- 1Department of Environmental Sciences, University of South Africa (UNISA), Johannesburg, South Africa
- 2Scientific Services, Research & Development Division, Magalies Water, Brits, South Africa
Phthalate esters (PEs) are by far the most produced and extensively used synthetic organic chemicals with notable applications in many industrial products such as vinyl upholstery, adhesives, food containers, packaging materials, printing inks, adhesives, cosmetics, paints, pharmaceuticals munitions, and insecticides among other. PEs have long been recognised as ubiquitous organic pollutants of prime environmental concern, with urbanisation amongst the main cause and source of these compounds. Due to their notoriety, these compounds are known to pose devastating effects to living organisms including humans. The presence of PEs and their metabolites in the aquatic ecosystems is of concern primarily due to their endocrine disrupting and carcinogenicity properties. Several research studies have reported prevalence, exposure pathways, toxicity, and impacts of PEs in aquatic ecosystems and humans. Their principal routes of exposure could be direct or indirect, of which the direct route include contact, eating, and drinking contaminated foods, and the indirect route constitute aerosols, leaching and other forms of environmental contamination. PEs find way into water systems through means such as effluent discharges, urban and agricultural land runoff, leaching from waste dumps and other diffuse sources. High-end instrumentation and improved methodologies on the other hand have resulted in increased ability to measure trace levels (μg/L) of PEs and their metabolites in different matrices and ecological compartments of water or aquatic ecosystems such as lakes, oceans, rivers, sediments, wetlands and drinking water samples. In light of the above, this article provides an informed and focused information on the prevalence of phthalate esters in aquatic systems and related effects on living organisms and humans. Furthermore, techniques that have enabled the extraction and analysis of these PEs in aquatic samples are also explained. Future research outlooks and needs are also highlighted in this manuscript. This information will be used to better understand their temporal and spatial distributions in the aquatic systems and aid in devising prudent means to curtail their ecological footprints.
Introduction
Phthalate esters (PEs) belong to a group of industrial organic compounds with a common chemical structure, dialkyl or alkyl/aryl esters of 1,2-benzenedicarboxylic acid (Annamalai and Vasudevan, 2020; Giuliani et al., 2020). They are esters of phthalic acid produced by reacting phthalic anhydride with an appropriate alcohol (Howdeshell et al., 2008; Vats et al., 2013; Annamalai and Vasudevan, 2020). The polar carboxyl group contributes little to the physical properties of PEs, except when R and R’ are very small alkyl groups (such as methyl and ethyl). The general chemical structure of PEs is shown in Figure 1, where R and R’ represents alkyl groups. The most commonly utilized PEs in consumer products are dimethyl phthalate (DMP), diethyl phthalate (DEP), diisobutyl phthalate (DIP), di-n-butyl phthalate (DBP), benzylbutyl phthalate (BBP), dicyclohexyl phthalate (DCHP), di-n-hexyl phthalate (DHP), bis (2-ethylhexyl) phthalate (DEHP), di-n-octyl phthalate (DOP), diisodecyl phthalate (DiDP) and diisononyl phthalate (DiNP) (Wang Y. et al., 2019; Dutta et al., 2020; Zhang et al., 2021). Table 1 highlights the chemical structures and uses of some of the most commonly used PEs.
PEs’ different chemical and physical properties account for many of their potential individual applications (Wang Y. et al., 2019). Their general low melting and high boiling points give them applications as heat-transfer fluids and carriers (Giuliani et al., 2020). Both linear and branched PEs are utilized in the production of plastics; with linear esters having outstanding flexibilities at low temperatures as they are less volatile. PEs with alkyl side chains lower than six carbons are not often used as plasticizers because volatility is a concern. PEs can be found in printing ink, paint, adhesives, vinyl flooring, and even in most food packaging materials, cosmetics, and pharmaceuticals products (Giuliani et al., 2020; Zhang et al., 2021).
Owing to their growing economic and commercial interest, PEs are by far the most used synthetic organic chemicals in a large variety of products since 1930s (Wang et al., 2018; Heo et al., 2020). Global production of PEs is estimated at 6 to 8 million tons per annum (Seyoum and Pradhan, 2019) with utilization by various industrial companies sitting at more than 3 million tons (Zhang et al., 2021). These compounds are mainly used for enteric coatings of nutritional supplements and pharmaceutical pills, as viscosity controlling agents, emulsifying agents, lubricants, binders, gelling agents, stabilizers film formers, dispersants, and suspending agents but primarily as plasticizers (Ji et al., 2014; Wang B. et al., 2015; Wang et al., 2018; Heo et al., 2020; USEPA, 2021). They are generally added to plastic materials such as polyethylene, polyethylene terephthalate, polyvinyl acetate and polyvinyl chloride (PVC), at the composition of up to 60% by weight of the PEs, to improve the elasticity and extensibility of the polymers (Giuliani et al., 2020). End applications comprise food products, building materials, children’s toys, medical devices, agricultural adjuvants, adhesives and glues, personal care products, detergents and surfactants, modeling clay, waxes, printing inks and coatings, paints, and textiles (Ling et al., 2007; Huang et al., 2008; Gao and Wen, 2016). A large variety of household applications, for example, vinyl upholstery, adhesives, shower curtains, floor tiles, cleaning materials, food holders and coverings contain PEs. PEs are also found in modern electronics (Chakraborty et al., 2019; Zhang et al., 2019) and clinical applications, for example, catheters and blood transfusion devices (Chiellini et al., 2013; Malarvannan et al., 2019). In light of their extensive uses and diverse applications, different studies have reported the presence of PEs residues in different receiving ecosystems (Salaudeen et al., 2018; Ai et al., 2021; Li et al., 2021), though here the aquatic ecosystems are reviewed. Furthermore, their magnificent bio-availability and degradability nature would indispensably foster high possibility for these compounds to accumulate in aquatic organisms and humans (Arambourou et al., 2019; He et al., 2020; Zhang et al., 2021).
This review was conducted through an exhaustive online database literature search from ScienceDirect (https://www.sciencedirect.com/), Google Scholar (https://scholar.google.com), international monitoring bodies databases such as World Health Organization (WHO), United States Environmental Protection Agency (USEPA) and United Nations Environmental Program (UNEP) to identify relevant and most recent articles and reports of PEs prevalence and their impacts on aquatic ecosystems worldwide. Studies considered were those published in accredited scientific journals and reports from recognized international bodies and majority of them were limited to a decade old (2011–2021), with preference given to those that are not older than 5 years. The following set of keywords were used to search and select the most suitable studies; phthalate esters, phthalate ester metabolites, phthalate esters in water, phthalate esters in sediments, phthalate esters in aquatic ecosystems, phthalate esters toxicity, phthalate esters bioaccumulation, phthalate esters biodegradation, health impacts of phthalate esters, treatment of phthalate esters. Based on the above criteria, more than 4,000 articles were found of which 184 articles deemed more relevant (including scientific reports) were used in the current article. The current state of knowledge regarding the occurrence, different extraction and analytical techniques and impacts of PEs in aquatic ecosystems is highlighted so as to provide a better understanding of their temporal and spatial distributions in aquatic systems and to aid in devising prudent means to curtail their ecological footprints.
Prevalence of Phthalate Esters in Aquatic Ecosystems
Due to their widespread domestic and industrial applications, it is not surprising that ubiquity of PE contaminants is noted in different aquatic ecosystem compartments such as: marine water, sediments, rivers, lakes, and wetlands, and from wastewater treatment plants (Gao and Wen, 2016; Al-Saleh et al., 2017; Wang et al., 2017; Salaudeen et al., 2018; Arfaeinia et al., 2019; Zhu Q. et al., 2019; Cheng et al., 2019; Zacharia, 2019; WHO, 2021). PEs are readily released during production, use and waste disposal, and can easily leach out into water (Vats et al., 2013; Salaudeen et al., 2018; Henkel et al., 2019). Specifically, they are easily released into the environment because there is no covalent bond between them and polymers in which they are mixed in (Koniecki et al., 2011; Zheng et al., 2014; Wang X. et al., 2015; Chi and Gao, 2015; Gao L. et al., 2019). Furthermore, as plastics age and breakdown, the release of PEs into the environment gets accelerated. Since PEs plasticizers are not chemically bound to PVC, they also easily filter into aquatic ecosystems among others (Olkowska et al., 2017; Chen et al., 2018; Kashyap and Agarwal, 2018). PEs enter the environment through several pathways including losses during manufacturing processes and weathering, leaching or volatilization from final products (Kashyap and Agarwal, 2018; Das et al., 2021). Several aquatic ecosystems such as lakes (Lee Y.-M. et al., 2019; Gao X. et al., 2019), wetlands (Gao and Wen, 2016; Wang et al., 2017), marine environments and rivers (Arfaeinia et al., 2019; Zacharia, 2019; Ai et al., 2021; WHO, 2021) have been characterized as highly polluted by PEs among other organic pollutants.
In the aquasphere, PEs occur at wide concentration range from trace (μg/L) to mg/L depending on the degree of PEs application and products. Large variety and abundance of PEs can be observed in aquatic ecosystems in different countries; however, the pollution levels and occurrences are mild to moderate. Adeniyi et al. (2011) reported the presence of up to 2,705 μg/L of PEs from river water in Nigeria, while Liu et al. (2015) reported between 0.02 and 0.77 μg/L in waterworks from China, from undetectable to 0.43 μg/L on surface water in Iran by Abtahi et al. (2019). Specifically, communities which are located adjacent to industries that use or produce these compounds tend to be greatly exposed to the PEs. Eventually, these compounds will find their way into the waterways since they are receptors of all effluents and pollutants in runoff. With PEs containing products and effluents being anthropogenically exorbitant in the aquatic ecosystems, it is also essential to understand the extent of occurrence and fate, biodegradation, bioaccumulation, and persistence.
In light of the above, an array of literature has investigated the temporal and spatial distribution of these compounds in different aquatic ecosystems to demonstrate the presence and distribution of these compounds in different countries globally (Paluselli et al., 2018; Abtahi et al., 2019; Mi et al., 2019; He et al., 2020; Ai et al., 2021). Furthermore, studies in the past decade that have reported the presence of PEs in aqueous and edaphic matrices including their concentration levels as reported in Tables 2, 3. DMP, DEP, DBP, BBP, and DEHP are the most dominant PEs in both aquatic sediments and waters with some DEHP concentrations frequently exceeding the annual average environmental water (freshwater and marine) quality standard of 1.3 μg/L (EU directive 2008/105/EC) (UNEP, 2020). The high number of recent PEs studies and PEs detection rate in Asia could probably be attributed to the higher volume of PEs produced and used in these environments, because of the absence of stricter regulations on usage of the PEs designated as priority pollutants unlike in developed countries of Europe and United States of America (UNEP, 2020; Das et al., 2021; Li et al., 2021). However, most values do not exceed the trigger threshold values of 5,100, 1,300, and 64.6 μg/L for DMP, DEP, and BBP respectively for protection of about 80% of aquatic species proposed by Australian and New Zealand guidelines (Net et al., 2015a). Ground and surface water bodies receive notable levels of PEs from different human activities such as agriculture, solid waste disposal, manufacturing industries and wastewater treatment plants (WWTPs) among others (Liu et al., 2015; Gao and Wen, 2016; Al-Saleh et al., 2017; Zhu Q. et al., 2019). A recent South African study by Salaudeen et al. (2018), revealed gross water pollution by PEs in the wastewater treatment plants (WWTPs) of Amathole Municipality in Eastern Cape province. In this study, the authors reported the presence of DMP, DEP, DBP, BBP, DEHP, and DOP of with DBP and DEHP as the most abundant PEs. The reported concentration in the influents were in the range of 2.7–2,488 μg/L and 130–1,094 μg/g dry weight in the sludge which exceeded acceptable limits of 100 μg/g for dry weight as recommended by the European Union for agriculture use (EU legislation 86/278/EEC). Furthermore, Salaudeen et al. (2018) reported the levels of PEs to be much higher in the WWTP unit processes than upstream of the WWTP and the effluents discharging point hence suggesting that the WWTPs are among the key source of anthropogenic PEs in the environment and they could be identified as one of the hotspots and source for PEs in aquatic ecosystems. In particular, the concentration of PEs ranged from undetectable to 17 μg/L upstream, 2.7–2,488 μg/L in the WWTPs, and undetectable to 25 μg/L in the effluent (Salaudeen et al., 2018). Considering the magnitude of their toxicity and impacts thereof, these compounds will drastically affect the receiving aquatic ecosystem and its integrity to foster life. Moreover, their composition could grossly be attributed to the level of anthropogenic activities, such as industries, that discharge their effluents to different handling facilities such as domestic and industrial WWTPs (Olijimi et al., 2012; Liu et al., 2015; Gao and Wen, 2016; Al-Saleh et al., 2017; Zhu Q. et al., 2019).
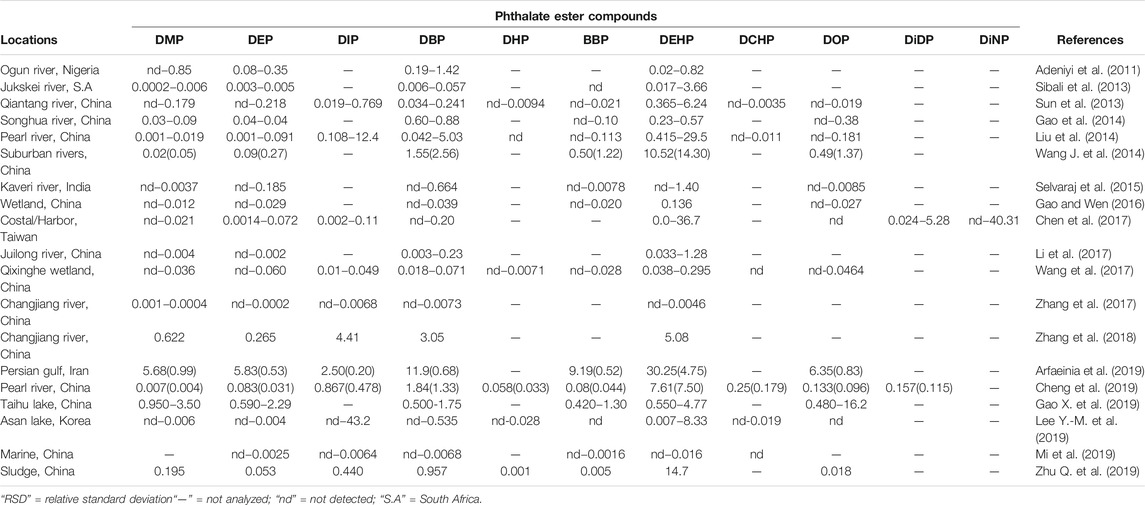
TABLE 3. Mean (RSD)/range concentrations of some PEs in sediments samples (μg/g dry weight) and standard deviations.
In aquatic ecosystem, some PEs are stable, persistent, and resistant to natural degradation, hence previous studies demonstrated the feasibility of PEs from contaminated water to bio-accumulate and bio-magnify in the food chain (Ma et al., 2013; Sun et al., 2015). Albeit, biodegradation is the dominant route for PEs degradation in the environment (Staples et al., 1997a; Chang et al., 2004; Xu et al., 2020; Das et al., 2021). Documented studies have reported that marine microalgal (Gao and Chi, 2015) and some microbial species possess the capability to degrade PEs (Ren et al., 2018; Yu et al., 2020), with microbial degradation considered the main route of PEs transformation (Yu et al., 2020). Microbial degradation is however, generally affected by external environmental factors and typical lack of specific degrading bacteria in the aquatic ecosystem, which in turn makes PEs degradation difficult under natural conditions, hence their long half-life, that range from a few years to several hundred years (Fang et al., 2018; Das et al., 2021; Zhang et al., 2021).
Interestingly, recent studies confirmed the ability of Bacillus mojavensis B1811 (Zhang et al., 2018a) and Paracoccus kondratievae BJQ0001 bacterium (Xu et al., 2020) to have high efficiency for the degradation of PEs. The results of the study by Zhang et al. (2018a), showed that at 0.5 μg/L; DEHP, DBP, BBP, and DPP could almost be completely degraded by strain B1811 within 4 days in mineral salt medium under shaking conditions, while only 5.9% of the DMP and 42.9% of DEP present with short alkyl chains, were degraded by strain B1811 under the same conditions with DMP and DEP degraded to 0.471 and 0.285 μg/L respectively. Xu et al. (2020), also found that strain BJQ0001 is also capable of successfully degrading substrates DMP, DEP, DBP, DIBP, and DEHP simultaneously when they are coexisting in the fermentation system at 0.2 μg/L of the PEs mixture, but with slightly reduced degradation rates. He et al. (2020), studied bioaccumulation of PEs in muscle tissues of domestic livestock (pig, cattle and chicken) and fish in China. In their study, the authors reported that most PEs congeners are not likely to accumulate in organisms due to their bioaccumulation factors being ≤2 except for DEP, DMP and BBP which were reported to comprise the BAFs values of ≥2, with the pig showing more capacity to accumulate PEs than the other animals’ tissues. This could be attributed to the fact that the pig generally has more fat contents than both the cattle and chicken and PEs are lipophilic.
Environmental and Human Health Risks Associated With Phthalate Esters
Phthalate Esters Impacts on Aquatic Ecosystems
Aquatic ecosystems such as rivers, dams, lakes and wetlands that are located within or passes through agricultural, industrial, residential and urban environments are exposed to a lot of different types of PEs from various anthropogenic inputs (Salaudeen et al., 2018; Ai et al., 2021; Li et al., 2021). Although both the cyanobacteria and freshwater algae are capable of producing DBP or monoethylhexyl phthalate (MEHP) under natural conditions, which can then be released to aquatic ecosystem, anthropogenic activities pollution such as agricultural pesticides, industrial effluents, commercial wastewaters, landfills (Zhang et al., 2021) and household-electronic and solid wastes (Zhang et al., 2019) all act as the main avenues of PEs into these aquatic ecosystems. Due to their high octanol-water partition (Kow/logKow25) (1.61–9.46) and low vapor pressures (Pa25) (1.84 × 10−6–0.263), most PEs entering the water environment have extremely low volatility hence can easily migrate into various water bodies and enter aquatic organisms (Net et al., 2015a; Das et al., 2021; Zhang et al., 2021). Since the value of KOW increases with increasing alkyl chain length, this results in increased hydrophobicity for the higher molecular weight PEs, hence their higher sorption to organic matter (Das et al., 2021; Zhang et al., 2021).
Upon entering the aquatic ecosystem, PEs directly come into contact with aquatic organisms. PEs can enter high-nutrient-grade aquatic organisms through ingestion and pass along the food chain (Zhang et al., 2021). PEs affect the aquatic ecosystem in diverse ways. Such is demonstrated by their ability to elicit their toxicity (immunotoxin, neurotoxic, genotoxic, endocrine, metabolic, and developmental toxicities) to fish and aquatic invertebrates with BBP, DEHP, and DBP eliciting the most effects (Staples et al., 1997b; Aarab et al., 2006; European Commission, 2008; Arambourou et al., 2019; Zhang et al., 2021). Thenceforth, the introduction of these compounds poses massive toxicity problem for the aquatic ecosystems and living organisms in there (Crafford and Avenant-Oldewage, 2010). As reviewed by Staples et al. (1997b), DMP, DEP, DAP, DBP, DIBP, and BBP exposure were found to have acute or chronic effects across, algae, invertebrates, and fish species. A recent review by Zhang et al. (2021) also noted acute and chronic toxic effects on aquatic organisms, with symptoms such as crooked tails, necrosis, cardiac edema, lack of tactile response and death on aquatic animal embryos, while on adult organisms, PEs exposure could lead to adverse effects on reproduction, damage to the liver, kidney, and other organs (Gao et al., 2018). It was noted that lower molecular weight PEs were acutely and chronically toxic at concentrations below their aqueous solubilities and that toxicity increased with increasing alkyl chain length up to and including four carbon atoms (Staples et al., 1997b). Reviewed ecotoxicity studies for these PEs showed adverse effects to aquatic organisms with broad range of endpoints and at much lower concentration levels (ng/L to μg/L) than that are being obtained in recent environmental studies (Oehlmann et al., 2009; Zhang et al., 2021).
Toxicity of chemical substances to aquatic organisms is determined by the median lethal concentration (LC50) (Huang et al., 2020; Zhang et al., 2021). As reviewed by Zhang et al. (2021), the common carp 96 h LC50 ranges for DEP, DBP, DOP, and DEHP are 34.8–53, 16.30–35, 7.95, and 37.95 mg/L respectively, while the lethal concentrations of DEP and DBP in marine flounder and Nile tilapia are also similar to those of carp. Contrary to this, lethal concentration of other small-sized fish such as juvenile triangular Bream (Magalobrame tarminalis) is significantly lower, with 96 h LC50 values for DMP, DEP, DBP, and DEHP as low as 3.29, 6.60, 2.08, and 5.41 mg/L respectively. They are also toxic for many other aquatic species with DEP’s LC50/EC50 values of 3 and 132 mg/L for marine algae and protozoan respectively, with the lowest NOECs (1.7–4 mg/L) for algae, fish and invertebrates (Staples et al., 1997b; Net et al., 2015a). Some of these toxicity studies on aquatic organisms are listed in Table 4 with their health effects. However, some aquatic organisms like micro-algae have PEs degradation capabilities. Instead of being toxicologically affected, benthic diatom (Cylindrotheca closterium) demonstrated the ability to accelerate degradation of DEP and DBP (Li et al., 2015). Research indicate that most PEs (with short carbon-chains) are degradable in the environment, however some tend to bioaccumulate in biological samples (He et al., 2020; UNEP, 2020).
While PEs tend to be ubiquitous in the biosphere, there is limited information about their route of exposure, bioaccumulation behavior, and human exposure. Furthermore, eco-toxicological studies have played a pivotal role in determining the degree at which these compounds accumulate in biota, specifically the plants and animals. Net et al. (2015a), indicated that a high capacity to accumulate the PEs by species could be obtained when the BAFs values are larger than 1,000 [log (BAFs) > 3]. In their study, the authors reported the mean log (BAFs) values which ranged between 0.91 and 2.96, thus implying that most PEs congeners are not likely to be accumulative in organisms. It is worth noting that large variances of BAFs values of individual PEs can also be found in other studies as reported by Net et al. (2015a), and for this, the explanation could be based on the discrepancies between the physicochemical properties of individual PEs, variances in temporal bioavailability, and species-specific in metabolic capability, resulting in individually and/or jointly impacting on the bioaccumulation of PEs in the biota (Adeogun et al., 2015; Net et al., 2015a). This further necessitates the need for improved and continual monitoring of PEs in aquatic ecosystems, especially those being utilized by communities for various basic functions such as fishing, irrigation, drinking and bathing. Such is evidenced by the recent study by He et al. (2020), in a rural agricultural area of western China where people are dependent on river water for domestic and household uses and found that their pigs, cattle and chicken meat samples all contained PEs. A significant correlation was also observed between the biota and river water samples, indicating that river water heavily influenced PEs uptake and accumulation in the biological samples (He et al., 2020). PEs, notably high molecular weight ones, have exceptional ability to accumulate in sediments; where sorption to particles and the low-oxygen conditions slows PEs degradation process by reducing their degradation rates (UNEP, 2020).
Phthalate Esters Exposure, Toxicity and Human Health Effects
Humans are continually exposed to PEs daily as these compounds are found in little quantities in literally thousands of industrial and household products ranging from cosmetics, toys, curtains, electrical appliances, food, packaging materials, beverages and drinking water (Baloyi, 2012; Gong et al., 2018; Luo et al., 2018; Abtahi et al., 2019; He et al., 2020). Humans are exposed to PEs from aquatic ecosystems via different routes, namely; dermal skin absorption, inhalation, and ingestion from food and drinking water (Alharbi et al., 2018; Paluselli et al., 2018; Abtahi et al., 2019; Giuliani et al., 2020). Upon exposure or uptake, effects exerted range from mutagenicity, teratogenicity, and carcinogenicity. In fact, global concern about PEs arise due to their hazardous, toxicity, mutagenic, teratogenic and carcinogenic characteristics to humans on exposure (Gao and Wen, 2016; Zarean et al., 2016; Zacharia, 2019; Li et al., 2020; USEPA, 2021). PEs at varying levels of exposure may adversely affect human health by acting as endocrine disruptors (Zarean et al., 2016; De Toni et al., 2017; Jiang et al., 2018; Ren et al., 2018; Zhu M. et al., 2019; Zhang et al., 2021). Endocrine disruptors are sometimes also referred to as hormonally active agents that act like hormones in the endocrine system, disrupting the physiological functioning of endogenous hormones (Balaguer et al., 2019; Darbre, 2019; Xu and Yin, 2019; Talia et al., 2021; You and Song, 2021). The endocrine system in humans and most groups of animal life, including aquatic organisms, consists of glands that secrete hormones, and receptors that detect and react to the hormones (Hiller-Sturmhöfel and Bartke, 1998). Studies have associated endocrine disruptors to severe biological effects in animals, giving rise to concerns that low-level exposure to PEs might cause similar effects in human beings (Krimsky, 2001; Lin et al., 2018; Balaguer et al., 2019; USEPA, 2021) since PEs have similar effects on the endocrine system (WHO/UNEP, 2013).
The theory of endocrine disruption posits that low-dose exposure to chemicals that interact with hormone receptors can interfere with reproduction, development, and other hormonally mediated processes (Zoeller et al., 2014; Gore et al., 2015; Xu and Yin, 2019). Furthermore, since endogenous hormones are typically present in the body in relatively tiny concentrations, the theory also holds that exposure to relatively small amounts of exogenous hormonally active substances such as PEs could disrupt proper functioning of the body’s endocrine system (Diamanti-Kandarakis et al., 2009; Marty et al., 2011). Thus, endocrine disruptors such as PEs elicit adverse effects at much lower doses than a toxicant acting through a different mechanism. The half-life of PEs diesters in human biomonitoring studies in blood plasma and/or urine of humans and rodents is less than 24 h (Wang Y. et al., 2019; Zhang et al., 2019; Giuliani et al., 2020; Shin et al., 2020). Other studies have reviewed pharmacokinetics of PEs and found that diesters are rapidly hydrolyzed to monoesters in the gastrointestinal tract (Latini, 2005; Frederiksen et al., 2007; Dodson et al., 2014; Cequier et al., 2015). While binding of DEHP metabolites to blood plasma proteins, biliary excretion, and enterohepatic circulation in humans have been suggested (Frederiksen et al., 2007), urinary excretion remains the major elimination pathway of PEs (Wang Y. et al., 2019). In one study by Jiang et al. (2018), prolonged blood clotting time, decreased hemoglobin concentrations, and increased likelihood of anemia were all associated with high urinary phthalate metabolites in pregnant women on their late third trimester.
PEs exposure timing is presumed to be critical, since different hormone pathways are active during different stages of development (WHO/UNEP, 2013). High doses of certain PEs have shown hormonal activity in rodents’ studies with reported liver and testicles damages and birth defects. Exposure to PEs during pregnancy could result in birth defects such as venereal enlargement of the male offspring and various forms of altered genitals developments, low sperm count and quality (Swan et al., 2005; Philippat et al., 2012; Sathyanarayana et al., 2014; Qureshi et al., 2016; Huang et al., 2017; Hoffman et al., 2018; Rehman et al., 2018; Xie et al., 2019). A study by Colon et al. (2000), found that Puerto Rican girls with premature breast development had higher levels of PEs in their blood compared to other girls, suggesting an association between PEs exposure and abnormal reproductive organs development. Swan et al. (2005) also found that baby boys born to mothers with highest levels of PEs were seven times more likely to have a shortened anogenital distance. This authenticates that one way or another, prenatal PEs exposure could and could’ve played a role in most cases of abnormal reproductive organs development, miscarriage and adverse birth outcomes (Toppari et al., 2006; Tilson, 2008; Mu et al., 2015; Hoffman et al., 2018). Therefore, it is very important not to only screen and monitor but also put preventative measures in place to limit exposure to such important endocrine disruptors as this could influence the fetal health (Chen et al., 2019).
PEs have also been linked with endometriosis and reduced female fertility (Cobellis et al., 2003; Reddy et al., 2006), obesity, preterm birth and low birth weight (Mu et al., 2015; Stojanoska et al., 2017; Hoffman et al., 2018), diabetes in adults (Svensson et al., 2011; Stojanoska et al., 2017), autism spectrum disorders (Jeddi et al., 2016), changes in sex hormone levels, breast cancer and prostate cancer (Lopez-Carrillo et al., 2010; Zhu M. et al., 2019), adverse effects on immune system, bronchial obstruction and asthma symptoms and neuropsychological development (Cho et al., 2010; Tellez-Rojo et al., 2013; Wang I.-J. et al., 2014; Kim et al., 2018; Zhang et al., 2019). PEs exposure could also interfere with production of the male sex hormones and testosterone, which is necessary for proper development and functioning of the male reproductive organs (Xie et al., 2019). Interference with testosterone activity, especially early in life, can have irreversible undesirable effects on male reproduction organs.
Some of the suggestions on avoiding and/or minimizing exposure to PEs for concerned people, are based on the available literature on occurrence and levels of PEs. Although with limited evidence that this will positively affect one’s health, suggestions to minimize effects include among other strategies; eating a balanced diet, reduced consumption of canned and plastic packaged food, no nutritional supplements, no microwaved food, frequent hand washing, minimal application of personal care products such as cosmetics, moisturizers and perfumes that contains PEs (Koniecki et al., 2011; Chen et al., 2015; Braun, 2017; Wang Y. et al., 2019). In Taiwan, Chen et al. (2015) explored these PEs exposure reduction strategies in 30 (4–13 years old) young girls and found that pre-intervention dominant urinary PEs metabolites were significantly lower post the one-week intervention period. The study found that frequent hand washing (p = 0.009) and reduced intake of beverages in plastic cups (p = 0.016) were the most effective ways to reduce PEs metabolites in urine, particularly DEHP and mono-n-butyl phthalate (MBP) metabolites (Chen et al., 2015). However, avoiding personal care products containing PEs can be tricky and impossible as countries such as the United States do not require that PEs be disclosed on the list of the ingredients (Dodson et al., 2012). It is also recommended to avoid and minimize utilization of untreated water from the rivers as they can act as a major source of exposure to human by PEs, especially in rural areas where access to clean water is limited. A study by He et al. (2020), in China, found that untreated river water accounted for 79, 83, and 88% of the estimated PEs (DMP, DEP, BBP, DIBP, DNBP, DEHP, and DOP) daily intakes in toddlers, children and adults respectively, which exhibited an increasing trend with age, and this agreed with another study, also in China by Ji et al. (2014), where water ingestion and/or absorption was the major source of exposure to DBP, DEHP, and DOP. It is also worth noting that domestic animal meat can also serve as another route of PEs exposure, especially pork, beef, chicken and some vegetables (He et al., 2020) and some crops producing eaten grains such as rice, maize and wheat (Sun et al., 2015; Xu et al., 2020), so it is befitting to suggest and recommend consumption of organic farm produces which contain no synthetic products.
An assessment report by UNEP (2020) on chemicals and waste issues posing risks to human health and the environment has reviewed measures taken by several countries to reduce the use and exposure of PEs through various means. Among these measures, Denmark successfully reduced the use and exposure to PEs by introducing tax on products containing PVC and phthalates, although the tax was then repealed in 2019, in part due to reductions in the overall utilization of PEs. The Korean Ministry of Food and Drug Safety adopted and prohibited the production, import sale or use medical devices containing DBP, BBP, and DEHP. China, through National Food Safety Standard prohibited the use of DMP, DBP, DOP, and DiDP as additives in food contact materials, and banned the use of DBP, BBP, DEHP, DOP, DiNP, and DiDP in infants and children’s textile products. Canadian government has already banned the use of DEHP in cosmetics and medical devices and restricts concentrations of BBP, DBP, DEHP, DOP, DiNP, and DiDP to below 1 mg/kg in childcare articles. Columbia and Peru also restrict contents of BBP, DBP, DOP, DEHP, DiNP, and DiDP in flexible plastic for children (UNEP, 2020). The widespread utilization, potential exposure and effects of PEs also necessitated the need for monitoring by international agencies such as the United Nations Environmental Program (UNEP), International POPs Elimination Network (IPEN), United States National Health and Nutrition Examination Survey (NHANES), United States Centers for Disease Control and prevention (USCDC), United States Environmental Protection Agency (USEPA) and Stockholm Convention, and for the Agency for Toxic Substances and Disease Registry (ATSDR) and European Food Safety Authority (EFSA) to regulate and recommend toxicity limits for some of the most utilized PEs. Table 5 lists recommended levels of some individual PEs as set out by ATSDR (2021), EFSA (2019), with regulated concentration in the form of Minimal Risk Levels (MRLs) and Tolerable Daily Intake (TDI). A study by the United States National Academies of Sciences, Engineering and Medicine (2017) found that although the current toxicity test methods can identify DEHP hazard, they may not accurately and conclusively be able to predict the levels at which humans are affected, implying that some of the reference doses and other “safe” limits established by regulatory agencies based on animal testing, may in fact be not safe to humans (UNEP, 2020). Such discovery calls urgently for improved and reliable methods for PEs biomonitoring studies on human samples. Statistically representative sample of United States population in a 2015–2016 NHANES survey revealed that high concentration of PEs was prevalent among people living below the poverty line (USCDC, 2018; USEPA, 2018). Similar trend of high exposure to PEs among children of low socio-economic income household was also found to be prevalent in Canada (Navaranjan et al., 2019; UNEP, 2020). It is worth noting that there are no known or documented MRLs/DTI and/or toxicity endpoints for majority of the PE compounds yet. Worryingly, these compounds are not regulated in most developing countries, specifically the low-and middle-income countries (LMIC) hence their impacts might be catastrophic, yet they are not captured in any epidemiological report or assay.
Extraction and Analysis of Phthalate Esters in Aquatic Ecosystems
Extraction of PEs
Like most organic compounds contaminants that are of environmental concern, PEs are also lipophilic, and thus typically extract into lipid soluble compounds. These esters have been detected in very low ranges of parts per billions (ppb) and parts per million (ppm) in different environmental samples (Sibali et al., 2013; Lee Y.-M. et al., 2019; Arfaeinia et al., 2019; Dong et al., 2019; Zhang et al., 2019; Annamalai and Vasudevan, 2020; He et al., 2020). Reliable and sensitive analytical methods for trace detection are therefore paramount. PEs analysis procedure generally involve a three-step process; extraction, column clean-up and chromatographic determination and/or quantification. Different methods and procedures for PEs determination have been reviewed and findings are well documented (Arfaeinia et al., 2019; Annamalai and Vasudevan, 2020; He et al., 2020). All these studies employed a typical separation approach comprising of sample collection, extraction of target compounds from the samples and extracts clean-up process, then qualitative/quantitative analysis. The most common and basic methods are briefly explained below.
Solid Phase Extraction
SPE is a sample preparation process by which analytes are isolated from wide variety of matrices (compounds) in the mixture according to their physical and chemical properties (Falenas, 2019). SPE is advocated for due to its ease of implementation, ability to save time and solvent (Net et al., 2015b). SPE has been used to isolate PEs with great success from river and tap waters (Dominguez-Morueco et al., 2014; Selvaraj et al., 2015; Wang R. et al., 2019). Advances have been made recently to improve applicability and reliability of SPE by utilizing different covalent organic framework (COF) adsorbents (Santana-Mayor et al., 2018; Tong et al., 2019; Pang et al., 2020). Examples of such are the successful development and applications among others of fabricated COF-(TpBD)/Fe3O4 (rapid magnetic separation and reusable after washing with methanol) to magnetic solid phase extraction of 15 PEs with detection limit as low as 0.005 µg/L and good recoveries of 79.3–121.8% (Pang et al., 2020); 0.01–0.28 µg/L detection limit and recoveries of 80–112.8% with fabricated core-shelled Fe3O4 graphitic carbon sub-microcube (Tong et al., 2019); 0.006–0.178 µg/L detection limit and recoveries of 70–120% with Fe3O4 nanoparticles coated with reduced-graphene oxide (Santana-Mayor et al., 2018). However, the downsides to these advances are that they are delicate and expensive therefore they are not practical especially for developing countries.
Solid Phase Micro-Extraction
SPME employs a miniature automatic device to integrate sampling, enrichment and separation, purification, concentration and injection into one simple procedure which is more sensitive and environmentally friendly (Yang et al., 2015). Introduction of modulating agent into the synthesis fibers enhances PEs extraction efficiency significantly which is evident achieved satisfactory recoveries (Wang X. et al., 2019; Guo et al., 2019). In early 21st century, novel SPME systems with new selective coatings received much attention in the development of SPME (Mehdinia and Aziz-Zanjani, 2013). In 2010, a headspace SPME approach was established and optimized for analysis of sixteen PEs compounds in oil matrices with a special fiber as sorbent. This approach had the major advantage of not requiring any sample manipulation thereby minimizing cross contamination from glassware, solvents and samples. With minor modifications to the method, it has been employed by several other researchers in China for PEs analysis in marine water samples with relative standard deviations of less than 10% and different satisfactory recoveries; 68–114% (Zhang et al., 2017), 55.4–110.9% (Zhang et al., 2018b), in South Africa for WWTPs effluents; 75–116% (Salaudeen et al., 2018). However, the method’s main drawbacks are that the fibers are rather expensive and tend to break (Yang et al., 2015).
Soxhlet Extraction
This method is applicable to the extraction of non-volatile and semi volatile organic compounds from solids such as sediments, sludges, soils and solid wastes (Sibali, 2008). Soxhlet extraction uses relatively affordable glassware and organic solvents. Once loaded it requires no hands-on manipulation and provides efficient extraction. In one study by Liu et al. (2014), SE was used for sixteen PEs analysis from urban lakes and rivers in China, with reported recoveries of 61–112% and average relative standard deviations of less than 10% for water and sediments samples. In South Africa, SE optimized and applied for PEs extraction on soil and sediments samples from Muledane open dump, Thohoyandou, Limpopo province, with recoveries of between 88–90% (Adeniyi et al., 2008; Fatoki et al., 2010). Also, in Venda, Limpopo province, South Africa, Fatoki et al. (2010). With minor modifications and recoveries of 90–122%, SE was also optimized and applied for PEs extraction from sediments samples in Jukskei river catchment in Gauteng province of South Africa (Sibali et al., 2013).
Liquid-Liquid Extraction
LLE, also known as solvent extraction is a basic technique in the laboratory and a traditional method used to separate compounds based on their preferences for two different immiscible liquids, usually water and an organic solvent. It is an extraction of lipophilic compounds from aquatic medium which results in the water rich and the organic rich phases (Cai et al., 2007). LLE has been used in a couple of environmental studies involving the analysis of PEs in aquatic ecosystems (Cai et al., 2007; Fatoki et al., 2010; Hadjmohammadi and Ranbari, 2011; Sibali et al., 2013). Fatoki et al. (2010), optimized and applied LLE for PEs analysis from Venda, Limpopo province, South Africa, with recoveries of 81–90% and relative standard deviation of less than 5%. In Africa, with minor modifications, Sibali et al. (2013) also successfully optimized and utilized the LLE method to extract PEs from water samples collected in Jukskei river catchment in Gauteng province of South Africa with recoveries of 95–122% and relative standard deviation of less than 10% while Nantaba et al. (2021) obtained recoveries of 83–115% in Lake Victoria of Uganda. Some of the benefits for LLE are the easy phase separation, rapid partition equilibrium, less toxicity and good compatibility for the subsequent determination (Cai et al., 2007).
Ultra-Sonication Extraction
Selvaraj et al. (2015), utilized the method to extract PEs from the sediments with good recoveries of 79–121% in India. With minor modifications, USE method was also applied to extract PEs from sediments in China, recoveries of 69–123% and relative standard deviations of less than 10% were reported (Wang et al., 2017; Zhang et al., 2017; Zhang et al., 2018b; Weizhen et al., 2020). Li et al. (2015), also employed the method on biodegradation of both diethyl phthalate and dibutyl phthalate esters from marine sediments albeit with no percentage recovery reported, this demonstrate the applicability of the method.
Sample Clean-Up and PEs Analysis
Extracts from environmental samples usually contain large amount of other potential interfering organic compounds which could give rise to high background during instrumental analysis, hence a clean-up process is necessary before analysis. More often, this is done by adsorption chromatography in the form of aluminum oxide, florosil or silica gel columns which traps unwanted compounds. Should the clean-up step be omitted the crude extracts are likely to contaminate the capillary column during instrumental analysis. Generally, the glass column is prepared by packing it with glass wool at its base and about 1–5 g of silica gel/florisil/aluminum oxide slurry in extracting solvent, anhydrous sodium sulfate is added above the column packing material (about 1 cm) to dehydrate the extracts. The column is saturated with extracting solvent, extract loaded on to the column and eluted with enough extracting solvent to ensure that all target compounds are eluted. The final eluate is concentrated to about 1 ml before Gas chromatography (GC) analysis (Fatoki et al., 2010; Sibali et al., 2013; Liu et al., 2014; Lee Y.-M. et al., 2019) or liquid chromatography (LC) such as high performance or Ultra performance coupled with mass spectroscopy (MS) (Santana-Mayor et al., 2018; He et al., 2020).
Development of gas chromatograph fused-silica capillary columns has resulted in an increased separation powers for PEs (Liu et al., 2013; Yang et al., 2015). This is greatly due to the general low molecular weight, relatively low polarity and thermal stability of PEs. GC methods combined with mass spectroscopy (MS), MS/MS, Electron capture detector (ECD) or flame ionization detectors (FID) have steadily become the main tool for identification and quantification of PEs in the 21st century (Yang et al., 2015; Salaudeen et al., 2018; Weizhen et al., 2020), and to some extent, LC coupled to MS detector (He et al., 2020). Common methods used for determination and analysis of PEs from different environmental matrices are based on GC-MS/FID (Sibali et al., 2013; Qureshi et al., 2016; De Toni et al., 2017; Zhang et al., 2018c; Salaudeen et al., 2018; Li et al., 2020), GC-ECD (Weizhen et al., 2020), Stir-Bar Sorptive Extraction-Thermal Desorption (SBSE-TD)-GC/MS (Heo et al., 2020), Ultra Performance Liquid Chromatography (UPLC) coupled to a Quadrupole Time of Flight Mass Spectrometer (TOF-MS) (He et al., 2020), ultra-high-performance liquid chromatography coupled to triple quadrupole tandem mass spectrometry (UHPLC-QqQ-MS/MS) (Santana-Mayor et al., 2018). Quantification of PEs is generally performed using internal standard calibration method (Liu et al., 2014; Lee Y.-M. et al., 2019; He et al., 2020; Li et al., 2020; Weizhen et al., 2020). Table 6 gives a summary of various extraction methods used on different environmental matrices, percentage recovery rate, detection limits and analytical instrument used.
All methods discussed demonstrate their fair share in terms of applicability and reliability for PEs extractions from different environmental matrices by yielding good and acceptable recoveries, especially the solid phase extraction. However, development of covalent organic framework absorbents for solid phase extraction is a delicate and time-consuming process. USE has also yielded great recoveries, but the downside is the need for hands-on and multiple extraction processes to concentrate the extracts. Although solid phase micro-extraction is regarded as an innovative solvent free extraction method with satisfactory recoveries, it is very technological which limits the applicability of the method, especially to under-developed and developing countries without access to state-of-the-art instrumentations. It was also noted from the reviewed research that LLE was barely utilized in the last decade, instead SPE and SPME have become the most preferred methods for PEs extraction from aquatic water matrices.
Conclusion
Occurrence of PEs in the environment is undoubtedly of great concern and more so in aquatic ecosystem where diverse sources are responsible for input of these toxic pollutants into the water and sediments. Biomonitoring studies elsewhere have clearly demonstrated that both human and aquatic life exposure from PEs polluted water is ubiquitous. PEs toxicity in aquatic ecosystems has been shown to affect biota therein and is also associated with human exposure and health risks that result from human contact, ingestion of contaminated food (e.g. fish and crops irrigated with PEs polluted water), and inhalation. Different toxicity studies have characterized the adverse effects (hatchability, embryonic development, and sex ratio) of PEs on different tissues, organs, and systems of aquatic organisms. The need to protect water resources and the aquatic ecosystem is well demonstrated through the multitude monitoring and toxicity data availed from various researches. Different sources of exposure to PEs are well documented but several questions about cumulative exposures, contributions of various sources in toxicity and mixed exposures remain partially answered. While the review here has demonstrated that there has been a lot of research characterizing and monitoring PEs in aquatic ecosystems and their effects on humans and other living organisms, very limited action has been implemented toward strict regulation and regulatory enforcement for these compounds in many developing countries. Although several countries have made efforts to regulate and limit the distribution and application of PEs by various industrial fields, through the establishment of various policy thresholds that restrict and limit the amount of PEs on certain products, and tolerable intake through MRLs and TDI for individual PEs per person. However, these efforts seem to be ineffective as exposure to PEs is exacerbated by the expanding human population and increasing PEs production and widespread application in various industries. There is ongoing need for regulatory agencies to put in place more research informed environmental, health-protective and legally binding regulations as well as enforcing them.
Author Contributions
All the authors contributed to the drafting, revision and approved the submitted article version. NB took responsibility of handling the compilation of article as first author while MT took the lead role on submission and revision of the article as corresponding senior author.
Conflict of Interest
The authors declare that the research was conducted in the absence of any commercial or financial relationships that could be construed as a potential conflict of interest.
References
Aarab, N., Lemaire-Gony, S., Unruh, E., Hansen, P. D., Larsen, B. K., Andersen, O. K., et al. (2006). Preliminary Study of Responses in Mussel (Mytilus Edilus) Exposed to Bisphenol A, Diallyl Phthalate and Tetrabromodiphenyl Ether. Aquat. Toxicol. 78, S86–S92. doi:10.1016/j.aquatox.2006.02.021
Abtahi, M., Dobaradaran, S., Torabbeigi, M., Jorfi, S., Gholamnia, R., Koolivand, A., et al. (2019). Health Risk of Phthalates in Water Environment: Occurrence in Water Resources, Bottled Water, and Tap Water, and burden of Disease from Exposure through Drinking Water in Tehran, Iran. Environ. Res. 173, 469–479. doi:10.1016/j.envres.2019.03.071
Adeniyi, A. A., Okedeyi, O. O., and Yusuf, K. A. (2011). Flame Ionization Gas Chromatographic Determination of Phthalate Esters in Water, Surface Sediments and Fish Species in the Ogun River Catchments, Ketu, Lagos, Nigeria. Environ. Monit. Assess. 172, 561–569. doi:10.1007/s10661-010-1354-2
Adeniyi, A., Dayomi, M., Siebe, P., and Okedeyi, O. (2008). An Assessment of the Levels of Phthalate Esters and Metals in the Muledane Open Dump, Thohoyandou, Limpopo Province, South Africa. Chem. Cent. J. 2 (9), 1–9. doi:10.1186/1752-153X-2-9
Adeogun, A. O., Ibor, O. R., Omogbemi, E. D., Chukwuka, A. V., Adegbola, R. A., Adewuyi, G. A., et al. (2015). Environmental Occurrence and Biota Concentration of Phthalate Esters in Epe and Lagos Lagoons, Nigeria. Mar. Environ. Res. 108, 24–32. doi:10.1016/j.marenvres.2015.04.002
Ai, S., Gao, X., Wang, X., Li, J., Fan, B., Zhao, S., et al. (2021). Exposure and Tiered Ecological Risk Assessment of Phthalate Esters in the Surface Water of Poyang Lake, China. Chemosphere 262, 127864. doi:10.1016/j.chemosphere.2020.127864
Al-Saleh, I., Elkhatib, R., Al-Rajoudi, T., and Al-Qudaihi, G. (2017). Assessing the Concentration of Phthalate Esters (PAEs) and Bisphenol A (BPA) and the Genotoxic Potential of Treated Wastewater (Final Effluent) in Saudi Arabia. Sci. Total Environ. 578, 440–451. doi:10.1016/j.scitotenv.2016.10.207
Alharbi, O. M. L., Basheer, A. A., Khattab, R. A., and Ali, I. (2018). Health and Environmental Effects of Persistent Organic Pollutants. J. Mol. Liquids 263, 442–453. doi:10.1016/j.molliq.2018.05.029
Annamalai, J., and Vasudevan, N. (2020). Detection of Phthalate Esters in PET Bottled Drinks and lake Water Using esterase/PANI/CNT/CuNP Based Electrochemical Biosensor. Analytica Chim. Acta 1135, 175–186. doi:10.1016/j.aca.2020.09.041
Arambourou, H., Planelló, R., Llorente, L., Fuertes, I., Barata, C., Delorme, N., et al. (2019). Chironomus Riparius Exposure to Field-Collected Contaminated Sediments: from Subcellular Effect to Whole-Organism Response. Sci. Total Environ. 671, 874–882. doi:10.1016/j.scitotenv.2019.03.384
Arfaeinia, H., Fazlzadeh, M., Taghizadeh, F., Saeedi, R., Spitz, J., and Dobaradaran, S. (2019). Phthalate Acid Esters (PAEs) Accumulation in Coastal Sediments from Regions with Different Land Use Configuration along the Persian Gulf. Ecotoxicology Environ. Saf. 169, 496–506. doi:10.1016/j.ecoenv.2018.11.033
Arukwe, A., Ibor, O. R., and Adeogun, A. O. (2017). Biphasic Modulation of Neuro- and Interrenal Steroidogenesis in Juvenile African Sharptooth Catfish (Clarias gariepinus) Exposed to Waterborne Di-(2-ethylhexyl) Phthalate. Gen. Comp. Endocrinol. 254, 22–37. doi:10.1016/j.ygcen.2017.09.007
ATSDR (2019). Minimal Risk Levels (MRLs) for Hazardous Substances. Available at: https://www.atsdr.cdc.gov/mrls/mrllist.asp (Accessed 09 October, 2019).
ATSDR (2021). Minimal Risk Levels (MRLs) for Hazardous Substances. Available at: https://wwwn.cdc.gov/TSP/MRLS/mrlsListing.aspx (Accessed April 21, 2021).
Balaguer, P., Delfosse, V., and Bourguet, W. (2019). Mechanisms of Endocrine Disruption through Nuclear Receptors and Related Pathways. Curr. Opin. Endocr. Metab. Res. 7, 1–8. doi:10.1016/j.coemr.2019.04.008
Baloyi, N. D. (2012). Prevalence of Endocrine Disrupting Phthalate Esters in Selected Foods and Food Wrappers from Some Supermarkets Around Pretoria, South Africa. South Africa: University of South Africa. [master’s dissertation].
Bhatia, H., Kumar, A., Du, J., Chapman, J., and McLaughlin, M. J. (2013). Di-n-butyl Phthalate Causes Antiestrogenic Effects in Female Murray Rainbowfish (Melanotaenia fluviatilis). Environ. Toxicol. Chem. 32, 2335–2344. doi:10.1002/etc.2304
Bhatia, H., Kumar, A., Ogino, Y., Gregg, A., Chapman, J., McLaughlin, M. J., et al. (2014). Di-n-butyl Phthalate Causes Estrogenic Effects in Adult Male Murray Rainbowfish (Melanotaenia fluviatilis). Aquat. Toxicol. 149, 103–115. doi:10.1016/j.aquatox.2014.01.025
Braun, J. M. (2017). Early-life Exposure to EDCs: Role in Childhood Obesity and Neurodevelopment. Nat. Rev. Endocrinol. 13 (3), 161–173. doi:10.1038/nrendo.2016.186
Cai, Y., Cai, Y., Shi, Y., Liu, J., Mou, S., and Lu, Y. (2007). A Liquid–Liquid Extraction Technique for Phthalate Esters with Water-Soluble Organic Solvents by Adding Inorganic Salts. Microchim Acta 157 (1-2), 73–79. doi:10.1007/s00604-006-0625-7
Cartwright, C. D., Owen, S. A., Thompson, I. P., and Burns, R. G. (2000). Biodegradation of Diethyl Phthalate in Soil by a Novel Pathway. FEMS Microbiol. Lett. 186, 27–34. doi:10.1111/j.1574-6968.2000.tb09077.x
Cequier, E., Sakhi, A. K., Marcé, R. M., Becher, G., and Thomsen, C. (2015). Human Exposure Pathways to Organophosphate Triesters - A Biomonitoring Study of Mother-Child Pairs. Environ. Int. 75, 159–165. doi:10.1016/j.envint.2014.11.009
Chakraborty, P., Sampath, S., Mukhopadhyay, M., Selvaraj, S., Bharat, G. K., and Nizzetto, L. (2019). Baseline Investigation on Plasticizers, Bisphenol A, Polycyclic Aromatic Hydrocarbons and Heavy Metals in the Surface Soil of the Informal Electronic Waste Recycling Workshops and Nearby Open Dumpsites in Indian Metropolitan Cities. Environ. Pollut. 248, 1036–1045. doi:10.1016/j.envpol.2018.11.010
Chang, B. V., Yang, C. M., Cheng, C. H., and Yuan, S. Y. (2004). Biodegradation of Phthalate Esters by Two Bacteria Strains. Chemosphere 55, 533–538. doi:10.1016/j.chemosphere.2003.11.057
Chen, C.-F., Chen, C.-W., Ju, Y.-R., and Dong, C.-D. (2017). Determination and Assessment of Phthalate Esters Content in Sediments from Kaohsiung Harbor, Taiwan. Mar. Pollut. Bull. 124, 767–774. doi:10.1016/j.marpolbul.2016.11.064
Chen, C.-Y., Chou, Y.-Y., Lin, S.-J., and Lee, C.-C. (2015). Developing an Intervention Strategy to Reduce Phthalate Exposure in Taiwanese Girls. Sci. Total Environ. 517, 125–131. doi:10.1016/j.scitotenv.2015.02.021
Chen, Y., Jiang, L., Lu, S., Kang, L., Luo, X., Liu, G., et al. (2019). Organophosphate Ester and Phthalate Ester Metabolites in Urine from Primiparas in Shenzhen, China: Implications for Health Risks. Environ. Pollut. 247, 944–952. doi:10.1016/j.envpol.2019.01.107
Chen, Y., Lv, D., Li, X., and Zhu, T. (2018). PM2.5-bound Phthalates in Indoor and Outdoor Air in Beijing: Seasonal Distributions and Human Exposure via Inhalation. Environ. Pollut. 241, 369–377. doi:10.1016/j.envpol.2018.05.081
Cheng, Z., Liu, J.-B., Gao, M., Shi, G.-Z., Fu, X.-J., Cai, P., et al. (2019). Occurrence and Distribution of Phthalate Esters in Freshwater Aquaculture Fish Ponds in Pearl River Delta, China. Environ. Pollut. 245, 883–888. doi:10.1016/j.envpol.2018.11.085
Chi, J., and Gao, J. (2015). Effects of Potamogeton Crispus L.-bacteria Interactions on the Removal of Phthalate Acid Esters from Surface Water. Chemosphere 119, 59–64. doi:10.1016/j.chemosphere.2014.05.058
Chiellini, F., Ferri, M., Morelli, A., Dipaola, L., and Latini, G. (2013). Perspectives on Alternatives to Phthalate Plasticized Poly(vinyl Chloride) in Medical Devices Applications. Prog. Polym. Sci. 38, 1067–1088. doi:10.1016/j.progpolymsci.2013.03.001
Cho, S.-C., Bhang, S.-Y., Hong, Y.-C., Shin, M.-S., Kim, B.-N., Kim, J.-W., et al. (2010). Relationship between Environmental Phthalate Exposure and the Intelligence of School-Age Children. Environ. Health Perspect. 118, 1027–1032. doi:10.1289/ehp.0901376
Cobellis, L., Latini, G., De Felice, C., Razzi, S., Paris, I., Ruggieri, F., et al. (2003). High Plasma Concentrations of Di-(2-ethylhexyl)-phthalate in Women with Endometriosis. Hum. Reprod. 18 (7), 1512–1515. doi:10.1093/humrep/deg254
Colón, I., Caro, D., Bourdony, C. J., Rosario, O., and Rossario, O. (2000). Identification of Phthalate Esters in the Serum of Young Puerto Rican Girls with Premature Breast Development. Environ. Health Perspect. 108 (9), 895–900. doi:10.1289/ehp.00108895
Crafford, D., and Avenant-Oldewage, A. (2010). Bioaccumulation of Non-essential Trace Metals in Tissue and Organs of Clarias gariepinus (Sharp Tooth Catfish) from the Vaal River System-Strontium, Aluminium, lead and Nickel. Water SA 36 (5), 621–640. doi:10.4314/wsa.v36i5.61996
Darbre, P. D. (2019). The History of Endocrine-Disrupting Chemicals. Curr. Opin. Endocr. Metab. Res. 7, 26–33. doi:10.1016/j.coemr.2019.06.007
Das, M. T., Kumar, S. S., Ghosh, P., Shah, G., Malyan, S. K., Bajar, S., et al. (2021). Remediation Strategies for Mitigation of Phthalate Pollution: Challenges and Future Perspectives. J. Hazard. Mater. 409, 124496. doi:10.1016/j.jhazmat.2020.124496
De Toni, L., Tisato, F., Seraglia, R., Roverso, M., Gandin, V., Marzano, C., et al. (2017). Phthalates and Heavy Metals as Endocrine Disruptors in Food: A Study on Pre-packed Coffee Products. Toxicol. Rep. 4, 234–239. doi:10.1016/j.toxrep.2017.05.004
Diamanti-Kandarakis, E., Bourguignon, J.-P., Giudice, L. C., Hauser, R., Prins, G. S., Soto, A. M., et al. (2009). Endocrine-disrupting Chemicals: An Endocrine Society Scientific Statement. Endocr. Rev. 30 (4), 293–342. doi:10.1210/er.2009-0002
Dodson, R. E., Nishioka, M., Standley, L. J., Perovich, L. J., Brody, J. G., and Rudel, R. A. (2012). Endocrine Disruptors and Asthma-Associated Chemicals in Consumer Products. Environ. Health Perspect. 120 (7), 935–943. doi:10.1289/ehp.1104052
Dodson, R. E., Van den Eede, N., Covaci, A., Perovich, L. J., Brody, J. G., and Rudel, R. A. (2014). Urinary Biomonitoring of Phosphate Flame Retardants: Levels in California Adults and Recommendations for Future Studies. Environ. Sci. Technol. 48, 13625–13633. doi:10.1021/es503445c
Domínguez-Morueco, N., González-Alonso, S., and Valcárcel, Y. (2014). Phthalate Occurrence in Rivers and Tap Water from central Spain. Sci. Total Environ. 500-501, 139–146. doi:10.1016/j.scitotenv.2014.08.098
Dong, W., Guo, R., Sun, X., Li, H., Zhao, M., Zheng, F., et al. (2019). Assessment of Phthalate Ester Residues and Distribution Patterns in Baijiu Raw Materials and Baijiu. Food Chem. 283, 508–516. doi:10.1016/j.foodchem.2019.01.069
Dutta, S., Haggerty, D. K., Rappolee, D. A., and Ruden, D. M. (2020). Phthalate Exposure and Long-Term Epigenomic Consequences: A Review. Front. Genet. 11, 405. doi:10.3389/fgene.2020.00405
European Commission (2008). European Union Risk Assessment Report Bis(2-Ethylhexyl) Phthalate (DEHP), CAS-No. 117-81-7. 1:80-85. EU R 233 84 EN. Luxembourg: Office for Official Publications of the European Communities.
European Food Safety Authority (2019). Update of the Risk Assessment of Di‐butyl Phthalate (DBP), Butyl‐benzyl‐phthalate (BBP), Bis(2‐ethylhexyl) Phthalate (DEHP), Di‐isononyl Phthalate (DINP) and Di‐isodecyl Phthalate (DIDP) for Use in Food Contact Materials. J. Efsa. 17 (12), 1–85. doi:10.2903/j.efsa.2019.5838
Falenas, F. (2019). Discussion: Automated Filtration or Really Just Solid Phase Extraction. J. Chromatogr. A 1601, 388–389. doi:10.1016/j.chroma.2019.03.052
Fang, C., Chu, Y., Jiang, L., Wang, H., Long, Y., and Shen, D. (2018). Removal of Phthalic Acid Diesters through a Municipal Solid Waste Landfill Leachate Treatment Process. J. Mater. Cycles Waste Manag. 20, 585–591. doi:10.1007/s10163-017-0625-1
Fatoki, O. S., Bornman, M., Ravandhalala, L., Chimuka, L., Genthe, B., and Adeniyi, A. (2010). Phthalate Ester Plasticizers in Freshwater Systems of Venda, South Africa and Potential Health Effects. Wsa 36 (1), 117–126. doi:10.4314/wsa.v36i1.50916
Forget-Leray, J., Landriau, I., Minier, C., and Leboulenger, F. (2005). Impact of Endocrine Toxicants on Survival, Development, and Reproduction of the Estuarine Copepod Eurytemora affinis (Poppe). Ecotoxicology Environ. Saf. 60, 288–294. doi:10.1016/j.ecoenv.2004.06.008
Frederiksen, H., Skakkebaek, N. E., and Andersson, A.-M. (2007). Metabolism of Phthalates in Humans. Mol. Nutr. Food Res. 51, 899–911. doi:10.1002/mnfr.200600243
Gao, D.-W., and Wen, Z.-D. (2016). Phthalate Esters in the Environment: A Critical Review of Their Occurrence, Biodegradation, and Removal during Wastewater Treatment Processes. Sci. Total Environ. 541, 986–1001. doi:10.1016/j.scitotenv.2015.09.148
Gao, D., Li, Z., Wang, H., and Liang, H. (2018). An Overview of Phthalate Acid Ester Pollution in China over the Last Decade: Environmental Occurrence and Human Exposure. Sci. Total Environ. 645, 1400–1409. doi:10.1016/j.scitotenv.2018.07.093
Gao, D., Li, Z., Wen, Z., and Ren, N. (2014). Occurrence and Fate of Phthalate Esters in Full-Scale Domestic Wastewater Treatment Plants and Their Impact on Receiving Waters along the Songhua River in China. Chemosphere 95, 24–32. doi:10.1016/j.chemosphere.2013.08.009
Gao, J., and Chi, J. (2015). Biodegradation of Phthalate Acid Esters by Different marine Microalgal Species. Mar. Pollut. Bull. 99, 70–75. doi:10.1016/j.marpolbul.2015.07.061
Gao, L., Tang, Y., Wang, C., Yao, L., Zhang, J., Gao, R., et al. (2019). Highly-efficient Amphiphilic Magnetic Nanocomposites Based on a Simple Sol-Gel Modification for Adsorption of Phthalate Esters. J. Colloid Interf. Sci. 552, 142–152. doi:10.1016/j.jcis.2019.05.031
Gao, X., Li, J., Wang, X., Zhou, J., Fan, B., Li, W., et al. (2019). Exposure and Ecological Risk of Phthalate Esters in the Taihu Lake basin, China. Ecotoxicology Environ. Saf. 171, 564–570. doi:10.1016/j.ecoenv.2019.01.001
Giuliani, A., Zuccarini, M., Cichelli, A., Khan, H., and Reale, M. (2020). Critical Review on the Presence of Phthalates in Food and Evidence of Their Biological Impact. Ijerph 17 (16), 5655. doi:10.3390/ijerph17165655
Golshan, M., Hatef, A., Socha, M., Milla, S., Butts, I. A. E., Carnevali, O., et al. (2015). Di-(2-ethylhexyl)-phthalate Disrupts Pituitary and Testicular Hormonal Functions to Reduce Sperm Quality in Mature Goldfish. Aquat. Toxicol. 163, 16–26. doi:10.1016/j.aquatox.2015.03.017
Gong, C.-b., Wei, Y.-b., Chen, M.-j., Liu, L.-t., Chow, C.-f., and Tang, Q. (2018). Double Imprinted Photoresponsive Polymer for Simultaneous Detection of Phthalate Esters in Plastics. Eur. Polym. J. 108, 295–303. doi:10.1016/j.eurpolymj.2018.09.009
Gore, A. C., Chappell, V. A., Fenton, S. E., Flaws, J. A., Nadal, A., Prins, G. S., et al. (2015). EDC-2: the Endocrine Society's Second Scientific Statement on Endocrine-Disrupting Chemicals. Endocr. Rev. 36, E1–E150. doi:10.1210/er.2015-1010
Guo, H., Song, N., Wang, D., Ma, J., and Jia, Q. (2019). A Modulation Approach for Covalent Organic Frameworks: Application to Solid Phase Microextraction of Phthalate Esters. Talanta 198, 277–283. doi:10.1016/j.talanta.2019.02.025
Hadjmohammadi, M. R., and Ranbari, E. (2011). Utilization of Homogeneous Liquid–Liquid Extraction Followed by HPLC-UV as a Sensitive Method for the Extraction and Determination of Phthalate Esters in Environmental Water Samples. Int. J. Environ. Anal. Chem. 2 (11), 1312–1324. doi:10.1080/03067319.2011.603049
He, M.-J., Lu, J.-F., Wang, j., Wei, S.-Q., and Hageman, K. J. (2020). Phthalate Esters in Biota, Air and Water in an Agricultural Area of Western China, with Emphasis on Bioaccumulation and Human Exposure. Sci. Total Environ. 698, 134264. doi:10.1016/j.scitotenv.2019.134264
He, W., Qin, N., Kong, X., Liu, W., He, Q., Ouyang, H., et al. (2013). Spatio-temporal Distributions and the Ecological and Health Risks of Phthalate Esters (PAEs) in the Surface Water of a Large, Shallow Chinese lake. Sci. Total Environ. 461-462, 672–680. doi:10.1016/j.scitotenv.2013.05.049
Henkel, C., Hüffer, T., and Hofmann, T. (2019). The Leaching of Phthalates from PVC Can Be Determined with an Infinite Sink Approach. MethodsX 6, 2729–2734. doi:10.1016/j.mex.2019.10.026
Heo, H., Choi, M. J., Park, J., Nam, T., and Cho, J. (2020). Anthropogenic Occurrence of Phthalate Esters in Beach Seawater in the Southeast Coast Region, South Korea. Water J. 12 (122), 1–13.
Herrero, Ó., Planelló, R., and Morcillo, G. (2015). The Plasticizer Benzyl Butyl Phthalate (BBP) Alters the Ecdysone Hormone Pathway, the Cellular Response to Stress, the Energy Metabolism, and Several Detoxication Mechanisms in Chironomus Riparius Larvae. Chemosphere 128, 266–277. doi:10.1016/j.chemosphere.2015.01.059
Hiller-Sturmhöfel, S., and Bartke, A. (1998). The Endocrine System, an Overview. Alcohol. Res. Health 22 (3), 153–164. doi:10.1007/978-3-319-24612-3_300828
Hoffman, K., Stapleton, H. M., Lorenzo, A., Butt, C. M., Adair, L., Herring, A. H., et al. (2018). Prenatal Exposure to Organophosphates and Associations with Birthweight and Gestational Length. Environ. Int. 116, 248–254. doi:10.1016/j.envint.2018.04.016
Howdeshell, K. L., Rider, C. V., Wilson, V. S., and Gray, L. E. (2008). Mechanisms of Action of Phthalate Esters, Individually and in Combination, to Induce Abnormal Reproductive Development in Male Laboratory Rats. Environ. Res. 108 (2), 168–176. doi:10.1016/j.envres.2008.08.009
Huang, P.-C., Tien, C.-J., Sun, Y.-M., Hsieh, C.-Y., and Lee, C.-C. (2008). Occurrence of Phthalates in Sediment and Biota: Relationship to Aquatic Factors and the Biota-Sediment Accumulation Factor. Chemosphere 73, 539–544. doi:10.1016/j.chemosphere.2008.06.019
Huang, P. C., Kuo, P. L., Guo, Y. L., Liao, P. C., and Lee, C. C. (2017). Associations between Urinary Phthalate Monoesters and Thyroid Hormones in Pregnant Women. Hum. Reprod. 22 (10), 2715–2722. doi:10.1093/humrep/dem205
Huang, X., Cui, H., and Duan, W. (2020). Ecotoxicity of Chlorpyrifos to Aquatic Organisms: a Review. Ecotoxicology Environ. Saf. 200, 110731. doi:10.1016/j.ecoenv.2020.110731
Jeddi, M. Z., Janani, L., Memari, A. H., Akhondzadeh, S., and yunesian, M. (2016). The Role of Phthalate Esters in Autism Development: A Systematic Review. Environ. Res. 151, 493–504. doi:10.1016/j.envres.2016.08.021
Ji, Y., Wang, F., Zhang, L., Shan, C., Bai, Z., Sun, Z., et al. (2014). A Comprehensive Assessment of Human Exposure to Phthalates from Environmental media and Food in Tianjin, China. J. Hazard. Mater. 279, 133–140. doi:10.1016/j.jhazmat.2014.06.055
Jiang, M., Li, Y., Zhang, B., Zhou, A., Zhu, Y., Li, J., et al. (2018). Urinary Concentrations of Phthalate Metabolites Associated with Changes in Clinical Hemostatic and Hematologic Parameters in Pregnant Women. Environ. Int. 120, 34–42. doi:10.1016/j.envint.2018.07.021
Jianlong, W., Xuan, Z., and Weizhong, W. (2004). Biodegradation of Phthalic Acid Esters (PAEs) in Soil Bioaugmented with Acclimated Activated Sludge. Process Biochem. 39 (12), 1837–1841. doi:10.1016/j.procbio.2003.08.005
Kashyap, D., and Agarwal, T. (2018). Concentration and Factors Affecting the Distribution of Phthalates in the Air and Dust: A Global Scenario. Sci. Total Environ. 635, 817–827. doi:10.1016/j.scitotenv.2018.04.158
Kim, Y.-M., Kim, J., Cheong, H.-K., Jeon, B.-H., and Ahn, K. (2018). Exposure to Phthalates Aggravates Pulmonary Function and Airway Inflammation in Asthmatic Children. PLoS ONE 13 (12), e0208553. doi:10.1371/journal.pone.0208553
Koniecki, D., Wang, R., Moody, R. P., and Zhu, J. (2011). Phthalates in Cosmetic and Personal Care Products: Concentrations and Possible Dermal Exposure. Environ. Res. 111 (3), 329–336. doi:10.1016/j.envres.2011.01.013
Krimsky, S. (2001). An Epistemological Inquiry into the Endocrine Disruptor Thesis. Ann. N. Y. Acad. Sci. 948, 130–141. doi:10.1111/j.1749-6632.2001.tb03994.x
Latini, G. (2005). Monitoring Phthalate Exposure in Humans. Clinica Chim. Acta 361, 20–29. doi:10.1016/j.cccn.2005.05.003
Lee, H., Lee, J., Choi, K., and Kim, K.-T. (2019). Comparative Analysis of Endocrine Disrupting Effects of Major Phthalates in Employed Two Cell Lines (MVLN and H295R) and Embryonic Zebrafish Assay. Environ. Res. 172, 319–325. doi:10.1016/j.envres.2019.02.033
Lee, Y.-M., Lee, J.-E., Choe, W., Kim, T., Lee, J.-Y., Kho, Y., et al. (2019). Distribution of Phthalate Esters in Air, Water, Sediments, and Fish in the Asan Lake of Korea. Environ. Int. 126, 635–643. doi:10.1016/j.envint.2019.02.059
Li, J., Wang, Z., Wang, Q., Guo, L., Wang, C., Wang, Z., et al. (2021). Construction of Hypercrosslinked Polymers for High-Performance Solid Phase Microextraction of Phthalate Esters from Water Samples. J. Chromatogr. A 1641, 461972. doi:10.1016/j.chroma.2021.461972
Li, R., Liang, J., Gong, Z., Zhang, N., and Duan, H. (2017). Occurrence, Spatial Distribution, Historical Trend and Ecological Risk of Phthalate Esters in the Jiulong River, Southeast China. Sci. Total Environ. 580, 388–397. doi:10.1016/j.scitotenv.2016.11.190
Li, Y., Gao, J., Meng, F., and Chi, J. (2015). Enhanced Biodegradation of Phthalate Acid Esters in marine Sediments by Benthic Diatom Cylindrotheca Closterium. Sci. Total Environ. 508, 251–257. doi:10.1016/j.scitotenv.2014.12.002
Li, Y. Y., He, W., Liu, W. X., Yang, B., He, Q. S., Yang, C., et al. (2020). Impacts of Anthropogenic Activities on Spatial Variations of Phthalate Esters in Water and Suspended Particulate Matter from China's Lakes. Sci. Total Environ. 724, 1–11. doi:10.1016/j.scitotenv.2020.138281
Lin, L.-Y., Tsai, M.-S., Chen, M.-H., Ng, S., Hsieh, C.-J., Lin, C.-C., et al. (2018). Childhood Exposure to Phthalates and Pulmonary Function. Sci. Total Environ. 615, 1282–1289. doi:10.1016/j.scitotenv.2017.08.318
Ling, W., Gui-Bin, C., Ya-Qi, H., Bin, Y., Wei, W., and Da-Zhong, S. (2007). Cloud point Extraction Coupled with HPLC-UV for the Determination of Phthalate Esters in Environmental Water Samples. J. Environ. Sci. 19, 874–878. doi:10.1016/S1001-0742(07)60145-4
Liu, H., Cui, K., Zeng, F., Chen, L., Cheng, Y., Li, H., et al. (2014). Occurrence and Distribution of Phthalate Esters in Riverine Sediments from the Pearl River Delta Region, South China. Mar. Pollut. Bull. 83 (1), 358–365. doi:10.1016/j.marpolbul.2014.03.038
Liu, X., Shi, j., Bo, T., Li, H., and Crittenden, J. C. (2015). Occurrence and Risk Assessment of Selected Phthalates in Drinking Water from Waterworks in China. Environ. Sci. Pollut. Res. 22, 10690–10698. doi:10.1007/s11356-015-4253-9
Liu, Y., Wang, S., and Wang, L. (2013). Development of Rapid Determination of 18 Phthalate Esters in Edible Vegetable Oils by Gas Chromatography Tandem Mass Spectrometry. J. Agric. Food Chem. 61 (6), 1160–1164. doi:10.1021/jf3053884
López-Carrillo, L., Hernández-Ramírez, R. U., Calafat, A. M., Torres-Sánchez, L., Galván-Portillo, M., Needham, L. L., et al. (2010). Exposure to Phthalates and Breast Cancer Risk in Northern Mexico. Environ. Health Perspect. 118, 539–544. doi:10.1289/ehp.0901091
Luo, Q., Liu, Z.-h., Yin, H., Dang, Z., Wu, P.-x., Zhu, N.-w., et al. (2018). Migration and Potential Risk of Trace Phthalates in Bottled Water: A Global Situation. Water Res. 147, 362–372. doi:10.1016/j.watres.2018.10.002
Ma, T. T., Christie, P., Luo, Y. M., and Teng, Y. (2013). Phthalate Esters Contamination in Soil and Plants on Agricultural Land Near an Electronic Waste Recycling Site. Environ. Geochem. Health 35, 465–476. doi:10.1007/s10653-012-9508-5
Malarvannan, G., Onghena, M., Verstraete, S., van Puffelen, E., Jacobs, A., Vanhorebeek, I., et al. (2019). Phthalate and Alternative Plasticizers in Indwelling Medical Devices in Pediatric Intensive Care Units. J. Hazard. Mater. 363, 64–72. doi:10.1016/j.jhazmat.2018.09.087
Mankidy, R., Wiseman, S., Ma, H., and Giesy, J. P. (2013). Biological Impact of Phthalates. Toxicol. Lett. 217 (1), 50–58. doi:10.1016/j.toxlet.2012.11.025
Marty, M. S., Carney, E. W., and Rowlands, J. C. (2011). Endocrine Disruption: Historical Perspectives and its Impact on the Future of Toxicology Testing. Toxicol. Sci. 120 (1), S93–S108. doi:10.1093/toxsci/kfq329
Mehdinia, A., and Aziz-Zanjani, M. O. (2013). Advances for Sensitive, Rapid and Selective Extraction in Different Configurations of Solid-phase Microextraction. Trac Trends Anal. Chem. 51, 13–22. doi:10.1016/j.trac.2013.05.013
Mi, L., Xie, Z., Zhao, Z., Zhong, M., Mi, W., Ebinghaus, R., et al. (2019). Occurrence and Spatial Distribution of Phthalate Esters in Sediments of the Bohai and Yellow Seas. Sci. Total Environ. 653, 792–800. doi:10.1016/j.scitotenv.2018.10.438
Mu, D., Gao, F., Fan, Z., Shen, H., Peng, H., and Hu, J. (2015). Levels of Phthalate Metabolites in Urine of Pregnant Women and Risk of Clinical Pregnancy Loss. Environ. Sci. Technol. 49, 10651–10657. doi:10.1021/acs.est.5b02617
Nantaba, F., Palm, W.-U., Wasswa, J., Bouwman, H., Kylin, H., and Kümmerer, K. (2021). Temporal Dynamics and Ecotoxicological Risk Assessment of Personal Care Products, Phthalate Ester Plasticizers, and Organophosphorus Flame Retardants in Water from Lake Victoria, Uganda. Chemosphere 262, 127716. doi:10.1016/j.chemosphere.2020.127716
Navaranjan, G., Takaro, T. K., Wheeler, A. J., Diamond, M. L., Shu, H., Azad, M. B., et al. (2019). Early Life Exposure to Phthalates in the Canadian Healthy Infant Longitudinal Development (CHILD) Study: a Multi-City Birth Cohort. J. Expo. Sci. Environ. Epidemiol. 30 (1), 70–85. doi:10.1038/s41370-019-0182-x
Net, S., Delmont, A., Sempéré, R., Paluselli, A., and Ouddane, B. (2015b). Reliable Quantification of Phthalates in Environmental Matrices (Air, Water, Sludge, Sediment and Soil): A Review. Sci. Total Environ. 515-516, 162–180. doi:10.1016/j.scitotenv.2015.02.013
Net, S., Dumoulin, D., El-Osmani, R., Rabodonirina, S., and Ouddane, B. (2014). Case Study of PAHs, Me-PAHs, PCBs, Phthalates and Pesticides Contamination in the Somme River Water. France Int. J. Environ. Res. 8, 1159–1170. doi:10.22059/IJER.2014.809
Net, S., Sempéré, R., Delmont, A., Paluselli, A., and Ouddane, B. (2015a). Occurrence, Fate, Behavior and Ecotoxicological State of Phthalates in Different Environmental Matrices. Environ. Sci. Technol. 49 (7), 4019–4035. doi:10.1021/es505233b
NICNAS (2008). Phthalates hazard Compendium; a Summary of Physiochemical and Human Helath hazard Data for Diisodecyl Phthalate. Sydney: National Industrial Chemicals Notification and Assessment Scheme.
Oehlmann, J., Schulte-Oehlmann, U., Kloas, W., Jagnytsch, O., Lutz, I., Kusk, K. O., et al. (2009). A Critical Analysis of the Biological Impacts of Plasticizers on Wildlife. Phil. Trans. R. Soc. B 364 (1526), 2047–2062. doi:10.1098/rstb.2008.0242
Olijimi, O. O., Fatoki, O. S., Odendaal, J. P., and Daso, A. P. (2012). Chemical Monitoring and Temporal Variation in Levels of Endocrine Disrupting Chemicals (Priority Phenols and Phthalate Esters) from Selected Wastewater Treatment Plant and Freshwater Systems in Republic of South Africa. Microchem. J. 101, 11–23.
Olkowska, E., Ratajczyk, J., and Wolska, L. (2017). Determination of Phthalate Esters in Air with thermal Desorption Technique - Advantages and Disadvantages. Trac Trends Anal. Chem. 91, 77–90. doi:10.1016/j.trac.2017.04.002
Olujimi, O. O., Fatoki, O. S., Ondendaal, J. P., and Okonkwo, J. O. (2010). Endocrine Disrupting Chemicals (Phenol and Phthalates) in the South African Environment: a Need for More Monitoring. Water SA 36 (5), 671–682. doi:10.4314/wsa.v36i5.62001
Paluselli, A., Aminot, Y., Galgani, F., Net, S., and Sempéré, R. (2018). Occurrence of Phthalate Acid Esters (PAEs) in the Northwestern Mediterranean Sea and the Rhone River. Prog. Oceanography 163, 221–231. doi:10.1016/j.pocean.2017.06.002
Pang, Y.-H., Yue, Q., Huang, Y.-y., Yang, C., and Shen, X.-F. (2020). Facile Magnetization of Covalent Organic Framework for Solid-phase Extraction of 15 Phthalate Esters in Beverage Samples. Talanta 206, 120194. doi:10.1016/j.talanta.2019.120194
Philippat, C., Mortamais, M., Chevrier, C., Petit, C., Calafat, A. M., Ye, X., et al. (2012). Exposure to Phthalates and Phenols during Pregnancy and Offspring Size at Birth. Environ. Health Perspect. 120, 464–470. doi:10.1289/ehp.1103634
Qureshi, M. S., Yusoff, A. R. b. M., Wirzal, M. D. H., Barek, J., Afridi, H. I., Üstündag, Z., et al. (2016). Methods for the Determination of Endocrine-Disrupting Phthalate Esters. Crit. Rev. Anal. Chem. 46 (2), 146–159. doi:10.1080/10408347.2015.1004157
Reddy, B., Rozati, R., Reddy, B., and Raman, N. (2006). General Gynaecology: Association of Phthalate Esters with Endometriosis in Indian Women. Int. J. Gynecol. Obstet. 113, 515–520. doi:10.1111/j.1471-0528.2006.00925.x
Rehman, S., Usman, Z., Rehman, S., AlDraihem, M., Rehman, N., Rehman, I., et al. (2018). Endocrine Disrupting Chemicals and Impact on Male Reproductive Health. Transl. Androl. Urol. 7 (3), 490–503. doi:10.21037/tau.2018.05.17
Ren, L., Lin, Z., Liu, H., and Hu, H. (2018). Bacteria-mediated Phthalic Acid Esters Degradation and Related Molecular Mechanisms. Appl. Microbiol. Biotechnol. 102, 1085–1096. doi:10.1007/s00253-017-8687-5
Rudel, R. A., and Perovich, L. J. (2009). Endocrine Disrupting Chemicals in Indoor and Outdoor Air. Atmos. Environ. 43 (1), 170–181. doi:10.1016/j.atmosenv.2008.09.025
Salaudeen, T., Okoh, O., Agunbiade, F., and Okoh, A. (2018). Fate and Impact of Phthalates in Activated Sludge Treated Municipal Wastewater on the Water Bodies in the Eastern Cape, South Africa. Chemosphere 203, 336–344. doi:10.1016/j.chemosphere.2018.03.176
Santana-Mayor, Á., Socas-Rodríguez, B., Afonso, M. d. M., Palenzuela-López, J. A., and Rodríguez-Delgado, M. Á. (2018). Reduced Graphene Oxide-Coated Magnetic-Nanoparticles as Sorbent for the Determination of Phthalates in Environmental Samples by Micro-dispersive Solid-phase Extraction Followed by Ultra-high-performance Liquid Chromatography Tandem Mass Spectrometry. J. Chromatogr. A 1565, 36–47. doi:10.1016/j.chroma.2018.06.031
Santhi, V. A., and Mustafa, A. M. (2013). Assessment of Organochlorine Pesticides and Plasticisers in the Selangor River basin and Possible Pollution Sources. Environ. Monit. Assess. 185, 1541–1554. doi:10.1007/s10661-012-2649-2
Sathyanarayana, S., Barrett, E., Butts, S., Wang, C., and Swan, S. H. (2014). Phthalate Exposure and Reproductive Hormone Concentrations in Pregnancy. Hum. Reprod. 147, 401–409. doi:10.1530/rep-13-0415
Selvaraj, K. K., Sundaramoorthy, G., Ravichandran, P. K., Girijan, G. K., Sampath, S., and Ramaswamy, B. R. (2015). Phthalate Esters in Water and Sediments of the Kaveri River, India: Environmental Levels and Ecotoxicological Evaluations. Environ. Geochem. Health 37, 83–96. doi:10.1007/s10653-014-9632-5
Seyoum, A., and Pradhan, A. (2019). Effect of Phthalates on Development, Reproduction, Fat Metabolism and Lifespan in Daphnia magna. Sci. Total Environ. 654, 969–977. doi:10.1016/j.scitotenv.2018.11.158
Shin, H.-M., Dhar, U., Calafat, A. M., Nguyen, V., Schmidt, R. J., and Hertz-Picciotto, I. (2020). Temporal Trends of Exposure to Phthalates and Phthalate Alternatives in California Pregnant Women during 2007-2013: Comparison with Other Populations. Environ. Sci. Technol. 54 (20), 13157–13166. doi:10.1021/acs.est.0c03857
Sibali, L. L. (2008). Determination of Endocrine Disrupting Compounds in Water and Sediments from Jukskei River Catchment Area. South Africa: Tshwane University of Technology. [PhD thesis].
Sibali, L. L., Okonkwo, J. O., and McCrindle, R. I. (2013). Determination of Selected Phthalate Esters Compounds in Water and Sediments by Capillary Gas Chromatography and Flame Ionization Detector. J. Environ. Sci. Health A 48, 1365–1377. doi:10.1080/10934529.2013.781884
Sohn, J., Kim, S., Koschorreck, J., Kho, Y., and Choi, K. (2016). Alteration of Sex Hormone Levels and Steroidogenic Pathway by Several Low Molecular Weight Phthalates and Their Metabolites in Male Zebrafish (Danio rerio) And/or Human Adrenal Cell (H295R) Line. J. Hazard. Mater. 320, 45–54. doi:10.1016/j.jhazmat.2016.08.008
Staples, C. A., Adams, W. J., Parkerton, T. F., Gorsuch, J. W., Biddinger, G. R., and Reinert, K. H. (1997b). Aquatic Toxicity of Eighteen Phthalate Esters. Environ. Toxicol. Chem. 16 (5), 875–891. doi:10.1002/etc.5620160507
Staples, C. A., Peterson, D. R., Parkerton, T. F., and Adams, W. J. (1997a). The Environmental Fate of Phthalate Esters: a Literature Review. Chemosphere 35, 667–749. doi:10.1016/s0045-6535(97)00195-1
Stojanoska, M. M., Milosevic, N., Milic, N., and Abenavoli, L. (2017). The Influence of Phthalates and Bisphenol A on the Obesity Development and Glucose Metabolism Disorders. Endocrine 55, 666–681. doi:10.1007/s12020-016-1158-4
Sun, J., Huang, J., Zhang, A., Liu, W., and Cheng, W. (2013). Occurrence of Phthalate Esters in Sediments in Qiantang River, China and Inference with Urbanization and River Flow Regime. J. Hazard. Mater. 248-249, 142–149. doi:10.1016/j.jhazmat.2012.12.057
Sun, J., Wu, X., and Gan, J. (2015). Uptake and Metabolism of Phthalate Esters by Edible Plants. Environ. Sci. Technol. 49, 8471–8478. doi:10.1021/acs.est.5b01233
Svensson, K., Hernández-Ramírez, R. U., Burguete-García, A., Cebrián, M. E., Calafat, A. M., Needham, L. L., et al. (2011). Phthalate Exposure Associated with Self-Reported Diabetes Among Mexican Women. Environ. Res. 111, 792–796. doi:10.1016/j.envres.2011.05.015
Swan, S. H., Main, K. M., Liu, F., Stewart, S. L., Kruse, R. L., Calafat, A. M., et al. (2005). Decrease in Anogenital Distance Among Male Infants with Prenatal Phthalate Exposure. Environ. Health Perspect. 113 (8), 1056–1061. doi:10.1289/ehp.8100
Talia, C., Connolly, L., and Fowler, P. A. (2021). The Insulin-like Growth Factor System: A Target for Endocrine Disruptors?. Environ. Int. 147, 106311. doi:10.1016/j.envint.2020.106311
Téllez-Rojo, M. M., Cantoral, A., Cantonwine, D. E., Schnaas, L., Peterson, K., Hu, H., et al. (2013). Prenatal Urinary Phthalate Metabolites Levels and Neurodevelopment in Children at Two and Three Years of Age. Sci. Total Environ. 461-462, 386–390. doi:10.1016/j.scitotenv.2013.05.021
Tilson, H. A. (2008). EHP Papers of the Year, 2008. Environ. Health Perspect. 116 (6), 234. doi:10.1289/ehp.11684
Tong, Y., Liu, X., and Zhang, L. (2019). Green Construction of Fe3O4@GC Submicrocubes for Highly Sensitive Magnetic Dispersive Solid-phase Extraction of Five Phthalate Esters in Beverages and Plastic Bottles. Food Chem. 277, 579–585. doi:10.1016/j.foodchem.2018.11.021
Toppari, J., Virtanen, H., Skakkebaek, N. E., and Main, K. M. (2006). Environmental Effects on Hormonal Regulation of Testicular Descent. J. Steroid Biochem. Mol. Biol. 102 (1-5), 184–186. doi:10.1016/j.jsbmb.2006.09.020
UNEP (2020). An Assessment Report on Issues of Concern: Chemicals and Waste Issues Posing Risks to Human Health and the Environment. Available at: https://wedocs.unep.org/bitstream/handle/20.500.11822/33807/ARIC.pdf?sequence=1&isAllowed=y (Accessed May 19, 2021).
US National Academies of Sciences Engineering and Medicine (2017). Application of Systematic Review Methods in an Overall Strategy for Evaluating Low-Dose Toxicity from Endocrine Active Chemicals. Washington, DC: The National Academies Press.
USCDC (2018). National Health and Nutrition Examination Survey 2015-2016 Data Documentation, Codebook, and Frequencies. Phthalates and Plasticizers Metabolites - Urine (PHTHTE_I). Available at: https://wwwn.cdc.gov/Nchs/Nhanes/2015-2016/PHTHTE_I.htm (Accessed May 21, 2021).
USEPA (2018). America’s Children and the Environment. Appendix A: Data Tables – Biomonitoring – Phthalates. Available at: https://www.epa.gov/americaschildrenenvironment/data-tables-biomonitoring-phthalates (Accessed May 21, 2021).
USEPA (2021). Bis (2-ethylhexyl) Phthalate (DEHP). Available at: https://www.epa.gov/sites/production/files/2016-09/documents/bis-2-ethylhexyl-phthalate.pdf (Accessed March 11, 2021).
Vats, S., Singh, R. K., and Tyagi, P. (2013). Phthalates - A Priority Pollutant. Int. J. Adv. Biol. 3 (1), 1–8.
Wang, B., Wang, H., Zhou, W., Chen, Y., Zhou, Y., and Jiang, Q. (2015a). Urinary Excretion of Phthalate Metabolites in School Children of China: Implication for Cumulative Risk Assessment of Phthalate Exposure. Environ. Sci. Technol. 49, 1120–1129. doi:10.1021/es504455a
Wang, H., Liang, H., and Gao, D. (2017). Occurrence and Distribution of Phthalate Esters (PAEs) in Wetland Sediments. J. For. Res. 28 (6), 1241–1248. doi:10.1007/s11676-017-0371-1
Wang, I.-J., Lin, C.-C., Lin, Y.-J., Hsieh, W.-S., and Chen, P.-C. (2014). Early Life Phthalate Exposure and Atopic Disorders in Children: a Prospective Birth Cohort Study. Environ. Int. 62, 48–54. doi:10.1016/j.envint.2013.09.002
Wang, J., Bo, L., Li, L., Wang, D., Chen, G., Christie, P., et al. (2014). Occurrence of Phthalate Esters in River Sediments in Areas with Different Land Use Patterns. Sci. Total Environ. 500-501, 113–119. doi:10.1016/j.scitotenv.2014.08.092
Wang, R., Ma, X., Zhang, X., Li, X., Li, D., and Dang, Y. (2019c). C8-modified Magnetic Graphene Oxide Based Solid-phase Extraction Coupled with Dispersive Liquid-Liquid Microextraction for Detection of Trace Phthalate Acid Esters in Water Samples. Ecotoxicology Environ. Saf. 170, 789–795. doi:10.1016/j.ecoenv.2018.12.051
Wang, W., Leung, A. O. W., Chu, L. H., and Wong, M. H. (2018). Phthalates Contamination in China: Status, Trends and Human Exposure-With an Emphasis on Oral Intake. Environ. Pollut. 238, 771–782. doi:10.1016/j.envpol.2018.02.088
Wang, X., Feng, J., Tian, Y., Li, C., Ji, X., Luo, C., et al. (2019). Melamine-formaldehyde Aerogel Functionalized with Polydopamine as In-Tube Solid-phase Microextraction Coating for the Determination of Phthalate Esters. Talanta 199, 317–323. doi:10.1016/j.talanta.2019.02.081
Wang, X., Song, M., Guo, M., Chi, C., Mo, F., and Shen, X. (2015b). Pollution Levels and Characteristics of Phthalate Esters in Indoor Air in Hospitals. J. Environ. Sci. 37, 67–74. doi:10.1016/j.jes.2015.02.016
Wang, Y., Zhu, H., and Kannan, K. (2019). A Review of Biomonitoring of Phthalate Exposures. Toxics 7 (2), 21. doi:10.3390/toxics7020021
Weizhen, Z., Xiaowei, Z., Peng, G., Ning, W., Zini, L., Jian, H., et al. (2020). Distribution and Risk Assessment of Phthalates in Water and Sediment of the Pearl River Delta. Environ. Sci. Pollut. Res. 27, 12550–12565. doi:10.1007/s11356-019-06819-y
Wen, Z., Huang, X., Gao, D., Liu, G., Fang, C., Shang, Y., et al. (2018). Phthalate Esters in Surface Water of Songhua River Watershed Associated with Land Use Types, Northeast China. Environ. Sci. Pollut. Res. 25, 7688–7698. doi:10.1007/s11356-017-1119-3
WHO (2021). Food Safety and Zoononess, Areas of Work Chemical Risks. Available at: https://www.who.int/foodsafety/areas_work/chemical-risks/pops/en/(Accessed March 10, 2021).
WHO/UNEP (2013). An Assessment of the State of the Science of Endocrine Disruptors Prepared by a Group of Experts for the. Switzerland: WHO Press.
Xie, F., Chen, X., Weng, S., Xia, T., Sun, X., Luo, T., et al. (2019). Effects of Two Environmental Endocrine Disruptors Di-n-butyl Phthalate (DBP) and Mono-N-Butyl Phthalate (MBP) on Human Sperm Functions In Vitro. Reprod. Toxicol. 83, 1–7. doi:10.1016/j.reprotox.2018.10.011
Xu, T., and Yin, D. (2019). The Unlocking Neurobehavioral Effects of Environmental Endocrine-Disrupting Chemicals. Curr. Opin. Endocr. Metab. Res. 7, 9–13. doi:10.1016/j.coemr.2019.04.010
Xu, Y., Minhazul, K. A. H. M., Wang, X., Liu, X., Li, X., Meng, Q., et al. (2020). Biodegradation of Phthalate Esters by Paracoccus Kondratievae BJQ0001 Isolated from Jiuqu (Baijiu Fermentation Starter) and Identification of the Ester Bond Hydrolysis Enzyme. Environ. Pollut. 263, 114506. doi:10.1016/j.envpol.2020.114506
Yang, J., Li, Y., Wang, Y., Ruan, J., Zhang, J., and Sun, C. (2015). Recent Advances in Analysis of Phthalate Esters in Foods. Trac Trends Anal. Chem. 72, 10–26. doi:10.1016/j.trac.2015.03.018
Ye, T., Kang, M., Huang, Q., Fang, C., Chen, Y., Shen, H., et al. (2014). Exposure to DEHP and MEHP from Hatching to Adulthood Causes Reproductive Dysfunction and Endocrine Disruption in marine Medaka (Oryzias Melastigma). Aquat. Toxicol. 146, 115–126. doi:10.1016/j.aquatox.2013.10.025
You, H. H., and Song, G. (2021). Review of Endocrine Disruptors on Male and Female Reproductive Systems. Comp. Biochem. Physiol. C: Toxicol. Pharmacol. 244, 109002. doi:10.1016/j.cbpc.2021.109002
Yu, H., Wang, L., Lin, Y., Liu, W., Tuyiringire, D., Jiao, Y., et al. (2020). Complete Metabolic Study by Dibutyl Phthalate Degrading Pseudomonas Sp. DNB-S1. Ecotoxicology Environ. Saf. 194, 110378. doi:10.1016/j.ecoenv.2020.110378
Zacharia, J. T. (2019). Degradation Pathways of Persistent Organic Pollutants (POPs) in the Environment. Available at: https://www.intechopen.com/books/persistent-organic-pollutants/degradation-pathways-of-persistent-organic-pollutants-pops-in-the-environment (Accessed March 19, 2021).
Zarean, M., Keikha, M., Poursafa, P., Khalighinejad, P., Amin, M., and Kelishadi, R. (2016). A Systematic Review on the Adverse Health Effects of Di-2-ethylhexyl Phthalate. Environ. Sci. Pollut. Res. 23, 24642–24693. doi:10.1007/s11356-016-7648-3
Zhang, B., Zhang, T., Duan, Y., Zhao, Z., Huang, X., Bai, X., et al. (2019). Human Exposure to Phthalate Esters Associated with E-Waste Dismantling: Exposure Levels, Sources, and Risk Assessment. Environ. Int. 124, 1–9. doi:10.1016/j.envint.2018.12.035
Zhang, J., Zhang, C., Zhu, Y., Li, J., and Li, X. (2018a). Biodegradation of Seven Phthalate Esters by Bacillus Mojavensis B1811. Int. Biodeterioration Biodegradation 132, 200–207. doi:10.1016/j.ibiod.2018.04.006
Zhang, Y., Jiao, Y., Li, Z., Tao, Y., and Yang, Y. (2021). Hazards of Phthalates (PAEs) Exposure: A Review of Aquatic Animal Toxicology Studies. Sci. Total Environ. 771, 145418. doi:10.1016/j.scitotenv.2021.145418
Zhang, Z.-M., Zhang, H.-H., Li, J.-L., and Yang, G.-P. (2017). Determination of Phthalic Acid Esters in Seawater and Sediment by Solid-phase Microextraction and Gas Chromatography-Mass Spectrometry. Chin. J. Anal. Chem. 45 (3), 348–356. doi:10.1016/s1872-2040(17)60999-x
Zhang, Z.-M., Zhang, H.-H., Zhang, J., Wang, Q.-W., and Yang, G.-P. (2018b). Occurrence, Distribution, and Ecological Risks of Phthalate Esters in the Seawater and Sediment of Changjiang River Estuary and its Adjacent Area. Sci. Total Environ. 619-620, 93–102. doi:10.1016/j.scitotenv.2017.11.070
Zhang, Z.-M., Zhang, H.-H., Zou, Y.-W., and Yang, G.-P. (2018c). Distribution and Ecotoxicological State of Phthalate Esters in the Sea-Surface Microlayer, Seawater and Sediment of the Bohai Sea and the Yellow Sea. Environ. Pollut. 240, 235–247. doi:10.1016/j.envpol.2018.04.056
Zheng, H., Ma, J., Zhu, C., Zhang, Z., Liu, L., Sun, Y., et al. (2014). Synthesis of Anion Polyacrylamide under UV Initiation and its Application in Removing Dioctyl Phthalate from Water through Flocculation Process. Sep. Purif. Tech. 123, 35–44. doi:10.1016/j.seppur.2013.12.018
Zheng, X., Yan, Z., Liu, P., Li, H., Zhou, J., Wang, Y., et al. (2019). Derivation of Aquatic Life Criteria for Four Phthalate Esters and Their Ecological Risk Assessment in Liao River. Chemosphere 220, 802–810. doi:10.1016/j.chemosphere.2018.12.047
Zhou, J., Chen, B., and Cai, Z. (2015). Metabolomics-based Approach for Assessing the Toxicity Mechanisms of Dibutyl Phthalate to Abalone (Haliotis Diversicolor Supertexta). Environ. Sci. Pollut. Res. 22, 5092–5099. doi:10.1007/s11356-014-3859-7
Zhu, M., Wu, J., Ma, X., Huang, C., Wu, R., Zhu, W., et al. (2019). Butyl Benzyl Phthalate Promotes Prostate Cancer Cell Proliferation through miR-34a Downregulation. Toxicol. Vitro 54, 82–88. doi:10.1016/j.tiv.2018.09.007
Zhu, Q., Jia, J., Zhang, K., Zhang, H., and Liao, C. (2019). Spatial Distribution and Mass Loading of Phthalate Esters in Wastewater Treatment Plants in China: An Assessment of Human Exposure. Sci. Total Environ. 656, 862–869. doi:10.1016/j.scitotenv.2018.11.458
Keywords: aquatic ecosystem, aquatic organisms, carcinogenic, endocrine disruptor, health risks, phthalate esters, phthalate esters toxicity
Citation: Baloyi ND, Tekere M, Maphangwa KW and Masindi V (2021) Insights Into the Prevalence and Impacts of Phthalate Esters in Aquatic Ecosystems. Front. Environ. Sci. 9:684190. doi: 10.3389/fenvs.2021.684190
Received: 22 March 2021; Accepted: 28 June 2021;
Published: 15 July 2021.
Edited by:
Sabrina Saponaro, Politecnico di Milano, ItalyReviewed by:
Roberta Guerra, University of Bologna, ItalyPengfei W. U, Hong Kong Baptist University, China
Copyright © 2021 Baloyi, Tekere, Maphangwa and Masindi. This is an open-access article distributed under the terms of the Creative Commons Attribution License (CC BY). The use, distribution or reproduction in other forums is permitted, provided the original author(s) and the copyright owner(s) are credited and that the original publication in this journal is cited, in accordance with accepted academic practice. No use, distribution or reproduction is permitted which does not comply with these terms.
*Correspondence: M. Tekere, dGVrZXJtQHVuaXNhLmFjLnph