., and Liu, Y. (2021). Performance Assessment on Anaerobic Co-digestion of Cannabis Ruderalis and blackwater: Ultrasonic Pretreatment and Kinetic Analysis. Resour. Conservation Recycling 169, 105506. doi:10.1016/j.resconrec.2021.105506
Romero-Güiza, M. S., Astals, S., Mata-Alvarez, J., and Chimenos, J. M. (2015). Feasibility of Coupling Anaerobic Digestion and Struvite Precipitation in the Same Reactor: Evaluation of Different Magnesium Sources. Chem. Eng. J. 270, 542–548. doi:10.1016/j.cej.2015.02.057
Sánchez-Ramírez, J. E., Pastor, L., Martí, N., Claros, J., Doñate, S., and Bouzas, A. (2019). Analysis of Uncontrolled Phosphorus Precipitation in Anaerobic Digesters under Thermophilic and Mesophilic Conditions. Environ. Technol. 42, 1836–1845. doi:10.1080/09593330.2019.1681522
Stratful, I., Scrimshaw, M. D., and Lester, J. N. (2001). Conditions Influencing the Precipitation of Magnesium Ammonium Phosphate. Water Res. 35 (17), 4191–4199. doi:10.1016/S0043-1354(01)00143-9
Tao, W., Fattah, K. P., and Huchzermeier, M. P. (2016). Struvite Recovery from Anaerobically Digested Dairy Manure: A Review of Application Potential and Hindrances. J. Environ. Manage. 169, 46–57. doi:10.1016/j.jenvman.2015.12.006
Uludag-Demirer, S., Demirer, G. N., Frear, C., and Chen, S. (2008). Anaerobic Digestion of Dairy Manure with Enhanced Ammonia Removal. J. Environ. Manage. 86 (1), 193–200. doi:10.1016/j.jenvman.2006.12.002
Wijesinghe, D. T. N., Dassanayake, K. B., Scales, P., Sommer, S. G., and Chen, D. (2018). Removal of Excess Nutrients by Australian Zeolite during Anaerobic Digestion of Swine Manure. J. Environ. Sci. Health A 53 (4), 362–372. doi:10.1080/10934529.2017.1401398
Yuan, T., Cheng, Y., Wang, X., Yu, Y., Zhang, Z., Lei, Z., et al. (2019). A Novel Anaerobic Digestion System Coupling Biogas Recirculation with MgCl2 Addition for Multipurpose Sewage Sludge Treatment. J. Clean. Prod. 230, 499–507. doi:10.1016/j.jclepro.2019.05.124
Keywords: anaerobic digestion, struvite, zeolite, bischofite, pig slurry
Citation: Palominos N, Castillo A, Guerrero L, Borja R and Huiliñir C (2021) Coupling of Anaerobic Digestion and Struvite Precipitation in the Same Reactor: Effect of Zeolite and Bischofite as Mg2+ Source. Front. Environ. Sci. 9:706730. doi: 10.3389/fenvs.2021.706730
Received: 07 May 2021; Accepted: 12 November 2021;
Published: 02 December 2021.
Edited by:
Cidália Maria Botelho, University of Porto, PortugalReviewed by:
Muhammad Hassan, Southwest Jiaotong University, ChinaCopyright © 2021 Palominos, Castillo, Guerrero, Borja and Huiliñir. This is an open-access article distributed under the terms of the Creative Commons Attribution License (CC BY). The use, distribution or reproduction in other forums is permitted, provided the original author(s) and the copyright owner(s) are credited and that the original publication in this journal is cited, in accordance with accepted academic practice. No use, distribution or reproduction is permitted which does not comply with these terms.
*Correspondence: C. Huiliñir, Y2VzYXIuaHVpbGluaXJAdXNhY2guY2w=
Disclaimer: All claims expressed in this article are solely those of the authors and do not necessarily represent those of their affiliated organizations, or those of the publisher, the editors and the reviewers. Any product that may be evaluated in this article or claim that may be made by its manufacturer is not guaranteed or endorsed by the publisher.
Research integrity at Frontiers
Learn more about the work of our research integrity team to safeguard the quality of each article we publish.