- 1World Agroforestry, Hanoi, Vietnam
- 2International Centre for Environmental Management (ICEM), Hanoi, Vietnam
- 3Food and Agriculture Organization of the United Nations Regional Office for Asia and the Pacific, Bangkok, Thailand
Entering the UN Decade on Ecosystem Restoration, interventions referred to as nature-based solutions (NBS) are at the forefront of the sustainability discourse. While applied in urban, natural forest or wetland ecosystems, they are underutilized in agricultural landscapes. This paper presents a technical framework to characterise NBS in agricultural systems. NBS in the agriculture sector is proposed as “the use of natural processes or elements to improve ecosystem functions of environments and landscapes affected by agricultural practices, and to enhance livelihoods and other social and cultural functions, over various temporal and spatial scales.” The framework emerges from a review of 188 peer-reviewed articles on NBS and green infrastructure published between 2015 and 2019 and three international expert consultations organized in 2019–2020. The framework establishes four essential functions for NBS in agriculture: 1) Sustainable practices — with a focus on production; 2) Green Infrastructure — mainly for engineering purposes such as water and soil, and slope stabilization; 3) Amelioration — for restoration of conditions for plants, water, soil or air and climate change mitigation; and 4) Conservation — focusing on biodiversity and ecosystem connectivity. The framework connects the conventional divide between production and conservation to add functionality, purpose and scale in project design. The review confirmed limited evidence of NBS in agricultural systems particularly in developing country contexts, although specific technologies feature under other labels. Consultations indicated that wider adoption will require a phased approach to generate evidence, while integrating NBS in national and local policies and agricultural development strategies. The paper concludes with recommended actions required to facilitate such processes.
Introduction
Report upon report stress the urgent and pressing state of the world’s rapidly degrading natural resources (FAO, 2017; FAO, 2018; FAO, 2019; IPBES, 2019; IPCC, 2019). As we enter the UN decade of Ecosystem Restoration, attention is brought to approaches that integrate natural ecosystems and ecosystems that sustain livelihoods and food production (Sonneveld et al., 2018), conserve or rehabilitate natural ecosystems, and enhance natural processes in modified ecosystems (Cecchi, 2015; Cohen-Shacham et al., 2016; GCA, 2019; IUCN, 2020).
Beginning in the 2000s, and emerging strongly in development discourses around 2017, nature-based solutions (NBS) gained ground both as a principle and an umbrella of approaches and technologies (Hanson et al., 2020). Deeply rooted in the discourse on ecosystem goods and services (MEA, 2005; Nesshöver et al., 2017), the International Union for Conservation of Nature (IUCN) specified eight principles for NBS (Cohen-Shacham et al., 2016; Cohen-Shacham et al., 2019) which, summarized, embrace nature conservation norms, offer inclusive and context-specific landscape-scale solutions, address societal challenges that produce equitable societal benefits, draw on local and scientific knowledge, address temporal tradeoffs between ecosystem and economic benefits, and are an integral part of policy and regulatory frameworks. As an umbrella, NBS is used to bridge similar concepts and practices for natural and managed ecosystems from different disciplines and for different needs (Cohen-Shacham et al., 2016). For example, the Special Report on Climate Change and Land (IPCC, 2019 p. 739) considers ecosystem-based adaptation (EbA) as “a set of nature-based methods” for adaptation and food security that is closely associated with sustainable land management and water security.
When it comes to specific practices, references to NBS predominantly feature in urban landscapes (Cecchi, 2015; IPBES, 2019) or for conservation and rehabilitation of water and forest ecosystems (Chausson et al., 2020; OECD, 2020; UNDRR, 2020). In particular for urban environments, work has advanced with planning and impact evaluation frameworks for NBS, (Raymond et al., 2017; Albert et al., 2020). Despite its increasing popularity, there is little compiled evidence on the potential of NBS to address problems associated with environmental degradation, disaster and climate vulnerability in agricultural (production) landscapes. For instance, only two out of ten NBS are for agriculture land uses in IUCN’s seminal work by Cohen-Shacham et al. (2016). In a systematic approach to categorize interventions for difference ecosystems, Eggermont et al. (2015) practically lay out a typology for how different interventions can maximize the return of ecosystem services from natural and managed ecosystems to the inclusive design of new agroecosystems that can meet the challenges ahead (Table 1). This typology provides dynamic benchmarks for many hybrid NBS to enhance their flexibility and problem-solving capacity in agriculture and has been adapted widely. For instance, FAO’s framework for NBS in agricultural water management presents a scoring guide for evaluating the success or failure of 21 NBS interventions, where the score represents the degree of ecosystem intervention, benefits of ecosystem services to stakeholders, degree of transdisciplinarity, stability of institutional collaboration, and financing (Sonneveld et al., 2018). This guide focuses on learning lessons and identifying good practice. One benefit of the typology by Eggermont et al. (2015) is it admits the inclusion of the many autonomous ‘NBS-like’ interventions that smallholder farmers have practiced for centuries, known as local knowledge (Hiwasaki et al., 2014; van Noordwijk et al., 2020), and responds to a multitude of environmental and socioeconomic challenges beyond climate change adaptation (Shah et al., 2019).
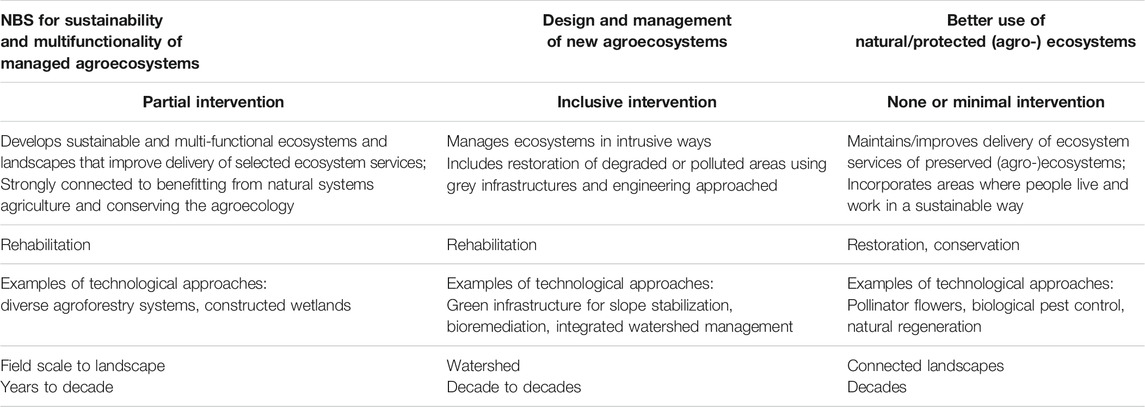
TABLE 1. Typologies of interventions within NBS for agricultural landscapes, with selected examples relevant for this paper (shaded background). Adapted from Eggermont et al. (2015) to reflect the framework presented in Table 2.
Recently, there has been a trend of various “good practices” increasingly being branded as NBS (O’Sullivan et al., 2020), often as compilations of short descriptive cases with elusive criteria for how it qualifies as NBS, good practice, or can be upscaled. For instance, the NBS Coalition of the 2019 UN Climate Action Summit gathered 200 NBS actions for scaling up for mitigation, resilience and adaptation in agriculture, forests, terrestrial and hydrological ecosystems (NBS-Facilitation Team, 2019). Some reports conclude that NBS are flexible, cost-effective and offer multiple solutions (GCA, 2019), and that NBS with safeguards can provide 37% of climate change mitigation until 2030 (IPBES, 2019 p. 10). On the other hand, as corporate and public funding are being availed for NBS, critical voices warn it may mislead as a new ”quick fix” as certain “tree-planting” initiatives disqualify as NBS for lack of biodiversity and people-centered considerations (Seddon et al., 2021). Such critique demonstrate challenges with economic valuation of ecosystems (Sonneveld et al., 2018) and also a lacking evidence base on the effectiveness of NBS in the Global South (Chausson et al., 2020; Seddon et al., 2021). Stressing the need for integrated ecosystems approaches adapted for developing country contexts, Supplementary Box 1 is provided to exemplify the pressing state of agroenvironments across Asia. It also reflects differences and diversity in land use and governance as compared to agroecosystems in Europe and North America, where the theoretical underpinnings of NBS originate.
This paper addresses two of these shortcomings. First, we propose a normative framework for NBS-practices in agriculture, bridging the conventional divide between production and conservation and exemplifying the specific problems NBS offer solutions to. Moreover, we frame NBS as (possibly underutilized) solutions for pressing issues in developing countries. We refer to NBS in the agriculture sector as “the use of natural processes or elements to improve ecosystem functions of environments and landscapes affected by agricultural practices, and to enhance livelihoods and other social and cultural functions, over various temporal and spatial scales.” We recognize that the NBS-concept does not substitute nature conservation or conventional “grey” engineering. Instead it offers a way to identify, prioritize and stage solutions that combine traditional, conventional and natural solutions in combinations to generate positive, cumulative biophysical interactions and social benefits.
Methods
This section outlines the scope of the framework, the iterative process of literature review and the outcomes of expert consultations which helped to refine the framework. The process employed to refine the framework also helped to pinpoint opportunities and barriers for adoption. While developed through interactions with key stakeholders in Asia, we regard it having wider application beyond any one region.
Particularly in developing country contexts, a useful framework should address challenges across a spectrum from production to conservation landscapes, and include land use functions 1) that maintain a high degree of local knowledge and relatively low levels of interventions; 2) for conservation and restoration pathways, and 3) for production systems with various land use management technologies towards restoration and sustainable land uses (Table 1). These conditions guided the framework formulation process.
Literature Review and Framework Formulation
The literature search involved three strategies for delineation: 1) Inclusion. NBS feature under different names and concepts, at landscape scale and as practices or technologies within a system (as exemplified in Supplementary Table 1). To keep the review manageable, we searched for practices referred to as NBS or GI. This would capture solutions across the spectra of conservation—production agroecosystems as well as engineering based NBS-technologies rarely used for solving problems in agriculture that potentially could lead to innovative land uses or “new agroecosystems” (Eggermont et al., 2015, Table 1). 2) Exclusion. Studies from urban contexts or lacking agricultural purpose, having marginal reference to NBS or branded as NBS without theoretical reference were excluded. 3) Screening. Articles remaining after title and abstract screening, underwent full text screening. For remaining articles the reviewers noted name and description of the practice(s) and intention; location, type of landscape and spatial scale; project duration; and for empirical papers, evidence provided for social, economic and ecological benefits. The review team marked references for fulltext review and point to examples 1) implemented in Asia, 2) implemented elsewhere but technically relevant for Asia, 3) providing new insights on economic, social or environmental impacts, or 4) as uncertain for case-by-case exclusion. Empirical studies failing to provide concrete information were ignored. Review articles were used for further references.
The first round of search aimed to establish a working definition and outline the framework. We searched ScienceDirect for abstracts and titles with “green infrastructure” or “nature-based solution.” This rendered 3,511 articles, in which the majority referred to urban environments. Adding “’NOT’ urban,” the result narrowed to 419 articles. After screening, 43 articles remained, and permitted a systematic grouping of practices according to their essential functions and primary purpose. Following the underlying division outlined in Table 1, the categories emerged as 1) sustainable practices (must have a productive element), 2) green infrastructure (must have a civil engineering function), 3) Amelioration (must have a beneficial biochemical, biological or microbial function), and 4) Conservation (must have a species preservation benefit), each with two to three qualifying functions.
The second round of search aimed to build more material to work with. We expanded the search to the practices identified as NBS or GI by searching for “practice name X” “AND” [“agriculture” “OR” “fisheries” “OR” “forestry” “OR” “animal husbandry”]. This allowed the inclusion of literature where the practice was not explicitly referred to as NBS or GI. The 25 most recent results in ScienceDirect for each type of practice published between 2005 and 2019 were assessed. In total, 181 out of 1,450 peer-reviewed articles were subject to in-depth review in this step, along with seven of the original 43 articles from the definition stage, a total of 188. This search approach may have excluded practices that are not yet associated with NBS in the literature, although they could have high potential. Furthermore, since the main review was done in 2019, the number of publications on NBS soared. Some updates were made after the consultations in 2020 in preparation for this paper. Making longitudinal or thematic comparisons of search term results was beyond scope in this study. Although the reviewed literature represented some global spread (Figure 1), most were based in Europe or North America. Most studies reported for 1) limited spatial (pilot, plot or part of catchment) and temporal scales; 2) ‘one’ technology rather than sets of NBS-technologies integrated in a landscape or interconnected; 3) one or few monitored environmental indicators, and 4) limited socioeconomic analysis. Similar conclusions were drawn based on the review by Hanson et al. (2020), where 10% of 112 NBS-articles included arable land uses.
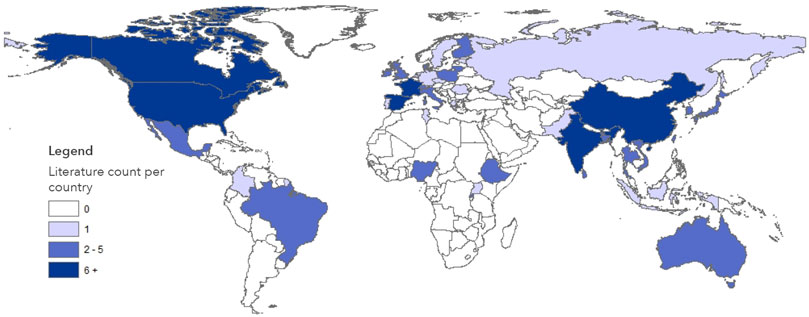
FIGURE 1. Countries represented in the NBS review (129 out of 188 papers with country-specific field experiments, excluding literature reviews and laboratory experiments).
Consultations and Further Refinement
The second stage of the framework development involved two phases of consultations. First, the draft framework was presented, tested, and modified at a regional 2-day consultation in Hanoi in July 2019. The 35 stakeholders represented practitioners involved in designing and implementing NBS on the ground, national level policy makers, and UN agencies from Indonesia, Lao PDR, Myanmar, Nepal, and Vietnam. The second consultation took place in 2020 with two rounds of online meetings. First with the FAO regional office for Asia-Pacific and its network of country offices followed by a global meeting with the FAO Technical Network on Climate Change, which included representatives from across FAO’s technical divisions and global network of regional and country offices.
The consultation rendered important insights for a more problem-based rationale of the framework. First, it is important that any NBS framework be designed with application in mind. In practice, this means that the framework should support the design and implementation of measures (solutions) that will address needs or problems, isolated and cumulative, which result from ongoing and continuous management of agricultural production systems. As an example, one may consider a business-as-usual scenario with conventional farming practices that manages “resource inputs (i.e. fertilizer, irrigation water, amendments, pesticides) uniformly, ignoring the naturally inherent spatial heterogeneity of soil and crop conditions between and within fields [and the] uniform application of inputs results in over and applications of resources” (Corwin and Scudiero, 2019, section 5.8.3).
Related to this, an overarching takeaway from the sessions was the need for human focus in the framework, particularly regarding its utility as a tool for implementation. Both practitioners and policy makers perceived people-centered frameworks to have better chances for implementation and wider uptake than concepts considered as top-down, complex, vague, technocratic, or bureaucratic. These views reflected findings in the review, that NBS-studies from Europe often integrated the general public as beneficiaries of cultural and rural ecosystem services, for recreation and well-being for example. Conversely, studies from developing countries had farmers and local communities as the primary, often only direct users and beneficiaries of ecosystem services, but they rarely interacted in negotiations with the larger society. On the other hand, some argued that too much livelihoods focus risks becoming “another” development project that takes focus away from environmental degradation. Responding to this, two categories were more explicitly integrated into the framework: the spatial and temporal scale of NBS. Incorporating a temporal scale acknowledges that NBS have different effects over different time periods, e.g. short—such as one crop season, medium (1–10 years), or long-term (decades) and has implications for planning of successions of interventions. The full benefits of NBS often emerge on a longer timescale, while unsustainable practices can bring quick short-term gains that hide longer-term negative effects. The spatial scale considers in-situ and ex-situ impacts. For example, grass strips can have in-situ (costs and) benefits for the farmer in the field, and wider ex-situ effects, such as amelioration of pollutants or sediments in a river, which will be experienced further downstream. At the largest scale, carbon sequestration measures need to have discernible effects at aggregated scales, up to global. The need for adding scales to the framework also led to the realization of three additional functions: biological pest control and pollination, and, land management practices for the purposes of above and below ground carbon sequestration to the amelioration category.
Discussion also took place at the conceptual level. One question emerged of what NBS could add to EbA, which was considered a more established concept. The question is warranted, as many reviewed papers presented unclear or confounded definitions of NBS and GI (if definitions were present at all). In the agriculture sector, we particularly note inconsistent naming conventions and similar practices and concepts referred to by different names (c.f. Supplementary Table 1). Compared to the cases in the Global North, many concepts such as ecosystem services, agroecology, climate-smart agriculture, and NBS have not had the chance to become fully mainstreamed in policy. Therefore, although technically many practices are known, when framed as a new concept it often must undergo a policy integration cycle. Concept fatigue was reflected particularly among the decision makers, who were questioning the need for another concept when policy makers are still struggling to integrate “sustainability” or “ecosystems” in the legislation. A framework with compilations of NBS-practices as in Supplementary Table 2, presents a menu of alternatives to and pathways from conventional agricultural practices while also illustrating the bridging function of the concept.
A third discussion centered around a possibility of NBS being promoted regardless of the root cause of the problem. This, many argued, run the risk of making NBS technology-oriented and supply-driven, rather than outcome-oriented and demand-driven, and as such, legitimising “lack of capacity” as a barrier for adoption among reluctant farmers. In contrast, when farmers experience demand for a product, they often find their own ways to overcome technical capacity gaps. The motivation to accept uncertainties involved with changing practices can vary considerably, even among homogenous groups of farmers, as shown in a European case study (e.g. Gatto et al., 2019).
Other key points that were raised include whether NBS is considered as a disruptive solution, or whether it can work alongside industrialized monoculture to reduce negative ecological impacts; and the importance of establishing policy drivers and incentives for NBS including comprehensive economic costing to establish clear evidence of benefits to decision makers and establish commercial viability to the private sector and farmers. These issues are discussed in further depth in Considerations for Implementation of NBS. Each consultation concluded that a technical framework could be a helpful tool to identify and match nature-based “solutions” with immediate intentions against a longer-term view.
The Nature-Based Solutions Framework
This section outlines the framework with consultation feedback incorporated and provides explanation of the essential functions with examples of measures as emerged from the literature review. The full list of practices mapped to specific functions is stated in detail in Supplementary Table 2.
Framework Overview and Technical Dimensions
The framework (Table 2) builds on the use of NBS in response to challenges in agricultural landscapes (Figure 2), and consists of four essential functions that can be used to gradually add functionality, purpose and scale in project design (Table 1) with measures categorized according to their essential, or primary function:
1) Sustainable practices—primarily for production purposes, including natural nutrient and microclimate management, e.g. agroforestry and windshields. Anticipated benefits to people include more diverse and/or higher production quality, more stable productivity, safeguarded livelihoods, and reduced damage by temperature stress.
2) Green infrastructure—primarily for engineering purposes, including physical regulation of water and soil, and slope stabilization, e.g. grass strips, hedgerows, or terraces using natural material. Benefits include reduced damage by mass movement or additional fodder grass.
3) Amelioration—primarily for restoration of conditions for plants, water, soil or air and climate change mitigation, e.g. bio- and phytoremediation and mangroves. Benefits include safe water, reduced health impacts stemming from biological pest control and carbon sequestration.
4) Conservation—primarily for maintenance or increase of ecological health at field or landscape scales, e.g. natural fallow or regeneration. Benefits include general well-being, cultural and spiritual benefits, safeguarded biodiversity, supported nutrient cycles, and increased resilience to environmental stress.
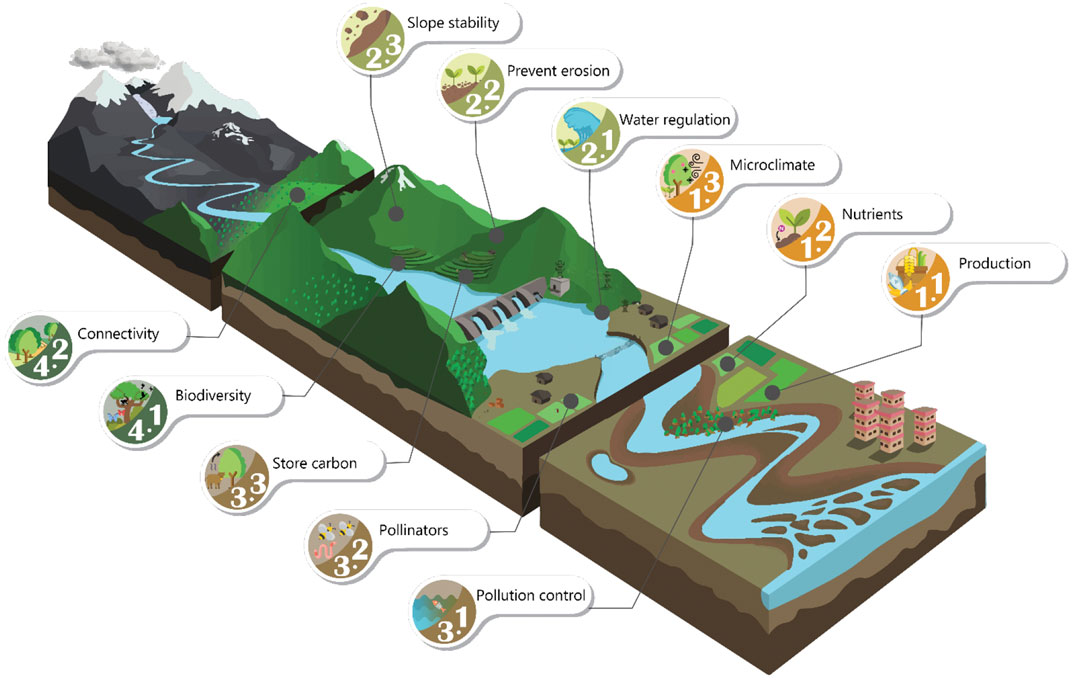
FIGURE 2. NBS for agriculture landscapes. The corresponding numbers are found in Table 2. Source: Landscape from ICIMOD (http://www.icimod.org/?q=rps_riverbasins). Wave (2.1) Abstract vector created by freepik - www.freepik.com. Landslide (2.3) original icon from www.clipartmax.com. Bee (3.2) and tree (4.1) original icons made by Smashicons from www.flaticon.com. Cow (3.3) icon made by Nhor Phai from www.flaticon.com. All other icons made by Freepik from www.flaticon.com.
The production (1) and conservation-oriented (4) purposes frequently appear in the literature and easily lend themselves to be contrasted, such as in Table 1. “Conservation” in agricultural landscapes should be distinguished from “conservation” and protection of natural ecosystems. However, NBS are multifunctional and provide synergy benefits. For example, perennial systems could contribute to all four NBS-categories. Similarly, root causes to declining agriculture productivity can often be traced to neglected management in all four categories.
The establishment of an ecologically functional system can be achieved by systematically building up ecosystem functions through different components over time (succession) or joining areas (connectivity). As such, the framework can stimulate multidisciplinary action towards higher social and environmental outcomes. Spanning from the first category there is more focus on socioeconomic co-benefits, in companion with the second group many solutions can be implemented on small scale with return to the landowner/user. Further towards the third and fourth groups come increasing biodiversity co-benefits, the focus shifts more on planning for successions that 1) take longer time, 2) and/or require larger landscapes, and to build-up of natural buffers/conditions for those ecosystems to restore functions and return benefits to people. Such objectives are more effective through organised groups of land users/owners or communities, as socioeconomic benefits are possible, e.g. amelioration and carbon sequestration with multifunctional plants that generates non-(timber)-tree products. Similarly, there can be trade-offs involved between the four essential functions. For example, root causes to declining agriculture productivity can often be traced to neglected management in all four categories. If agriculture production is a subsidiary priority, or can be compensated for, the interventions can target larger spatial scales or aim for achieving higher environmental values, i.e. amelioration and conservation goals. The nature of some challenges may call for immediate action to avoid further environmental deterioration, such as removing toxic substances or reducing natural hazard risks.
The Functions
Sustainable Practices
First, “production”-oriented practices make use of the multiple ecosystem functions of trees, plants and (wild or domesticated) animals for agricultural production, while minimizing the negative environmental impacts of the production (Daryanto et al., 2018) such as regenerative agriculture and conservation agriculture.
For example, trees in alley cropping can play multiple roles: 1) tree crops for food and fodder production, 2) perennial alley crops, 3) trees for crop facilitation via shade, and 4) within-system tree diversity (Wolz and DeLucia, 2018). In agroforestry and sloping agriculture land technologies, in addition to production contributions, plants may also perform green infrastructure functions if, for example, planted as grass strips, or nitrogen-fixing legumes used as green mulch and fruit trees, planted along contours (McIvor et al., 2017; Are et al., 2018; Geussens et al., 2019).
Green Infrastructure
In the reviewed examples, GI practices were used for structural stabilization of slopes and controlling the flow of water and soil at field or catchment scale. Often GI entails the use of selected species which maximize their purpose such as root structure and morphology for erosion control, slope reinforcement or wave energy reduction. In the non-agriculture sphere, one main purpose of GI is disaster mitigation. Common examples were those of constructed wetlands for water regulation, storage and flood control. For example, in the United States and New Zealand, ecological infrastructure of wetlands with riparian forest, floodplains and constructed wetlands (Mander et al., 2005; Watson et al., 2016). Mangroves also have documented direct and indirect benefits for coastal protection and adaptation for both urban and rural livelihoods, small-scale fisheries, and ecosystems (Tran and Bui, 2013; Diop et al., 2018; Rahman and Mahmud, 2018).
Viewing NBS from the perspective of “design of new agroecosystems”, we searched for evidence of engineered technologies essentially qualifying for multiple agriculture and non-agriculture purposes.
Agronomic Measures
When agriculture species plays the role of vegetation in GI, multiple functions are rendered. For example, grass strips control soil erosion and return crop yields (Are et al., 2018), where vetiver grass also can act as phytoremediation to trap phosphorous (Huang et al., 2019), or cut for animal feed. The efficiency of a catch crop also depends on physical elements, such as slope gradient (Novara et al., 2019) and root structure. Some papers related micro-terraces and built terraces as green infrastructure for agriculture (Zuazo et al., 2011; Liu et al., 2018). In northern India for example, simple weed strips and weed mulch also created micro terraces, which resulted in reduced soil erosion and higher yields (Lenka et al., 2017).
Engineering Structures
Agricultural waste can also be used as construction material for green infrastructure. For example, geotextiles made from local material such as bamboo, rice and wheat straw, and maize stalks were used to stabilize slopes in Lithuania, China, Thailand, and Vietnam, sometimes in combination with contour planting, with reported higher biomass production and crop yields, compared to no geotextiles (Bhattacharyya et al., 2012). There were no examples among the reviewed literature, but it is possible to imagine green slope stabilization measures on non-agriculture soils, providing non-timber forest products.
Amelioration—Phyto- and Bioremediation
Phytoremediation — the use of living green plants, and bioremediation — the use of microorganisms to break down or degrade contaminants, are considered cost-effective and environmentally friendly technologies for cleaning up polluted sites or preparing sludge before it is reintroduced to the environment. In the United States and Indonesia, a set of methods to control agricultural runoff, such as vegetated swales, enhanced stream buffers, denitrifying bioreactors, and constructed wetlands were referred to as GI (Anbumozhi et al., 2005), while according to our framework, their main functions place this measure into the category of amelioration. Many reviewed bio- and phytoremediation interventions were local, and studies therefore species-focused.
Bioremediation
The number of patents for new bioremediation technologies for water and soils are increasing at a fast rate, especially in China. A review showed that patents for using bioremediation agents, such as bacteria, enzymes, and fungi were more common than algae, plants and protozoa, as most patents targeted oil contaminants (Quintella et al., 2019). Specifically, in agricultural environments, anaerobic denitrifying bioreactors (hydraulic retention and biochar) can remove agricultural pollutants from farmland to surface waters, such as pesticides (Villaverde et al., 2018; Hassanpour et al., 2019). Of the 25 reviewed papers on bioremediation, most were concerned with removing nitrates, and with three Asian countries represented: China (5), India (1) and Pakistan (1). Within bioremediation, site selection and design are two important aspects. For example, denitrifying bioreactors require design that is resistant to differences in water flow during storm events to avoid leakage (Pluer et al., 2019). Among the literature featured many laboratory experiments, which suggests that this is an area where new and more advanced technology can be expected. Promising results were shown with rice straw instead of woodchip as carbon source in the bioreactor (Liang et al., 2015).
Phytoremediation
In phytoremediation, plants are purposely selected to extract pollutants from soil and water, or to exclude pollutants from biomass, or a combination of both (Jonsson and Haller, 2014). Through the search, we identified 14 studies on phytoremediation, most with the primary objectives being pollution control and desalinization. The extraction capacity of plants is important to inform about the potential use of remediation plants for feed or food. For example, to recover pesticide contaminated cotton soils in Nicaragua, scientists compared the distribution of persistent organic pollutants in different vegetative organs in three cultivars of amaranth. Overall, although the type and amount of pollutant that each cultivar extracted from the soil varied significantly, parts that could provide feed, stems and leaves, accumulated higher concentrations than the roots and seeds (Haller et al., 2017). The uptake and translocation of antibiotics in maize is another example of the potential use of agricultural crops for phytoremediation (Zhang et al., 2019). A recent review illustrates the efficiency of agriculture and forestry plants in metal extraction from mercury-contaminated soils and water, and also risks of accumulation in edible tissues for animal and human health (Tiodar et al., 2021).
In constructed wetlands, different riparian vegetation types such as coniferous, deciduous broad leaf or evergreen broad leaf forests, aquatic or herbaceous plants play different roles that are designed for controlling and managing water pollution (Wang et al., 2018). For some purposes, phytoremediation in constructed wetlands may perform better together with other technologies for removing toxic agrochemicals, such as bio-mixtures for biopurification (Gikas et al., 2018). Functions of riparian zones and buffer strips and their designs are described by Mander et al. (2017). Specifically, the width of the vegetated buffers, which may vary between 1 and 4,000 m, matters for protecting water sources and crops against pesticides depending on the habitat — something which is not reflected in legal documents (Gene et al., 2019).
Climate Change Mitigation
While many practices have production or conservation purposes (e.g. FAO, 2016a; Zomer et al., 2016; Hernández-Morcillo et al., 2018; Rosenstock et al., 2018), their contributions to climate change mitigation appear underestimated in the reviewed NBS and GI practices—or conversely, were seldom referred to as NBS or GI in the literature search. However, when tree planting for carbon sequestration comes at the cost of biodiversity and local rights to resources, it is a distraction from the meaning and intentions of NBS (Seddon et al., 2021). One exception was hedgerows, which increase soil organic carbon but often struggle to get recognition as a mitigation contributor (Hernández-Morcillo et al., 2018).
Possible explanations, despite numerous policy and funding mechanisms, could be that the scale of interventions necessary for a significant global impact is difficult to monitor, conflict with landscape diversification, or compete with other land uses and ecosystem goods and services (Namirembe et al., 2015; Cohen-Shacham et al., 2016).
Conservation
For the conservation category, in landscapes with human impact the main purpose is to build up connected ecosystems, ecosystems functions and biodiversity, temporarily such as natural fallows, long-term or permanently, such as natural forest regeneration. Various landscape approaches aim to achieve multiple goals from ecological intensification of crop production with biodiversity focus (Garibaldi et al., 2019) to ecosystem services within payments for ecosystem services (PES) schemes (Holt et al., 2016; Karabulut et al., 2019). One particular intention with practices under this essential function, is to ecologically connect conservation agriculture on field-units across larger landscape mosaics in landscape approaches (Holt et al., 2016).
The review illustrated that integration of practices can connect patches in the landscape. First, in Europe with functional agrobiodiversity approaches, where permanent grassland and crop diversification within ecological focus areas involved a certain percent of arable land that was set aside to be used for field margins, hedges, trees, fallow land, landscape features, biotopes, buffer strips, and afforested area (Delbaere et al., 2014). Similarly, connectivity was achieved with ecological infrastructures, such as woodland hedges, rosaceous hedges, grass strips, wildflower strips, and field margin (Rosas-Ramos et al., 2018). In Pakistan, an example of EbA included connecting landscapes through practices such as crop rotation, intercropping, agroforestry, crop diversification, live fencing, and wind barriers by trees (Shah et al., 2019). These examples show that many biodiversity conservation practices also contribute to ameliorative functions, such as carbon sequestration and pollinators (IPCC, 2019), that build up multiple ecosystem values over time.
Temporal and Spatial Scales: Sequencing, Successions, and Connections
Foreseen and unforeseen risks affect land use decisions across spatial and temporal scales. As conservation challenges are rarely foreseen, “best practice” solutions, which denotes predictability, are ill-suited for complex systems (Game et al., 2014). Instead, NBS need to be designed as a series of interventions to reinforce the resilience of ecosystems in order to prevent, reduce, respond to, or adapt to existing or anticipated stressors. An important aspect of the “conservation” function in the framework is therefore the process of connecting or expanding NBS-measures to cover larger timescales and areas of the landscape. These scales can be considered as a mosaic onto which we may overlay physical disruptors, e.g. environmental degradation, invasive species, pest and disease pathways, and interventions that connect landscapes, e.g. biodiversity corridors and constructed wetlands.
Prioritisation includes identifying the sequencing order for a stable succession. For example, natural regrowth and root development in riparian wetlands take years (Frątczak et al., 2019) and the full effect of trees for slope stabilisation comes decades later (Stokes et al., 2010). Timing the interventions thus depends on natural regeneration processes, as well as when implementers expect to see certain benefits. The benefits (or dis-benefits), and urgency of them, can be perceived and prioritized differently by certain groups at various scales from field-farm-farmer to landscape-ecosystem-community scales (UNEP, 2021). Important aspects of successful NBS involves responsive decentralized management (Game et al., 2014), removing barriers that focus on short-term economic returns to cover investments and to focus on an affordable succession of NBS practices that pay back over time.
Considerations for Implementation of Nature-Based Solutions
This section moves from consolidation of definitions of practices under the framework, to considerations for implementation. Factors and gaps emerging from the literature are discussed, as well as insight which emerged from the consultation workshops.
Economic Dimensions
The economic argument for adoption, which was stressed in consultations from the dual perspectives of farmers and decision makers of developing countries was not reflected in the literature. Ten reviewed papers included economic assessments of the practice itself or of the environmental values of the practices. Among these are economic estimates calculated on management approaches to reduce sediment loads (Mtibaa et al., 2018) and agriculture runoff (Gikas et al., 2018; Irwin et al., 2018). A study in Tunisia by Mtibaa et al. (2018) found that while contour ridges alone halved the sediment yield, the most cost-effective option was a combination of practices, including buffer strips, conversion to orchard, and grass strip cropping. Similarly, Gikas et al. (2018) showed that two low-cost options with plants in constructed wetlands, performed better when combined with bio-mixtures containing coconut fibre for biopurification. Other estimates, such as those by Irwin et al. (2018), related the improvement in water quality from reduced agriculture runoff with an associated value for residents and recreation users. Here, ten percent improved water quality resulted in a “lifetime cost benefit ratio” of 2.9.
Shortcomings in economic assessments can be attributed several issues:
First, difficulties in correctly evaluating ecosystem values. For example, the effects and valuation of agroforestry ecosystem services were clearer at the farm/plot scale, whereas attribution easily got blurred in the mixed land uses at landscape scale (Kay et al., 2019). The scales add challenges when negotiating economic and socio-cultural stakes in landscapes with diverse tenure and management.
Second, difficulties extrapolating results from smaller empirical studies, e.g. the role of pollinator services for global scale food production. To overcome this, Melathopoulos et al. (2015) devised an approach to estimate values of pollinator services from three different assumptions: 1) the degree of dependency of crops on pollinators; 2) pollinators need different habitats and pollinate different crops (wild versus domesticated) hence the cost to retain them will vary; 3) whether the price of the ecosystem service is aligned with the risk, e.g. the value depends on the probability of a bee pollinator collapse.
Third, underlying economic assumptions of grey versus green infrastructure depend on how risk, investment costs and value of losses are calculated. For example, Onuma and Tsuge (2018) tried to determine when green infrastructure is preferable to grey for disaster risk reduction. This was done by developing parameters to compare the two options in view of hazard, population potentially affected, and associated vulnerability. Although their primary focus was not on agriculture, similar valorization principles can have applications for GI in agriculture. For example, grey infrastructure is designed as a defense to one particular natural hazard and breaks at a certain magnitude, while mixing grey with green infrastructure can be more durable. Additionally, costs are often lower for recovering green infrastructure after a disaster event.
Lastly, NBS interventions need to consider surrounding land-use change, such as increasing rents on intensive agriculture land, which will likely drive costs for conservation and carbon credit compensations (Phelps et al., 2013). One review pointed out that many studies, especially in developing countries, fail to specify baseline conditions to which cost-effectiveness evaluations are made. This is partly due to a shortage of available georeferenced data on agriculture management, costs and prices (Ovando and Brouwer, 2019). Data shortage also risks misinterpreting conservation vis-a-vis production interests (sparing versus sharing debates), where the historical management contexts are required to understand the ecological values and trade-offs (Angelstam and Lazdinis, 2017; Naumov et al., 2018), not the least in the light of potential tenure issues (FAO, 2016b; Carter et al., 2017; Borelli et al., 2019). Furthermore, the ongoing rapid land-use changes across Asia (Tenneson et al., 2021) may make it difficult to determine a baseline or an “ecological equilibrium” to reflect “ecological health.” More studies involving a long-term lens on economic assessments can contribute to better estimates of avoided loss and damage by NBS and similar interventions and stimulate adoption.
Social Dimensions and Long-Term Adoption
Several studies in the NBS review indicate that farmers may not adopt sustainable practices despite having witnessed ecosystem benefits, because of increased initial costs, labour inputs, or customs and preferences (Chapman and Darby, 2016; McWilliam and Balzarova, 2017; Cerdà et al., 2018). To overcome this, farmers’ willingness to adopt new practices can be influenced by presenting cost-benefit assessments of different management options. Examples included cover crops in various ecosystems (Daryanto et al., 2018) and a system-dynamics modelling study on paddy field management from Vietnam, where the dynamics between farmers and their rice agriculture operations were integrated with the role of fluvial sediment deposition within their dyke compartment (Chapman and Darby, 2016). The latter study found that triple-cropping was only optimal for the wealthier farmers and in the short-term, while sluice gate management to enable soil nutrient replenishment would be a more economically and environmentally sustainable practice.
Despite a vast body of literature concerned with piloting different types of compensations for land use conversion, particularly PES, few mentioned NBS. In Uganda, Geussens et al. (2019) investigated farmers willingness to accept eight practices (qualifying as sustainable production or GI in this framework: i.e. minimum tillage, mulching, contouring, trenches, grass strips, agroforestry, and riverbank protection) under nine different compensation levels, or PES contracts. The study drew two important lessons for NBS. First, the biggest difference between willing and reluctant PES-adopters, concerned their perceived benefits. Their preferences depended on the intervention, the compensation level, and whether they received community funds or individual compensation. Second, project designers contrasted willingness to adopt and the reduced effectiveness of scattered practices. Hence, a minimum number of farmers were required for landscape benefits. The willingness to accept was high when the need for a different solution had reached a certain threshold, such as severity of degradation (the Uganda example), or when farmers have run out of other viable options. Ultimately, PES schemes would benefit land uses with high ecosystem values by combining marketable and non-marketable ecosystem services, such as biomass production and groundwater, soil quality, carbon sequestration, or penalizing land-uses with dis-benefits (Kay et al., 2019).
Illustrating complex trade-offs in transparent ways can help to reach negotiation solutions. For instance, Rosa-Schleich et al. (2019) reviewed the economic and environmental trade-offs among nine diversified farming practices. For each practice, they developed a matrix of ecological and economic benefits, which were converted into two axes. The space showed what clusters of practices were perceived to give high ecological benefits (agroforestry), high economic benefits (structural elements), or high in both (organic agriculture). Similarly, for the purpose of restoring an environmentally degraded mangrove ecosystem in Bangladesh, scientists developed a relative environmental and economic matrix with a quantitative cost-benefit study on four silvo-fishery systems under different restoration scenarios: integrated mangrove-shrimp, crab-mangrove, mangrove bio-filtering, and nypa-shrimp over three periods between 0 and 10+ years (Rahman and Mahmud, 2018). Both studies showed that combinations of practices with multiple functions were beneficial, particularly when the introduction of structural elements have insignificant economic or productive motives. Moreover, interventions that require decades to mature, such as mangrove restoration, also strongly depend on community participation and governance commitment (Rahman and Mahmud, 2018).
Policy Dimensions
Among the evidence for long-term adoption and transformation, the review raised examples of where NBS-practices were embedded in institutional and policy decisions that went beyond subsidies and conservation goals. For example, Albert et al. (2017) identified four premises for economic valuation of ecosystem services: 1) an institutional analysis to establish uses of nature and incentives of different stakeholders, 2) cost-and-benefits associated with the change in nature, 3) public and private sources of incentives to land managers, and 4) trade-off assessments between societal goals to establish winners and losers coming with the policy package, in their case the Common Agriculture Policy. The benefit of long-duration policies was shown similarly in an 18 year-long study from Italy, which concluded that through a persistent government policy, the different needs of different farmer typologies could be met, from early to late adopters (Gatto et al., 2019). Their study on implementing and maintaining hedgerows, reported that early adopters required that the compensation could be integrated with their income-generating activities, while the next group of adopters were those who received support to plant new hedgerows rather than those who maintained their existing ones. The third phase of adopters were motivated by social pressure and public acknowledgement of farmers’ work, and the late adopters followed when they felt pressure from neighbor farmers rather than the public. The role of governments for setting policies and long-term pathways is repeated also for regulating public goods where PES-markets are limited, such as fish and fish habitats (Mulazzani et al., 2019).
Some studies found that blanket policies fail to reflect the complex realities and trade-offs (Holt et al., 2016). The consultation workshops generated more practical insights to this literature. First, underlying causes of farmers’ reluctance, such as control over resources, are rarely addressed and instead generally “solved” by training and sensitization. For example, tenure insecurity is known to restrict smallholder farmers’ longer-term investments in diverse perennial farming systems (Borelli et al., 2019). Second, existing governance barriers, such as rigid policies and institutional silos, were overlooked in many studies. Such barriers can demotivate both decisionmakers and grassroot initiatives. For example, a structured analysis within seven Indonesian government institutions identified broad gaps and inconsistencies for institutionalizing valuation into policy (Phelps et al., 2017). Third, the workshop participants were largely in agreement that sufficient, stable and long-term support was lacking at the landscape-scale NBS across Asia. Exemplifying the importance of this as a precondition included Vietnam’s national PES policy, which after almost a decade of implementation still has difficulties reaching impact at scale. Among the reasons raised were that no compliance is required, and the net benefits are so low (fixed, non-negotiable compensation) that often only community-based payments are viable to payout. Moreover, while community compensation is often preferred by the poorer households, this is unlikely to motivate adopters in the long run if living standards improve. Incentives and policies to change from short to long-term sustainable behaviors are urgently needed, notably from government or companies buying the products. The Uganda case suggested, that since PES compensations are generally low and may be subject to changing compensation levels, (wealthier) farmers who do not need payments, should not receive them even if they make interventions (Geussens et al., 2019). Decision support tools seem to be used in the initial stages of research projects, while the review gave little evidence for them becoming permanently integrated in decision processes. Four papers concerned tools for negotiating human-environmental-governance relationships, typically trade-off models for anticipating or assessing policy impacts on ecosystems (Rega et al., 2018; Karabulut et al., 2019).
Nature-Based Solutions as a Disruptive Solution
The consultation workshops confirmed an urgent need for system-level interventions in agriculture that can effectively address multiple challenges simultaneously. One concern expressed in the first consultations was that some (decision makers, private sector interests) may view NBS as a troublemaker if promoted as a replacement for industrialized monoculture or “grey” infrastructure. Some argued, if NBS can appear alongside monocultures, it could gradually and more easily be “mainstreamed” into large-scale agriculture landscapes, such as rice-cultivated deltas, to mitigate some of the most harmful impacts. Others commented that such entry points would limit opportunities to fully use nature to restore ecosystem services, such as providing habitat for pollinators and natural predators. Following the need to address complex challenges, the consultations indicated that one selling point of the NBS framework is to demonstrate how to break spirals where agriculture cause environmental problems (e.g. overuse of agrochemicals spilling into waters) which create new problems for agriculture production (e.g. polluted soils and water impacting on pollinators and food safety), and how these problems are connected across landscapes. Few of the reviewed papers made substantial references to how NBS interventions could contribute to international commitments. Conceptually, the NBS-framework provides entry points to harmonize goals of several UN Conventions, such as on climate change, land degradation, biological diversity, and Sustainable Development Goals.
A concrete example to bypass two persistent obstacles for adoption: financial support and technical knowledge, could be to use decision support tools for comparing when GI is preferable to grey infrastructure (Onuma and Tsuge, 2018). This can be translated into loss and damage recommendations from, for example, economic assessments of benefits from GI for flood control (Watson et al., 2016), or post-disaster assessments of impacts on watershed services and water security (HLPE, 2019). Like the consultations pointed out, higher level public officers may be motivated to co-invest in implementation if NBS can attract private investments (FOLU, 2019). More importantly, to sustain long-term effects of NBS and GI, studies often highlight governance and the role of community, private and public sector engagement (IUCN, 2020; Monteiro et al., 2020; Dumitru et al., 2021). The nature of such relationships is fundamentally diverse across the globe, and each setting need to find their own new modalities.
Transboundary Challenges and Opportunities of Nature-Based Solutions
The literature review did not present solutions to the transboundary nature of many challenges, especially water-related ones, although many NBS examples seem fit for such purposes. Certain lessons can be drawn from catchment projects, such as PES, about acceptable compensation levels and their duration. Furthermore, successful NBS implementation will likely benefit from breaking up some institutional silos. This requires a common terminology and international policy frameworks. To illustrate this process is the development of ASEAN agroforestry guidelines, where ministers agreed on a regional strategy. Subsequent work nationally is described in Catacutan et al. (2018) and Singh et al. (2016). Further, NBS overlap with some of the Committee on World Food Security’s principles on guiding frameworks on rights, livelihoods and tenure (CFS, 2014). For instance, in relation to Principle 6 “Conserve and sustainably manage natural resources, increase resilience, and reduce disaster risks,” NBS can represent a set of environmentally sound practices that also can reduce the negative impacts of agriculture. Additionally, a stronger rights and co-investment perspective can be added to the NBS framework from the Responsible Investment in Agriculture and Food Systems (RIAFS), which offer a set of non-binding principles to promote responsible investments that specifically contribute to food security and nutrition.
Proof of Evidence From the Top Down and Bottom Up
The consultations pointed out that proof of evidence was viewed vital for the initial adoption of NBS. Details of such evidence must be worked out with various stakeholders in an agricultural landscape (Table 3), as the interests and motivation vary among land owners and users, decision makers, private sector, and the public.
Approaches need to accommodate both stable policies that motivate change and community engagement that ensures local problems are addressed. Although positive spill-over effects on adoption were noted over time in some European studies (e.g. Gatto et al., 2019), prerequisites for NBS-adoption outside European contexts need to be better understood. Collecting good practices could aim to fill specific data gaps on e.g. measurable benefits and ecological health. As evidence is generated, what counts as NBS will likely continue to evolve over time. Allowing a credible degree of flexibility within a concept is necessary, as an over-reliance on best practices recommendations can hinder creativity, co-learning, and may result in maladaptation (Game et al., 2014; Schipper, 2020; Eriksen et al., 2021). Furthermore, it was discussed whether landscape diversity requires a certain degree of homogeneity or heterogeneity among farmers, farm sizes or their activities. To opt for scaling of best practices may not always be desirable or achievable given the diversity of situations and problems in any specific agricultural area and community. Therefore, there was strong agreement among consultation participants that NBS need specific entry points to pursue opportunities to transition from short to long term impacts. For instance, through environmental economics accounting, “green GDP,” or capping a maximum for environmental debts that can be moved into the future. Another entry point was “urgency triggers,” as certain practices may only be adopted once a certain ecological (or economic) state worsens in a location or group, such as after a disaster, when human and environmental health needs demanded or were pushed by consumers or farmer organisations. Urgent entry points relate to the importance of a well-established baseline and setting common goals and success indicators—all essential parts of planning tools. One suggestion was that NBS-landscapes can be planned where a minimum level of “success” of NBS can be considered when resiliently building up vital ecosystem functions while delivering the social and ecosystem benefits people expected.
More transparent value chains were seen a precondition, where social media was perceived a tool to remove some distorted market information, especially when the policy development process was too slow. Equally important to identifying entry points, is the development of a common vision for NBS as part of broader efforts to support more sustainable and resilient food systems. This framework for nature-based solutions in agricultural systems is a response to a gap in available tools and guidance on how NBS can be applied to the agriculture sector.
This NBS framework is designed to provide policy makers and practitioners with guidance to develop inclusive, multi-purpose and nature-positive solutions to support the improved management and long-term sustainability of agricultural production systems. It is currently being tested with a companion project planning tool by FAO in five South and Southeast Asian countries to facilitate such intentions.
Looking ahead, Table 3 outlines categories of needed actions and possible concrete examples based on consultations. The framework developed in this report can provide needed guidance to inform this work.
Conclusion
The literature review and case studies presented at the regional workshops indicated that NBS approaches to date have been small in scale and focused on marginal lands at the fringes of major production landscapes. Empirical evidence on NBS and GI for agroecosystems is biased to western contexts. Few reviewed papers presented evidence of socioeconomic benefits of NBS.
The consultations identified limits to and potentials for adoption of the framework in major production landscapes with significant agroecosystem degradation. The consultations recommended that planning of successions is critical for achieving resilient impacts at scale and over time, to 1) select and sequence what and how to intervene to generate positive biophysical interactions and social benefits in and between agroecosystems, and 2) sustainably expand connectivity of positive interactions. In developing contexts, a gradual approach, based on decentralized piloting and demonstration of NBS approaches in a range of ecozones and socioecological contexts, would allow a mosaic of small-scale cases to be connected through a process of exchange and adaptive learning via networks and ecological interconnectedness. Such gradual approach would build up much needed evidence from practices and landscapes on the scalability of best practices and how to adapt NBS principles for implementation in developing countries.
To be effective, NBS in agriculture will require the identification of entry points with the support of a wide range of actors in the production landscape (farmers, communities and resource managers, local government extension workers and advisors at farm and landscapes scales, downstream value chain actors at local and global levels and national policy makers). Partnerships of actors, public and private, based on mutual interest in restoring major production landscapes through NBS are needed to ensure a wide support and the most potential to lead to lasting change in management practice. Policies can support the long-term commitments needed for restorative NBS approaches. The NBS-framework can facilitate the documentation of promising designs and practices for an overarching program of action. The next steps in testing the application of this framework involves reviewing evidence from Asia on the potential contributions of NBS to national polices for climate resilient agriculture, land restoration, biodiversity and sustainable development targets.
Author Contributions
JC-R and BD conceptualised the original idea. BD, JC-R, MC, JH, and ES led consultation workshops. ES led the research and lead-authored this manuscript. JH, CP-G, HT, and MV conducted the review. CP-G, JH, and MV prepared the figure and tables. All authors contributed to analyse results, develop the framework, and write the paper.
Funding
This research was funded by the Food and Agriculture Organization of the United Nations (FAO) under a regional programme on “Identifying nature-based solutions (NBS) and green infrastructure (GI) in the agriculture sector for more resilient rural communities in Asia”.
Author Disclaimer
The views, conclusions and recommendations are those of the authors, not of their organisations.
Conflict of Interest
The authors declare that the research was conducted in the absence of any commercial or financial relationships that could be construed as a potential conflict of interest.
Publisher’s Note
All claims expressed in this article are solely those of the authors and do not necessarily represent those of their affiliated organizations, or those of the publisher, the editors and the reviewers. Any product that may be evaluated in this article, or claim that may be made by its manufacturer, is not guaranteed or endorsed by the publisher.
Acknowledgments
The authors are sincerely grateful for the critical and constructive comments from consultation participants on the technical report leading to this article. The authors also wish to acknowledge two reviewers for valuable comments which have improved the manuscript.
Supplementary Material
The Supplementary Material for this article can be found online at: https://www.frontiersin.org/articles/10.3389/fenvs.2021.678367/full#supplementary-material
References
Albert, C., Brillinger, M., Guerrero, P., Gottwald, S., Henze, J., Schmidt, S., et al. (2020). Planning Nature-Based Solutions: Principles, Steps, and Insights. Ambio 50 (8), 1441–1461. doi:10.1007/s13280-020-01365-1
Albert, C., Schröter-Schlaack, C., Hansjürgens, B., Dehnhardt, A., Döring, R., Job, H., et al. (2017). An Economic Perspective on Land Use Decisions in Agricultural Landscapes: Insights from the TEEB Germany Study. Ecosystem Serv. 25, 69–78. doi:10.1016/j.ecoser.2017.03.020
Anbumozhi, V., Radhakrishnan, J., and Yamaji, E. (2005). Impact of Riparian Buffer Zones on Water Quality and Associated Management Considerations. Ecol. Eng. 24, 517–523. doi:10.1016/j.ecoleng.2004.01.007
Angelstam, P., and Lazdinis, M. (2017). Tall Herb Sites as a Guide For Planning, Maintenance and Engineering of Riparian Continuous Forest Cover. Ecol. Eng. 103, 470–477. doi:10.1016/j.ecoleng.2016.06.099
Are, K. S., Oshunsanya, S. O., and Oluwatosin, G. A. (2018). Changes in Soil Physical Health Indicators of an Eroded Land as Influenced by Integrated Use of Narrow Grass Strips and Mulch. Soil Tillage Res. 184, 269–280. doi:10.1016/j.still.2018.08.009
Bhattacharyya, R., Yi, Z., Yongmei, L., Li, T., Panomtaranichagul, M., Peukrai, S., et al. (2012). Effects of Biological Geotextiles on Aboveground Biomass Production in Selected Agro-Ecosystems. Field Crops Res. 126, 23–36. doi:10.1016/j.fcr.2011.09.006
Borelli, S., Simelton, E., Aggarwal, S., Olivier, A., Conigliaro, M., Hillbrand, A., et al. (2019). Agroforestry and Tenure. Rome, Italy: FAO and ICRAF.
Carter, S., Manceur, A., Seppelt, R., Hermans-Neumann, K., Herold, M., and Verchot, L. (2017). Large Scale Land Acquisitions and REDD+: A Synthesis of Conflicts and Opportunities. Environ. Res. Lett. 12, 035010. doi:10.1088/1748-9326/aa6056
Catacutan, D., Finlayson, R., Gassner, A., Perdana, A., Lusiana, B., Leimona, B., et al. (2018). ASEAN Guidelines for Agroforestry Development. Jakarta, Indonesia: ASEAN Secretariat.
Cecchi, C. (2015). “Towards an EU Research and Innovation Policy Agenda for Nature-based Solutions & Re-naturing Cities: Final Report of the Horizon 2020 Expert Group on 'Nature-based Solutions and Re-Naturing Cities,” in Directorate-General for Research and Innovation (European Commission) (Bruxelles: Publications Office of the European Union).
Cerdà, A., Rodrigo-Comino, J., Giménez-Morera, A., Novara, A., Pulido, M., Kapović-Solomun, M., et al. (2018). Policies Can Help to Apply Successful Strategies to Control Soil and Water Losses. The Case of Chipped Pruned Branches (CPB) in Mediterranean Citrus Plantations. Land Use Policy 75, 734–745. doi:10.1016/j.landusepol.2017.12.052
CFS (2014). Principles for Responsible Investment in Agriculture and Food Systems. Rome, Italy: The Committee on World Food Security (CFS).
Chapman, A., and Darby, S. (2016). Evaluating Sustainable Adaptation Strategies for Vulnerable Mega-Deltas Using System Dynamics Modelling: Rice Agriculture in the Mekong Delta’s An Giang Province, Vietnam. Sci. Total Environ. 559, 326–338. doi:10.1016/j.scitotenv.2016.02.162
Chausson, A., Turner, B., Seddon, D., Chabaneix, N., Girardin, C. A. J., Kapos, V., et al. (2020). Mapping the Effectiveness of Nature‐Based Solutions for Climate Change Adaptation. Glob. Change Biol. 26, 6134–6155. doi:10.1111/gcb.15310
Cohen-Shacham, E., Andrade, A., Dalton, J., Dudley, N., Jones, M., Kumar, C., et al. (2019). Core Principles for Successfully Implementing and Upscaling Nature-Based Solutions. Environ. Sci. Pol. 98, 20–29. doi:10.1016/j.envsci.2019.04.014
Cohen-Shacham, E., Walters, G., Janzen, C., and Maginnis, S. (2016). Nature-Based Solutions to Address Global Societal Challenges. Gland, Switzerland: IUCN.
Corwin, D. L., and Scudiero, E. (2019). “Review of Soil Salinity Assessment for Agriculture Across Multiple Scales Using Proximal and/or Remote Sensors,” in Advances in Agronomy. Editor D. L. Sparks (Cambridge, MA: Academic Press), 1–130.
Daryanto, S., Fu, B., Wang, L., Jacinthe, P.-A., and Zhao, W. (2018). Quantitative Synthesis on the Ecosystem Services of Cover Crops. Earth Sci. Rev. 185, 357–373. doi:10.1016/j.earscirev.2018.06.013
Delbaere, B., Mikos, V., and Pulleman, M. (2014). European Policy Review: Functional Agrobiodiversity Supporting Sustainable Agriculture. J. Nat. Conserv. 22, 193–194. doi:10.1016/j.jnc.2014.01.003
Diop, B., Blanchard, F., and Sanz, N. (2018). Mangrove increases resiliency of the French Guiana shrimp fishery facing global warming. Ecol. Model. 387, 27–37. doi:10.1016/j.ecolmodel.2018.08.014
Dumitru, A., Wendling, L., Eiter, S., and Pilla, F. (2021). “Evaluating the Impact of Nature-Based Solutions,” in A Handbook for Practitioners. (Belgium: Directorate-General for Research and Innovation).
Eggermont, H., Balian, E., Azevedo, J. M. N., Beumer, V., Brodin, T., Claudet, J., et al. (2015). Nature-Based Solutions: New Influence for Environmental Management and Research in Europe. GAIA - Ecol. Perspect. Sci. Soc. 24, 243–248. doi:10.14512/gaia.24.4.9
Eriksen, S., Schipper, E. L. F., Scoville-Simonds, M., Vincent, K., Adam, H. N., Brooks, N., et al. (2021). Adaptation Interventions and Their Effect on Vulnerability in Developing Countries: Help, Hindrance or Irrelevance?. World Dev. 141, 105383. doi:10.1016/j.worlddev.2020.105383
FAO (2016a). “The Agriculture Sectors in the Intended Nationally Determined Contributions: Analysis,” in Environment and Natural Resources Management Working Paper No. 62. Editors R. Strohmaier, J. Rioux, A. Seggel, A. Meybeck, M. Bernoux, M. Salvatore, J. Miranda, and A. Agostini (Rome: Food and Agriculture Organization of the United Nations).
FAO (2016b). “Negotiation, Environment and Territorial Development. Green Negotiated Territorial Development (GreenNTD),” in Land and Water Divison Working Paper 16a. Editors D. Tarrason, G. Andrian, and P. Groppo (Rome, Italy: Food and Agriculture Organization of the United Nations), 40.
FAO (2017). The State of Food and Agriculture: Climate Change, Agriculture and Food Security. Rome: Food and Agriculture Organization of the United Nations.
FAO (2018). The State of the World’s Forests 2018 Forest Pathways to Sustainable Development. Rome: Food and Agriculture Organization of the United Nations.
FOLU (2019). “Growing Better: Ten Critical Transitions to Transform Food and Land Use,” in The Global Consultation Report of the Food and Land Use Coalition September 2019. (Food and Land Use Coalition).
Frątczak, W., Michalska-Hejduk, D., Zalewski, M., and Izydorczyk, K. (2019). Effective Phosphorous Reduction by a Riparian Plant Buffer Zone Enhanced With a Limestone-Based Barrier. Ecol. Eng. 130, 94–100.
Game, E. T., Meijaard, E., Sheil, D., and McDonald-Madden, E. (2014). Conservation in a Wicked Complex World; Challenges and Solutions. Conserv. Lett. 7, 271–277. doi:10.1111/conl.12050
Garibaldi, L. A., Pérez-Méndez, N., Garratt, M. P. D., Gemmill-Herren, B., Miguez, F. E., and Dicks, L. V. (2019). Policies for Ecological Intensification of Crop Production. Trends Ecol. Evol. 34, 282–286. doi:10.1016/j.tree.2019.01.003
Gatto, P., Mozzato, D., and Defrancesco, E. (2019). Analysing the Role of Factors Affecting Farmers’ Decisions to Continue With Agri-Environmental Schemes from a Temporal Perspective. Environ. Sci. Pol. 92, 237–244. doi:10.1016/j.envsci.2018.12.001
GCA (2019). Adapt Now: A Global Call for Leadership on Climate Resilience. Rotterdam and Washington, DC: Global Commission on Adapatation and World Resources Institute.
Gene, S. M., Hoekstra, P. F., Hannam, C., White, M., Truman, C., Hanson, M. L., et al. (2019). The Role of Vegetated Buffers in Agriculture and Their Regulation Across Canada and the United States. J. Environ. Manage. 243, 12–21. doi:10.1016/j.jenvman.2019.05.003
Geussens, K., Van den Broeck, G., Vanderhaegen, K., Verbist, B., and Maertens, M. (2019). Farmers’ Perspectives on Payments for Ecosystem Services in Uganda. Land Use Policy 84, 316–327. doi:10.1016/j.landusepol.2019.03.020
Gikas, G. D., Pérez-Villanueva, M., Tsioras, M., Alexoudis, C., Pérez-Rojas, G., Masís-Mora, M., et al. (2018). Low-Cost Approaches for the Removal of Terbuthylazine from Agricultural Wastewater: Constructed Wetlands and Biopurification System. Chem. Eng. J. 335, 647–656. doi:10.1016/j.cej.2017.11.031
Haller, H., Jonsson, A., Romero, M., and Pascua, M. (2017). Bioaccumulation and Translocation of Field-Weathered toxaphene and Other Persistent Organic Pollutants in Three Cultivars of amaranth (A. cruentus ‘R127 México’, A. cruentus ‘Don León’ y A. caudatus ‘CAC 48 Perú’) – A field Study from Former Cotton Fields in Chinandega, Nicaragua. Ecol. Eng. 121, 65–71. doi:10.1016/j.ecoleng.2017.07.019
Hanson, H. I., Wickenberg, B., and Alkan Olsson, J. (2020). Working on the Boundaries-How do Science Use and Interpret the Nature-Based Solution Concept?. Land Use Policy 90, 104302. doi:10.1016/j.landusepol.2019.104302
Hassanpour, B., Geohring, L. D., Klein, A. R., Giri, S., Aristilde, L., and Steenhuis, T. S. (2019). Application of Denitrifying Bioreactors for the Removal of Atrazine in Agricultural Drainage Water. J. Environ. Manage. 239, 48–56. doi:10.1016/j.jenvman.2019.03.029
Hernández-Morcillo, M., Burgess, P., Mirck, J., Pantera, A., and Plieninger, T. (2018). Scanning Agroforestry-Based Solutions for Climate Change Mitigation and Adaptation in Europe. Environ. Sci. Pol. 80, 44–52. doi:10.1016/j.envsci.2017.11.013
Hiwasaki, L., Luna, E., Syamsidik, , and Marcal, J. A. (2014). Local and Indigenous Knowledge on Climate-Related Hazards of Coastal and Small Island Communities in Southeast Asia. Climatic Change 128, 35–56. doi:10.1007/s10584-014-1288-8
HLPE (2019). Agroecological and Other Innovative Approaches for Sustainable Agriculture and Food Systems that Enhance Food Security and Nutrition. Rome, Italy: High Level Panel of Experts on Food Security and Nutrition of the Committee on World Food Security.
Holt, A. R., Alix, A., Thompson, A., and Maltby, L. (2016). Food Production, Ecosystem Services and Biodiversity: We Can’t Have it All Everywhere. Sci. Total Environ. 573, 1422–1429. doi:10.1016/j.scitotenv.2016.07.139
Huang, Z., Oshunsanya, S. O., Li, Y., Yu, H., and Are, K. S. (2019). Vetiver Grass Hedgerows Significantly Trap P But LIttle N from Sloping Land: Evidenced from a 10-Year Field Observation. Agric. Ecosyst. Environ. 281, 72–80. doi:10.1016/j.agee.2019.05.005
IPCC (2019). Climate Change and Land,” in IPCC Special Report on Climate Change, Desertification, Land Degradation, Sustainable Land Management, Food Security, and Greenhouse Gas Fluxes in Terrestrial Ecosystems. Geneva, Switzerland: Intergovernmental Panel of Climate Change.
Irwin, N. B., Irwin, E. G., Martin, J. F., and Aracena, P. (2018). Constructed Wetlands for Water Quality Improvements: Benefit Transfer Analysis from Ohio. J. Environ. Manage. 206, 1063–1071. doi:10.1016/j.jenvman.2017.10.050
IPBES (2019). Summary for Policymakers of the Global Assessment Report on Biodiversity and Ecosystem Services of the Intergovernmental Science-Policy Platform on Biodiversity and Ecosystem Services. Editors S. Díaz, J. Settele, E. Brondizio, H. Ngo, M. Guèze, J. Agardet al. (Bonn, Germany: IPBES Secretariat).
FAO (2019). “The State of the World’s Biodiversity for Food and Agriculture,” in FAO Commission on Genetic Resources for Food and Agriculture Assessments. Editors J. Bélanger, and D. Pilling (Rome: Food and Agriculture Organization of the United Nations), 572.
Jonsson, A., and Haller, H. (2014). “Sustainability Aspects of In-Situ Bioremediation of Polluted Soil in Developing Countries and Remote Regions,” in Environmental Risk Assessment of Soil Contamination. Editor M. Soriano (London: IntechOpen). doi:10.5772/57315
Karabulut, A. A., Udias, A., and Vigiak, O. (2019). Assessing the Policy Scenarios for the Ecosystem Water Food Energy (EWFE) Nexus in the Mediterranean Region. Ecosyst. Serv. 35, 231–240. doi:10.1016/j.ecoser.2018.12.013
Kay, S., Graves, A., Palma, J. H. N., Moreno, G., Roces-Díaz, J. V., Aviron, S., et al. (2019). Agroforestry is Paying Off - Economic Evaluation of Ecosystem Services in European Landscapes With and Without Agroforestry Systems. Ecosyst. Serv. 36, 100896. doi:10.1016/j.ecoser.2019.100896
Lenka, N. K., Satapathy, K. K., Lal, R., Singh, R. K., Singh, N. A. K., Agrawal, P. K., et al. (2017). Weed Strip Management for Minimizing Soil Erosion and Enhancing Productivity in the Sloping Lands of North-Eastern India. Soil Tillage Res. 170, 104–113. doi:10.1016/j.still.2017.03.012
Liang, X., Lin, L., Ye, Y., Gu, J., Wang, Z., Xu, L., et al. (2015). Nutrient Removal Efficiency in a Rice-Straw Denitrifying Bioreactor. Bioresour. Tech. 198, 746–754. doi:10.1016/j.biortech.2015.09.083
Liu, H., Yao, L., Lin, C., Wang, X., Xu, W., and Wang, H. (2018). 18-Year Grass Hedge Effect on Soil Water Loss and Soil Productivity on Sloping Cropland. Soil Tillage Res. 177, 12–18. doi:10.1016/j.still.2017.11.007
Mander, Ü., Hayakawa, Y., and Kuusemets, V. (2005). Purification Processes, Ecological Functions, Planning and Design of Riparian Buffer Zones in Agricultural Watersheds. Ecol. Eng. 24, 421–432. doi:10.1016/j.ecoleng.2005.01.015
Mander, Ü., Tournebize, J., Sauvage, S., and Sánchez-Perez, J. M. (2017). Wetlands and Buffer Zones in Watershed Management. Ecol. Eng. 103, 289–295. doi:10.1016/j.ecoleng.2016.12.005
McIvor, I., Youjun, H., Daoping, L., Eyles, G., and Pu, Z. (2017). Agroforestry: Conservation Trees and Erosion Prevention. Reference Module in Food Science. Amsterdam, Netherlands: Elsevier.
McWilliam, W., and Balzarova, M. (2017). The Role of Dairy Company Policies in Support of Farm Green Infrastructure in the Absence of Government Stewardship Payments. Land Use Policy 68, 671–680. doi:10.1016/j.landusepol.2017.08.030
MEA (2005). Millennium Ecosystem Assessment - Ecosystems and Human Well-being: Synthesis. Washington, DC: World Resources Institute, Island Press, 137.
Melathopoulos, A. P., Cutler, G. C., and Tyedmers, P. (2015). Where is the Value in Valuing Pollination Ecosystem Services to Agriculture?. Ecol. Econ. 109, 59–70. doi:10.1016/j.ecolecon.2014.11.007
Monteiro, R., Ferreira, J. C., and Antunes, P. (2020). Green Infrastructure Planning Principles: An Integrated Literature Review. Land 9, 525. doi:10.5194/gmd-2020-302-rc1
Mtibaa, S., Hotta, N., and Irie, M. (2018). Analysis of the Efficacy and Cost-Effectiveness of Best Management Practices for Controlling Sediment Yield: A Case Study of the Joumine Watershed, Tunisia. Sci. Total Environ. 616-617, 1–16. doi:10.1016/j.scitotenv.2017.10.290
Mulazzani, L., Camanzi, L., and Malorgio, G. (2019). Multifunctionality in Fisheries and the Provision of Public Goods. Ocean Coastal Manag. 168, 51–62. doi:10.1016/j.ocecoaman.2018.10.037
Namirembe, S., McFatridge, S., Duguma, L., Bernard, F., Minang, P., Sassen, M., et al. (2015). “Agroforestry: An attractive REDD+ Policy Option?,” in ICRAF & UNEP WCMC. Editors S. Hussain, K. Sharma, and I. Mulder. Geneva: The Economics of Ecosystems and Biodiversity (TEEB).
Naumov, V., Manton, M., Elbakidze, M., Rendenieks, Z., Priednieks, J., Uhlianets, S., et al. (2018). How to Reconcile Wood Production and Biodiversity Conservation? The Pan-European Boreal Forest History Gradient as an “Experiment”. J. Environ. Manage. 218, 1–13. doi:10.1016/j.jenvman.2018.03.095
NBS-Facilitation Team (2019). “Compendium of Contributions Nature-Based Solutions,” in Nature-Based Solutions (NBS) Facilitation Team of the 2019 UN Climate Action Summit. Nairobi: NBS-Facilitation Team UNON, Publishing Services Section.
Nesshöver, C., Assmuth, T., Irvine, K. N., Rusch, G. M., Waylen, K. A., Delbaere, B., et al. (2017). The Science, Policy and Practice of Nature-Based Solutions: An Interdisciplinary Perspective. Sci. Total Environ. 579, 1215–1227. doi:10.1016/j.scitotenv.2016.11.106
Novara, A., Minacapilli, M., Santoro, A., Rodrigo-Comino, J., Carrubba, A., Sarno, M., et al. (2019). Real Cover Crops Contribution to Soil Organic Carbon Sequestration in Sloping Vineyard. Sci. Total Environ. 652, 300–306. doi:10.1016/j.scitotenv.2018.10.247
O’Sullivan, F., Mell, I., and Clement, S. (2020). Novel Solutions or Rebranded Approaches: Evaluating the Use of Nature-Based Solutions (NBS) in Europe. Front. Sust. Cities 2. doi:10.3389/frsc.2020.572527
OECD (2020). Nature-Based Solutions for Adapting to Water-Related Climate Risks, OECD Environment Policy Papers, No. 21. Paris: OECD Publishing.
Onuma, A., and Tsuge, T. (2018). Comparing Green Infrastructure as Ecosystem-Based Disaster Risk Reduction with Gray Infrastructure in Terms of Costs and Benefits Under Uncertainty: A Theoretical Approach. Int. J. Disaster Risk Reduct. 32, 22–28. doi:10.1016/j.ijdrr.2018.01.025
Ovando, P., and Brouwer, R. (2019). A Review of Economic Approaches Modeling the Complex Interactions Between Forest Management and Watershed Services. For. Pol. Econ. 100, 164–176. doi:10.1016/j.forpol.2018.12.007
Phelps, J., Carrasco, L. R., Webb, E. L., Koh, L. P., and Pascual, U. (2013). Agricultural Intensification Escalates Future Conservation Costs. Proc. Natl. Acad. Sci. 110, 7601–7606. doi:10.1073/pnas.1220070110
Phelps, J., Dermawan, A., and Garmendia, E. (2017). Institutionalizing Environmental Valuation Into Policy: Lessons from 7 Indonesian Agencies. Glob. Environ. Change 43, 15–25. doi:10.1016/j.gloenvcha.2017.01.004
Pluer, W. T., Morris, C. K., Walter, M. T., and Geohring, L. D. (2019). Denitrifying Bioreactor Response During Storm Events. Agric. Water Manag. 213, 1109–1115. doi:10.1016/j.agwat.2018.12.004
Quintella, C. M., Mata, A. M. T., and Lima, L. C. P. (2019). Overview of Bioremediation with Technology Assessment and Emphasis on Fungal Bioremediation of Oil Contaminated Soils. J. Environ. Manage. 241, 156–166. doi:10.1016/j.jenvman.2019.04.019
Rahman, M. M., and Mahmud, M. A. (2018). Economic Feasibility of Mangrove Restoration in the Southeastern Coast of Bangladesh. Ocean Coastal Manag. 161, 211–221. doi:10.1016/j.ocecoaman.2018.05.009
Raymond, C. M., Berry, P., Breil, M., Nita, M. R., Kabisch, N., de Bel, M., et al. (2017). “An Impact Evaluation Framework to Support Planning and Evaluation of Nature-based Solutions Projects,” in Report Prepared by the EKLIPSE Expert Working Group on Nature-based Solutions to Promote Climate Resilience in Urban Areas. (Wallingford, United Kingdom: Centre for Ecology & Hydrology).
Rega, C., Bartual, A. M., Bocci, G., Sutter, L., Albrecht, M., Moonen, A.-C., et al. (2018). A Pan-European Model of Landscape Potential to Support Natural Pest Control Services. Ecol. Indic. 90, 653–664. doi:10.1016/j.ecolind.2018.03.075
Rosa-Schleich, J., Loos, J., Mußhoff, O., and Tscharntke, T. (2019). Ecological-Economic Trade-Offs of Diversified Farming Systems - A Review. Ecol. Econ. 160, 251–263. doi:10.1016/j.ecolecon.2019.03.002
Rosas-Ramos, N., Baños-Picón, L., Tobajas, E., de Paz, V., Tormos, J., and Asís, J. D. (2018). Value of Ecological Infrastructure Diversity in the Maintenance of Spider Assemblages: A Case Study of Mediterranean Vineyard Agroecosystems. Agric. Ecosyst. Environ. 265, 244–253. doi:10.1016/j.agee.2018.06.026
Rosenstock, T., Wilkes, A., Jallo, C., Namoi, N., Bulusu, M., Suber, M., et al. (2018). Making Trees Count: Measurement, Reporting and Verification of Agroforestry Under the UNFCCC. Wageningen, Netherlands: CGIAR Research Program on Climate Change, Agriculture and Food Security (CCAFS).
Schipper, E. L. F. (2020). Maladaptation: When Adaptation to Climate Change Goes Very Wrong. One Earth 3, 409–414. doi:10.1016/j.oneear.2020.09.014
Seddon, N., Smith, A., Smith, P., Key, I., Chausson, A., Girardin, C., et al. (2021). Getting the Message Right on Nature-Based Solutions to Climate Change. Glob. Change Biol. 27, 1518–1546.doi:10.1111/gcb.15513
Shah, S. I. A., Zhou, J., and Shah, A. A. (2019). Ecosystem-Based Adaptation (EbA) Practices in Smallholder Agriculture; Emerging Evidence from Rural Pakistan. J. Clean. Prod. 218, 673–684. doi:10.1016/j.jclepro.2019.02.028
Singh, V., Sinha, R., Nayak, D., Neufeldt, H., van Noordwijk, M., and Rizvi, J. (2016). The National Agroforestry Policy of India: Experiential Learning in Development and Delivery Phases. New Delhi: World Agroforestry Centre.
Sonneveld, B. G. J. S., Merbis, M. D., Alfarra, A., Ünver, O., and Arnal, M. A. (2018). Nature-Based Solutions for Agricultural Water Management and Food Security. Rome: FAO, 66.
Stokes, A., Sotir, R., Chen, W., and Ghestem, M. (2010). Soil Bio- and Eco-Engineering in China: Past Experience and Future Priorities. Ecol. Eng. 36, 247–257. doi:10.1016/j.ecoleng.2009.07.008
Tenneson, K., Patterson, M. S., Jadin, J., Rosenstock, T., Mulia, R., Kim, J., et al. (2021). Commodity-Driven Forest Loss: A Study of Southeast Asia. Washington, DC: USAID, 196.
Tiodar, E. D., Văcar, C. L., and Podar, D. (2021). Phytoremediation and Microorganisms-Assisted Phytoremediation of Mercury-Contaminated Soils: Challenges and Perspectives. Int. J. Environ Res. Public Health 18, 2435. doi:10.3390/ijerph18052435
Tran, H. T., and Bui, D. T. (2013). Cost-Benefit Analysis of Mangrove Restoration in Thi Nai Lagoon, Quy Nhon City, Vietnam in Asian Cities Climate Resilience Working Paper. London: IIED.
UNDRR (2020). Ecosystem-Based Disaster Risk Reduction: Implementing Nature-based Solutions for Resilience. Thailand: United Nations Office for Disaster Risk Reduction – Regional Office for Asia and the Pacific.
UNEP (2021). Making Peace With Nature: A Scientific Blueprint to Tackle the Climate, Biodiversity and Pollution Emergencies. Nairobi, Kenya: UN Enviroment Programme.
van Noordwijk, M., Gitz, V., Minang, P. A., Dewi, S., Leimona, B., Duguma, L., et al. (2020). People-Centric Nature-Based Land Restoration Through Agroforestry: A Typology. Land 9, 251. doi:10.3390/land9080251
Villaverde, J., Rubio-Bellido, M., Lara-Moreno, A., Merchan, F., and Morillo, E. (2018). Combined Use of Microbial Consortia Isolated from Different Agricultural Soils and Cyclodextrin as a Bioremediation Technique for Herbicide Contaminated Soils. Chemosphere 193, 118–125. doi:10.1016/j.chemosphere.2017.10.172
Wang, Y., Hu, Y., Yang, C., and Chen, Y. (2018). Effects of Vegetation Types on Water-Extracted Soil Organic Matter (WSOM) from Riparian Wetland and Its Impacts on Riverine Water Quality: Implications for Riparian Wetland Management. Sci. Total Environ. 628-629, 1249–1257. doi:10.1016/j.scitotenv.2018.02.061
Watson, K. B., Ricketts, T., Galford, G., Polasky, S., and O’Niel-Dunne, J. (2016). Quantifying Flood Mitigation Services: The Economic Value of Otter Creek Wetlands and Floodplains to Middlebury, VT. Ecol. Econ. 130, 16–24. doi:10.1016/j.ecolecon.2016.05.015
Wolz, K. J., and DeLucia, E. H. (2018). Alley Cropping: Global Patterns of Species Composition and Function. Agric. Ecosyst. Environ. 252, 61–68. doi:10.1016/j.agee.2017.10.005
Zhang, C., Xue, J., Cheng, D., Feng, Y., Liu, Y., Aly, H. M., et al. (2019). Uptake, Translocation and Distribution of Three Veterinary Antibiotics in Zea Mays L. Environ. Pollut. 250, 47–57. doi:10.1016/j.envpol.2019.03.110
Zomer, R. J., Neufeldt, H., Xu, J., Ahrends, A., Bossio, D., Trabucco, A., et al. (2016). Global Tree Cover and Biomass Carbon on Agricultural Land: The Contribution of Agroforestry to Global and National Carbon Budgets. Sci. Rep. 6, 29987. doi:10.1038/srep29987
Zuazo, V. H. D., Pleguezuelo, C. R. R., Peinado, F. J. M., de Graaff, J., Martínez, J. R. F., and Flanagan, D. C. (2011). Environmental Impact of Introducing Plant Covers in the Taluses of Terraces: Implications for Mitigating Agricultural Soil Erosion and Runoff. CATENA 84, 79–88. doi:10.1016/j.catena.2010.10.004
Keywords: green infrastructure (GI), sustainable agriculture, nature-based solutions (nbs), restoration, agrobiodiversity conservation, people-centered, climate-resilience
Citation: Simelton E, Carew-Reid J, Coulier M, Damen B, Howell J, Pottinger-Glass C, Tran HV and Van Der Meiren M (2021) NBS Framework for Agricultural Landscapes. Front. Environ. Sci. 9:678367. doi: 10.3389/fenvs.2021.678367
Received: 09 March 2021; Accepted: 20 July 2021;
Published: 05 August 2021.
Edited by:
Fabrice Renaud, University of Glasgow, United KingdomReviewed by:
Francisco Comín, Superior Council of Scientific Investigations, SpainPeter William Clinton, New Zealand Forest Research Institute Limited (Scion), New Zealand
Copyright © 2021 Simelton, Carew-Reid, Coulier, Damen, Howell, Pottinger-Glass, Tran and Van Der Meiren. This is an open-access article distributed under the terms of the Creative Commons Attribution License (CC BY). The use, distribution or reproduction in other forums is permitted, provided the original author(s) and the copyright owner(s) are credited and that the original publication in this journal is cited, in accordance with accepted academic practice. No use, distribution or reproduction is permitted which does not comply with these terms.
*Correspondence: Elisabeth Simelton, e.simelton@cgiar.org