Commentary: Dryland Watershed Restoration With Rock Detention Structures: A Nature-Based Solution to Mitigate Drought, Erosion, Flooding, and Atmospheric Carbon
- 1Biophilia Foundation, Chester, MD, United States
- 2Cuenca Los Ojos, Pearce, AZ, United States
- 3Borderlands Restoration Network, Patagonia, AZ, United States
Historic land degradation is an ongoing threat to the Sky Islands of southern Arizona, US, and northern Sonora, Mexico, an area designated as a globally significant biodiversity hotspot. Land degradation has reduced ecosystem services provisioning, released carbon from disturbed soils into the atmosphere, and significantly diminished resilience to climate change. Private land managers in the region have developed methods to reverse degradation and restore biodiversity and ecosystem function. Land managers have used rock detention structures (RDS), technology adapted from traditional Indigenous practices in the region, as a tool for reversing desertification and watershed degradation. The structures were installed primarily for erosion control and water management, but they have had positive impacts on multiple biophysical systems. In this study, we analyze watershed-scale installation of RDS as a nature-based solution for climate change mitigation and adaptation. Case studies include four properties that offer examples of structures that have been in place over a period ranging from 1 to 40 years. We reviewed journal articles and other studies conducted at the four sites, supplemented with interviews, to catalogue the nature-based solutions provided by RDS. This study documents positive impacts on overall stream flow, reduction in peak runoff during inundation events, and increased sedimentation, which increase resilience to drought, erosion, and flooding. Data suggest potential impacts for climate change mitigation, though further research is needed. In addition, results suggest that watershed restoration with RDS offers a host of co-benefits, including an increase in biodiversity and wildlife abundance, an increase in vegetative cover, and increased surface water provisioning over time to support the land-based livelihoods of downstream neighbors. In the discussion, we consider barriers to replication and scalability using the strategy of the UN Decade on Ecosystem Restoration as a guiding framework, discussing issues of awareness, legislation and policy, technical capacity, finance, and gaps in knowledge.
Introduction
Ecosystems of the arid southwestern United States and northern Mexico have suffered extensive land degradation. This degradation has been a continuing threat to the Sky Islands of southern Arizona and northern Sonora, an area that is both a biodiversity hotspot and a harbinger of the deleterious effects of climate change (Deyo et al., 2012; Falk, 2013).
One of the most visible symptoms of land degradation in the Sky Islands has been the incision of streambeds and the declining health of the riparian areas that depend upon them. Though the region is arid, it was crossed with important rivers and wetlands (ciénegas) at the time of European arrival (Minckley et al., 2009). Even in a degraded state, these perennial and ephemeral waterways are critical to maintaining the region’s biodiversity. For example, in the Sonoran Desert, dry washes occupy less than 5% of land area, but they support 90% of bird life, even though washes may carry water for only a few hours a year (Dimmit 2015).
Healthy riparian areas play important roles in regulating the flow and quality of surface water, providing water for domesticated animals and wildlife, maintaining water tables, recharging aquifers, and preventing erosion. Globally, wetlands, including riparian areas, constitute only 9% of landscapes, but they are estimated to deliver 23% of global ecosystem service values (Zedler and Kercher 2005; Costanza et al., 2014). When watersheds are degraded, the provision of these ecosystem services is diminished. Degraded watersheds are characterized by disturbances to hydrology brought about by poor soil stability due to a lack of vegetation, unsustainable timber harvest, over-allocation of surface and groundwater, overgrazing, intentional burning for agriculture, a legacy of fire suppression, habitat fragmentation, and extirpation of beaver (Castor canadensis, Cole and Cole 2015).
In recent years, scientists and policy makers have begun addressing loss of ecosystem functionality through nature-based solutions (NbS), which are “actions to protect, sustainably manage, and restore natural or modified ecosystems that address societal challenges effectively and adaptively, simultaneously providing human well-being and biodiversity benefits” (IUCN 2020). Recognized societal challenges that can be ameliorated through NbS include climate change mitigation and adaptation, disaster risk reduction, economic and social development, human health, food security, water security, environmental degradation, and biodiversity loss (IUCN 2020).
NbS have the potential to remedy societal problems that have traditionally been addressed with grey infrastructure. Unlike grey infrastructure, however, NbS often deliver a host of co-benefits (Chausson et al., 2020). For example, on vulnerable coastlines, both dykes and restored mangroves can address erosion and storm surges, but restored mangroves also benefit biodiversity, carbon sequestration, and food security (McGinn 2019). Research has found that most NbS have positive outcomes, such as increased number of species, functional diversity, or greater plant or animal productivity (Chausson et al., 2020). NbS can also foster a sense of place and strengthen social infrastructure (Tidball et al., 2018). In this study, we use NbS as a lens for examining the impacts of watershed restoration on ecosystem functionality.
Indigenous peoples in this region have installed rock detention structures (RDS, a subset of the more general category of erosion control structures) for centuries, most famously (in this region) around Trincheras, Sonora (Phillips, 2009). The archaeological literature on the American Southwest contains thousands of records of check dams, and the Zuni people of the Southwest continue to use check dams to spread the flow of water more evenly over the land, as they and others have for centuries (Doolittle, 2010).
Most recently, restoration practitioners have been installing RDS at our case study sites (see Methods) and elsewhere to increase water availability, reduce the number and severity of flooding events, promote vegetative growth, and decrease erosion. RDS are simple structures, usually composed of stone found on site, and they include a variety of types, such as check dams, one rock dams, and gabions Figure 1. When the structures installed are of small or medium size, such as those illustrated in Figure 1, RDS-based watershed restoration is an example of low-tech, process-based restoration (Wheaton et al., 2019), which is defined as the use of simple, cost effective, hand-built solutions that help repair degraded streams and that are “designed to kickstart the processes that allow the stream to repair itself” (Wheaton et al., 2019, p.4). While their construction is an act of craftsmanship, and proper placement requires expertise, volunteers can be trained to construct high quality structures. Their impact can be profound: “Viewed in terms of environmental change, there may well be no deliberate human activity that has a greater impact given its minimal input than the construction of check dams. The simple act of dropping an obstacle across a small water course affects land both upstream and downstream” (Doolittle, 2010).
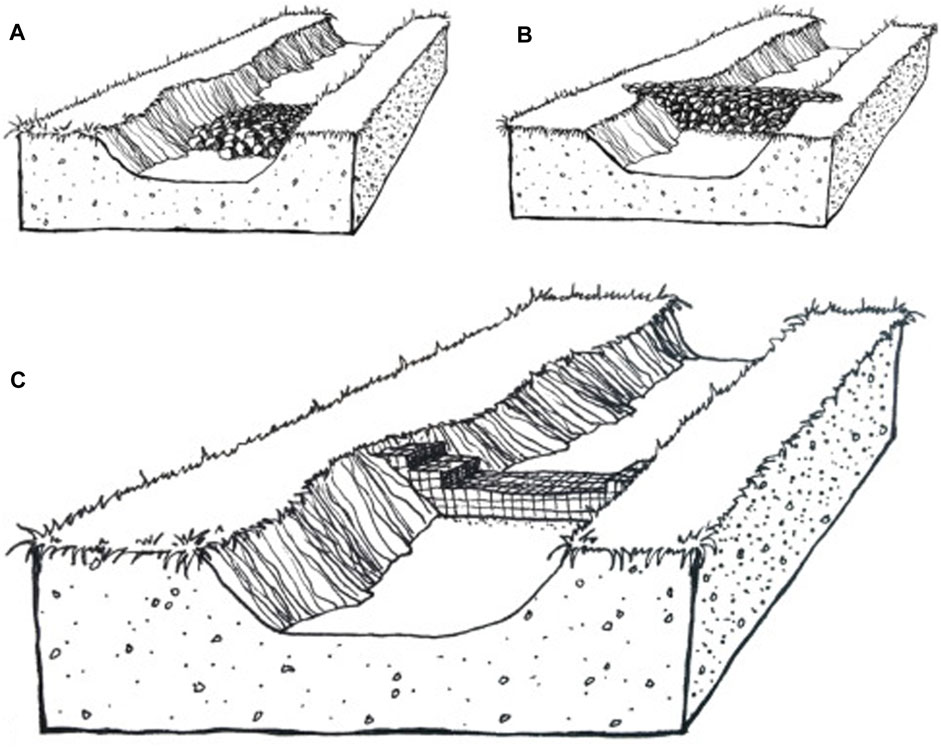
FIGURE 1. Sketches of rock-detention structures, including: (A) spreader (or one-rock dam), (B) loose-rock check dams (or gully plugs), and (C) larger rock-filled wire baskets (gabions) (reprinted from Norman et al., (2017), Figure 1, Chloé Fandel).
The “low tech” aspects of this solution make it worthy of further investigation for several reasons. First, globally more than 2 billion hectares of previously productive land is now degraded (UNCCD, 2020), and much of that land is occupied by the world’s poor. Relative to “high tech,” engineered alternatives, solutions with low-cost materials and minimal training can be more rapidly deployed and used to better engage communities in land stewardship. Additionally, in many regions of the world, people already use RDS for erosion control and to improve land for agriculture (WOCAT, 2021). Documenting the additional utility of watershed-scale installation extends their applicability to restoring other ecosystem functions. Lastly, because RDS slow and spread water, their use in arid and semi-arid regions may help foster resilience to current and future challenges associated with a changing climate.
The aims of this paper are to identify 1) the multiple societal impacts of watershed-scale installation of RDS in order to understand their function as an NbS and 2) factors that affect replicability and scalability in the Sky Islands. To address the first aim, we consolidate empirical evidence from case study sites to catalogue the societal problems for which RDS can serve as a solution. We investigated four case studies in northern Sonora and southern Arizona that offer examples of RDS that have been in place over a period ranging from 1 to 40 years. Using both interview data and analyses from 18 journal articles, theses, and other publications based on data collected at these sites, we document the impacts on overall stream flow, peak flow during inundation events, sedimentation and the resulting effects on resilience to drought, erosion, and flooding. In addition, data suggest that watershed restoration with RDS offers a host of other co-benefits, including an increase in biodiversity and wildlife abundance, an increase in vegetative cover, and increased surface water provisioning over time to support the land-based livelihoods of downstream neighbors.
To address the second aim, we consider barriers to widespread implementation using the strategy for the United Nations Decade on Ecosystem Restoration as a guiding framework, informed by our experience with watershed restoration in the Sky Islands. We consider how barriers can be overcome, including guiding principles to inform policy decisions and the potential of carbon markets as a financing mechanism.
Methods
Sky Islands Ecosystem
All four case studies are located within the Sky Islands/Madrean Archipelago in southeastern Arizona and northeastern Sonora. This region lies at the convergence of several major biogeographic zones, including the Sonoran Desert, Chihuahuan Desert, the Rocky Mountains and Colorado Plateau, and the Sierra Madre Occidental. It is home to more than 60 isolated mountain ranges with elevations that rise to over 3,000 m.
The Sky Islands sustain exceptional biodiversity. For example, approximately one-third of all bird species in North America occur in the Chiricahua Mountains, an isolated range in southeastern Arizona (Moore 2015), and one-quarter of all species native to Mexico occur in the Madrean pine-oak woodlands (Wilson, 2016). The entire region is thought to be home to 3,600 species of plants (Moore, 2015). The Sonoran portion is less well known, but new species and genera continue to be discovered in ongoing explorations.
In Tucson, AZ, temperatures increased 0.25C per decade between 1949 and 2011 (Moore, 2015). As a consequence, there have been more large fires, widespread outbreaks of bark beetles, spread of invasive species, shifts in plant flowering times, and plant species being pushed to higher elevation, potentially causing local extinctions of high-elevation endemics and other evolutionary unique lineages (Moore, 2015).
The Sky Islands have been designated an urgent conservation priority due to the region’s high biodiversity and high level of threat. In the last 200 years, the Sky Islands have been degraded due to the pressures of unsustainable grazing, farming, mining, and eradication of keystone species, such as beaver (Castor canadensis, Cole and Cole, 2015). Ciénegas, or desert wetlands, and meandering waterways were drained by the historical incision of rivers (Hendrickson and Minckley, 1985), which depleted surface and ground water, altered aquatic habitats, and led to a decline in the wildlife that depend on them (Cole and Cole, 2015). Conservation International, the Critical Ecosystem Partnership Fund, and the Key Biodiversity Areas Partnership have designated the area a global biodiversity hotspot, highlighting its plant diversity and threat of further degradation (Critical Ecosystem Partnership Fund, 2020; Conservation International, 2020; Birdlife International, 2020).
Case Study Sites
In this paper we analyze evidence for the impacts of RDS at four case study sites with which we are familiar. The sites are the Cuenca Los Ojos protected area in northeastern Sonora, Mexico, and the El Coronado Ranch, Smith Canyon, and the Babacomari Ranch in southeastern Arizona, United States.
Cuenca Los Ojos
Cuenca Los Ojos (CLO) is a nonprofit privately protected area in northern Sonora, Mexico, immediately south of the U.S.-Mexico border. The 53,000-ha area consists of nine former cattle ranches, ranging in elevation from 900 to 2,400 m. Low elevation desert grasslands transition to mesquite grasslands and pinyon-juniper woodlands, and upper elevations support dense coniferous forests. CLO lands provide habitat for at least 13 endangered or threatened species. Species counts include more than 70 mammals, 250 birds, 20 amphibians, 70 reptiles, 8 native fish (4 endemic), 900 invertebrates, and an estimated 3,000 plants (pers. comm.).
Prior land uses, including grazing, significantly degraded the land, accelerated erosion and attendant release of carbon, and reduced water infiltration, which depleted water tables. Restoration efforts have included changes to grazing practices and installation of RDS in eroded areas, beginning in the mid-1990s. To date, CLO has constructed more than 50 large gabions and 40,000 small rock dams in lower order washes and hillsides. It is one of the largest-scale applications of this method known; DeLong and Henderson, (2012) noted, “we are unaware of a comparable attempt to use gabions and berms for the sole purpose of ecological restoration along >10 km of arroyo channels draining watersheds on the order of ∼400 km2 and larger.” Current threats include climate change, adjacent land use change to irrigated cropland, and habitat fragmentation by both the U.S.-Mexico border wall and the widening of Highway 2 in Mexico.
El Coronado
El Coronado (EC) is a 6,600-ha ranch on the western slope of the Chiricahua mountains in southeastern Arizona, including both privately owned land and the leased West Turkey Creek allotment from the U.S. Forest Service. The proprietors began installation of RDS structures in the Turkey Pen watershed in 1983 to mitigate erosion caused by previous cattle grazing and forest clearing, eventually installing approximately 2,000 structures. After successful demonstration of watershed restoration at El Coronado, proprietors founded CLO to extend their impact into northern Mexico.
Smith Canyon
Smith Canyon is a tributary of Sonoita Creek located about three miles north of Patagonia, Arizona. It is within the Coronado National Forest, which is open to the public for recreational uses including hiking, camping, birdwatching, mountain biking, fishing and boating, and visiting historic areas (USFWS, 2021). Restoration treatments focus on the use of RDS to reduce erosion impacts and sediment pollution downstream and to assess effectiveness and impacts on ecosystem services. The area has been grazed in the past, but cattle are now excluded by fencing.
This watershed provides an opportunity for large-scale experimentation due to its structurally repetitive landscape consisting of roughly 90 physically similar sub-basins, each approximately 2–5 ha. The project includes treatment and control sites. The project is a collaboration between Borderlands Restoration Network, the Biophilia Foundation, and the US Geological Survey (USGS).
Babacomari Ranch
The Babacomari Ranch, located in Santa Cruz County, is one of the oldest land grant ranches in Arizona. The land is still used for cattle grazing, with management in collaboration with the University of Arizona and the National Resources Conservation Service. The Vaughn Canyon channel restoration includes “sandbagging,” the construction of 5 gabions, and other smaller RDS. Restoration has been a collaboration between Borderlands Restoration Network and the USGS, funded in part by the Walton Family Foundation (Petrakis et al., 2021).
Data
For this study we utilized a database of publications on El Coronado and Cuenca Los Ojos that had previously been compiled by the authors. The database was populated in early 2020 with publications provided by the property owners, supplemented by searches for property and parcel names (e.g., El Coronado, Cuenca Los Ojos, Rancho San Bernardino) in Scopus and Web of Science. Publications included peer-reviewed literature and student theses and reports. It has been maintained with additional contribution from the property owners and managers, who are aware of research conducted on the properties; publication alerts; and reference tracing. In 2021 we added sources related to Smith Canyon and Babacomari using the same methods. Eighteen records in the database were relevant to our research question and were included in this analysis.
Because not all impacts resulting from RDS-based restoration had been documented in the scientific literature, we supplemented the literature review with informal interviews with three key stakeholders who had installed, monitored, and/or observed the interventions for a period of at least 10 years and as much as 40 years (Supplementary Table S1, for interview data). The three interviewees were selected for their long history with and depth of knowledge about the case study sites. To provide structure and reduce bias, interviews were conducted using the Intergovernmental Science-Policy Platform on Biodiversity and Ecosystem Services (IPBES) categories of nature’s contributions to people (NCP; see Supplementary Table S2) (Díaz et al., 2018) as a list of prompts. Interviewees were cued with each IPBES category and asked to provide their knowledge of relevant impacts, positive or negative. We note in our results which impacts were documented by published studies and which were observational.
Analytical Methods
Our objective for this study was to identify the multiple societal benefits of watershed-scale use of RDS as an NbS. Following Smith et al. (2019), we analyzed our source material to identify the contribution of the intervention (RDS-based watershed restoration) in terms of ecosystem functions, nature-based solutions, and the Sustainable Development Goals. In this stage, we also evaluated the data for any indication of trade-offs between competing ecosystem services (Chausson et al., 2020).
In phase 1, we reviewed each publication that met the source criteria (see Data) and interviewee observations for evidence of ecosystem functions served by restored watersheds. We catalogued all relevant research findings in a spreadsheet, labeling the ecosystem function and noting the evidence to support each. We then consolidated the catalogued list of ecosystem services to standardize terminology and eliminate duplicates.
In phase 2, we utilized Díaz et al. (2018) framework for categorizing NCPs, a form of NbS, to group similar categories of the ecosystem services we identified in phase 1. Following methods developed by Smith et al. (2019), we associated each ecosystem function with the relevant NCP. These associations are indicated by numerical tags in Figure 2.
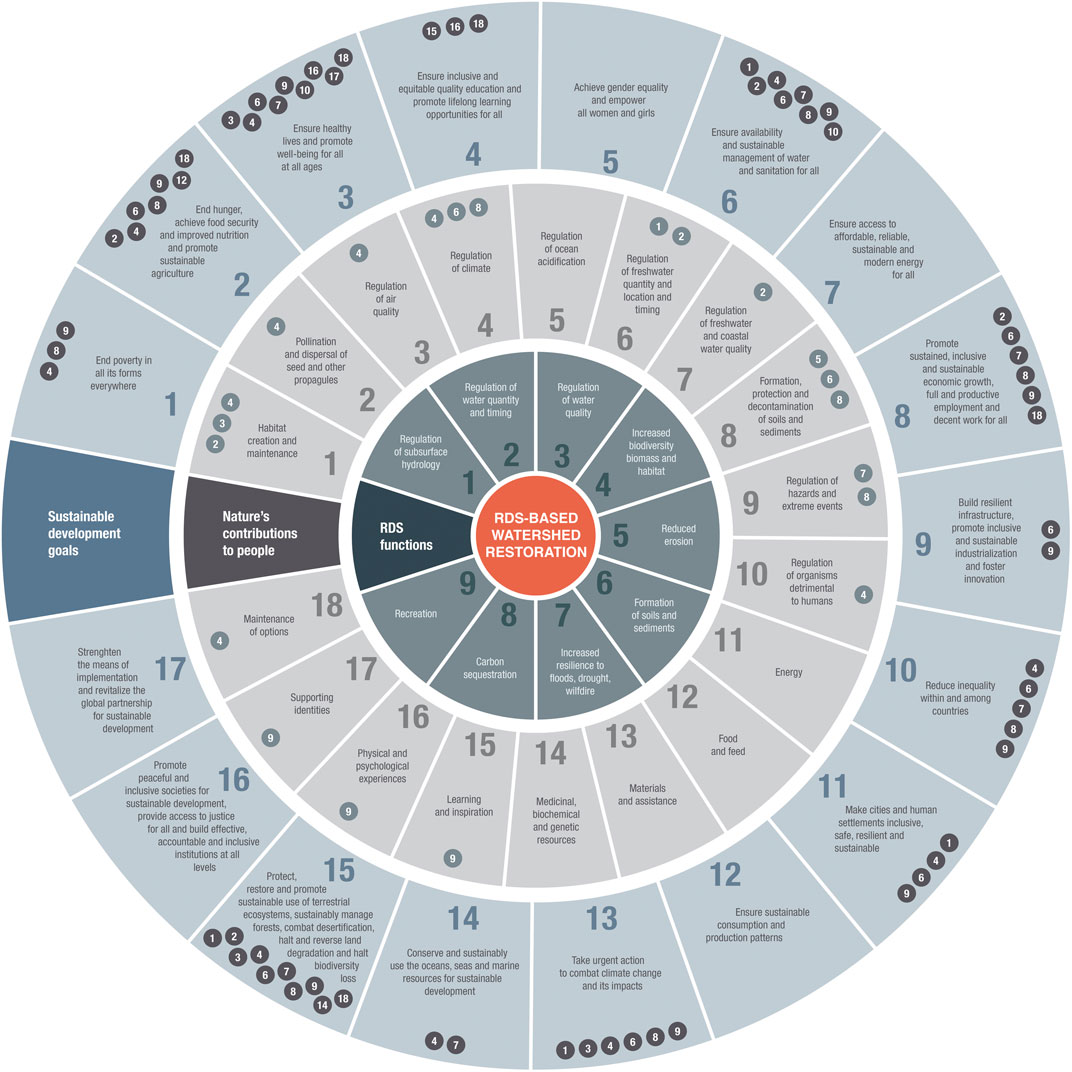
FIGURE 2. Summary of the impact of RDS-based watershed restoration on ecosystem functions, on nature’s contributions to people (NCPs), and on the United Nations Sustainable Development Goals (SDGs), showing the impact of each function on each NCP, and the contribution of the NCPs to each of the SDGs. Figure adapted from similar figure by Smith et al. (2019).
Finally, in phase 3, following Smith et al. (2019), we assessed the impacts of the RDS-based watershed restoration on the Sustainable Development Goals (SDGs). Each of the connections between the NCPs identified in phase 2 are indicated by numerical tags in Figure 2.
This study was conducted in adherence with the code of ethics of the Society for Ecological Restoration.
Results
Ecosystem Functions
Here, we report the ecosystem functions served by RDS-based watershed restoration in drylands, as identified through our analysis of scientific literature and interviews associated with the four case study sites. Some ecosystem functions could be categorized under more than one NbS; for purposes of simplification, we have placed each according to the NbS with which it was most closely aligned (Díaz et al., 2018).
Formation, Protection, and Decontamination of Soils and Sediments
Erosion
Riparian areas and dry washes at our study sites were subject to significant erosion due to historical land uses. Incision of waterways ranged from a few centimeters to 9 m (Barry, 2014). The earliest RDS installed at El Coronado were constructed specifically for the purpose of controlling erosion (Barry, 2014). RDS help replicate the functions of healthy riparian ecosystems, where tree roots and vegetative debris would normally stabilize the soil, slow the speed of water, and reduce the energy of water moving downstream, reducing the water’s ability to transport sediments. Some years after the installation of RDS, vegetation that has re-established can once again serve these functions (US Fish and Wildlife Service, US Bureau of Reclamation, US Forest Service, and Cross Watershed Network, 2018).
Modeling of the 7.7 km2 Turkey Pen watershed at EC using the Soil and Water Assessment Tool (SWAT) found that 356–483 tons of sediment would likely be yielded from this watershed annually, given no management for erosion control, i.e., had no check dams been installed (Norman and Niraula, 2016). Modeling showed the installed RDS reduced sediment yield by 178–242 tons, or approximately by half. Conversely, a LiDAR study of the Babacomari Ranch found equal amounts of erosion (528 m2) and deposition (497 m2) surrounding a large gabion (∼10 m wide); however, the authors note that this likely reflects the impacts of additional construction and rehabilitation of gabions during the study period, which may have increased erosion temporarily (Norman et al., 2019).
Formation of Soils and Sediments
In addition to preventing erosion, RDS can also help reverse the effects of past erosion by trapping sediments upstream of the structures (Fandel, 2016). This sedimentation causes streambeds to rise, reversing past incision of waterways (US Fish and Wildlife Service, US Bureau of Reclamation, US Forest Service. and Cross Watershed Network, 2018). Installation of RDS can be iterative and adaptive. If the structures reach their full capacity in trapping sediments, additional structures can be built atop older structures (Fandel, 2016), raising the streambed even further, ultimately (or ideally) reattaching the streambed to its floodplain.
Storing, Filtering, and Transforming Nutrients
Climate modeling has predicted increases in extent, frequency, and severity of wildfires in the western United States, leading to erosion and depletion of soils. Elsewhere, these fires have led to the permanent loss of N and C through geomorphic tipping points (Falk, 2013; Youberg et al., 2013). Delivery of nutrient-rich sediments to downstream water bodies negatively impacts water quality and aquatic ecosystems. A study of the ability of RDS to capture carbon and nutrients following wildfire found that both organic carbon and nitrogen in the sediments trapped post wildfire are twice (at 0.1-m depth) to 10 times (at 0.3-m depth) greater behind RDS than in off-channel soils in recently installed structures, though structures that had been in place for 30–40 years had in-channel organic carbon levels that more closely resembled off-channel levels (Callegary et al., 2021). RDS afforded the opportunity for quick reburial of mobilized biomass, soil organic matter, and charred organic matter following an occurrence of wildfire. If undisturbed, buried carbon is subject to soil and plant microbial processes, which result in some CO2 being respired back into the atmosphere, some incorporated into plant roots and mycelia, and some mineralized or left inorganically in long term storage in soil, depending on conditions.
Regulation of Freshwater Quantity, Location, and Timing
Freshwater Quantity and Timing
Strong evidence documents positive impacts on freshwater quantity and availability throughout the year. Multiple studies have observed an overall increase in stream flow in areas where RDS have been installed throughout the landscape (Barry, 2014; Buckley and Nabhan, 2016; Norman et al., 2016; Wilson and Norman, 2018). At El Coronado, flow volume increased 28% per unit of watershed area, relative to an untreated watershed (Norman et al., 2016). Duration of flow has also increased; a once-dry river now flows perennially for 9 km at CLO (Barry, 2014; Norman et al., 2019). These effects extend both downstream (5–10 km) and upstream (1 km) from the restoration area (Wilson and Norman, 2018). There have also been observations of pools of water present on hillsides during dry months (US Fish and Wildlife Service, US Bureau of Reclamation, US Forest Service. and Cross Watershed Network, 2018), and interviews indicate that the San Bernardino ciénega, historically a much larger wetland than in recent times, located in a watershed that has now been restored using RDS, has at least doubled in size since 2005.
Subsurface Hydrological Processes
Because RDS slow the flow of water over land and through waterways, they create conditions in which more water can soak into the soil, affecting subsurface hydrology. Using a heat transport method to measure infiltration flux, Fandel (2016) ran a series of weather simulations which, when combined with field measurements, found that a single gabion could increase total aquifer recharge, with simulations ranging from no impact to +225%, with the most likely scenarios depicting a 10.8% increase (Fandel, 2016). Modeling also shows increased subsurface hydraulic conductivity and accentuated lateral flow contributions to streamflow (Norman et al., 2019).
Observations indicate a decrease in the depth to water table due to sedimentation (unquantified) (Barry, 2014), which CLO land managers report occurred during a 15-years drought. Similarly, gabions installed in the upstream tributaries are likely impacting areas further downstream by raising the water table (Norman et al., 2014). Other observations include increased soil moisture on treated hillslopes, with pools of water observed during dry months (US Fish and Wildlife Service, US Bureau of Reclamation, US Forest Service. and Cross Watershed Network, 2018).
Regulation of Freshwater Quality
Freshwater Quality
One study anecdotally reported lower turbidity in a watershed treated with RDS than in an untreated watershed. During the study, a rainfall event of 5.2 cm occurred, with an average rate of 20.8 mm/h, over 150 times the average for the season. After the peak, observations showed no significant sand or silt deposits in the treated waterway, and the water flow was reportedly clear (Norman et al., 2016).
Regulation of Hazards and Extreme Events
Flood Resilience
In a model versus field measurement experiment, researchers found that the Soil Water Assessment Tool (SWAT) accurately (within 2.3%) predicted the hydrograph of a control watershed during several storm events of the seasonal monsoon in a southeast Arizona watershed. The SWAT model overestimated the hydrograph of the Turkey Pen watershed, which had been treated with over 2000 RDS, for which the SWAT model had not been calibrated, by 119.8%. Thus, stream gauges installed in the treated and untreated (control) waterways during monsoon season indicated that during inundation events the treated watershed had a lower runoff response and reduced peak flow, as modeled (Norman et al., 2016). Though baseflows are higher in treated streams, as discussed above (3.1.2.1), peak flows appear to be dampened. During the study, the aforementioned heavy rain event had little observable impact on the treated watershed. The authors note that after the event the only evidence of such heavy flooding was that the grass in the floodplain “had lain down” (Norman et al., 2016).
Drought Resilience
Relative to an untreated watershed, vegetation in a treated watershed at CLO was more resilient to dry conditions (Wilson and Norman, 2018). In the treated watershed, vegetation greenness, as measured by the Landsat Thematic Mapper using the normalized difference vegetation index (NDVI), was decoupled from spring precipitation, remaining greener than the control site even during seasons with low rainfall. The study found that RDS installation has increased water availability in the restored area, allowing vegetation to be less dependent on precipitation. Land managers also note that storing water in vegetated soil is preferable to storing water in open ponds because it is subject to less evaporation and therefore provides another adaptation to drought.
Catastrophic Wildfire
Because treated watersheds are greener and plant vegetation contains more water (Norman et al., 2019), it has been speculated that wildfires may be less severe in RDS-treated watersheds than in watersheds that are degraded and untreated. Land managers have speculated that the Horseshoe 2 fire in 2011, for example, which burned through the Turkey Pen watershed, may have been less intense due to restoration; however, other management treatments in and round the area make it difficult to assess drivers. Our research found no studies of the impact of RDS-based watershed restoration on the intensity of wildfires at the case study sites to date.
Habitat Creation and Maintenance
Biomass/Habitat
Studies of vegetative biomass and habitat have reported denser vegetation (US Fish and Wildlife Service, US Bureau of Reclamation, US Forest Service. and Cross Watershed Network, 2018), higher levels of greenness that increase over time, measured by the NDVI (Wilson and Norman, 2018); higher water content in vegetation, measured by the normalized difference infrared index (NDII) (Wilson and Norman, 2018); and increased perennial vegetation cover, assessed by field observations (Wilson and Norman, 2019). A time series of remote sensing data using the NVDI as a proxy for plant biomass showed that treatment sites increased in vegetation cover despite a general trend of below-average precipitation, in contrast to untreated sites, where vegetation decreased (Figure 3) (Norman et al., 2014).
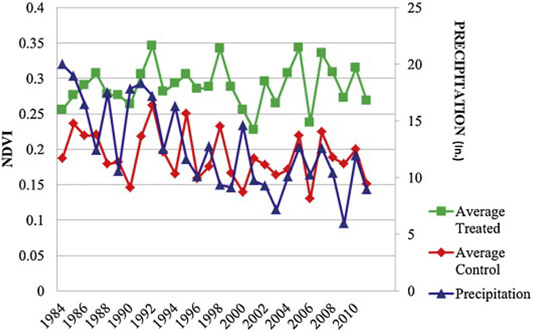
FIGURE 3. Average NDVI plotted over time in San Bernardino (CLO) at treated and control sites, in relationship to annual precipitation (reprinted from Norman et al., 2014).
Biodiversity (Species, Habitats, and Genes)
In addition to an overall increase in biomass, there was also an increase in biodiversity (Cárdenas-García and Olguín-Villa, 2013; Barry, 2014). Using transects and vegetative surveying, researchers have observed an increased richness of plant species in riparian habitats, including more grass species (both native and non-native), more young age classes of trees that were not present before gabions were constructed, and many more aquatic plant species (Norman et al., 2014). Waterway restoration enabled the recolonization of native fish (Barry, 2014). Land managers at CLO also report increased presence of endangered species following watershed restoration, including jaguar (Panthera onca, Main, 2021), ocelot (Leopardus pardalis, pers. comm.), and black bear (Ursus americanus, Coronel-Arellano et al., 2018).
Regulation of Climate
Soil Carbon
Two studies have assessed the impact of RDS treatment on carbon sequestration in soil. A study at El Coronado assessed the ability of RDS of differing ages to capture soil carbon post-wildfire (Callegary et al., 2021). Results showed higher levels of soil carbon behind more recently constructed RDS: in-channel soil organic matter was twice (at 0.1-m depth) to 10 times (at 0.3-m depth) greater behind RDS than in off channel soils. At older structures, which had less remaining capacity to trap new post-wildfire sediments, there was little difference between in-channel and off-channel sediments. Mean additional carbon storage in the Turkey Pen watershed was 746–935 tons of CO2 equivalent (tCO2e) per ha of sedimentation behind RDS1,2, an amount comparable to wetlands (Callegary et al., 2021).
A separate study at the same site found 40% greater soil organic carbon in a treated channel compared to a control channel (Leger et al., 2019). Multiple soil samples in a 290 m2 riparian area behind one RDS contained an average of 5.6 tCO2e, but there was high variability (2.1-17.4 tCO2e). The mean value equated to soil carbon storage of 193 tCO2e per ha over an estimated 30 years of active sediment entrapment, a lower value than that found by Callegary et al. (2021) (Table 1). Differences in the studies’ sample sizes and sampling methodologies and potential localized effects of post-fire, carbon-rich sedimentation confound the results and highlight the need for additional research.
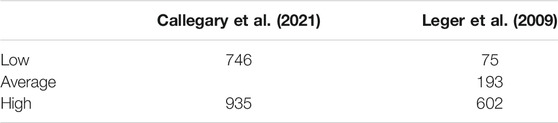
TABLE 1. Potential soil carbon storage as a result of RDS-based riparian restoration, reported in studies by Callegary et al. (2021) and Leger et al. (2009), converted to tCO2e per ha
Regulation of Micro-climate
Restoration of riparian vegetation that results from RDS installation creates a micro-climate along waterways, reducing local water and air temperatures through shading and evapotranspiration. A study of the endangered Yaqui catfish (Ictalurus pricei) found that the species was more likely to be present in areas with native riparian restoration and less likely where the waterways were bordered by cropland or shrubland (Hafen, 2018). Land managers have observed that the increase in native riparian vegetation following restoration creates cooler aquatic conditions and habitat more suitable to native species.
Physical and Psychological Experiences
Physical and Mental Health
A study that mapped social value preferences of residents of Patagonia, Arizona, within the Sonoita Creek watershed, which includes Smith Canyon, showed that across 12 measured values (aesthetics, biological diversity, cultural, economic, future generations, historical, intrinsic, learning, life sustaining, recreational, spiritual, and therapeutic), residents placed a high social value along Sonoita Creek and its main tributaries, particularly within the town of Patagonia and surrounding Patagonia Lake. Life sustaining, future generations, aesthetics, and biological diversity garnered the highest scores (Petrakis et al., 2020). Access to natural green spaces has been shown elsewhere to address both physical and mental health through multiple mechanisms (Staats, 2012).
Additionally, land managers have observed that volunteers and others who construct RDS find satisfaction in doing so. One restoration project manager who supervised a 10-person inmate crew wrote that “a unique rapport took shape, along with unanticipated levels of respect and pride in the work at hand” and detailed many anecdotes of comradery, humor, and realization of the positive impact that was being made (Seibert, 2015).
Supporting Identities
Preserving and Utilizing Indigenous Knowledge
The RDS techniques deployed at the four study sites were adapted from techniques developed and utilized by Indigenous peoples in the region. The use of these techniques in modern conservation draws attention to the wisdom and skill of the peoples who inhabited these lands for centuries (Phillips, 2009). In addition, workshops offered by CLO to tribes in the region have enabled RDS techniques to be reintroduced on other tribal lands.
Preserving Ranching Heritage
For the last 300 years, the Sky Islands grasslands have been used by ranchers for sheep and cattle grazing (Cole and Cole, 2015). The use of unsustainable practices has led to land degradation and made the region less hospital for ranching, increasing the likelihood of conversion for other, even less sustainable uses, such as irrigated agriculture (Pool et al., 2014). Watershed restoration on ranchlands, coupled with sustainable ranching practices, can help make working lands more hospitable to wildlife and more viable over the long term, thereby maintaining ranching culture and heritage (Vásquez-León et al., 2003).
Maintenance of Options
Option Value
Protection of a wide variety of species, populations, and genotypes provides options for future generations to enjoy and utilize natural resources (Faith, 2016). Biodiversity also increases resilience to threats, such as a warmer climate (Isbell et al., 2015). Interviewees reported that current extractive economic activity (e.g., mining) is reducing options for the region’s significant nature-based economy, which is based on tourism, outdoor recreation, sustainable harvest, and land restoration. By restoring landscapes in which nature-based economic activity can take place, RDS-based watershed restoration increases options for a sustainable economy, both now and in the future.
Contribution to Sustainable Development Goals
Following Smith et al. (2019), we traced the impacts of RDS-based watershed restoration and nature’s contributions to people (Díaz et al., 2018) to the United Nations Sustainable Development Goals (SDGs). Relating ecosystem restoration to the SDGs underscores their potential contribution to factors that improve the lives of people. Results are shown in Figure 2.
Trade-Offs
A significant concern in NbS research is whether solutions to one problem incur trade-offs in the form of increased salience of another problem. For example, Chausson et al. (2020) found that in some cases NbS aiming for increased water availability came at a cost to protection against climate impacts. However, Chausson et al. (2020) suggested that interventions using natural or semi-natural ecosystems, as compared to non-native species, showed more synergies than trade-offs. Our findings support this conclusion. The only trade-off we identified was temporal: one study observed a construction-related decrease in vegetation near gabions until approximately 2–4 years after installation, after which there was a net increase in vegetation (Wilson and Norman, 2018). We found no evidence of consequential trade-offs among these factors in publications or interviews with stakeholders. On the four case study sites, we found water availability, biomass cover, erosion control, flood resilience, and carbon sequestration were mutually increased as a result of the intervention.
Costs
A first approximation of costs can be calculated using information from an RDS construction project conducted at the Babacomari site. Figures provided by the Borderlands Restoration Network indicate that medium-sized check dams could be built for approximately $65 USD each in 2019, assuming a work crew (1 foreman, 5 laborers) dedicating 2.25 h per medium-sized check dam (D. Seibert, pers. comm.). Given the density of structures installed at EC and CLO, labor costs for a 1-km stretch of waterway could be restored for approximately $1,600 USD. These calculations do not include the costs of any materials or equipment. Consistent with many conservation interventions (Cook et al., 2017; Iacona et al., 2018), further documentation of costs is necessary to fully assess the costs and benefits of restoration.
Discussion
Summary
RDS have been utilized around the world, for centuries or longer (Abbasi et al., 2019; Norman, 2020). Their use to plug gullies, control erosion, increase sedimentation, trap precipitation, increase water infiltration, and make land more productive and suitable for agriculture has been documented in various manuals and solutions databases, such as the World Overview of Conservation Approaches and Technologies (WOCAT) Sustainable Land Management database (WOCAT, 2021). Yet there has been little attention on their potential use as an NbS for climate mitigation and adaption.
Over 40% of the earth’s land area is classified as desert, semi desert, arid and semi-arid grasslands or rangelands, containing 44% of the world’s cultivated systems (Reid et al., 2005). One third of the earth’s human population inhabit these areas, 40% of whom have livelihoods directly affected by desertification. Ephemeral and intermittent streams, the lifeblood for these areas, make up more than half the combined length of all rivers and streams globally (Acuna et al., 2014). The evidence from our case studies suggests that RDS-based interventions offer a means to adapt to the conditions of an altered climate, including higher temperatures, more variable precipitation, and more extreme weather events. More research is necessary to assess the range of eligible application, but their use in drylands across the globe suggest they could provide relief and resilience for some of the 2.1 billion human inhabitants of drylands worldwide.
Addressing Barriers to Replication and Scalability
This Frontiers special research topic, Nature-Based Solutions for Natural Hazards and Climate Change, emphasizes the need for research to identify and scale replicable options for NbS. As conservation practitioners and funders, we are particularly interested in issues of replicability and scalability and ask: if RDS-based watershed restoration is feasible, cost-effective, and impactful, why is it not more widely utilized?
Currently, there is no analysis of barriers to restoration in the Sky Islands region, so we used the United Nations Decade on Ecosystem Restoration (hereafter, UN Decade) strategy document (United Nations Environment Program, 2020), which identifies global barriers to widespread ecosystem restoration, as a guiding framework. Here we elaborate on five of the barriers: 1) limited awareness of land degradation and the benefits of restoration, 2) lack of legislation, policies, and regulation, 3) limited technical capacity, 4) limited finance, and 5) need for more research.3
Limited Awareness of Land Degradation and the Benefits of Restoration
Because land degradation in the Sky Islands has occurred over a period of 200–300 years, changes to the land can be less obvious within a single generation (i.e., shifting baseline, Pauly, 1995). As a result, there is limited awareness of the historical functionality of ecosystems, the extent and costs of degradation, or the potential societal benefits that could accrue with restoration (United Nations Environment Program, 2020).
Positive impacts of RDS-based watershed restoration have been documented in scientific papers across multiple disciplines. Our aim with this paper was to catalog and synthesize the evidence in support of the intervention, based on work at four case study sites that have collectively been the subject of research in multiple disciplines. We found strong evidence for impacts on freshwater quantity and timing. Highlights include a 28% increase in watershed flow volume (Norman et al., 2016) and longer annual duration of flow (Barry, 2014). We also found positive impacts on formation of soils and sediments, freshwater quantity and timing, resilience to flooding and drought, vegetation, biodiversity, pollination and seed dispersal, and human use of the landscape. We hope this paper and associated outreach materials help address the barrier of awareness of the societal value and benefits that accrue as a result of RDS-based watershed restoration.
Lack of Legislation, Policies, and Regulation
The UN Decade identifies a lack of institutional mechanisms that incentivize investments in large-scale restoration (United Nations Environment Program, 2020). The IUCN Global Standard for Nature-based Solutions (IUCN, 2020) provides definitional criteria, a systematic learning framework, and recommendations for governance. In addition, the guiding principles offered by the Nature-Based Solutions Initiative, which have been endorsed by 20 organizations (Nature Based Solutions Initiative, 2020) and which are described below, can be used to inform the development of new legislative, policy, and regulatory tools in the region.
1) NbS are not a substitute for a rapid fossil fuel phase-out. Carbon markets are the most likely source of short-term funding for NbS (Seddon et al., 2021). However, over the medium to long term, we recommend constraint of restoration in the Sky Islands as an offset for carbon emissions elsewhere, with emphasis instead on phasing out fossil fuels.
2) NbS involve the protection and/or restoration of a wide range of naturally occurring ecosystems. Recent global NbS implementation has included afforestation at the expense of other vital ecosystems, such as grasslands. Restoration of woody vegetation associated with RDS installation in the Sky Islands should be done in riparian and wetland ecosystems where trees grew in the past or where they regenerate naturally.
3) NbS are implemented with full engagement and consent of Indigenous peoples and local communities, apply social safeguards, and build human capacity to adapt to climate change. RDS originated in the region as Indigenous technology, and their use resonates with many local communities. IUCN guidance provides best practices for Indigenous and community conservation areas (Borrini et al., 2013) and privately protected areas (Mitchell et al., 2018).
4) NbS sustain, enhance, or support biodiversity. Project goals at case study sites included restoration of habitat for threatened and endangered wildlife, providing a template for regional efforts. RDS can support different constellations of goals and resources, but attention must be given to potential trade-offs between biodiversity and ecosystem services.
Limited Technical Capacity
Limited technical capacity to design and implement restoration initiatives can hinder their widespread use (United Nations Environment Program, 2020). As an example of low-tech, process-based restoration (Wheaton et al., 2019), many of the structural interventions of RDS-based watershed restoration can be built by hand. Their placement requires expertise – though not to the same degree as engineered solutions – but RDS can be built by volunteers or workers with few pre-existing labor market skills. Much of the wisdom on placement and construction of RDS originated in Indigenous communities, where knowledge and skills were communicated orally and experientially. Organizations such as Cuenca Los Ojos and the Borderlands Restoration Network are using similar methods, through workshops, peer-to-peer exchanges, volunteer workdays, and youth trainings, to share the knowledge today.
While low-tech methods are not a complete solution to watershed restoration, their use opens opportunities for community engagement in restoration, which has potentially transformational effects on communities and their local political economy (Pritzlaff, 2018) and the potential for restoration across a larger land area than would otherwise be possible. As an illustration, low-tech, process-based restoration techniques are considered applicable in wadable streams (i.e., fifth order streams or less), which account for roughly 90% of the perennial stream length in a typical drainage network (Wheaton et al., 2019, p.4).
Limited Finance
Lack of financial resources for conservation is a perpetual concern (Clark, 2007), and investment can be difficult to find due to mismatches in terms of timescale and beneficiary. Restoration at our four case study sites has been funded primarily through philanthropy, including funding from private landowners, charitable foundations, and government agencies. Funding from these sources are limited, however, and additional sources of revenue are needed. One option is payments for ecosystem services (PES) schemes, including carbon credits. Based on more than 2 years of investigation into this topic, including consultation with researchers, restoration professionals, and experts on market creation, we believe carbon markets are a viable financing mechanism for three reasons.
1)Preliminary evidence suggests carbon can be sequestered by RDS. Based on our case study sites, anywhere from 70-930 tCO2e per hectare can be stored in soils alone, which at the upper end is comparable with sequestration rates in wetlands, which currently receive substantial investment from carbon markets (Zomer et al., 2017)
2)Some suitable carbon markets are already in place, and new markets are coming online each year. Carbon markets are seeing record market volume and value in 2021, with markets on track to reach $1 billion in transactions this year (Ecosystem Marketplace, 2021).
3)Carbon market funding is scalable. We estimate that 37 million hectares of watersheds in the Sky Island region need restoration, potentially storing 13.8 million tCO2e. Callegary et. al. (2021) estimated 40 million tCO2e could potentially be stored in restored watersheds of forested regions of the entire southwestern United States. These figures approximate carbon captured in soils, not carbon stored in standing trees of restored riparian areas, where some researchers suggest most carbon is stored (please see Supplementary Material (Calculations)). Carbon sequestration is but one potential benefit of RDS-based watershed restoration, but with demand for carbon credits growing over time, carbon markets offer a feasible mechanism to finance regional-scale restoration.
Need for More Research
There is evidence of positive impacts of RDS-based watershed restoration on hazard mitigation and climate resilience at our case study sites. Yet many research gaps remain. At the case study sites, there has been less attention on impacts on freshwater quality, biodiversity, and resilience to catastrophic wildfire. Given anticipated impacts on these factors due to climate change, these gaps urgently need to be filled. Other ecosystem functions, such as pollination, seed dispersal, and air quality, have not yet been investigated.
More research to assess the carbon sequestration potential of RDS-based watershed restoration is necessary, particularly to understand the contribution of both above ground and below ground plant biomass and fallen large wood. Additionally, most studies on the ecosystem impacts of restoration have been retroactive. More experiments like the study recently initiated at Smith Canyon are needed (Petrakis et al., 2021).
Finally, although we reported some information about the cost of constructing RDS, we lack sufficient documentation of the costs of watershed-scale restoration, information that is necessary to assess the financial viability of PES programs and carbon market-financed restoration projects.
Conclusion
Dryland watershed restoration provides a range of environmental and social benefits. This low tech restoration method has been practiced for centuries with success, and the skills can easily be taught to the inhabitants of the areas where it is most needed, providing local employment and livelihood opportunities. The materials needed for construction are often found on site. The practices themselves are adaptable, should field monitoring suggest alterations based on changing hydrologic or other conditions.
Dryland watershed restoration was not included by Fargione et al. (2018) as a natural climate solution for the United States. We believe the results of the case studies presented here illustrate the range of ecosystem functions served by RDS-based restoration and provide a basis for exploring opportunities to pursue dryland watershed restoration as nature-based solution to climate mitigation and adaptation.
Data Availability Statement
The original contributions presented in the study are included in the article and its Supplementary Material, and further inquiries can be directed to the corresponding author.
Ethics Statement
Ethical review and approval was not required for the study on human participants in accordance with the local legislation and institutional requirements. Written informed consent for participation was not required for this study in accordance with the national legislation and the institutional requirements.
Author Contributions
Both authors contributed to review and summary of published literature and to writing the manuscript. JG developed the theoretical framework, conducted interviews, and analyzed interview data. RP analyzed carbon sequestration and carbon finance potential. Both authors have made substantial, direct, and intellectual contribution to the work and approved it for publication.
Conflict of Interest
RP is founder and chairman and JG is president/CEO of the Biophilia Foundation, which has funded some of the work conducted at the case study sites. JG has worked as a conservation strategist for a coalition of organizations in the region that includes Cuenca Los Ojos, the Biophilia Foundation, and the Borderlands Restoration Network, which are closely involved with the case study sites.
Publisher’s Note
All claims expressed in this article are solely those of the authors and do not necessarily represent those of their affiliated organizations, or those of the publisher, the editors and the reviewers. Any product that may be evaluated in this article, or claim that may be made by its manufacturer, is not guaranteed or endorsed by the publisher.
Acknowledgments
The authors would like to thank the proprietors of the case study sites for their support of the research cited herein and their ongoing work restoring the Sky Islands ecosystem.
Supplementary Material
The Supplementary Material for this article can be found online at: https://www.frontiersin.org/articles/10.3389/fenvs.2021.679189/full#supplementary-material
Footnotes
1To facilitate comparison, we have converted units of measurement from both studies to CO2 equivalent (CO2e) and hectares.
2Restored area was calculated to be 2.6 ha, a sum of sediment areas behind 2000 RDS in the 770-ha watershed (0.34% of land area).
3The UN Decade identifies an additional barrier that we do not discuss here: low pressure to invest in ecosystem restoration relative to other sectors, such as health care or education (United Nations Environment Program, 2020). We recognize the extent of this issue in the US, where environmental causes received only 12% of philanthropy, as of 2009 (Ramutsindela et al., 2011), but lack sufficient knowledge of other sectors in the Sky Islands to compare.
References
Abbasi, N. A., Xu, X., Lucas-Borja, M. E., Dang, W., and Liu, B. (2019). The Use of Check Dams in Watershed Management Projects: Examples from Around the World. Sci. Total Environ. 676, 683–691. doi:10.1016/j.scitotenv.2019.04.249
Acuña, V., Datry, T., Marshall, J., Barceló, D., Dahm, C. N., Ginebreda, A., et al. (2014). Conservation. Why Should We Care about Temporary Waterways. Science 343 (343), 1080–1081. doi:10.1126/science.1246666
Barry, T. (2014). Transborder Drylands Restoration: Vision and Reality after Three Decades of Innovative Partnerships on the U.S.-Mexico Border. SAPIENS 7 (2), 1–8.
BirdLife International (2020). The World Database of Key Biodiversity Areas. Developed by the Key Biodiversity Areas Partnership: BirdLife International, IUCN, Amphibian Survival Alliance, Conservation International, Critical Ecosystem Partnership Fund, Global Environment Facility, Global Wildlife Conservation, NatureServe, Royal Society for the Protection of Birds, World Wildlife Fund and Wildlife Conservation Society. Available at: http://www.keybiodiversityareas.org (Accessed June 20, 2020).
Borrini, G., Dudley, N., Jaeger, T., Lassen, B., Pathak, N., Phillips, A., et al. (2013). Governance of Protected Areas: From Understanding to Action. Gland, Switzerland: IUCN.
Buckley, S., and Nabhan, G. P. (2016). Food Chain Restoration for Pollinators: Regional Habitat Recovery Strategies Involving Protected Areas of the Southwest. Nat. Areas J. 36 (4), 489–497. doi:10.3375/043.036.0414
Callegary, J. B., Norman, L. M., Eastoe, C. J., Sankey, J. B., and Youberg, A. (2021). Post-wildfire Carbon and Nitrogen Sequestration Potential in Entisols of forest Ecosystems of Southwest USA. Air Soil Water Res. 14, 1–18. doi:10.1177/11786221201001768
Cárdenas-García, M., and Olguín-Villa, M. C. (2013). “Bird List of San Bernardino Ranch in Agua Prieta, Sonora, Mexico. 2013,” in Comps. Merging Science and Management in a Rapidly Changing World: Biodiversity and Management of the Madrean Archipelago III. 2012 May 1-5; Tucson, AZ. Proceedings. RMRS-P-67. Editors G. J. Gottfried, P. F. Ffolliott, B. S. Gebow, L. G. Eskew, and L. C. Collins (Fort Collins, CO: U.S. Department of Agriculture, Forest Service, Rocky Mountain Research Station).
Critical Ecosystem Partnership Fund (2020). Madrean pine-oak Woodlands. Available at: https://www.cepf.net/our-work/biodiversity-hotspoadreanean-pine-oak-woodlands(Accessed June 20, 2020).
Chausson, A., Turner, B., Seddon, D., Chabaneix, N., Girardin, C. A. J., Kapos, V., et al. (2020). Mapping the Effectiveness of Nature‐based Solutions for Climate Change Adaptation. Glob. Change Biol. 26 (11), 6134–6155. doi:10.1111/gcb.15310
Conservation International (2020). Biodiversity Hotspots. Available at: https://www.conservation.org/priorities/biodiversity-hotspots (Accessed December 4, 2020).
Cole, A. T., and Cole, C. (2015). An Overview of Aridland Cienagas with Proposals for Their Classification, Restoration, and Preservation. New Mexico Botanist. (Special Issue 4).
Cook, C. N., Pullin, A. S., Sutherland, W. J., Stewart, G. B., and Carrasco, L. R. (2017). Considering Cost Alongside the Effectiveness of Management in Evidence-Based Conservation: A Systematic Reporting Protocol. Biol. Conservation 209, 508–516. doi:10.1016/j.biocon.2017.03.022
Coronel-Arellano, H., Lara-Díaz, N. E., Moreno, C. E., Gutiérrez-González, C. E., and López-González, C. A. (2018). Biodiversity Conservation in the Madrean Sky Islands: Community Homogeneity of Medium and Large Mammals in Northwestern Mexico. J. Mammalogy 99 (2), 465–477. doi:10.1093/jmammal/gyx151
Costanza, R., de Groot, R., Sutton, P., van der Ploeg, S., Anderson, S. J., Kubiszewski, I., et al. (2014). Changes in the Global Value of Ecosystem Services. Glob. Environ. Change 26, 152–158. doi:10.1016/j.gloenvcha.2014.04.002
DeLong, S., and Henderson, W. (2012). Can Erosion Control Structures in Large Dryland Arroyo Channels lead to Resilient Riparian and Cienega Restoration? Observations from LiDAR, Monitoring and Modeling at Rancho San Bernardino, SonoraMX (#EP23C-0825). San Francisco, California: Poster presented at the American Geophysical Union (AGU), Fall meeting. Available at: http://fallmeeting.agu.org/2012/eposters/eposter/ep23c-0825/.
Deyo, N. S., Van Devender, T. R., Smith, A., and Gilbert, E. (2012). “Documenting the Biodiversity of the Madrean Archipelago: An Analysis of a Virtual flora and Fauna,” in Comps. 2013. Merging Science and Management in a Rapidly Changing World: Biodiversity and Management of the Madrean Archipelago III; 2012 May 1-5. Editors G. J. Gottfried, P. F. Ffolliott, B. S. Gebow, L. G. Eskew, and L. C. Collins (Tucson, AZ: US Department of Agriculture, Forest Service, Rocky Mountain Research Station). Proceedings.
Díaz, S., Pascual, U., Stenseke, M., Martín-López, B., Watson, R. T., Molnár, Z., et al. (2018). Assessing Nature's Contributions to People. Science 359 (6373), 270–272. doi:10.1126/science.aap8826
Dimmitt, M. A. (2015). “Biomes and Communities of the Sonoran Desert Region,” in A Natural History of the Sonoran Desert. Editors S. J. Phillips, P. Wentworth Comus, and L. M. Brewer. 2nd edition (Tucson: Arizona-Sonora Desert Museum Press).
Doolittle, W. E. (2010). “Traditional Use of Check Dams: A Global and Historical Introductio,” inCheck Dams, Morphological Adjustments and Erosion Control in Torrential Streams. Editors C. C. Garcia, and M. A. Lenzi (New York: Nova Science Publishers).
Ecosystem Marketplace (2021). Voluntary Carbon Markets Rocket in 2021, on Track to Break $1B for First Time. 2021-09-15. Available at: https://www.ecosystemmarketplace.com/articles/press-release-voluntary-carbon-markets-rocket-in-2021-on-track-to-break-1b-for-first-time/ (Accessed 10 2021, 01).
Faith, D. P. (2016). “A General Model for Biodiversity and its Value,” in The Routledge Handbook of Philosophy of Biodiversity. Editors J. Garson, A. Plutynski, and S. Sarkar. 1st edition (London: Routledge).
Falk, D. A. (2013). “Are Madrean Ecosystems Approaching Tipping Points? Anticipating Interactions of Landscape Disturbance and Climate Change,” in ”Merging Science and Management in a Rapidly Changing World: Biodiversity and Management of the Madrean Archipelago III; 2012 May 1-5; Tucson, AZ. Loa C., Comps. 2013. Proceedings. RMRS-P-67. Editors G. J. Gottfried, P. F. Ffolliott, B. S. Gebow, L. G. Eskew, and L. C. Collins (Fort Collins, CO: U.S. Department of Agriculture, Forest Service, Rocky Mountain Research Station).
Fandel, C. A. (2016). The Effect of Gabion Construction on Infiltration of Ephemeral Streams. [master’s thesis]. Tucson, AZ]: University of Arizona.
Fargione, J. E., Bassett, S., Boucher, T., Bridgham, S. D., Conant, R. T., Cook-Patton, S. C., et al. (2018). Natural climate solutions for the United States. Sci. Adv. 4 (11), eaat1869. doi:10.1126/sciadv.aat1869
Hafen, T. (2018). Landscape-scale Factors Affecting Detection and Occurrence of Threatened Yaqui Catfish in the Yaqui River basin. [master’s thesis]. Mexico[Norman, OK]: University of Oklahoma.
Hendrickson, D. A., and Minckley, W. L. (1985). Ciénegas: Vanishing climax Communities of the American Southwest. Desert Plants 6 (3), 131–174.
Iacona, G. D., Sutherland, W. J., Mappin, B., Adams, V. M., Armsworth, P. R., Coleshaw, T., et al. (2018). Standardized Reporting of the Costs of Management Interventions for Biodiversity Conservation. Conservation Biol. 32 (5), 979–988. doi:10.1111/cobi.13195
Isbell, F., Craven, D., Connolly, J., Loreau, M., Schmid, B., Beierkuhnlein, C., et al. (2015). Biodiversity Increases the Resistance of Ecosystem Productivity to Climate Extremes. Nature 526 (7574), 574–577. doi:10.1038/nature15374
IUCN (2020). Global Standard for Nature-Based Solutions: A User-Friendly Framework for the Verification, Design and Scaling up of NbS. First edition. Gland, Switzerland: IUCN.
Leger, A., Lopez, O., Lagarda, G., and Austin, V. (2019). Sampling for the Effect of Rock Erosion-Control Structures on Soil Carbon: Do Rock Structures Add Organic Matter to Our Soils? Preliminary Report for Cuenca Los Ojos and Borderlands Restoration Network (Chester, MD: Biophilia Foundation).
Main, D. (2021). Why a New Jaguar Sighting Near the Arizona-Mexico Border Gives Experts hope. National Geographic, 23. March Available at: https://www.nationalgeographic.com/animals/article/jaguar-near-arizona-border-wall-mexico (Accessed March 25, 2021).
McGinn, A. (2019). Nature as Resilient Infrastructure: An Overview of Nature-Based Solutions. Environmental and Energy Study Institute. Available at: https://www.eesi.org/papers/view/fact-sheet-nature-as-resilient-infrastructure-an-overview-of-nature-based-solutions (Accessed February 1, 2021).
Minckley, T. A., Clementz, M. T., Brunelle, A., and Klopfenstein, G. A. (2009). Isotopic Analysis of Wetland Development in the American Southwest. The Holocene 19, 737–745. doi:10.1177/0959683609105297
Mitchell, B. A., Stolton, S., Bezuary-Creel, J., Bingham, H. C., Cumming, T. L., Dudley, N., et al. (2018). Guidelines for Privately Protected Areas. Gland, Switzerland: IUCN.
Moore, W. (2015). “Sky Islands,” in A Natural History of the Sonoran Desert. Editors S. J. Phillips, P. Wentworth Comus, and L. M. Brewer. 2nd edition (Tucson: Arizona-Sonora Desert Museum Press).
Nature Based Solutions Initiative (2020). Nature-based Solutions to Climate Change: Key Messages for Decision Makers in 2020 and beyond. Available at: https://www.naturebasedsolutionsinitiative.org/wp-content/uploads/2020/03/NBS_Guidelines_2020.pdf (Accessed December 10, 2020).
Norman, L., Callegary, J., Lacher, L., Wilson, N., Fandel, C., Forbes, B., et al. (2019). Modeling Riparian Restoration Impacts on the Hydrologic Cycle at the Babacomari Ranch, SE Arizona, USA. Water 11, 381. doi:10.3390/w11020381
Norman, L. M. (2020). Ecosystem services of riparian restoration: a review of rock detention structures in the Madrean Archipelago Ecoregion. Air, Soil and Water Res. 13, 1178622120946337.
Norman, L. M., Brinkerhoff, F., Gwilliam, E., Guertin, D. P., Callegary, J., Goodrich, D. C., et al. (2016). Hydrologic Response of Streams Restored with Check Dams in the Chiricahua Mountains, Arizona. River Res. Applic. 32 (4), 519–527. doi:10.1002/rra.2895
Norman, L. M., and Niraula, R. (2016). Model Analysis of Check Dam Impacts on Long-Term Sediment and Water Budgets in Southeast Arizona, USA. Ecohydrology & Hydrobiology 16, 125–137. doi:10.1016/j.ecohyd.2015.12.001
Norman, L. M., Sankey, J. B., Dean, D., Caster, J., DeLong, S., DeLong, W., et al. (2017). Quantifying Geomorphic Change at Ephemeral Stream Restoration Sites Using a Coupled-Model Approach. Geomorphology 283, 1–16. doi:10.1016/j.geomorph.2017.01.017
Norman, L., Villarreal, M., Pulliam, H. R., Minckley, R., Gass, L., Tolle, C., et al. (2014). Remote Sensing Analysis of Riparian Vegetation Response to Desert Marsh Restoration in the Mexican Highlands. Ecol. Eng. 70, 241–254. doi:10.1016/j.ecoleng.2014.05.012
Pauly, D. (1995). Anecdotes and the Shifting Baseline Syndrome of Fisheries. Trends Ecol. Evol. 10 (10), 430. doi:10.1016/s0169-5347(00)89171-5
Petrakis, J. E., Norman, L. M., Vaughn, K., Pritzlaff, R., Weaver, C., Rader, A., et al. (2021). Hierarchical Clustering for Paired Watershed Experiments: Case Study in Southeastern Arizona, U.S.A.. Water 13, 2955. doi:10.3390/w13212955
Petrakis, R., Norman, L., Lysaght, O., Sherrouse, B. C., Semmens, D., and Pritzlaff, R. (2020). Mapping Perceived Social Values to Support a Respondent-Defined Restoration Economy: Case Study in southeastern Arizona,USA. Air Soil Water Res. 13, 1–16. doi:10.1177/1178622120913318
Phillips, D. (2009). Archaeology and Prehistory of Northwest Mexico: A ‘Rough Essay. University of New Mexico. Available at: https://www.unm.edu/∼dap/nwm/trin.html (Accessed September 24, 2021).
Pool, D. B., Panjabi, A. O., Macias-Duarte, A., and Solhjem, D. M. (2014). Rapid Expansion of Croplands in Chihuahua, Mexico Threatens Declining North American Grassland Bird Species. Biol. Conservation 170, 274–281. doi:10.1016/j.biocon.2013.12.019
Pritzlaff, R. G. (2018). A Meta-Analysis of Successful Community-Based Payment for Ecosystem Services Programs. [doctoral thesis]. [Prescott, AZ]: ProQuest Dissertations Publishing, 10822Prescott College.
Reid, W. V., Mooney, H. A., Cropper, A., Capistrano, D., Carpenter, S. R., Chopra, K., et al. (2005). Ecosystems and human well-being-Synthesis: A report of the Millennium Ecosystem Assessment Washington, D.C.: Island Press
Seddon, N., Giardin, C., and Smith, S. (2021). Value and Limits of Working with Nature to Address Climate Change, 25. Presentation to the Oxford Martin School. Available at: https://talks.ox.ac.uk/talks/id/e9487051-db69-4198-ae12-7c6d1f26061f/ (Accessed January 4, 2021).
Seibert, D. (2015). Post-fire Habitat Restoration with the Department of Corrections Inmate Crew. Patagonia, AZ: Seibert Ecological Restoration LLC. (Unpublished).
Smith, P., Adams, J., Beerling, D. J., Beringer, T., Calvin, K. V., Fuss, S., et al. (2019). Land-management Options for Greenhouse Gas Removal and Their Impacts on Ecosystem Services and the Sustainable Development Goals. Annu. Rev. Environ. Resour. 44, 1–10. doi:10.1146/annurev-environ-101718-033129
Staats, H. (2012). “Restorative Environments,” in The Oxford Handbook of Environmental and Conservation Psychology. Editor S. D. Clayton (New York: Oxford University Press). doi:10.1093/oxfordhb/9780199733026.013.0024
Tidball, K. G., Metcalf, S., Bain, M., and Elmqvist, T. (2018). Community-led Reforestation: Cultivating the Potential of Virtuous Cycles to Confer Resilience in Disaster Disrupted Social-Ecological Systems. Sustain. Sci. 13 (3), 797–813. doi:10.1007/s11625-017-0506-5
UNCCD (2020). 2020 Desertification and Drought Day. Available at: https://www.unccd.int/actions17-june-desertification-and-drought-day/2020-desertification-and-drought-day (Accessed 02 2021, 27).
United Nations Environment Program (2020). United Nations Decade on Ecosystem Restoration Strategy. Available at: https://wedocs.unep.org/bitstream/handle/20.500.11822/31813/ERDStrat.pdf?sequence=1&isAllowed=y (Accessed 10 2021, 01).
USFWS (2021). Available at: https://www.fs.usda.gov/recmain/coronado/recreation (Accessed June 20, 2021).
US Fish and Wildlife Service, US Bureau of Reclamation, US Forest Service. and Cross Watershed Network (2018). Cuenca de los Ojos: three decades of restoring water flows on private land in the U.S. and Mexico. Report. Available at: https://lccnetwork.org/sites/default/files/Resources/Cuenca%20de%20los%20Ojos_Three%20Decades%20of%20Restoring%20Water%20Flows%20on%20Private%20Land%20in%20the%20U.S.%20and%20Mexico.pdf (Accessed December 4, 2020).
Vásquez-León, M., West, C. T., and Finan, T. J. (2003). A Comparative Assessment of Climate Vulnerability: Agriculture and Ranching on Both Sides of the US-Mexico Border. Glob. Environ. Change 13 (3), 159–173. doi:10.1016/s0959-3780(03)00034-7
Wheaton, J. M., Bennett, S. M., Bouwes, N., Maestas, J. D., and Shahverdian, S. M. (2019). Low-Tech, Process-Based Restoration of Riverscapes: Design Manual. Version 1.0 (Logan, UT: Utah State University).
Wilson, N. R., and Norman, L. M. (2018). Analysis of Vegetation Recovery Surrounding a Restored Wetland Using the Normalized Difference Infrared Index (NDII) and Normalized Difference Vegetation Index (NDVI). Int. J. Remote Sensing 39 (10), 3243–3274. doi:10.1080/01431161.2018.1437297
Wilson, N. R., and Norman, L. M. (2019). Vegetation Response to Landscape Conservation in the Sky Islands. Washington, D.C.: The Plant Press Winter, 27–31.
WOCAT (2021). Global Database on Sustainable Land Management. Available at: https://www.wocat.net/en/global-slm-database/ (Accessed 01 2021, 07).
Youberg, A., Neary, D. G., Koestner, K. A., and Koestner, P. E. (2013). “Post-wildfire Erosion in the Chiricahua Mountains,” in Merging Science and Management in a Rapidly Changing World: Biodiversity and Management of the Madrean Archipelago III; 2012 May 1-5; Tucson, AZ. Editors G. J. Gottfried, P. F. Ffolliott, B. S. Gebow, L. G. Eskew, and L. C. Collins. (Fort Collins, CO: U.S. Department of Agriculture, Forest Service, Rocky Mountain Research Station). comps. 2013. Proceedings. RMRS-P-67.
Zedler, J. B., and Kercher, S. (2005). Wetland Resources: Status, Trends, Ecosystem Services, and Restorability. Annu. Rev. Environ. Resour. 30, 39–74. doi:10.1146/annurev.energy.30.050504.144248
Keywords: natural climate solution, ecosystem services (ES), erosion control structure, riverine, wetlands, carbon sequestration, carbon market, conservation finance
Citation: Gooden J and Pritzlaff R (2021) Dryland Watershed Restoration With Rock Detention Structures: A Nature-based Solution to Mitigate Drought, Erosion, Flooding, and Atmospheric Carbon. Front. Environ. Sci. 9:679189. doi: 10.3389/fenvs.2021.679189
Received: 11 March 2021; Accepted: 18 October 2021;
Published: 04 November 2021.
Edited by:
Borja Gonzalez Reguero, University of California, Santa Cruz, United StatesReviewed by:
Peter William Clinton, New Zealand Forest Research Institute Limited (Scion), New ZealandThomas Sisk, Northern Arizona University, United States
Copyright © 2021 Gooden and Pritzlaff. This is an open-access article distributed under the terms of the Creative Commons Attribution License (CC BY). The use, distribution or reproduction in other forums is permitted, provided the original author(s) and the copyright owner(s) are credited and that the original publication in this journal is cited, in accordance with accepted academic practice. No use, distribution or reproduction is permitted which does not comply with these terms.
*Correspondence: Jennifer Gooden, jennifer@biophiliafoundation.org