- 1Key Laboratory of Genetics and Germplasm Innovation of Tropical Special Forest Trees and Ornamental Plants, Ministry of Education, College of Forestry, Hainan University, Haikou, China
- 2Ministry of Education Key Laboratory for Biodiversity Science and Ecological Engineering, School of Life Sciences, Fudan University, Shanghai, China
- 3State Key Laboratory of Grassland Agro-Ecosystem, Institute of Innovation Ecology, Lanzhou University, Lanzhou, China
Global nitrogen eutrophication, which is disrupting the intimate plant–arbuscular mycorrhizal fungi (AMF) symbiosis, can alter the diversity and physiological functions of soil AMF greatly. However, shifts of beta diversity and the intrinsic patterns of AMF community dissimilarities in response to nitrogen addition remain unclear. Based on a 7-year nitrogen addition experiment in a Qinghai–Tibet Plateau alpine meadow, we detected the changes in soil AMF alpha diversity (richness and genus abundance) and the community composition beta diversity by partitioning the two components of Simpson and nestedness dissimilarities along (turnover) and within (variation) nitrogen addition treatments, and fitted with environmental factor dissimilarities. We found that nitrogen addition decreased AMF richness by decreasing the most dominant AMF genus of Glomus but increasing the abundance of the rare genera. The turnover of the AMF community overall beta diversity along the nitrogen addition gradients was induced by the increased nestedness dissimilarity, while the variation within treatments was explained by both increased Simpson and nestedness dissimilarities, which was significantly correlated with soil pH. Our study found both Simpson and nestedness dissimilarities worked on the AMF community dissimilarity after nitrogen addition and the significant variation within the same treatment, which would be important in the future for predicting global AMF or microbial diversity changes in response to nitrogen eutrophication.
Introduction
Arbuscular mycorrhizal fungi, which belong to the phylum Glomeromycota, are widely distributed in soil and plant roots (Smith and Read, 2008; Davison et al., 2015). The fungi, forming symbiosis with plants to swap nutrients by supplying soil nitrogen and phosphorus and capturing the photosynthetic products in return, can protect plants from abrupt environmental changes or biotic attacks such as pathogens, which is an essential part of ecosystem processes (Johnson, 2010; Tedersoo et al., 2020). Global changes in nitrogen eutrophication caused by anthropogenic activities have largely impacted the physiological functions and diversity of AMF (Treseder, 2004; Jiang et al., 2018), yet the underlying mechanisms of these processes are still not fully understood.
Previous studies have shown that nitrogen addition changed the AMF alpha diversity. Most studies illustrated a decreased AMF colonization rate after nitrogen addition, owing to the decreased dependence of plants (Treseder, 2004; Liu et al., 2012), which could also result in a decrease in AMF alpha diversity in soil. However, a meta-analysis showed a negative but nonsignificant relationship between the AMF richness and nitrogen addition (Han et al., 2020), which may be due to variation among different ecosystems, treatment forms, rates, soil properties (Egerton-Warburton et al., 2007; Camenzind et al., 2014; Zheng et al., 2014), and/or the abundance shift of specific AMF taxons with specific functions. In plant roots, the more redundant AMF families may decrease in abundance more quickly, while the less abundant taxa increase in abundance after nitrogen addition, even though the overall richness remains unchanged (Lu et al., 2020). Hence, identifying the changes in alpha diversity and taxon abundance of AMF species in soil under long-term nitrogen addition is meaningful but not useful for discovering the functional properties and their role in the ecosystem process.
Beta diversity, which links the biodiversity between local (alpha diversity) and regional (gamma diversity) diversity, reflects the degree of species similarity (or dissimilarity) among species assemblages at different places and times (Whittaker, 1960; Whittaker, 1972; Vellend, 2010). Despite the low endemism of global AMF species (Davison et al., 2015), fungi still showed compositional dissimilarities due to spatiotemporal heterogeneity (Hiiesalu et al., 2014; Bahram et al., 2015). For example, AMF community composition differs among different nitrogen addition treatments by interrupting the intimate mutualistic relationship (Jiang et al., 2018; Lu et al., 2020). Actually, two metrics of beta diversity have been proposed for measuring composition dissimilarity (Anderson et al., 2011). The first one measures directional turnover (thereafter “turnover”) or changes in community composition along an environment or treatment gradient, from one sampling unit to another (Vellend, 2001). The other is defined as the non-directional variation (thereafter “variation”) within a specific region or gradient range, which was directly related to the multivariate dispersion of community composition (Legendre et al., 2005; Anderson et al., 2006). Different measures emphasize particular properties (Anderson et al., 2011), but whether nitrogen addition affects turnover and/or variation of AMF communities remains unknown.
The overall beta diversity can additionally be partitioned into two intrinsic components, that is, the Simpson and nestedness dissimilarities (Baselga, 2010). The Simpson dissimilarity, previously referred to as “spatial turnover” (Baselga, 2010), defines the replacement of some species by others as a consequence of spatial and historical constraints or environmental differentiation, with species losses balancing the gains (Lennon et al., 2001; Koleff et al., 2003). The nestedness dissimilarity emphasizes that the biotas of sites with fewer species are subsets of the richer sites, forming an inverted stepped structure (Baselga, 2010; Baselga, 2012). Both Simpson and nestedness dissimilarities can exist in turnover and variation. Exploring the intrinsic causes of AMF community beta diversity can provide a better understanding of whether community dissimilarity is due to difference in species diversity and/or function replacement or the loss and gain of AMF species, which requires opposing biodiversity conservation strategies (Thébault and Fontaine, 2010; Baselga, 2012). Likewise, many previous experiments also failed to disentangle the contribution of Simpson and nestedness beta diversity patterns to the overall AMF community dissimilarity under nitrogen addition.
Environmental changes (abiotic or biotic) after nitrogen addition played important roles in shaping the beta diversity of microbiota (Xu et al., 2016; Fierer, 2017). First, nitrogen addition can reduce plant species richness and change community composition (Tilman, 1987; Stevens et al., 200), and it led to a reduction in AMF root colonization via both the direct AMF physiological effects and shift in plant community composition (Liu et al., 2012; Lu et al., 2020). Second, the alternation of soil chemical properties after nitrogen addition is also closely related to the AMF diversity (Egerton-Warburton et al., 2007; Zheng et al., 2014). For instance, soil pH was considered an important factor shaping AMF community composition, with a higher pH value being positive to AMF diversity (Xu et al., 2016; Chen et al., 2017). The content of different nitrogen forms and other microbes in soil can also affect soil AMF abundance and composition (Egerton-Warburton et al., 2007; Zheng et al., 2014; Chen et al., 2017). However, it remains unknown whether and how the environmental factors affect overall and partitioned AMF community beta diversity, which may help detect and understand any complicated diversity changes after nitrogen addition.
Alpine meadows are known as a “hot spot” for above- and belowground biodiversity. However, the sophisticated alpha and beta diversity changes of the AMF community under nitrogen addition and the intrinsic patterns have not been fully explored. Here, based on a 7-year nitrogen addition experiment in a Qinghai–Tibet Plateau alpine meadow, we investigate the soil AMF alpha diversity (richness and taxon abundance change) and beta diversity by partitioning the two components of Simpson and nestedness dissimilarities along (i.e., turnover) and within (i.e., variation) nitrogen addition treatment and their relationships with environmental factors. We aim to test the following hypotheses: 1) nitrogen addition decreases AMF richness by decreasing the dominant AMF taxon but increasing the abundance of the rare taxa; 2) the partitioned Simpson and nestedness beta dissimilarities may provide different explanation powers on the soil AMF community overall dissimilarity along and within different nitrogen addition treatments; and 3) soil AMF beta diversity is related to dissimilarities in environmental factors.
Materials and Methods
Study Site
The study was conducted at the Gannan Grassland Ecosystem Field Science Observation and Research Station of the Ministry of Education (101° 52’ E, 33° 40’ N; 3500 m. a.s.l.) in Maqu County, Gansu Province, the eastern part of the Qinghai–Tibet Plateau, China. The site is a typical alpine meadow vegetation ecosystem, and the nitrogen-limited soils are classified as “chestnut” or “subalpine meadow” soils, with an average thickness of 80 cm (Liu et al., 2017). The annual mean precipitation is 620 mm, which mainly falls during the short growing season from May to September. The annual mean temperature is 1.2°C, ranging from–10.7°C in January to 11.7°C in July. The alpine meadow is dominated by some perennial herbaceous species such as Asteraceae, Poaceae, and Ranunculaceae, and herbivore animals include yaks, marmots, and zokors (Lu et al., 2020).
Experimental Design
The long-term nitrogen addition experiment had been established on a south-facing meadow since June 2011, with grazing (mainly yaks) permitted only in winter. We regularly arranged twenty-four 5 × 5-m plots in a 50 × 50-m area with roughly the same plant community composition, and the adjacent edges of the plots were separated by a 1-m buffer zone. Four concentrations of nitrogen addition of ammonium nitrate (NH4NO3) with six replicates were randomly assigned to the 24 plots: 0 (ambient), 5, 10, or 15 gm−2 yr−1 (Liu et al., 2016; Jiang et al., 2018). Nitrogen addition has been applied annually in mid-June since 2011.
Data Collection
In August 2017, we randomly arranged one 0.5 × 0.5-m subplot in each of the 24 plots, parallel to but at least 0.5 m away from the plot edges. We harvested all the aboveground stems in each subplot and recorded the species richness and abundance (i.e., number of individuals) of each species. The 7-year nitrogen addition treatment had significantly changed the plant species richness and community composition and soil chemical properties (Liu et al., 2020; Lu et al., 2020). Additionally, we drilled four soil cores in each plot which were 5 cm in diameter and 10 cm in depth, mixing into one soil sample. The soil samples were transported to the laboratory for measuring soil chemical properties and molecular AMF diversity identification.
Soil chemical properties include soil pH (measured using a pH meter forming a slurry with a dry soil-to-water ratio of 1:5), soil moisture (%; measured as the percent change of weight after 72 h of desiccation at 70°C), soil ammonium nitrogen (NH4+–N mg/kg; measured using the indophenol blue colorimetric method), soil nitrate nitrogen (NO3––N mg/kg; measured using the salicylate colorimetric method), soil microbial biomass carbon and nitrogen (MBC and MBN mg/kg; measured using the chloroform fumigation–direct extraction method), and soil total carbon and total nitrogen (TC and TN, g/kg, measured using an element analyzer) (Elementar Vario EL III, Hanau, Germany).
Molecular and Bioinformatic Analysis
For each composite soil sample, around 50 g root-free soil was resampled for DNA extraction. The AMF community diversity identification was followed by a polymerase chain reaction (PCR) sequencing approach described by Lu et al. (2020). In brief, the soil DNA from each sample was extracted using a Qiagen Plant DNeasy Kit (Qiagen, Hilden, Germany) and evaluated with 1% (w/v) agarose gel to confirm efficiency. The fungal 18 S rRNA genes of distinct regions were amplified by PCR using the specific primer pair AMV4.5NF and AMDGR (Sato et al., 2005; Lumini et al., 2010), with a 12-bp barcode. After detection, pooling, and purification of PCR products, we generated sequencing libraries using an NEBNext® Ultra™ DNA Library Prep Kit for Illumina® (New England Biolabs, United States). At last, the library was sequenced on an IlluminaHiseq2500 platform, and 250-bp paired-end reads were generated.
High-quality clean reads were obtained using the Trimmomatic (V0.33) quality control process that sequences with no valid primer sequencing, that is, an average quality score of <25 or length <200 bp was discarded. We merged paired-end clean reads with FLASH (V1.2.11) and assigned sequences to each sample with mothur software (V1.35.1) based on the unique barcode and primer. Sequences with a similarity threshold above 97% were clustered to the same operational taxonomic units (OTUs) by removing potential chimeric and singleton reads. The representative sequences for all 1313 detected OTUs were compared using the SILVA database for further annotation (Pruesse et al., 2007). To compare our data with those of other published studies, AMF OTUs were blasted and classified into the virtual taxa (i.e., VT) using the online database MaarjAM (http://maarjam.botany.ut.ee), and an e-value less than 1e–50 was considered significant (Öpik et al., 2010). The representative sequence of each AMF VT has been deposited in the NCBI Sequence Read Archive (SRA) database (accession code SRP318342).
Statistical Analysis
We rarefied each sample to a minimum of 7000 sequences in total for downstream analysis to allow for equalization of different sequencing depths and to enable comparison between samples with the rarecurve function in the vegan package (Oksanen et al., 2013). For each plot, the AMF VT richness, Shannon diversity, Simpson diversity, and the relative abundance of each AMF genus were calculated, and we used linear models to test the effects of nitrogen addition on them.
Based on the community matrix of AMF VT, the beta diversity between samples was calculated with the abundance-weighted (i.e., relative sequence reads) Bray–Curtis, and Sørensen dissimilarity matrices. The Sørensen dissimilarity represents the sum of dissimilarities due to spatial turnover (i.e., Simpson dissimilarity) and nestedness (Baselga, 2010; Baselga, 2012). We partitioned the Sørensen dissimilarity of the AMF community into Simpson and nestedness diversity using the beta. pair function (index.family = “sorensen”) in the betapart package (Baselga and Orme, 2012). To confirm our results, we also employed another beta partition method that separates the overall Jaccard dissimilarity (corresponds to Sørensen dissimilarity) into species replacement (corresponds to Simpson dissimilarity) and richness difference (corresponds to nestedness dissimilarity) (Podani and Schmera, 2011; Carvalho et al., 2012) with function beta.multi in the BAT package (Cardoso et al., 2015) and built linear models to detect the correlations of the three sets of data.
To identify the turnover of the AMF community beta diversity along four nitrogen addition treatments, we calculated the treatment distance as the difference between the intensities of different nitrogen addition treatments. We built linear models with the nitrogen addition treatment distance as the independent variable, and the Bray–Curtis dissimilarity (β-bray; abundance-weighted), Sørensen dissimilarity (β-sor; presence/absence), and partitioned Simpson (β-sim), and nestedness (β-nes) dissimilarities as the response variables, separately. Similarly, variation of AMF community beta diversity among replicates at each nitrogen addition level was also tested with linear models, with the β-bray, β-sor, β-sim, and β-nes dissimilarities among the same treatment samples as response variables, and the exact nitrogen addition level (i.e., within treatment) as the independent variable. The significance of the linear models was evaluated using the information-to-theory evidence ratio (ER), calculating the relative support between the linear slope model and the intercept-only (null) model, which was better than the arbitrary p-value, and ER > 1.5 means a significant relationship (Burnham et al., 2011). The goodness of fit of the linear models was calculated with the percent deviance explained (De) in the response variable. We also used one-way ANOVA to decompose the Simpson and nestedness components of the overall Sørensen dissimilarities after nitrogen addition with the turnover and variation patterns, respectively (Lepš et al., 2011; Lu et al., 2020). The percentage explained in the Sørensen dissimilarity partitioned by Simpson and nestedness dissimilarities and their covariation was calculated.
We used PERMANOVA (permutation multivariate analysis) with 9999 permutations for β-bray, β-sor, β-sim, and β-nes dissimilarity matrices to test for the beta diversity differences of AMF communities under different nitrogen addition treatments, using the adonis function in the vegan package (Oksanen et al., 2013). To graphically illustrate the AMF community β-bray, β-sor, β-sim, and β-nes dissimilarities between and within nitrogen addition treatments, dissimilarity matrices were formed by PCoA (principal coordinate analysis) with the pcoa function in the ape package (Paradis et al., 2004).
We calculated Spearman’s rank correlation between the environmental factor dissimilarities of plant community composition, soil chemical properties (pH, moisture, NH4+–N, NO3––N, MBC, MBN, TC, and TN), and β-bray, β-sor, β-sim, and β-nes dissimilarities (Oksanen et al., 2013). The plant community dissimilarities were calculated in line with the AMF community dissimilarities, with β-bray, β-sor, β-sim, and β-nes dissimilarities. The soil chemical property dissimilarities were calculated by subtracting between samples, and the correlations of the soil properties were tested using the cor. test function by Pearson’s rank correlation examination. All the calculations were done in R version 3.5.1 (R Development Core Team, 2014).
Results
Out of the 24 soil samples, a total of 885, 077 sequencing reads of the phylum Glomeromycota and 114 AMF virtual taxa (VTs) belonging to nine genera were observed after rarefaction. Nitrogen addition significantly decreased the soil AMF VT richness (ER = 1.98 × 103; De = 52.4; Figure 1A) but not the Shannon (ER = 0.30; De = 0.92) and Simpson diversities (ER = 0.46; De = 4.41; Supplementary Figure S2). The abundance of different AMF genera also had a significant variation along and within nitrogen addition gradients, with the AMF genera of Ambispora (p < 0.001) and Glomus (p < 0.001) decreasing significantly but the genera of Archaeospora (p = 0.03), Claroideoglomus (p < 0.001), and Paraglomus (p < 0.001) increasing significantly, while the genera of Acaulospora (p = 0.493), Diversispora (p = 0.637), Pacispora (p = 0.665), and Scutellospora (p = 0.283) did not change with nitrogen addition (Figure 1B).
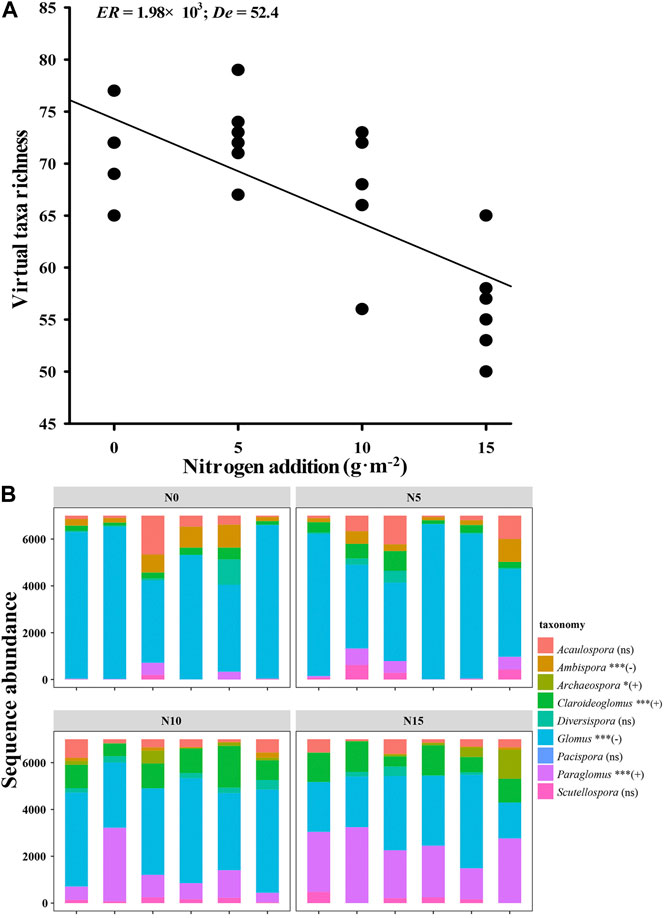
FIGURE 1. (A) Virtual taxa richness decreased with nitrogen addition (information-to-theory evidence ratio (ER) = 1.98 × 103; percent deviance explained as (De) = 52.4; with some overlapping points). ER > 1.5 means a significant relationship. (B) AMF abundance change of the rarified sequence assigned to the represented genera with four nitrogen addition treatments. The “+” or “–” sign in parentheses means significant increase or decrease in sequence abundance with nitrogen addition, while “ns” means insignificance.
We found that the results obtained using the beta partition method proposed by Baselga (2010) and those obtained using the method proposed by Podani and Schmera (2011) and Carvalho et al. (2012) showed significant correlations, with β-sor highly correlated with Jaccard dissimilarity (p < 0.001), β-sim correlated with replacement (p < 0.001), and β-nes correlated with richness difference (p < 0.001) (Supplementary Figure S3). Hence, we only report the results of β-sor, β-sim, and β-nes below.
Based on linear models, the turnover of the AMF community β-bray (abundance-weighted) (ER = 3.54 × 1035; De = 45.15) and the β-sor (presence/absence data) (ER = 6.95 × 109; De = 15.77) dissimilarities both significantly increased with nitrogen addition, which can be attributed to the significant increase in β-nes (ER = 5.56 × 1011; De = 18.4) but not in β-sim (ER = 0.88; De = 0.64) dissimilarities (Figure 2). The results of one-way ANOVA also showed that β-nes (p < 0.001; De = 22.1) significantly increased the overall β-sor dissimilarity but not β-sim (p = 0.183; De = 0.53) along the nitrogen treatment distance (Supplementary Table S2).
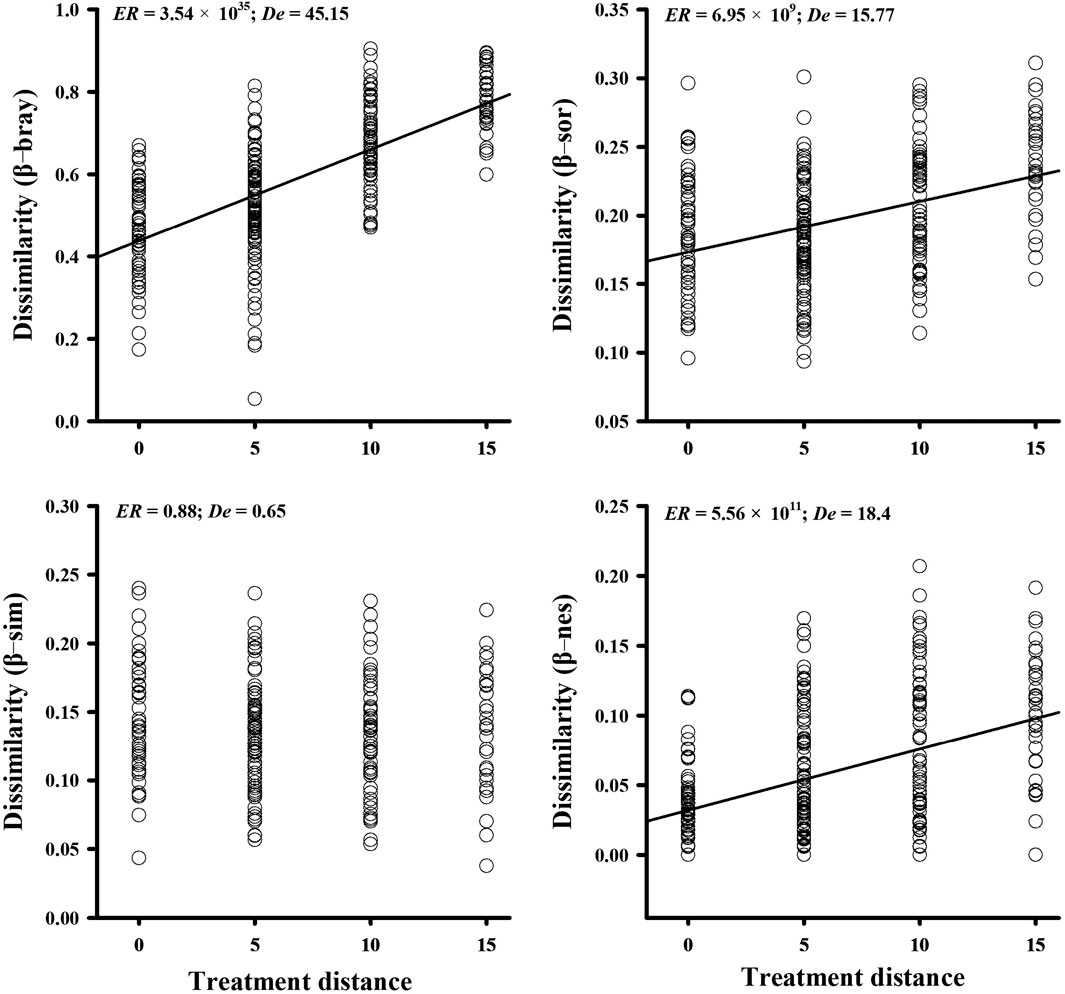
FIGURE 2. Turnover of the soil AMF community beta diversity of Bray–Curtis (β-bray; abundance-weighted), Sørensen (β-sor; presence/absence-based), partitioned Simpson (β-sim), and nestedness (β-nes) along the nitrogen addition gradient. ER > 1.5 means a significant relationship.
β-sor within the nitrogen addition treatments showed a significant increase with nitrogen addition (ER = 2.76 × 103; De = 26), which can be explained by the increase in both β-sim (ER = 13.58; De = 11.65) and β-nes (ER = 3.2; De = 7.3) dissimilarities. The results of one-way ANOVA also showed that both β-sim (p = 0.008; De = 11.4) and β-nes (p = 0.037; De = 3.16) significantly increased the overall β-sor dissimilarities (Supplementary Table S3). However, there was no significant variation in abundance-weighted β-bray metrics within the treatments (ER = 0.58; De = 1.86) (Figure 3).
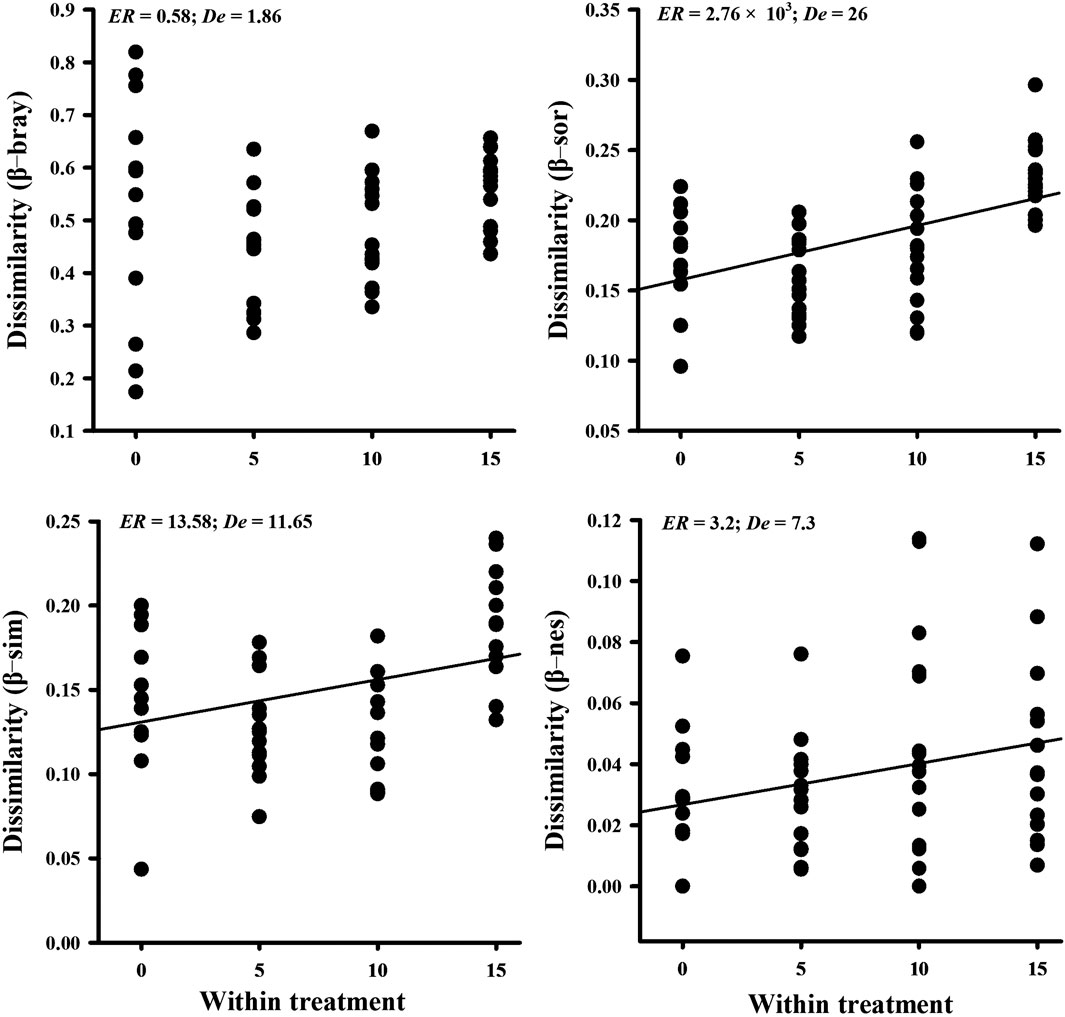
FIGURE 3. Variation of the soil AMF community beta diversity of Bray–Curtis (β-bray; abundance-weighted), Sørensen (β-sor; presence/absence-based), partitioned Simpson (β-sim), and nestedness (β-nes) within four nitrogen addition treatments. ER > 1.5 means a significant relationship.
The PCoA results of PERMANOVA depicted an overview of the AMF community dissimilarity between and within treatments. Nitrogen addition significantly affected the β-bray (F = 5.18; p < 0.003) and β-sor (F = 1.91; p = 0.003) dissimilarities, which was explained more by the partitioned effect of β-nes (F = 15.1; p < 0.001) but not β-sim (F = –0.2; p = 0.996) (Figure 4).
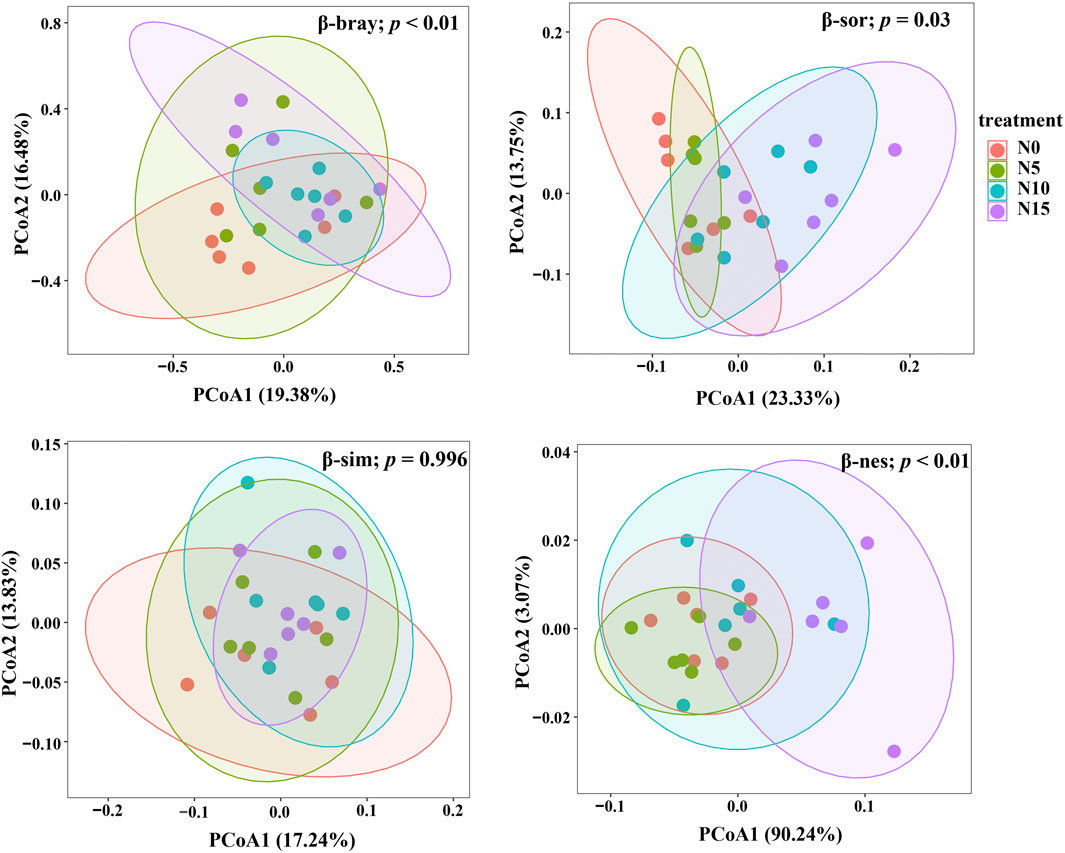
FIGURE 4. Principal coordinate analysis (PCoA) by PERMANOVA showing the association of soil AMF community dissimilarities along and within nitrogen addition treatments. Significance of the Bray–Curtis (β-bray; abundance-weighted), Sørensen (β-sor; presence/absence-based), partitioned Simpson (β-sim), and nestedness (β-nes) dissimilarities with nitrogen addition are shown with p values (p ≤ 0.05 means a significant effect).
The Mantel test showed that AMF community beta diversity was correlated with environmental dissimilarities. The dissimilarity of the plant community composition was positively correlated with the β-bray dissimilarities (p = 0.012) but had a weak correlation with β-sor (p = 0.095) and partitioned β-sim (p = 0.094) but no significant correlation with β-nes (p = 0.236) dissimilarities. Soil pH was the only factor that was significantly correlated with all four beta dissimilarities (p < 0.001 for β-bray and β-sor, respectively), having a negative relationship with partitioned β-sim (p = 0.014) and a positive relationship with β-nes (p < 0.001). Soil moisture was significantly correlated with β-bray (p = 0.007) and β-nes (p = 0.024) but not β-sor (p = 0.136) and β-sim (p = 0.066). Dissimilarities of NH4+–N, NO3––N, MBC, and MBN all correlated significantly with β-bray, β-sor, and partitioned β-nes dissimilarities (all p < 0.05), but NH4+–N and NO3––N correlated negatively, while MBC and MBN correlated positively with AMF community dissimilarities. Soil TN (p = 0.043) and TC (p = 0.003) correlated positively with β-bray but not with β-sor, as the partitioned β-sim (TN, p = 0.017; TC, p = 0.006) and β-nes (TN, p = 0.017; TC, p = 0.001) components changed in opposite directions (Table 1).
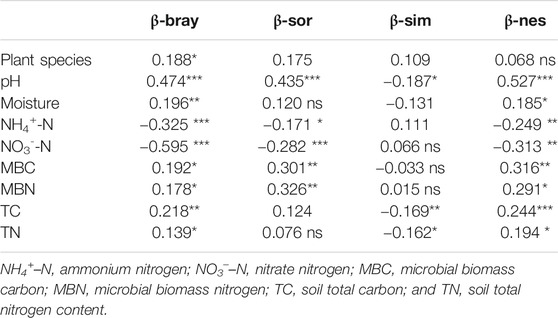
TABLE 1. Mantel tests for the soil arbuscular mycorrhizal fungi community Bray–Curtis (β-bray; abundance-weighted), Sørensen (β-sor; presence/absence-based), partitioned Simpson (β-sim), and nestedness (β-nes) dissimilarities with environmental factor dissimilarities. The Spearman correlation r-value and significant effects are shown (. p < 0.1; *p ≤ 0.05; **p ≤ 0.01; ***p ≤ 0.001; ns, insignificant).
Discussion
We found that AMF richness decreased and the relative abundance of different AMF genera was altered differently after nitrogen addition. By disentangling multiple aspects of soil AMF diversity along a nitrogen addition gradient, we found that nitrogen addition significantly increased the AMF community dissimilarities. The turnover of the AMF community along the treatment gradient was explained by an increase in nestedness but not Simpson dissimilarity, while the variation of the AMF community within treatments was explained by the increase in both Simpson and nestedness dissimilarities. Under nitrogen addition, overall and partitioned AMF community dissimilarities also significantly correlated with dissimilarities of the plant community and soil chemical properties.
Decreased AMF richness suggests that plants reduce their dependence on AMF and tend to absorb nutrients through their own roots as soil nutrient availability is enriched (Johnson, 2010), which supports previous findings (Egerton-Warburton and Allen, 2007; Camenzind et al., 2014; Chen et al., 2017). After short-term nitrogen fertilization, an insignificant or even positive relationship between AMF richness may still persist (Zheng et al., 2014; Xiang et al., 2016) because plant species may still have a friendly interaction with AMF (Jiang et al., 2018). However, after long-term nitrogen fertilization, the loss of some plant species caused by nitrogen addition may lead to the corresponding loss of associated specific AMF species because of host identification (Hiiesalu et al., 2014; Martínez-García et al., 2015).
The functional equilibrium model emphasized that aboveground plants still need to rely on some functionally specific AMF groups to obtain other scarce elements (e.g., phosphorus) when only some elements (e.g., nitrogen) of the soil are enriched (Johnson, 2010). This may result in the abundance and functional turnover of the AMF taxa (Lilleskov et al., 2019). The genus of Glomus was considered the most dominant and redundant in natural conditions (Davison et al., 2015; Gosling et al., 2016); thus, taxa belonging to this genus may be prone to extinction because of competitive exclusion of functionally and/or phylogenetically similar groups after nitrogen addition (Liu et al., 2015). Previous global-scale meta-analysis also proposed that Glomeraceae (including the Glomus genus) would be lost under increasing soil nitrogen (Han et al., 2020). Other genera of Ambispora, Claroideoglomus, and Paraglomus, on the contrary, are less abundant in natural conditions but can increase significantly after nitrogen addition probably because of their specific functional traits in helping plants grow by absorbing more scarce elements (Dodd et al., 2000; Chagnon et al., 2013). Genus-specific response of AMF in abundance after nitrogen addition may also be affected by soil properties, like the soil nitrogen/phosphorus ratio (Egerton-Warburton and Allen, 2007; Han et al., 2020).
We found that the dissimilarities of the AMF community with presence/absence and abundance-weighted data significantly increased with nitrogen addition, which is consistent with previous studies (Chen et al., 2017; Jiang et al., 2018; Lu et al., 2020). This may be because long-term nitrogen addition leads to changes of some AMF species in abundance due to environmental filtering or competitive exclusion (Liu et al., 2015; Chen et al., 2017). Nevertheless, the partitioned nestedness dissimilarity increased significantly, indicating that the AMF species remaining in the higher nitrogen addition treatment is, to some extent, a subset of those remaining in relatively lower N addition treatments. However, the Simpson dissimilarity of AMF along the nitrogen addition gradient did not change significantly, which may be because the variation within the same treatments was large and diluted the effect between different treatments. Moreover, as more AMF species got lost at higher nitrogen addition levels, the increase of taxa cannot compensate for the loss of species, so there was no significant change in spatial turnover (β-sim) (Baselga, 2010).
We found that AMF community composition was more dissimilar within high nitrogen addition treatments, which could be explained by the increased Simpson and nestedness dissimilarities. The fungal diversity can vary greatly even over a small geographical distance because of changes in the microenvironment (e.g., soil environments or animal movements) (Bahram et al., 2015; Fierer, 2017) or stochastic processes like random drifts (Zhou and Ning, 2017). Increased dissimilarity means more unshared species within sampling units, which may be conducive to the conservation of AMF diversity (Anderson et al., 2011). However, we found that the abundance-weighted variation of the AMF community structure did not change significantly within nitrogen treatments, indicating that the dominant groups were still dominant and vice versa, even though the Simpson and nestedness of species richness both changed after nitrogen addition.
Attributing different patterns of beta diversity to the respective biological processes is fundamental to understanding the mechanisms of biodiversity maintenance. For conservation purposes, it is also essential to evaluate the relative importance of the Simpson and nestedness dissimilarities, which requires opposing conservation strategies (Wright and Reeves, 1992; Baselga, 2010). Although different methods have been proposed for partitioning the overall beta diversity (Podani and Schmera, 2011; Carvalho et al., 2012; Baselga and Leprieur, 2015), they generally provide positively corrected results (Wu et al., 2017), and they also resulted in consistent results along the nitrogen addition gradient in this study. Hence, even though global AMF diversity shows low endemism, the partition methods assessing beta diversity may be a vital method in the extrapolation of global AMF richness (Davison et al., 2015).
Different environmental factors affect the AMF diversity, like an environmental filter, and possess different powers in predicting AMF community dissimilarity (Stegen et al., 2012; Chen et al., 2017). We found that the plant community dissimilarity positively correlated with the composition dissimilarity of the AMF community (presence/absence) but not with that measured with abundance or partitioned components. There may be a coherence of plant and AMF species that meant that more abundant plants also contained more dominant AMF groups (Hiiesalu et al., 2014). Soil pH, moisture, and total carbon and nitrogen dissimilarities were all positively correlated with the overall AMF dissimilarities, but Simpson dissimilarity negatively correlated, while nestedness dissimilarity positively correlated with AMF. The microbial biomass carbon and nitrogen were found to have a collaboratively opposite relationship with ammonium nitrogen and nitrate nitrogen (by negatively correlating with overall AMF community composition, structure, and nestedness). We confirmed a strong relationship between soil AMF and pH (Pan et al., 2020), but it may also be influenced by other surroundings and explained more by the partitioning of the Simpson and nestedness components.
In summary, our experiments demonstrate a decreased soil AMF richness and abundance of different genera in a 7-year nitrogen addition experiment in an alpine meadow. We found that decomposing the overall AMF community beta diversity into Simpson and nestedness dissimilarities along (turnover) and within (variation) nitrogen addition treatments uncovered more intrinsic mechanisms of AMF diversity variation. The turnover of the AMF community overall beta diversity among treatments was explained by an increased nestedness dissimilarity, while the variation of the community overall dissimilarity within treatments was due to both increased Simpson and nestedness dissimilarities. The overall and partitioned AMF community beta diversity also correlated with environmental factors, respectively. Our results provide a deep detection of AMF diversity change after nitrogen addition, which may be a vital key for global AMF diversity conservation.
Data Availability Statement
The datasets presented in this study can be found in online repositories. The data presented in the study are deposited in the (NCBI SRA BioProject) repository, accession number (PRJNA726639).
Author Contributions
SZ, YL, and XL designed this experiment. YL and XL collected the data. YL, XL, and SZ analyzed the data and wrote the manuscript. All authors approved the final manuscript.
Funding
The study was supported by the National Natural Science Foundation of China (31770518) and Hainan University (RZ2000009932).
Conflict of Interest
The authors declare that the research was conducted in the absence of any commercial or financial relationships that could be construed as a potential conflict of interest.
Acknowledgments
The field experiment was conducted in the Gannan Grassland Ecosystem Field Science Observation and Research Station of the Ministry of Education. We thank Dexin Sun, Fei Chen, Li Zhang, and Mengjiao Huang for helping with the sampling and Jiajia Liu for advice.
Supplementary Material
The Supplementary Material for this article can be found online at: https://www.frontiersin.org/articles/10.3389/fenvs.2021.701653/full#supplementary-material
References
Anderson, M. J., Crist, T. O., Chase, J. M., Vellend, M., Inouye, B. D., Freestone, A. L., et al. (2011). Navigating the Multiple Meanings of β Diversity: a Roadmap for the Practicing Ecologist. Ecol. Lett. 14, 19–28. doi:10.1111/j.1461-0248.2010.01552.x
Anderson, M. J., Ellingsen, K. E., and McArdle, B. H. (2006). Multivariate Dispersion as a Measure of Beta Diversity. Ecol. Lett. 9, 683–693. doi:10.1111/j.1461-0248.2006.00926.x
Bahram, M., Peay, K. G., and Tedersoo, L. (2015). Local‐scale Biogeography and Spatiotemporal Variability in Communities of Mycorrhizal Fungi. New Phytol. 205, 1454–1463. doi:10.1111/nph.13206
Baselga, A., and Leprieur, F. (2015). Comparing Methods to Separate Components of Beta Diversity. Methods Ecol. Evol. 6, 1069–1079. doi:10.1111/2041-210x.12388
Baselga, A., and Orme, C. D. L. (2012). Betapart : an R Package for the Study of Beta Diversity. Methods Ecol. Evol. 3, 808–812. doi:10.1111/j.2041-210x.2012.00224.x
Baselga, A. (2010). Partitioning the Turnover and Nestedness Components of Beta Diversity. Glob. Ecol. Biogeogr. 19, 134–143. doi:10.1111/j.1466-8238.2009.00490.x
Baselga, A. (2012). The Relationship between Species Replacement, Dissimilarity Derived from Nestedness, and Nestedness. Glob. Ecol. Biogeogr. 21, 1223–1232. doi:10.1111/j.1466-8238.2011.00756.x
Burnham, K. P., Anderson, D. R., and Huyvaert, K. P. (2011). AIC Model Selection and Multimodel Inference in Behavioral Ecology: Some Background, Observations, and Comparisons. Behav. Ecol. Sociobiol. 65, 23–35. doi:10.1007/s00265-010-1029-6
Camenzind, T., Hempel, S., Homeier, J., Horn, S., Velescu, A., Wilcke, W., et al. (2014). Nitrogen and Phosphorus Additions Impact Arbuscular Mycorrhizal Abundance and Molecular Diversity in a Tropical Montane forest. Glob. Change Biol. 20, 5203646–5203659. doi:10.1111/gcb.12618
Cardoso, P., Rigal, F., and Carvalho, J. C. (2015). BAT - Biodiversity Assessment Tools, an R Package for the Measurement and Estimation of Alpha and Beta Taxon, Phylogenetic and Functional Diversity. Methods Ecol. Evol. 6, 232–236. doi:10.1111/2041-210x.12310
Carvalho, J. C., Cardoso, P., and Gomes, P. (2012). Determining the Relative Roles of Species Replacement and Species Richness Differences in Generating Beta-Diversity Patterns. Glob. Ecol. Biogeogr. 21, 760–771. doi:10.1111/j.1466-8238.2011.00694.x
Chagnon, P.-L., Bradley, R. L., Maherali, H., and Klironomos, J. N. (2013). A Trait-Based Framework to Understand Life History of Mycorrhizal Fungi. Trends Plant Sci. 18, 484–491. doi:10.1016/j.tplants.2013.05.001
Chen, Y.-L., Xu, Z.-W., Xu, T.-L., Veresoglou, S. D., Yang, G.-W., and Chen, B.-D. (2017). Nitrogen Deposition and Precipitation Induced Phylogenetic Clustering of Arbuscular Mycorrhizal Fungal Communities. Soil Biol. Biochem. 115, 233–242. doi:10.1016/j.soilbio.2017.08.024
Davison, J., Moora, M., Öpik, M., Adholeya, A., Ainsaar, L., Bâ, A., et al. (2015). Global Assessment of Arbuscular Mycorrhizal Fungus Diversity Reveals Very Low Endemism. Science 349, 970–973. doi:10.1126/science.aab1161
Dodd, J. C., Boddington, C. L., Rodriguez, A., Gonzalez-Chavez, C., and Mansur, I. (2000). Mycelium of Arbuscular Mycorrhizal Fungi (AMF) from Different Genera: Form, Function and Detection. Plant Soil 226, 131–151. doi:10.1023/a:1026574828169
Egerton-Warburton, L. M., Johnson, N. C., and Allen, E. B. (2007). Mycorrhizal Community Dynamics Following Nitrogen Fertilization: a Cross-Site Test in Five Grasslands. Ecol. Monogr. 77, 527–544. doi:10.1890/06-1772.1
Fierer, N. (2017). Embracing the Unknown: Disentangling the Complexities of the Soil Microbiome. Nat. Rev. Microbiol. 15, 579–590. doi:10.1038/nrmicro.2017.87
Gosling, P., Jones, J., and Bending, G. D. (2016). Evidence for Functional Redundancy in Arbuscular Mycorrhizal Fungi and Implications for Agroecosystem Management. Mycorrhiza 26, 77–83. doi:10.1007/s00572-015-0651-6
Han, Y., Feng, J., Han, M., and Zhu, B. (2020). Responses of Arbuscular Mycorrhizal Fungi to Nitrogen Addition: A Meta‐analysis. Glob. Change Biol. 26, 7229–7241. doi:10.1111/gcb.15369
Hiiesalu, I., Pärtel, M., Davison, J., Gerhold, P., Metsis, M., Moora, M., et al. (2014). Species Richness of Arbuscular Mycorrhizal Fungi: Associations with Grassland Plant Richness and Biomass. New Phytol. 203, 233–244. doi:10.1111/nph.12765
Jiang, S., Liu, Y., Luo, J., Qin, M., Johnson, N. C., Öpik, M., et al. (2018). Dynamics of Arbuscular Mycorrhizal Fungal Community Structure and Functioning along a Nitrogen Enrichment Gradient in an alpine Meadow Ecosystem. New Phytol. 220, 1222–1235. doi:10.1111/nph.15112
Johnson, N. C. (2010). Resource Stoichiometry Elucidates the Structure and Function of Arbuscular Mycorrhizas across Scales. New Phytol. 185, 631–647. doi:10.1111/j.1469-8137.2009.03110.x
Koleff, P., Gaston, K. J., and Lennon, J. J. (2003). Measuring Beta Diversity for Presence-Absence Data. J. Anim. Ecol. 72, 367–382. doi:10.1046/j.1365-2656.2003.00710.x
Legendre, P., Borcard, D., and Peres-Neto, P. R. (2005). Analyzing Beta Diversity: Partitioning the Spatial Variation of Community Composition Data. Ecol. Monogr. 75, 435–450. doi:10.1890/05-0549
Lennon, J. J., Koleff, P., Greenwood, J. J. D., and Gaston, K. J. (2001). The Geographical Structure of British Bird Distributions: Diversity, Spatial Turnover and Scale. J. Anim. Ecol. 70, 966–979. doi:10.1046/j.0021-8790.2001.00563.x
Lepš, J., de Bello, F., Šmilauer, P., and Doležal, J. (2011). Community Trait Response to Environment: Disentangling Species Turnover vs Intraspecific Trait Variability Effects. Ecography 34, 856–863. doi:10.1111/j.1600-0587.2010.06904.x
Lilleskov, E. A., Kuyper, T. W., Bidartondo, M. I., and Hobbie, E. A. (2019). Atmospheric Nitrogen Deposition Impacts on the Structure and Function of forest Mycorrhizal Communities: A Review. Environ. Pollut. 246, 148–162. doi:10.1016/j.envpol.2018.11.074
Liu, X., Lyu, S., Zhou, S., and Bradshaw, C. J. A. (2016). Warming and Fertilization Alter the Dilution Effect of Host Diversity on Disease Severity. Ecology 97, 1680–1689. doi:10.1890/15-1784.1
Liu, X., Lyu, S. M., Sun, D. X., Bradshaw, C. J. A., and Zhou, S. R. (2017). Species Decline under Nitrogen Fertilization Increases Community-Level Competence of Fungal Diseases. Proc. R. Soc. B 284, 2016–2621. doi:10.1098/rspb.2016.2621
Liu, X., Zhang, L., Huang, M. J., and Zhou, S. R. (2020). Plant Diversity Promotes Soil Fungal Pathogen Richness under Fertilization in an alpine Meadow. J. Plant Ecol. 14 (2), 323–336. doi:10.1093/jpe/rtaa099
Liu, Y., Johnson, N. C., Mao, L., Shi, G., Jiang, S., Ma, X., et al. (2015). Phylogenetic Structure of Arbuscular Mycorrhizal Community Shifts in Response to Increasing Soil Fertility. Soil Biol. Biochem. 89, 196–205. doi:10.1016/j.soilbio.2015.07.007
Liu, Y., Shi, G., Mao, L., Cheng, G., Jiang, S., Ma, X., et al. (2012). Direct and Indirect Influences of 8 Yr of Nitrogen and Phosphorus Fertilization on Glomeromycota in an alpine Meadow Ecosystem. New Phytol. 194, 523–535. doi:10.1111/j.1469-8137.2012.04050.x
Lu, Y., Liu, X., Chen, F., and Zhou, S. (2020). Shifts in Plant Community Composition Weaken the Negative Effect of Nitrogen Addition on Community-Level Arbuscular Mycorrhizal Fungi Colonization. Proc. R. Soc. B. 287, 20200483. doi:10.1098/rspb.2020.0483
Lumini, E., Orgiazzi, A., Borriello, R., Bonfante, P., and Bianciotto, V. (2010). Disclosing Arbuscular Mycorrhizal Fungal Biodiversity in Soil through a Land-Use Gradient Using a Pyrosequencing Approach. Environ. Microbiol. 12, 2165–2179. doi:10.1111/j.1462-2920.2009.02099.x
Martínez-García, L. B., Richardson, S. J., Tylianakis, J. M., Peltzer, D. A., and Dickie, I. A. (2015). Host Identity Is a Dominant Driver of Mycorrhizal Fungal Community Composition during Ecosystem Development. New Phytol. 205, 1565–1576. doi:10.1111/nph.13226
Oksanen, J., Blanchet, F. G., Kindt, R., Legendre, P., Minchin, P. R., O’Hara, R. B., et al. (2013). Vegan: Community Ecology Package. R Package Version 2.0-7. Available at: http://CRAN.R-project.org/package=vegan Accessed October 10, 2020.
Öpik, M., Vanatoa, A., Vanatoa, E., Moora, M., Davison, J., Kalwij, J. M., et al. (2010). The Online Database MaarjAM Reveals Global and Ecosystemic Distribution Patterns in Arbuscular Mycorrhizal Fungi (Glomeromycota). New Phytol. 188, 223–241. doi:10.1111/j.1469-8137.2010.03334.x
Pan, S., Wang, Y., Qiu, Y., Chen, D., Zhang, L., Ye, C., et al. (2020). Nitrogen‐induced Acidification, Not N‐nutrient, Dominates Suppressive N Effects on Arbuscular Mycorrhizal Fungi. Glob. Change Biol. 26, 6568–6580. doi:10.1111/gcb.15311
Paradis, E., Claude, J., and Strimmer, K. (2004). APE: Analyses of Phylogenetics and Evolution in R Language. Bioinformatics 20, 289–290. doi:10.1093/bioinformatics/btg412
Podani, J., and Schmera, D. (2011). A New Conceptual and Methodological Framework for Exploring and Explaining Pattern in Presence - Absence Data. Oikos 120, 1625–1638. doi:10.1111/j.1600-0706.2011.19451.x
Pruesse, E., Quast, C., Knittel, K., Fuchs, B. M., Ludwig, W., Peplies, J., et al. (2007). SILVA: a Comprehensive Online Resource for Quality Checked and Aligned Ribosomal RNA Sequence Data Compatible with ARB. Nucleic Acids Res. 35, 7188–7196. doi:10.1093/nar/gkm864
R Development Core Team (2014). A Language and Environment for Statistical Computing. Vienna, Austria: R Foundation for Statistical Computing.
Sato, K., Suyama, Y., Saito, M., and Sugawara, K. (2005). A New Primer for Discrimination of Arbuscular Mycorrhizal Fungi with Polymerase Chain Reaction-Denature Gradient Gel Electrophoresis. Grassland Sci. 51, 179–181. doi:10.1111/j.1744-697x.2005.00023.x
Stegen, J. C., Lin, X., Konopka, A. E., and Fredrickson, J. K. (2012). Stochastic and Deterministic Assembly Processes in Subsurface Microbial Communities. Isme J. 6, 1653–1664. doi:10.1038/ismej.2012.22
Stevens, C. J., Dise, N. B., Mountford, J. O., and Gowing, D. J. (2004). Impact of Nitrogen Deposition on the Species Richness of Grasslands. Science 303, 1876–1879. doi:10.1126/science.1094678
Tedersoo, L., Bahram, M., and Zobel, M. (2020). How Mycorrhizal Associations Drive Plant Population and Community Biology. Science 367, 367. doi:10.1126/science.aba1223
Thébault, E., and Fontaine, C. (2010). Stability of Ecological Communities and the Architecture of Mutualistic and Trophic Networks. Science 329, 853–856. doi:10.1126/science.1188321
Tilman, D. (1987). Secondary Succession and the Pattern of Plant Dominance along Experimental Nitrogen Gradients. Ecol. Monogr. 57, 189–214. doi:10.2307/2937080
Treseder, K. K. (2004). A Meta‐analysis of Mycorrhizal Responses to Nitrogen, Phosphorus, and Atmospheric CO 2 in Field Studies. New Phytol. 164, 347–355. doi:10.1111/j.1469-8137.2004.01159.x
Vellend, M. (2001). Do commonly Used Indices of β‐diversity Measure Species Turnover? J. Vegetation Sci. 12, 545–552. doi:10.2307/3237006
Vellend, M. (2010). Conceptual Synthesis in Community Ecology. Q. Rev. Biol. 85, 183–206. doi:10.1086/652373
Whittaker, R. H. (1960). Vegetation of the Siskiyou Mountains, Oregon and California. Ecol. Monogr. 30, 279–338. doi:10.2307/1943563
Whittaker, R. H. (1972). Evolution and Measurement of Species Diversity. Taxon 21, 213–251. doi:10.2307/1218190
Wright, D. H., and Reeves, J. H. (1992). On the Meaning and Measurement of Nestedness of Species Assemblages. Oecologia 92, 416–428.
Wu, L., Si, X., Didham, R. K., Ge, D., and Ding, P. (2017). Dispersal Modality Determines the Relative Partitioning of Beta Diversity in Spider Assemblages on Subtropical Land-Bridge Islands. J. Biogeogr. 44, 2121–2131. doi:10.1111/jbi.13007
Xiang, X., Gibbons, S. M., He, J. S., Wang, C., He, D., Li, Q., et al. (2016). Rapid Response of Arbuscular Mycorrhizal Fungal Communities to Short-Term Fertilization in an alpine Grassland on the Qinghai-Tibet Plateau. PeerJ 4, e2226. doi:10.7717/peerj.2226
Xu, T., Veresoglou, S. D., Chen, Y., Rillig, M. C., Xiang, D., Ondřej, D., et al. (2016). Plant Community, Geographic Distance and Abiotic Factors Play Different Roles in Predicting AMF Biogeography at the Regional Scale in Northern China. Environ. Microbiol. Rep. 8, 1048–1057. doi:10.1111/1758-2229.12485
Zheng, Y., Kim, Y.-C., Tian, X.-F., Chen, L., Yang, W., Gao, C., et al. (2014). Differential Responses of Arbuscular Mycorrhizal Fungi to Nitrogen Addition in a Near Pristine Tibetan alpine Meadow. FEMS Microbiol. Ecol. 89, 594–605. doi:10.1111/1574-6941.12361
Keywords: beta diversity, community composition, nestedness, turnover, nitrogen addition
Citation: Lu Y, Liu X and Zhou S (2021) Nitrogen Addition and Arbuscular Mycorrhizal Fungi Beta Diversity: Patterns and Mechanisms. Front. Environ. Sci. 9:701653. doi: 10.3389/fenvs.2021.701653
Received: 28 April 2021; Accepted: 21 May 2021;
Published: 17 June 2021.
Edited by:
Wenxing Long, Hainan University, ChinaCopyright © 2021 Lu, Liu and Zhou. This is an open-access article distributed under the terms of the Creative Commons Attribution License (CC BY). The use, distribution or reproduction in other forums is permitted, provided the original author(s) and the copyright owner(s) are credited and that the original publication in this journal is cited, in accordance with accepted academic practice. No use, distribution or reproduction is permitted which does not comply with these terms.
*Correspondence: Shurong Zhou, emhzaHJvbmdAaGFpbmFudS5lZHUuY24=
†ORCID: Yawen Lu, orcid.org/0000-0002-3112-0705; Xiang Liu,orcid.org/0000-0003-3914-5824; Shurong Zhou, orcid.org/0000-0002-7093-1703