- 1Department of Ecology and Evolutionary Biology, University of Toronto, Toronto, ON, Canada
- 2Ocean Conservancy, Washington, DC, United States
- 3Centre for Marinelife Conservation and Community Development, Hanoi, Vietnam
Vietnamese rivers are among the top ten contributors of anthropogenic debris to the ocean. However, there is limited empirical research documenting debris and its effects in Northern Vietnam. The goal of our research was to conduct the first baseline assessment of anthropogenic debris in the Red River. We aimed to understand the sources, accumulation patterns, and ecological effects of anthropogenic debris in the Red River (Song Hong) estuary. To assess debris patterns, we conducted standing stock debris surveys at sites in the mouth, and upstream of the Red River. To assess the ecological effects of anthropogenic debris on mangrove ecosystems, we measured mangrove diameter, canopy cover, and number of crab burrows/m2 in the same debris transects. Anthropogenic debris was found at all sites, and plastic was the most common material. We identified a non-significant trend, whereby ecological indices declined with increasing amounts of debris. Overall, our results demonstrate that anthropogenic debris is ubiquitous in the Red River estuary, composition varies among sites, and this debris may have adverse or neutral ecological effects on mangrove ecosystem health. Future work should conduct debris assessments at larger spatial scales, and assess ecological responses at the community or population level over extended time periods.
Introduction
Anthropogenic debris, defined as any man-made item produced by people that is discarded or abandoned in the environment (Coe and Rogers, 1997), has been documented in marine environments around the world for decades (Bergmann et al., 2015; Seeruttun et al., 2021). It is now so ubiquitous that it is found in remote ecosystems, including gyres in the middle of the oceans (Law et al., 2014; Pham et al., 2014; Lebreton et al., 2018; Eriksen et al., 2019), beaches of remote islands (Gregory, 1978), the Arctic and Antarctic (Bergmann et al., 2017), and the deep sea (Chiba et al., 2018). Marine debris has also been found smothering, entangling, or in the stomachs of flora and fauna (Gall and Thompson, 2015; Poeta et al., 2017; Battisti et al., 2019b; Staffieri et al., 2019) which continues to raise concerns about its impacts to human and environmental health.
Given it’s ubiquity in the environment, considerable efforts have gone into identifying the major sources of marine anthropogenic debris (hereinafter referred to as marine debris). This is because understanding the sources of marine debris can inform mitigation. Generally, it is agreed upon that marine debris comes from land-based or ocean-based sources (Coe and Rogers, 1997; Sheavly and Register, 2007; Willis et al., 2017), which can be determined by the composition of items and diversity of material types (Cheshire et al., 2009; Battisti et al., 2017). Land-based debris items tend to be made up of consumer products composed of diverse materials, including glass (e.g., bottles), metal (e.g., cans), and plastic (Honorato-Zimmer et al., 2019a). Single-use items [e.g., plastic cutlery, food containers, beverage containers; (Honorato-Zimmer et al., 2019a; Terzi et al., 2020)] tend to be the most common. Another common type of land-based debris is plastic preproduction resin pellets (Karlsson et al., 2018). Land-based sources represent the majority of marine debris found in marine environments (Kershaw and Rochman, 2016; Meijer et al., 2020). On the contrary, ocean-based debris is dominated by more durable plastic items, with metal and glass typically less prevalent (Honorato-Zimmer et al., 2019a). Items such as fishing nets, lines, and buoys are characteristic of ocean-based debris (Battisti et al., 2019a). Irrespective of source, plastic represents a large proportion of the total marine debris found on beaches, and in mangrove ecosystems (Sheavly and Register, 2007; Kershaw and Rochman, 2016; Chen et al., 2020; Seeruttun et al., 2021).
A major pathway for land-based plastic debris to get to the oceans is via rivers and streams (Lebreton et al., 2017; Meijer et al., 2020; Terzi et al., 2020). Recent research suggests that approximately 80% of the plastic debris in the oceans comes from rivers (Meijer et al., 2020). Many of these rivers are found in rapidly developing countries in Asia, where increased production and consumption of plastic, waste importation, insufficient waste collection systems, and illegal dumping have resulted in large amounts of plastic pollution (Lebreton et al., 2017; Dauvergne, 2018; Meijer et al., 2020). This marine debris can accumulate in rivers, transport to the oceans, be washed up along beaches, or trapped in coastal ecosystems such as mangrove forests (Martin et al., 2019; Li et al., 2020; Seeruttun et al., 2021). Given that a high proportion of debris comes from rivers in Southeast Asia, local research in this region is needed to understand sources, pathways, and accumulation patterns in these “debris hotspots” to inform mitigation strategies.
In addition to better understanding the sources and fate of marine debris, it is important to understand whether any ecological impacts are associated with it. Currently, we know that marine debris interacts with hundreds of species worldwide (Gall and Thompson, 2015; Poeta et al., 2017; Battisti et al., 2019a; Staffieri et al., 2019), and that the impacts of marine debris to biota occur at the individual or population level. Examples of these impacts include entanglement, ingestion, and the provision of new habitat for dispersal of macro- and micro-biota (Gall and Thompson, 2015). Despite the fact that marine debris is recognized as a threat to marine ecosystems (Whitacre, 2012; Pham et al., 2014), it is still unclear whether marine debris, and in particular plastic, impacts biota at higher levels of biological organization (e.g., community or ecosystem; Koelmans et al., 2017). Assuming that individual and population level effects will “trickle up” to higher levels of biological organization, we might expect that marine debris will have impacts at the community, or ecosystem level (Browne et al., 2015b).
Vietnamese rivers are expected to be a significant source of plastic debris to the oceans (Jambeck et al., 2015; Meijer et al., 2020). In fact, a recent study ranked Vietnam eighth in terms of annual plastic emissions to the ocean (Meijer et al., 2020). Prior to beginning this research, there were no empirical assessments of anthropogenic debris in the Red River. This research represents the first documented baseline assessment of anthropogenic debris in the Red River. Here, we aimed to understand the sources, accumulation patterns, and ecological effects of marine debris in the Red River estuary–the second largest river in Vietnam. Passing through two major urban areas including the capital, Hanoi, and Nam Dinh city, it serves as an ideal site to address these questions about marine debris. Located at the mouth of the Red River (Song Hong) is Xuan Thuy National Park (XTNP), which is home to 14 species of mangroves, 116 species of water and shore birds, 111 aquatic plant species, and over 500 species of benthos and zooplankton, including shrimp, fish, crab, and oysters. The park also provides habitat for rare species of otter, porpoise, and whale (Xuan Thuy National Park, 2019). This site is of significant ecological importance which is highlighted by its designation as the first Ramsar site in South East Asia. Our first objective was to assess the amount and composition of anthropogenic debris in the park in order to identify potential sources. We hypothesized that the Red River was a source of land-based marine debris to XTNP, and that fisheries in the region may be a source of ocean-based debris. Thus, we predicted that land-based items would be found within and close to the river mouth, and ocean-based items would be found further from the river mouth and at sites facing the open ocean. We also expected that the most marine debris would be found near the high tide line (McDermid and McMullen, 2004; Browne et al., 2015a; Lavers and Bond, 2017). Our second objective was to determine whether marine debris had ecological effects on mangrove ecosystems. We hypothesized that anthropogenic debris would impact mangrove health. We predicted that diversity indices (mangrove diameter, mangrove canopy cover, crab burrow holes/m2) would decrease as debris concentrations increased. Combined, we aim for this work to inform future mitigation strategies relevant to reducing marine debris and protecting affected ecosystems.
Materials and Methods
Study Site
Our research was conducted within and just outside the border of Xuan Thuy National Park (XTNP), in Nam Dinh province, Vietnam. XTNP is located along, and at the mouth of, the Red River Estuary, approximately 150 km southeast of Hanoi. As the first Ramsar site in southeast Asia, the park was formally established in 1989 due to its significance for creating migratory bird habitat. Dominated by mangrove ecosystems and mud flats, the park is home to over 100 bird species, and countless other biota including invertebrates and plants (Xuan Thuy National Park, 2019). Despite being a conservation area, there remains a significant amount of economic activity within the park. Shrimp and clam aquaculture covers approximately 9 and 9.5% of the park lands respectively (Department of Agriculture and Rural Development, 2015; Department of Agriculture and Rural Development, 2018). Artisanal fishers enter the park to dig for clams in non-aquaculture areas.
To quantify and characterize debris and its ecological impacts in the park, we conducted two types of surveys: anthropogenic debris and ecological surveys. Debris surveys were conducted to quantify the amount, distribution, and composition of debris. Ecological surveys were conducted to understand the impacts of the debris on mangrove ecosystems. We selected five sites within the park that we felt would be informative regarding the potential sources of marine debris (Figure 1). Sites were located upstream of the Red River mouth (Site 5, Figure 1), at the river mouth (Site 1, Figure 1), facing the open ocean (Site 2, Figure 1), protected but ocean-facing (Site 3, Figure 1), and ocean-facing close to fishing boats (Site 4, Figure 1). By choosing sites both upstream of the mouth of the river, near the mouth, and beyond the river mouth, we were able to assess the potential for both land-based and ocean-based sources of marine debris. At each site, we completed between three and five replicate debris surveys. Ecological surveys were carried out at Sites 1–4, and only included mangroves where feasible (Sites 1–3).
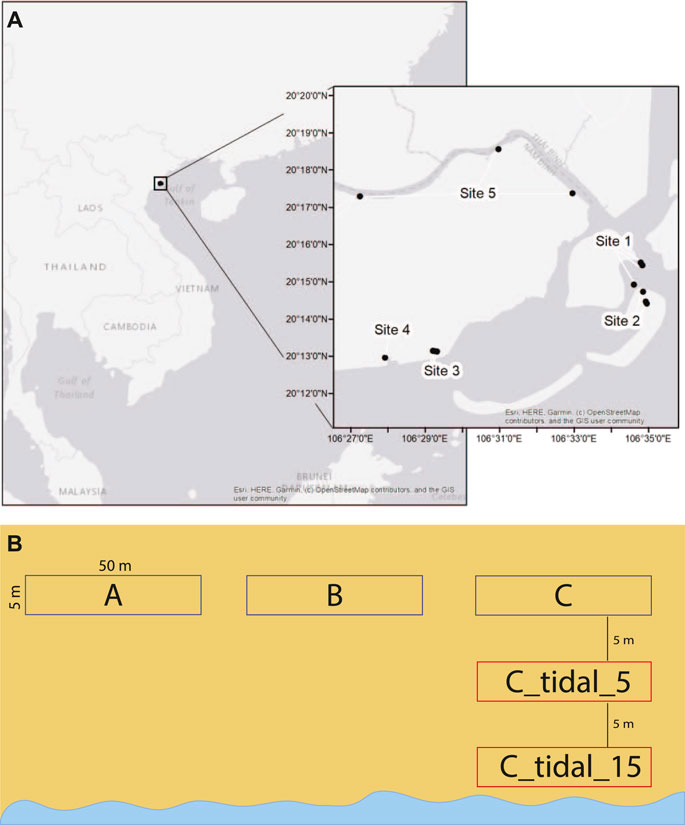
FIGURE 1. (A) Site overview. Five sites were surveyed for anthropogenic debris (1–5) and four were surveyed for ecological indices (1 – 4 for crab burrows and 1 – 3 for mangrove health). (B) Blue rectangles demarcate where triplicate transects were surveyed for anthropogenic debris and ecological health in transects that include the high tide line. These transects were located within the mangroves where possible. Red rectangles demarcate where transects were surveyed for anthropogenic debris along the tidal flats moving away from the high tide line. Tidal flat transects, located 5 and 15 m below the high tide transects, were only surveyed at Sites 1, 2, and 3.
Debris Surveys
Across all five sites, we conducted assessments in a total of 19 transects. We used a modified standing stock survey protocol from NOAA (Opfer et al., 2012), reducing transect size to 50 × 5 m or smaller when necessary (5A, 5B, 5C), due to topography or large amounts of debris. At each site, except Site 4, we conducted triplicate standing stock surveys parallel to the coastline (Figure 1B, blue). We included the high tide line in the middle of the transect (1A, 1B, 1C, 2A, 2B, 2C, 3A, 3B, 3C, 5A, 5B, 5C; Figure 1B, blue). At Sites 1–3, we conducted two additional standing stock surveys (1C_tidal_5, 1C_tidal_15, 2C_tidal_5, 2C_tidal_15, 3A_tidal_5, 3A_tidal_15) that were positioned moving toward the ocean from one of the transects at high tide (Figure 1B, red). These two transects were spaced approximately 5 m apart and were located approximately 5 and 15 m from the high tide line along the tidal flat toward the ocean (Figure 1B, red). For Site 4, we did not conduct the tidal flat surveys nor the triplicate standing stock debris surveys because this site was a pilot site. Here, we conducted a single debris survey and included it in our dataset due to the large amount of debris. For Site 5, along the river, our three transects were conducted further apart (>10 m) compared to other sites because we were limited to sites with accessibility (Figure 1A).
To quantify and characterize marine debris, we laid out the transect tape, marked the four corners of the transect, recorded the GPS coordinates of each corner, and took photographs of the transect. We then split the transect into three approximately equal sections, and three pairs of researchers surveyed one section each. Each team had one counter and one recorder. The counter walked within the transect in a zig zag motion, scanning their section for any visible debris items larger than 2.5 cm. As items were identified, the counter verbally reported to the recorder to document each item on a datasheet. All items were recorded to product and material type where possible (e.g., plastic straw, plastic container, metal can). For analyses, debris amounts were expressed as the number of items/m2.
Ecological Surveys
To measure how debris impacts mangrove ecosystem health, we measured crab burrows, mangrove diameter, and mangrove canopy cover in transects where crab burrows and/or mangroves were present. Crab burrows were measured in all transects, except for those at Site 5, i.e., the sites along the river, where crabs were absent. Mangrove indices were measured in transects at the high tide line at Sites 1–3. These bioindicators have been used in previous assessments of mangrove ecosystem health (Environmental Protection (Water) Policy 2009; Gül and Griffen 2018). To measure the number of crab burrows, we randomly dropped a 1 m by 1 m quadrat and counted the number of crab holes larger than 1 cm in diameter. This was repeated three times in each transect to obtain an average number of crab holes/m2. To obtain an average measure of mangrove diameter, we recorded the diameter around the trunk of ten mangrove trees, spaced approximately 5 m apart along the length of each transect. Although diameter at breast height (DBH) is the standard measurement used for mangrove health, we had to modify DBH measurements because the mangroves in XTNP are much shorter compared to those measured in standard monitoring protocols (Environmental Protection (Water) Policy 2009). For bush-like mangroves, the largest stem of the mangrove was selected and measured below where it first began to branch. For tree-like mangroves, the main trunk was measured below where it began to branch. Measures from all ten trees within the transect were then averaged to obtain a measure of mangrove diameter per transect. Last, a proxy for canopy cover was determined by subtracting the light intensity underneath the canopy from the light intensity of the open air. We used the Light meter Pro App (v2; Elena Polyanskaya, iOS, United States) on our smart phones to obtain measurements. We collected ten measurements for each transect, from the same trees that were measured above, and used these values to calculate an average light intensity for each transect. In general, the larger the light intensity difference between the canopy and open air, the more leaves are found in the canopy, reflecting better mangrove health. Small light intensity differences reflect fewer leaves in the canopy, indicating poorer mangrove health.
Statistical Analysis
All statistical analyses were performed using R Statistical Software version 3.5.2 using RStudio version 1.1.463 (RStudio Team, 2016). To assess patterns of debris composition across sites, we visualized our data in an nMDS plot using the Bray-Curtis dissimilarity metric plotted in two dimensions. Data was not transformed, and we measured stress to see if the pattern within the plot was a good fit. We used the function “metaMDS” in the vegan community ecology package (Oksanen et al., 2008) in R. To determine whether there were significant differences between the types of debris among sites, we ran a PERMANOVA. Differences were considered significant when p < 0.05. We used the function “adonis2” in the vegan community ecology package (Oksanen et al., 2008) in R. To better assess what debris types were driving these patterns, we visualized the top five most common debris items for each site. Data from all transects surveyed within a site were pooled, to obtain one list of debris items per site. To determine whether there was a significant difference in the total amount of debris in the transects that included the high tide line compared to those in the tidal flats, we performed a single factor ANOVA. For Sites 1, 2, and 3, we included transects in the high tide line that were directly above the tidal transects (n = 3), transects that were 5 m from the high tide line (n = 3), and transects that were 15 m from the high tide line (n = 3). We used the “aov” function in base R. To assess the ecological effects of anthropogenic debris on the mangrove ecosystems, we ran simple linear regressions to assess the relationship between the amount of marine debris and each ecological index individually, i.e., crab holes, mangrove diameter, and light intensity difference (canopy cover).
Results
Debris Surveys
Marine debris was found at all sites within and around the Red River in XTNP (Supplementary Table S1). Overall, the number of debris items varied from 0.14 to 16.9 items/m2, with an average of 2.81 items/m2. From greatest to least, Site five had the highest average debris density (14.25 items/m2 ± 2.72 SD), followed by Site 4 (6.15 items/m2), Site 2 (4.85 items/m2 ± 4.98 SD), Site 1 (2.16 items/m2 ± 3.59 SD), and Site 3 (0.76 items/m2 ± 0.63 SD). The density of debris was highest in transects that included the high tide line (Table 1; Figure 2), although the trend was not significant [F(2,6) = 1.256, p = 0.35]. Transects that included the high tide line (1A, 1B, 1C, 2A, 2B, 2C, 3A, 3B, 3C, 5A, 5B, 5C) had an average of 5.10 ± 6.99 debris items/m2, while transects five and 15 m toward the ocean (1C_tidal_5, 1C_tidal_15, 2C_tidal_5, 2C_tidal_15, 3A_tidal_5, 3A_tidal_15) had an average of 0.87 ± 1.19, and 0.21 ± 0.12 items/m2 respectively. Across all sites, plastic comprised the majority of all surveyed debris, making up 86.6% of surveyed debris items (Figure 2). The top five debris items across all sites, in order of most to least, were plastic food wrappers, foam fragments, plastic bags, fabric pieces, pieces of plastic rope and nets (Figure 3).
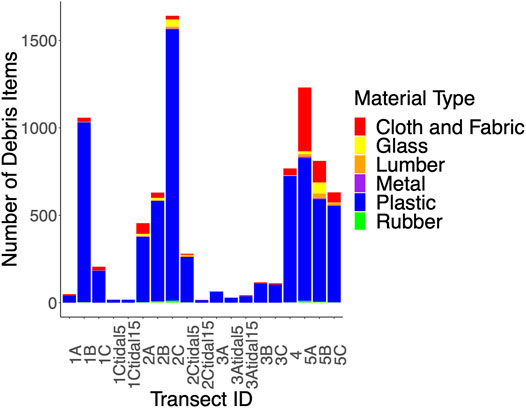
FIGURE 2. Bar graph showing the total amount of items of anthropogenic debris per m2 surveyed in each transect within all five sites. The colors represent the proportion of each material type surveyed in the transect.
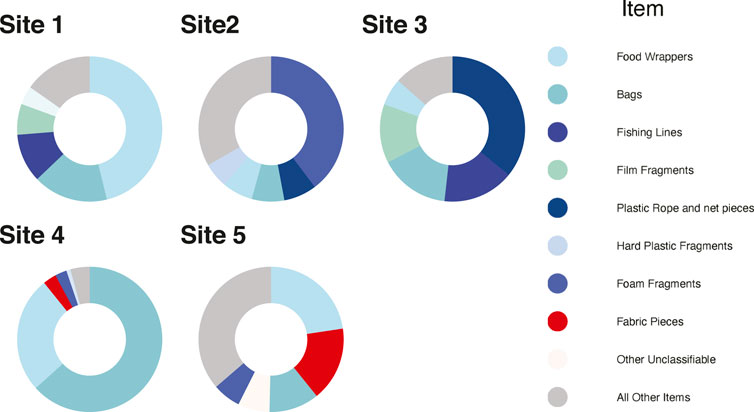
FIGURE 3. Top five debris items found in each site. The types of debris are characterized by color and the legend shows which color matches which debris item (right). Plastic items shown in shades of blue represent the majority of the top five items.
Overall, the PERMANOVA showed that the composition of debris items varied significantly between sites (Figure 4; p = 0.004). The patterns of similarities in debris composition between sites can be seen in the nMDS plot (Figure 4). Sites plotted closer together have more similar debris composition. The sites cluster into two groups; Sites 1, 2, and 3; and Sites 4 and 5 (Figure 4). This suggests that debris compositions are similar amongst these groups of sites. Foam, fishing lines, and rope were common amongst Sites 1, 2, and 3; food wrappers, plastic bags, and fabric pieces were common amongst Sites 4 and 5 (Figure 3). Fabric/cloth pieces were uniquely dominant in Sites 4 and 5 (Figure 3). Although Site 4 is plotted close to Site 5 (Figure 4), it is closer geographically to Sites 1, 2, and 3 (Figure 1).
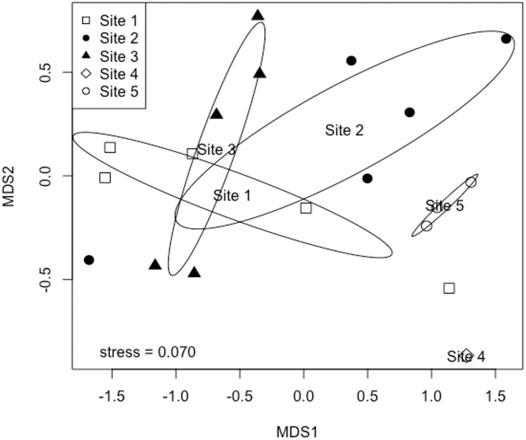
FIGURE 4. An nMDS plot created using data collected regarding all debris items across all locations within each of the five sites using the Bray-Curtis dissimilarity metric and plotted in two dimensions. Data was not transformed. Each point on the figure represents a transect, and the different shapes represent each of the five sites.
Ecological Surveys
All ecological indices showed a trend whereby the ecological index reduced with increasing amounts of marine debris. Still, none of these trends were significant. Light intensity difference represents the amount of light reaching the base of the mangrove tree; a smaller light intensity difference infers a lower density of leaves, indicating a less healthy plant. We observed a non-significant decrease in light intensity difference with increasing debris density represented by a decrease in light intensity difference (Figure 5B; r2 = 0.0756, p = 0.51). We observed a weak, non-significant negative relationship between mangrove diameter and debris density (Figure 5A; r2 = 0.0565, p value = 0.61). Similarly, we observed a negative, non-significant relationship between crab burrows and marine debris (Figure 6; r2 = 0.1321, p = 0.17).
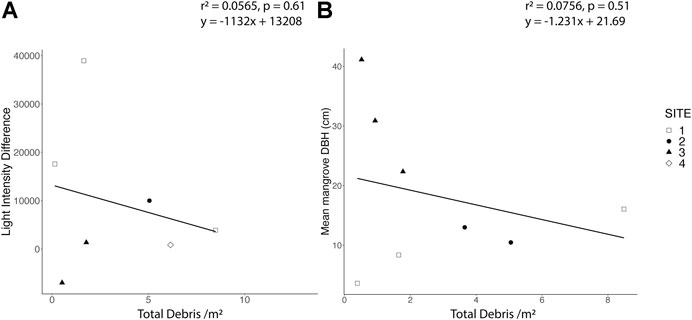
FIGURE 5. Scatter plots with the total number of debris items per m2 plotted on the x-axis and mangrove health index (DBH, light intensity) plotted on the y-axis. A trendline for a linear regression is shown with the equation, r2 value, and p value denoting significance for each graph. (A) Average light intensity difference vs. total debris. Light intensity difference is a proxy for canopy cover. Smaller differences in light intensity infers lower density of leaves, and poorer canopy cover. (B) Average mangrove diameter at breast height (cm) vs. total debris.
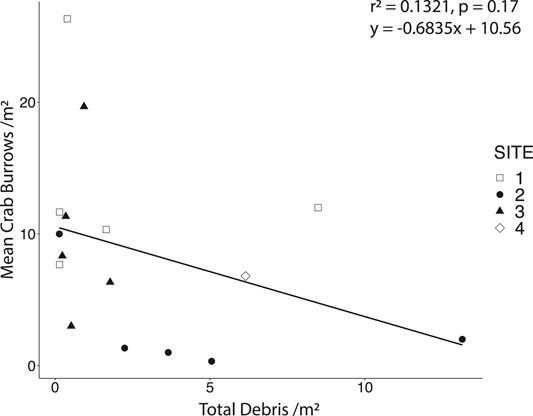
FIGURE 6. A scatter plot with the total number of debris items per m2 plotted on the x-axis and the number of crab holes per m2 plotted on the y-axis. A trendline for a linear regression is shown with the equation, R2 value, and p value denoting significance.
Discussion
The aim of our project was to quantify and characterize patterns of marine debris and assess ecological effects in the Red River, Vietnam. Our results demonstrate that debris is ubiquitous in the Red River, composition varies among sites, and that debris may have adverse or neutral ecological effects on mangrove ecosystem health. Based on the debris we found, our observations suggest plastic is the most abundant material found, and according to the type of items, Sites 4 and 5 had mostly land-based debris sources, and Sites 1, 2 and 3 had mostly ocean-based sources of debris (Honorato-Zimmer et al., 2019a; Chen et al., 2020; Terzi et al., 2020). According to the ecological surveys, it appears that marine debris has a negative impact on mangrove canopy cover, DBH, and crab burrows (Figures 5, 6); however, none of these relationships were significant. Overall, our results support our first hypothesis related to the distribution and composition of debris, but were unable to provide complete support for our second hypothesis that anthropogenic marine debris impacts mangrove ecosystems.
The preliminary nature of this study led to some limitations. First, there were relatively few sites surveyed, with some survey locations geographically close to each other, as we were limited to surveying sites that we could access, and with limited time. Future work should conduct sampling with broader spatial scales over longer periods of time (e.g., wet vs. dry season). Further, the ecological indices used in this study are rapid assessment tools, and may not fully capture the ecological dynamics taking place in this system. For example, it is possible that wave-action impacted the condition of the mangroves, rather than the debris. These methods were selected due to limited access to sample collection, and limited time in the field. Future work should investigate ecological effects over longer time periods, using more robust assessment methods, such as biological sampling of ecological communities. As such, this assessment serves as the first baseline assessment of marine debris in the Red River and its potential ecological effects, and should inform and motivate future work.
Debris Surveys
Our first objective was to quantify and characterize anthropogenic debris in and upstream of XTNP and identify potential sources. Overall, the range of debris densities measured in our study were comparable to beach debris surveys in China (e.g., Chen et al., 2020). We expected that the source of debris items at sites found within, or close to the river mouth (Sites 1 and 5) would be land-based, whereas the source of debris in sites facing the open ocean and further from the river would be ocean-based. It is generally agreed upon that item type (e.g., bag, fishing line) is indicative of the source of debris (Battisti et al., 2019a; Honorato-Zimmer et al., 2019a; Terzi et al., 2020). Thus, we expected that Sites 1 and 5 (within and close to the river) would have a high proportion of single-use consumer materials. Our results matched our predictions for Site 5, where nearly half of the plastic debris was comprised of single-use items such as food wrappers and plastic bags. This suggests that the land is a source of debris to Site 5. Although the debris at Site 1 included these items, it also included fishing-related items that were similar to Sites 2 and 3. This suggests that both land- and ocean-based sources (i.e., fishing and aquaculture) are also a source of debris to Site 1 (Battisti et al., 2019a). The debris composition of Site 4 was more similar to Site 5 (Figure 4). This suggests most debris encountered in Site 4 originated from land-based sources.
We predicted that debris composition in sites facing the ocean (Sites 2, 3, and 4), would reflect ocean-based sources (Martin et al., 2019). If true, we expected these sites would have a high proportion of plastic (∼80%; Honorato-Zimmer et al., 2019a), and specifically more ocean-based debris items (i.e., fishing nets, styrofoam coolers, buoys; Battisti et al., 2019a). Our results matched our predictions for Sites 2 and 3, where plastic comprised nearly all of the surveyed items, and fishery-related items such as foam pieces, fishing line and nets, and plastic rope were common. This suggests that fishing and aquaculture are a source of debris to Sites 2 and 3 (Figure 3). As previously mentioned, the debris composition of Site 4 was not consistent with our predictions, suggesting that the land is a source of debris to Site 4. Site 4 was located on a sandy beach, situated close to a docking area with many fishing boats, so it is possible that consumables from the fishing community are a source of debris to Site 4. As mentioned above, Site 1 held both a land-based and ocean-based signature suggesting that the ocean and the land are both sources of marine debris to this location.
Interestingly, fabric scraps and clothing items were common in Sites 4 and 5. Leakage of scrap fabric from textile industries may provide an explanation for the large amounts of fabric and cloth found at these sites. Pieces of scrap fabric are created when textile industries cut patterns for clothing (personal communication; Diana Rosenberg from GAP). Further research is required to identify where textile industries exist along the Red River and whether scrap fabric leakage is a viable explanation. Overall, the high proportion of single-use items and fabric scraps found at Sites 4 and 5 suggests that upstream sources of land-based debris deliver debris to XTNP, as do ocean-based sources.
Overall, our results demonstrate that the composition of debris can inform its source. The data we generated also support our hypothesis that the Red River is a source of debris to XTNP. Sites 4 and 5, and to a lesser extent Site 1, had high proportions of land-based debris. These results are consistent with other studies that have found that mangrove forests and beaches close to industrialized rivers have high proportions of land-based debris, while locations further from industrialized rivers have predominantly ocean-based sources of debris (Honorato-Zimmer et al., 2019a; Chen et al., 2020). In the Yangzte River, a highly industrialized river, 90% of debris found on beaches came from land-based sources (Chen et al., 2020), while in Chilean and German beaches far from industrialized rivers, ocean-based sources of debris dominated the beaches (e.g., Honorato-Zimmer et al., 2019a).
Our second prediction was that the concentration of debris would be highest in the transects that include the high tide line. Our results were consistent with this prediction; all transects that included the high tide line had higher debris densities than the transects surveyed outside of the high tide line (Figure 2). Still, the relationship was not significant. This could be due to the large standard deviation associated with debris densities in the high tide line. This is consistent with other beach survey studies that have found higher debris counts at the high tide line (McDermid and McMullen, 2004; Browne et al., 2015a; Lavers and Bond, 2017).
Ecological Surveys
Our second objective was to determine whether marine debris had ecological effects on the mangrove ecosystems. We predicted that anthropogenic debris would have negative impacts on mangrove health. If true, we expected that ecosystem health indices (canopy cover, mangrove diameter, crab burrow holes/m2) would decrease as marine debris increased. Overall, our results matched our predictions, where ecological indices declined as debris concentrations increased. Still, none of the relationships were significant.
We observed a negative, non-significant relationship between canopy cover, DBH, and debris density (Figure 2). Although there is little known about how mangroves respond to anthropogenic debris, we know that they respond to other stressors such as heavy metals through cellular changes (i.e., changes in protein production/expression), alterations in growth, photosynthesis, reproduction, and survival (Das et al., 2016; Yan et al., 2017; Nikalje and Suprasanna, 2018). Plastic macrodebris may break down into microplastics and sorb harmful chemicals (Rochman et al., 2013), which could reduce survival and growth of mangrove trees (Li et al., 2020). Due to sampling limitations, we were unable to assess changes to cellular processes, but the differences observed in light intensity difference (canopy cover) and mangrove diameter, proxies for ecosystem health, suggest that the debris has negative impacts on mangrove health. Alternatively, marine debris can cause hypoxia or anoxia by smothering the mangrove trees (Gregory, 2009). When plastic sheeting and bags blanket the seafloor, they limit gas exchange; similar processes may occur on the forest floor which could ultimately result in reduced growth of mangrove trees (Gregory, 2009). Many of the mangroves at our sites had debris hanging from the branches or entrained in the roots, which could provide an explanation for reduced diameter and canopy cover. Overall, macrodebris, or components of the debris such as microplastics and associated chemicals, may have caused stress to mangroves through direct or indirect mechanisms that may have impeded canopy and trunk growth. It is important to note that these measures are best employed in long term studies and with more replication. We only had six transects with mangrove measurements which does not allow for robust statistical analyses. Thus, we recommend continued monitoring of these forests, and with greater sample sizes, to assess changes to mangrove health over extended periods of time.
We observed a negative, non-significant relationship between crab burrows and debris density. Crab burrow density is commonly used in environmental monitoring as a proxy for mangrove ecosystem health (Environmental Protection Policy 2009; Gül and Griffen 2018). In general, a lower density of crab burrows is correlated with poor mangrove health. Debris, particularly plastic bags and food wrappers, may have smothered the crab habitat (Ivar do Sul et al., 2014; Lavers et al., 2020) or caused toxicity by ingestion of microplastics (Li et al., 2020). When ingested by organisms such as sea turtles, marine debris can cause a number of adverse effects, including nutrient dilution, bowel perforation, wounds, skin lesions, sores, and more (Tomas et al., 2002; Sigler, 2014). Due to this non-significant trend, we recommend that further research includes higher spatial coverage and replication and/or community-level ecological data to better understand whether such an effect may be predicted in this or other regions.
Mangrove forests serve an important role in estuarine ecosystems, by providing habitat and protection for species of fish, birds, and other organisms. They also provide ecosystem services for coastal communities through flood control, water filtration, and recreation (Costanza et al., 2014). Overall, our results suggest that marine debris may have negative or neutral impacts on mangrove ecosystem health. Because mangrove forests may trap and sequester anthropogenic debris for longer periods of time than beaches (Martin et al., 2019), it is important to further investigate how anthropogenic debris impacts these vulnerable ecosystems. Such studies might include long-term monitoring using the indices from this study or debris removal experiments.
Relevance to Policy
There is no doubt that the ubiquity of anthropogenic debris in marine ecosystems is a global problem, and requires both local and global efforts to be addressed. Scientists and policy makers can work together to accomplish this; scientists contribute to fundamental and applied understandings of the distribution, fate, and ecological effects of marine debris, while policy makers use these new scientific understandings to create relevant and effective policies and mitigation strategies (Rochman et al., 2016). Vietnam recently developed and issued a National Action Plan on marine debris, in which it identifies rivers as a potential avenue for high impact interventions (Promulgating the National Action Plan for Marine Plastic Waste Management to 2030, 2019). In this document, annual monitoring of debris is listed as a specific objective that should be implemented. Prior to our study, there was no previous data quantifying anthropogenic debris in the Red River; all relevant research had been conducted in rivers in Southern Vietnam (e.g., van Emmerik et al., 2018; van Emmerik et al., 2019; Lahens et al., 2018; van Emmerik and Schwarz, 2020). Further monitoring should be done to determine sources and hotspots for debris to inform prevention and clean-up strategies. Our research begins to fill these data gaps, by introducing replicable monitoring methodologies and empirical data that policy makers will be able to use to identify priority areas, and evaluate the efficacy of future mitigation practices. Our science can, and has been used by local governments and stakeholders to address the issue of anthropogenic marine debris and its ecological impacts in Vietnam as a whole.
Conclusion
Our goal was to conduct the first baseline assessment of anthropogenic debris and its ecological impacts in XTNP. We observed anthropogenic debris in all sites throughout the park, and our results suggest it originated from both ocean and land-based sources, depending on the site. We were able to identify potential sources by identifying the dominant types of debris at each site. Results indicated that sites located near to, or within the river had debris items indicative of land-based sources, whereas sites located far from the river or facing the ocean had debris items from ocean-based sources. Rapid ecological assessments of the mangrove ecosystems indicated that increasing debris densities were associated with increased ecological stress, including reductions in mangrove growth, canopy cover, and crab burrows. The results of this study directly address objectives in Vietnam’s National Action Plan for Marine Debris, which aims to reduce debris by 2030. Ultimately, this work contributes the first data on anthropogenic debris and potential ecological impacts in the Red River, which may eventually inform global mitigation, and conservation strategies.
Data Availability Statement
The original contributions presented in the study are included in the article/Supplementary Material, further inquiries can be directed to the corresponding author.
Author Contributions
RG conceived of the idea, developed the methods, conducted the experiment, ran analyses and wrote the first draft of the manuscript. CR conceived of the idea, developed the methods, conducted the experiment, ran analyses, contributed resources and edited the manuscript. CT, CVN, NT, and TT conceived of the idea, conducted the experiment and edited the manuscript. All authors approved the submitted version of the manuscript and agreed to be listed.
Funding
This work was supported Global Environment Fund (Project ID: 96812017). The Funding source was not involved in planning the study design; collection, analysis and interpretation of data; writing of the report; nor the decision to submit the article for publication.
Conflict of Interest
The authors declare that the research was conducted in the absence of any commercial or financial relationships that could be construed as a potential conflict of interest.
Acknowledgments
We would like to thank Sandra Whitehouse and Keondra Bills Freemyn for their support in planning this project. We would also like to thank Phan Xuan Long for assisting with site access, and Mai Kien Dinh for his assistance with conducting our field work. Thank you to Britta Baechler for providing comments on earlier drafts of this manuscript, and Xia Zhu for creating Figure 1A. We are grateful to two reviewers and the editor for their comments that improved this manuscript.
Supplementary Material
The Supplementary Material for this article can be found online at: https://www.frontiersin.org/articles/10.3389/fenvs.2021.679530/full#supplementary-material
References
Battisti, C., Bazzichetto, M., Poeta, G., Pietrelli, L., and Acosta, A. T. R. (2017). Measuring Non-biological Diversity Using Commonly Used Metrics: Strengths, Weaknesses and Caveats for Their Application in Beach Litter Management. J. Coast. Conserv. 21, 303–310. doi:10.1007/s11852-017-0505-9
Battisti, C., Kroha, S., Kozhuharova, E., De Michelis, S., Fanelli, G., Poeta, G., et al. (2019a). Fishing Lines and Fish Hooks as Neglected Marine Litter: First Data on Chemical Composition, Densities, and Biological Entrapment from a Mediterranean Beach. Environ. Sci. Pollut. Res. 26, 1000–1007. doi:10.1007/s11356-018-3753-9
Battisti, C., Staffieri, E., Poeta, G., Sorace, A., Luiselli, L., and Amori, G. (2019b). Interactions between Anthropogenic Litter and Birds: A Global Review with a ‘black-List’ of Species. Mar. Pollut. Bull. 138, 93–114. doi:10.1016/j.marpolbul.2018.11.017
Bergmann, M., Gutow, L., and Klages, M. (2015). “Alfred-Wegener-Institut,” in Marine Anthropogenic Litter. Editor G. universitet (Cham Heidelberg New York Dordrecht London: Springer Open. Springer).
Bergmann, M., Lutz, B., Tekman, M. B., and Gutow, L. (2017). Citizen Scientists Reveal: Marine Litter Pollutes Arctic Beaches and Affects Wild Life. Mar. Pollut. Bull. 125, 535–540. doi:10.1016/j.marpolbul.2017.09.055
Browne, M. A., Chapman, M. G., Thompson, R. C., Amaral Zettler, L. A., Jambeck, J., and Mallos, N. J. (2015a). Spatial and Temporal Patterns of Stranded Intertidal Marine Debris: Is There a Picture of Global Change? Environ. Sci. Technol. 49, 7082–7094. doi:10.1021/es5060572
Browne, M. A., Underwood, A. J., Chapman, M. G., Williams, R., Thompson, R. C., and van Franeker, J. A. (2015b). Linking Effects of Anthropogenic Debris to Ecological Impacts. Proc. R. Soc. B Biol. Sci. 282, 20142929. doi:10.1098/rspb.2014.2929
Chen, H., Wang, S., Guo, H., Lin, H., and Zhang, Y. (2020). A Nationwide Assessment of Litter on China’s Beaches Using Citizen Science Data. Environ. Pollut. 258, 113756. doi:10.1016/j.envpol.2019.113756
Cheshire, A., Adler, E., Barbière, J., and United Nations Environment Programme, (2009). UNEP/IOC Guidelines on Survey and Monitoring of Marine Litter. Nairobi: UNEP.
Chiba, S., Saito, H., Fletcher, R., Yogi, T., Kayo, M., Miyagi, S., et al. (2018). Human Footprint in the Abyss: 30 Year Records of Deep-Sea Plastic Debris. Mar. Pol. 96, 204–212. doi:10.1016/j.marpol.2018.03.022
Coe, J. M., and Rogers, D. B. (1997). Marine Debris: Sources, Impacts and Soltuion. New York: Springer-Verlag.
Costanza, R., de Groot, R., Sutton, P., van der Ploeg, S., Anderson, S. J., Kubiszewski, I., et al. (2014). Changes in the Global Value of Ecosystem Services. Glob. Environ. Change 26, 152–158. doi:10.1016/j.gloenvcha.2014.04.002
Das, S. K., Patra, J. K., and Thatoi, H. (2016). Antioxidative Response to Abiotic and Biotic Stresses in Mangrove Plants: A Review: Antioxidative Response to Abiotic and Biotic Stresses. Int. Rev. Hydrobiol. 101, 3–19. doi:10.1002/iroh.201401744
Dauvergne, P. (2018). Why Is the Global Governance of Plastic Failing the Oceans? Glob. Environ. Change 51, 22–31. doi:10.1016/j.gloenvcha.2018.05.002
Department of Agriculture & Rural Development, (2018). Master Planning for Aquaculture for Giao Thuy District. Hanoi, Vietnam
Department of Agriculture and Rural Development, (2015). Report on Situation of Clam Aquaculture in Giao Thuy District (Nam Dinh Province). Hanoi, Vietnam
D. M. Whitacre (Editior) (2012). Reviews of Environmental Contamination and Toxicology, Reviews of Environmental Contamination and Toxicology (New York, NY: Springer New York). doi:10.1007/978-1-4614-3414-6
Eriksen, M., Thiel, M., and Lebreton, L. (2019). “Nature of Plastic Marine Pollution in the Subtropical Gyres,” in Hazardous Chemicals Associated with Plastics in the Marine Environment, the Handbook of Environmental Chemistry. Editors H. Takada, and H. K. Karapanagioti (Cham: Springer International Publishing). doi:10.1007/978-3-319-95568-1
Environmental Protection (Water) Policy (2009). Monitoring and Sampling ManualQueensland Government.
Gall, S. C., and Thompson, R. C. (2015). The Impact of Debris on Marine Life. Mar. Pollut. Bull. 92, 170–179. doi:10.1016/j.marpolbul.2014.12.041
Gregory, M. R. (2009). Environmental Implications of Plastic Debris in Marine Settings—Entanglement, Ingestion, Smothering, Hangers-On, Hitch-Hiking and Alien Invasions. Philos. Trans. R. Soc. B Biol. Sci. 364, 2013–2025. doi:10.1098/rstb.2008.0265
Gregory, M. R. (1978). Virgin Plastic Granules on South-West Pacific Beaches and Their Possible Environmental Implications. Presented Tenth Int. Congress Sedimentology 1, 270–271.
Gül, M. R., and Griffen, B. D. (2018). Impacts of Human Disturbance on Ghost Crab Burrow Morphology and Distribution on Sandy Shores. PLOS ONE 13, e0209977. doi:10.1371/journal.pone.0209977
Honorato-Zimmer, D., Kruse, K., Knickmeier, K., Weinmann, A., Hinojosa, I. A., and Thiel, M. (2019a). Inter-hemispherical Shoreline Surveys of Anthropogenic Marine Debris – A Binational Citizen Science Project with Schoolchildren. Mar. Pollut. Bull. 138, 464–473. doi:10.1016/j.marpolbul.2018.11.048
Ivar do Sul, J. A., Costa, M. F., Silva-Cavalcanti, J. S., and Araújo, M. C. B. (2014). Plastic Debris Retention and Exportation by a Mangrove Forest Patch. Mar. Pollut. Bull. 78, 252–257. doi:10.1016/j.marpolbul.2013.11.011
Jambeck, J. R., Geyer, R., Wilcox, C., Siegler, T. R., Perryman, M., Andrady, A., et al. (2015). Plastic Waste Inputs from Land into the Ocean. Science 347, 768–771. doi:10.1126/science.1260352
Karlsson, T. M., Arneborg, L., Broström, G., Almroth, B. C., Gipperth, L., and Hassellöv, M. (2018). The Unaccountability Case of Plastic Pellet Pollution. Mar. Pollut. Bull. 129, 52–60. doi:10.1016/j.marpolbul.2018.01.041
Kershaw, P., and Rochman, C., (2016). Sources, Fate and Effects of Microplastics in the Marine Environment: Part Two of a Global Assessment,(London: INTERNATIONAL MARITIME ORGANIZATION), (IMO/FAO/UNESCO-IOC/UNIDO/WMO/IAEA/UN/UNEP/UNDP Joint Group of Experts on the Scientific Aspects of Marine Environmental Protection. 93).
Koelmans, A. A., Besseling, E., Foekema, E., Kooi, M., Mintenig, S., Ossendorp, B. C., et al. (2017). Risks of Plastic Debris: Unravelling Fact, Opinion, Perception, and Belief. Environ. Sci. Technol. 51, 11513–11519. doi:10.1021/acs.est.7b02219
Lahens, L., Strady, E., Kieu-Le, T.-C., Dris, R., Boukerma, K., Rinnert, E., et al. (2018). Macroplastic and Microplastic Contamination Assessment of a Tropical River (Saigon River, Vietnam) Transversed by a Developing Megacity. Environ. Pollut. 236, 661–671. doi:10.1016/j.envpol.2018.02.005
Lavers, J. L., and Bond, A. L. (2017). Exceptional and Rapid Accumulation of Anthropogenic Debris on One of the World’s Most Remote and Pristine Islands. Proc. Natl. Acad. Sci. 114, 6052–6055. doi:10.1073/pnas.1619818114
Lavers, J. L., Sharp, P. B., Stuckenbrock, S., and Bond, A. L. (2020). Entrapment in Plastic Debris Endangers Hermit Crabs. J. Hazard. Mater. 387, 121703. doi:10.1016/j.jhazmat.2019.121703
Law, K. L., Morét-Ferguson, S. E., Goodwin, D. S., Zettler, E. R., DeForce, E., Kukulka, T., et al. (2014). Distribution of Surface Plastic Debris in the Eastern Pacific Ocean from an 11-Year Data Set. Environ. Sci. Technol. 48, 4732–4738. doi:10.1021/es4053076
Lebreton, L. C. M., van der Zwet, J., Damsteeg, J.-W., Slat, B., Andrady, A., and Reisser, J. (2017). River Plastic Emissions to the World’s Oceans. Nat. Commun. 8, 15611. doi:10.1038/ncomms15611
Lebreton, L., Slat, B., Ferrari, F., Sainte-Rose, B., Aitken, J., Marthouse, R., et al. (2018). Evidence that the Great Pacific Garbage Patch Is Rapidly Accumulating Plastic. Sci. Rep. 8, 4666. doi:10.1038/s41598-018-22939-w
Li, R., Yu, L., Chai, M., Wu, H., and Zhu, X. (2020). The Distribution, Characteristics and Ecological Risks of Microplastics in the Mangroves of Southern China. Sci. Total Environ. 708, 135025. doi:10.1016/j.scitotenv.2019.135025
Martin, C., Almahasheer, H., and Duarte, C. M. (2019). Mangrove Forests as Traps for Marine Litter. Environ. Pollut. 247, 499–508. doi:10.1016/j.envpol.2019.01.067
McDermid, K. J., and McMullen, T. L. (2004). Quantitative Analysis of Small-Plastic Debris on Beaches in the Hawaiian Archipelago. Mar. Pollut. Bull. 48, 790–794. doi:10.1016/j.marpolbul.2003.10.017
Meijer, L., van Emmerik, T., van der Ent, R., Schmidt, C., and Lebreton, L. (2020). Over 1000 Rivers Accountable for 80% of Global Riverine Plastic Emissions into the Ocean. EarthArXiv [Preprint].
Nikalje, G. C., and Suprasanna, P. (2018). Coping with Metal Toxicity – Cues from Halophytes. Front. Plant Sci. 9, 777. doi:10.3389/fpls.2018.00777
Oksanen, J., Blanchet, F. G., Friendly, M., Kindt, R., Legendre, P., Mcglinn, D., et al. (2008). Package ‘ Vegan.
Opfer, S., Arthur, C., and Lippiatt, S. (2012). NOAA Marine Debris Shoreline Survey Field GuideNOAA Marine Debris Program.
Pham, C. K., Ramirez-Llodra, E., Alt, C. H. S., Amaro, T., Bergmann, M., Canals, M., et al. (2014). Marine Litter Distribution and Density in European Seas, from the Shelves to Deep Basins. PLoS ONE 9, e95839. doi:10.1371/journal.pone.0095839
Poeta, G., Staffieri, E., Acosta, A. T. R., and Battisti, C. (2017). Ecological Effects of Anthropogenic Litter on Marine Mammals: A Global Review with a “black-List’’ of Impacted Taxa. Hystrix It. J. Mamm. 29 (2), 253–264.
Promulgating the National Action Plan for Marine Plastic Waste Management to 2030 (2019). (No. 1746/QD-TTg). Hanoi, Vietnam.
Rochman, C. M., Cook, A.-M., and Koelmans, A. A. (2016). Plastic Debris and Policy: Using Current Scientific Understanding to Invoke Positive Change: Plastic Debris and Policy. Environ. Toxicol. Chem. 35, 1617–1626. doi:10.1002/etc.3408
Rochman, C. M., Manzano, C., Hentschel, B. T., Simonich, S. L. M., and Hoh, E. (2013). Polystyrene Plastic: A Source and Sink for Polycyclic Aromatic Hydrocarbons in the Marine Environment. Environ. Sci. Technol. 47, 13976–13984. doi:10.1021/es403605f
RStudio Team (2016). RStudio Integr. Dev. Environ. R. Available at: https://rstudio.com/ (Accessed 15 4, 20).
Seeruttun, L. D., Raghbor, P., and Appadoo, C. (2021). First Assessment of Anthropogenic Marine Debris in Mangrove Forests of Mauritius, a Small Oceanic Island. Mar. Pollut. Bull. 164, 112019. doi:10.1016/j.marpolbul.2021.112019
Sheavly, S. B., and Register, K. M. (2007). Marine Debris & Plastics: Environmental Concerns, Sources, Impacts and Solutions. J. Polym. Environ. 15, 301–305. doi:10.1007/s10924-007-0074-3
Sigler, M. (2014). The Effects of Plastic Pollution on Aquatic Wildlife: Current Situations and Future Solutions. Water Air Soil Pollut. 225, 2184. doi:10.1007/s11270-014-2184-6
Staffieri, E., de Lucia, G. A., Camedda, A., Poeta, G., and Battisti, C. (2019). Pressure and Impact of Anthropogenic Litter on Marine and Estuarine Reptiles: an Updated “Blacklist” Highlighting Gaps of Evidence. Environ. Sci. Pollut. Res. 26, 1238–1249. doi:10.1007/s11356-018-3616-4
Terzi, Y., Erüz, C., and Özşeker, K. (2020). Marine Litter Composition and Sources on Coasts of South-Eastern Black Sea: A Long-Term Case Study. Waste Manag. 105, 139–147. doi:10.1016/j.wasman.2020.01.032
Tomas, J., Guitart, R., Mateo, R., and Raga, J. (2002). Marine Debris Ingestion in Loggerhead Sea Turtles, Caretta caretta, from the Western Mediterranean. Mar. Pollut. Bull. 44, 211–216. doi:10.1016/s0025-326x(01)00236-3
van Emmerik, T., Kieu-Le, T.-C., Loozen, M., van Oeveren, K., Strady, E., Bui, X.-T., et al. (2018). A Methodology to Characterize Riverine Macroplastic Emission into the Ocean. Front. Mar. Sci. 5, 372. doi:10.3389/fmars.2018.00372
van Emmerik, T., and Schwarz, A. (2020). Plastic Debris in Rivers. WIREs Water 7. doi:10.1002/wat2.1398
van Emmerik, T., Strady, E., Kieu-Le, T.-C., Nguyen, L., and Gratiot, N. (2019). Seasonality of Riverine Macroplastic Transport. Sci. Rep. 9, 13549. doi:10.1038/s41598-019-50096-1
Willis, K., Denise Hardesty, B., Kriwoken, L., and Wilcox, C. (2017). Differentiating Littering, Urban Runoff and Marine Transport as Sources of Marine Debris in Coastal and Estuarine Environments. Sci. Rep. 7, 44479. doi:10.1038/srep44479
Xuan Thuy National Park (2019). The First Ramsar site of Vietnam. Available at: http://www.vuonquocgiaxuanthuy.org.vn/ (Accessed June 8, 2019)
Keywords: anthropogenic marine debris, ecological impact, environmental stressor, plastic pollution, mangrove, estuary, river
Citation: Giles RK, Nguyen CAT, Hồ TTY, Nguyến CV, Ngô NT and Rochman CM (2021) Source-Specific Patterns of Marine Debris and Associated Ecological Impacts in the Red River Estuary of Xuan Thuy National Park, Vietnam. Front. Environ. Sci. 9:679530. doi: 10.3389/fenvs.2021.679530
Received: 12 March 2021; Accepted: 03 May 2021;
Published: 28 May 2021.
Edited by:
Daniel González-Fernández, University of Cádiz, SpainReviewed by:
Setyo Budi Kurniawan, Universiti Kebangsaan Malaysia, MalaysiaCorrado Battisti, Roma Tre University, Italy
Copyright © 2021 Giles, Nguyen, Hồ, Nguyến, Ngô and Rochman. This is an open-access article distributed under the terms of the Creative Commons Attribution License (CC BY). The use, distribution or reproduction in other forums is permitted, provided the original author(s) and the copyright owner(s) are credited and that the original publication in this journal is cited, in accordance with accepted academic practice. No use, distribution or reproduction is permitted which does not comply with these terms.
*Correspondence: Rachel K. Giles, cmFjaGVsLmsuZ2lsZXNAZ21haWwuY29t