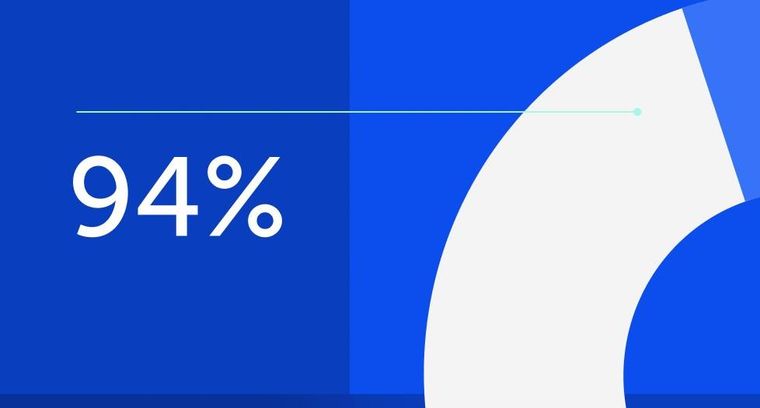
94% of researchers rate our articles as excellent or good
Learn more about the work of our research integrity team to safeguard the quality of each article we publish.
Find out more
ORIGINAL RESEARCH article
Front. Environ. Sci., 25 May 2021
Sec. Freshwater Science
Volume 9 - 2021 | https://doi.org/10.3389/fenvs.2021.686334
Recent studies suggest that water hyacinths can influence the transport of macroplastics in freshwater ecosystems at tropical latitudes. Forming large patches of several meters at the water surface, water hyacinths can entrain and aggregate large amounts of floating debris, including plastic items. Research on this topic is still novel and few studies have quantified the role of the water hyacinths in plastic transport. In this study, we present the findings of a six-week monitoring campaign, combining the use of visual observations and Unmanned Aerial Vehicle imagery in the Saigon river, Vietnam. For the first time, we provide observational evidence that the majority of macroplastic is transported by water hyacinth patches. Over the study period, these fast-growing and free-floating water plants transported 78% of the macroplastics observed. Additionally, we present insights on the spatial distribution of plastic and hyacinths across the river width, and the different characteristics of entrapped items compared with free-floating ones. With this study, we demonstrate the role of water hyacinths as a river plastic aggregator, which is crucial for improving the understanding of plastic transport, and optimizing future monitoring and collection strategies.
Rapid growth in plastic production and consumption has made plastic pollution an ubiquitous issue across the globe (Lebreton and Andrady, 2019). Plastics can directly harm aquatic and terrestrial species, and cause serious economic damage (van Emmerik and Schwarz, 2020). Alarmingly, studies predict major increases in plastic emissions due to low recycling rates, global increases in plastic consumption, and difficulties in large scale plastic reduction efforts (Borrelle et al., 2020; Lau et al., 2020). Plastic pollution monitoring efforts are being conducted at various scales, but these initiatives focus mostly on marine and coastal areas, rather than riverine ecosystems (Blettler et al., 2018). Despite rivers assumed to account for the majority of the plastic emitted into oceans (Lebreton and Andrady, 2019), many aspects on plastic transport and fates in river systems remain unknown. Yet, quantifying riverine plastic loads is essential for assessing the efficiency of plastic reduction measures such as reduction of plastic consumption and improving of waste disposal. In addition, quantitative data about plastic in rivers is crucial to better understand the exposure of riverine ecosystems to plastic waste.
Understanding of the role of various factors in plastic transport variability in rivers is expanding, and studies are exploring different drivers for different sizes of plastic debris. It is often assumed that hydrometeorological variables are important drivers of plastic flux (Meijer et al., 2021). River discharge was related to plastic transport in the Seine, France (van Emmerik et al., 2019b) and changes in dry/wet seasons correspond to significant plastic debris fluctuations in the Wonorejo river, Indonesia (Kurniawan and Imron, 2019). In the United Kingdom, flood events were found to be responsible for microplastic export (Hurley et al., 2018). Other drivers, such as sediment loads have also been recently examined in Australia (He et al., 2020), China (Liu et al., 2021) and Amazonian rivers (Gerolin et al., 2020) in relation to microplastic transport. Mangrove forests and coastal vegetation habitats can also be an important sink of plastic debris (Ivar do Sul et al., 2014; Cozzolino et al., 2020; Martin et al., 2020). In the Saigon river, Vietnam, macroplastic (>5 mm) transport was correlated to the abundance of floating vegetation material found (mainly drifting water hyacinths), rather than hydrological variables (van Emmerik et al., 2019a). The monthly estimates of plastic and water hyacinths abundance were both found to vary with an order of magnitude, suggesting that drifting hyacinths may act as the main carrier for floating macroplastic. For better understanding of plastic transport dynamics in the (sub) tropics, exploring the role of water hyacinths is thus crucial.
Water hyacinth (Eichhornia crassipes) is a macrophyte species native from the Amazon, which has spread to most freshwater ecosystems in the tropics and subtropics (Thamaga and Dube, 2018). Water hyacinths are aquatic weeds that tend to aggregate and form large patches floating at the water surface. They are considered one of the most invasive vegetation species in the world, and have already spread to 15 Asian and 36 African countries (IUCN, 2017; CABI, 2020). More recently, these floating water plants have been observed in North America and Southern Europe (CABI, 2020; EDDMapS, 2020). Despite water hyacinths being considered a nuisance, their absorbent capacities offer an opportunity to use them as ecological indicators, and their role in the absorption of pollutants such as heavy metals has been well ascertained (Sharma et al., 2016). Better understanding the relation between hyacinths and to plastic pollution is crucial for three main reasons. We hypothesize that hyacinths substantially influence spatiotemporal variation in riverine plastic transport as they drift downstream carrying floating macroplastic. Thus understanding this relationship is key for improved plastic transport quantification. Secondly, the detectability of large floating vegetation aggregations from satellite (Dogliotti et al., 2018; Ongore et al., 2018; Thamaga and Dube, 2018; Winton et al., 2020; Kleinschroth et al., 2021) is an opportunity to use hyacinth patches as a proxy for plastic pollution. This could be an important step in scaling-up plastic monitoring efforts. Lastly, if the role of hyacinths in aggregating plastic is ascertained, clean-up efforts could utilize the plant as a means for efficient plastic co-removal in inland waters.
In this paper, we provide a first assessment of the role of drifting water hyacinths in riverine macroplastic transport. We present findings of a six-week measurement campaign that combined visual observations and Unmanned Aerial Vehicle (UAV) surveys. We monitored the contribution of plastic entangled in hyacinths relative to the total plastic transport, the accumulation of plastics in hyacinths, and composition of plastics within and outside hyacinths. With this paper we aim to: 1) demonstrate the substantial role of water hyacinths in plastic transport, and 2) provide insights on the characteristics (polymer composition and size distribution) of plastics that are transported by hyacinths.
We focused on the Saigon river, considered one the most plastic polluted watershed in Vietnam, the 8th highest contributing country in terms of river plastic emissions worldwide (Meijer et al., 2021). The Saigon river is part of the Saigon–Dong Nai river system that traverses Ho Chi Minh City (HCMC). The Saigon river is controlled upstream by a reservoir and is subjected to diurnal asymmetric tides. Its net annual mean water discharge was estimated of 50 m3 s−1, fluctuated monthly from 4 to 222 m3 s−1 (Nguyen et al., 2020). Plastic pollution in the Saigon river has already been monitored by several studies, thus enabling investigation of transport dynamics (van Emmerik et al., 2018; van Emmerik et al., 2019a).
We used two complementary measurement methods to quantify both the role of water hyacinths in plastic accumulation and in transport (Table 1). Visual observations enabled to estimate macroplastic transport flux at the water surface for 15 individual days. The Unmanned Aerial Vehicle (UAV) survey, on the other hand, allowed finer characterization on the plastic entangled in water hyacinths, such as spatial distribution along the river width, plastic categories, item size and plastic density within vegetation patches. Visual counting can be used for categorizing plastic as well, but becomes challenging in a context of high plastic flux (van Emmerik et al., 2018). The UAV survey uses photos and can be used to determine the plastic concentration at the water surface (Geraeds et al., 2019).
TABLE 1. Overview of methods used to characterize macroplastic transport and storage in water hyacinths for the Saigon river.
The visual measurement campaign took place between the 27 April and the 8 June 2020 (n = 15). The plastic items counting protocol was adapted from the methodology developed by van Emmerik et al. (van Emmerik et al., 2018). The counting was done from the Thu Thiem bridge in HCMC (10°47′08.3″N, 106°43′06.2″E), see Figure 1. The UAV images were taken on the May 23, 2020 only. A total of ten UAV flights were conducted on that day, with the four initial flights 100 m downstream the bridge, and the six subsequent surveys 80 m upstream. The UAV survey method that we present is similar to the method tested by Geraeds et al. (Geraeds et al., 2019), with the alteration of splitting the cross-sectional flights to two locations, due to tidal influences.
FIGURE 1. Localization of visual observation points and 22 Unmanned aerial vehicle survey waypoints. Right and left river banks refer to the hand sides when facing downstream. Source: Bing imagery.
The visual counting method was adapted from (González-Fernández and Hanke. (González-Fernández and Hanke, 2017). It is a well-established and simple observation procedure, easily replicable at various locations. The visual observations were conducted from three different locations along the Thu Thiem bridge over a period of 6 weeks. It is estimated that at each location, the surveyor was able to detect all floating plastic items along a 15 m width section. Overall, 13% (45 m out of 340 m) of the river cross-section was covered by the visual counting. The bridge is approximately 14 m above the water level and the counting was done facing downstream. From this height, the average minimum item size was estimated to be 1 cm.
One experienced surveyor conducted the visual counting at the bridge. During a time frame of two minutes and at one bridge location, the surveyor counted all plastic items passing below the bridge and entrapped in drifting hyacinth patches (entangled macroplastic flux). This observation sequence was followed by another 2-min time frame to count plastic debris flowing downstream and not carried by hyacinth patches (free-floating macroplastic flux). The visual contrast between floating items with both water and the drifting hyacinth patches was considered sufficient for accurate identification of surface macroplastics. When the nature of the floating debris was uncertain, it was not counted as a plastic item. After the visual counting at one observation point, the surveyor proceeded to the next location. For each measurement day, several cross-sectional profiles of the plastic flux were made. In total, 256 two-minute observations of were conducted over the 15 individual days. Subsequent data analysis included extrapolation of plastic flux for the whole river width and scaling to obtain hourly values (Supplementary Text S1).
A DJI Phantom 4 Pro (DJI, Shenzhen, China; http://www.dji.com) Unmanned Aerial Vehicle (UAV) was used to acquire aerial imagery upstream and downstream of the Thu Thiem bridge on May 23, 2020. A total of 3,936 images were taken at 22 locations (henceforth referred to as “waypoints”) across the river width. We defined 22 waypoints every 15 m. Overall, the flights covered the full width of the river. Blurry images were discarded (n = 261) and ultimately, only images with visible plastic items were retained for analysis (n = 128). The low number of images analyzed is due to the removal of overlapping images at the same waypoint. When images taken at a waypoint showed differences in the number of plastic items, they were both analyzed, making sure that items present on both images were not counted double. The UAV survey was conducted at two altitudes. The scans along the river transepts were scheduled at 5 m from the chart datum (derived from the lowest astronomical tide). A few images (n = 11) were captured at 15 m of altitude above the chart datum, usually in the middle section of the river. These images were retained for items count analysis and discarded for statistics on spatial distribution as they were not taken at the same river transepts than the rest of the UAV images. More details on UAV data acquisition are found in Supplementary Information (Supplementary Text S2).
The selected images were manually labelled with the open source Visual Geometry Group Image Annotator (VIA) tool (Dutta and Zisserman, 2019). Two categories of plastic were distinguished: “free-floating” items – plastic debris flowing freely at the river surface – and “entangled” items – plastic waste trapped within hyacinth. Rectangular polygons were drawn around each identified plastic item for size and area estimates (Supplementary Figure S1). Information on the plastic categories was also filled in during the manual labelling. Seven plastic types were distinguished, following the categorization used in van Emmerik, Strady, et al. (van Emmerik et al., 2019a): 1) E-PS (expanded polystyrene), 2) PO hard (hard polyolefins), 3) PO soft (soft polyolefins), 4) PS (polystyrene), 5) PET (polyethylene terephthalate), 6) Multilayer plastics, and 7) Rest plastics. The items were categorized based on color, shape and other visual properties such as transparency (Supplementary Table S1). When the plastic category of the item was uncertain, it was categorized as part of the category “Rest”.
To estimate the aquatic vegetation coverage area, color filtering was performed using the Open CV library in Python over a selection of images (n = 75) (Supplementary Text S3; Supplementary Figure S2). Ultimately, the ground sampling distance was determined, based on the flying elevation, the image width in pixels, sensor width and focal length of the camera (Supplementary Text S3). This allowed us to estimate both vegetation and plastic item areas. It should be noted that for the entire image collection, the average spatial resolution on UAV images was estimated at 0.175 cm/pixel. This allowed to spot debris as small as 5 mm of size.
For both the visual counting and the UAV survey, only floating items that were undoubtfully of plastic composition were included in the study. Both the aerial images and the bridge measurements provided satisfactory visibility to detect items either entangled in water hyacinths or free-floating. Only macroplastic items entrained in hyacinth patches were considered as entangled items, whereas floating items in contact with other debris (such as leaves, branches or coconuts) were counted as free-floating macroplastics.
Estimated mean plastic flows show large temporal variability during the visual counting period. Mean daily plastic flux calculated from visual observations varied between 15 and 1,080 items/minute (Figure 2A), with the lowest mean plastic flux on 11 May and peak mean plastic flux on 27 April. No steady and consistent temporal trend is noticeable over the 6-week period: days with low plastic flux can be followed by increases in plastic flux and then register a significant drop at the next measurement day. Overall, the mean plastic flux over the study period was estimated at 170 items/min for entangled items and 48 items/min for free-floating debris. The total mean macroplastic flux was thus approximately of 218 items/min.
FIGURE 2. (A) Mean plastic flux and entanglement ratios per measurement day as measured from visual observations. (B) Entangled and free-floating plastic flux in relation to total plastic flux. Each data point represents one visual counting observation.
The entrapped fraction of transported macroplastic varies greatly, from 15 to 93% depending on the measurement day (Figure 2A), with days registering low ratios broadly corresponding to low plastic daily flux (6 May, 11 May, 22 May, 27 May, and 8 June). During the 6-week period of visual counting, water hyacinths carried an average of 78% of the observed plastic items. In addition, we found that plastic transported by hyacinths shows a variability in mean flux of three orders of magnitude (2–1,002 items/min). This is considerably higher than the variability in free-floating flux, of one order of magnitude (12–160 items/min). These results demonstrate that the transport of floating macroplastic entrapped in hyacinths has a substantial influence on the total floating macroplastic transported along the river.
We found a highly positive correlation between entangled macroplastics and total macroplastic items (Figure 2B), the latter being likely mainly driven by the amount of hyacinths present. Data on the transport of free-floating items, on the other hand, show a lower positive correlation (r = 0.48, p < 0.01) with total plastic flux. These results suggest that total floating macroplastic transport in the Saigon river flanking Ho Chi Minh City is heavily impacted by macroplastics accumulated in hyacinths.
For the month of May specifically, we estimate that the floating macroplastic flux averaged 162 items/min. Interestingly, this estimation is close to the mean flux values (117–133 items/min) found by van Emmerik, Strady, et al. (van Emmerik et al., 2019a) for May 2018 at the same location. The slightly higher flux that we measured in 2,020 might be attributable to variability in environmental drivers (for example, rainfall for water flow, temperature for hyacinth growth) or increases in plastic consumption, mismanagement and leakage between observed years.
Overall, we found large temporal variations in the ratio of entangled macroplastic and in entangled plastic fluxes. Our measurement campaign lasted six weeks and took place between the end of April and the beginning of June, a season which marks the transition from the high plastic loads of the dry season to the relatively low plastic flux characteristics of the wet months (van Emmerik et al., 2019a). Further quantification of the macroplastic flux transported in water hyacinths at different seasons is needed. Longer field measurement campaigns could provide more accurate estimates of the average flux transported by hyacinth, which could then be used as a proxy indicator for estimating plastic transport and emissions.
The spatial distribution of plastic debris and vegetation captured by the UAV survey on 23 May shows large heterogeneity in hyacinth and plastic concentrations along the river width, with clear accumulation zones. For the UAV flights done downstream of the bridge, debris and vegetation patches accumulated in largest amounts close to the right river bank at waypoint 1. Approximately 57% of detected hyacinth patches, 42% of macroplastic items entangled in hyacinth, and 31% free-floating items were observed along the first 35 m from the right river bank (Figure 3A). Vegetation and plastic concentration zones were also observable at 80 m (waypoint 6) from the north river bank, and waypoint 16 at 230 m (closer to the left river bank). For the section upstream of the bridge, concentration near the right river bank was even higher (Figure 3A). Most plastic items accumulated in the first 35 m from the north river bank (87% for entangled items and 65% for free-floating ones), with just a few items counted at 230 and 275 m. The hyacinths were all concentrated at the right bank for the upstream surveys. A likely explanation is that one UAV flight detected a higher number of macroplastic items (n = 193, flight n.7) compared to the average number of detected items (n = 40) for all other flights. The items detected during the 7th flight were primarily located close to the right river bank, thus influencing the overall data for upstream surveys.
FIGURE 3. (A) Distribution of vegetation and macroplastics along the river width [%] upstream and downstream the bridge. (B) Correlation matrix of measured metrics. The Pearson correlation coefficient was used. Red shows highest correlation scores between variables, while blue shows lower scores. All p-values are <0.01 so all correlation scores show significant relationships between variables (C) Plastic accumulation within hyacinth patches: number of plastic items per m2 of vegetation in relation to the estimated vegetation area in m2.
Our results show that macroplastic and hyacinth patches accumulate at the same sections across the river width. Wind speed, direction and flow velocity could be governing factors in the patterns observed, but have yet to be studied. The heterogeneity in the spatial distribution of plastic debris is in accordance with previous studies in riverine environments (van Emmerik et al., 2019a; Geraeds et al., 2019). In addition, it remains unclear if the observed accumulation zones on the right bank is the result of the absence of hyacinths in the mid-channel sections (given that this section of the river is heavily navigated) or if right bank sections are natural accumulation zones due to the combined influence of flow velocity, wind characteristics, river shape and the presence of hyacinths. Understanding what determines the spatial distribution of the water hyacinths could provide new insights on macroplastic accumulation zones and thus inform plastic removal measures. Additionally, a better understanding of the drifting dynamics of hyacinth patches in rivers is also needed. For the monitoring period studied, hyacinths functioned mainly as floating macroplastic carriers, as they drifted downstream. However, hyacinth patches could also accumulate in slowly moving water, for instance close to the riverbanks. In this configuration, this aquatic weed would act more as a barrier for dispersion than a means for macroplastic transport.
For the entire river width, the UAV survey found that 39% (n = 313) of floating macroplastic were accumulated in hyacinth on the 23rd of May. Considering only items of a size >2.5 cm, the entrapment rate rose to 44%. Note that this “snapshot” entanglement ratio cannot be compared directly with the ratio of entangled macroplastic flux measured over 6 weeks. Nonetheless, the UAV results on spatial distribution are consistent with the visual counting results on transport temporal variability: lower total transport flux on 22 and 27 May plus higher free-floating plastic transport compared with hyacinth-entangled flux.
Overall, the data (Figure 3B) shows that the spatial macroplastic distribution is strongly correlated with the hyacinth presence (Pearson’s r = 0.89 for the total macroplastic count and r = 0.59 for macroplastic area). Furthermore, the correlations between macroplastic and water hyacinth abundance are higher when considering only entrapped items (Pearson’s r = 0.94 for items count and r = 0.65 for area).
The accumulation of floating macroplastic in vegetation patches varies considerably, from 0 to 98 items per m2 (Figure 3C). On average, the accumulation density is eight items per m2 of vegetation. Interestingly, the data shows that largest vegetation patches have lowest plastic accumulation densities. Visual examination of the aerial imagery showed that plastic litter is mostly entrapped at the edges of the patches, with a sparser presence of items in the central area. This could indicate that free-floating items interact with the edges of the vegetation patches during their transport. Long term monitoring efforts should shed light on the spatial interactions between hyacinths and plastics and the entrapment mechanisms.
The plastic debris we detected in UAV imagery consisted mainly of E-PS (38%), in similar proportions compared to previous studies conducted in the Saigon river (van Emmerik et al., 2019a). The predominance of E-PS is the result of both the extensive use of single-use food containers in Vietnam (Lahens et al., 2018) as well as the high floatability of this low-density polymer type. Foamed items were predominantly below 1 cm in size (Figure 4B), suggesting a higher level of fragmentation that ultimately contributes to a large share of E-PS items. In addition, E-PS made up for 32% of free-floating items and almost 49% of entangled items (Figure 4A). Its low density might explain how and why it becomes entrained in hyacinth patches. Hyacinth appears to entangle PET items at higher rates compared with the proportion found in open water, possibly because the items we observed in the UAV imagery were of a larger size (>10 cm). Overall, PET was the least abundant (2%) plastic category found among floating macroplastic, a likely effect of re-use or recycling mechanisms targeting this category of plastic debris. This is in agreement with other studies that observed lower quantities of PET items in aquatic environments than in the production composition (Schwarz et al., 2019; van Calcar and van Emmerik, 2019). The share of items classified as “Rest” is higher (30%) within the hyacinth patches than outside (21%). Poor identification of entangled plastic materials were likely caused by the partial coverage of plastic debris by leaves and roots. PS, PO soft and Multilayer items were more frequently observed as free-floating debris, (respectively, 16, 16, and 6%) than entangled (3, 8, and 1%), indicating that bags and foil items are not easily entrained/entangled. Only PO hard particles were found in similar proportions in both entangled and free-floating items, (respectively, 8 and 9%). This is a considerably lower estimate than found in previous studies, where PO hard accounted for approximately 20% of detected items (van Emmerik et al., 2019a). Possible explanations for this could be observer bias from the manual labelling, or a relative scarcity of PO hard items during the measurement day (23 May). Given that plastic composition is subject to temporal variability (van Emmerik et al., 2019a), higher temporal coverage and frequency on the plastic composition of entangled plastic items is needed to inform plastic reduction strategies.
FIGURE 4. Plastic composition of observed items (A), in relation to size categories (B) and overall size distribution (C).
Overall, larger plastic particles were more frequently found entrapped in hyacinths than otherwise observed among free-floating items (Figure 4C). Nearly 50% of plastic debris within vegetation patches exceeded 5 cm in size, whereas 67% of free-floating debris were smaller than 5 cm. There are two possible explanations for this. The first assumes that the plastic items have been flowing freely for some distance. During their transportation, the large items get trapped in the vegetation patches, due to contact and interference. On the contrary, the small debris items appear to be more mobile on the water surface, and perhaps more influenced by flow. The second explanation considers that most of the plastic litter is leaked into the river system via the vegetation patches. Indeed, hyacinths are often located close to the riverbanks, where waste may be more conveniently dumped. Some items then fragment into smaller particles, disentangle from the hyacinths, and enter the open water. A coupling of these accumulation-transport patterns is not to be excluded.
In this study, we observed important temporal variations in plastic fluxes of several orders of magnitude, highlighting the need for long-term continuous plastic monitoring. The importance of seasonal variability in macroplastic transport in the Saigon river, as already shown by van Emmerik, Strady, et al. (van Emmerik et al., 2019a), prompts for investigations into the seasonality of water hyacinths and its role in plastic flux variability. We recommend that further research efforts focus on large-scale detection of floating vegetation, using Earth Observation (EO) satellites. Research shows that floating patches of hyacinth as well as plastics are detectable from space (Dogliotti et al., 2018; Biermann et al., 2020; Winton et al., 2020; Kleinschroth et al., 2021). The disintegration and growth cycles of hyacinths could also explain sudden peaks in plastic emitted into the oceans, but have yet to be studied.
Monitoring efforts may also be expanded to other locations along the Saigon river to better understand plastic transport-sink patterns. Our study focused on one location, but the role of hyacinth may differ in upstream and downstream river segments. Furthermore, it is unclear if the plastic debris is introduced at HCMC or further upstream. Better understanding of the plastic input sources is required for a comprehensive monitoring scheme. Investigating other river systems is also crucial to ascertain whether the dominant role of floating vegetation in plastic transport is specific to the Saigon river or not. Given the important overlap between rivers that have been invaded by hyacinth and highly plastic polluted waterways, we hypothesize that hyacinth may act as a plastic carriers in many other watersheds.
We showed that hyacinths aggregate and carry the majority of floating macroplastic transported downstream. However, it is possible that in certain configurations–for instance large floating patches close to the riverbanks, with low flow velocity–the hyacinths act more as a barrier than a means of transport. Recent studies prove the role of mangrove forests in trapping anthropogenic litter in estuarine (Ivar do Sul et al., 2014) and coastal environments (Martin et al., 2019; Martin et al., 2020). Focus on the residence time, and spatial interactions between hyacinths and plastic and degradation rates could be beneficial in that sense. The routes of macroplastic and transport-storage-remobilization patterns related to hyacinth dynamics should certainly be investigated at different scales (Liro et al., 2020). Again, EO may prove to be a crucial complementary approach for understanding the river system at synoptics scales. Additional field measurements, such as physical sampling, on the other hand, would be necessary for finer characterization of the fate of plastics once entangled in hyacinths.
This study provides the first observational evidence that water hyacinths act as macroplastic carriers in rivers. Water hyacinths play a substantial role in macroplastic transport in the Saigon river, as 78% of the observed items were found to be transported by hyacinth patches. In addition, the high positive correlation (Pearson’s r = 0.98) between entangled macroplastic and total macroplastic flux suggests that hyacinths have a considerable impact on the spatiotemporal variation of floating macroplastic transport. The item composition between entangled and free-floating macroplastic differs, with larger items and mainly E-PS plastics predominantly associated with hyacinth patches, and foils and soft plastics more frequently observed as free-floating items. We observed high temporal variability in fluxes of macroplastic carried by hyacinths (of three orders of magnitude–from 1 100 to 1 103 items/min) and in the share of plastic entangled in hyacinths (between 15 and 93%), highlighting the need for long-term field measurement campaigns. More accurate estimates of the average flux transported by hyacinth patches could then be used as a proxy indicator for estimating plastic transport and emissions. Moreover, additional research is required on the scalability and transferability of our findings to other locations along the Saigon, and other river systems worldwide.
With this paper, we demonstrate that floating vegetation function as a major carrier for floating macroplastic in the Saigon river. The combined use of visual observations and UAV imagery allows for the assessment of contribution of vegetation to plastic transport and accumulation. Furthermore, our insights into the role of hyacinths as plastic aggregators and carriers in the Saigon river could inform clean-up strategies at the local level.
The original contributions presented in the study are included in the article/Supplementary Material, further inquiries can be directed to the corresponding author.
TvE, LS, ES, and T-CK-L: design and conceptualization of the study. TLN, N-AP, and T-CK-L: data collection. LS and ES: data analysis. LS: writing. MvP, TvE, ES, LB, and T-CK-L: editing and reviewing.
The work of LS was supported by NWO Open Mind grant 18127. The work of TvE and MvP was supported by the 4TU.Federation Plantenna project. TvE is supported by the Veni research program The River Plastic Monitoring Project with project number 18211, which is (partly) funded by the Dutch Research Council (NWO). This study is part of the Plastic Plants project, supported by the Discovery Element of the European Space Agency’s Basic Activities (ESA contract no. 4000132682/20/NL/GLC).
The authors declare that the research was conducted in the absence of any commercial or financial relationships that could be construed as a potential conflict of interest.
We thank the two reviewers for their constructive feedback which helped improving the paper considerably.
The Supplementary Material for this article can be found online at: https://www.frontiersin.org/articles/10.3389/fenvs.2021.686334/full#supplementary-material
Biermann, L., Clewley, D., Martinez-Vicente, V., and Topouzelis, K. (2020). Finding Plastic Patches in Coastal Waters Using Optical Satellite Data. Sci. Rep. 10 (1), 5364. doi:10.1038/s41598-020-62298-z
Blettler, M. C. M., Abrial, E., Khan, F. R., Sivri, N., and Espinola, L. A. (2018). Freshwater Plastic Pollution: Recognizing Research Biases and Identifying Knowledge Gaps. Water Res. 143, 416–424. doi:10.1016/j.watres.2018.06.015
Borrelle, S. B., Ringma, J., Law, K. L., Monnahan, C. C., Lebreton, L., McGivern, A., et al. (2020). Predicted Growth in Plastic Waste Exceeds Efforts to Mitigate Plastic Pollution. Science 369 (6510), 1515–1518. doi:10.1126/science.aba3656
CABI (2020). “Eichhornia crassipes (Water Hyacinth),” in Invasive Species Compendium (Wallingford, UK: CAB International).
Cozzolino, L., Nicastro, K. R., Zardi, G. I., and de los Santos, C. B. (2020). Species-specific Plastic Accumulation in the Sediment and Canopy of Coastal Vegetated Habitats. Sci. Total Environ. 723, 138018. doi:10.1016/j.scitotenv.2020.138018
Dogliotti, A., Gossn, J., Vanhellemont, Q., and Ruddick, K. (2018). Detecting and Quantifying a Massive Invasion of Floating Aquatic Plants in the Río de la Plata Turbid Waters Using High Spatial Resolution Ocean Color Imagery. Remote Sensing 10, 1140. doi:10.3390/rs10071140
Dutta, A., and Zisserman, A. (2019). “The VIA Annotation Software for Images, Audio and Video,” In Proceedings of the 27th ACM International Conference on Multimedia, October 2019, Nice, France. Available at: http://www.robots.ox.ac.uk/. doi:10.1145/3343031.3350535
EDDMapS (2020). Early Detection & Distribution Mapping System. The University of Georgia - Center for Invasive Species and Ecosystem HealthAvailable at: http://www.eddmaps.org/ (Accessed November 6, 2020).
Geraeds, M., van Emmerik, T., de Vries, R., and bin Ab Razak, M. S. (2019). Riverine Plastic Litter Monitoring Using Unmanned Aerial Vehicles (UAVs). Remote Sensing 11 (17), 2045–2048. doi:10.3390/rs11172045
Gerolin, C. R., Pupim, F. N., Sawakuchi, A. O., Grohmann, C. H., Labuto, G., and Semensatto, D. (2020). Microplastics in Sediments from Amazon Rivers, Brazil. Sci. Total Environ. 749, 141604. doi:10.1016/j.scitotenv.2020.141604
González-Fernández, D., and Hanke, G. (2017). Toward a Harmonized Approach for Monitoring of Riverine Floating Macro Litter Inputs to the marine Environment. Front. Mar. Sci. (MAR) 4, 86. doi:10.3389/fmars.2017.00086
He, B., Wijesiri, B., Ayoko, G. A., Egodawatta, P., Rintoul, L., and Goonetilleke, A. (2020). Influential Factors on Microplastics Occurrence in River Sediments. Sci. Total Environ. 738, 139901. doi:10.1016/j.scitotenv.2020.139901
Hurley, R., Woodward, J., and Rothwell, J. J. (2018). Microplastic Contamination of River Beds Significantly Reduced by Catchment-wide Flooding. Nat. Geosci 11 (4), 251–257. doi:10.1038/s41561-018-0080-1
IUCN (2017). Water Hyacinth, an Invasive Plant in the Lake Tanganyika Basin. Available at: http://www.iucngisd.org (November 4, 2020).
Ivar do Sul, J. A., Costa, M. F., Silva-Cavalcanti, J. S., and Araújo, M. C. B. (2014). Plastic Debris Retention and Exportation by a Mangrove forest Patch. Mar. Pollut. Bull. 78 (1–2), 252–257. doi:10.1016/j.marpolbul.2013.11.011
Kleinschroth, F., Winton, R. S., Calamita, E., Niggemann, F., Botter, M., Wehrli, B., et al. (2021). Living with Floating Vegetation Invasions. Ambio 50 (1), 125–137. doi:10.1007/s13280-020-01360-6
Kurniawan, S. B., and Imron, M. F. (2019). Seasonal Variation of Plastic Debris Accumulation in the Estuary of Wonorejo River, Surabaya, Indonesia. Environ. Techn. Innovation 16, 100490. doi:10.1016/j.eti.2019.100490
Lahens, L., Strady, E., Kieu-Le, T.-C., Dris, R., Boukerma, K., Rinnert, E., et al. (2018). Macroplastic and Microplastic Contamination Assessment of a Tropical River (Saigon River, Vietnam) Transversed by a Developing Megacity. Environ. Pollut. 236, 661–671. doi:10.1016/j.envpol.2018.02.005
Lau, W. W. Y., Shiran, Y., Bailey, R. M., Cook, E., Stuchtey, M. R., Koskella, J., et al. (2020). Evaluating Scenarios toward Zero Plastic Pollution. Science 369 (6510), 1455–1461. doi:10.1126/science.aba9475
Lebreton, L., and Andrady, A. (2019). Future Scenarios of Global Plastic Waste Generation and Disposal. Palgrave Commun. 5 (1), 1–11. doi:10.1057/s41599-018-0212-7
Liro, M., Emmerik, T. v., Wyżga, B., Liro, J., and Mikuś, P. (2020). Macroplastic Storage and Remobilization in Rivers. Water 12 (7), 2055. doi:10.3390/w12072055
Liu, Y., Zhang, J., Tang, Y., He, Y., Li, Y., You, J., et al. (2021). Effects of Anthropogenic Discharge and Hydraulic Deposition on the Distribution and Accumulation of Microplastics in Surface Sediments of a Typical Seagoing River: The Haihe River. J. Hazard. Mater. 404, 124180. doi:10.1016/j.jhazmat.2020.124180
Martin, C., Almahasheer, H., and Duarte, C. M. (2019). Mangrove Forests as Traps for marine Litter. Environ. Pollut. 247, 499–508. doi:10.1016/j.envpol.2019.01.067
Martin, C., Baalkhuyur, F., Valluzzi, L., Saderne, V., Cusack, M., Almahasheer, H., et al. (2020). Exponential Increase of Plastic Burial in Mangrove Sediments as a Major Plastic Sink. Sci. Adv. 6 (44), eaaz5593. doi:10.1126/sciadv.aaz5593
Meijer, L. J. J., van Emmerik, T., van der Ent, R., Schmidt, C., and Lebreton, L. (2021). More Than 1000 Rivers Account for 80% of Global Riverine Plastic Emissions into the Ocean. Sci. Adv. 7 (18), eaaz5803. doi:10.1126/sciadv.aaz5803
Nguyen, T. T. N., Némery, J., Gratiot, N., Garnier, J., Strady, E., Nguyen, D. P., et al. (2020). Nutrient Budgets in the Saigon-Dongnai River basin: Past to Future Inputs from the Developing Ho Chi Minh Megacity (Vietnam). River Res. Applic 36 (6), 974–990. doi:10.1002/rra.3552
Ongore, C. O., Aura, C. M., Ogari, Z., Njiru, J. M., and Nyamweya, C. S. (2018). Spatial-temporal Dynamics of Water Hyacinth, Eichhornia crassipes (Mart.) and Other Macrophytes and Their Impact on Fisheries in Lake Victoria, Kenya. J. Great Lakes Res. 44 (6), 1273–1280. doi:10.1016/j.jglr.2018.10.001
Schwarz, A. E., Ligthart, T. N., Boukris, E., and van Harmelen, T. (2019). Sources, Transport, and Accumulation of Different Types of Plastic Litter in Aquatic Environments: A Review Study. Mar. Pollut. Bull. 143, 92–100. doi:10.1016/j.marpolbul.2019.04.029
Sharma, A., Aggarwal, N. K., Saini, A., and Yadav, A. (2015). Beyond Biocontrol: Water Hyacinth-Opportunities and Challenges. J. Environ. Sci. Techn. 9 (1), 26–48. doi:10.3923/jest.2016.26.48
Thamaga, K. H., and Dube, T. (2018). Remote Sensing of Invasive Water Hyacinth (Eichhornia crassipes): A Review on Applications and Challenges. Remote Sensing Appl. Soc. Environ. 10, 36–46. doi:10.1016/j.rsase.2018.02.005
van Calcar, C. J., and van Emmerik, T. H. M. (2019). Abundance of Plastic Debris across European and Asian Rivers. Environ. Res. Lett. 14 (12), 124051. doi:10.1088/1748-9326/ab5468
van Emmerik, T., Kieu-Le, T.-C., Loozen, M., van Oeveren, K., Strady, E., Bui, X.-T., et al. (2018). A Methodology to Characterize Riverine Macroplastic Emission into the Ocean. Front. Mar. Sci. 5 (OCT), 372. doi:10.3389/fmars.2018.00372
van Emmerik, T., and Schwarz, A. (2020). Plastic Debris in Rivers. WIREs Water 7 (1), e1398. doi:10.1002/wat2.1398
van Emmerik, T., Strady, E., Kieu-Le, T.-C., Nguyen, L., and Gratiot, N. (2019a). Seasonality of Riverine Macroplastic Transport. Sci. Rep. 9 (1), 1–9. doi:10.1038/s41598-019-50096-1
van Emmerik, T., Tramoy, R., van Calcar, C., Alligant, S., Treilles, R., Tassin, B., et al. (2019b). Seine Plastic Debris Transport Tenfolded During Increased River Discharge. Front. Mar. Sci. 6 (October), 1–7. doi:10.3389/fmars.2019.00642
Keywords: macroplastic, microplastic, riverine pollution, aquatic vegetation, observations, field data
Citation: Schreyers L, van Emmerik T, Nguyen TL, Castrop E, Phung N-A, Kieu-Le T-C, Strady E, Biermann L and van der Ploeg M (2021) Plastic Plants: The Role of Water Hyacinths in Plastic Transport in Tropical Rivers. Front. Environ. Sci. 9:686334. doi: 10.3389/fenvs.2021.686334
Received: 26 March 2021; Accepted: 11 May 2021;
Published: 25 May 2021.
Edited by:
Sergi Sabater, University of Girona, SpainReviewed by:
Tomoya Kataoka, Tokyo University of Science, JapanCopyright © 2021 Schreyers, van Emmerik, Nguyen, Castrop, Phung, Kieu-Le, Strady, Biermann and van der Ploeg. This is an open-access article distributed under the terms of the Creative Commons Attribution License (CC BY). The use, distribution or reproduction in other forums is permitted, provided the original author(s) and the copyright owner(s) are credited and that the original publication in this journal is cited, in accordance with accepted academic practice. No use, distribution or reproduction is permitted which does not comply with these terms.
*Correspondence: Louise Schreyers, bG91aXNlLnNjaHJleWVyc0B3dXIubmw=
Disclaimer: All claims expressed in this article are solely those of the authors and do not necessarily represent those of their affiliated organizations, or those of the publisher, the editors and the reviewers. Any product that may be evaluated in this article or claim that may be made by its manufacturer is not guaranteed or endorsed by the publisher.
Research integrity at Frontiers
Learn more about the work of our research integrity team to safeguard the quality of each article we publish.