- 1Colombia Program, Wildlife Conservation Society, Cali, Colombia
- 2Ecology and Territory Department, Pontificia Universidad Javeriana, Bogotá, Colombia
Land-use change in the Colombian Llanos due to agro-industrial expansion affects biodiversity. This change alters species occurrence probability, consequently impacting species’ composition. For some species, the occurence probability increases with land-use changes, while it stays unchanged or decreases for others. This interspecific variation in the response to land-use change may be mediated by functional traits, among other factors. We investigated response functional traits to land-use changes and their influence on the occurrence probability of bird species in the Colombian Orinoquia region. We compiled data for 13 morphological and life-history traits of 364 species recorded in forests, savannas, rice fields, palm oil crops, and livestock pastures in the piedmont and flooded savanna landscapes. We used a novel framework to identify response functional traits (i.e., traits with a significant effect on occurrence probability) through multiple statistical tests. We used random forest models to identify response functional traits to land-use change for pairwise comparisons of natural vs. agricultural land use types. For the functional traits, we estimated the influence of their states as trait attributes on species’ responses to land-use changes. We identified functional groups based on hierarchical clustering analysis. Functional groups corresponded to different levels of response, that is, different changes in probability occurrence. Land-use changes altered the multidimensional space of bird traits (i.e., functional diversity), implying modifications in species' composition, functional redundancy, and functional group turnover. Functional traits were similar for random forest classifications of the same natural cover but differed among landscapes. In the piedmont forests, social behavior—migratory status—was a functional trait combination common to all classifications, while foraging behavior-nest location trait combination was common to all forests scenarios in flooded savannas landscape classifications. Migratory status was a functional trait for all savanna classifications. Functional groups described the impacts of land-use changes on bird assemblages. Identification and characterization of these groups using trait attributes can help predict species' responses to land-use changes and guide conservation efforts toward groups with decreased occurrence probability, including recommendations for agricultural practices that can reduce impacts on the Orinoquia biodiversity.
Introduction
The loss and degradation of natural habitats due to land-use changes are among the main drivers of biodiversity loss (Foley et al., 2005; Millenium Ecosystem Assessment, 2005; Baan et al., 2013; Deinet et al., 2018). It is estimated that vertebrate populations decreased by at least 60% worldwide and 89% in the Neotropics between 1970 and 2014, where more than half was due to loss and degradation of natural habitats (Deinet et al., 2018). Agriculture is the primary driver of land-use changes (Lambin et al., 2001; Meyfroidt et al., 2013). Understanding its effects on biodiversity can help identify practices that lessen these impacts, predict and mitigate future biodiversity changes, and design monitoring approaches for conservation strategies, including sustainable agriculture (Wezel et al., 2014). The Sustainable Development Goals program promotes sustainable agriculture and reduces its effects on the degradation of natural habitats. Such sustainability could be supported by developing indicators that aid in understanding and predicting the impacts of anthropic pressures and the design and monitoring of conservation strategies (Burtchart et al., 2010; Biodiversity Indicators Partnership, 2011).
Traditionally, land-use impacts on biodiversity are measured using taxonomic metrics (based on the species count), limiting the possibility of thoroughly understanding the effects of anthropic activities (Branquinho et al., 2019). However, the impact may occur at different levels of biodiversity (e.g., genetic, species, and ecosystem), as well as in the underlying mechanisms that sustain it, leaving taxonomic metrics telling only part of the story (Flynn et al., 2009; Mouillot et al., 2013; Pearson et al., 2014; Titeux et al., 2016). Taxonomic metrics do not take into account the multidimensional space that each species represents and assume them as units of ecological equivalence, for instance, regarding their effects on the ecosystem and their survival probabilities (Chave, 2004; De Souza et al., 2013; Luck et al., 2013; Córdova-Tapia and Zambrano, 2015). Accordingly, the taxonomic approach should be complemented with additional ecological metrics to better understand the impacts of land cover transformations on biodiversity, leading to better-informed strategies to prevent or mitigate them (Pereira et al., 2013).
Functional ecology studies assign values to each species based on functional traits and their different states as the range of functional attributes it occupies within the multidimensional space representing species assemblage (Luck et al., 2012). We refer to trait attributes as different values, levels, or states of a trait. Functional traits can be classified into effect or response traits (Luck et al., 2012). The former describes the effect patterns of a species assemblage on ecosystem processes (e.g., dispersal strategy), while response traits describe responses of a species assemblage to environmental disturbances or changes (Hooper et al., 2002; Lavorel and Garnier, 2002; Naeem and Wright, 2003). Therefore, the effect trait analysis allows describing the ecosystem functions, while the response trait analysis is useful in understanding the assemblage's responses to the impacts of environmental disturbances. The functional response refers to the influence of functional traits on the response of the assemblage to environmental changes. Functional trait analyses are based on the collection of multiple individual traits and the assessment of their effects on ecosystems or responses to ecosystem disturbance, leading to the identification of the functional groups of species that represent particular effects or response patterns (Diaz and Cabido, 2001; Hooper et al., 2002; Luck et al., 2012). These add up to our understanding of how ecosystems function and their tolerance to disturbances. Global meta-analyses conducted to assess the impacts of anthropic interventions on bird assemblages show a decrease in functional diversity and describe the implications of these changes for the provision of ecosystem services (Flynn et al., 2009; Newbold et al., 2013; Bregman et al., 2014; Matuoka et al., 2020).
The Colombian Llanos or the Orinoquia region is a region of great environmental heterogeneity that supports a high biological diversity (Romero Ruíz et al., 2004; Lasso et al., 2010, 2011). In recent decades, socioeconomic development has accelerated the expansion of livestock farming, oil exploitation, and agribusiness related to oil palm crops, rice, and others, which have exposed the region to the socioecological transformation processes of uncertain environmental and socioeconomic impacts (Romero Ruíz et al., 2004, 2011; Andrade et al., 2009). Furthermore, current national policies promote the Orinoquia region as the focal area for agricultural expansion, with 56% of this region being part of the agricultural frontier and available for agricultural activities (UPRA, 2021). This generates an urgency for developing mechanisms to assess and account for the potential impacts that this transformation could impose on the biodiversity and for integrating this information into decisions that support the region’s sustainable development.
We identified “response functional groups” to land-use changes from natural to agricultural land cover types to identify and understand patterns of changes in bird assemblages in response to land cover transformation. We determined the response of a set of functional traits (functional response traits) to changes of natural land cover (forests and savannas) to agriculture (livestock, rice, and oil palm crops) in the Llanos piedmont (LP) and flooded savanna (FS) landscapes of the Colombian Llanos. We identified the functional response patterns to land-use changes and the functional traits and attributes that modulate such responses. These functional groups and response patterns are helpful indicators to assess land-use change impacts and monitor biodiversity in response to agricultural activities.
Materials and Methods
Study Area
The Colombian Llanos is a savanna-dominated region with high ecosystem diversity, it is bounded by the Orinoco River basin in the north of South America and extends for about seventeen million hectares and covering three general types of large-scale landscapes (i.e., LP, FS, and the Altillanura) (Romero Ruíz et al., 2004). Due to its extension, there are sites with a greater number of bird records, for instance, there are more complete datasets of avifauna both in natural and agricultural covers for LP and FS than Altillanura. Therefore, our study focused on LP and FS landscapes (Figure 1), which include around 54 and 69% of all bird species present in the entire region, respectively (Acevedo-Charry et al., 2014). The Colombian Llanos has an annual average temperature ranging between 25 and 28°C, showing a binomial rain regime with a dry period between January and April, and a rainy season from March to December with higher precipitation in June (IDEAM, 2021).
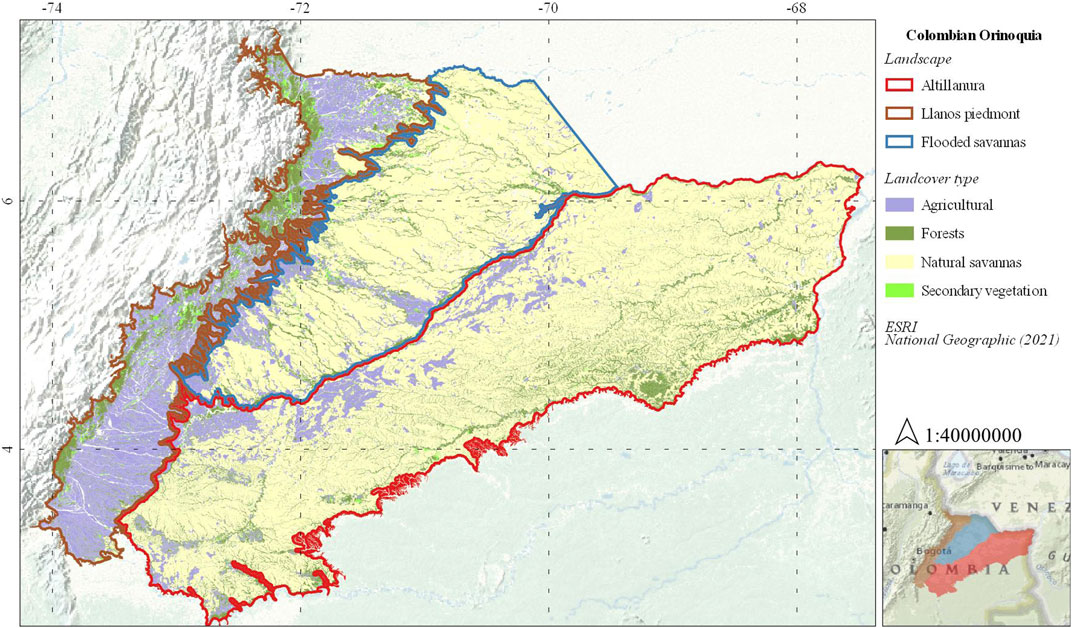
FIGURE 1. Study area. Colombian Orinoquia landscapes. Modified from (Romero Ruíz et al., 2004; IDEAM, 2017).
The Llanos piedmont is an ecotone landscape between the mountain ranges of the Andes at 1,100 m elevation and the Orinoco plains at 400 m, characterized by erosional ridges, alluvial terraces, mountains, and piedmont forests (Romero Ruíz et al., 2004). However, anthropic intervention changed the natural landscape by turning over 80% of the area into a matrix dominated by livestock pastures, crops, and degraded natural ecosystems. Forests currently represent around 10% of the landscape distributed in small fragments and riparian vegetation (IDEAM, 2017).
The flooded savanna corresponds to a “flooded landscape” between 0 and 400 m elevation characterized by seasonally flooded savannas, riverine forests, wetlands, and swamps (Figure 1) (Romero Ruíz et al., 2004). Anthropic intervention in this landscape has transformed at least 20% of the area, making it fit mainly for livestock pastures, and crops, degrading natural ecosystems until reduce them as patches around rivers. In the FS landscape, forests comprise around 10% of the territory, while seasonally flooded savannas represent around 60% (Figure 1) (IDEAM, 2017).
Data Collection
We compiled species occurrence data from bird surveys taken between 2000 and 2018 in the FS and LP landscapes. We generated an occurrence database from survey data and referred publications that included bird records at the species level with associated data on the date, locality, coordinates, and land cover types (Gilroy et al., 2015; López-Ricaurte et al., 2017; GBIF.org, 2018; Diaz Lopez et al., 2020; Rodríguez Posada et al., 2020). We classified records in the FS landscape as natural (forest or savanna) or agricultural (livestock pastures, rice fields, or oil palm crops). In the LP region, records of the natural land cover corresponded only to forests, while agricultural land uses include the same classes used in the FS landscape. We verified species occurrences based on the most updated bird species checklist for this region (Acevedo-Charry et al., 2014) and followed the taxonomical classification proposed by Remsen et al. (2021).
Trait Data
We performed a systematic literature review on studies concerning functional diversity in birds. We examined with particular emphasis on studies covering the Colombian LLanos region and global studies that analyzed functional traits in the anthropic cover, compared functional diversity between natural and anthropic covers, or analyzed functional response traits to anthropic disturbances. We chose 13 potential response traits to environmental changes, following the selection framework for vertebrate response traits as proposed by Luck et al. (2012), including morphological and life-history traits that could influence birds’ responses to land-use changes based on a literature review (see Supplementary Material S1). The life-history traits considered were social behavior, diet, migratory status, foraging stratum, foraging behavior, and nest location, which were compiled from the scientific literature (Hilty and Brown, 1986; Ridgely et al., 1989; Restall et al., 2007; Ridgely and Tudor, 2009; Birds of the World, 2021). Morphological traits included bill depth, bill width, wing length, tail length, culmen length, tarsus length, and weight. These were measured on specimens collected in the Orinoquia region and preserved in Colombia’s biological collections at the Instituto de Ciencias Naturales of the Universidad Nacional de Colombia, Instituto Alexander von Humboldt, and Museo de Historia Natural of the Pontificia Universidad Javeriana (Stiles et al., 2017; Córdoba Córdoba et al., 2018; Laverde Rodríguez et al., 2018). We measured six adult specimens of every species, three females and three males, depending on availability. If not enough individuals were available, we would complete the information with measures reported in the literature. We averaged morphometric species values for each species. Morphometric measures were taken only by the first author to minimize interobserver bias. However, for 5% of the species, we obtained morphometric data from field reports, which may have added bias due to differences in measurements between preserved and live individuals or between observers. The databases of life-history and morphometric traits collected are available in the EDI data repository (Rincon-Parra, 2021).
Data Analysis
We generated pairwise classification models between each natural and agricultural land cover type for each landscape. These predictive models
The model
For every pairwise classification model, we considered all the compiled species traits as the independent variables
RF models with high success rates represent a high probability of species with a particular trait pattern occurring differentially in the classified land cover types. In contrast, RF models with low success rates suggest the lack of significant differences in trait patterns among compared land cover types. High success rates also indicate a high probability of change in the species occurrence as a response to land-use changes as determined by their traits.
We estimated the importance of attributes’ combinations of response functional traits through partial dependence analysis (Breiman, 2001; Liaw and Wiener, 2002; Eric and Archer, 2020). We standardized partial dependence values on a scale between −1 and 1, in which an attribute's combination closer to 1 indicated an increased probability of species with those attributes occurring in the agricultural land cover. In contrast, values closer to −1 indicated an increased probability of species with those attributes occurring in the natural cover. Thus, in a land-use change scenario (e.g., forests to livestock pastures, and savannas to oil palm crops), species with attribute combinations with values closer to −1 would experience a decrease in their occurrence probability, while those with attribute combinations with values closer to 1 would result in increased occurrence probability. On the other hand, species with attribute combinations with partial dependence values around zero do not show a particular response pattern to land-use changes.
We applied a hierarchical cluster analysis to the species characterized by the partial dependence values of attributes combinations to identify functional response groups. The number of functional groups was defined by multiscale bootstrap resampling (Hennig, 2020). Functional groups corresponded to cluster-wise, highly stable groups (i.e., stability values higher than 0.75) (Hennig, 2007; Hennig, 2020).
Results
We collected 11,444 records representing 364 species, 193 in LP and 357 in FS. The two landscapes shared 186 species, while 171 were exclusively reported in FS, and only seven were recorded in LP. In the two landscapes, natural land covers showed greater species richness than agricultural ones, accounting for 21% of the total bird species recorded in LP, and for 38 and 36% of the total bird species in forests and savannas of FS, respectively (Figure 2).
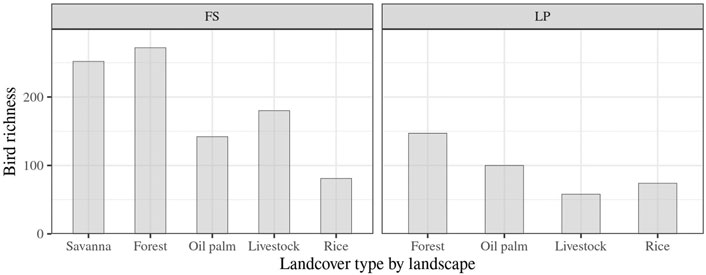
FIGURE 2. Bird species richness collected according to the land cover type and landscape. Landscapes: LP: Llanos piedmont; FS: flooded savannas.
Of the 13 traits measured and estimated for all species, 11 corresponded to response functional traits alone or in trait combinations for at least one classification. Tail length and weight were the only traits that did not show a functional response to land-use changes. Overall, functional response traits were similar for RF pairwise classifications of the same natural cover but differed among landscapes (Figure 3). For a given natural land cover, all possible classifications’ included at least one response trait or a trait combination common to all for a given natural land cover. In LP forests, social behavior–migratory status was identified as a response trait combination in all classifications. For LP forests’ livestock classification, foraging the stratum diet and migratory status–culmen length correspond to additional trait combinations showing a functional response, while foraging behavior–nest location and foraging stratum–tarsus length were added to social behavior–migratory status in the forests’ rice classification (Figure 3). Foraging behavior–nest location (FB-NL) was common to all RF forest models and is the only trait combination in classifications with livestock and oil palm crops. In the FS landscape forests’ rice model, supplementary trait combinations corresponded to bill width–foraging stratum–nest location, bill depth–foraging stratum–nest location, and nest location–wing length (Figure 3). Despite nest location and foraging stratum being shared across trait combinations in this latter classification, we found no correlation between them. In savannas of the FS landscape, the migratory status was in pairwise classifications, combined with social behavior in the livestock and oil palm classification and with the foraging stratum in the rice comparison (Figure 3). Higher numbers of identified functional response traits corresponded to higher success rates in RF models (Figures 3, 4). These rates were the highest (>0.7) for forests’ rice scenarios in both landscapes (i.e., there is, on average, a 70% chance of species differing between land cover classes, given their response traits), followed by the FS savannas’ rice classification (0.65) (Figure 4). All RF classifications, including oil palm, resulted in similar success rates in both landscapes, that is, there is, on average, a 50% probability of species differing between the natural land cover and oil palm, given their response traits. The forests’ livestock classification showed a slightly higher success rate in LP (0.6) than in FS (0.55). The success rate of the FS savannas’ livestock (0.51) suggested a close to random chance of correctly classifying species into savanna or livestock based on their attributes of response traits (Figure 4). However, these rates represent the classification probabilities for all species in the assemblages, overlooking the differences in the functional group's responses.
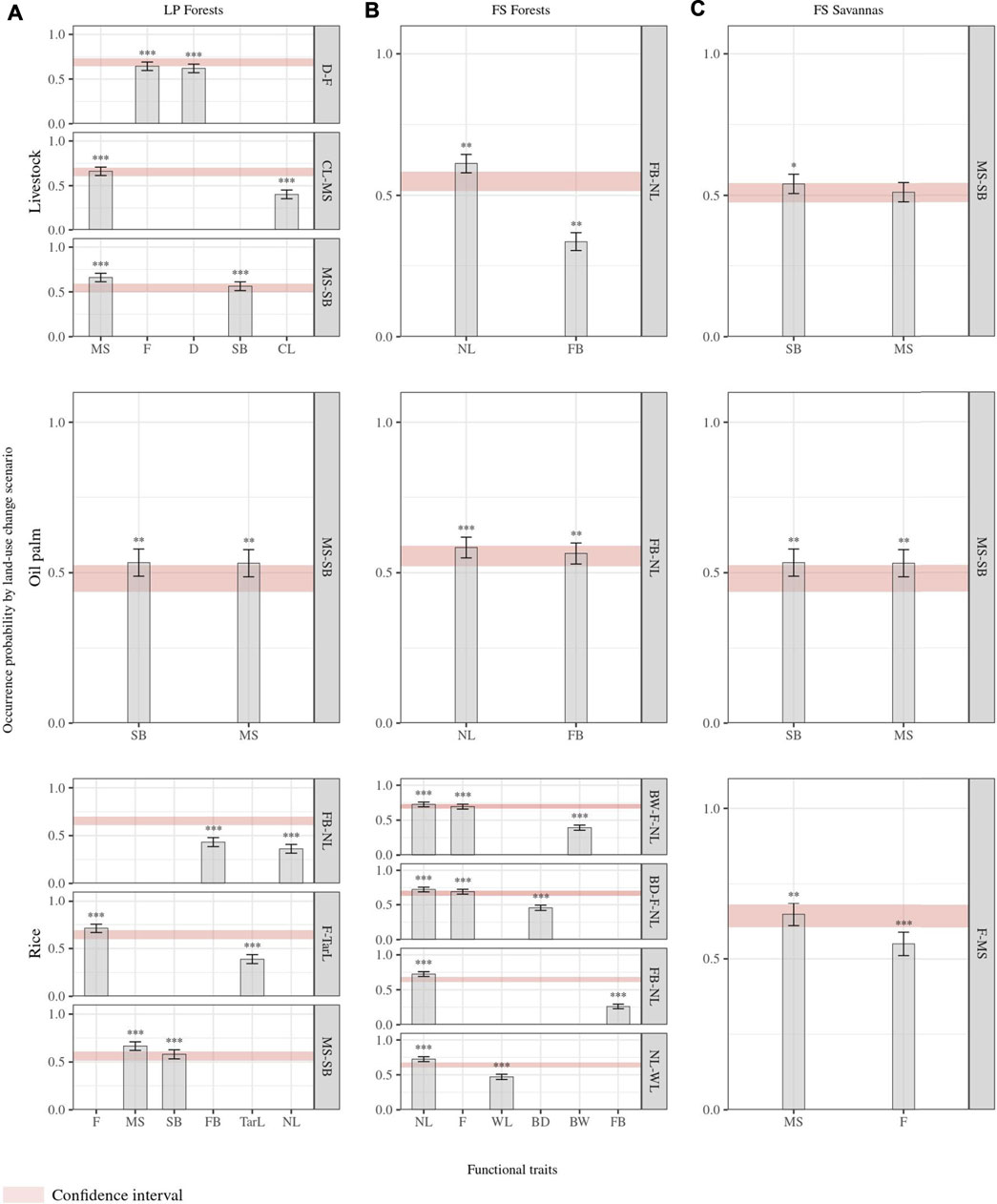
FIGURE 3. Importance of response functional traits. The y-axis represents the probability of influence of each trait to predict changes in species occurrence due to land-use changes. Confidence intervals represent the probabilities of the influence of trait combinations of each trait to predict species occurrence changes. We calculated probability values from RF models with individual and combined traits. (A). Land-use change scenarios from forests to agricultural covers in the Llanos piedmont (LP) landscape; (B). land-use change scenarios from forests to agricultural covers in the flooded savanna (FS) landscape; (C). land-use change scenarios from natural savannas to agricultural covers in the FS landscape. Traits: BD: bill depth length at the base level; BW: bill width length at the base level; WL: wing length; CL: culmen length; Tarl: tarsus length; SB: social behavior; D: diet; MS: migratory status; F: foraging stratum; FB: foraging behavior; NL: nest location. Asterisk brackets indicate significance values of traits estimated from the mean decrease accuracy metric (MDA) after five thousand permutation null models for each combination trait by scenario. * p-value < 0.1, ** p-value < 0.05, *** p-value < 0.01.
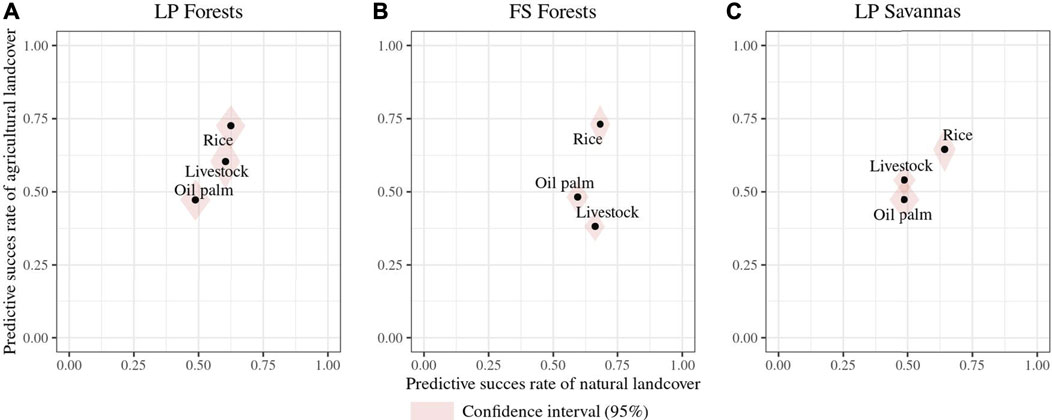
FIGURE 4. Predictive success rates of the classification of RF models for changes in species occurrence probabilities (according to traits) in land-use change scenarios. The x-axis represents predictive success to classify species in natural covers, and y-axis represents predictive success to classify species in agricultural covers. The points represent the predictive success of each scenario in which high predictive rates mean a high probability of the change of species occurrence probabilities as a response to land-use changes. (A). Land-use change scenarios from forests to agricultural covers in the Llanos piedmont (LP) landscape; (B). land-use change scenarios from forests to agricultural covers in the flooded savanna (FS) landscape; (C). land-use change scenarios from natural savannas to agricultural covers in the FS landscape.
We found similar numbers of functional groups (10–13) for all pairwise classifications (i.e., land-use change scenarios), except for forests’ rice and savannas’ rice scenarios in the FS landscape, for which the number of functional groups reached 20 and 17, respectively (Figure 5, Supplementary Material S2). Most scenarios produced homogeneous functional groups with low variability in their partial dependence values. Scenarios resulting in groups with more variable partial dependence values corresponded to classification models with morphometric traits as functional response traits (Figure 5). For example, LP forests’ livestock response traits included culmen length, LP forest’s rice included tarsus length, and FS forest rice included bill width, bill depth, and wing length.
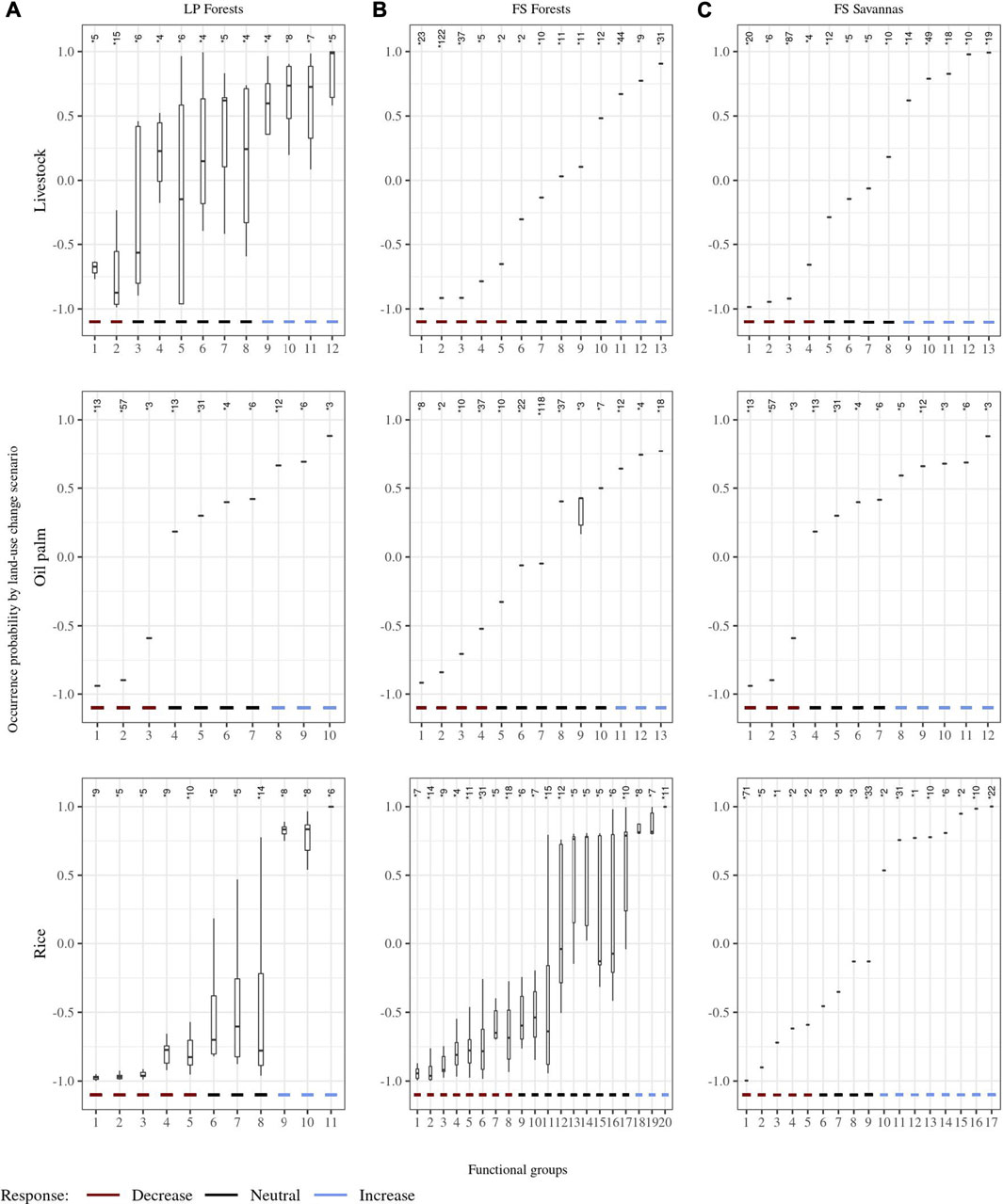
FIGURE 5. Response functional groups to land-use change scenarios. The x-axis represents the functional groups by land-use change and the y-axis the range of their partial responses to change. Responses nearest to 1 and -1 mean groups with species with a high probability to increase or decrease, respectively, their occurrence probability after the change. * Number of species by group. (A). Land-use change scenarios from forests to agricultural covers in the Llanos piedmont (LP) landscape; (B). land-use change scenarios from forests to agricultural covers in the flooded savanna (FS) landscape; (C). land-use change scenarios from natural savannas to agricultural covers in the FS landscape.
In all cases, land-use changes resulted in groups’ turnover, with the occurrence probability decreasing for a proportion of groups (i.e., partial dependence value closer to −1) and increasing (i.e., partial dependence value closer to 1) or staying unchanged for others (i.e., partial dependence values around zero) (Figure 5). In terms of functional groups, LP forests’ rice scenario resulted in the largest proportion of groups (0.45), suffering a reduction in occurrence probability. In contrast, a change of LP forests to livestock reduced the occurrence probability in 17% of functional groups and 27% of species, representing the smallest proportion of groups with reduced occurrence probability across all land-use change scenarios. Savannas’ rice scenario in FS savannas’ rice scenario resulted in the largest proportion of functional groups (0.47) with increased occurrence probability, while only 15% groups in the FS forests’ rice scenario, the smallest proportion among all scenarios, experienced an increment (Figure 5).
On average, the proportion of functional groups with decreased occurrence probability (0.32) was similar to that of groups with increased occurrence probability (0.31). Land-use change scenarios involving forest transformation resulted in similar or slightly larger proportions of groups with decreased occurrence probability than increased probability. However, FS savannas changes showed a higher proportion of groups with increased occurrence probability (0.42) than decreased probability (0.28).
The number of species differed among functional groups, influencing the response pattern for functional groups across land-use change scenarios (Figure 5). The largest proportion of species (0.59) with decreased occurrence probability corresponded to the FS forests’ livestock scenario, followed by the FS forests’ rice scenario (0.51), and the LP forests’ oil palm scenario (0.49). The highest proportion of species with increased occurrence probability appeared in the FS savannas’ livestock scenario (0.42), followed by FS savannas’ rice scenario (0.40). The average proportion of species across all scenarios with decreased occurrence probability was higher (0.42) than that of species with increased occurrence probability (0.25). In contrast with the results by functional groups, in the FS savannas scenarios, the proportion of species with decreased occurrence probability (0.43) was larger than that with increased probability (0.34) (Figure 5). On average, the occurrence probability for around a third of the functional groups (0.37) and species (0.33) remained unchanged across land-use change scenarios.
In all LP forest scenarios, functional groups with reduced occurrence probability included the traits and attribute combinations: (1) resident migratory status and solitary social behavior or (2) resident migratory status and opportunistic gregarious social behavior. Accordingly, functional groups with increased occurrence probability corresponded to 1) local migratory and social behavior in flocks or 2) continental migratory and social behavior in flocks. In LP forests’ livestock scenarios, functional groups with increased occurrence probability were also characterized by a long culmen, piscivore or insectivore diet, and foraging in water and ground. At the same time, groups with decreased occurrence probability corresponded showed such as a short culmen, insectivore diet, and foraging in the understory. The LP forests’ rice scenario exhibited increased occurrence probability in groups that forage in water and have long tarsus, while groups with short tarsus and foraging in all forest levels characterized groups with decreased occurrence probability. Similarly, this probability decreased for groups characterized by gatherer foraging behavior and nesting in cavities and branches but increased for groups with hunter foraging behavior (Figure 3, Supplementary Material S3). In FS forest scenarios with livestock and oil palm, functional groups with gatherer foraging behavior, nest location in branches, and cavities had reduced occurrence probability. However, functional groups with gatherer foraging behavior and foraging on the ground resulted in increased probability. In addition to these traits, groups with short wings, foraging in all forest levels, narrow bill, and hunter foraging behavior had reduced occurrence probability in FS forests’ rice scenarios. This probability increased for groups with long wings, a long bill or narrow bill, and foraging in water (Figure 3, Supplementary Material S3).
In all savannas scenarios of the FS landscape, functional groups characterized by a resident migratory status showed lower occurrence probability, while groups with a local migratory status had increased probability. Moreover, both livestock and palm scenarios reduced probability for groups with solitary social behavior and increased probability for those with opportunistic social behavior or in flocks. In FS savannas rice scenarios, occurrence probability decreased for groups with foraging in the understory and increased for groups with foraging in water (Figure 3, Supplementary Material S3).
Discussion
Our results described probabilistic response patterns of bird assemblages to land-use changes and indicate that these responses are nonrandom but rather deterministic trait-mediated. This is the first study studying functional response traits of birds in the Orinoquia region to estimate functional groups’ responses to land-use change scenarios and identifying the traits and attributes that determine them. It should be noted that, since morphological traits obtained from collection specimens could not be linked to specific land cover types, we assumed no intraspecific variation in these traits across different land cover types. For future studies, we recommend that morphometric variables are measured directly in the field, considering the land cover type on which individuals are sampled.
Shared response traits or trait combinations identified for all pairwise classifications of the same natural land cover type, indicate the presence of common functional response patterns of species assemblages to environmental disturbances. Changing forests to agricultural lands in LP influenced species occurrence probabilities based on their migratory status and social behavior, independently of the agricultural land cover type. Resident and solitary or opportunistic gregarious species would tend to disappear when replacing forests with agriculture, which could be associated with higher forest specialization in resident species and increased detectability of solitary species in more open vegetation, resulting in increased predation risk and reduced food availability (Hutto, 1989; Newton, 1998; Thiollay and Jullien, 1998; Sridhar et al., 2009; Barbe et al., 2018). This also explains why local or continental migratory species that flock are favored by transformation to agriculture (Smith et al., 2001; Goldstein et al., 2003; Barbe et al., 2018). Similarly, forest conversion to agriculture in the FS landscape affected species occurrence based on foraging behavior and nest location, while migratory status influenced species responses in all savanna classifications. Additional trait combinations mediated the distinct responses exhibited in individual land-use change scenarios.
Classification models differed in their success rates, with models including rice showing higher rates than others. High rates indicate that selected response traits can effectively explain the differences in species occurrence probabilities between natural and agricultural land cover types. However, the success rates for models involving oil palm crops and cattle were close to 0.5, suggesting a poor classification performance of the RF model. Potential explanations for this poor performance include missing functional traits that could be important for the classifications considered in this study and model limitations by focusing solely on traits while ignoring other factors influencing species occurrence probabilities (Newbold et al., 2013). However, identifying functional groups and their responses suggests a potential bias in success rate estimation when all species are assumed equal. When functional group responses are highly variable, their increasing and decreasing response patterns cancel each other out, which does not allow the RF model to classify assemblages between land cover types differentially. This highlights the idea that posterior functional group evaluation can provide a better picture of the impact of environmental pressures on species assemblages.
Different studies have shown that the functional diversity of bird communities varies depending on particular environmental conditions such as environmental gradients and the transformation of the landscape (Clavero and Brotons, 2010; Bregman et al., 2014; Jarzyna et al., 2020). Our results show that changes in land use determine the occurrence of species with particular traits because anthropic disturbances produce changes in the functional spectrum of traits (Mayfield et al., 2010; Aiba et al., 2016). The scale of analysis of this research encompasses the response functional patterns to land-use changes of natural covers by anthropic ones as a process related to the transformation of the landscape. However, functional diversity analysis at finer scales could consider environmental gradients or of anthropic transformation to obtain more detailed results. For example, from a taxonomic perspective, the Colombian Llanos present the greatest changes in bird species toward areas with a greater altitudinal and latitudinal gradient (Acevedo-Charry, 2017; Trujillo and Henao-Cárdenas, 2018), for which it would be interesting to analyze patterns of functional diversity in future studies.
The identity of natural systems is determined by the functional traits that make them up, and their resilience is based on maintaining their functional space (Allen et al., 2005; Gladstone-Gallagher et al., 2019; Roberts et al., 2019). Differential responses between functional groups result in a turnover of functional groups and species, modifying the functional space of traits between land cover types, thus resulting in changes in the species number, composition, functional diversity, and functional redundancy (i.e., frequency of representation of the same attribute traits) (Figure 5). The direction of these changes would depend on the proportion of functional groups showing a particular response (increase, decrease, or neutral) in occurrence probability and the number of species represented in these groups. When a larger proportion of functional groups containing a larger proportion of species increased their occurrence probability, an increase in functional diversity and species number increase as expected. However, none of the scenarios considered in this study showed this pattern. In the FS savannas rice scenario, a larger proportion of functional groups increased their occurrence probability, but the proportion of species represented in these groups (0.4) was similar to those in groups experiencing a decrease in occurrence probability (0.38), suggesting an increase in functional diversity and a species turnover. When a larger proportion of functional groups increases their occurrence probability, but the proportion of species represented in these groups is the smallest (e.g., FS savannas oil palm), the functional diversity might increase but with low functional redundancy and a reduction in species numbers.
Consequently, a larger proportion of functional groups with decreased occurrence probability than with increased probability suggests a decline in functional diversity. This occurred in all FS forest scenarios and LP forests’ rice scenarios. In these scenarios, the proportion of species in the groups with decreased occurrence probability was also larger, indicating a reduction in species numbers. This decline in species richness was greater for the FS forests’ livestock scenario, followed by the FS forests’ rice scenario, where these groups accounted for more than 50% of the species. In contrast, in the FS forests’ oil palm scenario, a smaller percentage of species (20%) are represented in the functional groups with decreased occurrence probability, while 68% are included in the groups with neutral responses, suggesting smaller changes in species composition.
Land-use change scenarios that caused stronger responses on the functional group occurrence probability implied significant changes in the vegetation structure, mainly resulting in a simplification of the habitat complexity (Batisteli et al., 2018). This can be related to the habitat hypothesis, in which higher habitat heterogeneity, as indicated by its structural complexity, provides higher diversity of niches and thus sustains a higher diversity (Hurlbert, 2004; Tews et al., 2004). In turn, this may be reflected in the functional niche of organisms and groups so that higher spatial heterogeneity can lead to higher functional richness (Wiescher et al., 2012; Nooten et al., 2019). Increased diversity due to habitat complexity is not generalizable from taxonomic metrics (Tews et al., 2004; Cousin and Phillips, 2008), but complex habitats provide a high diversity of conditions from a functional perspective, increasing the diversity of functional groups. It should be noted that habitat structural complexity is just one heterogeneity dimension; thus, analyzing other landscape configuration metrics and environmental variability would lead to a better understanding of the species assemblages’ responses (Stein et al., 2014; Tuanmu and Jetz, 2015).
The identification of the functional trait combination nest location–foraging behavior in all FS forest scenarios illustrates the importance of assessing combined traits. Neither nest location nor foraging behavior was significant as individual traits in any forest scenario. Species that obtain resources through active search in different substrates (gatherer foraging behavior) were common in different land-use scenarios as part of functional groups that differed in their responses to land-use changes, based on the nest location attribute (Martin and Possingham, 2005; Hua et al., 2016; Sitters et al., 2016). Groups that forage by gathering and nest in tree cavities would suffer a decrease in occurrence probability because they usually forage vertically in the forests. The loss of vertical structures by deforestation would reduce habitat availability for foraging and nesting. In contrast, groups that forage by gathering and nest on the ground showed higher occurrence probabilities in agricultural areas as they would find an increased availability of open areas for nesting with sparse vegetation on which they scratch in search of food.
The methodological framework we used relied on probabilistic models to describe the impact of land-use changes on bird assemblages and the functional groups, traits, and attributes that explain the species response pattern. RF models estimate the response probabilities of bird assemblages and the occurrence probabilities of groups of species with particular traits that mediate the response to land-use changes. This method allows one to overcome one of the most significant challenges in studying functional diversity, that is, developing statistical procedures to assess and identify functional traits (Ricotta and Moretti, 2010; Luck et al., 2012; Villéger et al., 2017). Unlike previous studies on functional response diversity (Mouillot et al., 2013), our analyses estimated response functional groups, not just as species clusters with similar traits, but rather as groups of species with similar responses influenced by their traits. Thus, functional response groups should be defined based on their responses rather than on their traits (Hooper et al., 2002; Lavorel and Garnier, 2002; Naeem and Wright, 2003).
Our results coincide with previous studies assessing the impacts of agricultural transformation on birds in this region and showing a decrease in bird species richness in agricultural land cover types compared to natural ones (Edwards et al., 2015; Gilroy et al., 2015; Tamaris-Turizo et al., 2017). Few studies explored the impacts of land-use changes on functional diversity in the Orinoquia region. Prescott et al. (2016) concluded that functional diversity is greater in forests than in oil palm crops and livestock pastures in the FS landscape, while López-Ricaurte et al. (2017) found a decrease in taxonomic richness and functional diversity in livestock pastures and oil palm crops for bird assemblages of savannas.
Potential applications of our results include using species that represent response functional groups (e.g., in LP little wrens are vulnerable while medium size water birds of prey benefit from changing forest to livestock) as indicators to monitor impacts of land-use change on the Llanos biodiversity (Supplementary Appendix S4). In addition, changes in the composition of response functional traits associated with agricultural land cover could reflect changes in functional processes of ecosystems that may impact the provision of ecosystem services. We have explored how the different bird communities within and between land-cover respond to change. However, there is room to inquire about how these remaining functional communities’ impact or affect the land-cover themself. We suggest assessing effect functional traits to understand these effects on ecosystem processes and services. Our results indicate that agricultural practices can be improved to reduce impacts on species with particular response trait attributes that are mostly affected by land-use change (Supplementary Material S4).
The analysis of response functional groups can be used as a predictive tool on the impact and/or monitoring of land-use changes on biodiversity, so that strategies to avoid or mitigate them can be designed and implemented. Furthermore, the functional response traits and species representing functional groups can be used as indicators of the assemblage responses to changue as improved agricultural practices or conservation actions in agricultural areas. Patterns of functional traits expose conditions that can be targeted in the design of management strategies to minimize the impacts of agricultural expansion, while the identification of response functional groups support the identification of indicator species for monitoring the impact of such strategies on biodiversity in the Colombian Llanos.
Data Availability Statement
The original contributions presented in the study are included in the article/Supplementary Materials; further inquiries can be directed to the corresponding author
Ethics Statement
Ethical review and approval was not required for the animal study because this research was carried out using secondary information and biological collections.
Author Contributions
SA proposed the main idea of this study. VR-P reviewed the literature, proposed the methodological framework, collected the data, and performed the computations and analysis. SA and ME-G supervised the findings of this research. All the authors contributed to the discussion on the results and the preparation of the manuscript.
Funding
This study was supported by the “Land Use Change in the Orinoquia” working group of the Science for Nature and People Partnership (SNAPP) and funded by Arcadia Foundation and Fundación Santo Domingo.
Conflict of Interest
The authors declare that the research was conducted in the absence of any commercial or financial relationships that could be construed as a potential conflict of interest.
Publisher’s Note
All claims expressed in this article are solely those of the authors and do not necessarily represent those of their affiliated organizations, or those of the publisher, the editors, and the reviewers. Any product that may be evaluated in this article, or claim that may be made by its manufacturer, is not guaranteed or endorsed by the publisher.
Acknowledgments
We are grateful to O. Laverde-R for providing constructive feedback around the design and conceptual framework in the early stages of this study. We thank Polygrow Colombia S.AS, M. E. Rodriguez-Posada, L. Lopez-Ricaurte, and G. Prescott for sharing their valuable data collected in the Colombian Llanos. Finally, we acknowledge the support provided by F. G. Stiles, S. Córdoba-Córdoba, and D Forero, who, as museum curators at the time, permitted us to access the bird collections.
Supplementary Material
The Supplementary Material for this article can be found online at: https://www.frontiersin.org/articles/10.3389/fenvs.2021.689745/full#supplementary-material
References
Acevedo-Charry, O. (2017). Birds of Río Tame of the Andes-Orinoco Transitional Region: Species Check-List, Biogeographic Relationship and Conservation. Ornitol. Colomb. 16, eA03–1.
Acevedo-Charry, O., Pinto-Gómez, A., and Rangel-Ch, O. (2014). Las aves de la Orinoquia colombiana: Una revisión de sus registros. Colomb. Divers. Biótica 14, 691–750. Available at: https://www.researchgate.net/publication/265643904.
Aiba, M., Kurokawa, H., Onoda, Y., Oguro, M., Nakashizuka, T., and Masaki, T. (2016). Context-dependent Changes in the Functional Composition of Tree Communities along Successional Gradients after Land-Use Change. J. Ecol. 104, 1347–1356. doi:10.1111/1365-2745.12597
Allen, C. R., Gunderson, L., and Johnson, A. R. (2005). The Use of Discontinuities and Functional Groups to Assess Relative Resilience in Complex Systems. Ecosystems 8, 958–966. doi:10.1007/s10021-005-0147-x
Andrade, G. I., Castro Gutierrez, L. G., Duran Duran, A., Rodríguez Becerra, M., Rudas Lleras, G., Uribe Botero, E., et al. (2009). La mejor Orinoquia que podemos construir, Elementos para la Sostenibilidad Ambiental del Desarrollo. Bogotá D.C, Colombia: Facultad de administración Universidad de los Andes. Corporación Autonoma Regional de la Orinoquia CORPORINOQUIA Available at: https://www.researchgate.net/publication/265077456.
Barbe, L., Morel, R., Rantier, Y., Lebas, J.-F., and Butet, A. (2018). Bird Communities of a Temperate forest: Spatio-Temporal Partitioning between Resident and Migratory Species. J. Ornithol. 159, 457–469. doi:10.1007/s10336-017-1523-y
Batisteli, A., Tanaka, M., and Souza, A. (2018). Bird Functional Traits Respond to forest Structure in Riparian Areas Undergoing Active Restoration. Diversity 10, 90–11. doi:10.3390/d10030090
Biodiversity Indicators Partnership (2011). Guidance for National Biodiversity Indicator Development and Use. Cambridge, UK: UNEP World Conservation Monitoring Centre. UNEP World Conservation Monitoring Centre Available at: www.bipnational.net.
Branquinho, C., Serrano, H. C., Nunes, A., Pinho, P., and Matos, P. (2019). “Chapter 7: Essential Biodiversity Change Indicators for Evaluating the Effects of Anthropocene in Ecosystems at a Global Scale,” in From Assessing to Conserving Biodiversity, Conceptual and Practical Challenges. Editors E. Casseta, J. Marques da Silva, and D. Vecchi (Cham, Switzerland: Springer International Publishing), 137–163.
Bregman, T. P., Sekercioglu, C. H., and Tobias, J. A. (2014). Global Patterns and Predictors of Bird Species Responses to forest Fragmentation: Implications for Ecosystem Function and Conservation. Biol. Conservation 169, 372–383. doi:10.1016/j.biocon.2013.11.024
Buskirk, W. H. (1976). Social Systems in a Tropical forest Avifauna. The Am. Naturalist 110, 293–310. doi:10.1086/283065
Butchart, S. H. M., Walpole, M., Collen, B., van Strien, A., Scharlemann, J. P. W., Almond, R. E. A., et al. (2010). Global Biodiversity: Indicators of Recent Declines. Science 328, 1164–1168. doi:10.1126/science.1187512
Chave, J. (2004). Neutral Theory and Community Ecology. Ecol. Lett. 7, 241–253. doi:10.1111/j.1461-0248.2003.00566.x
Claramunt, S., Derryberry, E. P., Remsen, J. V., and Brumfield, R. T. (2012). High Dispersal Ability Inhibits Speciation in a continental Radiation of Passerine Birds. Proc. R. Soc. B. 279, 1567–1574. doi:10.1098/rspb.2011.1922
C. Lasso, J. S. Usma, F. Trujillo, and A. Rial (2010). Biodiversidad de la cuenca del Orinoco: bases científicas para la identificación de áreas prioritarias para la conservación y uso sostenible de la biodiversidad (Bogotá D. C., Colombia: Instituto de Investigación de Recursos Biológicos Alexander von Humboldt - IAvH, Ministerio del Ambiente, Vivienda y Desarrollo Territorial - MADS, World Wild Fund for Nature - WWF Colombia, Fundación Omacha, Fundación La Salle de Ciencias Naturales e Ins). Available at: https://www.researchgate.net/publication/299505707.
C. Lasso, A. Rial, C. Matallana, W. Ramirez, J. Señaris, A. Diaz-Pulidoet al. (2011). Biodiversidad de la cuenca del Orinoco: II. Áreas prioritarias para la conservación y uso sostenible (Bogotá D. C., Colombia: Instituto de Investigación de Recursos Biológicos Alexander von Humboldt - IAvH, Ministerio del Ambiente, Vivienda y Desarrollo Territorial - MADS, World Wild Fund for Nature - WWF Colombia, Fundación Omacha, Fundación La Salle de Ciencias Naturales e Ins). Available at: https://www.researchgate.net/publication/299560450.
Clavero, M., and Brotons, L. (2010). Functional Homogenization of Bird Communities along Habitat Gradients: Accounting for Niche Multidimensionality. Glob. Ecol. Biogeogr. 19, 684–696. doi:10.1111/j.1466-8238.2010.00544.x
Córdoba Córdoba, S., Borja-Acosta, K. G., and Sierra Buitrago, S. (2018). Colección de Aves del Instituto de Investigación de Recursos Biológicos Alexander von Humboldt (IAvH-A). Inst. Investig. Recur. Biológicos Alexander von Humboldt - IAvH. doi:10.15468/dl.6knqpz
Córdova-Tapia, F., and Zambrano, L. (2015). Functional Diversity in Community Ecology. Ecos 24, 78–87. doi:10.7818/ECOS.2015.24-3.1010.7818/ecos.2015.24-3.10
Cousin, J. A., and Phillips, R. D. (2008). Habitat Complexity Explains Species-specific Occupancy but Not Species Richness in a Western Australian woodland. Aust. J. Zool. 56, 95–102. doi:10.1071/ZO07065
Cutler, D. R., Edwards, T. C., Beard, K. H., Cutler, A., Hess, K. T., Gibson, J., et al. (2007). Random Forests for Classification in Ecology. Ecology 88, 2783–2792. doi:10.1890/07-0539.1
de Baan, L., Alkemade, R., and Koellner, T. (2013). Land Use Impacts on Biodiversity in LCA: a Global Approach. Int. J. Life Cycle Assess. 18, 1216–1230. doi:10.1007/s11367-012-0412-0
De Souza, D. M., Flynn, D. F. B., Declerck, F., Rosenbaum, R. K., De Melo Lisboa, H., and Koellner, T. (2013). Land Use Impacts on Biodiversity in LCA: Proposal of Characterization Factors Based on Functional Diversity. Int. J. Life Cycle Assess. 18, 1231–1242. doi:10.1007/s11367-013-0578-0
Deinet, S., McRae, L., and Freeman, R. (2018). “Chapter 3: Biodiversity in a Changing World,” in Living Planet Report - 2018: Aiming Higher. Editors M. Grooten, and R. E. Almond (Gland, Switzerland: World Wildlife Fund WWF), 89–105. Available at: worldwildlife.org.
Diaz Lopez, D. L., Mora Fernández, C., and Rodríguez Posada, M. E. (2020). Caracterización de Fauna y Flora en ecosistemas de piedemonte, montaña y humedales en el departamento del Casanare, Version 1.4. Fund. Reserv. Nat. La. Palmita - Cent. Investig. Occur. Dataset. doi:10.15472/FVMXMO
Diaz, S., and Cabido, M. (2001). Vive la différence: plant functional diversity matters to ecosystem processes. Trends Ecol. Evol. 16, 646–655. doi:10.1016/S0169-5347(01)02283-210.1016/s0169-5347(01)02181-4
Edwards, D. P., Gilroy, J. J., Thomas, G. H., Medina, C. A., Edwards, D. P., Gilroy, J. J., et al. (2015). Land-Sparing Agriculture Best Protects Avian Phylogenetic Diversity Report Land-Sparing Agriculture Best Protects Avian Phylogenetic Diversity. Curr. Biol. 25, 2384–2391. doi:10.1016/j.cub.2015.07.063
Edwards, F. A., Edwards, D. P., Hamer, K. C., and Davies, R. G. (2013). Impacts of Logging and Conversion of Rainforest to Oil palm on the Functional Diversity of Birds in Sundaland. Ibis 155, 313–326. doi:10.1111/ibi.12027
Eric, A., and Archer, M. E. (2020). rfPermute: Estimate Permutation P-Values for Random Forest Importance Metrics. R Packag. Available at: https://cran.r-project.org/package=rfPermute.
Flather, C. H., and Sauer, J. R. (1996). Using Landscape Ecology to Test Hypotheses about Large-Scale Abundance Patterns in Migratory Birds. Ecology 77, 28–35. doi:10.2307/2265651
Flynn, D. F. B., Gogol-Prokurat, M., Nogeire, T., Molinari, N., Richers, B. T., Lin, B. B., et al. (2009). Loss of Functional Diversity under Land Use Intensification across Multiple Taxa. Ecol. Lett. 12, 22–33. doi:10.1111/j.1461-0248.2008.01255.x
Foley, J. A., DeFries, R., Asner, G. P., Barford, C., Bonan, G., Carpenter, S. R., et al. (2005). Global Consequences of Land Use. Science 309, 570–574. doi:10.1126/science.1111772
Friedman, J. H. (2001). Greedy Function Approximation: A Gradient Boosting Machine. Ann. Statist. 29, 1189–1232. doi:10.1214/aos/1013203451
Genuer, R., Poggi, J.-M., and Tuleau-malot, C. (2010). Variable Selection Using Random Forests. Pattern Recognition Lett. 31, 2225–2236. doi:10.1016/j.patrec.2010.03.014
Gilroy, J. J., Prescott, G. W., Cardenas, J. S., Castañeda, P. G. D. P., Sánchez, A., Rojas-Murcia, L. E., et al. (2015). Minimizing the Biodiversity Impact of Neotropical Oil palm Development. Glob. Change Biol. 21, 1531–1540. doi:10.1111/gcb.12696
Gladstone-Gallagher, R. V., Pilditch, C. A., Stephenson, F., and Thrush, S. F. (2019). Linking Traits across Ecological Scales Determines Functional Resilience. Trends Ecol. Evol. 34, 1080–1091. doi:10.1016/j.tree.2019.07.010
Goldstein, M. I., Corson, M. S., Lacher, T. E., and Grant, W. E. (2003). Managed Forests and Migratory Bird Populations: Evaluating Spatial Configurations through Simulation. Ecol. Model. 162, 155–175. doi:10.1016/S0304-3800(03)00005-X
Hapfelmeier, A., and Ulm, K. (2013). A New Variable Selection Approach Using Random Forests. Comput. Stat. Data Anal. 60, 50–69. doi:10.1016/j.csda.2012.09.020
Hastie, T., Tibshirani, R., and Friedman, J. (2009). “Boosting and Additive Trees,” in The Elements of Statistical Learning Data, Data Mining, Inference, and Prediction. Editors T. Hastie, R. Tibshirani, and J. Friedman (New York, USA: Springer Series in Statistics), 337–387. doi:10.1007/978-0-387-84858-7_10
Henle, K., Davies, K. F., Kleyer, M., Margules, C., and Settele, J. (2004). Predictors of Species Sensitivity to Fragmentation. Biodiversity and Conservation 13, 207–251. doi:10.1023/B:BIOC.0000004319.91643.9e
Hennig, C. (2020). Fpc: Flexible Procedures for Clustering. R Packag. version 2.2-5. Available at: https://cran.r-project.org/package=fpc.
Hennig, C. (2007). Cluster-wise Assessment of Cluster Stability. Comput. Stat. Data Anal. 52, 258–271. doi:10.1016/j.csda.2006.11.025
Herrel, A., Podos, J., Huber, S. K., and Hendry, A. P. (2005). Bite Performance and Morphology in a Population of Darwin's Finches: Implications for the Evolution of Beak Shape. Funct. Ecol. 19, 43–48. doi:10.1111/j.0269-8463.2005.00923.x
Higginson, A. D. (2017). Conflict over Non-partitioned Resources May Explain Between-Species Differences in Declines: the Anthropogenic Competition Hypothesis. Behav. Ecol. Sociobiol. 71, 71–99. doi:10.1007/s00265-017-2327-z
Hilty, S. L., and Brown, W. L. (1986). A Guide to the Birds of Colombia. New Jersey, USA: Princeton University Press.
Hooper, D. U., Solan, M., Symstad, A., Diaz, S., Gessner, M. O., Buchmann, N., et al. (2002). “Species Diversity, Functional Diversity, and Ecosystem Functioning,” in Biodiversity and Ecosystem Functioning: Synthesis and Perspectives. Editors M. Loreau, S. Naeem, and P. Inchausti (Oxford, UK: Oxford University Press), 192–208. Available at: https://www.researchgate.net/publication/27272026.
Hua, F., Yong, D. L., Janra, M. N., Fitri, L. M., Prawiradilaga, D., and Sieving, K. E. (2016). Functional Traits Determine Heterospecific Use of Risk‐related Social Information in forest Birds of Tropical South‐East Asia. Ecol. Evol. 6, 8485–8494. doi:10.1002/ece3.2545
Hurlbert, A. H. (2004). Species-energy Relationships and Habitat Complexity in Bird Communities. Ecol. Lett. 7, 714–720. doi:10.1111/j.1461-0248.2004.00630.x
Hutto, R. L. (1989). The Effect of Habitat Alteration on Migratory Land Birds in a West Mexican Tropical Deciduous forest: A Conservation Perspective. Conservation Biol. 3, 138–148. doi:10.1111/j.1523-1739.1989.tb00066.x
IDEAM (2017). Mapa de ecosistemas continentales, costeros y marinos de Colombia (MEC) [mapa]. Versión 2.1, escala 1:100.000. Inst. Hidrol. Meteorol. y Estud. Ambient. - IDEAM. Inst. Investig. Recur. Biológicos Alexander von Humboldt - IAvH. Inst. Geográfico Agustín Codazzi - IGAC. Minist. Ambient. y Desarro. Sosten. - MADS. Available at: http://www.siac.gov.co/.
IDEAM (2021). Bienvenidos al Sistema de Información para la gestión de datos Hidrológicos y Meteorológicos – DHIME!. Inst. Hidrol. Meteorol. y Estud. Ambient. - IDEAM. Available at: http://dhime.ideam.gov.co/.
Jarzyna, M. A., Quintero, I., and Jetz, W. (2020). Global Functional and Phylogenetic Structure of Avian Assemblages across Elevation and Latitude. Ecol. Lett. 24, 196–207. doi:10.1111/ele.13631
Jullien, M., and Clobert, J. (2000). The Survival Value of Flocking in Neotropical Birds: Reality or Fiction?. Ecology 81, 3416–3430. doi:10.2307/17750410.1890/0012-9658(2000)081[3416:tsvofi]2.0.co;2
Lambin, E. F., Turner, B. L., Geist, H. J., Agbola, S. B., Angelsen, A., Bruce, J. W., et al. (2001). The Causes of Land-Use and Land-Cover Change: Moving beyond the Myths. Glob. Environ. Change 11, 261–269. doi:10.1016/S0959-3780(01)00007-3
Laverde Rodríguez, O. A., Herrera Collazos, E. E., and Rodriguez Morales, M. A. (2018). Colección de aves del Museo de Historia Natural de la Pontificia Universidad Javeriana. Pontif. Univ. Javeriana. doi:10.15472/EOQDL9
Lavorel, S., and Garnier, E. (2002). Predicting Changes in Community Composition and Ecosystem Functioning from Plant Traits: Revisiting the Holy Grail. Funct. Ecol. 16, 545–556. doi:10.1046/J.1365-2435.2002.00664.X
Leck, C. F. (1972). The Impact of Some North American Migrants at Fruiting Trees in Panama. The Auk 89, 842–850. doi:10.2307/4084113
Lederer, R. J. (1975). Bill Size, Food Size, and Jaw Forces of Insectivorous Birds. The Auk 92, 385–387. doi:10.2307/4084573
Liaw, A., and Wiener, M. (2002). Classification and Regression by randomForest. R. News 2, 18–22. Available at: https://www.researchgate.net/publication/228451484.
López Ordoñez, J. P., Stiles, F. G., and Parra Vergara, J. L. (2016). “Protocolo para la medición de rasgos funcionales en aves,” in in La ecología funcional como aproximación al estudio, manejo y conservación de la biodiversidad: protocolos y aplicaciones. Editor B. Salgado-Negret (Bogotá, D. C. Colombia: Instituto de Investigación de Recursos Biológicos Alexander von Humboldt - IAvH), 81–125. doi:10.7818/ECOS.2015.24-3.10
López-Ricaurte, L., Edwards, D. P., Romero-Rodríguez, N., and Gilroy, J. J. (2017). Impacts of Oil palm Expansion on Avian Biodiversity in a Neotropical Natural savanna. Biol. Conservation 213, 225–233. doi:10.1016/j.biocon.2017.07.009
Luck, G. W., Carter, A., and Smallbone, L. (2013). Changes in Bird Functional Diversity across Multiple Land Uses: Interpretations of Functional Redundancy Depend on Functional Group Identity. PLoS One 8, e63671–11. doi:10.1371/journal.pone.0063671
Luck, G. W., Lavorel, S., Mcintyre, S., and Lumb, K. (2012). Improving the Application of Vertebrate Trait-Based Frameworks to the Study of Ecosystem Services. J. Anim. Ecol. 81, 1065–1076. doi:10.1111/j.1365-2656.2012.01974.x
Mainwaring, M. C., Hartley, I. R., Lambrechts, M. M., and Deeming, D. C. (2014). The Design and Function of Birds' Nests. Ecol. Evol. 4, 3909–3928. doi:10.1002/ece3.1054
Martin, T. G., and Possingham, H. P. (2005). Predicting the Impact of Livestock Grazing on Birds Using Foraging Height Data. J. Appl. Ecol. 42, 400–408. doi:10.1111/j.1365-2664.2005.01012.x
Martínez, A. E., Gomez, J. P., Ponciano, J. M., and Robinson, S. K. (2016). Functional Traits, Flocking Propensity, and Perceived Predation Risk in an Amazonian Understory Bird Community. Am. Naturalist 187, 607–619. doi:10.1086/685894
Matuoka, M. A., Benchimol, M., Almeida-Rocha, J. M. d., and Morante-Filho, J. C. (2020). Effects of Anthropogenic Disturbances on Bird Functional Diversity: A Global Meta-Analysis. Ecol. Indicators 116, 106471. doi:10.1016/j.ecolind.2020.106471
Mayfield, M. M., Bonser, S. P., Morgan, J. W., Aubin, I., McNamara, S., and Vesk, P. A. (2010). What Does Species Richness Tell Us about Functional Trait Diversity? Predictions and Evidence for Responses of Species and Functional Trait Diversity to Land-Use Change. Glob. Ecol. Biogeogr. 19, 423–431. doi:10.1111/j.1466-8238.2010.00532.x
Meyfroidt, P., Lambin, E. F., Erb, K.-H., and Hertel, T. W. (2013). Globalization of Land Use: Distant Drivers of Land Change and Geographic Displacement of Land Use. Curr. Opin. Environ. Sustainability 5, 438–444. doi:10.1016/j.cosust.2013.04.003
Millenium Ecosystem Assessment (2005). Ecosystems and Human Well-Being: Biodiversity Synthesis. Washington, DC, USA: World Resources Institute. doi:10.1057/9780230625600
Moermond, T. C., and Denslow, J. S. (1985). Neotropical Avian Frugivores: Patterns of Behavior, Morphology, and Nutrition, with Consequences for Fruit Selection. Ornithological Monogr. 36, 865–897. doi:10.2307/40168322
Morse, D. H. (1977). Feeding Behavior and Predator Avoidance in Heterospecific Groups. Bioscience 27, 332–339. doi:10.2307/1297632
Mouillot, D., Graham, N. A. J., Villéger, S., Mason, N. W. H., and Bellwood, D. R. (2013). A Functional Approach Reveals Community Responses to Disturbances. Trends Ecol. Evol. 28, 167–177. doi:10.1016/j.tree.2012.10.004
Naeem, S., and Wright, J. P. (2003). Disentangling Biodiversity Effects on Ecosystem Functioning: Deriving Solutions to a Seemingly Insurmountable Problem. Ecol. Lett. 6, 567–579. doi:10.1046/j.1461-0248.2003.00471.x
Naranjo, L. G., Amaya, J. D., Eusse González, D., and Cifuentes-Sarmiento, Y. (2012). Guía de las Especies Migratorias de la Biodiversidad en Colombia -Aves, Vol. 1. Bogotá, D. C.: Colombia: Ministerio de Ambiente y Desarrollo Sostenible -MADS, World Wildlife Fundation - WWF.
Newbold, T., Scharlemann, J. P. W., Butchart, S. H. M., Şekercioğlu, Ç. H., Alkemade, R., Booth, H., et al. (2013). Ecological Traits Affect the Response of Tropical forest Bird Species to Land-Use Intensity. Proc. R. Soc. B. 280, 20122131–20122138. doi:10.1098/rspb.2012.2131
Newton, I. (1998). “Social Systems and Status,” in Population Limitation in Birds. Editor I. Newton (San Diego, California, USA: Academic Press), 31–43. doi:10.1016/b978-012517365-0/50002-8
Nooten, S. S., Shultheiss, P., Rowe, R. C., Facey, S. L., and Cook, J. M. (2019). Habitat Complexity Affects Functional Traits and Diversity of Ant Assemblages in Urban green Spaces (Hymeno Ptera: Formicidae). Myrmecological News 29, 67–77. doi:10.25849/myrmecol.news_029:067
Pearson, R. G., Stanton, J. C., Shoemaker, K. T., Aiello-lammens, M. E., Ersts, P. J., Horning, N., et al. (2014). Life History and Spatial Traits Predict Extinction Risk Due to Climate Change. Nat. Clim Change 4, 217–221. doi:10.1038/NCLIMATE2113
Pereira, H. M., Ferrier, S., Walters, M., Geller, G. N., Jongman, R. H. G., Scholes, R. J., et al. (2013). Essential Biodiversity Variables. Science 339, 277–278. doi:10.1126/science.1229931
Powell, G. V. N. (1985). Sociobiology and Adaptive Significance of Interspecific Foraging Flocks in the Neotropics. Ornithological Monogr. 36, 713–732. doi:10.2307/40168313
Prescott, G. W., Gilroy, J. J., Haugaasen, T., Medina Uribe, C. A., Foster, W. A., and Edwards, D. P. (2016). Reducing the Impacts of Neotropical Oil palm Development on Functional Diversity. Biol. Conservation 197, 139–145. doi:10.1016/j.biocon.2016.02.013
Remsen, J. V., Areta, J. I., Bonaccorso, E., Claramunt, S., Jaramillo, A., Pacheco, J. F., et al. (2021). A Classification of the Bird Species of South America. Version [9 Febr. 2021]. American Ornithological Society. Available at: http://www.museum.lsu.edu/∼Remsen/SACCBaseline.htm (Accessed January 20, 2021).
Restall, R. L., Rodner, C., and Lentino, R., M. (2007). Birds of Northern South America : An Identification Guide, Volumen 1. Yale University Press.
Ricotta, C., and Moretti, M. (2010). Assessing the Functional Turnover of Species Assemblages with Tailored Dissimilarity Matrices. Oikos 119, 1089–1098. doi:10.1111/j.1600-0706.2009.18202.x
Ridgely, R. S., Tudor, G., and Brown, W. L. (1989). The Birds of South America: Volume 1: The Oscine Passerines. Austin, TX: University of Texas Press.
Ridgely, R. S., and Tudor, G. (2009). Field Guide to the Songbirds of South America: The Passerines. Austin, TX: University of Texas Press.
Rincon-Parra, V. J. (2021). Dataset of Traits of Some Birds Species of Llanos Piedmont and Flooded Savannas in the Colombian Llanos. Environ. Data Initiat.. doi:10.6073/pasta/3c13d83163859f517882e6376a037953
Roberts, C. P., Twidwell, D., Angeler, D. G., and Allen, C. R. (2019). How Do Ecological Resilience Metrics Relate to Community Stability and Collapse? Ecol. Indicators 107, 105552. doi:10.1016/j.ecolind.2019.105552
Robinson, S. K., and Holmes, R. T. (1982). Foraging Behavior of Forest Birds: The Relationships Among Search Tactics, Diet, and Habitat Structure. Ecology 63, 1918–1931. doi:10.2307/1940130
Rodríguez Posada, M. E., Fernández Rodríguez, R. C., Carantón, D., and Mora Fernandez, C. (2020). Diagnóstico y monitoreo de la diversidad biológica de las sabanas inundables en Casanare. Primera etapa: mamíferos y aves, Version 1.1. Fund. Reserv. Nat. La. Palmita - Cent. Investig. Occur. Dataset. doi:10.15472/S8GB08
Romero Ruíz, M. H., Galindo García, G., Otero García, J., and Armenteras Pascual, D. (2004). Ecosistemas de la cuenca del orinoco colombiano. Bogotá D. C., Colombia: Instituto de Investigación de Recursos Biológicos Alexander von Humboldt - IAvH. Available at: https://www.researchgate.net/publication/236173780.
Romero-Ruiz, M. H., Flantua, S. G. A., Tansey, K., and Berrio, J. C. (2012). Landscape Transformations in Savannas of Northern South America: Land Use/cover Changes since 1987 in the Llanos Orientales of Colombia. Appl. Geogr. 32, 766–776. doi:10.1016/j.apgeog.2011.08.010
Root, R. B. (1967). The Niche Exploitation Pattern of the Blue‐Gray Gnatcatcher. Ecol. Monogr. 37, 317–350. doi:10.2307/1942327
Sitters, H., York, A., Swan, M., Christie, F., and Di StefanoDi, J. (2016). Opposing Responses of Bird Functional Diversity to Vegetation Structural Diversity in Wet and Dry forest. PLoS One 11, e0164917–18. doi:10.1371/journal.pone.0164917
Birds of the World (2021). in Cornell Laboratory of Ornithology. Editors S. M. Billerman, B. K. Keeney, P. G. Rodewald, and T. S. Schulenberg Ithaca (NY, USA. Available at: https://birdsoftheworld.org/bow/home (Accessed October 1, 2020).
Smith, A. L., Ortiz, J. S., and Robertson, R. J. (2001). Distribution Patterns of Migrant and Resident Birds in Successional Forests of the Yucatan Peninsula, Mexico1. Biotropica 33, 153–170. doi:10.1111/j.1744-7429.2001.tb00165.x
Speiser, J. L., Miller, M. E., Tooze, J., and Ip, E. (2019). A Comparison of Random forest Variable Selection Methods for Classification Prediction Modeling. Expert Syst. Appl. 134, 93–101. doi:10.1016/j.eswa.2019.05.028
Sridhar, H., Beauchamp, G., and Shanker, K. (2009). Why Do Birds Participate in Mixed-Species Foraging Flocks? A Large-Scale Synthesis. Anim. Behav. 78, 337–347. doi:10.1016/j.anbehav.2009.05.008
Stein, A., Gerstner, K., and Kreft, H. (2014). Environmental Heterogeneity as a Universal Driver of Species Richness across Taxa, Biomes and Spatial Scales. Ecol. Lett. 17, 866–880. doi:10.1111/ele.12277
Stiles, F., Raz, L., and Agudelo, H. (2017). Colección Ornitológica del Instituto de Ciencias Naturales (ICN-MHN-Or). Inst. Ciencias Nat. Univ. Nac. Colomb. doi:10.15472/DI7ZED
Sun, Y., Wong, A. K. C., and Kamel, M. S. (2009). Classification of Imbalanced Data: A Review. Int. J. Patt. Recogn. Artif. Intell. 23, 687–719. doi:10.1142/S0218001409007326
Swaddle, J. P., and Lockwood, R. (1998). Morphological Adaptations to Predation Risk in Passerines. J. Avian Biol. 29, 172–176. doi:10.2307/3677195
Tamaris-Turizo, D. P., López-arévalo, H. F., and Romero Rodríguez, N. (2017). Efecto de la estructura del cultivo de palma de aceite Elaeis guineensis (Arecaceae) sobre la diversidad de aves en un paisaje de la Orinoquía colombiana. Rev. Biol. Trop. 65, 1569–1581. doi:10.15517/RBT.V65I4.26735
Tellería, J. L., Hera, I. D. L., and Pérez-Tris, J. (2013). Morphological Variation as a Tool for Monitoring Bird Populations: A Review. Ardeola 60, 191–224. doi:10.13157/arla.60.2.2013.191
Tews, J., Brose, U., Grimm, V., Tielbörger, K., Wichmann, M. C., Schwager, M., et al. (2004). Animal Species Diversity Driven by Habitat Heterogeneity/diversity: The Importance of keystone Structures. J. Biogeogr. 31, 79–92. doi:10.1046/j.0305-0270.2003.00994.x
Thiollay, J.-M., and Jullien, M. (1998). Flocking Behaviour of Foraging Birds in a Neotropical Rain forest and the Antipredator Defence Hypothesis. Ibis (Lond. 1859) 140, 382–394. doi:10.1111/j.1474-919x.1998.tb04599.x
Thomas, A. (1993). On the Aerodynamics of Birds' Tails. Phil. Trans. R. Soc. Lond. B 340, 361–380. doi:10.1098/rstb.1993.0079
Tillé, A. Y., and Matei, A. (2016). Sampling: Survey Sampling. R Packag. version 2.8. Available at: https://cran.r-project.org/package=sampling.
Titeux, N., Henle, K., Mihoub, J.-B., Regos, A., Geijzendorffer, I. R., Cramer, W., et al. (2016). Biodiversity Scenarios Neglect Future Land-Use Changes. Glob. Change Biol. 22, 2505–2515. doi:10.1111/gcb.13272
Trujillo, W. F., and Henao, M. M. (2018). Riqueza florística y recambio de especies en la vertiente orinoquense de los Andes, Colombia. Colomb. For. 21, 18–33. doi:10.14483/2256201X.11848
Tuanmu, M.-N., and Jetz, W. (2015). A Global, Remote Sensing-Based Characterization of Terrestrial Habitat Heterogeneity for Biodiversity and Ecosystem Modelling. Glob. Ecol. Biogeogr. 24, 1329–1339. doi:10.1111/geb.12365
UPRA (2021). “Sistema Para la Planificación Rural Agropecuaria - SIPRA,” in Unidad Planif. Rural Agropecu. - UPRA. Available at: https://sipra.upra.gov.co/#nacional.
Villéger, S., Brosse, S., Mouchet, M., Mouillot, D., and Vanni, M. J. (2017). Functional Ecology of Fish: Current Approaches and Future Challenges. Aquat. Sci. 79, 783–801. doi:10.1007/s00027-017-0546-z
Wezel, A., Casagrande, M., Celette, F., Vian, J.-F., Ferrer, A., and Peigné, J. (2014). Agroecological Practices for Sustainable Agriculture. A Review. Agron. Sustain. Dev. 34, 1–20. doi:10.1007/s13593-013-0180-7
Wiescher, P. T., Pearce-Duvet, J. M. C., and Feener, D. H. (2012). Assembling an Ant Community: Species Functional Traits Reflect Environmental Filtering. Oecologia 169, 1063–1074. doi:10.1007/s00442-012-2262-7
Willson, M. F. (1971). Seed Size Preference in Finches. Wilson Bull. 84, 449–455. Available at: https://www.jstor.org/stable/4160257.
Keywords: functional diversity, functional traits, trait attributes, machine learning classification, Orinoquia
Citation: Rincon-Parra VJ, Echeverry-Galvis MA and Alvarez SJ (2022) Functional Responses of Bird Assemblages to Land-Use Change in the Colombian Llanos Region. Front. Environ. Sci. 9:689745. doi: 10.3389/fenvs.2021.689745
Received: 01 April 2021; Accepted: 10 August 2021;
Published: 20 January 2022.
Edited by:
Carlos Hernando Montenegro, University of Los Andes, ColombiaReviewed by:
Orlando Acevedo-Charry, Alexander von Humboldt Biological Resources Research Institute, ColombiaChong Leong Puan, Putra Malaysia University, Malaysia
Copyright © 2022 Rincon-Parra, Echeverry-Galvis and Alvarez. This is an open-access article distributed under the terms of the Creative Commons Attribution License (CC BY). The use, distribution or reproduction in other forums is permitted, provided the original author(s) and the copyright owner(s) are credited and that the original publication in this journal is cited, in accordance with accepted academic practice. No use, distribution or reproduction is permitted which does not comply with these terms.
*Correspondence: Victor Julio Rincon-Parra, cmluY29uLXZAamF2ZXJpYW5hLmVkdS5jbw==