- iES Landau (Institute for Environmental Sciences), University of Koblenz-Landau, Landau, Germany
A climatic shift from temperate to arid conditions is predicted for Northwest Africa. Water temperature, salinity, and river intermittency are likely to increase, which may impact freshwater communities, ecosystem functioning, and related ecosystem services. Quantitative data and information on the impact of climate change on insect communities (e.g., richness, taxonomic and trait composition) are still scarce for Northwest Africa. In this study, we extracted information on freshwater insect occurrence and environmental variables in Northwest Africa from the results of a literature search to study potential consequences of changing climatic conditions for these communities. Our data set covered 96 families in 165 sites in Morocco and Algeria. We quantified the impact of several explanatoryvariables (climate, altitude, water temperature, conductivity, intermittency, flow, aridity, dams, and land cover) on richness, taxonomic and functional trait composition using negative binomial regression models and constrained ordination. Family richness in arid sites was on average 37 % lower than in temperate sites in association with flow, river regulation, cropland extent, conductivity, altitude, and water temperature. With 36 % of the studied temperate sites predicted to turn arid by the end of the century, a loss of insect families can be predicted for Northwest Africa, mainly affecting species adapted to temperate environments. Resistance and resilience traits such as small body size, aerial dispersal, and air breathing promote survival in arid climates. Future research should report insect occurrences on species level to allow for better predictions on climate change effects.
Introduction
Northwest Africa is characterized by the temperate Mediterranean and arid Saharan climate and is subject to droughts (Schilling et al., 2012; Waha et al., 2017). In this region, rivers can be considered the arteries of life because all life is clustered around them. Arid climate is predicted to extend northwards covering increasing parts of the Maghreb until the mid- and late-21st century (Born et al., 2008; Beck et al., 2018). This will likely lead to increased salinity levels and flow intermittency in rivers (Williams, 1999) potentially affecting biodiversity and dependent human livelihoods (Arthington et al., 2010; Berger et al., 2018) through changes in the supply of ecosystem goods and services. Besides climate change, water abstraction (Dewson et al., 2007), flow modification (Martínez et al., 2013) and additional human-induced forms of secondary salinisation (Cañedo-Argüelles et al., 2013) related to human land use in the river catchment may exacerbate salinity levels and flow intermittency.
Benthic macroinvertebrates play an important role in the functioning of freshwater ecosystems (Wallace & Webster, 1996; Covich et al., 1999). By processing dead and living material and transferring the energy to freshwater and adjacent terrestrial food webs, macroinvertebrates contribute to ecosystem stability (Wallace and Webster, 1996; Giller et al., 2004). Macroinvertebrate abundance and richness has been shown to decrease with environmental stressors such as high water temperature (Durance and Ormerod, 2007), high salinity) (Kefford, 1998; Arribas et al., 2019), and flow intermittency (Beauchard et al., 2003; Stubbington et al., 2009; Smeti et al., 2019). This, in turn, has been associated with a reduction in ecosystem functions such as nutrient cycling (Cao et al., 2018) and organic matter breakdown (Smeti et al., 2019). The anticipated increase in water temperature, salinity, and flow intermittency with future climate change suggests a reduction in macroinvertebrate abundance and richness, which may result in the local extinction of species in Northwest Africa. A loss of species can potentially be compensated by functionally similar species (Solan, 2004) as long as this species can maintain or increase its abundance. However, in the absence of compensation, the reduction of ecosystem functions through the loss of insect abundance and richness could adversely affect ecosystem services and human health (Strange et al., 1999).
Species that can thrive in arid climates show adaptations to drought and salinity or the ability to quickly recolonize after droughts. Resistance traits (e.g., desiccation resistance) can enable species to withstand long droughts (Stubbington et al., 2017). Ovoviviparity, multi-voltinism, and filter- and deposit-feeding, have been associated with increased salinity (Piscart et al., 2006; Szöcs et al., 2014). High female dispersal and short life cycles promote recolonization after droughts (Stubbington et al., 2017). Traits that increase resistance and resilience typically increase in communities that persist in harsh climatic conditions, because species that lack such traits go locally extinct. Notwithstanding, the occurrence of specific traits is not only driven by environmental conditions but complex abiotic and biotic interactions under phylogenetic constraints (Hamilton et al., 2020).
Studies on macroinvertebrates in Northwest Africa are still scarce and often focus on water quality and anthropogenic stressors on a local scale, and less on climate effects in the whole region. Trends in macroinvertebrate distribution and richness patterns in North Africa can be assessed by using a combination of climatic and hydrological variables (Beauchard et al., 2003). However, little is known about the impact that climate change can have on insect communities. While insect richness and abundance are decreasing as a response to anthropogenic land use intensification (Seibold et al., 2019), macroinvertebrate richness may increase in temperate regions of Europe by a northward shift of species from the highly diverse Mediterranean climate (Bonada et al., 2007). As a consequence of the increasing arid climate in Northwest Africa (Beck et al., 2018), a loss of richness, however, can be expected. Understanding the effects of arid and temperate climate on insect communities in Northwest Africa may help establish future scenarios for insect communities and identify consequences for food webs, ecosystem functions, and related ecosystem services.
We compiled data for Northwest Africa to quantify the associations between aquatic insect communities and environmental variables. The aim of our study was to study the response of aquatic insect richness to a change of climates based on an increase of arid conditions. Furthermore, we aimed to identify insect families that are likely vulnerable to climate change and traits that enable insects to thrive under arid conditions.
We hypothesized that (i) aquatic insect richness in Northwest Africa is lower in arid than in temperate climates; (ii) insect family composition differs between arid and temperate climates, in association with (iii) changes in specific traits, such as small body size, short life cycles, resistance against drought and salinity, and the ability for air breathing.
Materials and Methods
Data Collection
Literature Search and Insect Occurrence Data
We used Web of Knowledge, Google Scholar, and the database MacroMED (Blanco-Garrido et al., 2013) to search for publications about macroinvertebrates in Northwest Africa using the keywords “macroinvertebrate*,” “invertebrate*,” and “insect*” together with “Algeria,” “Morocco,” and “Tunisia,” as well as their French translations. Given that most publications were limited to aquatic insects and Morocco and Algeria, we limited our study to this group in these two countries (Figure 1). Moreover, the references of the retrieved publications were screened for additional studies, called footnote chasing. The literature search was completed on June 15th, 2020. Publications that reported 1) presence/absence data for aquatic insects (not restricted to specific orders), 2) water temperature and conductivity, (iii) and were conducted in intermittent or permanent rivers, were used in the analysis (Supplementary Figure S1). This resulted in 18 publications (Supplementary Table S1). In all studies, aquatic insects were sampled by using either a Surber sampler or hand/kick net, except for Khebiza et al. (2006) that used a membrane pump. The sampling method was not reported in Giudicelli and Dakki (1984).
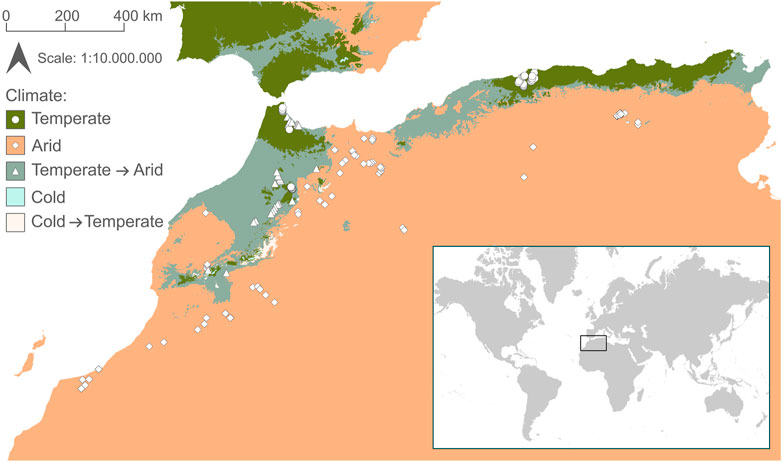
FIGURE 1. Map of sample sites in Northwest Africa. Shape of points indicates climatic region (dots = temperate; diamonds = arid; triangles = temperate climate predicted to shift to arid by 2071–2100). Climate classification is based on Beck et al. (2018).
Insect and Environmental Variables
Insect and environmental variables were sampled one to 12 times per site. Therefore, we included “Number of samples per site” as a variable, to allow for checking the effect of the sampling frequency per site on family richness. Based on the given taxonomic resolution, we used the family level.
11 variables were gathered for analysis (Table 1), which describe the hydrological, geographic, and climatic conditions, as well as land cover. Water temperature and water electrical conductivity as a surrogate measure for salinity (hereafter conductivity) were extracted directly from tables or graphs in the selected papers using WebPlotDigitizer (Rohatgi, 2020). In the case of repeated measurements, a mean was calculated, because several publications already reported mean values. Coordinates (World Geodetic System 1984 Coordinate system (WGS-84)) and altitude were extracted directly from the publications. If information was missing, the location and altitude of the sites were estimated based on the presented maps. The general flow regime (permanent or intermittent) was extracted from the publications. River area, degree of regulation, Global Aridity Index, cropland extent, and urban extent (spatial extent of cropland/urban areas in reach catchment), were extracted from the RiverATLAS (version 1.0; Linke et al., 2019) using QGIS 3.8.2-Zanzibar (QGIS.org, 2021). Finally, the current and future climatic class of each site (arid, temperate) was assigned using the Köppen-Geiger climate classification map for present-day and future climate (Beck et al., 2018).
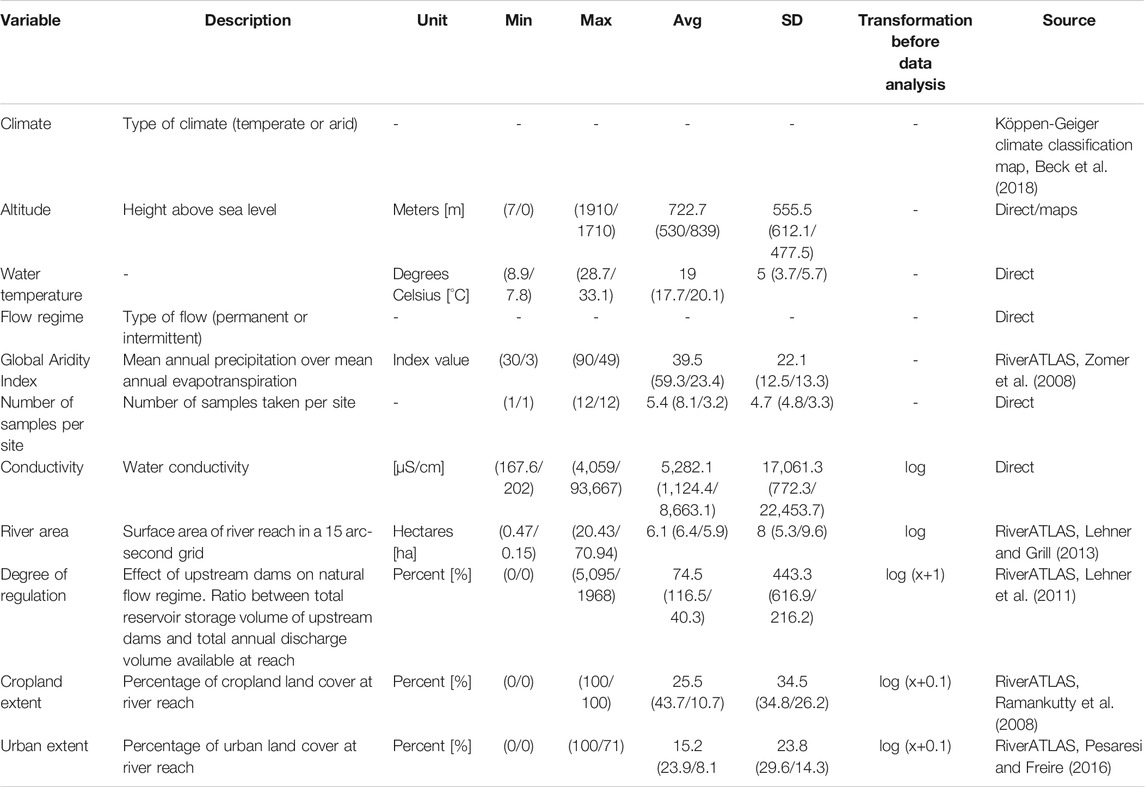
TABLE 1. Environmental and anthropogenic variables used in the analyses with their unit, minimum (Min), maximum (Max) and average (Avg) values, standard deviation (SD), used transformation before data analysis and the source of the variables (“direct” indicates direct extraction from publications). Values for temperate and arid climate are reported in brackets, separated by a slash.
Trait Data
We used the trait database of Tachet et al. (2010) to obtain information for the insect families, including 11 biological traits with 60 modalities (Supplementary Table S2) associated with resilience and resistance to disturbances (Bonada et al., 2007). Among these are included traits such as size, life-cycle duration, reproduction, and feeding behavior. For each of these biological traits, different trait modalities are defined and the affinity of the taxa to each trait modality is represented by fuzzy coded scores (0 = no affinity, 1 = low affinity, 2 = moderate affinity, 3 = high affinity). These codes were transformed to relative frequencies for a given family. If traits were defined for a lower taxonomic level than family in the trait database, we assigned traits as follows: i) If only one species of a family occurred in the study region and traits were available for the species, these traits were assigned to the family. ii) In the case of multiple species from a family, we calculated a weighted mean based on its relative frequency of occurrence with respect to the total number of sites. For each site, we calculated an insect assemblage trait profile based on the relative frequencies of trait modalities for each family and the presence data of these families at a given site, by taking the sum for each trait modality from all present insect families and transforming these sums into relative frequencies for each trait (similar to Mondy et al., 2016). Trait information was missing for 15 out of 96 families and for four families few specific traits were missing. These families or traits were not considered for the calculation of the insect assemblage trait profiles.
Data Analysis
For all data analyses, we used RStudio (version 1.2.5019) and the packages “Vegan” (Oksanen et al., 2019), “car” (Fox and Weisberg, 2018), “DHARMa” (Hartig, 2020), “MASS” (Venables and Ripley, 2002), and “faraway” (Faraway, 2016), “reghelper” (Hughes and Team, 2017), “modEvA” (Márcia Barbosa et al., 2013), “indicspecies” (De Cáceres et al., 2016), “beanplot” (Kampstra, 2008), “MuMIn” (Barton and Barton, 2015).
Association Between Family Richness and Environmental Variables
To analyze for differences in the richness of insect families between arid and temperate climate (hypothesis 1), we used Welch’s t-test given heterogeneous variances (Figure 2). To identify environmental variables that can explain family richness, we used negative binomial regression models. The variable “Climate” was removed from analyses due to collinearity (VIF >5) with the Global Aridity Index, which captures climatic conditions in terms of evaporation and precipitation. Five variables were log-transformed prior analysis because they were left-skewed (Table 1). We used a backward stepwise model selection by AIC to select the best fitting model. The resulting best-fit model was checked for model assumptions.
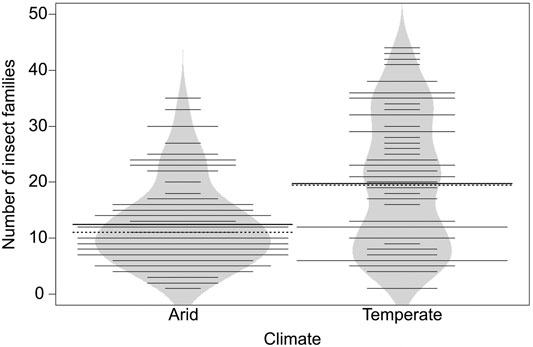
FIGURE 2. Beanplot for number of insect families for arid (n = 91) and temperate climate (n = 74) in Northwest Africa. Bold line indicates mean of each group (arid = 12.44; temperate = 19.76), dashed lines indicates median of each group (arid = 11; temperate = 19.5), thin lines individual observations, polygon and length of lines density of observations. Means differ significantly between the two climates (Welch’s t-test, n = 165, t (116.47) = −4.6, p = < 0.001). For details on beanplots see Kampstra (2008).
Association Between Family Composition and Environmental Variables
Before analysis, we excluded rare families that occurred in less than five percent of sites (<8 sites) from the analysis (Legendre and Gallagher, 2001). Canonical Correspondence Analysis (CCA) was used to analyze the relationship between insect families and environmental variables (hypothesis 2). We selected CCA because a detrended correspondence analysis (DCA) showed a unimodal gradient (gradient length of 3.29) (Legendre and Legendre, 2012). The best fit model was selected using a stepwise model selection with the adjusted R2 as the goodness of fit metric.
To identify families that are characteristic for arid and temperate climate, we used an Indicator species analysis. For each family, an indicator value was calculated, which is highest when taxa are either present in one of the climates but not the other or present in all sites of one climate (Borcard et al., 2018). We checked for a significant association of families with the climate types by using a permutational test.
Association Between Traits and Environmental Variables
To analyze the association of traits with climate change-related environmental variables (hypothesis 3), we used Redundancy Analysis (RDA) given a gradient length of 1.37 in DCA (Legendre & Legendre, 2012), by using the insect assemblage trait profiles. As in CCA, stepwise model selection was used to identify the best-fit model.
Results
The literature search resulted in 766 publications, of which 724 were excluded after screening titles and abstracts (Supplementary Figure S1). Of the remaining 42 publications, we included 18 in our analysis that met the inclusion criteria (Supplementary Table S1). Most publications were excluded based on missing taxa lists or environmental parameters. The number of sites in the publications ranged from 1 to 28 (mean = 9.2). In total there were 165 sites covering a wide range of environmental conditions (Table 1). 91 sites were located in arid climate and 74 in temperate climate, of which 27 (36%) are predicted to shift towards arid climate by 2071–2,100 (Figure 1).
Association Between Family Richness and Environmental Variables
The number of families per site ranged from 1 to 44 (mean = 15.72, SD = 10.33), with 96 families in total. Family richness was 37% lower in arid (n = 91, mean = 12.44, SD = 7.39, median = 11) compared with temperate (n = 74, mean = 19.76, SD = 11.94, median = 19.5) climate (Welch’s t-test, n = 165, t (116.47) = −4.6, p < 0.001) (Figure 2).
Variation in family richness was associated with altitude, flow regime, conductivity, cropland extent, degree of regulation, and water temperature. Family richness was lower in sites with higher altitude, higher regulation, higher conductivity, and intermittent flow, but increased with higher water temperature and larger extent of cropland areas (Table 2). The variables “Number of samples per site,” “Global Aridity Index,” “River area,” and “Urban extent” were not included in the best-fit model.
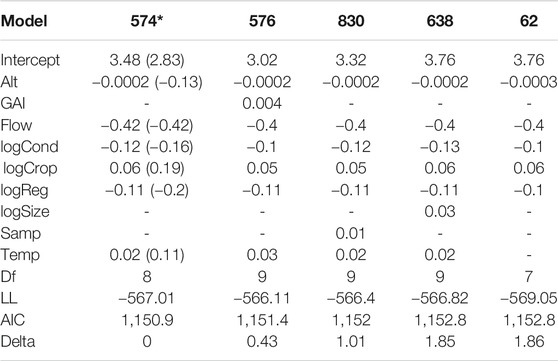
TABLE 2. AIC model selection with regression estimates for the included environmental variables (altitude (Alt), Global Aridity Index (GAI), intermittent flow regime (Flow), conductivity (log-transformed; logCond), cropland extent (log-transformed; logCrop), degree of regulation (log-transformed; logReg), river size (log-transformed; logSize), number of samples per site (Samp), water temperature (Temp)), degrees of freedom (Df), Log-likelihood (LL), information score of the model (AIC), delta and AIC weight for all models within a delta of 2 (* = final model). Regression estimates with standardized variables are indicated in brackets for the final model.
Association Between Family Composition and Environmental Variables
The best-fitting CCA model for community composition included water temperature, number of samples per site, altitude, flow regime, conductivity, cropland extent, urban extent, Global Aridity Index, river area and degree of regulation. These variables explained 18% of the variation, where 7.1% of the total variation (39% of constrained variation) was explained by the first two axes. Axis 1 shows a gradient of water temperature, conductivity, and aridity (Figure 3). Axis 2 shows a gradient of number of samples per site and urban extent. Most insect families occurred in less arid sites with low or medium water temperature and conductivity (Figure 3) such as Ephemeroptera and Plecoptera. The family Micronectidae was associated with aridity and high water temperature and conductivity. Most families of Diptera occurred more frequently in sites characterized by a higher extent of croplands and urbanization.
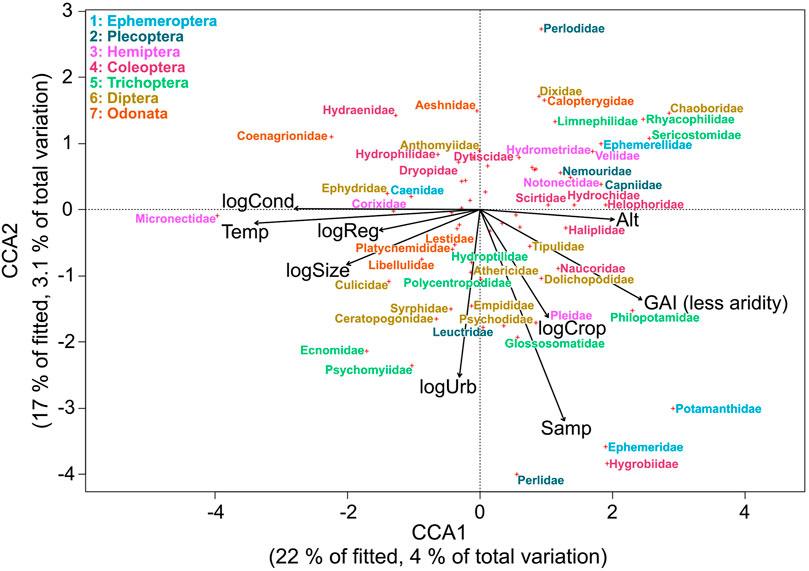
FIGURE 3. CCA ordination distance triplot (scaling 1) with the relationship of environmental variables (altitude (Alt), water temperature (Temp), conductivity (log-transformed; logCond), number of samples per site (Samp), river area (log-transformed; logSize), degree of regulation (log-transformed; logReg), urban extent (log-transformed; logUrb), cropland extent (log-transformed; logCrop), Global Aridity Index (GAI)), and aquatic insect families in Northwest Africa. To avoid cluttering, overlapping labels were omitted from the plot.
In the Indicator Species Analysis, one family (Micronectidae) was associated with arid climate and 33 (48%) families were associated with temperate climate (Table 3).
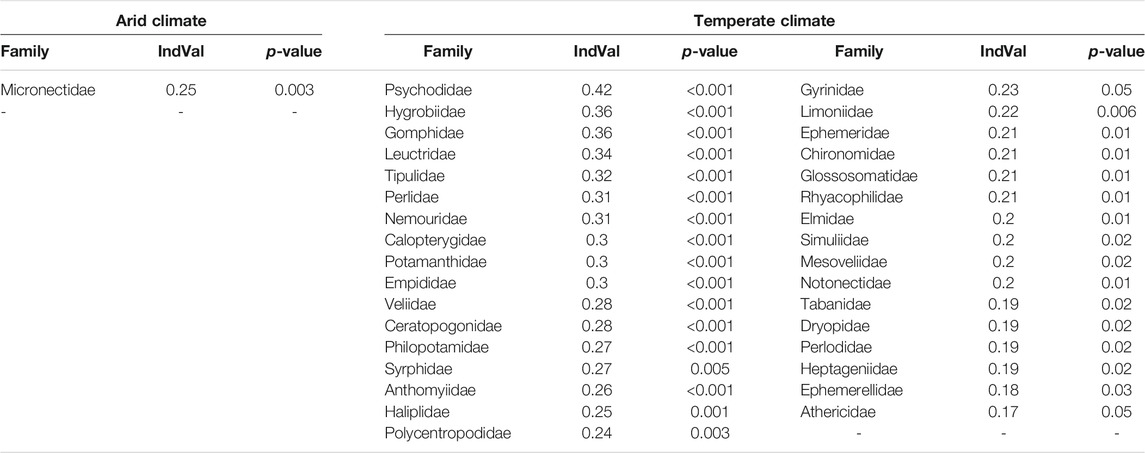
TABLE 3. Results of Indicator Species Analysis showing families associated with arid and temperate climate, test statistics and indicator value (IndVal).
Association Between Traits and Environmental Variables
In the best-fitting RDA model, the assemblage trait profile was explained by conductivity, degree of regulation, water temperature, urban extent, aridity, and flow regime. These variables explained 19% of the variation, of which 16% of the total variation (82% of the constrained variation) was explained by the first two axes. Axis 1 represents a gradient of conductivity urban area extent and degree of regulation (Figure 4), whereas axis 2 shows a gradient of water temperature, conductivity, degree of regulation, and flow regime. The traits “Life cycle duration,” “Reproduction,” and “Respiration” were mainly associated with axis 1, whereas “Potential number of cycles/year,” “Dispersal,” and “Food” were mainly associated with axis 2 (Figure 4).
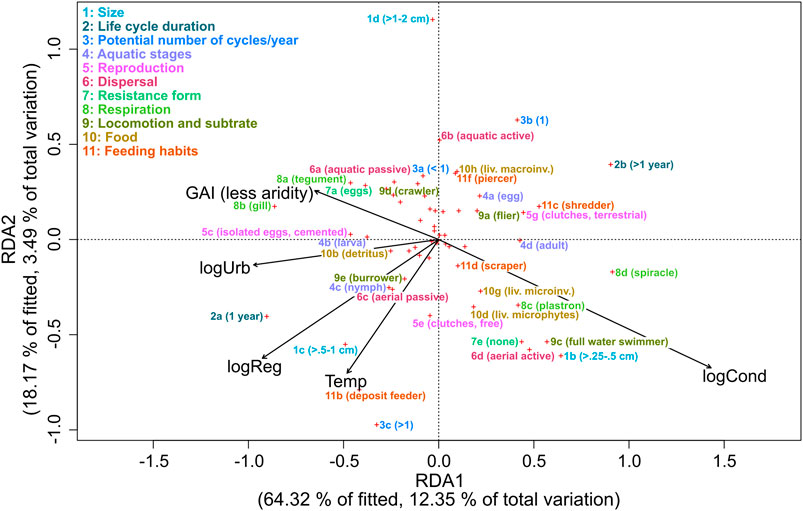
FIGURE 4. RDA ordination distance triplot (Scaling 1) with the relationship of environmental variables (conductivity (log-transformed; logCond), degree of regulation (log-transformed; logReg), water temperature (Temp) urban extent (log-transformed; logUrb), and Global Aridity Index (GAI)), and traits in Northwest Africa. Only traits that correlate strongly with the environmental variables are displayed with names.
Discussion
Association Between Family Richness and Environmental Variables
In Northwest Africa, large parts of temperate climate are predicted to turn to arid climate by the end of the century (Beck et al., 2018), and this will likely affect 36% of the temperate sites in our study. On average family richness was 37% lower in arid than in temperate climates, supporting our first hypothesis. Hence, the family richness of sites that will shift towards an arid climate will likely decrease over time. Although the loss of some families could be partly compensated by the invasion of other families adapted to arid environments, this may be insufficient to maintain the current level of richness (Dornelas et al., 2019; Morghad et al., 2019).
The loss of insect family richness was associated with a shift towards intermittent flow and higher conductivity. These findings are in accordance with several studies on macroinvertebrate richness in intermittent (Beauchard et al., 2003; Soria et al., 2017) and highly saline rivers (Velasco et al., 2006; Arribas et al., 2019; Berger et al., 2021). With the decrease of precipitation and the increase of evapotranspiration predicted in future climate scenarios for Northwest Africa (Terink et al., 2013), intermittency is likely to increase (Döll & Schmied, 2012). An increase in water and soil salinity can also be expected in association with droughts, as well as a reduction in flow due to human alterations of rivers, and water abstraction for irrigation of agricultural lands (Williams, 1999; Pitman and Läuchli, 2002; Herbert et al., 2015). This suggests future species loss. With family richness being on average 37% lower in arid than in temperate sites, our model suggests that intermittent flow and increasing conductivity will play major roles in the loss of richness.
Water temperature increased insect family richness in our model, whereas altitude had a negative effect. We have hypothesized a decrease of insect families with rising water temperatures based on the assumption of impaired oxygen uptake in species lacking respiratory adaptations to cope with reduced oxygen, such as plastron and spiracle (Datry et al., 2017). The increase of family richness with increasing water temperature may be explained by the low family richness of high altitude streams with colder water temperature (Jacobsen, 2004) in our study. By contrast, other studies found an increase in family richness with altitude in Northwest Africa (Beauchard et al., 2003; Benzina et al., 2019), while abundance decreased (Benzina et al., 2019). In our dataset, we further saw a decrease of family richness in water temperatures exceeding around 20°C. If rising water temperatures exceed the temperature tolerances of specific insect families, these could be threatened by local extinction (Dallas and Ketley, 2011).
Variables related to human activities, such as a higher degree of regulation, were negatively associated with insect family richness, while the extent of cropland showed a positive association. This is in line with previous findings that hydromorphological alterations of rivers can reduce macroinvertebrate richness (Martínez et al., 2013), due to reduced water quality, disconnection of river sections, and changes in flow regime (McAllister et al., 2001; Navarro-Llácer et al., 2010). Increasing water demand under climate change in Northwest Africa reinforces the importance of water reservoirs for agricultural and potable water use likely resulting in more water regulation (Ayt Ougougdal et al., 2020). This reflects a global trend of increasing river regulation putting freshwater biodiversity at risk (Zarfl et al., 2015). We were surprised to find a positive association between the extent of cropland and insect family richness because most studies suggest a negative impact of agricultural activities on family richness (Genito et al., 2002; Hepp et al., 2010). In our study, cropland areas were more frequently located in temperate areas with lower aridity, which may have masked the negative impact of agriculture.
We used family level data to study associations with environmental variables. Taxonomic richness at the species level and the use of abundance data could show stronger associations with environmental conditions and help to detect variability on a smaller scale (Leung and Dudgeon, 2011).
Association Between Family Composition and Environmental Variables
The Indicator Species analysis showed that nearly half of the insect families in our study were associated with temperate climate and only rarely or never occurred in arid climate, which is in line with the finding of reduced family richness in the latter. These families will likely be foremost affected by changing environmental conditions, whereas only a few families, like Micronectidae, seem to better tolerate arid conditions and could potentially profit from an extension of arid climate (Domisch et al., 2013). The occurrence of insect families is mostly associated with lower aridity, and lower to medium water temperatures and conductivity, explaining their absence in arid environments. Thus, local extinction of species by loss of suitable habitats in Northwest Africa could be minimized by limiting global warming to 1.5°C (Warren et al., 2018).
However, the variance explained by the CCA was relatively low, probably due to the variability of single species inside a family with species showing different associations with environmental variables. Missing stressors (such as local pollution and pesticides) which we did not cover in our model and biogeographic limitations in species distribution could further explain the low variance. As “number of samples per site” was also explaining the distribution of families, consistent sampling methods (e.g., AQEM Consortium (2002)) and the use of metabarcoding for species identification (Elbrecht et al., 2017) could help to overcome these problems.
While the orders of Ephemeroptera, Plecoptera, and Trichoptera (EPT taxa) were mainly associated with low aridity, low water temperature, and low conductivity, we saw a higher variability in the other insect orders. This matches the results of Díaz et al. (2007), where the EPT taxa were more associated with pristine mountain environments than with saline sites. Also, 12 out of 23 EPT families included in the analysis were associated with temperate climate in the Indicator Species Analysis, which suggests these taxa to be good indicators for measuring effects of present and future environmental changes (Wallace and Webster, 1996) in North Africa.
Climate conditions shifting along altitudinal gradients may increase the importance of high altitude habitats with low water temperatures as potential refuge habitats for lowland species (Walther et al., 2009). Insect families, such as Capniidae, were associated with high altitudes and low water temperatures. While species from lowland sites could escape increasing water temperatures by moving upwards to colder mountain streams, suitable habitats for families associated with high altitudes and low water temperatures in our analysis could get lost by changes in environmental conditions (Domisch et al., 2011; Domisch et al., 2013).
Our results suggest that many insect families benefit from agricultural and urban areas in the catchment, This could be due to rich food sources entering the river from the surrounding cropland (MacFarlane, 1983) and suitable conditions in areas of human settlements. Kietzka et al. (2018) found that the loss of most dragonfly species mainly affected endemics, while generalists can cope with the increase of anthropogenic land use. Similarly, other generalist insect species were found to better cope with the change in environmental conditions than endemic species (Domisch et al., 2013). However, we do not know if endemic species were less able to cope with environmental stressors in our case since we only looked at the family level.
Some aquatic insects such as the vector mosquito Culex pipiens transmit diseases like malaria, West Nile virus, and Zika virus (Farajollahi et al., 2011). In our analysis, the family Culicidae (mosquitoes) was positively correlated with higher water temperature and conductivity and thus may profit from climate change as was previously reported (Ramasamy and Surendran 2012). However, not all species within the family of Culicidae are vectors of diseases. Species level identification is required in future studies to better understand potential human health effects through an increase of disease-transmitting mosquitoes (Marselle et al., 2021).
Association Between Traits and Environmental Variables
Environmental conditions shape trait profiles of insect communities by acting as a filter (Statzner et al., 2001). In our study, conductivity, degree of regulation, water temperature, urban extent, aridity, and flow regime explained the differences in insect trait profiles. Similarly, conductivity and water temperature were among the most influential variables of trait profiles in a study from a Mediterranean semi-arid climate (Díaz et al., 2007).
Resilience traits can promote recolonization after natural events such as droughts (Leigh et al., 2016; Stubbington et al., 2017). The environmental variables related to arid climate, aridity, high water temperatures, and conductivity were associated with active aerial dispersal in Redundancy Analysis (RDA). Also, the degree of regulation was positively correlated to aerial dispersal. Aerial dispersal can promote recolonization and help to maintain local populations (Bogan et al., 2017). If large parts of rivers dry out, aquatic insects with the ability to fly, such as coleopterans and hemipterans, are the main colonizers (Díaz et al., 2007; Stubbington et al., 2017).
Resistance traits can help to cope with stressors such as drying rivers and high salinity (Leigh et al., 2016; Stubbington et al., 2017). In the RDA, full water swimmers, respiratory adaptations, and small body size were associated with aridity and high conductivity. The ability to swim (full water swimmers) becomes more important when flow recedes and river sections are separated (Datry et al., 2017) because it allows to reach food sources that are no longer transported by the water current. Due to a fast ion-uptake in saline water and lower oxygen levels in standing or slow running water of intermittent streams, special strategies of respiration are required (Kefford et al., 2016; Datry et al., 2017). In the RDA, adaptations that allow air breathing such as plastron and spiracle show to be associated with high conductivity and aridity (Chessman, 2015; Datry et al., 2017), whereas tegument and gill show the opposite affinity in the model. Resistance against droughts like housing against desiccation and terrestrial clutches also support survival in dry conditions (Díaz et al., 2007; Vidal-Abarca et al., 2013; Datry et al., 2017). However, these traits were non-responsive in our analysis to the environmental variables related to arid climate. Matching the concept of r-strategists (MacArthur & Wilson, 1967), smaller body size and a higher number of reproductive cycles per year were associated with an increase arid environmental stressors, suggesting that these traits provide resistance in arid environments (Díaz et al., 2007; Stubbington et al., 2017).
With a change of environmental conditions towards arid environments with higher water temperature and conductivity, aquatic insect communities need to adapt to changing food sources by a change of feeding habits (Díaz et al., 2007). Similar to Díaz et al. (2007), we found a change of food sources from macroinvertebrates to microphytes, along a gradient of rising water temperatures and conductivity. Further, deposit feeders were associated with high water temperatures, which is in accordance with the river continuum concept (Vannote et al., 1980).
Conclusion
Our results show that a change towards hot and arid climate will likely reduce aquatic insect family richness in temperate regions of Northwest Africa. Climate change induced aridity, high water temperatures, high conductivity and intermittent flow could be intensified by a higher water demand and hydromorphological alterations. Droughts and reduced water quantity and quality could put various insect families, mainly endemics from temperate regions, at risk of local extinction. Traits that increase the ability to survive in arid environments (such as small size, aerial dispersal, ability for air breathing) increase the potential to cope with high water temperatures and conductivity, whereas species lacking such adaptations could be highly affected by a changing environment. A loss of family richness could lead to negative impacts on nature's contribution to human well-being. Protecting and restoring river ecosystems and promoting sustainable water use could limit species loss driven by climate change. Future studies should analyze abundances and use species level identification for a more refined assessment of climate change impact on local insect communities, which could be achieved using metabarcoding.
Data Availability Statement
The original contributions presented in the study are included in the article/Supplementary Material, further inquiries can be directed to the corresponding author.
Author Contribution
All authors contributed to conception and design of the study, contributed to article and statistical analysis revision, read, and approved the submitted version. NK performed literature search, data collection and statistical analysis and wrote the article.
Funding
The study was funded by the SALIDRAAjuj-01UU project through the Program on Social-Ecological Research by the German Ministry of Education and Research (Grant # 01UU1906). The publication was funded by the Open Access Fund of the University of Koblenz-Landau.
Conflict of Interest
The authors declare that the research was conducted in the absence of any commercial or financial relationships that could be construed as a potential conflict of interest.
Publisher’s Note
All claims expressed in this article are solely those of the authors and do not necessarily represent those of their affiliated organizations, or those of the publisher, the editors and the reviewers. Any product that may be evaluated in this article, or claim that may be made by its manufacturer, is not guaranteed or endorsed by the publisher.
Acknowledgments
We kindly thank the authors of the 18 publications for the original data we used in the analysis and for providing additional data. Further, we thank our colleagues for critical and helpful discussions and Stefan Kunz for helping with assigning the traits to taxa. We thank the Germany Ministry of Education and Research (BMBF) for financial support (SALIDRAAjuj-01UU 1906). Finally, we are very thankful for the constructive critique by the two reviewers and would like to thank the organizers of this special issue.
Supplementary Material
The Supplementary Material for this article can be found online at: https://www.frontiersin.org/articles/10.3389/fenvs.2021.671715/full#supplementary-material
References
AQEM Consortium (2002). Manual for the Application of the AQEM System. A Comprehensive Method to Assess European Streams Using Benthic Macroinvertebrates, Developed for the Purpose of the Water Framework Directive.
Arribas, P., Gutiérrez-Cánovas, C., Botella-Cruz, M., Cañedo-Argüelles, M., Carbonell, J. A., Millán, A., et al. (2019). Insect Communities in saline Waters Consist of Realized but Not Fundamental Niche Specialists. Philos. T. Roy. Soc. B. 374 (1764), 20180008. doi:10.1098/rstb.2018.0008
Arthington, A. H., Naiman, R. J., McClain, M. E., and Nilsson, C. (2010). Preserving the Biodiversity and Ecological Services of Rivers: New Challenges and Research Opportunities. Freshw. Biol 55 (1), 1–16. doi:10.1111/j.1365-2427.2009.02340.x
Ayt Ougougdal, H., Yacoubi Khebiza, M., Messouli, M., and Lachir, A. (2020). Assessment of Future Water Demand and Supply under IPCC Climate Change and Socio-Economic Scenarios, Using a Combination of Models in Ourika Watershed, High Atlas, Morocco. Water 12 (6), 1751. doi:10.3390/w12061751
Beauchard, O., Gagneur, J., and Brosse, S. (2003). Macroinvertebrate Richness Patterns in North African Streams: Stream Macroinvertebrate Richness in North Africa. J. Biogeogr. 30 (12), 1821–1833. doi:10.1111/j.1365-2699.2003.00954.x
Beck, H. E., Zimmermann, N. E., McVicar, T. R., Vergopolan, N., Berg, A., and Wood, E. F. (2018). Present and Future Köppen-Geiger Climate Classification Maps at 1-km Resolution. Sci. Data 5 (1), 1–12. doi:10.1038/sdata.2018.214
Benzina, I., Bachir, A. S., Ghazi, C., Santoul, F., and Céréghino, R. (2019). How Altitudinal Gradient Affects the Diversity and Composition of Benthic Insects in Arid Areas Streams of Northern East Algeria? Biologia 75, 567–577. doi:10.2478/s11756-019-00326-8
Berger, E., Bossenbroek, L., Beermann, A. J., Schäfer, R. B., Znari, M., Riethmüller, S., et al. (2021). Social-ecological Interactions in the Draa River Basin, Southern Morocco: Towards Nature Conservation and Human Well-Being Using the IPBES Framework. Sci. Total. Environ. 769, 144492. doi:10.1016/j.scitotenv.2020.144492
Berger, E., Haase, P., Schäfer, R. B., and Sundermann, A. (2018). Towards Stressor-specific Macroinvertebrate Indices: Which Traits and Taxonomic Groups Are Associated with Vulnerable and Tolerant Taxa? Sci. Total. Environ. 619, 144–154. doi:10.1016/j.scitotenv.2017.11.022
Blanco-Garrido, F., Zamora-Muñoz, C., and Bonada, N. (2013). MacroMED: Macroinvertebrates in Mediterranean Climate Watercourses.
Bogan, M. T., Chester, E. T., Datry, T., Murphy, A. L., Robson, B. J., and Ruhi, A. (2017). “Resistance, Resilience, and Community Recovery in Intermittent Rivers and Ephemeral Streams,” in In Intermittent Rivers and Ephemeral Streams (Cambridge, MA: Academic Press), 349–376. doi:10.1016/B978-0-12-803835-2.00007-3
Bonada, N., Dolédec, S., and Statzner, B. (2007). Taxonomic and Biological Trait Differences of Stream Macroinvertebrate Communities between Mediterranean and Temperate Regions: Implications for Future Climatic Scenarios. Glo. Change Biol. 13 (8), 1658–1671. doi:10.1111/j.1365-2486.2007.01375.x
Borcard, D., Gillet, F., and Legendre, P. (2018). Numerical Ecology with R. Princeton, NJ: Springer.
Born, K., Fink, A. H., and Paeth, H. (2008). Dry and Wet Periods in the Northwestern Maghreb for Present Day and Future Climate Conditions. Meteorol. Z. 17 (5), 533–551. doi:10.1127/0941-2948/2008/0313
Cañedo-Argüelles, M., Kefford, B. J., Piscart, C., Prat, N., Schäfer, R. B., and Schulz, C.-J. (2013). Salinisation of Rivers: An Urgent Ecological Issue. Environ. Pollut. 173, 157–167. doi:10.1016/j.envpol.2012.10.011
Cao, X., Chai, L., Jiang, D., Wang, J., Liu, Y., and Huang, Y. (2018). Loss of Biodiversity Alters Ecosystem Function in Freshwater Streams: Potential Evidence from Benthic Macroinvertebrates. Ecosphere 9 (10), e02445. doi:10.1002/ecs2.2445
Chessman, B. C. (2015). Relationships between Lotic Macroinvertebrate Traits and Responses to Extreme Drought. Freshw. Biol. 60 (1), 50–63. doi:10.1111/fwb.12466
Covich, A. P., Palmer, M. A., and Crowl, T. A. (1999). The Role of Benthic Invertebrate Species in Freshwater Ecosystems: Zoobenthic Species Influence Energy Flows and Nutrient Cycling. BioScience 49 (2), 119–127. doi:10.2307/1313537
Dallas, H. F., and Ketley, Z. A. (2011). Upper thermal Limits of Aquatic Macroinvertebrates: Comparing Critical thermal Maxima with 96-LT50 Values. J. Therm. Biol. 36 (6), 322–327. doi:10.1016/j.jtherbio.2011.06.001
Datry, T., Bonada, N., and Boulton, A. (2017). Intermittent Rivers and Ephemeral Streams: Ecology and Management. Cambridge: Academic Press (Elsevier.
Dewson, Z. S., James, A. B. W., and Death, R. G. (2007). A Review of the Consequences of Decreased Flow for Instream Habitat and Macroinvertebrates. J. N. Am. Benthol. Soc. 26 (3), 401–415. doi:10.1899/06-110.1
Díaz, A. M., Alonso, M. L. S., and Gutiérrez, M. R. V.-A. (2007). Biological Traits of Stream Macroinvertebrates from a Semi-arid Catchment: Patterns along Complex Environmental Gradients. Freshw. Biol 53 (1), 1–21. doi:10.1111/j.1365-2427.2007.01854.x
Döll, P., and Schmied, H. M. (2012). How Is the Impact of Climate Change on River Flow Regimes Related to the Impact on Mean Annual Runoff? A Global-Scale Analysis. Environ. Res. Lett. 7 (1), 014037. doi:10.1088/1748-9326/7/1/014037
Domisch, S., Araújo, M. B., Bonada, N., Pauls, S. U., Jähnig, S. C., and Haase, P. (2013). Modelling Distribution in European Stream Macroinvertebrates under Future Climates. Glob. Change Biol. 19 (3), 752–762. doi:10.1111/gcb.12107
Domisch, S., Jähnig, S. C., and Haase, P. (2011). Climate-change Winners and Losers: Stream Macroinvertebrates of a Submontane Region in Central Europe: Climate Change Effects on Stream Macroinvertebrates. Freshw. Biol 56 (10), 2009–2020. doi:10.1111/j.1365-2427.2011.02631
Dornelas, M., Gotelli, N. J., Shimadzu, H., Moyes, F., Magurran, A. E., and McGill, B. J. (2019). A Balance of Winners and Losers in the Anthropocene. Ecol. Lett. 22 (5), 847–854. doi:10.1111/ele.13242
Durance, I., and Ormerod, S. J. (2007). Climate Change Effects on upland Stream Macroinvertebrates over a 25-year Period. Glob. Change Biol. 13, 942–957. doi:10.1111/j.1365-2486.2007.01340.x
Elbrecht, V., Vamos, E. E., Meissner, K., Aroviita, J., and Leese, F. (2017). Assessing Strengths and Weaknesses of DNA Metabarcoding‐based Macroinvertebrate Identification for Routine Stream Monitoring. Methods Ecol. Evol. 8 (10), 1265–1275. doi:10.1111/2041-210X.12789
Farajollahi, A., Fonseca, D. M., Kramer, L. D., and Marm Kilpatrick, A. (2011). “Bird Biting” Mosquitoes and Human Disease: A Review of the Role of Culex pipiens Complex Mosquitoes in Epidemiology. Infect. Gen. Evo. 11 (7), 1577–1585. doi:10.1016/j.meegid.2011.08.013
Faraway, J. (2016). Faraway: Functions and Datasets for Books by Julian Faraway. R. Package Version 1 (7).
Fox, J., and Weisberg, S. (2018). An R Companion to Applied Regression. Thousand Oaks, CA: Sage publications.
Genito, D., Gburek, W. J., and Sharpley, A. N. (2002). Response of Stream Macroinvertebrates to Agricultural Land Cover in a Small Watershed. J. Freshw. Ecol. 17 (1), 109–119. doi:10.1080/02705060.2002.9663874
Giller, P. S., Hillebrand, H., Berninger, U.-G., Gessner, O. M., Hawkins, S., Inchausti, P., et al. (2004). Biodiversity Effects on Ecosystem Functioning: Emerging Issues and Their Experimental Test in Aquatic Environments. Oikos 104 (3), 423–436. doi:10.1111/j.0030-1299.2004.13253.x
Giudicelli, J., and Dakki, M. (1984). Les Sources du Moyen Atlas et de Rif (Maroc): Faunistique (Description de Deux Espèces Nouvelles de Trichoptères), Écologie. Intérêt Biogéographique. Bijdr. Dierkd. 54, 83–100. doi:10.1163/26660644-05401007
Hamilton, A. T., Schäfer, R. B., Pyne, M. I., Chessman, B., Kakouei, K., Boersma, K. S., et al. (2020). Limitations of Trait‐based Approaches for Stressor Assessment: The Case of Freshwater Invertebrates and Climate Drivers. Glob. Change Biol. 26 (2), 364–379. doi:10.1111/gcb.14846
Hartig, F. (2020). DHARMa: Residual Diagnostics for Hierarchical (Multi-Level/Mixed) Regression Models. The Comprehensive R Archive Network (CRAN).R package version 0.3. 2.0.
Hepp, L. U., Milesi, S. V., Biasi, C., and Restello, R. M. (2010). Effects of Agricultural and Urban Impacts on Macroinvertebrates Assemblages in Streams (Rio Grande Do Sul, Brazil). Zoologia-Curitiba 27 (1), 106–113. doi:10.1590/S1984-46702010000100016
Herbert, E. R., Boon, P., Burgin, A. J., Neubauer, S. C., Franklin, R. B., Ardón, M., et al. (2015). A Global Perspective on Wetland Salinization: Ecological Consequences of a Growing Threat to Freshwater Wetlands. Ecosphere 6 (10), 1–43. doi:10.1890/ES14-00534.1
Hughes, J., and Team, R. C. (2017). Reghelper: Helper Functions for Regression Analysis. R. Package Version 0 3, 3.
Jacobsen, D. (2004). Contrasting Patterns in Local and Zonal Family Richness of Stream Invertebrates along an Andean Altitudinal Gradient. Freshw. Biol 49 (10), 1293–1305. doi:10.1111/j.1365-2427.2004.01274.x
Kampstra, P. (2008). Beanplot: A Boxplot Alternative for Visual Comparison of Distributions. J. Stat. Soft. 28 (1), 1–99. doi:10.18637/jss.v028.c01
Kefford, B. J., Buchwalter, D., Cañedo-Argüelles, M., Davis, J., Duncan, R. P., Hoffmann, A., et al. (2016). Salinized Rivers: Degraded Systems or New Habitats for Salt-Tolerant Faunas? Biol. Lett. 12 (3), 20151072. doi:10.1098/rsbl.2015.1072
Kefford, B. J. (1998). The Relationship between Electrical Conductivity and Selected Macroinvertebrate Communities in Four River Systems of South-West Victoria, Australia. Int. J. Salt Lake Res. 7 (2), 153–170. doi:10.1007/BF02441884
Khebiza, M. Y., Boughrous, A. A., Gabbanini, C., Messouli, M., and Messana, G. (2006). Impact of Waste Discharges on the Water Quality and Interstitial Community Structure of Two Mediterranean Rivers. Ital. J. Zool. 73 (2), 153–166. doi:10.1080/11250000600679462
Kietzka, G. J., Pryke, J. S., and Samways, M. J. (2018). Comparative Effects of Urban and Agricultural Land Transformation on Odonata Assemblages in a Biodiversity Hotspot. Basic Appl. Ecol. 33, 89–98. doi:10.1016/j.baae.2018.08.008
Legendre, P., and Gallagher, E. D. (2001). Ecologically Meaningful Transformations for Ordination of Species Data. Oecologia 129 (2), 271–280. doi:10.1007/s004420100716
Lehner, B., and Grill, G. (2013). Global River Hydrography and Network Routing: Baseline Data and New Approaches to Study the World’s Large River Systems. Hydrol. Process. 27 (15), 2171–2186. doi:10.1002/hyp.9740
Lehner, B., Liermann, C. R., Revenga, C., Vörösmarty, C., Fekete, B., Crouzet, P., et al. (2011). High‐resolution Mapping of the World’s Reservoirs and Dams for Sustainable River‐flow Management. Front. Ecol. Environ. 9 (9), 494–502. doi:10.1890/100125
Linke, S., Lehner, B., Ouellet Dallaire, C., Ariwi, J., Grill, G., Thieme, M., et al. (2019). Global hydro-environmental sub-basin and river reach characteristics at high spatial resolution. Sci Data 6 (283), 121. doi:10.1038/s41597-019-0300-6
Leigh, C., Bonada, N., Boulton, A. J., Hugueny, B., Larned, S. T., Vander Vorste, R., et al. (2016). Invertebrate Assemblage Responses and the Dual Roles of Resistance and Resilience to Drying in Intermittent Rivers. Aquat. Sci. 78 (2), 291–301. doi:10.1007/s00027-015-0427-2
Leung, A. S. L., and Dudgeon, D. (2011). Scales of Spatiotemporal Variability in Macroinvertebrate Abundance and Diversity in Monsoonal Streams: Detecting Environmental Change: Spatiotemporal Variability of Macroinvertebrates in Monsoonal Streams. Freshw. Biol 56 (6), 1193–1208. doi:10.1111/j.1365-2427.2010.02556.x
MacArthur, R. H., and Wilson, E. O. (1967). The Theory of Island Biogeography, Vol. 1. Princeton university press.
MacFarlane, M. B. (1983). Structure of Benthic Macroinvertebrate Communities in a Midwestern Plains Stream. Freshw. Invert. Biol. 2 (3), 147–153. doi:10.2307/1467089
Márcia Barbosa, A., Real, R., Muñoz, A.-R., and Brown, J. A. (2013). New Measures for Assessing Model Equilibrium and Prediction Mismatch in Species Distribution Models. Divers. Distrib 19 (10), 1333–1338. doi:10.1111/ddi.12100
Marselle, M. R., Hartig, T., Cox, D. T. C., de Bell, S., Knapp, S., Lindley, S., et al. (2021). Pathways Linking Biodiversity to Human Health: A Conceptual Framework. Environ. Int. 150, 106420. doi:10.1016/j.envint.2021.106420
Martínez, A., Larrañaga, A., Basaguren, A., Pérez, J., Mendoza-Lera, C., and Pozo, J. (2013). Stream Regulation by Small Dams Affects Benthic Macroinvertebrate Communities: from Structural Changes to Functional Implications. Hydrobiologia 711 (1), 31–42. doi:10.1007/s10750-013-1459-z
McAllister, D. E., Craig, J. F., Davidson, N., Delany, S., and Seddon, M. (2001). Biodiversity Impacts of Large Dams. Int. Union Conserv. Nat. Nat. Resour./UN Environ. Programme Rep., 1–68.
Mondy, C. P., Muñoz, I., and Dolédec, S. (2016). Life-history Strategies Constrain Invertebrate Community Tolerance to Multiple Stressors: A Case Study in the Ebro basin. Sci. Total. Environ. 572, 196–206. doi:10.1016/j.scitotenv.2016.07.227
Morghad, F., Samraoui, F., Touati, L., and Samraoui, B. (2019). The Times They Are a Changin’: Impact of Land-Use Shift and Climate Warming on the Odonate Community of a Mediterranean Stream over a 25-year Period. Vie Milieu 69 (1), 25–33.
Navarro-Llácer, C., Baeza, D., and de las Heras, J. (2010). Assessment of Regulated Rivers with Indices Based on Macroinvertebrates, Fish and Riparian forest in the Southeast of Spain. Ecol. Indic. 10 (5), 935–942. doi:10.1016/j.ecolind.2010.02.003
Oksanen, J., Blanchet, F. G., Friendly, M., Kindt, R., Legendre, P., and McGlinn, D. (2019). Vegan: Community Ecology Package. R Package Version 2, 5–6.
Pesaresi, M., and Freire, S. (2016). GHS Settlement Grid Following the REGIO Model 2014 in Application to GHSL Landsat and CIESIN GPW V4-Multitemporal (1975-1990-2000-2015). Brussels: European Commission, Joint Research Centre.
Piscart, C., Usseglio-Polatera, P., and Moreteau, J. C. B. (2006). The Role of Salinity in the Selection of Biological Traits of Freshwater Invertebrates. Archiv Hydrobio 166 (2), 185–198. doi:10.1127/0003-9136/2006/0166-0185
Pitman, M. G., and Läuchli, A. (2002). “Global Impact of Salinity and Agricultural Ecosystems,” in Salinity: Environment - Plants - Molecules. Editors A. Läuchli, and U. Lüttge (Dordrecht: Kluwer Academic Publishers), 3–20. doi:10.1007/0-306-48155-3_1
QGIS.org (2021). QGIS Geographic Information System. QGIS Associationhttp://www.qgis.org (Accessed February 4, 2021).]
Ramankutty, N., Evan, A. T., Monfreda, C., and Foley, J. A. (2008). Farming the Planet: 1. Geographic Distribution of Global Agricultural Lands in the Year 2000. Glob. Biogeochem. Cycles 22 (1), GB1022. doi:10.1029/2007GB002952
Ramasamy, R., and Surendran, S. N. (2012). Global Climate Change and its Potential Impact on Disease Transmission by Salinity-Tolerant Mosquito Vectors in Coastal Zones. Front. Physio. 3, 198. doi:10.3389/fphys.2012.00198
Rohatgi, A. (2020). WebPlotDigitizer. Available: https://automeris.io/WebPlotDigitizer (accessed July 31, 2020).
Schilling, J., Freier, K. P., Hertig, E., and Scheffran, J. (2012). Climate Change, Vulnerability and Adaptation in North Africa with Focus on Morocco. Agricult. Ecos. Environ. 156, 12–26. doi:10.1016/j.agee.2012.04.021
Seibold, S., Gossner, M. M., Simons, N. K., Blüthgen, N., Müller, J., Ambarlı, D., et al. (2019). Arthropod Decline in Grasslands and Forests Is Associated with Landscape-Level Drivers. Nature 574 (7780), 671–674. doi:10.1038/s41586-019-1684-3
Smeti, E., von Schiller, D., Karaouzas, I., Laschou, S., Vardakas, L., Sabater, S., et al. (2019). Multiple Stressor Effects on Biodiversity and Ecosystem Functioning in a Mediterranean Temporary River. Sci. Total. Environ. 647, 1179–1187. doi:10.1016/j.scitotenv.2018.08.105
Solan, M. (2004). Extinction and Ecosystem Function in the Marine Benthos. Science 306 (5699), 1177–1180. doi:10.1126/science.1103960
Soria, M., Leigh, C., Datry, T., Bini, L. M., and Bonada, N. (2017). Biodiversity in Perennial and Intermittent Rivers: a Meta-Analysis. Oikos 126 (8), 1078–1089. doi:10.1111/oik.04118
Statzner, B., Hildrew, A. G., and Resh, V. H. (2001). Species Traits and Environmental Constraints: Entomological Research and the History of Ecological Theory. Annu. Rev. Entomol. 46 (1), 291–316. doi:10.1146/annurev.ento.46.1.291
Strange, E. M., Fausch, K. D., and Covich, A. P. (1999). Sustaining Ecosystem Services in Human-Dominated Watersheds: Biohydrology and Ecosystem Processes in the South Platte River Basin. Environ. Manage. 24 (1), 39–54. doi:10.1007/s002679900213
Stubbington, R., Bogan, M. T., Bonada, N., Boulton, A. J., Datry, T., Leigh, C., et al. (2017). “The Biota of Intermittent Rivers and Ephemeral Streams: Aquatic Invertebrates,” in Intermittent Rivers and Ephemeral Streams. Elsevier, Amsterdam: 217–243. doi:10.1016/B978-0-12-803835-2.00007-3
Stubbington, R., Greenwood, A. M., Wood, P. J., Armitage, P. D., Gunn, J., and Robertson, A. L. (2009). The Response of Perennial and Temporary Headwater Stream Invertebrate Communities to Hydrological Extremes. Hydrobiologia 630 (1), 299–312. doi:10.1007/s10750-009-9823-8
Szöcs, E., Coring, E., Bäthe, J., and Schäfer, R. B. (2014). Effects of Anthropogenic Salinization on Biological Traits and Community Composition of Stream Macroinvertebrates. Sci. Total. Environ. 468, 943–949. doi:10.1016/j.scitotenv.2013.08.058
Tachet, H., Richoux, P., Bournaud, M., and Usseglio-Polatera, P. (2010). Invertébrés D'eau Douce: Systématique, Biologie, Écologie, Vol. 15. Paris: CNRS editions.
Terink, W., Immerzeel, W. W., and Droogers, P. (2013). Climate Change Projections of Precipitation and Reference Evapotranspiration for the Middle East and Northern Africa until 2050. Int. J. Climatol. 33 (14), 3055–3072. doi:10.1002/joc.3650
Vannote, R. L., Minshall, G. W., Cummins, K. W., Sedell, J. R., and Cushing, C. E. (1980). The River Continuum Concept. Can. J. Fish. Aquat. Sci. 37 (1), 130–137. doi:10.1139/f80-017
Velasco, J., Millán, A., Hernández, J., Gutiérrez, C., Abellán, P., Sánchez, D., et al. (2006). Response of Biotic Communities to Salinity Changes in a Mediterranean Hypersaline Stream. Saline Syst. 2 (1), 1–15. doi:10.1186/1746-1448-2-12
Venables, W. N., and Ripley, B. D. (2002). Modern Applied Statistics with S. Editors J. Chambers, W. Eddy, W. Härdle, S. Sheather, and L. Tierney, Fourth Edition. New York: Springer.
Vidal-Abarca, M. R., Sánchez-Montoya, M. M., Guerrero, C., Gómez, R., Arce, M. I., García-García, V., et al. (2013). Effects of Intermittent Stream Flow on Macroinvertebrate Community Composition and Biological Traits in a Naturally saline Mediterranean Stream. J. Arid. Environ. 99, 28–40. doi:10.1016/j.jaridenv.2013.09.008
Waha, K., Krummenauer, L., Adams, S., Aich, V., Baarsch, F., and Schleussner, C. F. (2017). Climate Change Impacts in the Middle East and Northern Africa (MENA) Region and Their Implications for Vulnerable Population Groups. Reg. Environ. Change 17 (6), 1623–1638. doi:10.1007/s10113-017-1144-2
Wallace, J. B., and Webster, J. R. (1996). The Role of Macroinvertebrates in Stream Ecosystem Function. Annu. Rev. Entomol. 41 (1), 115–139. doi:10.1146/annurev.en.41.010196.000555
Walther, G.-R., Roques, A., Hulme, P. E., Sykes, M. T., Pyšek, P., Kühn, I., et al. (2009). Alien Species in a Warmer World: Risks and Opportunities. Trends Ecol. Evol. 24 (12), 686–693. doi:10.1016/j.tree.2009.06.008
Warren, R., Price, J., Graham, E., Forstenhaeusler, N., and VanDerWal, J. (2018). The Projected Effect on Insects, Vertebrates, and Plants of Limiting Global Warming to 1.5°C rather Than 2°C. Science 360 (6390), 791–795. doi:10.1126/science.aar3646
Williams, W. D. (1999). Salinisation: A Major Threat to Water Resources in the Arid and Semi-arid Regions of the World. Lakes Reservoirs 4 (3-4), 85–91. doi:10.1046/j.1440-1770.1999.00089.x
Zarfl, C., Lumsdon, A. E., Berlekamp, J., Tydecks, L., and Tockner, K. (2015). A Global Boom in Hydropower Dam Construction. Aquat. Sci. 77 (1), 161–170. doi:10.1007/s00027-014-0377-0
Keywords: macroinvertebrates, river intermittency, Northwest Africa, climate change, aridity, insect richness
Citation: Kaczmarek N, Schäfer RB and Berger E (2021) Environmental Change Threatens Freshwater Insect Communities in Northwest Africa: A Meta-Analysis. Front. Environ. Sci. 9:671715. doi: 10.3389/fenvs.2021.671715
Received: 24 February 2021; Accepted: 15 July 2021;
Published: 27 July 2021.
Edited by:
Eugenia López-López, Instituto Politécnico Nacional de México (IPN), MexicoReviewed by:
Kai Chen, Nanjing Agricultural University, ChinaMaria Filomena Magalhães, University of Lisbon, Portugal
Copyright © 2021 Kaczmarek, Schäfer and Berger. This is an open-access article distributed under the terms of the Creative Commons Attribution License (CC BY). The use, distribution or reproduction in other forums is permitted, provided the original author(s) and the copyright owner(s) are credited and that the original publication in this journal is cited, in accordance with accepted academic practice. No use, distribution or reproduction is permitted which does not comply with these terms.
*Correspondence: Nils Kaczmarek, a2Fjem1hcmVrQHVuaS1sYW5kYXUuZGU=